- 1Department of Tropical Medicine, University of Hawai'i, Honolulu, HI, United States
- 2Hawaii Center for AIDS, University of Hawai'i, Honolulu, HI, United States
- 3Department of Native Hawaiian Health, University of Hawai'i, Honolulu, HI, United States
- 4Office of Biostatistics and Quantitative Health Sciences, John A. Burns School of Medicine, University of Hawai'i, Honolulu, HI, United States
Cognitive dysfunction persists in 30–50% of chronically HIV-infected individuals despite combination antiretroviral therapy (ART). Although monocytes are implicated in poor cognitive performance, distinct biological mechanisms associated with cognitive dysfunction in HIV infection are unclear. We previously showed that a regulatory region of the interferon regulatory factor-8 (IRF8) gene is hyper-methylated in HIV-infected individuals with cognitive impairment compared to those with normal cognition. Here, we investigated IRF-8 protein expression and assessed relationships with multiple parameters associated with brain health. Intracellular IRF-8 expression was measured in cryopreserved peripheral blood mononuclear cells from chronically HIV-infected individuals on ART using flow cytometry. Neuropsychological performance was assessed by generating domain-specific standardized (NPZ) scores, with a global score defined by aggregating individual domain scores. Regional brain volumes were obtained by magnetic resonance imaging and soluble inflammatory factors were assessed by immunosorbent assays. Non-parametric analyses were conducted and statistical significance was defined as p < 0.05. Twenty aviremic (HIV RNA<50 copies/ml) participants, 84% male, median age 51 [interquartile range (IQR) 46, 55], median CD4 count 548 [439, 700] were evaluated. IRF-8 expression was highest in plasmacytoid dendritic cells (pDCs). Assessing cognitive function, lower IRF-8 density in classical monocytes significantly correlated with worse NPZ_learning memory (LM; rho = 0.556) and NPZ_working memory (WM; rho = 0.612) scores, in intermediate monocytes with worse NPZ_LM (rho = 0.532) scores, and in non-classical monocytes, lower IRF-8 correlated with worse global NPZ (rho = 0.646), NPZ_LM (rho = 0.536), NPZ_WM (rho = 0.647), and NPZ_executive function (rho = 0.605) scores. In myeloid DCs (mDCs) lower IRF-8 correlated with worse NPZ_WM (rho = 0.48) scores and in pDCs with worse NPZ_WM (rho = 0.561) scores. Declines in IRF-8 in classical monocytes significantly correlated with smaller hippocampal volume (rho = 0.573) and in intermediate and non-classical monocytes with smaller cerebral white matter volume (rho = 0.509 and rho = 0.473, respectively). IRF-8 density in DCs did not significantly correlate with brain volumes. Among biomarkers tested, higher soluble ICAM-1 levels significantly correlated with higher IRF-8 in all monocyte and DC subsets. These data may implicate IRF-8 as a novel transcription factor in the neuropathophysiology of brain abnormalities in treated HIV and serve as a potential therapeutic target to decrease the burden of cognitive dysfunction in this population.
Introduction
Cognitive performance is compromised in ~30–50% of chronically HIV-infected individuals despite access to combination antiretroviral therapy (ART) (1, 2). These cognitive, behavioral, and motor deficits are not only widespread, but also impact everyday functioning, increase morbidity and mortality and have lasting critical public health effects (3–5). Since clinically approved therapies for HIV-associated cognitive impairment are not available (6), there is need to identify novel therapeutic targets.
The transmigration of both infected and uninfected monocytes into the central nervous system (CNS) is thought to be a significant mediator of the development of cognitive disorders during HIV infection, primarily by promoting viral seeding of CNS resident cells and promoting neuroinflammatory responses (7–14). Furthermore, myeloid cells, including monocytes and dendritic cells (DCs), play crucial roles in maintaining homeostasis along with inducing and controlling neuroinflammatory responses when recruited to the CNS (15–17). The implications of myeloid cells entering the CNS can differ depending on the pathological state and their peripheral phenotype, either inducing neuroinflammatory cytotoxic effects or promoting neural regeneration (18–20). Therefore, a better understanding of molecular mechanisms linking peripheral myeloid cells to the CNS is crucial to further elucidate the pathophysiology of HIV-associated brain injury.
Previously, we identified 1,032 differentially methylated loci in monocytes from persons with and without HIV-associated cognitive impairment. IRF8, the gene encoding for interferon regulatory factor-8 (IRF-8), had regions of significant hypermethylation in HIV-infected individuals with cognitive impairment compared to those with normal cognition, suggesting a potential role for this otherwise constitutively expressed transcription factor in HIV-related cognitive dysfunction (21). IRF-8 plays critical roles in the regulation of lineage commitment and differentiation during myeloid cell maturation and response to stimuli (22–24). For example, IRF-8 expression is elevated in the brains of a Alzheimer's Disease rodent model and in the context of accelerated aging and Alzheimer's Disease, IRF-8 was found to be one of the biomarkers with the highest correlation coefficient (25–27). Here, we wish to extend our IRF8 epigenetic findings and evaluate IRF-8 protein expression in myeloid cells and the relationship to several measures of cognition, CNS injury, and inflammation in virally suppressed chronic HIV individuals on stable ART.
Materials and Methods
Cohort Description
This study consisted of 20 chronically HIV infected individuals from the Hawaii Aging with HIV—Cardiovascular cohort study. The study, which has been previously described (28), was approved by the University of Hawaii Manoa Committee on Human Studies. Entry criteria to the study required subjects to have documentation of HIV infection, be ≥40 years, and to be on stable ART for≥6 months. Two study participants had a history of Hepatitis C infection. The selection of participants for our study was based on the availability of neuropsychological (NP) testing data and banked cryopreserved peripheral blood mononuclear cells (PBMCs) and plasma.
Neuropsychological Assessments
NP testing was conducted at the UH Clint Spencer Clinic by trained psychometrists. In order to minimize the risk of distraction and fatigue, NP testing was conducted in a quiet room and participants were provided breaks as needed throughout the testing session. The test battery was comprised of measures known to be sensitive to HIV infection including psychomotor speed, executive function, learning and memory, and working memory (29, 30). All raw NP scores were transformed to standard z-scores (NPZ score) using normative data (31, 32) and a global score was defined by aggregating the domain scores.
Regional Brain Volume Assessments by Magnetic Resonance Imaging
T1-weighted MRI data were processed with FreeSurfer (version 5.0, https://surfer.nmr.mgh.harvard.edu) (33–36) to obtain volumes of the caudate, putamen, hippocampus, amygdala, cortical gray matter, cerebral white matter, cerebellar gray matter, cerebellar white matter, and total subcortical gray matter, FreeSurfer's processing steam involves skull-stripping (37), intensity normalization (38), Talairach transformation, segmentation of subcortical white matter and deep gray matter (34, 35), and reconstruction of the cortical gray/white matter boundary and pial surface (33). Total regional volumes were computed by summing over the left and right hemispheres. An estimate of total intracranial volume (ICV) was obtained and used to normalize the regional volumes of interest (39). Each regional volume was expressed as a fraction of ICV (i.e., volume/ICV) to adjust for inter-individual head size variability.
IRF-8 Intracellular Staining
Cryopreserved PBMCs were placed in 96 well-polypropylene round bottom plates and stained with Live/Dead® Fixable Red Dead Cell Stain for 15 min at room temperature followed by a 30 min room temperature with conjugated monoclonal antibodies (mAbs) against CD3 [Brilliant Violet (BV)711], CD4 (PE-Texas Red), CD8 (PE-Cy5), CD7 (PE-Cy7), CD19 (PE-Cy7), CD20 (PE-Cy7), CD11c (AlexaFluor700), CD123 (FITC), CD11b (BV510), HLA-DR (APC-H7), CD14 (BV605), CD16 (BV421). Cells were then fixed and permeabilized with BD FACS Lysing Solution and Permeabilizing Solution 2 (BD Bioscience, San Jose, USA), respectively, then stained with an anti-IRF-8 antibody or isotype control (PerCP-eF710). Cells were fixed with 1% PFA and samples were acquired on a custom 4-laser BD LSRFortessa (BD Bioscience, San Jose, USA). Compensation and gating analyses were performed using FlowJo (FlowJo LCC, Ashland, USA). Reagents were purchased from BD Bioscience, San Jose, USA (mAbs CD123 Catalog Number [Cat] 558663, CD8 Cat 555368, CD19 Cat 557835, CD20 Cat 560735), Invitrogen, Carlsbad, USA (Live/Dead Stain, CD4 Cat MHCD0417), BioLegend, San Diego, USA (mAbs CD3 Cat 317328, CD7 Cat 343113, CD11b Cat 301333, HLA-DR Cat 307618, CD14 Cat 301834, CD16 Cat 302038) and eBioscience, San Diego, USA (mAbs CD11c Cat 56-0116-42, IRF-8 Cat 46-9852-80, isotype control Cat 46-4714-82). The implemented phenotyping gating strategy is shown in Supplementary Figure 1.
Quantification of Plasma Markers
The plasma soluble biomarkers, matrix metallopeptidase-9, myeloperoxidase, tissue plasminogen activator inhibitor-1, C-reactive protein, serum amyloid A, serum amyloid P, interleukin (IL)-1β, IL-6, IL-8, IL-10, tumor necrosis factor (TNF)-α, soluble E-selectin, soluble vascular cell adhesion molecule-1, soluble intercellular cell adhesion molecule-1 (sICAM-1), monocyte chemoattractant protein-1 (MCP-1), vascular endothelial growth factor, interferon-gamma (IFN-γ), and N-terminal pro-brain natriuretic peptide were measured by Milliplex Human Cardiovascular Disease panels (EMD Millipore, Temecula, CA) as outlined in the manufacturer's protocols as previously described (40).
Statistical Analysis
Demographic and clinical characteristics were presented as a median and interquartile range (IQR) except for gender, which was presented as a percentage. Comparisons between continuous variables were carried out using Kruskal-Wallis tests, and for categorical variables, chi-squared tests. For IRF-8 outcomes, Kruskal Wallis tests were used to compare between groups. Associations between two continuous variables were evaluated by Spearman correlation. Statistical analyses were performed using R v3.2.2 or Prism GraphPad version 7 (GraphPad Software, San Diego, California).
Results
Participant Characteristics and IRF-8 Expression in Blood
Clinical and demographic characteristics of the participants are shown in Table 1. The twenty participants included in this study had undetectable viral loads (<48 copies/ml), were 84% male, with a median age of 51 ([IQR 47, 55]), a median CD4 count of 548 [439, 700]), and had a range of cognitive performance [global score of −0.04 (−0.46, 0.55)]. IRF-8 density in each myeloid subset from HCV-infected and HCV-uninfected subjects are shown in Supplementary Figure 2.
Among the myeloid subsets analyzed, plasmacytoid DCs (pDCs) had the highest IRF-8 expression (geometric mean fluorescence intensity (GMF) 1501 [1273, 1651]), which was significantly higher than all 3 monocyte subsets and myeloid DCs (mDCs) (GMF 250 [222,277]) in concordance with a previous murine study (p's <0.001; Figures 1A,B) (41). Non-classical monocytes had the lowest IRF-8 expression (GMF 202 [192, 230]) compared to intermediate monocytes (GMF 244 [211, 273], not significant) and classical monocytes (GMF 270 [236, 289]; p < 0.01) (Figures 1A,B), while CD4+ and CD8+ T cells had undetectable IRF-8 levels (Figure 1B). Frequencies of each myeloid subset are show in Supplementary Table 1. IRF-8 expression in all myeloid subsets did not associate with age, CD4 count or nadir, CD8 count, CD4/CD8 ratio, or total self-reported years of infection or on ART (data not shown).
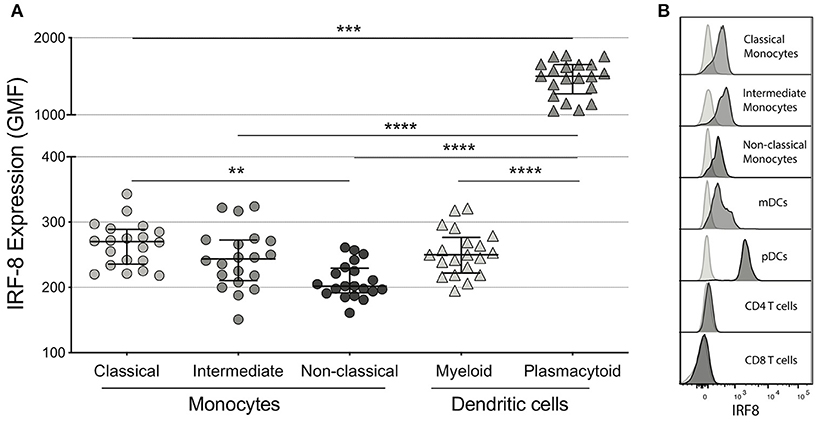
Figure 1. IRF-8 expression on monocytes and dendritic cells. (A) Intracellular IRF-8 levels, expressed as geometric mean fluorescence intensity (GMF), on monocyte subsets (classical, intermediate, and non-classical) and dendritic cell (DC) subsets (myeloid and plasmacytoid). The hash mark indicates the median and error bars are the interquartile range. **p < 0.01; ***p < 0.001, ****p < 0.0001. (B) Representative histograms of IRF-8 expression in each subset.
IRF-8 Density in Myeloid Cells Correlates With Cognitive Performance
Next, correlations of IRF-8 density in myeloid cells and neurocognitive functions were analyzed (Figure 2 and Supplementary Table 2). Higher IRF-8 expression on classical monocytes correlated with better NPZ_learning and memory (LM) (rho = 0.556, p = 0.013) and working memory (WM) (rho = 0.612, p = 0.004; Figure 2A) scores. Higher IRF-8 expression on intermediate monocytes also correlated with better NPZ_LM scores (rho = 0.532, p = 0.019; Figure 2B). Greater IRF-8 expression on non-classical monocytes also correlated with increased NP testing (global: rho = 0.646; p = 0.004; LM: rho = 0.536, p = 0.018, WM: rho = 0.647, p = 0.002 and executive function: rho = 0.605; p = 0.005; Figure 2C). Higher IRF-8 expression on both mDCs and pDCs also correlated with better NPZ_WM scores (rho = 0.484, p = 0.030 and rho = 0.561, p = 0.010, respectively; Figures 2D,E). Age did not show significant correlations with any NPZ testing data (data not shown).
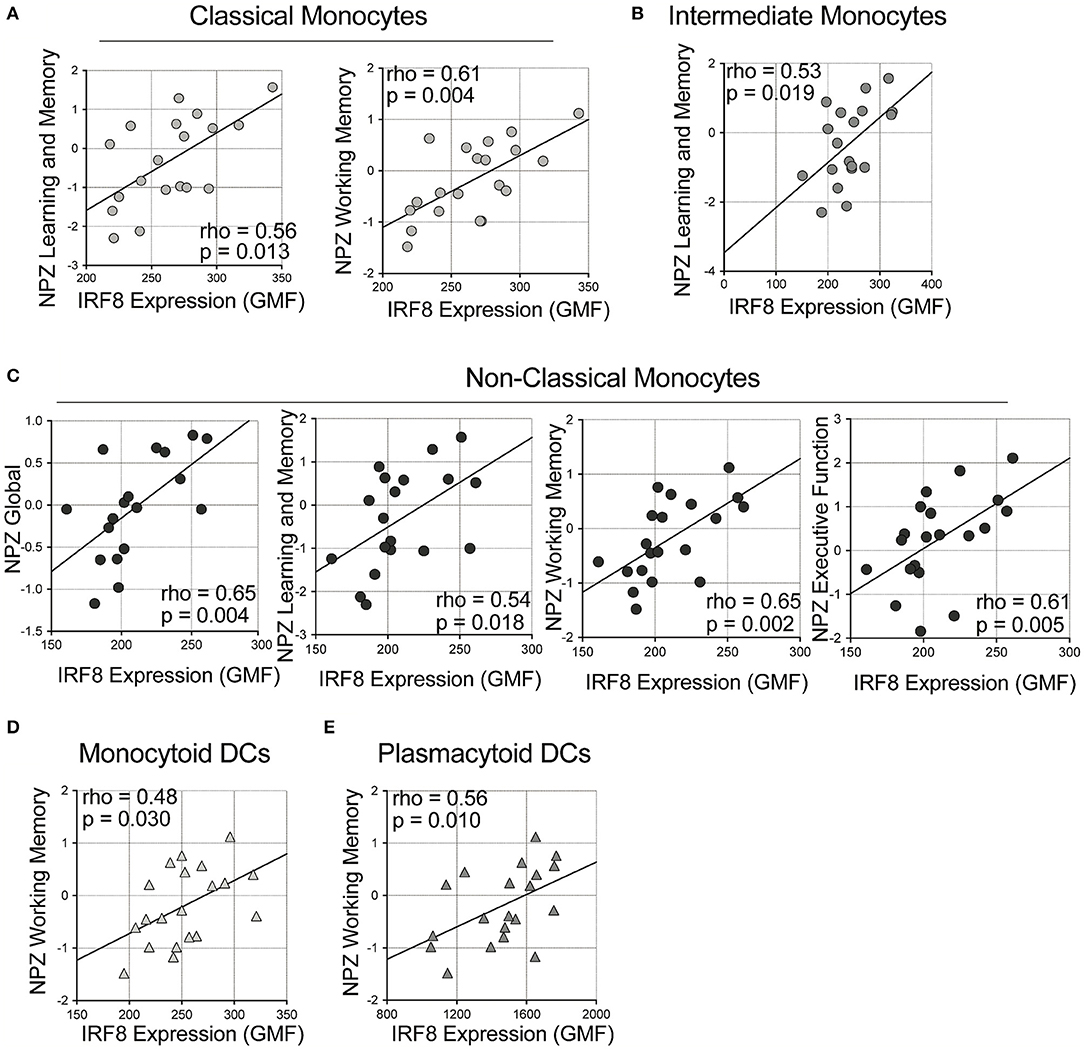
Figure 2. Correlations between IRF-8 expression on myeloid cells and neuropsychological testing. Correlations between IRF-8 expression of (A) classical, (B) intermediate, and (C) non-classical monocytes, and (D) myeloid and (E) plasmacytoid dendritic cells and NPZ scores.
IRF-8 Density in Monocytes Correlates With Brain Volumes
We analyzed correlations of IRF-8 density with regional brain volumes (Figure 3 and Supplementary Table 3). Higher IRF-8 expression in classical monocytes correlated with larger ICV-adjusted hippocampal volume (rho = 0.573; p = 0.008; Figure 3A). Higher IRF-8 expression in intermediate and non-classical monocytes correlated with larger cerebral white matter volume corrected for ICV (rho = 0.509; p = 0.022 and rho = 0.473; p = 0.035, respectively; Figures 3B,C). DC IRF-8 expression did not correlate with regional brain volumes (Supplementary Table 3). Age did not correlate with volumes of hippocampus or cerebral white matter (data not shown).
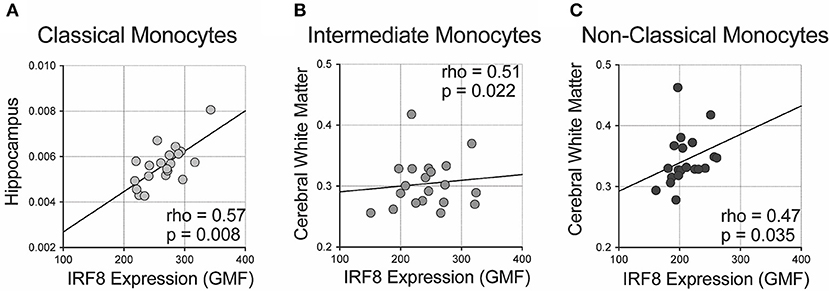
Figure 3. Correlations between IRF-8 expression on myeloid cells and regional brain volumes. Correlations between IRF-8 expression of (A) classical, (B) intermediate, and (C) non-classical monocytes and regional brain volumes corrected for intracranial volume.
IRF-8 Density in Myeloid Cells Correlates With Plasma Inflammatory Mediators
Finally, correlations of IRF-8 density and plasma cytokine levels were analyzed (Figure 4 and Supplementary Table 4). Higher IRF-8 density in classical, intermediate and non-classical monocytes all correlated with higher sICAM-1 levels (rho = 0.756; p = 0.0001; rho = 0.600, p = 0.005 and rho = 0.534, p = 0.015, respectively; Figure 4A). Higher IRF-8 density in mDCs and pDCs also correlated with higher sICAM-1 (rho = 0.490, p = 0.030; rho = 0.490, p = 0.028, respectively; Figures 4B,C). Higher IRF-8 density in intermediate monocytes also correlated with higher IFN-γ levels (rho = 0.453; p = 0.045; Figure 4D). No correlations between IRF-8 and the other measured inflammatory mediators were observed (Supplementary Table 4). However, separately, higher sICAM-1 levels correlated with higher learning memory NPZ scores (rho = 0.484, p = 0.036) (Supplementary Table 5). Higher IL-8 and MMP-9 levels correlated with lower NPZ_LM scores (rho = −0.523, p = 0.022) and NPZ_WM (rho = −0.502, p = 0.024) scores, respectively (Supplementary Table 5). Actual values of NPZ scores and soluble inflammatory markers are shown in Supplementary Tables 6, 7, respectively.
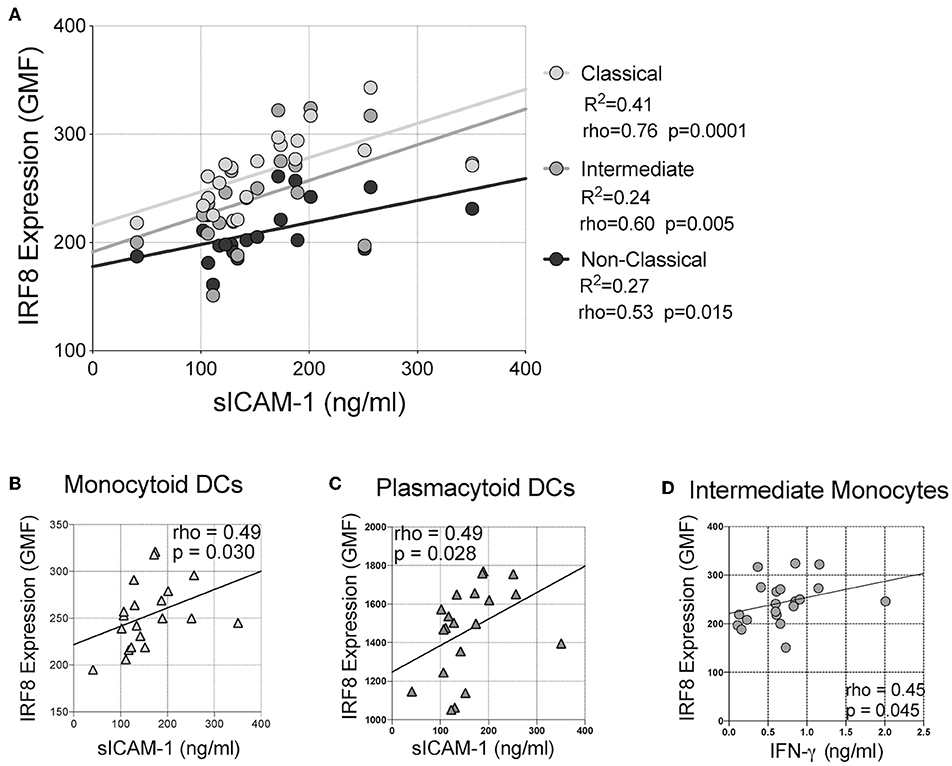
Figure 4. Correlations between IRF-8 expression on myeloid cells and soluble inflammatory markers. Correlations between IRF-8 expression of (A) classical, intermediate, and non-classical monocytes, (B) myeloid and (C) plasmacytoid DCs and soluble intercellular cell adhesion molecule-1 (sICAM-1). (D) Correlation between IRF-8 expression in intermediate monocytes and plasma interferon-gamma (IFN-γ) level.
Discussion
Given our intriguing methylation data demonstrating a link between the IRF8 gene and HIV-associated cognitive impairment, the protein expression of intracellular IRF-8 was investigated in peripheral myeloid cells in virally suppressed HIV-infected individuals with multi-dimensional measures of cognitive performance and brain volumes. Although constitutive IRF-8 expression has been previously reported in myeloid cells, to our knowledge, our data are the first to link reduced IRF-8 protein expression in monocytes and DCs to worse NP testing performance and smaller brain volumes in virally suppressed HIV-infected individuals, suggesting this transcription factor is relevant in the neuropathology of brain health in treated HIV possibly by secretion of cytokines beneficial for CNS function and control of proviral quiescence of HIV.
Both in humans and mice, IRF8 gene mutations are associated with depletions in circulating monocytes and dendritic cells (42) with IRF-8 driving myeloid differentiation. The maintenance of the monocytic homeostatic pool is not affected by deletion of IRF-8 in mice (23, 43, 44). In fact, in our cohort, total and subset myeloid, both monocyte and DC, frequencies were not affected by IRF-8 expression (data not shown).
The functional consequences of higher IRF-8 expression as well as the relationship to CNS measures merits further study. Indicative of systemic inflammation, the presence of inflammatory cytokines can drive IRF-8 expression, which may further influence functional differences in monocyte and DC subsets (45–47). Indeed, IRF-8 is essential for controlling infection in both mice and humans (48, 49). Mutations in IRF8 are associated with increased susceptibility to M. tuberculosis infection (47). Moreover, IRF-8 is crucial for the optimal activation of NLRC4 inflammasome and deletion of the gene induce susceptibility to Salmonella Typhimurium and Burkholderia thailandensis in mice (49). Finally, in the context of viral control, IRF-8 in the cytosol is crucial for NF-kB activation via the TLR9-MyD88 dependent pathway (50). IRF-8 also acts as a transcription factor for the induction of Il12b gene upon TLR stimulation (51, 52). In addition, monocyte cytokine responses to pathogenic stimuli, such as interferon-beta (IFN-β) secretion, can also be synergistically regulated by IRF-8 and IRF-3 binding of the ETS/IRF composite element of the IFN-β promoter (53).
Although inflammatory responses are historically viewed as undesirable in the context of pathological conditions, recent studies, on the other hand, have shown the importance of inflammatory responses that bridge subsequent anti-inflammatory responses, which suggests complicated nature of inflammation (54, 55). In HIV-infected individuals, a previous report highlighted positive correlations of higher IFN-γ, MCP-1, and TNF-α level to higher volumes of the putamen, pallidum and amygdala, whereas higher IL-1β, IL-6, and IL-18 plasma levels were related to lower volumes of the putamen, pallidum, thalamus, hippocampus, and amygdala (56). In line with this report, our data showed the positive correlation between IRF-8, which expression is driven by cytokines including IFN-γ, and brain volume. In a cutaneous wound healing murine model, the down-regulation of IRF-8 by in vivo miRNA-induced silencing complex administration decreased transcription of inflammatory mediators associated with M1 macrophages (IL-1β, IL-6, iNOS, TNF-α) and impaired wound healing at the site of injury (57). Yuan et al. has previously reported that plasma soluble ICAM-5 level was increased in HIV-infected subjects with cognitive impairment compared to HIV-infected subjects with normal cognition (58). Since sICAM-5 suppresses immune activation status (58) whereas sICAM-1 activates the immune system, this supports our results (59). Intriguingly, we found a correlation such that higher plasma sICAM-1 levels correlate with better learning memory NPZ scores (Supplementary Table 5). Furthermore, our findings point toward an interesting correlation found among MMP-9 and learning memory NPZ score such that higher MMP-9 correlated with lower learning memory NPZ scores (Supplementary Table 5). Similarly, higher IL-8 negatively correlated with working memory scores (Supplementary Table 5). MMP-9 has previously been described as inhibiting IL-23 mediated pro-inflammatory responses in dendritic cells while IL-8 is essential for mounting a sufficient T helper cell response (60–62). It has been described that IRF-8 is involved in CCL4 expression which in turn promotes MMP-9 expression in macrophages (63). Additional studies into networked IRF-8, MMP-9, IL-23, and IL-8 activities to decipher the nature of inflammation in people living with HIV are warranted.
Additionally, IRF-8 activity may regulate HIV provirus production. In vitro HIV infection of Jurkat cells has been shown to significantly increase IRF-1 gene expression (42). However, when HIV-infected Jurkat cells were stably transfected with IRF-8, p24 production was decreased compared to the control vector, due to the ability of IRF-8 to inhibit the binding of IRF-1 to Tat, suggesting that IRF-8 is a dominant negative regulator of IRF-1 activity and can block HIV-1 transcription (42). When the chronically HIV-infected pro-monocytic cell line, U1, was treated with a histone deacetylase inhibitor, a concomitant decrease in IRF-8 gene and protein expression was observed along with an increase in gag gene expression (64). This decreased expression of IRF-8 following reactivation of latency suggests a role of IRF-8 in maintaining of proviral quiescence of HIV. Higher IRF-8 levels in the myeloid cells may permit better control of HIV levels within the monocytes which may be a principle mechanism that may maintain viral quiescence and limit neuroinflammation leading to CNS injury manifesting as cognitive impairment (65).
Our observed associations between IRF-8 density and both cognitive performance and regional brain volumes are supported by functional relationships between the relevant regions and NP domains. Consistent with its relationship to better working memory and learning and memory, higher IRF-8 expression in classical monocytes correlated with larger volumes of hippocampus. The involvement of the hippocampus in working memory is well-known (66): in Alzheimer's disease, decline in this domain is associated with hippocampal atrophy (67), as is learning and memory impairment (68). Hippocampal volume has also been positively correlated with memory performance in healthy young individuals (69). The active role played by white matter in learning and memory (70) supports the correlations of IRF-8 expression in intermediate and non-classical monocytes with cerebral white matter volume and with learning and memory. Similarly, correlations of non-classical monocyte expression of IRF-8 with cerebral white matter volume, working memory, executive function, and global NP performance are in line with the literature relating white matter reductions to cognitive decline (71).
Correlations linking the periphery and brain might reflect monocytes migrating into the tissues and carrying out a protective role with regards to HIV infection. IRF-8 expression in monocytes and DCs tracking with NP performance suggests a role for IRF-8 in HIV-related cognitive dysfunction and may highlight a potential therapeutic target. In Vogt-Koyanagi-Harada disease (VKH), monocyte-derived dendritic cells (MDDCs) from active VKH patients have decreased IRF-8 mRNA expression in association with higher methylation levels compared with normal controls or individuals with inactive VKH. Treating DCs the demethylation reagent, 5-Aza-2'-deoxycytidine (DAC), increased IRF-8 mRNA expression by reducing the methylation level of the IRF-8 gene (72). Hypermethylation of IRF-8 in myeloid cells in the context of VKH is similar to our previously published methylation data in HIV (21). Pharmacological interventions targeting demethylation of IRF8 may offer a novel strategy in the context HIV-associated cognitive dysfunction. This may be feasible with the use of microRNA interference as evidenced by murine studies that have enriched pDC frequencies with administration of miR-103 (73). An evaluation of IRF-8 regulatory activity and characterization of affected genomic elements will be beneficial to understand precise mechanisms of IRF-8 function in myeloid cells. Lin et al. demonstrated identification of DC lineage-specific transcription factor regulatory networks for IRF-8 with studies examining consecutive changes of stage-specific expression for key DC regulators are associated with specific histone modifications in promoter and enhancer sequences (74).
Here we report the lowest levels of IRF-8 in peripheral myeloid cells from virally suppressed individuals with chronic HIV correlates with worse cognitive performance. The conclusions of this study are limited by the small sample size of only 20 participants. In addition, the design of this study did not include an HIV-uninfected control group, which limits our ability to conclusively define a causal link between IRF-8 expression and HIV infection. Future longitudinal studies are also necessary to examine the causal relationships between the high IRF-8 expression and HIV-related cognition dysfunction.
Data Availability Statement
All datasets generated for this study are included in the article/Supplementary Material.
Ethics Statement
The study, which has been previously described (25), was approved by the University of Hawaii Manoa Committee on Human Studies. The patients/participants provided their written informed consent to participate in this study.
Author Contributions
MD'A, KK, MC, DO-A, DC, CS, and LN conceived and designed the study. MD'A, KK, and TP performed the experiments. MD'A, KK, TP, TF, EL, and VK analyzed the data. MD'A, TF, and EL wrote the manuscript.
Funding
This work was funded in part by a 2018 New Investigator award (to MD'A) from the University of Washington/Fred Hutch Center for AIDS Research, an NIH funded program under award number AI027757 which was supported by the following NIH Institutes and Centers: NIAID, NCI, NIMH, NIDA, NICHD, NHLBI, NIA, NIGMS, NIDDK. LN receives support from the National Institute of Mental Health (award R01MH104141).
Conflict of Interest
The authors declare that the research was conducted in the absence of any commercial or financial relationship that could be construed as a potential conflict of interest.
Supplementary Material
The Supplementary Material for this article can be found online at: https://www.frontiersin.org/articles/10.3389/fimmu.2019.02789/full#supplementary-material
Supplementary Figure 1. Representative gating strategy for T cells, monocytes, dendritic cells using flow cytometry. After excluding doublets and dead cells, CD4+ and CD8+ T cells were identified from the CD3+ cell population. From the CD3− population, after the exclusion natural killer (NK) and B cells (CD7, CD19, or CD20 positive cells), monocytes, positive for HLA-DR and CD11b, were subset by CD14 and CD16 expression: Classical monocytes (CD14++CD16−), intermediate monocytes (CD14++CD16+) and non-classical monocytes (CD14+CD16+). Dendritic cells (DCs; CD3− CD7− CD19− CD20− CD14− HLA-DR+) were subset into myeloid (CD11c+) or plasmacytoid (CD123+) DCs. IRF-8 expression was then assessed on T cells subsets (CD4+ and CD8+), DC subsets and monocyte subsets.
Supplementary Figure 2. IRF-8 density in each myeloid subset from HCV+ and HCV- subjects. Intracellular IRF-8 expression in (A) monocyte each subset and (B) DC each subset from HCV+ and HCV- subjects.
Supplementary Table 1. Frequencies of each myeloid subset. After performing gating strategy as detailed in Supplementary Figure 1, frequencies of myeloid subsets with respect to total PBMCs are given here.
Supplementary Table 2. Complete analyses of correlations of IRF-8 density and neuropsychological testing correlation. Correlations using Kruskal Wallis testing was used to evaluate associations between IRF-8 expression density and measures of neuropsychological tests, including: learning memory, working memory, executive function, psychomotor, and a composite neuropsychological score of the four domains referred to as global. The values reflected in Figure 2 are reflected here in table format as this table.
Supplementary Table 3. Complete analyses of correlations of IRF-8 density and regional brain volumes. Correlations using Kruskal Wallis testing was used to evaluate associations between IRF-8 expression density and regional brain volumes listed in the table. Values reflected in Figure 3 are reflected here in table format as this table.
Supplementary Table 4. Complete analyses of correlations of IRF-8 density and soluble inflammatory markers. Correlations using Kruskal Wallis testing was used to evaluate associations between IRF-8 expression density and soluble inflammatory markers, including: soluble E-Selectin (sE-Selectin), soluble vascular cell adhesion protein 1 (sVCAM-1), soluble intercellular adhesion molecule 1 (sICAM-1), metallomatrixprotein-9 (MMP-9), myeloperoxidase (MPO), plasminogen activator inhibitor-1 (tPAI-1), C-reactive protein (CRP), serum amyloid A (SAA), serum amyloid P component (SAP), interleukins (IL-1β, IL-6, IL-8, IL-10), Tumor necrosis factor alpha (TNF-α), monocyte chemoattractant protein-1 (MCP-1), vascular endothelial growth factor (VEGF), interferon gamma (IFN-γ). Values reflected in Figure 4 are reflected here in table format as this table.
Supplementary Table 5. Complete analyses of correlations of neuropsychological testing and soluble inflammatory markers. Correlations using Kruskal Wallis testing was used to evaluate associations between neuropsychological testing scores and regional soluble inflammatory markers listed in the table.
Supplementary Table 6. Values of NPZ scores in each subject. Actual values of NPZ scores in each subject are shown in the table.
Supplementary Table 7. Values of soluble inflammatory cytokine levels in each subject. Actual values of soluble inflammatory cytokine levels measured in each subject are shown in the table.
References
1. Tozzi V, Balestra P, Salvatori MF, Vlassi C, Liuzzi G, Giancola ML, et al. Changes in cognition during antiretroviral therapy: comparison of 2 different ranking systems to measure antiretroviral drug efficacy on HIV-associated neurocognitive disorders. J Acquir Immune Defic Syndr. (2009) 52:56–63. doi: 10.1097/QAI.0b013e3181af83d6
2. Heaton RK, Clifford DB, Franklin DR Jr, Woods SP, Ake C, Vaida F, et al. HIV-associated neurocognitive disorders persist in the era of potent antiretroviral therapy: CHARTER Study. Neurology. (2010) 75:2087–96. doi: 10.1212/WNL.0b013e318200d727
3. Heaton RK, Marcotte TD, Mindt MR, Sadek J, Moore DJ, Bentley H, et al. The impact of HIV-associated neuropsychological impairment on everyday functioning. J Int Neuropsychol Soc. (2004) 10:317–31. doi: 10.1017/S1355617704102130
4. Ellis RJ, Deutsch R, Heaton RK, Marcotte TD, McCutchan JA, Nelson JA, et al. Neurocognitive impairment is an independent risk factor for death in HIV infection. San Diego HIV neurobehavioral research center group. Arch Neurol. (1997) 54:416–24. doi: 10.1001/archneur.1997.00550160054016
5. Ickovics JR, Hamburger ME, Vlahov D, Schoenbaum EE, Schuman P, Boland RJ, et al. Mortality, CD4 cell count decline, and depressive symptoms among HIV-seropositive women: longitudinal analysis from the HIV Epidemiology Research Study. JAMA. (2001) 285:1466–74. doi: 10.1001/jama.285.11.1466
6. Shapshak P, Kangueane P, Fujimura RK, Commins D, Chiappelli F, Singer E, et al. Editorial neuroAIDS review. Aids. (2011) 25:123–41. doi: 10.1097/QAD.0b013e328340fd42
7. Kusao I, Shiramizu B, Liang CY, Grove J, Agsalda M, Troelstrup D, et al. Cognitive performance related to HIV-1-infected monocytes. J Neuropsych Clin Neurosci. (2012) 24:71–80. doi: 10.1176/appi.neuropsych.11050109
8. Gonzalez-Scarano F, Martin-Garcia J. The neuropathogenesis of AIDS. Nat Rev Immunol. (2005) 5:69–81. doi: 10.1038/nri1527
9. Sevigny JJ, Albert SM, McDermott MP, McArthur JC, Sacktor N, Conant K, et al. Evaluation of HIV RNA and markers of immune activation as predictors of HIV-associated dementia. Neurology. (2004) 63:2084–90. doi: 10.1212/01.WNL.0000145763.68284.15
10. Cassol E, Misra V, Morgello S, Gabuzda D. Applications and limitations of inflammatory biomarkers for studies on neurocognitive impairment in HIV infection. J Neuroimmune Pharmacol. (2013) 8:1087–97. doi: 10.1007/s11481-013-9512-2
11. Thames AD, Briones MS, Magpantay LI, Martinez-Maza O, Singer EJ, Hinkin CH, et al. The role of chemokine C-C motif ligand 2 genotype and cerebrospinal fluid chemokine C-C motif ligand 2 in neurocognition among HIV-infected patients. AIDS. (2015) 29:1483–91. doi: 10.1097/QAD.0000000000000706
12. Gartner S. HIV infection and dementia. Science. (2000) 287:602–4. doi: 10.1126/science.287.5453.602
13. Anthony IC, Ramage SN, Carnie FW, Simmonds P, Bell JE. Influence of HAART on HIV-related CNS disease and neuroinflammation. J Neuropathol Exp Neurol. (2005) 64:529–36. doi: 10.1093/jnen/64.6.529
14. Burdo TH, Soulas C, Orzechowski K, Button J, Krishnan A, Sugimoto C, et al. Increased monocyte turnover from bone marrow correlates with severity of SIV encephalitis and CD163 levels in plasma. PLoS Pathog. (2010) 6:e1000842. doi: 10.1371/journal.ppat.1000842
15. Herz J, Filiano AJ, Smith A, Yogev N, Kipnis J. Myeloid cells in the central nervous system. Immunity. (2017) 46:943–56. doi: 10.1016/j.immuni.2017.06.007
16. Prinz M, Priller J. The role of peripheral immune cells in the CNS in steady state and disease. Nat Neurosci. (2017) 20:136–44. doi: 10.1038/nn.4475
17. Goldmann T, Wieghofer P, Jordao MJ, Prutek F, Hagemeyer N, Frenzel K, et al. Origin, fate and dynamics of macrophages at central nervous system interfaces. Nat Immunol. (2016) 17:797–805. doi: 10.1038/ni.3423
18. Henderson AP, Barnett MH, Parratt JD, Prineas JW. Multiple sclerosis: distribution of inflammatory cells in newly forming lesions. Ann Neurol. (2009) 66:739–53. doi: 10.1002/ana.21800
19. Gadani SP, Walsh JT, Smirnov I, Zheng J, Kipnis J. The glia-derived alarmin IL-33 orchestrates the immune response and promotes recovery following CNS injury. Neuron. (2015) 85:703–9. doi: 10.1016/j.neuron.2015.01.013
20. Shechter R, Miller O, Yovel G, Rosenzweig N, London A, Ruckh J, et al. Recruitment of beneficial M2 macrophages to injured spinal cord is orchestrated by remote brain choroid plexus. Immunity. (2013) 38:555–69. doi: 10.1016/j.immuni.2013.02.012
21. Corley MJ, Dye C, D'Antoni ML, Byron MM, Yo KL, Lum-Jones A, et al. Comparative DNA methylation profiling reveals an immunoepigenetic signature of HIV-related cognitive impairment. Sci Rep. (2016) 6:33310. doi: 10.1038/srep33310
22. Scheller M, Foerster J, Heyworth CM, Waring JF, Lohler J, Gilmore GL, et al. Altered development and cytokine responses of myeloid progenitors in the absence of transcription factor, interferon consensus sequence binding protein. Blood. (1999) 94:3764–71. doi: 10.1182/blood.V94.11.3764.423k03_3764_3771
23. Tamura T, Ozato K. ICSBP/IRF-8: its regulatory roles in the development of myeloid cells. J Interferon Cytokine Res. (2002) 22:145–52. doi: 10.1089/107999002753452755
24. Becker AM, Michael DG, Satpathy AT, Sciammas R, Singh H, Bhattacharya D. IRF-8 extinguishes neutrophil production and promotes dendritic cell lineage commitment in both myeloid and lymphoid mouse progenitors. Blood. (2012) 119:2003–12. doi: 10.1182/blood-2011-06-364976
25. Zhou JX, Lee CH, Qi CF, Wang H, Naghashfar Z, Abbasi S, et al. IFN regulatory factor 8 regulates MDM2 in germinal center B cells. J Immunol. (2009) 183:3188–94. doi: 10.4049/jimmunol.0803693
26. Zeng Q, Man R, Luo Y, Zeng L, Zhong Y, Lu B, et al. IRF-8 is Involved in Amyloid-beta1–40. (Abeta1–40)-induced microglial activation: a new implication in alzheimer's disease. J Mol Neurosci. (2017) 63:159–64. doi: 10.1007/s12031-017-0966-1
27. Zhou M, Xia X, Yan H, Li S, Bian S, Sha X, et al. The model of aging acceleration network reveals the correlation of alzheimer's disease and aging at system level. Biomed Res Int. (2019) 2019:4273108. doi: 10.1155/2019/4273108
28. Shikuma CM, Seto T, Liang CY, Bennett K, DeGruttola V, Gerschenson M, et al. Vitamin D levels and markers of arterial dysfunction in HIV. AIDS Res Hum Retrovirus. (2012) 28:793–7. doi: 10.1089/aid.2011.0086
29. Antinori A, Arendt G, Becker JT, Brew BJ, Byrd DA, Cherner M, et al. Updated research nosology for HIV-associated neurocognitive disorders. Neurology. (2007) 69:1789–99. doi: 10.1212/01.WNL.0000287431.88658.8b
31. Fastenau PS, Denburg NL, Hufford BJ. Adult norms for the Rey-Osterrieth Complex Figure Test and for supplemental recognition and matching trials from the extended complex figure test. Clin Neuropsychol. (1999) 13:30–47. doi: 10.1076/clin.13.1.30.1976
32. Robert K, Heaton IG, Charles G, Matthews. Comprehensive Norms for An Expanded Halstead-Reitan Battery: Demographic Corrections, Research Findings, And Clinical Applications. Odessa, FL: Psychological Assessment Resources (1991).
33. Dale AM, Fischl B, Sereno MI. Cortical surface-based analysis. I. Segmentation and surface reconstruction. Neuroimage. (1999) 9:179–94. doi: 10.1006/nimg.1998.0395
34. Fischl B, Salat DH, Busa E, Albert M, Dieterich M, Haselgrove C, et al. Whole brain segmentation: automated labeling of neuroanatomical structures in the human brain. Neuron. (2002) 33:341–55. doi: 10.1016/S0896-6273(02)00569-X
35. Fischl B, van der Kouwe A, Destrieux C, Halgren E, Segonne F, Salat DH, et al. Automatically parcellating the human cerebral cortex. Cereb Cortex. (2004) 14:11–22. doi: 10.1093/cercor/bhg087
36. Fischl B, Sereno MI, Dale AM. Cortical surface-based analysis. II: inflation, flattening, and a surface-based coordinate system. Neuroimage. (1999) 9:195–207. doi: 10.1006/nimg.1998.0396
37. Segonne F, Dale AM, Busa E, Glessner M, Salat D, Hahn HK, et al. A hybrid approach to the skull stripping problem in MRI. Neuroimage. (2004) 22:1060–75. doi: 10.1016/j.neuroimage.2004.03.032
38. Sled JG, Zijdenbos AP, Evans AC. A nonparametric method for automatic correction of intensity nonuniformity in MRI data. IEEE Trans Med Imaging. (1998) 17:87–97. doi: 10.1109/42.668698
39. Buckner RL, Head D, Parker J, Fotenos AF, Marcus D, Morris JC, et al. A unified approach for morphometric and functional data analysis in young, old, and demented adults using automated atlas-based head size normalization: reliability and validation against manual measurement of total intracranial volume. Neuroimage. (2004) 23:724–38. doi: 10.1016/j.neuroimage.2004.06.018
40. Shikuma CM, Chow DC, Gangcuangco LM, Zhang G, Keating SM, Norris PJ, et al. Monocytes expand with immune dysregulation and is associated with insulin resistance in older individuals with chronic HIV. PLoS ONE. (2014) 9:e90330. doi: 10.1371/journal.pone.0090330
41. Sichien D, Scott CL, Martens L, Vanderkerken M, Van Gassen S, Plantinga M, et al. IRF8 transcription factor controls survival and function of terminally differentiated conventional and plasmacytoid dendritic cells, respectively. Immunity. (2016) 45:626–40. doi: 10.1016/j.immuni.2016.08.013
42. Sgarbanti M, Borsetti A, Moscufo N, Bellocchi MC, Ridolfi B, Nappi F, et al. Modulation of human immunodeficiency virus 1 replication by interferon regulatory factors. J Exp Med. (2002) 195:1359–70. doi: 10.1084/jem.20010753
43. Tamura T, Tailor P, Yamaoka K, Kong HJ, Tsujimura H, O'Shea JJ, et al. IFN regulatory factor-4 and−8 govern dendritic cell subset development and their functional diversity. J Immunol. (2005) 174:2573–81. doi: 10.4049/jimmunol.174.5.2573
44. Schiavoni G, Mattei F, Sestili P, Borghi P, Venditti M, Morse HC III, et al. ICSBP is essential for the development of mouse type I interferon-producing cells and for the generation and activation of CD8alpha(+) dendritic cells. J Exp Med. (2002) 196:1415–25. doi: 10.1084/jem.20021263
45. Giese NA, Gabriele L, Doherty TM, Klinman DM, Tadesse-Heath L, Contursi C, et al. Interferon. (IFN) consensus sequence-binding protein, a transcription factor of the IFN regulatory factor family, regulates immune responses in vivo through control of interleukin 12 expression. J Exp Med. (1997) 186:1535–46. doi: 10.1084/jem.186.9.1535
46. Wang IM, Contursi C, Masumi A, Ma X, Trinchieri G, Ozato K. An IFN-gamma-inducible transcription factor, IFN consensus sequence binding protein. (ICSBP), stimulates IL-12 p40 expression in macrophages. J Immunol. (2000) 165:271–9. doi: 10.4049/jimmunol.165.1.271
47. Gupta M, Shin DM, Ramakrishna L, Goussetis DJ, Platanias LC, Xiong H, et al. IRF8 directs stress-induced autophagy in macrophages and promotes clearance of Listeria monocytogenes. Nat Commun. (2015) 6:6379. doi: 10.1038/ncomms7379
48. Hambleton S, Salem S, Bustamante J, Bigley V, Boisson-Dupuis S, Azevedo J, et al. IRF8 mutations and human dendritic-cell immunodeficiency. N Engl J Med. (2011) 365:127–38. doi: 10.1056/NEJMoa1100066
49. Karki R, Lee E, Place D, Samir P, Mavuluri J, Sharma BR, et al. IRF8 regulates transcription of naips for NLRC4 inflammasome activation. Cell. (2018) 173:920–33 e13. doi: 10.1016/j.cell.2018.02.055
50. Ikushima H, Negishi H, Taniguchi T. The IRF family transcription factors at the interface of innate and adaptive immune responses. Cold Spring Harb Symp Quant Biol. (2013) 78:105–16. doi: 10.1101/sqb.2013.78.020321
51. Tsujimura H, Tamura T, Kong HJ, Nishiyama A, Ishii KJ, Klinman DM, et al. Toll-like receptor 9 signaling activates NF-kappaB through IFN regulatory factor-8/IFN consensus sequence binding protein in dendritic cells. J Immunol. (2004) 172:6820–7. doi: 10.4049/jimmunol.172.11.6820
52. Savitsky D, Tamura T, Yanai H, Taniguchi T. Regulation of immunity and oncogenesis by the IRF transcription factor family. Cancer Immunol Immunother. (2010) 59:489–510. doi: 10.1007/s00262-009-0804-6
53. Henault J, Martinez J, Riggs JM, Tian J, Mehta P, Clarke L, et al. Noncanonical autophagy is required for type I interferon secretion in response to DNA-immune complexes. Immunity. (2012) 37:986–97. doi: 10.1016/j.immuni.2012.09.014
54. Eming SA, Wynn TA, Martin P. Inflammation and metabolism in tissue repair and regeneration. Science. (2017) 356:1026–30. doi: 10.1126/science.aam7928
55. Maxwell JR, Zhang Y, Brown WA, Smith CL, Byrne FR, Fiorino M, et al. Differential roles for interleukin-23 and interleukin-17 in intestinal immunoregulation. Immunity. (2015) 43:739–50. doi: 10.1016/j.immuni.2015.08.019
56. Gongvatana A, Correia S, Dunsiger S, Gauthier L, Devlin KN, Ross S, et al. Plasma cytokine levels are related to brain volumes in HIV-infected individuals. J Neuroimmune Pharmacol. (2014) 9:740–50. doi: 10.1007/s11481-014-9567-8
57. Guo Y, Yang Z, Wu S, Xu P, Peng Y, Yao M. Inhibition of IRF8 negatively regulates macrophage function and impairs cutaneous wound healing. Inflammation. (2017) 40:68–78. doi: 10.1007/s10753-016-0454-8
58. Yuan L, Wei F, Zhang X, Guo X, Lu X, Su B, et al. Intercellular adhesion molecular-5 as marker in HIV associated neurocognitive disorder. Aging Dis. (2017) 8:250–6. doi: 10.14336/AD.2016.0918
59. Diez-Ruiz A, Kaiser G, Jager H, Birkmann J, Tilz GP, Wachter H, et al. Increased levels of serum intercellular adhesion molecule 1 in HIV infection are related to immune activation. Int Arch Allergy Immunol. (1993) 102:56–60. doi: 10.1159/000236550
60. Twum DY, Colligan SH, Hoffend NC, Katsuta E, Cortes Gomez E, Hensen ML, et al. IFN regulatory factor-8 expression in macrophages governs an antimetastatic program. JCI Insight. (2019) 4:124267. doi: 10.1172/jci.insight.124267
61. Oriss TB, Krishnamoorthy N, Raundhal M, Morse C, Chakraborty K, Khare A, et al. Cutting Edge: MMP-9 inhibits IL-23p19 expression in dendritic cells by targeting membrane stem cell factor affecting lung IL-17 response. J Immunol. (2014) 192:5471–5. doi: 10.4049/jimmunol.1303183
62. Jensen SS, Gad M. Differential induction of inflammatory cytokines by dendritic cells treated with novel TLR-agonist and cytokine based cocktails: targeting dendritic cells in autoimmunity. J Inflamm. (2010) 7:37. doi: 10.1186/1476-9255-7-37
63. Wang Y, Liu T, Yang N, Xu S, Li X, Wang D. Hypoxia and macrophages promote glioblastoma invasion by the CCL4-CCR5 axis. Oncol Rep. (2016) 36:3522–8. doi: 10.3892/or.2016.5171
64. Munier S, Delcroix-Genete D, Carthagena L, Gumez A, Hazan U. Characterization of two candidate genes, NCoA3 and IRF8, potentially involved in the control of HIV-1 latency. Retrovirology. (2005) 2:73. doi: 10.1186/1742-4690-2-73
65. Valcour VG, Ananworanich J, Agsalda M, Sailasuta N, Chalermchai T, Schuetz A, et al. HIV DNA reservoir increases risk for cognitive disorders in cART-naive patients. PLoS ONE. (2013) 8:e70164. doi: 10.1371/journal.pone.0070164
66. Olafsdottir HF, Bush D, Barry C. The role of hippocampal replay in memory and planning. Curr Biol. (2018) 28:R37–50. doi: 10.1016/j.cub.2017.10.073
67. Mortimer JA, Gosche KM, Riley KP, Markesbery WR, Snowdon DA. Delayed recall, hippocampal volume and Alzheimer neuropathology: findings from the Nun Study. Neurology. (2004) 62:428–32. doi: 10.1212/01.WNL.0000106463.66966.65
68. Apostolova LG, Dutton RA, Dinov ID, Hayashi KM, Toga AW, Cummings JL, et al. Conversion of mild cognitive impairment to Alzheimer disease predicted by hippocampal atrophy maps. Arch Neurol. (2006) 63:693–9. doi: 10.1001/archneur.63.5.693
69. Pohlack ST, Meyer P, Cacciaglia R, Liebscher C, Ridder S, Flor H. Bigger is better! Hippocampal volume and declarative memory performance in healthy young men. Brain Struct Funct. (2014) 219:255–67. doi: 10.1007/s00429-012-0497-z
70. Fields RD. Neuroscience. Change in the brain's white matter. Science. (2010) 330:768–9. doi: 10.1126/science.1199139
71. Brickman AM, Zimmerman ME, Paul RH, Grieve SM, Tate DF, Cohen RA, et al. Regional white matter and neuropsychological functioning across the adult lifespan. Biol Psychiatr. (2006) 60:444–53. doi: 10.1016/j.biopsych.2006.01.011
72. Qiu Y, Yu H, Zhu Y, Ye Z, Deng J, Su W, et al. Hypermethylation of interferon regulatory factor 8. (IRF8) confers risk to vogt-koyanagi-harada disease. Sci Rep. (2017) 7:1007. doi: 10.1038/s41598-017-01249-7
73. Li HS, Greeley N, Sugimoto N, Liu YJ, Watowich SS. miR-22 controls Irf8 mRNA abundance and murine dendritic cell development. PLoS ONE. (2012) 7:e52341. doi: 10.1371/journal.pone.0052341
Keywords: HIV-1, IRF-8, interferon, monocytes, dendritic cells, cognition, central nervous system, anti-retroviral therapy
Citation: D'Antoni ML, Kallianpur KJ, Premeaux TA, Corley MJ, Fujita T, Laws EI, Ogata-Arakaki D, Chow DC, Khadka VS, Shikuma CM and Ndhlovu LC (2019) Lower Interferon Regulatory Factor-8 Expression in Peripheral Myeloid Cells Tracks With Adverse Central Nervous System Outcomes in Treated HIV Infection. Front. Immunol. 10:2789. doi: 10.3389/fimmu.2019.02789
Received: 22 August 2019; Accepted: 14 November 2019;
Published: 29 November 2019.
Edited by:
Paul Urquhart Cameron, The University of Melbourne, AustraliaReviewed by:
Laura Fantuzzi, National Institute of Health (ISS), ItalyThomas A. Angelovich, RMIT University, Australia
Copyright © 2019 D'Antoni, Kallianpur, Premeaux, Corley, Fujita, Laws, Ogata-Arakaki, Chow, Khadka, Shikuma and Ndhlovu. This is an open-access article distributed under the terms of the Creative Commons Attribution License (CC BY). The use, distribution or reproduction in other forums is permitted, provided the original author(s) and the copyright owner(s) are credited and that the original publication in this journal is cited, in accordance with accepted academic practice. No use, distribution or reproduction is permitted which does not comply with these terms.
*Correspondence: Lishomwa C. Ndhlovu, bG5kaGxvdnVAaGF3YWlpLmVkdQ==