- 1Division of Immunology and South African Medical Research Council (SAMRC) Immunology of Infectious Diseases, Department of Pathology, Faculty of Health Sciences, University of Cape Town, Cape Town, South Africa
- 2International Centre for Genetic Engineering and Biotechnology (ICGEB), Cape Town, South Africa
- 3Division of Immunology, Faculty of Health Sciences, Institute of Infectious Diseases and Molecular Medicine (IDM), University of Cape Town, Cape Town, South Africa
- 4Faculty of Health Sciences, Wellcome Centre for Infectious Diseases Research in Africa (CIDRI-Africa), Institute of Infectious Diseases and Molecular Medicine (IDM), University of Cape Town, Cape Town, South Africa
Asthma is a chronic debilitating airway disease affecting millions of people worldwide. Although largely thought to be a disease of the first world, it is now clear that it is on the rise in many middle- and lower-income countries. The disease is complex, and its etiology is poorly understood, which explains failure of most treatment strategies. We know that in children, asthma is closely linked to poor lung function in the first 3-years of life, when the lung is still undergoing post-natal alveolarization phase. Epidemiological studies also suggest that environmental factors around that age do play a critical part in the establishment of early wheezing which persists until adulthood. Some of the factors that contribute to early development of asthma in children in Western world are clear, however, in low- to middle-income countries this is likely to differ significantly. The contribution of fungal species in the development of allergic diseases is known in adults and in experimental models. However, it is unclear whether early exposure during perinatal or post-natal lung development influences a protective or promotes allergic asthma. Host immune cells and responses will play a crucial part in early development of allergic asthma. How immune cells and their receptors may recognize fungi and promote allergic asthma or protect by tolerance among other immune mechanisms is not fully understood in this early lung development stage. The aim of this review is to discuss what fungal species are present during early exposure as well as their contribution to the development of allergic responses. We also discuss how the host has evolved to promote tolerance to limit hyper-responsiveness to innocuous fungi, and how host evasion by fungi during early development consequentially results in allergic diseases.
Introduction
Asthma has largely been thought to be a genetic pre-disposition, however, over the last two decades it is becoming more clear that environment may equally play a significant role in the development of the disease (1). Genetic pre-disposition is supported by numerous epidemiological data showing that children born to both parents with asthma or low forced expiratory volume per second (FEV1) are at higher risk of developing asthma (2). Many studies have also linked specific gene loci or single nucleotide polymorphisms (SNPs) to asthma disease in different parts of the world (3). For example, HLA-DQ, SMAD3, IL-2RB1, IL-33, IL-1RL1, IL-4RA, ADAM33, FOXP3, STAT6, and 17q21 loci, have been linked to asthma diseases in diverse ethnic groups with varying degrees (3–5). What is intriguing about asthma is that it is mainly prevalent amongst urban dwellers compared to rural areas in different regions of the world. Although asthma has always been associated with first world countries, it is clear that it is on the rise in middle to lower income countries and in some of these countries the incidence rates may be as high as Western world countries (6, 7). This points to additional factors that can contribute to the rise in asthma disease, apart from genetic pre-disposition. Indeed, environmental factors are now considered as another possible factor that contributes to the increase in asthma. This stems from many epidemiological data mainly from North America, Scandinavian and Eastern European countries that have demonstrated that children raised in farm environments tend to be protected from asthma, whereas those from urban “cleaner” environments are at higher risks (8–10). A term now widely known as “hygiene hypothesis” coined by David Strachan in the late 80s (10–12). The hygiene hypothesis is primarily based on exposure to microbes or high endotoxin levels in the environment in early life which leads to reduced allergic asthma. Hygiene hypothesis may not fully explain the rise of asthma as some studies have disproved this early exposure to high endotoxins and reduced asthma risks (13, 14). A study by Litonjua et al. (15) in children under the age of 5 years exposed to high concentrations of endotoxin in house dust mites were at high risk of developing wheeze over a 46 months period. Other studies have also found variable influence of endotoxin levels in asthma and atopy development in early life depending on dose, exposure and timing (16). Apart from endotoxin level studies, other studies focusing on Bacilli Calmetté-Guerin vaccination and early Mycobacterium tuberculosis infection amongst children in both high- and low-income countries, could not establish an association between infection or immunization with allergen sensitization (17, 18). Other studies have also shown no association between previous infection with Toxoplasma gondii, herpes simplex virus, hepatitis A or Helicobacter pyroli with allergen sensitization and allergic diseases amongst Spanish and German university students (19). Other factors linked to increase in asthma are the rise of industrialization in many countries which generates large amounts of air pollution. In utero pregnant mothers and early life exposure to tobacco smoke is linked to poor lung function and development of chronic obstructive pulmonary disease (COPD) and early mortality (2, 20). Thus, the relationship between early life exposure to microbes or air pollutants and asthma is complex and is likely to be dependent on many factors, such as the nature of exposure, how and when it takes place, host genetic susceptibility and probably many other factors currently not defined (21).
All these do point to the environment being important in asthma pathogenesis. However, other microbes such as fungi have been linked to exacerbations of asthma in both children and adults, suggestive of a non-protective role (22, 23). Naturally, fungi is not detected in high levels in healthy human lung, which had lead researchers to assume that lungs contain no fungus. This is partly due to the fact that inhaled fungal spores are rapidly cleared by both active physical forces such as mucociliary lining the lung epithelium and clearance by innate immune phagocytic cells, such as macrophages. The lack of highly sensitive techniques to detect fungi in sputum or lavage fluid and cumbersome techniques to detect species, partly contributes to low detection of fungi in healthy human lung (24). Nevertheless, some studies have detected certain phyla in healthy respiratory airways including fungi belonging to the genera Cladosporium, Eurotium, Penicillium, and Aspergillus. Other genera such as Candida, Malassezia, and Pneumocystis have been detected, but in low abundance (25). It is likely that some of these species of fungi found in lung sampling may also be part of the oral mycobiome, as techniques used to sample lavage fluid or endoscopy can be contaminated with oral cavity fungi. Some fungi are often detected in sputum or lavage fluid in chronic respiratory diseases such as asthma. These fungal species exploit a compromised host defense system, often germinating, and colonizing the airways, causing mycosis which precedes sensitization and exacerbation of asthma symptoms (26, 27). Some of the fungal species associated with asthma are shown in Table 1. These include genera Aspergillus, Candida, Alternaria, Cladosporium, and Cryptococcus spp. amongst others (35, 40). Some of these species isolated from airways or sinusitis of asthmatic patients with TH2-type disease show viability when cultured in vitro, showing that infection is essential in disease manifestation (27). Importantly, these patients generally show increased fungal-specific IgE responses, memory phenotype when stimulated with fungal antigens and eosinophilia which is directly involved in fungal killing (28, 41). It is now clear that fungal colonization contributes largely to asthma and its severity, however, current methods used to determine colonization rely on culture methods for viable fungi and molecular methods which do not necessarily differentiate between active colonization and fungal fragments. This leads to underestimation of disease burden and bias in distinction between disease and colonization depending on detection methodology used. The mode of sensitization is mainly aerosol exposure, however, some fungal species are commonly found colonizing the skin, for example Malassezia spp. and Trichophyton spp. This suggests that there might have a different mode of sensitization when found on the skin which leads to asthma. These fungal species, although generally found colonizing the skin have also been detected in the both healthy and asthmatic lung and gut which may suggests diverse adaptation in multiple barrier sites (35). In this review, we mainly focus on how some fungi may cause or contribute to allergic asthma, we also discuss how fungi such as Malassezia and Trichophyton that mainly colonize the skin can be important sources of allergens that sensitizes the skin and cause allergic asthma through atopic march (42). We discuss some of the genetic risk factors associated with fungal asthma and tolerance mechanism that host has developed to limit hyperreactivity to these ubiquitous organisms.
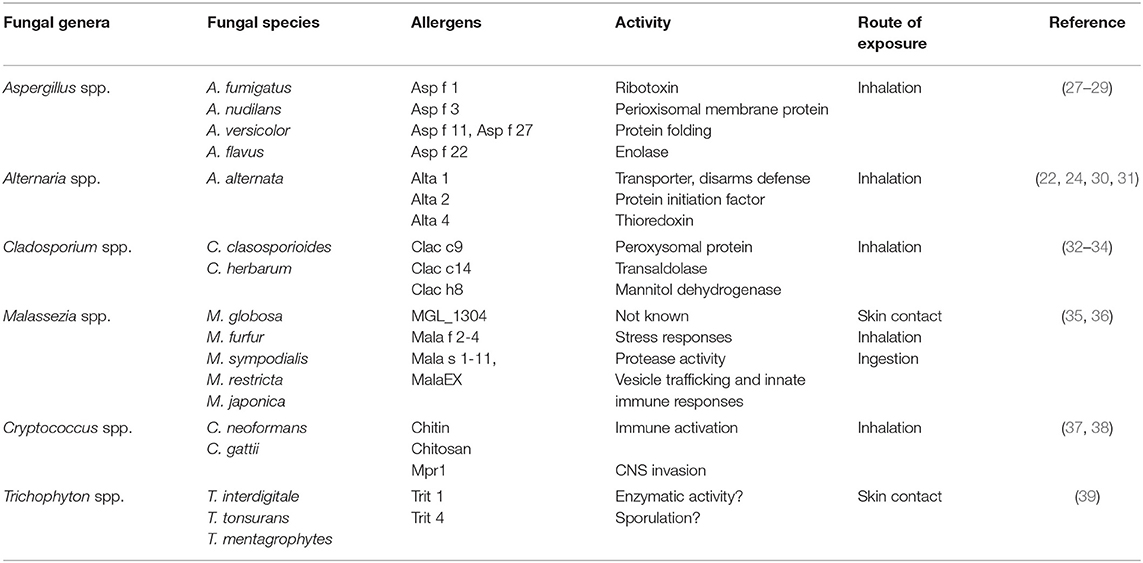
Table 1. Some of the fungal species associated with allergic asthma via various routes of sensitization.
Fungal Sensitization and Asthma Risks
Sensitization to fungi poses a major risk factor for asthma including severe form of asthma and death. Sensitization to fungi is estimated anywhere between 2 and 90% in asthmatics (24, 43). Factors that affects this variation include different exposure, batch of commercially available skin prick test (SPT) extracts and subjective methods used to measure exposure. There is also a high degree of multiple fungal species sensitization, which further complicates these estimates. For example a study done in multiple states within the United States of America using a Pharmacia Capsulated hydrophobic carrier polymer (CAP) system, did show a positive correlation between sensitization to outdoor Alternaria and indoor Cladosporium (44). Four fungal genera namely, Alternaria, Aspergillus, Penicillium, and Cladosporium are overly represented when it comes to measuring fungal allergen exposure (43, 45, 46). This is partly due to airborne abundance, distinct morphological characteristics, geographic locations, and whether indoor or outdoor allergens are being considered.
Highest asthma exacerbations are observed during sporulation seasons, however, most individuals are exposed to either sub-micron (>1 μm) or larger (<1 μm) fungal fragments (23, 43, 47). These fungal fragments are derived from broken or fractured conidia or hyphae and can be easily dislodged by penetrating deeper pockets of the lung at much higher concentrations than spores or conidia (46, 48, 49). This is particularly an efficient aerosolization model for some of the larger fungi such as Alternaria alternata that can be over 10 μm in size and can only penetrate upper respiratory airways (48, 50). Determining whether an individual patient has been sensitized through fungal fragments or live infection is difficult to ascertain without a positive culture, or biopsy for histopathology. However, several case reports have shown that fungal mucosal infection precludes sensitization to that fungus and exacerbates life-threatening allergic asthma (27, 51, 52). Treatment with antifungal drugs and low dose corticosteroids improves asthma symptoms and clears fungal infection, suggesting that airway mycosis may be important in subsequent sensitization (23, 51, 53). It is likely that infectious mycosis instigates sensitization through activation of TH2-type immune response, while fungal fragments derived from hyphae and conidia can exacerbate allergic asthma through their easily accessible fungal pathogen associated molecular patterns (PAMPs) (46, 49). Several animal model studies also support the idea that airway mycosis induces TH2-type airway disease and that some fungal PAMPs may exacerbate allergic asthma in the presence of allergens such as ovalbumin or house dust mite (28, 54–56).
Some studies have suggested that infants are likely to be exposed more than adults and more fungal fragments seem to seed deeper pockets of the lung, which may explain increased risk to fungal induced asthma (49, 57). However, variation in exposure and allergic asthma has also been observed in children from low-income and high-income homes, which may suggests that exposure is also biased based how often one visits a physician. Children from high income homes are likely to be diagnosed with fungal induced asthma simple because of access to healthcare, whereas children from low income homes may even have higher exposure due to living in damp, moldy homes, with less access to healthcare and diagnosis (58).
Genetic Basis for Fungal Induced Asthma
Asthma is a multifactorial complex disease whose origins and pathogenesis are still not clear. Multiple genetic risk factors involved in inflammatory pathway of allergic asthma have been reported. These polymorphisms associated with asthma risk are not present in all patients and seem to be influenced largely by environment. Interleukin 4 receptor alpha (IL-4Rα) and IL-13 polymorphisms are associated with hyper IgE and asthma severity (59, 60). There are currently eight known naturally occurring SNPs of the IL-4RA namely ile75val, glu400ala, cys431arg, ser436leu, ser503pro, gln576arg, ser752ala, and ser786pro (61, 62). Some of these SNPs are associated with A. alternata moderate to severe asthma in children (63). HLA-DQB1*03 and HLA-DRB1*13 have also been associated with A. alternata moderate to severe asthma in children, where HLA-DQB1*03 was reduced in frequency and HLA-DRB1*13 increased in frequency when compared to A. alternata mild asthmatic children (63). These SNPs were specific to fungal sensitization as they were not present in control Allergic Bronchopulmonary Aspergillosis (ABPA) or Chronic Pulmonary Aspergillosis (CPA). Other studies have shown susceptibility variants specific to innate immune responses and pattern recognition. Toll-like receptor 3(TLR-3), TLR-9, DECTIN-1 variants were shown to be associated with severe asthma with fungal sensitization (SAFS) induced by Aspergillus fumigatus in a Caucasian older cohort (64, 65). In another study, DECTIN-1 variant rs58677678 was found to be associated with reduced gene expression leading to unrestrained type 2 immune response and subsequently poor lung function (66). Other SNPs associated with SAFS in this cohort were genes associated with TH2 chemoattractant (CCL17) and monocyte recruitment (CCL2) or immune suppression (IL-10). Interestingly, even in this cohort, there were no correlation in the SNPs between SAFS, atopic asthma and healthy controls (64). These SNPs were specific to SAFS and there were major genetic differences even between closely related ABPA disease (64, 65). To fully appreciate the function of these variants in susceptibility to disease, it would be of great benefit to combine experimental models to delineate function of each variant in that gene. This was perfectly illustrated in patients with invasive Aspergillosis where variant rs35699176 in ZNF77 was shown to cause loss of integrity of the bronchial epithelium and allowed fungal growth (67). This variant was recapitulated in vitro by genetically-editing immortalized epithelial cells via CRISPR/cas9 which were subsequently infected with A. fumigatus conidia. Such studies in SAFS could lead to better understanding of gene function in disease susceptibility, especially in cases where human tissue is not easily accessible. It is worth noting that variants in the study by Knutsen et al. (63) were from 10 year old asthmatic children from multi-ethnic groups. This is relevant in Africa, where there are much larger genetic variations expected compared to Caucasian ancestry.
Several of the genetic polymorphisms associated with fungal allergic asthma are in the innate arm of the immune response to fungi, particularly pattern recognition receptors (PRRs) that recognize evolutionary conserved PAMPs. Although a full discussion is beyond this review, as we have recently reviewed this topic in great length (68), it is worth highlighting PRRs that are implicated in fungal induced asthma. Fungi is covered by cell wall which is mainly composed of outer mannan layer, a β-glucan layer and an inner chitin layer. β-glucan is a sugar found in most fungi and varies in content depending on the fungal species and stage of germination. Beta-glucan is recognized by Dectin-1 mainly by phagocytes and upon recognition initiates a cascade of events including phagocytosis, NF-κB activation, induction of pro-inflammatory genes including GM-CSF, TNF-α and activation of TH2 cytokine secreting cells. Studies in both human and mouse are contradictory regarding the role of Dectin-1 in the induction of TH2 cytokines (68). Several human studies support the idea that Dectin-1 engagement with its ligand favors TH2 environment either through instruction of naïve T cells to secrete TH2-type cytokines (69) or releasing of chemokines (CCL20) associated with TH2 cell recruitment (70). However, other studies suggest that Dectin-1 inhibits TH2 polarization through favoring a TH17 cell polarization or direct inhibition of IL-33 secretion (66, 69). In animal models, the role of Dectin-1 in allergic asthma is even more complicated, with several independent studies showing considerable inconsistences (54, 55, 71–76). Beta-glucan availability on the surface Aspergillus versicolor or Cladosporium cladosporioides live conidia determines the importance of Dectin-1 in TH2 airway immune responses (74). So, future studies should ascertain how each DECTIN-1 variant directly contributes to fungal induced asthma using both purified ligands and multiple fungal species with varying morphologies and growth stages as all these factors are likely to be important.
Toll-like receptors are another group of PRRs that have been shown to bind to fungi and induce pro-inflammatory cytokines (77–79). Several TLRs have been shown to recognize various PAMPs on the surface or released nucleic acid of fungi for example TLR2, TLR3, TLR4, TLR6, and TLR9 promote inflammation and clearance (80). TLRs can bind to fungal PAMPs individually or as heterodimeric clusters and have also been reported to cooperate with CLRs to induce immune responses (77, 81, 82). TLR3 SNP (rs10025405) rare G allele genotype results in susceptibility to SAFS. TLR3 is involved in direct recognition of A. fumigatus and upon recognition by epithelial cells activates a TRIF pathway leading to indoleamine 2,3-dioxygenase activation and cytokine production. Mice deficient of TLR-3 are susceptible to pulmonary A. fumigatus infection which may explain this susceptibility genotype (83). Currently, mechanisms of how TLR-3 or TLR3 SNP (rs100254050) can lead to susceptibility to severe asthma are unclear. TLR9 is an endosomal TLRs whose expression has been shown to be upregulated by Dectin-1 upon stimulation with A. fumigatus conidia or hyphae. TLR-9 regulates airway hyper-responsiveness and inflammation when sensitized and challenged with resting A. fumigatus conidia. Single nucleotide polymorphism in TLR-9 (rs352140) specifically the rare CC and CT genotypes are associated with SAFS (64). This suggests that TLR-9 plays an essential part in the modulating A. fumigatus induced allergic asthma. Mechanistically, it is likely that TLR-9 regulates allergic asthma induced by resisting A. fumigatus through regulating IL-5, IL-13, CCL11, and CCL21 and this process seems to be independent of Dectin-1 (84). During swollen A. fumigatus induced allergic asthma, TLR-9-deficient mice are more susceptible and show increased fungal growth. In this setting, TLR-9 completely regulates dectin-1 expression and by extension IL-17A expression, which explains increased fungal burden in the absence of TLR-9 (84).
Large birth cohort studies that primarily focus on the genetic-environmental interactions in the first year of life with follow-up of these infants up to at least 10 years of age can give greater insights into asthma development. Most risk genetic studies have ignored this crucial early phase where environmental exposure exerts most of its influence in the development of asthma. The importance of these studies was demonstrated in a 17q21 locus risk alleles ORMDL3 and GSDMB, where the same genotype associated with risk was influenced by environment to be protective (21). It would be of great interest to evaluate some of the fungal asthma associated risk alleles in birth cohort studies where environmental exposure at infancy if taken into account.
How Is Allergy Tolerance Generated to Fungal Species?
Fungi is ubiquitous in our environment and it is estimated that in some seasons an individual can be exposed to as high as 50,000 spores per day, yet most individuals do not react and remain non-sensitized (49). Development of tolerance to fungal allergens is intricately regulated by a balance between regulatory T cells (Tregs) which suppress exaggerated inflammation and effector TH2 cells, which are essential in preventing invasive airway mycosis (85). Allergy is usually associated with loss of tolerance to highly allergen-specific TH2 cells and B cells producing IgE (86). In fungal induced TH2-type allergic airway responses, loss of tolerance to fungal allergens can be viewed as an active process aimed at limiting suppressive immune responses in favor of a robust TH2 airway inflammation capable of containing invasive and dissemination of infection to other organs such as the brain (27). How the immune system choses to react to some innocuous antigens or maintain tolerance to majority of inhaled airborne particles is poorly understood. This is particularly of great interest in fungal induced asthma where both active TH2 immunity and immunoregulation are required for initial host protection against invasive fungal species and limiting detrimental pathology. In fact most allergic individuals are extremely tolerant to majority of airborne allergens encountered, except for few that cause aberrant allergic disease (85, 87). Studies specifically focusing on T reg function in allergic neonates suggests an early dysfunction of these cells due to reduced expression CTLA4, TGF-β, resulting in failure in restraining a skewed thymic and peripheral TH2 cells (88). This is also supported by primary immune-deficiency studies in atopic neonates with immune dysregulation, polyendocrinopathy, enteropathy, X-linked (IPEX) syndrome where FOXP3 gene is impaired. These patients present with multiple organ autoimmunity and neonatal development of allergies due to unrestrained TH2 responses at mucosal surfaces (89). There are various mechanisms in which T reg cells can induce immune tolerance to allergens. These mechanisms do include active tolerance where both thymic or peripheral T regs actively suppress allergen specific naïve T cells, allergen ignorance and immunosuppression through secretion of IL-10 by Type 1 T regs (Tr1) (86, 89). The latter is not induced de novo and only seem to play a role in an already developed TH2 allergic disease, which makes it less attractive as therapeutic intervention in most allergic disease, but may well be beneficial in fungal induced asthma, which needs a balanced TH2 immunity and reduced host pathology (86). Two mechanisms seem to be relevant to fungal tolerance, namely direct suppression of allergen-specific TH2 cells by allergen specific T regs and allergen ignorance (85). The former is supported by studies using antigen reactive T cell-enrichment (ARTE) assays in sensitized individuals, where A. fumigatus-specific Treg cells directly compete with A. fumigatus-specific TH2 cells (85). Interestingly, this allergen suppression seems to be specific to particulate A. fumigatus allergens and rare in soluble allergens (85, 90). Allergen ignorance mechanisms of tolerance does suggest that most major fungal allergens are ignored by the immune system of healthy individuals. Studies using MHC multimer enrichment showed that allergen-specific T cells from healthy individuals were neither clonally expanding nor proliferating when exposed to antigen in vitro or during allergen season and resembled a naïve phenotype when compared to asthmatic individuals (91). Currently, it is unclear whether only certain fungal allergens are specific for inducing T regs or all allergens have a potential to induce both TH2 cells and T regs depending on the local cytokine milieu or antigen load. It is likely that pre-disposed individuals without disease possess a reasonable Treg compartment which keeps all airborne allergens at bay. However, during a low-grade fungal infection, this stable T reg compartment is highjacked leading to TH2 allergic immune response which keeps fungal load low and prevent dissemination (27, 85). This idea is supported by studies where chronic exposure to low grade A. niger is able to break tolerance induced by inert ovalbumin antigen in favor of a TH2 immune response (56). It is likely that there are numerous ways in which the human host has co-evolved with fungal airborne species and developed an intricately balanced tolerance vs. necessary inflammation to keep the pathogens out. It is likely that these tolerance mechanisms develop from birth and are nurtured throughout life, acting alone or in synergy with others to limit immunopathology. How tolerance induced by T reg cells toward common ubiquitous fungal airborne allergens is abrogated is of significant interest in the field of fungal induced asthma. Understanding mechanisms of tolerance to airborne fungi initiated by both neonatal and adult human thymic and peripheral Tregs would lead to not only new therapeutics that prevent this break of tolerance, but also interventions that limit immunopathology without compromising active fungal clearance. Appropriate mouse models that closely mimic the spectrum of fungal induced allergic asthma need to be developed in order to delineate mechanisms of tolerance.
Fungal Asthma in Early-Life, How Can We Understand It?
Asthma is thought to be a disease that develops very early in life with almost a third of children under 5 years showing signs of wheezing. Lower respiratory infections contribute to poor lung function in infants who develop early onset of the disease. This early poor lung function is thought to persist until adulthood and is associated with premature mortality (20). Exposure to fungi in the first 2–3 months of life is generally associated with exacerbations of asthma in children (9, 22, 23, 30, 92). However, other studies in large birth cohorts that were followed from birth until age 13 have suggested that indoor fungal sensitization by certain dustborne yeasts were associated with protection (93). The mechanisms of protection to dustborne yeasts are unclear, but it is possible that because of hand-to-mouth feeding habits in infancy, these infants develop tolerance to such airborne yeast due to gut-specific immune responses that favor tolerance toward same ingested yeast (93). Other mechanisms of protection could be due to differences in species on dustborne vs. airborne fungal species which may also result in differential PAMPs exposure and subsequent stimulation of protective PRRs such as TLRs or C-type lectin receptors.
Lung is incompletely developed at birth and completes its post-natal development in the first 3 years of life (94, 95). This post-natal development is largely shaped by encountered external environment and means that the lung remains vulnerable to pathogen colonization during these first 3 years of life (96, 97). What is interesting about these early stages of life, is that pregnant mothers show a TH2 biased immune responses which is transferred to off springs who are also dominated by this skewed TH2 immunity (88). This inherited allergic status of infants from mothers is well-documented and is influenced by other factors including early exposure to microbes, cesarean delivery, breastfeeding, use of probiotics to name a few (96, 98). This TH2 skewed immune response reduces gradually during the first 2 years of life, however, allergic infants have been observed to retain this utero TH2 bias, which is thought to increase wheezing at this stage (99).
The data regarding immunological changes in the airways in early life is scarce and this is mainly due to invasive procedures required to get BAL samples or histopathology samples. This has also made it difficult to match clinical expression of asthma with changes in immune cells. The evidence which suggested both lung histopathological and physiological changes in children before age 3 who went on to develop asthma before pre-school gave some insights on early respiratory symptoms (100). Histopathological features of asthma have been observed after 3 years of age and include airway smooth muscle remodeling, variable reticular basement membrane and eosinophilic infiltration, a feature not seen in wheezing infants under 2 years old (100, 101). The lack of proper bronchoscopy control subjects in these studies makes it difficult to draw conclusions as it is unclear if this remodeling is due to the atopic disease or normal development of the lung. What also complicates these observations is that these infants are usually suspected of having severe respiratory symptoms and would be under corticosteroids, which may supress inflammatory cells such as eosinophils (96, 98).
To fully appreciate early determinants of asthma pathophysiology several studies have recapitulated these early events in animal models of allergic asthma (102–106). At steady state and during HDM exposure, innate lymphoid cells (ILC2s) producing IL-13 dominate the first 10 days of life in an ST2-dependent manner. This creates a TH2-biased immune phenotype, characterized by M2 macrophages that favor resolution of inflammation and protect the vulnerable developing lung (92, 102, 106). ILC2 have also been detected in sputum of children with severe form of asthma not responsive to corticosteroids, which suggests that these cells are essential in the early years in wheezing (107).
In humans, most studies in infants have mainly focussed on how certain bacterial taxa and viruses influence susceptibility to asthma in the first 3 years of life (96, 98). The information on fungal influence in the development of asthma at early stages is scarce and has only been looked at in the context of gut microbiome, where abundance of Candida and Rhodotorula species were associated with wheezing in 12 month old birth cohort (108). There are limited neonatal mouse studies focusing on how fungal species exacerbate allergic asthma. Those limited studies have solely focused on A. alternata, which is mainly associated with severe type of asthma, a disease not only restricted to children, but found in adults too. These studies have shown that A. alternata induces IL-33 dependent steroid resistant asthma (30, 105, 109, 110), which closely resemble that in children non-responsive to oral corticosteroids. This field is still in its infancy and more work is needed to combine asthma clinical data in pediatrics, fungal triggers in those environments and experimental neonatal immunology to decipher context specific disease mechanisms. Considering how little information is available in Africa on fungal sensitization and fungal species associated with different types of asthma, it is conceivable that disease mechanisms will differ, uncovering context specific drivers of allergic diseases. Below, we discuss some of the fungal species that have been associated with asthma.
Alternaria alternata in Asthma
Alternaria sp. belongs to Ascomycota phylum which is the second most abundant fungal species. It is a cosmopolitan organism that is often isolated with outdoor environments, easily growing on dead vegetation or soil samples particularly during cultivation season of grainy plants (111). Alternaria can be found in rural and urban places particularly in warm climates and at higher CO2 levels, conditions that favor sporulation and high antigen content per spore (111). Spores have also been found in indoor environments in places such as basements or evaporative coolers (air-conditioning) where it is thought to be an important source of spores that induce exacerbations (48). Although sensitization to most fungal species is associated with asthma exacerbations, sensitization to A. alternata appears to increase the risk of potentially fatal asthma leading to higher hospital admissions under intensive care units (22, 45, 112). In a 25 year birth cohort prospective study done in the US, sensitization to A. alternata at age 6 was associated with increased hyperresponsiveness from ages 11 to 26 years, even in children who did not show signs of asthma (113). In another prospective study in Western Australia in 9-year-old children, A. alternata sensitization was found to strongly correlate with poor lung function when compared to sensitization to other allergens. Increases in AHR was associated with increase in A. alternata spores during dry season with spore counts reaching 300 spores/m3/day over 1 month (114). In the UK, Central and Eastern Europe, asthma exacerbations, high oral steroid usage and hospitalization have been linked with high A. alternata spore content and high allergen load (30, 31). Other retrospective studies have found as high as 20% A. alternata sensitization amongst mid-year school children from different locations in the US using more sensitive tests such as CAP system (44).
Most of what we know in terms of immune responses to A. alternata has mainly come from mouse studies, where TH2 cells have been demonstrated to play a superior role in the pathogenesis (30, 110, 115–117). In adult asthmatics or children directly sensitized or acutely exposed to A. alternata, immune responses have often not been done. In one pediatric study of children under 16 years, who were sensitized to A. alternata and had SAFS and unresponsive to oral steroids, they were found to have increased levels of IL-33 (30, 109). The mechanisms of steroid resistance induced by A. alternata were further evaluated in a neonatal mouse model where mice were chronically exposed to A. alternata and treated with steroids. IL-33 was found to be essential in A. alternata induced steroid resistant asthma by promoting TH2 cells and ILC2 that were not inhibited by steroids (30). Other studies have also shown a critical part of TH2 cells, ILC2, signaling molecule STAT6 and ST2 receptor in A. alternata induced allergic asthma (110, 115, 116, 118). Although these studies shed some light on immune mechanisms that lead to steroid resistance, it is currently unclear what factors within A. alternata organism leads to severe forms and fatal asthma. This is further complicated by lack of understanding on the function of major allergens, Alta 1 and 2. Serine proteases released by A. alternata have been shown to cleave IL-33, therefore leading to TH2 allergic airway responses (30, 110). More studies are needed from different geographic locations, socioeconomic status and age groups to elucidate the link between early sensitization to A. alternata exposure and development of steroid resistant asthma (44, 113). The function of innate immune responses and PRRs is less studied in both animal models and human challenge models, which can be explained by lack of studies looking at the cell wall properties of this environmentally important fungus.
Aspergillus Species in Asthma
Up to 50% of asthmatics are also sensitized to fungi, presenting with increased IgE specific to fungi. The main culprit fungal species that are often found colonizing the lower airways of the asthmatic lung are A. fumigatus and other thermoresistant Aspergillus genera species (43). A. fumigatus is ubiquitous and is also found in airways of healthy people, where it does not cause any adverse effects and cleared. In A. fumigatus sensitized asthmatics, this fungus is associated with severe asthma which is characterized by frequent bronchiectasis, poor lung function and poor control of asthma. Sensitization to fungi, particularly Aspergillus with severe asthma symptoms has been classified as SAFS (43, 45, 119). Although SAFS is commonly used, however, this term is restricted to adult asthma and moderately used in children, partly because sensitization in children is often associated with immature immune system and difficulty in diagnoses. So, the term that has been adopted is fungal asthma (120).
Aspergillus-associated asthma is often masked or misdiagnosed with other Aspergillus associated diseases such as ABPA which in itself can be worsened by CPA (43, 119, 121, 122). Often, Aspergillus sensitized asthmatics do not meet the criteria for ABPA as their total IgE levels fall well-below threshold of (>410 IU/L or 1,000 ng/mL or >1,000 IU/L) and often present with normal levels of IgG (47, 121, 122). The other defining factors are lack of frequent pulmonary infections or bronchiectasis and fleeting shadows often seen in ABPA patients. CPA differs significantly from allergic asthma as it presents with morbidity associated features such as weight loss, productive cough, shortness of breath, pulmonary/pleural cavitation, localized fibrosis, and sometimes aspergilloma (47, 123). A few randomized clinical trials have shown a beneficial effect of antifungal drug (itraconazole) in more than 60% of patients with severe asthma sensitized to fungi, demonstrated by reduced IgE, exacerbations, and improved lung function and quality of life (124). Vericonazole, another antifungal drug have been shown to be effective against CPA (123), but had no effect in a randomized trial of moderate to severe asthmatics sensitized to A. fumigatus (125). Discontinuation of antifungal therapy is usually associated with rebound of asthma symptoms in both children and adults (124, 126), which highlights the difficulties in controlling environmental exposure to fungal allergens. Corticosteroids are commonly used to alleviate allergic asthma symptoms in SAFS, however they also pose a risk in developing invasive fungal dissemination (40). Immune responses to A. fumigatus associated sensitization are well-described in both animal models (28, 56) and human studies (127).
A. fumigatus lung infection is associated with TH2 cells, cytokines IL-4, IL-5, and IL-13, eosinophils and AHR. Severe form of the diseases is associated with TH17 cells, IL-17A and neutrophilic inflammation (128–130). However, some severe forms of the diseases are associated with hyper IgE and eosinophilia and resistance to corticosteroids (54). Recently, it was shown that there is a considerable cross-reactivity between Candida albican-specific TH17 cells residing in the gut and the vast majority of lung associated aero-allergens from Aspergillus spp. (127). These C. albican-specific T cells broadly promoted pathogenic TH17 cells against A. fumigatus in the lung and were associated with immunopathology. This gut/lung cross-talk has been observed in experimental mouse models where gut axis dysbiosis due to the use of antifungal drugs exacerbates allergic asthma (131, 132). What is characteristic of this lung responses is the proliferation of lung resident A. fumigatus and other aero-allergens as C. albicans disappear in the gut (131).
Cladosporium cladosporiodes in Asthma
Cladosporium spp. are dematiaceous fungi and are found in a wide variety of habits, mainly foods. Cladosporium spp. are prevalent in indoor environments including moldy homes and are associated with severe forms of asthma particularly during sporulating season (133). Cladosporium spp. have been isolated in people's homes and air conditioners at work places and in some cases chronic exposure lead to hypersensitivity pneumonitis, hemorrhagic pneumonia, pulmonary fungal balls, left bronchial obstruction and lesions (32, 33, 134, 135). In some cases, Cladosporium spp. were associated with death, although it is unlikely that it was the sole cause of death, as some of the patients had underlying conditions such as diabetes milieus or cavitary lungs (32, 135). Cladosporium cladosporiodes is the major species detected in most atopic asthmatics followed by Cladosporium herbarum. Some studies have estimated that as high as 38% of atopic asthmatics are sensitized to C. cladosporiodes, which is in a similar range to other aero-allergens such as Aspergillus (136). This is based on immunoblot serum reactivity to major allergens such as serine protease (Cla c14) or peroxisomal protein (Clac c9) (Table 1). It is worth mentioning that there is a certain degree of overlap in amino acid sequence and IgE cross-reactivity between C. cladosporiodes and Penicillium spp., which can complicate diagnosis as both these species have a similar habitat and modes of human exposure (34). The mechanisms of how Cladosporium spp. or its individual components cause or exacerbate asthma are scarce and require further research. Some studies using secreted proteases suggest that proteases can activate allergic airway immune responses by cleaving key components of the lung epithelial barrier such as occludins resulting in a leaky barrier and more access for other allergens which leads to a co-ordinated local inflammatory cascade (137). It is also likely that Cladosporium spp. proteases act in a similar fashion to other fungal proteases by degrading specific endogenous substrates of which their products can bind directly to TLR-4 or to protease activated receptor 2 which then activate downstream signals through their cytoplasmic tails leading to recruitment of eosinophils, innate type 2 lymphoid cells and systemic IgE release (137–140). The importance of C. cladosporiodes proteases in the induction of allergic airway responses has been demonstrated in mouse models of allergic asthma (74, 75). In these models, chronic exposure of mice to live conidia induced a strong TH2 allergic airway disease characterized by eosinophilia, AHR, elevated levels of IgE and mucus production. However, when C. cladosporiodes conidia is inactivated by heat killing, it induced less AHR and allergic responses due to increased exposure of fungal β-glucan and Dectin-1 dependent neutrophilic and TH17 responses (74). These studies suggested that the factor that promotes TH2 allergic asthma in C. cladosporiodes where less β-glucan is exposed is likely to be proteases, although this was not formally investigated in this study.
Cryptococcus neoformans in Asthma
Cryptococcosis is largely associated with immunocompromised patients and is the most common cause of HIV-related meningitis particularly in low CD4 count, killing over 150,000 people in Sub-Saharan Africa (141, 142). Cryptococcus neoformans and Cryptococcus gattii, are the causal agents. These are encapsulated basidiomycete found in the soil, tree bark and bird droppings. The spores or yeast form of fungus can easily be inhaled localizing in the alveoli space (37). M1 macrophages, TH1 and TH17 cells are essential in clearing infection (143). Although the immune response is sufficient to silence Cryptococcus to its dormancy, it is not sufficient to completely sterilize the lung (37, 143). Breakdown of immune defense leads to re-activation of this dormant stage and escape from lung, disseminating to other organs, particularly the brain (144). In immunocompetent individuals, Cryptococcus is thought to cause sub-clinical or asymptomatic pulmonary infections. This is often observed in urban areas that are densely populated and tend to have large pigeon colonies that live side-by-side with humans. Sub-clinical disease correlates with the presence of TH2 cells, M2 macrophages, and related cytokines and are detrimental in controlling dissemination and often associate with worse outcomes (38, 143, 145, 146). A few studies done in Northern America in Bronx area suggested that more than 50% of children over the age of 2 years were exposed to Cryptococcal antigens and their sera reacted to more than six antigens (147, 148). Cryptococcus exposure is associated with increased risk of developing asthma in children (147, 149). There is further evidence in mouse and rat animal models, where Cryptococcus infection exacerbates TH2 allergic airway inflammation (150). Cryptococcus virulent factors such as chitosan, proteases (Mpr1), laccases are the likely culprits in the development of TH2- type allergic asthma (Table 1), through favoring a defective immune arm (37). Encapsulation has been shown not to be essential in the initiation of TH2 type allergic asthma, despite its immune modulatory effects in IL-10 production and TH1 polarization (150, 151). Cryptococci cell wall is covered with chitin, which is known to have TH2-type stimulatory effects (37, 38). Human and mouse, both secrete Acidic Mammalian Chitinases (AMCase) known to digest huge chitin polymers and mediate TH2 airway immune responses (149, 152–154). Chitinases expression is increased in lung biopsies of adult asthmatics (152), although other studies have not shown comparable levels in BAL fluid (153). Children showing signs of exposure to Cryptococcal antigens, have elevated chitinases activity in the BAL fluid (149). A case study of a 10 year old boy also in the Bronx area who was sensitized to multiple fungal species including C. neoformans showed improvement in asthma symptoms such as reduced IgE and lung function when treated with itraconazole (126). Long term prospective studies are required to link early Cryptococcus exposure or infection to poor lung function and early acquisition of allergic asthma in children. What is currently known is that children are exposed to Cryptococcal antigen after the 2 years of life and make antibody responses to at least six antigens of similar nature to the ones observed in rat sera infected with C. neoformans (147, 150). The nature of these antigens is unclear and whether they can be used as diagnostic markers particularly for skin-prick tests or CAP to test for Cryptococcus sensitization. These studies should be strongly supported by experimental model studies to determine which Cryptococcal antigens are essential in establishing allergic asthma at an early age.
Fungal skin sensitization is common particularly in atopic dermatitis (AD) patients. AD patients are often pre-disposed to secondary allergic responses such as asthma. Atopic march is a term used to describe this progression from skin sensitization to allergic asthma (42). Here, we mention two fungal species that are mainly found colonizing the skin surface and often associated with AD and have been associated with allergic asthma. We briefly, discuss the mechanisms on how these skin resident fungi can cause allergic asthma.
Malassezia in Atopic Dermatitis and Asthma
The skin serves as the first barrier to potential harm and is exposed to the largest number of pathogens due to its surface area, yet it contains the most diverse microbiome (155). Although large part of work has been focussed on bacterial species that colonize the skin such as Staphylococcus epidermidis, fungi accounts for almost a quarter of normal skin flora (156). Malassezia spp. colonize the skin of healthy and AD patients (156), but have also been found in sputum of asthmatic patients, which suggests their involvement in allergic asthma (35). Malassezzia spp. are dimorphic, lipophilic skin resident fungi that belongs to the phylum Basidiomycota, with at least 14 species identified so far in human and animal skin (36). The distribution of the species is geographically determined and largely driven by environment for example Malassezia furfur is more prevalent in Japan, whereas Malassezia globota and Malassezia restricta are found in Europe and US (36). Malassezia colonizes the skin as early as 0 and 1 days after birth and increases with time to almost 90% of skin being colonized by teenage years. However, there is considerable discrepancies in the species colonization between different studies (157, 158). A study by Lee et al. showed M. restricta to be common in teenagers and M. globosa predominant in adults over 50 years, whereas Gupta et al. found M. globosa to be more prevalent in younger subjects. Malassezia spp. is associated with at least three skin conditions including Malassezia folliculitis, Seborrheic dermatitis, Pityriasis versicolor, psoriasis and AD. The mechanisms on how Malassezia spp. contribute to any of these diseases are less defined and it is unclear if Malassezia spp. are the root causes of these conditions. In AD, Malassezia spp. is implicated as an aggravating factor, where it is thought that it releases allergens rather than being an infectious agent. The mechanisms of how Malassezia or its allergens promotes pathophysiology in AD patients is not well-defined, but is thought to be due to dysfunctional skin barrier, environmental factors, and other disturbances of normal skin flora, due to immunosuppression that favor mycelia growth over yeast form (36, 159). Once Malassezia spp. allergens enter the disrupted skin barrier, they are recognized by keratinocytes, Langerhan cells and other dermal dendritic cells that recognizes allergens and transport to local draining lymph nodes. This results in TH2 polarization and secretion of IL-4, IL-5, IL-13, and IgE which migrate back to the sight of inflammation further exacerbating AD. Other mechanisms of sensitization that have been suggested, are trafficking of Malassezia spp. allergens via extracellular vesicles (160). These extracellular vesicles (MalaEx) directly interact with keratinocytes and monocytes and are engulfed, becoming a major source of allergens, further exacerbating AD (Table 1) (160). Malassezia spp. sensitization (observed by Malassezia specific IgE skin prick test) is less frequent in children compared to adults (161). M. sympodialis is the most frequently isolated species in AD skin lesions and patients on topical or systemic antifungal treatment show reduced severity of skin symptoms and IgE responses (161, 162). Both toll-like receptors (TLR-2 and TLR-4) and C-type lectin receptors, Mincle and Dectin-2 have been shown to bind specific ligands in Malassezia spp. and inducing specific pro-inflammatory cytokines in mouse macrophages (163, 164). However, these studies have not definitely shown whether these innate receptors promote Malassezia-specific IgE or promote IgE -independent skin inflammation that worsens AD.
Trichophyton interdigitale in Asthma
Trichophyton interdigitale is a dermatophyte that can cause cutaneous infections when absorbed through the skin in millions of people worldwide. Trichophyton can also be inhaled, although it is unable to colonize the lung, it is associated with severe form of asthma (39). In some studies T. interdigitale-specific IgE responses correlate with severity of asthma and history of sensitization suggests childhood exposure (165, 166). Trichophyton and fungal skin infections are more common in immunocompromised people such as those with HIV infection, those receiving systemic corticosteroids and diabetes mellitus (39). In an African setting, there is significantly more people who are immunocompromised due to HIV and diabetes mellitus and develop skin infections, which may influence sensitization to Trichophyton. Trichophyton infections particularly scalp ringworm are predominant in children of African Caribbean or African American descent (167). In Africa, epidemiological data is scarce, however, these infections are likely to be high and probably involve other species of Trichophyton not reported in European, North American and other parts of the world. This early exposure to Trichophyton spp. certainly has profound impact on sensitization and development of allergies.
The immune responses to Trichophyton spp. is not well-defined and has somewhat remained an enigma with some anecdotes regarding its onset (39). It is believed that Trichophyton antigens are absorbed through barrier defected skin and these antigens are then taken up by local Langerhan antigen presenting dendritic cells. These antigen presenting cells then migrate to local draining lymph nodes and present antigens to T cells (39, 165). There are two main dominant immune responses to Trichophyton spp., an immediate hypersensitivity (IH) and delayed hypersensitivity (DHT) (39, 168). Trichophyton-specific IgE and IgG4 and mast cell activation are the main characteristics of IH and also involve urticaria and flare and these are generally associated with TH2 cell activation and cytokines such as IL-4, IL-5 and are localized (166). DHT response usually takes 48 h to come up and is correlated with presence of TH1 cells and IFN-γ production by these activated cells (168). What is intriguing about immune responses to Trichophyton is that some allergens can drive both IH and DHT and stimulate both TH2 and TH1 cell subsets (168). What also remains elusive is how Trichophyton skin sensitized individuals develop allergic airway asthma. Atopic march is a common phenomenon in allergic diseases and is likely at play where the skin colonizers become an important source of chronic exposure. However, this has not been investigated in Trichophyton sensitized individuals with asthma symptoms and animal models have not been investigated, where an allergen, APC and T cell can be tracked in vivo.
Conclusions
Fungal species are ubiquitous, occupying at least 25% of Earth's biomass and are possibly found in every single niche of the human body cohabiting happily and forming part of the mucosal commensals. What is clear is that although the human body has learnt to tolerate fungi in most individuals, there are instances where this tolerance is dysregulated and cause various allergic pathologies. There is a lack of tools to detect fungal associated allergic sensitization and SPT for fungi are not routine. The use of artificial intelligence to predict IgE specific fungal allergens will likely have a bigger impact in hospital settings, particularly in prospective birth cohorts (169). In low- to middle-income countries, especially in Africa, these tools are currently beyond reach. Treatment options using both corticosteroids and antifungal drugs are promising but require further investigations. Antifungal drug access is also not widespread with some drugs either with no cheap generics, not available at all or only prescribed for life-threating infections such meningitis (170). Pharmacokinetics and drug-drug interactions between antifungal drugs and corticosteroids are not clear and require more randomized clinical trials in different population and age groups (120). Current FDA approved monoclonal therapy (omaluzimab or mepoluzimab) might be helpful in certain cases of severe hyper IgE, however, these are beyond reach for many African countries, so antifungal therapy remains the only attractive treatment method for fungal asthma (7, 120). It is important to note that only two countries in Africa have guidelines for asthma management in children, let alone fungal asthma (7, 171). Current SPT test in government hospitals do not include fungal allergens which makes it even harder to diagnose these asthma cases. There are limited studies focusing on the early development of fungal induced asthma and more work is needed to combine what is seen at clinics and experimental models. Long term birth cohort prospective studies are a valuable source of information regarding host genetics-environmental impact in the development of asthma (10, 172). In Africa, prospective birth cohorts to determine risk factors for asthma development are scarce (173, 174). More of these types of studies are needed to comprehensively evaluate impact of changing environment in the steady increase of asthma in Africa. Birth cohorts together with aerodynamic monitoring tools such as low-pressure impactor (ELPI) to specifically determine fungal allergen burden in indoor and outdoor environments will accelerate research in an African context and determine specific triggers of poor lung function and avoid a catastrophe (175).
Author Contributions
SH and FB wrote the manuscript.
Funding
This work was supported by ICGEB, Cape Town Component, Medical Research Council South Africa as well as support by the South African National Research Foundation (NRF) Research Chair initiative (SARChi) and Wellcome Trust CIDRI-Africa (203135Z/16/Z) to FB. NRF Thuthuka Grant (117721), MRC (SA) SIR grant, and Robert Bosch Stiftung Fellowship to SH.
Conflict of Interest
The authors declare that the research was conducted in the absence of any commercial or financial relationships that could be construed as a potential conflict of interest.
References
1. Anderson GP. Endotyping asthma: new insights into key pathogenic mechanisms in a complex, heterogeneous disease. Lancet. (2008) 372:1107–19. doi: 10.1016/S0140-6736(08)61452-X
2. Agustí A, Noell G, Brugada J, Faner R. Lung function in early adulthood and health in later life: a transgenerational cohort analysis. Lancet Respir Med. (2017) 5:935–45. doi: 10.1016/S2213-2600(17)30434-4
3. Torgerson DG, Ampleford EJ, Chiu GY, Gauderman WJ, Gignoux CR, Graves PE, et al. Meta-analysis of genome-wide association studies of asthma in ethnically diverse North American populations. Nat Genet. (2011) 43:887–92. doi: 10.1038/ng.888
4. Demenais F, Margaritte-Jeannin P, Barnes KC, Cookson WOC, Altmüller J, Ang W, et al. Multiancestry association study identifies new asthma risk loci that colocalize with immune-cell enhancer marks. Nat Genet. (2018) 50:42–50. doi: 10.1038/s41588-017-0014-7
5. Ferreira MA, Vonk JM, Baurecht H, Marenholz I, Tian C, Hoffman JD, et al. Shared genetic origin of asthma, hay fever and eczema elucidates allergic disease biology. Nat Genet. (2017) 49:1752–7. doi: 10.1038/ng.3985
6. Adeloye D, Chan KY, Rudan I, Campbell H. An estimate of asthma prevalence in Africa: a systematic analysis. Croat Med J. (2013) 54:519–31. doi: 10.3325/cmj.2013.54.519
7. Kwizera R, Musaazi J, Meya DB, Worodria W, Bwanga F, Kajumbula H, et al. Burden of fungal asthma in Africa: a systematic review and meta-analysis. PLoS ONE. (2019) 14:e0216568. doi: 10.1371/journal.pone.0216568
8. Schuijs MJ, Willart MA, Vergote K, Gras D, Deswarte K, Ege MJ, et al. Farm dust and endotoxin protect against allergy through A20 induction in lung epithelial cells. Science. (2015) 349:1106–10. doi: 10.1126/science.aac6623
9. Stein MM, Hrusch CL, Gozdz J, Igartua C, Pivniouk V, Murray SE, et al. Innate immunity and asthma risk in amish and hutterite farm children. N Engl J Med. (2016) 375:411–21. doi: 10.1056/NEJMoa1508749
10. Kirjavainen P V, Karvonen AM, Adams RI, Täubel M, Roponen M, Tuoresmäki P, et al. Farm-like indoor microbiota in non-farm homes protects children from asthma development. Nat Med. (2019) 25:1089–95. doi: 10.1038/s41591-019-0546-8
11. Wills-Karp M, Santeliz J, Karp CL. The germless theory of allergic disease: revisiting the hygiene hypothesis. Nat Rev Immunol. (2001) 1:69–75. doi: 10.1038/35095579
12. von Mutius E, Vercelli D. Farm living: effects on childhood asthma and allergy. Nat Rev Immunol. (2010) 10:861–8. doi: 10.1038/nri2871
13. Ramsey CD, Celedón JC. The hygiene hypothesis and asthma. Curr Opin Pulm Med. (2005) 11:14–20. doi: 10.1097/01.mcp.0000145791.13714.ae
14. Platts-Mills TAE, Erwin E, Heymann P, Woodfolk J. Is the hygiene hypothesis still a viable explanation for the increased prevalence of asthma? Allergy Eur J Allergy Clin Immunol Suppl. (2005) 60:25–31. doi: 10.1111/j.1398-9995.2005.00854.x
15. Litonjua AA, Milton DK, Celedon JC, Ryan L, Weiss ST, Gold DR. A longitudinal analysis of wheezing in young children: the independent effects of early life exposure to house dust endotoxin, allergens, and pets. J Allergy Clin Immunol. (2002) 110:736–42. doi: 10.1067/mai.2002.128948
16. Celedón JC, Milton DK, Ramsey CD, Litonjua AA, Ryan L, Platts-Mills TA, et al. Exposure to dust mite allergen and endotoxin in early life and asthma and atopy in childhood. J Allergy Clin Immunol. (2007) 120:144–9. doi: 10.1016/j.jaci.2007.03.037
17. Ota MO, van der Sande MA, Walraven GE, Jeffries D, Nyan OA, Marchant A, et al. Absence of association between delayed type hypersensitivity to tuberculin and atopy in children in The Gambia. Clin Exp Allergy. (2003) 33:731–6. doi: 10.1046/j.1365-2222.2003.01599.x
18. Bager P, Rostgaard K, Nielsen NM, Melbye M, Westergaard T. Age at bacille Calmette–Guérin vaccination and risk of allergy and asthma. Clin Exp Allergy. (2003) 33:1512–7. doi: 10.1046/j.1365-2222.2003.01796.x
19. Uter W, Stock C, Pfahlberg A, Guillén-Grima F, Aguinaga-Ontoso I, Brun-Sandiumenge C, et al. Association between infections and signs and symptoms of ‘atopic' hypersensitivity – results of a cross-sectional survey among first-year university students in Germany and Spain. Allergy. (2003) 58:580–4. doi: 10.1034/j.1398-9995.2003.00102.x
20. Vasquez MM, Zhou M, Hu C, Martinez FD, Guerra S. Low lung function in young adult life is associated with early mortality. Am J Respir Crit Care Med. (2017) 195:1399–401. doi: 10.1164/rccm.201608-1561LE
21. Loss GJ, Depner M, Hose AJ, Genuneit J, Karvonen AM, Hyvärinen A, et al. The early development of wheeze. environmental determinants and genetic susceptibility at 17q21. Am J Respir Crit Care Med. (2015) 193:889–97. doi: 10.1164/rccm.201507-1493OC
22. Black PN, Udy AA, Brodie SM. Sensitivity to fungal allergens is a risk factor for life-threatening asthma. Allergy. (2000) 55:501–4. doi: 10.1034/j.1398-9995.2000.00293.x
23. O'Driscoll BR, Hopkinson LC, Denning DW. Mold sensitization is common amongst patients with severe asthma requiring multiple hospital admissions. BMC Pulm Med. (2005) 5:4. doi: 10.1186/1471-2466-5-4
24. Weaver D, Gago S, Bromley M, Bowyer P. The human lung mycobiome in chronic respiratory disease: limitations of methods and our current understanding. Curr Fungal Infect Rep. (2019) 13:109–19. doi: 10.1007/s12281-019-00347-5
25. Tipton L, Ghedin E, Morris A. The lung mycobiome in the next-generation sequencing era. Virulence. (2017) 8:334–41. doi: 10.1080/21505594.2016.1235671
26. Porter PC, Ongeri V, Luong A, Kheradmand F, Corry DB. Seeking common pathophysiology in asthma, atopy and sinusitis. Trends Immunol. (2011) 32:43–9. doi: 10.1016/j.it.2010.11.007
27. Porter PC, Lim DJ, Maskatia ZK, Mak G, Tsai CL, Citardi MJ, et al. Airway surface mycosis in chronic TH2-associated airway disease. J Allergy Clin Immunol. (2014) 134:325–31.e9. doi: 10.1016/j.jaci.2014.04.028
28. Porter P, Susarla SC, Polikepahad S, Qian Y, Hampton J, Kiss A, et al. Link between allergic asthma and airway mucosal infection suggested by proteinase-secreting household fungi. Mucosal Immunol. (2009) 2:504–17. doi: 10.1038/mi.2009.102
29. Williams PB, Barnes CS, Portnoy JM, Environmental Allergens Workgroup. Innate and adaptive immune response to fungal products and allergens. J Allergy Clin Immunol Pract. (2016) 4:386–95. doi: 10.1016/j.jaip.2015.11.016
30. Castanhinha S, Sherburn R, Walker S, Gupta A, Bossley CJ, Buckley J, et al. Pediatric severe asthma with fungal sensitization is mediated by steroid-resistant IL-33. J Allergy Clin Immunol. (2015) 136:312–22.e7. doi: 10.1016/j.jaci.2015.01.016
31. Grewling Ł, Nowak M, Szymanska A, Kostecki Ł, Bogawski P. Temporal variability in the allergenicity of airborne Alternaria spores. Med Mycol. (2018) 57:403–11. doi: 10.1093/mmy/myy069
32. Chiba S, Okada S, Suzuki Y, Watanuki Z, Mitsuishi Y, Igusa R, et al. Cladosporium species-related hypersensitivity pneumonitis in household environments. Intern Med. (2009) 48:363–7. doi: 10.2169/internalmedicine.48.1811
33. Grava S, Lopes FAD, Cavallazzi RS, Grassi MFNN, Svidzinski TIE. A rare case of hemorrhagic pneumonia due to Cladosporium cladosporioides. J Bras Pneumol. (2016) 42:392–394. doi: 10.1590/S1806-37562016000000079
34. Chou H, Tam MF, Chiang C-H, Chou C-T, Tai H-Y, Shen H-D. Transaldolases are novel and immunoglobulin E cross-reacting fungal allergens. Clin Exp Allergy. (2011) 41:739–49. doi: 10.1111/j.1365-2222.2011.03698.x
35. van Woerden HC, Gregory C, Brown R, Marchesi JR, Hoogendoorn B, Matthews IP. Differences in fungi present in induced sputum samples from asthma patients and non-atopic controls: a community based case control study. BMC Infect Dis. (2013) 13:69. doi: 10.1186/1471-2334-13-69
36. Prohic A, Jovovic Sadikovic T, Krupalija-Fazlic M, Kuskunovic-Vlahovljak S. Malassezia species in healthy skin and in dermatological conditions. Int J Dermatol. (2016) 55:494–504. doi: 10.1111/ijd.13116
37. Wozniak KL, Olszewski MA, Wormley FL. Molecules at the interface of Cryptococcus and the host that determine disease susceptibility. Fungal Genet Biol. (2015) 78:87–92. doi: 10.1016/j.fgb.2014.10.013
38. Wiesner DL, Specht CA, Lee CK, Smith KD, Mukaremera L, Lee ST, et al. Chitin recognition via chitotriosidase promotes pathologic type-2 helper T cell responses to cryptococcal infection. PLoS Pathog. (2015) 11:1–28. doi: 10.1371/journal.ppat.1004701
39. Woodfolk JA. Allergy and dermatophytes. Clin Microbiol Rev. (2005) 2005:30–43. doi: 10.1128/CMR.18.1.30-43.2005
40. Fraczek MG, Chishimba L, Niven RM, Bromley M, Simpson A, Smyth L, et al. Corticosteroid treatment is associated with increased filamentous fungal burden in allergic fungal disease. J Allergy Clin Immunol. (2018) 142:407–14. doi: 10.1016/j.jaci.2017.09.039
41. Luong A, Davis LS, Marple BF. Peripheral blood mononuclear cells from allergic fungal rhinosinusitis adults express a Th2 cytokine response to fungal antigens. Am J Rhinol Allergy. (2009) 23:281–7. doi: 10.2500/ajra.2009.23.3311
42. Spergel JM. From atopic dermatitis to asthma: the atopic march. Ann Allergy Asthma Immunol. (2010) 105:99–106. doi: 10.1016/j.anai.2009.10.002
43. Knutsen AP, Bush RK, Demain JG, Denning DW, Dixit A, Fairs A, et al. Fungi and allergic lower respiratory tract diseases. J Allergy Clin Immunol. (2012) 129:280–91. doi: 10.1016/j.jaci.2011.12.970
44. Perzanowski MS, Sporik R, Squillace SP, Gelber LE, Call R, Carter M, et al. Association of sensitization to Alternaria allergens with asthma among school-age children. J Allergy Clin Immunol. (1998) 101:626–32. doi: 10.1016/S0091-6749(98)70170-8
45. Denning DW, O'Driscoll BR, Hogaboam CM, Bowyer P, Niven RM. The link between fungi and severe asthma: a summary of the evidence. Eur Respir J. (2006) 27:615–26. doi: 10.1183/09031936.06.00074705
46. Green BJ, Tovey ER, Sercombe JK, Blachere FM, Beezhold DH, Schmechel D. Airborne fungal fragments and allergenicity. Med Mycol. (2006) 44(Suppl. 1):245–55. doi: 10.1080/13693780600776308
47. Denning DW, Pashley C, Hartl D, Wardlaw A, Godet C, Del Giacco S, et al. Fungal allergy in asthma–state of the art and research needs. Clin Transl Allergy. (2014) 4:14. doi: 10.1186/2045-7022-4-14
48. Pringle A. Asthma and the diversity of fungal spores in air. PLoS Pathog. (2013) 9:1–4. doi: 10.1371/journal.ppat.1003371
49. Cho SH, Seo SC, Schmechel D, Grinshpun SA, Reponen T. Aerodynamic characteristics and respiratory deposition of fungal fragments. Atmos Environ. (2005) 39:5454–65. doi: 10.1016/j.atmosenv.2005.05.042
50. Fröhlich-Nowoisky J, Pickersgill DA, Després VR, Pöschl U. High diversity of fungi in air particulate matter. Proc Natl Acad Sci USA. (2009) 106:12814–9. doi: 10.1073/pnas.0811003106
51. Karim M, Sheikh H, Alam M, Sheikh Y. Disseminated bipolaris infection in an asthmatic patient: case report. Clin Infect Dis. (1993) 17:248–53. doi: 10.1093/clinids/17.2.248
52. Ribeiro NFF, Cousin GCS, Wilson GE, Butterworth DM, Woodwards RTM. Lethal invasive mucormycosis: case report and recommendations for treatment. Int J Oral Maxillofac Surg. (2001) 30:156–9. doi: 10.1054/ijom.2000.0010
53. Chishimba L, Niven RM, Cooley J, Denning DW. Voriconazole and posaconazole improve asthma severity in allergic bronchopulmonary aspergillosis and severe asthma with fungal sensitization. J Asthma. (2012) 49:423–33. doi: 10.3109/02770903.2012.662568
54. Zhang Z, Biagini Myers JM, Brandt EB, Ryan PH, Lindsey M, Mintz-Cole RA, et al. β-Glucan exacerbates allergic asthma independent of fungal sensitization and promotes steroid-resistant TH2/TH17 responses. J Allergy Clin Immunol. (2017) 139:54–65.e8. doi: 10.1016/j.jaci.2016.02.031
55. Hadebe S, Kirstein F, Fierens K, Redelinghuys P, Murray GI, Williams DL, et al. β-Glucan exacerbates allergic airway responses to house dust mite allergen. Respir Res. (2016) 17:35. doi: 10.1186/s12931-016-0352-5
56. Porter PC, Roberts L, Fields A, Knight M, Qian Y, Delclos GL, et al. Necessary and sufficient role for T helper cells to prevent fungal dissemination in allergic lung disease. Infect Immun. (2011) 79:4459–71. doi: 10.1128/IAI.05209-11
57. Kauffman HF, van der Heide S. Exposure, sensitization, and mechanisms of fungus-induced asthma. Curr Allergy Asthma Rep. (2003) 3:430–7. doi: 10.1007/s11882-003-0080-z
58. Barnes C. Fungi and atopy. Clin Rev Allergy Immunol. (2019) 1–10. doi: 10.1007/s12016-019-08750-z
59. Massoud AH, Charbonnier L, Lopez D, Pellegrini M, Phipatanakul W, Chatila TA. An asthma-associated IL4R variant exacerbates airway inflammation by promoting conversion of regulatory T cells to T H 17-like cells. Nat Med. (2016) 22:1–13. doi: 10.1038/nm.4147
60. Hershey GKK, Friedrich MF, Esswein LA, Thomas ML, Chatila TA. The association of atopy with a gain-of-function mutation in the α subunit of the interleukin-4 receptor. N Engl J Med. (1997) 337:1720–5. doi: 10.1056/NEJM199712113372403
61. Ober C, Yao TC. The genetics of asthma and allergic disease: a 21st century perspective. Immunol Rev. (2011) 242:10–30. doi: 10.1111/j.1600-065X.2011.01029.x
62. Ober C, Leavitt SA, Tsalenko A, Howard TD, Hoki DM, Daniel R, et al. Variation in the interleukin 4–receptor α gene confers susceptibility to asthma and atopy in ethnically diverse populations. Am J Hum Genet. (2000) 66:517–26. doi: 10.1086/302781
63. Knutsen AP, Vijay HM, Kariuki B, Santiago LA, Graff R, Wofford JD, et al. Association of IL-4RA single nucleotide polymorphisms, HLA-DR and HLA-DQ in children with Alternaria-sensitive moderate-severe asthma. Clin Mol Allergy. (2010) 8:5. doi: 10.1186/1476-7961-8-5
64. Overton NL, Simpson A, Bowyer P, Denning DW. Genetic susceptibility to severe asthma with fungal sensitization. Int J Immunogenet. (2017) 44:93–106. doi: 10.1111/iji.12312
65. Overton NLD, Denning DW, Bowyer P, Simpson A. Genetic susceptibility to allergic bronchopulmonary aspergillosis in asthma: a genetic association study. Allergy Asthma Clin Immunol. (2016) 12:47. doi: 10.1186/s13223-016-0152-y
66. Gour N, Lajoie S, Smole U, White M, Hu D, Goddard P, et al. Dysregulated invertebrate tropomyosin–dectin-1 interaction confers susceptibility to allergic diseases. Sci Immunol. (2018) 3:eaam9841. doi: 10.1126/sciimmunol.aam9841
67. Gago S, Overton NLD, Ben-Ghazzi N, Novak-Frazer L, Read ND, Denning DW, et al. Lung colonization by Aspergillus fumigatus is controlled by ZNF77. Nat Commun. (2018) 9:3835. doi: 10.1038/s41467-018-06148-7
68. Hadebe S, Brombacher F, Brown GD. C-type lectin receptors in asthma. Front Immunol. (2018) 9:733. doi: 10.3389/fimmu.2018.00733
69. Joo H, Upchurch K, Zhang W, Ni L, Li D, Xue Y, et al. Opposing roles of dectin-1 expressed on human plasmacytoid dendritic cells and myeloid dendritic cells in Th2 polarization. J Immunol. (2015) 195:1723–31. doi: 10.4049/jimmunol.1402276
70. Nathan AT, Peterson EA, Chakir J, Wills-Karp M. Innate immune responses of airway epithelium to house dust mite are mediated through β-glucan-dependent pathways. J Allergy Clin Immunol. (2009) 123:612–8. doi: 10.1016/j.jaci.2008.12.006
71. Werner JL, Gessner MA, Lilly LM, Nelson MP, Metz AE, Horn D, et al. Neutrophils produce interleukin 17A (IL-17A) in a Dectin-1- and IL-23-dependent manner during invasive fungal infection. Infect Immun. (2011) 79:3966–77. doi: 10.1128/IAI.05493-11
72. Lilly LM, Gessner MA, Dunaway CW, Metz AE, Schwiebert L, Weaver CT, et al. The β-glucan receptor dectin-1 promotes lung immunopathology during fungal allergy via IL-22. J Immunol. (2012) 189:3653–60. doi: 10.4049/jimmunol.1201797
73. Ryu JH, Yoo JY, Kim MJ, Hwang SG, Ahn KC, Ryu JC, et al. Distinct TLR-mediated pathways regulate house dust mite-induced allergic disease in the upper and lower airways. J Allergy Clin Immunol. (2013) 131:549–61. doi: 10.1016/j.jaci.2012.07.050
74. Mintz-Cole RA, Brandt EB, Bass SA, Gibson AM, Reponen T, Khurana Hershey GK. Surface availability of beta-glucans is critical determinant of host immune response to Cladosporium cladosporioides. J Allergy Clin Immunol. (2013) 132:159–69. doi: 10.1016/j.jaci.2013.01.003
75. Mintz-Cole RA, Gibson AM, Bass SA, Budelsky AL, Reponen T, Hershey GKK. Dectin-1 and IL-17A suppress murine asthma induced by Aspergillus versicolor but not Cladosporium cladosporioides due to differences in β-glucan surface exposure. J Immunol. (2012) 189:3609–17. doi: 10.4049/jimmunol.1200589
76. Ito T, Hirose K, Norimoto A, Tamachi T, Yokota M, Saku A, et al. Dectin-1 plays an important role in house dust mite-induced allergic airway inflammation through the activation of CD11b+ dendritic cells. J Immunol. (2017) 198:61–70. doi: 10.4049/jimmunol.1502393
77. Netea MG, Gow NA, Munro CA, Bates S, Collins C, Ferwerda G, et al. Immune sensing of Candida albicans requires cooperative recognition of mannans and glucans by lectin and Toll-like receptors. J Clin Invest. (2006) 116:1642–50. doi: 10.1172/JCI27114
78. Ferwerda G, Meyer-Wentrup F, Kullberg BJ, Netea MG, Adema GJ. Dectin-1 synergizes with TLR2 and TLR4 for cytokine production in human primary monocytes and macrophages. Cell Microbiol. (2008) 10:2058–66. doi: 10.1111/j.1462-5822.2008.01188.x
79. Hohl TM, Van Epps HL, Rivera A, Morgan LA, Chen PL, Feldmesser M, et al. Aspergillus fumigatus triggers inflammatory responses by stage-specific β-glucan display. PLoS Pathog. (2005) 1:e30. doi: 10.1371/journal.ppat.0010030
80. Mambula SS, Sau K, Henneke P, Golenbock DT, Levitz SM. Toll-like receptor (TLR) signaling in response to Aspergillus fumigatus. J Biol Chem. (2002) 277:39320–6. doi: 10.1074/jbc.M201683200
81. Sousa Mda G, Reid DM, Schweighoffer E, Tybulewicz V, Ruland J, Langhorne J, et al. Restoration of pattern recognition receptor costimulation to treat chromoblastomycosis, a chronic fungal infection of the skin. Cell Host Microbe. (2011) 9:436–43. doi: 10.1016/j.chom.2011.04.005
82. Dennehy KM, Ferwerda G, Faro-Trindade I, Pyz E, Willment JA, Taylor PR, et al. Syk kinase is required for collaborative cytokine production induced through Dectin-1 and Toll-like receptors. Eur J Immunol. (2008) 38:500–6. doi: 10.1002/eji.200737741
83. Carvalho A, De Luca A, Bozza S, Cunha C, D'Angelo C, Moretti S, et al. TLR3 essentially promotes protective class I–restricted memory CD8+ T-cell responses to Aspergillus fumigatus in hematopoietic transplanted patients. Blood. (2012) 119:967–77. doi: 10.1182/blood-2011-06-362582
84. Ramaprakash H, Ito T, Standiford TJ, Kunkel SL, Hogaboam CM. Toll-like receptor 9 modulates immune responses to Aspergillus fumigatus conidia in immunodeficient and allergic mice. Infect Immun. (2009) 77:108–19. doi: 10.1128/IAI.00998-08
85. Bacher P, Heinrich F, Stervbo U, Nienen M, Vahldieck M, Iwert C, et al. Regulatory T cell specificity directs tolerance versus allergy against Aeroantigens in humans. Cell. (2016) 167:1067–78.e16. doi: 10.1016/j.cell.2016.09.050
86. Bacher P, Scheffold A. The effect of regulatory T cells on tolerance to airborne allergens and allergen immunotherapy. J Allergy Clin Immunol. (2018) 142:1697–709. doi: 10.1016/j.jaci.2018.10.016
87. Palm NW, Rosenstein RK, Medzhitov R. Allergic host defences. Nature. (2012) 484:465–72. doi: 10.1038/nature11047
88. Tulic MK, Andrews D, Crook ML, Charles A, Tourigny MR, Moqbel R, et al. Changes in thymic regulatory T-cell maturation from birth to puberty: differences in atopic children. J Allergy Clin Immunol. (2012) 129:199–206.e4. doi: 10.1016/j.jaci.2011.10.016
89. Sakaguchi S, Yamaguchi T, Nomura T, Ono M. Regulatory T cells and immune tolerance. Cell. (2008) 133:775–87. doi: 10.1016/j.cell.2008.05.009
90. Bacher P, Kniemeyer O, Schönbrunn A, Sawitzki B, Assenmacher M, Rietschel E, et al. Antigen-specific expansion of human regulatory T cells as a major tolerance mechanism against mucosal fungi. Mucosal Immunol. (2013) 7:916. doi: 10.1038/mi.2013.107
91. Wambre E, Bajzik V, DeLong JH, O'Brien K, Nguyen QA, Speake C, et al. A phenotypically and functionally distinct human TH2 cell subpopulation is associated with allergic disorders. Sci Transl Med. (2017) 9:eaam9171. doi: 10.1126/scitranslmed.aam9171
92. Bachus H, Kaur K, Papillion AM, Marquez-Lago TT, Yu Z, Ballesteros-Tato A, et al. Impaired tumor-necrosis-factor-α-driven dendritic cell activation limits lipopolysaccharide-induced protection from allergic inflammation in infants. Immunity. (2019) 50:225–40.e4. doi: 10.1016/j.immuni.2018.11.012
93. Behbod B, Sordillo JE, Hoffman EB, Datta S, Webb TE, Kwan DL, et al. Asthma and allergy development: contrasting influences of yeasts and other fungal exposures. Clin Exp Allergy. (2015) 45:154–63. doi: 10.1111/cea.12401
94. Metzger RJ, Klein OD, Martin GR, Krasnow MA. The branching programme of mouse lung development. Nature. (2008) 453:745–50. doi: 10.1038/nature07005
95. Treutlein B, Brownfield DG, Wu AR, Neff NF, Mantalas GL, Espinoza FH, et al. Reconstructing lineage hierarchies of the distal lung epithelium using single-cell RNA-seq. Nature. (2014) 509:371–5. doi: 10.1038/nature13173
96. Lloyd CM, Saglani S. Development of allergic immunity in early life. Immunol Rev. (2017) 278:101–15. doi: 10.1111/imr.12562
97. Guerra S, Martinez FD. Epidemiology of the origins of airflow limitation in asthma. Proc Am Thorac Soc. (2009) 6:707–11. doi: 10.1513/pats.200908-085DP
98. Jackson DJ, Gern JE, Lemanske RF. Lessons learned from birth cohort studies conducted in diverse environments. J Allergy Clin Immunol. (2017) 139:379–86. doi: 10.1016/j.jaci.2016.12.941
99. Prescott SL, Macaubas C, Smallacombe T, Holt BJ, Sly PD, Loh R, et al. Reciprocal age-related patterns of allergen-specific T-cell immunity in normal vs. atopic infants. Clin Exp Allergy. (1998) 28(suppl. 5):39–44. doi: 10.1046/j.1365-2222.1998.028s5039.x
100. Saglani S, Malmström K, Pelkonen AS, Malmberg LP, Lindahl H, Kajosaari M, et al. Airway remodeling and inflammation in symptomatic infants with reversible airflow obstruction. Am J Respir Crit Care Med. (2005) 171:722–7. doi: 10.1164/rccm.200410-1404OC
101. Lezmi G, Gosset P, Deschildre A, Abou-Taam R, Mahut B, Beydon N, et al. Airway remodeling in preschool children with severe recurrent wheeze. Am J Respir Crit Care Med. (2015) 192:164–71. doi: 10.1164/rccm.201411-1958OC
102. de Kleer IM, Kool M, de Bruijn MJ, Willart M, van Moorleghem J, Schuijs MJ, et al. Perinatal activation of the interleukin-33 pathway promotes type 2 immunity in the developing lung. Immunity. (2016) 45:1285–98. doi: 10.1016/j.immuni.2016.10.031
103. Trompette A, Gollwitzer ES, Yadava K, Sichelstiel AK, Sprenger N, Ngom-Bru C, et al. Gut microbiota metabolism of dietary fiber influences allergic airway disease and hematopoiesis. Nat Med. (2014) 20:159–66. doi: 10.1038/nm.3444
104. Gollwitzer ES, Saglani S, Trompette A, Yadava K, Sherburn R, McCoy KD, et al. Lung microbiota promotes tolerance to allergens in neonates via PD-L1. (2014) 20:3568. doi: 10.1038/nm.3568
105. Saglani S, Gregory LG, Manghera AK, Branchett WJ, Uwadiae F, Entwistle LJ, et al. Inception of early-life allergen–induced airway hyperresponsiveness is reliant on IL-13 + CD4 + T cells. Sci Immunol. (2018) 3:eaan4128. doi: 10.1126/sciimmunol.aan4128
106. Saluzzo S, Gorki AD, Rana BMJ, Martins R, Scanlon S, Starkl P, et al. First-breath-induced type 2 pathways shape the lung immune environment. Cell Rep. (2017) 18:1893–905. doi: 10.1016/j.celrep.2017.01.071
107. Nagakumar P, Denney L, Fleming L, Bush A, Lloyd CM, Saglani S. Type 2 innate lymphoid cells in induced sputum from children with severe asthma. J Allergy Clin Immunol. (2016) 137:624–6.e6. doi: 10.1016/j.jaci.2015.06.038
108. Fujimura KE, Sitarik AR, Havstad S, Lin DL, Levan S, Fadrosh D, et al. Neonatal gut microbiota associates with childhood multisensitized atopy and T cell differentiation. Nat Med. (2016) 22:1187. doi: 10.1038/nm.4176
109. Saglani S, Lui S, Ullmann N, Campbell GA, Sherburn RT, Mathie SA, et al. IL-33 promotes airway remodeling in pediatric patients with severe steroid-resistant asthma. J Allergy Clin Immunol. (2013) 132:676–85.e13. doi: 10.1016/j.jaci.2013.04.012
110. Snelgrove RJ, Gregory LG, Peiró T, Akthar S, Campbell GA, Walker SA, et al. Alternaria-derived serine protease activity drives IL-33-mediated asthma exacerbations. J Allergy Clin Immunol. (2014) 134:583–92.e6. doi: 10.1016/j.jaci.2014.02.002
111. Wolf J, O'Neill RN, Rogers AC, Muilenberg LM, Ziska HL. Elevated atmospheric carbon dioxide concentrations amplify alternaria alternata sporulation and total antigen production. Environ Health Perspect. (2010) 118:1223–8. doi: 10.1289/ehp.0901867
112. Bush RK, Prochnau JJ. Alternaria-induced asthma. J Allergy Clin Immunol. (2004) 113:227–34. doi: 10.1016/j.jaci.2003.11.023
113. Hiranrattana A, Stern DA, Guerra S, Halonen M, Wright AL, Daines M, et al. Alternaria sensitisation at age 6 years is associated with subsequent airway hyper-responsiveness in non-asthmatics. Thorax. (2018) 73:1170–3. doi: 10.1136/thoraxjnl-2017-210325
114. Downs SH, Mitakakis TZ, Marks GB, Car NG, Belousova EG, Leüppi JD, et al. Clinical importance of alternaria exposure in children. Am J Respir Crit Care Med. (2001) 164:455–9. doi: 10.1164/ajrccm.164.3.2008042
115. Doherty TA, Khorram N, Sugimoto K, Sheppard D, Rosenthal P, Cho JY, et al. Alternaria induces STAT6-dependent acute airway eosinophilia and epithelial FIZZ1 expression that promotes airway fibrosis and epithelial thickness. J Immunol. (2012) 188:2622–9. doi: 10.4049/jimmunol.1101632
116. Doherty TA, Khorram N, Lund S, Mehta AK, Croft M, Broide DH. Lung type 2 innate lymphoid cells express cysteinyl leukotriene receptor 1, which regulates TH2 cytokine production. J Allergy Clin Immunol. (2013) 132:205–13. doi: 10.1016/j.jaci.2013.03.048
117. Löser S, Gregory LG, Zhang Y, Schaefer K, Walker SA, Buckley J, et al. Pulmonary ORMDL3 is critical for induction of Alternaria-induced allergic airways disease. J Allergy Clin Immunol. (2017) 139:1496–507.e3. doi: 10.1016/j.jaci.2016.07.033
118. Valladao AC, Frevert CW, Koch LK, Campbell DJ, Ziegler SF. STAT6 regulates the development of eosinophilic versus neutrophilic asthma in response to Alternaria alternata. J Immunol. (2016) 197:4541–51. doi: 10.4049/jimmunol.1600007
119. Denning DW, Pleuvry A, Cole DC. Global burden of allergic bronchopulmonary aspergillosis with asthma and its complication chronic pulmonary aspergillosis in adults. Med Mycol. (2013) 51:361–70. doi: 10.3109/13693786.2012.738312
120. Moss RB. Treatment options in severe fungal asthma and allergic bronchopulmonary aspergillosis. Eur Respir J. (2014) 43:1487–500. doi: 10.1183/09031936.00139513
121. Agarwal R, Nath A, Aggarwal AN, Gupta D, Chakrabarti A. Aspergillus hypersensitivity and allergic bronchopulmonary aspergillosis in patients with acute severe asthma in a respiratory intensive care unit in North India. Mycoses. (2010) 53:138–43. doi: 10.1111/j.1439-0507.2008.01680.x
122. Agarwal R, Gupta D. Severe asthma and fungi: current evidence. Med Mycol. (2011) 49(Suppl 1):150–7. doi: 10.3109/13693786.2010.504752
123. Camuset J, Nunes H, Dombret MC, Bergeron A, Henno P, Philippe B, et al. Treatment of chronic pulmonary aspergillosis by voriconazole in nonimmunocompromised patients. Chest. (2007) 131:1435–41. doi: 10.1378/chest.06-2441
124. Denning DW, O'Driscoll BR, Powell G, Chew F, Atherton GT, Vyas A, et al. Randomized controlled trial of oral antifungal treatment for severe asthma with fungal sensitization: the Fungal Asthma Sensitization Trial (FAST) study. Am J Respir Crit Care Med. (2009) 179:11–8. doi: 10.1164/rccm.200805-737OC
125. Agbetile J, Bourne M, Fairs A, Hargadon B, Desai D, Broad C, et al. Effectiveness of voriconazole in the treatment of Aspergillus fumigatus–associated asthma (EVITA3 study). J Allergy Clin Immunol. (2014) 134:33–9. doi: 10.1016/j.jaci.2013.09.050
126. Vicencio AG, Muzumdar H, Tsirilakis K, Kessel A, Nandalike K, Goldman DL. Severe asthma with fungal sensitization in a child: response to itraconazole therapy. Pediatrics. (2010) 125:1255–8. doi: 10.1542/peds.2009-2443
127. Bacher P, Hohnstein T, Beerbaum E, Röcker M, Blango MG, Kaufmann S, et al. Human anti-fungal Th17 immunity and pathology rely on cross-reactivity against Candida albicans. Cell. (2019) 176:1340–55.e15. doi: 10.1016/j.cell.2019.01.041
128. Chen F, Liu Z, Wu W, Rozo C, Bowdridge S, Millman A, et al. An essential role for the Th2-type response in limiting tissue damage during helmit infection. Natl Inst Heal. (2012) 18:260–6. doi: 10.1038/nm.2628
129. Fei M, Bhatia S, Oriss TB, Yarlagadda M, Khare A, Akira S, et al. TNF-alpha from inflammatory dendritic cells (DCs) regulates lung IL-17A/IL-5 levels and neutrophilia versus eosinophilia during persistent fungal infection. Proc Natl Acad Sci USA. (2011) 108:5360–5. doi: 10.1073/pnas.1015476108
130. Hadebe S, Kirstein F, Fierens K, Chen K, Drummond RA, Vautier S, et al. Microbial ligand costimulation drives neutrophilic steroid-refractory asthma. PLoS ONE. (2015) 10:e0137945. doi: 10.1371/journal.pone.0137945
131. Wheeler ML, Limon JJ, Bar AS, Leal CA, Gargus M, Tang J, et al. Immunological consequences of intestinal fungal dysbiosis. Cell Host Microbe. (2016) 19:865–73. doi: 10.1016/j.chom.2016.05.003
132. Li X, Leonardi I, Semon A, Doron I, Gao IH, Putzel GG, et al. Response to fungal dysbiosis by gut-resident CX3CR1+ mononuclear phagocytes aggravates allergic airway disease. Cell Host Microbe. (2018) 24:847–56.e4. doi: 10.1016/j.chom.2018.11.003
133. Chowdhary A, Agarwal K, Kathuria S, Gaur SN, Randhawa HS, Meis JF. Allergic bronchopulmonary mycosis due to fungi other than Aspergillus: a global overview. Crit Rev Microbiol. (2014) 40:30–48. doi: 10.3109/1040841X.2012.754401
134. Feldman GJ. Mucoid lesion obstructing left main bronchus associated with isolation of cladosporium fungal species. J Bronchology Interv Pulmonol. (1999) 6:9. doi: 10.1097/00128594-199907000-00009
135. Kwon-Chung KJ, Schwartz IS, Rybak BJ. A pulmonary fungus ball produced by Cladosporium cladosporioides. Am J Clin Pathol. (1975) 64:564–8. doi: 10.1093/ajcp/64.4.564
136. Chou H, Tam MF, Lee LH, Chiang CH, Tai HY, Panzani RC, et al. Vacuolar serine protease is a major allergen of Cladosporium cladosporioides. Int Arch Allergy Immunol. (2008) 146:277–86. doi: 10.1159/000121462
137. Li E, Knight JM, Wu Y, et al. Chapter four–airway mycosis in allergic airway disease. Adv Immunol. (2019) 142:85–140. doi: 10.1016/bs.ai.2019.05.002
138. Millien VO, Lu W, Shaw J, Yuan X, Mak G, Roberts L, et al. Cleavage of fibrinogen by proteinases elicits allergic responses through Toll-like receptor 4. Science. (2013) 341:792–6. doi: 10.1126/science.1240342
139. Lewkowich IP, Day SB, Ledford JR, Zhou P, Dienger K, Wills-Karp M, et al. Protease-activated receptor 2 activation of myeloid dendritic cells regulates allergic airway inflammation. Respir Res. (2011) 12:122. doi: 10.1186/1465-9921-12-122
140. Kheradmand F, Kiss A, Xu J, Lee S-H, Kolattukudy PE, Corry DB. A protease-activated pathway underlying Th cell type 2 activation and allergic lung disease. J Immunol. (2002) 169:5904–11. doi: 10.4049/jimmunol.169.10.5904
141. Park BJ, Wannemuehler KA, Marston BJ, Govender N, Pappas PG, Chiller TM. Estimation of the current global burden of cryptococcal meningitis among persons living with HIV/AIDS. AIDS. (2009) 23:525–30. doi: 10.1097/QAD.0b013e328322ffac
142. Rajasingham R, Smith RM, Park BJ, Jarvis JN, Govender NP, Chiller TM, et al. Global burden of disease of HIV-associated cryptococcal meningitis: an updated analysis. Lancet Infect Dis. (2017) 17:873–81. doi: 10.1016/S1473-3099(17)30243-8
143. Leopold Wager CM, Wormley Jr FL. Classical versus alternative macrophage activation: the Ying and the Yang in host defense against pulmonary fungal infections. Mucosal Immunol. (2014) 7:1023. doi: 10.1038/mi.2014.65
144. Walsh NM, Botts MR, McDermott AJ, Ortiz SC, Wüthrich M, Klein B, et al. Infectious particle identity determines dissemination and disease outcome for the inhaled human fungal pathogen Cryptococcus. PLOS Pathog. (2019) 15:e1007777. doi: 10.1371/journal.ppat.1007777
145. Müller U, Piehler D, Stenzel W, Köhler G, Frey O, Held J, et al. Lack of IL-4 receptor expression on T helper cells reduces T helper 2 cell polyfunctionality and confers resistance in allergic bronchopulmonary mycosis. Mucosal Immunol. (2012) 5:299. doi: 10.1038/mi.2012.9
146. Piehler D, Eschke M, Schulze B, Protschka M, Müller U, Grahnert A, et al. The IL-33 receptor (ST2) regulates early IL-13 production in fungus-induced allergic airway inflammation. Mucosal Immunol. (2016) 9:937–49. doi: 10.1038/mi.2015.106
147. Goldman DL, Khine H, Abadi J, Lindenberg DJ, Pirofski La, Niang R, et al. Serologic evidence for cryptococcus neoformans infection in early childhood. Pediatrics. (2001) 107:1–6. doi: 10.1542/peds.107.5.e66
148. Abadi J, Pirofski L. Antibodies reactive with the cryptococcal capsular polysaccharide glucuronoxylomannan are present in sera from children with and without human immunodeficiency virus infection. J Infect Dis. (2002) 180:915–9. doi: 10.1086/314953
149. Goldman DL, Li X, Tsirilakis K, Andrade C, Casadevall A, Vicencio AG. Increased chitinase expression and fungal-specific antibodies in the bronchoalveolar lavage fluid of asthmatic children. Clin Exp Allergy. (2012) 42:523–30. doi: 10.1111/j.1365-2222.2011.03886.x
150. Goldman DL, Davis J, Bommarito F, Shao X, Casadevall A. Enhanced allergic inflammation and airway responsiveness in rats with chronic cryptococcus neoformans infection: potential role for fungal pulmonary infection in the pathogenesis of asthma. J Infect Dis. (2006) 193:1178–86. doi: 10.1086/501363
151. Retini C, Kozel TR, Pietrella D, Monari C, Bistoni F, Vecchiarelli A. Interdependency of interleukin-10 and interleukin-12 in regulation of T-cell differentiation and effector function of monocytes in response to stimulation with Cryptococcus neoformans. Infect Immun. (2001) 69:6064–73. doi: 10.1128/IAI.69.10.6064-6073.2001
152. Zhu Z, Zheng T, Homer RJ, Kim YK, Chen NY, Cohn L, et al. Acidic mammalian chitinase in asthmatic Th2 inflammation and IL-13 pathway activation. Science. (2004) 304:1678–82. doi: 10.1126/science.1095336
153. Van Dyken SJ, Liang HE, Naikawadi RP, Woodruff PG, Wolters PJ, Erle DJ, et al. Spontaneous chitin accumulation in airways and age-related fibrotic lung disease. Cell. (2017) 169:497–509. doi: 10.1016/j.cell.2017.03.044
154. Vannella KM, Ramalingam TR, Hart KM, de Queiroz Prado R, Sciurba J, Barron L, et al. Acidic chitinase primes the protective immune response to gastrointestinal nematodes. Nat Immunol. (2016) 17:538–44. doi: 10.1038/ni.3417
155. Belkaid Y, Tamoutounour S. The influence of skin microorganisms on cutaneous immunity. Nat Rev Immunol. (2016) 16:353–66. doi: 10.1038/nri.2016.48
156. Oh J, Byrd AL, Deming C, Conlan S, Kong HH, et al. Biogeography and individuality shape function in the human skin metagenome. Nature. (2014) 514:59. doi: 10.1038/nature13786
157. Gupta AK, Kohli Y. Prevalence of Malassezia species on various body sites in clinically healthy subjects representing different age group. Med Mycol. (2004) 42:35–42. doi: 10.1080/13693780310001610056
158. Lee YW, Yim SM, Lim SH, Choe YB, Ahn KJ. Quantitative investigation on the distribution of Malassezia species on healthy human skin in Korea. Mycoses. (2006) 49:405–10. doi: 10.1111/j.1439-0507.2006.01239.x
159. Glatz M, Bosshard P, Schmid-Grendelmeier P. The role of fungi in atopic dermatitis. Immunol Allergy Clin North Am. (2017) 37:63–74. doi: 10.1016/j.iac.2016.08.012
160. Johansson HJ, Vallhov H, Holm T, Gehrmann U, Andersson A, Johansson C, et al. Extracellular nanovesicles released from the commensal yeast Malassezia sympodialis are enriched in allergens and interact with cells in human skin. Sci Rep. (2018) 8:1–11. doi: 10.1038/s41598-018-27451-9
161. Brodská P, Panzner P, Pizinger K, Schmid-Grendelmeier P. IgE-mediated sensitization to malassezia in atopic dermatitis: More common in male patients and in head and neck type. Dermatitis. (2014) 25:120–6. doi: 10.1097/DER.0000000000000040
162. Svejgaard E, Ølholm Larsen P, Deleuran M, Ternowitz T, Roed-Petersen J, Nilsson J. Treatment of head and neck dermatitis comparing itraconazole 200 mg and 400 mg daily for 1 week with placebo. J Eur Acad Dermatology Venereol. (2004) 18:445–9. doi: 10.1111/j.1468-3083.2004.00963.x
163. Yamasaki S, Matsumoto M, Takeuchi O, Matsuzawa T, Ishikawa E, Sakuma M, et al. C-type lectin Mincle is an activating receptor for pathogenic fungus, Malassezia. Proc Natl Acad Sci USA. (2009) 106:1897–902. doi: 10.1073/pnas.0805177106
164. Ishikawa T, Itoh F, Yoshida S, et al. Identification of distinct ligands for the C-type lectin receptors mincle and dectin-2 in the pathogenic fungus Malassezia. Cell Host Microbe. (2013) 13:477–88. doi: 10.1016/j.chom.2013.03.008
165. Matsuoka H, Niimi A, Matsumoto H, Ueda T, Takemura M, Yamaguchi M, et al. Specific IgE response to trichophyton and asthma severity. Chest. (2009) 135:898–903. doi: 10.1378/chest.08-1783
166. Ward Jr G, Rose G, Karlsson G, Platts-Mills T. Trichophyton asthma: sensitisation of bronchi and upper airways to dermatophyte antigen. Lancet. (1989) 333:859–62. doi: 10.1016/S0140-6736(89)92863-8
167. Fuller LC, Child FC, Midgley G, Higgins EM. Scalp ringworm in south-east London and an analysis of a cohort of patients from a paediatric dermatology department. Br J Dermatol. (2003) 148:985–8. doi: 10.1046/j.1365-2133.2003.05022.x
168. Woodfolk JA, Sung S-SJ, Benjamin DC, Lee JK, Platts-Mills TAE. Distinct human T cell repertoires mediate immediate and delayed-type hypersensitivity to the Trichophyton antigen, Tri r 2. J Immunol. (2000) 165:4379–87. doi: 10.4049/jimmunol.165.8.4379
169. Fontanella S, Frainay C, Murray CS, Simpson A, Custovic A. Machine learning to identify pairwise interactions between specific IgE antibodies and their association with asthma: a cross-sectional analysis within a population-based birth cohort. PLoS Med. (2018) 15:e1002691. doi: 10.1371/journal.pmed.1002691
170. Kneale M, Bartholomew JS, Davies E, Denning DW. Global access to antifungal therapy and its variable cost. J Antimicrob Chemother. (2016) 71:3599–606. doi: 10.1093/jac/dkw325
171. Kling S, Zar HJ, Levin ME, Green RJ, Jeena PM, Risenga SM, et al. Guideline for the management of acute asthma in children: 2013 update. S Afr Med J. (2013) 103(3 Pt 3):199–207. doi: 10.7196/SAMJ.6658
172. Illi S, von Mutius E, Lau S, Niggemann B, Grüber C, Wahn U, et al. Perennial allergen sensitisation early in life and chronic asthma in children: a birth cohort study. Lancet. (2006) 368:763–70. doi: 10.1016/S0140-6736(06)69286-6
173. Gray DM, Turkovic L, Willemse L, Visagie A, Vanker A, Stein DJ, et al. Gray Lung function in African infants in the Drakenstein child health study impact of lower respiratory tract illness. Am J Respir Crit Care Med. (2017) 195:212–20. doi: 10.1164/rccm.201601-0188OC
174. Beran D, Zar HJ, Perrin C, Menezes AM, Burney P. Burden of asthma and chronic obstructive pulmonary disease and access to essential medicines in low-income and middle-income countries. Lancet Respir Med. (2015) 3:159–70. doi: 10.1016/S2213-2600(15)00004-1
Keywords: allergy, severe asthma associated with fungal sensitivity, fungi, Low- and lower-middle-income countries, hyperreactivity
Citation: Hadebe S and Brombacher F (2019) Environment and Host-Genetic Determinants in Early Development of Allergic Asthma: Contribution of Fungi. Front. Immunol. 10:2696. doi: 10.3389/fimmu.2019.02696
Received: 16 July 2019; Accepted: 01 November 2019;
Published: 20 November 2019.
Edited by:
Joshua J. Obar, Dartmouth College, United StatesReviewed by:
Hirohito Kita, Mayo Clinic, United StatesDavid Corry, Baylor College of Medicine, United States
Copyright © 2019 Hadebe and Brombacher. This is an open-access article distributed under the terms of the Creative Commons Attribution License (CC BY). The use, distribution or reproduction in other forums is permitted, provided the original author(s) and the copyright owner(s) are credited and that the original publication in this journal is cited, in accordance with accepted academic practice. No use, distribution or reproduction is permitted which does not comply with these terms.
*Correspondence: Sabelo Hadebe, sabelo.hadebe@uct.ac.za; Frank Brombacher, brombacherfrank@gmail.com