- 1The School of Biomedical and Pharmaceutical Sciences, Guangdong University of Technology, Guangzhou, China
- 2Department of Immunology, Nanjing Medical University, Nanjing, China
The recognition of ω-3 polyunsaturated acids (PUFAs) as essential fatty acids to normal growth and health was realized more than 80 years ago. However, the awareness of the long-term nutritional intake of ω-3 PUFAs in lowering the risk of a variety of chronic human diseases has grown exponentially only since the 1980s (1, 2). Despite the overwhelming epidemiological evidence, many attempts of using fish-oil supplementation to intervene human diseases have generated conflicting and often ambiguous outcomes; null or weak supporting conclusions were sometimes derived in the subsequent META analysis. Different dosages, as well as the sources of fish-oil, may have contributed to the conflicting outcomes of intervention carried out at different clinics. However, over the past decade, mounting evidence generated from genetic mouse models and clinical studies has shed new light on the functions and the underlying mechanisms of ω-3 PUFAs and their metabolites in the prevention and treatment of rheumatoid arthritis, systemic lupus erythematosus (SLE), multiple sclerosis, and type 1 diabetes. In this review, we have summarized the current understanding of the effects as well as the underlying mechanisms of ω-3 PUFAs on autoimmune diseases.
Introduction
Polyunsaturated fatty acids (PUFAs) can be divided into two major classes: ω-3 PUFAs and ω-6 PUFAs, with the primary structural difference at the positions of their double bonds on the carbon chain. ω-6 PUFAs have the first double bonds starting at the sixth carbon, while ω-3 PUFAs starting at the third carbon, from the methyl end of the carbon chain (the ω-carbon) (3). The two major ω-6 PUFAs that are typically consumed in the diet are linoleic acid (18:2; ω-6; LA) and arachidonic acid (20:4; ω-6; AA). Western diets are dominated by ω-6 PUFAs but contain only small amounts of ω-3 PUFAs with the ratio of ω-6/ω-3 reaching as high as 20–30 (4). The three major ω-3 PUFAs are α-linolenic acid (18:3; ω-3; α-LA), eicosapentaenoic acid (20:5; ω-3; EPA), and docosahexaenoic acid (22:6; ω-3; DHA). The α-LA (18:3; ω-3) should not be confused with γ-linolenic acid (GLA) that is also 18:3 but an ω-6 fatty acid. LA (precursor to the ω-6 series of fatty acids) and α-LA (precursor to the ω-3 series of fatty acids) are considered essential fatty acids because they cannot be synthesized in mammals. Starting with LA, mammals can sequentially synthesize GLA via Δ6-desaturase (Fads2) then AA through elongase and Δ5-desaturase (Fads1) (5–7). On the other hand, α-LA is converted to stearidonic acid (C18:4; ω-3) by Δ6-desaturase; stearidonic acid is then elongated and converted to EPA via Δ5-desaturase. EPA add two carbons via elongase to form docosapentaenoic acid (22:5; ω-3; DPA), then add two more carbons via elongase followed by desaturation by Δ6-desaturase to produce tetracosahexaenoic acid (24:6; ω-3); the subsequent, removal of two carbons by β-oxidation yields DHA. Although Δ6-desaturase is widely considered as rate-limiting for the sequential synthesis from ALA to DHA (8–11), a recent study also revealed that elongase reactions (primarily mediated by elongase-5 in humans) were control points as well (12). These enzymatic constraints limit the tissue levels of EPA and DHA. Consequently, we still need to rely on dietary intake to meet the physiological demands of EPA and DHA.
The beneficial effects of ω-3 PUFAs are mediated by themselves as well as by their bioactive metabolites, such as resolvins, protectins, and maresins. Among them, the most studied anti-inflammation metabolites are resolvins which have two classes. E-series resolvins include RvE1, RvE2, and RvE3, all of which are synthesized from EPA by such enzymes as cytochrome P450 (CYP 450), cyclocygenase-2 (COX-2), and 15-lipoxygenase (15-LOX) (13, 14). The D series resolvins (RvD1–RvD6) are derived from DHA by such enzymes as COX-2, 12/15 LOX, and 5-LOX (13, 15–17). These metabolites from ω-3 PUFAs compete with those of ω-6 PUFAs to promote the resolution of the inflammatory cycle (18, 19), and have been increasingly recognized as the important players in the attenuation of inflammation and regulation of autoimmunity (18, 19).
Mounting evidence from both human and animal studies has demonstrated that ω-3 PUFAs, primarily EPA and DHA, can suppress inflammation and have beneficial roles in a variety of human diseases, including autoimmune diseases, diabetes, cancers, Alzheimer's disease (AD), and stroke (3, 20–26). Fish oil is the oil derived from the tissues of oily ocean fish. The main ω-3 PUFAs in fish oil are EPA and DHA. Researchers often use fish oil as the source of ω-3 PUFAs in clinical interventions. Although the effects of ω-3 PUFAs on these diseases have been extensively investigated in the past few decades, a series of recent studies involving the usage of a transgenic genetic model, fat-1 (or mfat-1) transgenic mice, have shed new light into the mechanisms underlying the protective effects of ω-3 PUFAs. The fat-1 transgenic mice, initially generated in Kang's lab (27) [and later the mfat-1 mouse model independently designed by our group (28)] carry a globally expressed Caenorhabditis elegans fat-1 transgene that encodes an ω-3 fatty acid desaturase. The FAT-1 enzyme can convert ω-6 PUFAs to the corresponding ω-3 forms by adding a double bond to the ω-3 position, thus allowing endogenous production of ω-3 PUFAs while reducing the ω-6: ω-3 ratio in tissues without special dietary adjustment (27, 28). The fat-1 transgenic model allows researchers to bypass the traditional lengthy dietary approach of feeding fish-oil to the animals, and have been widely applied to the studies related to autoimmune diseases, tumorigenesis, metabolic, and cardiovascular diseases, as well as neurological diseases (24, 27, 29, 30).
In this review, we choose to focus on the current understanding of the effects of ω-3 fatty acids on such debilitating diseases as rheumatoid arthritis (RA) (31), systemic lupus erythematosus (SLE) (32), type 1 diabetes (T1D) (33), and multiple sclerosis (MS) (34). Although there have been a large body of clinical research articles related to the application of ω-3 PUFAs in different indications, perhaps it should come with no surprise that not all published studies reached the same conclusions. The reasons behind such discrepancy are still unclear although different dose, source, and duration of ω-3 PUFAs treatment may have all played roles in the clinical outcomes. Unfortunately, many of these studies did not report the blood concentrations of EPA/DHA of the enrolled patients, making it difficult to evaluate if the enrolled patients for their studies have indeed gained sufficient EPA/DHA. Thus, for the benefit of readers, we have offered several formatted tables (by no means complete, but certainly representative) detailing the number of patients, dose, duration, and outcomes of each trial (Tables 1–4).
ω-3 PUFAs and Rheumatoid Arthritis
Rheumatoid arthritis (RA) is a chronic autoimmune disease manifested by swollen and painful joints, bone erosion, and functional impairment. The joint lesions are characterized by infiltration of T lymphocytes, macrophages, and B lymphocytes into the synovium, as well as by synovial inflammation induced by PUFAs metabolites, cytokines, and matrix metalloproteinases (58–60). The intervention of RA with ω-3 PUFAs has attracted increasing attention. One of the earliest studies about ω-3 PUFAs' impact on rheumatoid arthritis was reported in Lancet in 1985 (35) (Table 1). In this pilot study, seventeen RA patients consumed a daily supplement of 1.8 g of EPA for 12 weeks. At the end of treatment, the EPA intervention significantly shortened morning stiffness time than the control group at 12 weeks and lessened the number of tender joints at 12 weeks from baseline. Several years following this study, Kremer et al. reported in 1990 that the number of tender joints significantly improved from baseline after both the low- and high-dose intervention with fish oil supplement (36) (Table 1). In addition to such beneficial effects, an ω-6 PUFAs-derived metabolite, leukotriene B4 (LTB4), generated by the neutrophils of the fish oil-treated patients, decreased significantly (36) (Table 1). In a prospective study, sixty patients with active RA were enrolled in a randomized 12-week trial, who were given either fish oil supplement, or fish oil supplement with primrose evening oil (enriched in GLA), or no intervention (37) (Table 1). Daily supplementation of fish oil alone or in combination with primrose evening oil significantly improved the number of tender joints and visual analog scale (VAS) score. There was a sharp decline in the ratio of ω-6/ω-3 fatty acids in the plasma samples from the groups of subjects receiving fish oil, which was strongly linked to the clinical improvements. Beyer et al. reported in a nutrition study involving 78 RA patients, of which 58% had active RA, that consuming the recommended seafood intake was correlated with better RA disease outcome (38) (Table 1). In a separate study, sixteen patients received a daily dose of 2.04 g EPA and 1.32 g DHA for 12 weeks, the results also showed a significant reduction in joint swelling index and duration of early morning stiffness as well as a decrease of LTB4 production by the neutrophils isolated from patients' blood (39) (Table 1). A meta-analysis of 23 similar clinical studies revealed a fairly consistent finding that ω-3 PUFAs had a beneficial effect on joint swelling, pain and morning stiffness, and that there was a significant reduction in the required dose of steroidal anti-inflammatory drugs (40) (Table 1).
In an animal model study, fish-oil feeding in mice delayed the onset and reduced the incidence and severity of type II collagen-induced arthritis compared with the vegetable oil-fed group (61). In the susceptible DBA/1 mouse strain, daily intake of marine ω-3 PUFAs in the form of phospholipids delayed the onset of arthritis, decreased the severity, reduced paw swelling, and knee joint pathology in collagen-induced arthritis (62). Both EPA and DHA have also been shown to suppress Streptococcal-induced arthritis in rats, although EPA appeared to be more effective than DHA (63). As additional proof, endogenous production of ω-3 PUFAs in the fat-1 transgenic mice drastically attenuated arthritis as well as local and systemic levels of inflammatory cytokines following the establishment of RA, whereas the wild type control mice developed overt arthritis (64). Thus, in both animal models and patients, ω-3 PUFAs were able to decrease not only the incidence but also the severity of RA.
The mechanisms underlying ω-3 PUFAs-initiated regulation of immunity in RA can be several. Most studies focused on molecular mediators, including cytokines, metabolites, and reactive oxygen species; others have interrogated the roles of immune cells such as T cells and antigen presenting cells. In the study by Espersen et al. (41) (Table 1), the level of interleukin-1β (IL-1β) in plasma was significantly suppressed in the patients who consumed fish oil by week 6 of the trial, and a further significant decrease was observed a few weeks later. Even in healthy volunteers, a clinical study involving supplementation of fish oil has demonstrated reduction in tumor necrosis factor-alpha (TNF-α), IL-1β, and interleukin-6 (IL-6) in endotoxin-stimulated monocytes cells (65), although the findings from a few other studies did not corroborate these results (66–68). Recently, interleukin-17 (IL-17) has been proposed to be a key cytokine in the onset and development of RA (69, 70). Studies using fish oil supplement found that DHA and EPA have anti-inflammation benefit by reducing the population of interferon-γ (IFN-γ) and IL-17-producing CD4+ T cells in humans and animals (71–74). Thus, suppression of inflammatory cytokines is likely an important contributing factor to the amelioration of clinical signs and symptoms in RA patients. In cultured cells, studies also reported that EPA and DHA could inhibit the production of TNF-α, IL-1β, and IL-6, the classic panel of pro-inflammatory cytokines (75–78). The results derived from these clinical and cellular studies were also corroborated by similar findings in other animal models (24, 30). For example, the fat-1 transgenic mice showed strong anti-inflammatory effects that rendered tissues strongly resistant to cytokines (IL-1β and TNF-α)-induced cell death (24, 30). The inflammatory metabolites, prostaglandin E2 (PGE2) and LTB4, were sharply decreased in the tissues of the fat-1 mice. Endogenous production of ω-3 PUFAs also markedly attenuated cytokine-induced activation of nuclear factor kappa-light-chain-enhancer of activated B cells (NF-κB) and extracellular signal–related kinase 1/2 (ERK1/2) (24, 30).
Our recent study and many other studies revealed that incubation of EPA and DHA with isolated human T cells could inhibit the proliferation of human T cells and their production of IL-2 (79–83). Similar findings were also made in the T-cells isolated from mice (80). In vivo, a dietary gain of ω-3 PUFAs has been shown to correct the imbalance of Th1 and Th2 ratios in such Th1-mediated autoimmune disease models as RA and EAE (84–86). Thus, both the proliferation and differentiation of T cells could be regulated by ω-3 PUFAs. EPA and DHA may have also influenced the function of antigen presenting cells. Studies derived from cultured cells have shown that the expression of major histocompatibility complex (MHC) II and antigen presentation via MHC II could be significantly reduced following the exposure to EPA and DHA (87–89). These findings are also supported by the observations in the mice fed fish-oil (90) or in humans with EPA/DHA supplementation (91). Taken together, these investigations supported the concept that ω-3 PUFAs can decrease levels of inflammatory cytokines, modulate T-cell differentiation, reduce antigen presentation via MHC II, and importantly correct a range of pathological conditions of RA.
ω-3 PUFAs and Systemic Lupus Erythematosus
In addition to the investigations of ω-3 PUFAs in the treatment for RA, there have also been a number of studies in animal models and clinical trials assessing the effects of dietary supplementation of ω-3 PUFAs in other autoimmune diseases, such as SLE (42, 92). SLE is a common autoimmune disorder with diverse clinical manifestations including inflammation, blood vessel abnormalities, and immune-complex deposition, which are all associated with autoantibodies against cellular components (93, 94). Since the earliest clinical trial in 1989 (Table 2), there have been seven major published clinical studies focusing on the relationship between ω-3 PUFAs and SLE (42–48) (Table 2). All but one of the clinical studies (48) (Table 2) reported beneficial effects, including the improvement in endothelial function, disease activity, or inflammatory markers following the implementation of ω-3 PUFAs in SLE patients. In all of the studies with positive outcomes, more than 12 weeks of intervention appeared to be necessary. In a recent randomized placebo-controlled 6-month trial involving fifty SLE patients (47) (Table 2), the patients who received ω-3 PUFAs intervention (2.25 g EPA and 2.25 g DHA) showed remarkable improvement in their scores derived from Physician Global Assessment (PGA) and RAND 36-Item Short Form Health Survey (RAND SF-36, Version 1.0). The circulating levels of several inflammatory markers, including IL-13 and IL-12, were significantly decreased (47) (Table 2). A clinical nutritional study of SLE patients found that dietary patterns low in ω-3 PUFAs and high in carbohydrates positively correlated with the severity of disease activity, adverse serum lipids, and the presence of plaque (46) (Table 2). A double-blind, double placebo-controlled factorial trial in 52 patients with SLE (45) (Table 2) reported a significant decline in SLAM-R score (revised Systemic Lupus Activity Measure) from 6.12 to 4.69 in the subjects receiving EPA/DHA compared to those on placebo. In the study carried out by Das and colleagues (44) (Table 2), daily oral supplementation of even moderate EPA and DHA (EPA 162 mg, DHA 144 mg) induced prolonged remission of SLE in ten patients. Furthermore, EPA and DHA also suppressed both T-cell proliferation and the production of IL-1, IL-2, as well as TNF-α in vitro and in vivo (44) (Table 2). A comparative clinical study compared fatty acid (FA) compositions in the red blood cells (RBC) and plasma between the female SLE patients and age-matched females with a history of cardiovascular disease (CVD) or those SLE patients with no history of CVD (SLE+CVD and SLE-CVD) (5). The plasma levels of EPA and ω-3 index (EPA+DHA) were significantly lower in the SLE patients than the CVD controls. In RBC, the ratio of AA to EPA was also significantly higher in the SLE patients than in the controls. Thus, although there was no intervention with ω-3 PUFA supplement, these SLE patients clearly had altered plasma and RBC FA compositions favoring inflammation pathology (5). Following this study, Luca Navarini et al. analyzed fatty acids or metabolites in the plasma samples from SLE patients (95). RvD1, the main metabolic product of DHA, was found to be remarkably lower in the samples from SLE patients than those from the non-SLE controls (95), further supporting the potential of using ω-3 PUFAs and its metabolites in the clinical management of SLE.
Mounting evidence built in SLE animal models strongly supports the preventative and therapeutic benefits of ω-3 PUFAs against SLE. Although several SLE models have been widely used in published animal studies, we decided to focus on the results derived from NZB/NZW F1 mice (herein after named B/W F1) because it is a classic spontaneous SLE model with close resemblance to the development of human SLE. Fernandes et al. found that a diet rich in long chain ω-3 fatty acids (10%) delayed the onset, attenuated the severity of autoimmune diseases, and prolonged the survival of female B/W F1 mice (96). Such amelioration of autoimmune disease was mainly attributed to the elevation of transforming growth factor β1 (TGF-β1) and IL-4 as well as the reduction of pro-inflammatory cytokines IL-2 from the splenocytes of B/W F1 mice (96). Perhaps not surprisingly, in their following study, the analysis of fatty acids revealed significantly elevated EPA and DHA levels in the kidney tissue samples of the EPA/DHA-fed animals, and further confirmed the decreased expression of TGF-β1 played a key role in the development of SLE (97). In a separate independent study, the B/W F1 mice, after fed linseed-oil (containing 70% ω-3 PUFAs), showed lower titers of antibodies to DNA as well as cardiolipin and less severe kidney damage than the B/W F1 mice fed control diets (98). Consistent with these findings, Halade et al. found that the concentrated EPA/DHA (Lovaza®) had a dose-dependent therapeutic effect on SLE progression: the diet containing 1% Lovaza extending maximal lifespan to 517 days; and the diet containing 4% Lovaza further prolonged both the median (502 d) and maximal (600 d) life span in B/W F1 mice compared to the same mice fed standard chow diet (301 d) (99). In addition, the diet containing 4% Lovaza significantly decreased anti-dsDNA antibodies, glomerulonephritis, and pro-inflammatory cytokines (IL-1β, IL-6, TNF-α) in the splenocytes. Notably, NF-κB activation and p65 nuclear translocation were also lowered by 4% Lovaza-containing diet when compared to that in the control diet. An independent study by Pestka et al. found that ω-3 PUFAs-enriched diets delayed the onset and markedly attenuated the severity of autoimmune glomerulonephritis, plasma autoantibodies, and proteinuria in the B/W F1 mice (100); importantly, such suppression of autoimmunity was found to correlate with a generalized reduction of CD4+ T cell-specific gene expression in the kidneys and/or spleens of the SLE mice (100). Thus, daily oral supplementation of ω-3 PUFAs has shown promising therapeutic effects, even at low dosages, by suppressing glomerulonephritis and extending life span in patients and SLE-prone animals, most likely via reducing inflammatory cytokines. These studies further demonstrated that ω-3 PUFAs could alleviate the severity and slow the progression of SLE by regulating.
ω-3 PUFAs and Type 1 Diabetes
T1D is a polygenic and organ-specific autoimmune disease, in which a certain subclass of T lymphocytes is involved in executing autoimmune attacks that lead to the destruction of pancreatic β cells (101, 102). Two recent large-scale clinical observational studies revealed clear preventative and therapeutic benefits against the development of T1D through early intervention with EPA/DHA-enriched agents to infants and children. Stene et al. initially reported in a large population-based case-control study that dietary supplement of cod-liver oil and vitamin D in the first year of life significantly reduced the incidence of childhood-onset T1D (49) (Table 3). In a separate longitudinal, observational study (part of the Diabetes Autoimmunity Study in the Young, DAISY), Norris and colleagues showed that EPA/DHA supplement starting from first year of life sharply lowered the incidence of islet autoimmunity and the titers of autoantibodies in children with high risk of T1D (50) (Table 3). In an attempt to treat a childhood onset T1D, Ricordi's group successfully normalized blood glucose and sharply reduced the dose of injected insulin to just once a day after a year and half of oral EPA/DHA and vitamin D supplement in an 8-year-old child (51) (Table 3). A multicenter, two-arm, randomized, double-blind pilot trial of DHA supplementation, beginning either in the last trimester of pregnancy (41 infants) or in the first 5 months after birth (57 infants) with a high genetic risk for T1D was conducted by Dr. Chase's group (52) (Table 3). There were no statistically significant reductions in the blood levels of inflammatory cytokines, such as IL-1β, TNF-α or interleukin-12 subunit p40 (IL-12p40). However, high-sensitivity C-reactive protein (hsCRP) was significantly lower in breast-fed DHA-treated infants compared to all formula-fed infants at age 12 months, suggesting that the nutritional matrix of breast milk, particularly the ones with increased DHA contents, has an anti-inflammatory effect (52) (Table 3).
A series of recent genetic and clinical studies have shed new light on the mechanisms and the therapeutic potential of ω-3 PUFAs in suppressing autoimmunity as well as protecting and enhancing islet functions. In animal studies, Otani et al. reported that low ratio of ω-6/ω-3 at 3.0 in the maternal diet during gestation and lactation delayed the onset of diabetes in NOD mice offspring (103). Their further study indicated that diet with a low ω-6/ω-3 ratio started immediately after the onset of overt diabetes prolonged survival of type 1 diabetes in NOD mice (104). However, a study by a different group failed to see a significant delay in the onset of diabetes with the supplementation of fish-oil (105). The author ascribed these results to the low power of statistical method and the possibility that EPA/DHA may have undergone oxidation after thawing from the −20°C storage condition (105). However, other factors, including the dosage and the concentrations of the administered EPA/DHA, should not be excluded.
The recent research coming from our own group revealed that elevation of cellular ω-3 PUFAs via the transgenic expression of fat-1 almost completely blocked the pancreatic β-cells from the attack of pro-inflammatory cytokines while significantly enhancing insulin secretion (30). These effects were primarily mediated via the reduction of PGE2 secretion from the islets and β-cells, suggesting that the control of inflammation through reduction of ω-6 PUFAs and their metabolites was the main theme in the regulation of β-cell functions (30). These findings derived from the fat-1 transgenic model was also consistent with the results from earlier nutritional studies that dietary gain of marine ω-3 PUFAs could restore palmitate acid-or LA-impaired insulin secretion (106, 107).
In our latest published report using the spontaneous type 1 diabetic NOD mice, an EPA/DHA-enriched diet and a fat-1 gene therapy not only delayed the onset but also reversed the autoimmunity and diabetes (80). Both methods of treatment, provided as either a preventative or a therapeutic modality, significantly suppressed Th1 and Th17 cell populations, reduced the secreted levels of IFN-γ and IL-17, increased the proportion of Th2 and regulatory T cells (Tregs). Thus, EPA/DHA-initiated global changes in CD4+ T-cells are likely the primary cellular mechanisms stalling the development of autoimmunity in T1D (80). Multiple molecular mechanisms may have contributed to the effects of ω-3 PUFAs on CD4+ T-cell differentiation. In particular, the metabolites of PUFAs played critical roles in the modulation of immune system. For example, arachidonic acid (an ω-6 PUFA)-derived eicosanoids, synthesized through the activities of such enzymes as lipoxygenase, cyclooxygenase, and cytochrome P450, often have a pro-inflammatory effect (108). In contrast, EPA/DHA-derived eicosanoids and docosanoids are less inflammatory than those from ω-6 PUFAs (16). In our analysis, different eicosanoids and docosanoids played distinct roles in CD4+ T-cell differentiation (80). Prostaglandin D3 (PGD3) (EPA-derived metabolite) had a strong inhibitory effect on Th1 and Th17 differentiation and increased the percentage of Th2 and Treg.17, 18-dihydroxy-5Z,8Z,11Z,14Z-eicosatetraenoic acid (17,18-DiHETE), another EPA-derived metabolite, only decreased the population of Th17. One of the DHA-derived metabolites, resolvin D1 (RvD1), had a strong inhibitory effect on Th1 differentiation and promoted Th2 and Tregs differentiation. In contrast, the pro-inflammatory eicosanoids (synthesized from AA), such as 15-Hydroxyeicosatetraenoic acid (15-HETE) and 20-Hydroxyeicosatetraenoic acid (20-HETE), had exactly the opposite effects on naive CD4+ T-cell differentiation than those of EPA/DHA-derived metabolites (80).
Olefsky' group has identified a receptor for ω-3 PUFA, which is G-protein coupled receptor 120 (GPR120), that can mediate at least in part the anti-inflammation effect of ω-3 PUFAs (103, 109). GPR120 couples to β-arrestin2 and inhibits TAK1 binding protein 1 (TAB1)-mediated activation of transforming growth factor-β activated kinase 1 (TAK1), providing a mechanism for inhibition of both the NF-κB related Toll-like receptor (TLR) signaling and TNF-α pro-inflammatory signaling (103, 109) (see the proposed model in Figure 1). Deficiency of GPR120 in mice and humans produced symptoms very similar to those of wild-type mice fed LOW ω-3 PUFA diets, including reduced energy expenditure, obesity, insulin resistance, and an elevated state of inflammation (114).
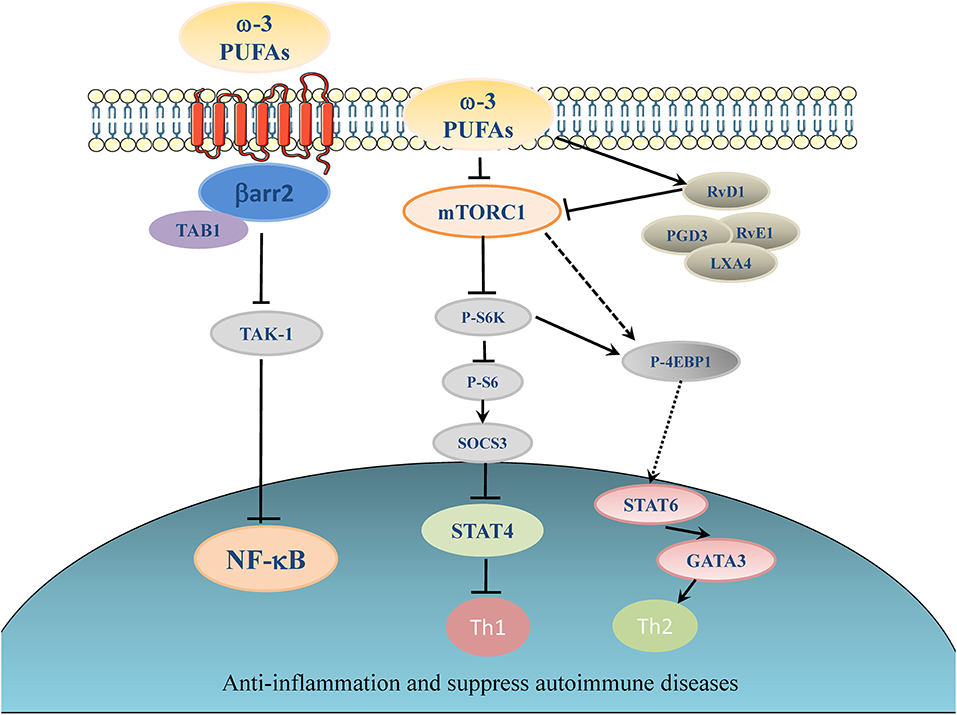
Figure 1. Proposed working model of ω-3 PUFAs induced inhibitory effect on inflammation and autoimmunity. Elevation of ω-3 PUFAs can stimulate one of its major receptors, GPR120. GPR120 couples to β-arrestin2 and inhibit TAB1-mediated activation of TAK1, providing a mechanism for inhibition of NF-κB related pro-inflammatory signaling pathways in autoimmune disease (103, 109). ω-3 PUFAs and their metabolites suppress the activation of Th1 cells through inhibiting of mTORC1 (80, 110, 111), which in turn activates suppressor of cytokine signaling (SOCS) (112). ω-3 PUFAs can inhibit signal transducer and activator of transcription (STAT) 4 which is essential for the development of Th1 cells from naive CD4+ T cells (113). In another signaling pathway, ω-3 PUFAs can dictate the differentiation toward Th2 cells by activating 4E-BP1 through dephosphorylation and then stimulate STAT6 (80).
Our recent studies further revealed that the mTOR has emerged as an important signaling switch for ω-3 PUFA-regulated differentiation of CD4+ T helper cells (110). In particular, application of rapamycin has been shown to inhibit the proliferation of T-cells and the differentiation to Th1 even in the presence of TCR co-stimulatory molecules, such as CD28 (82). T-cell specific mutation of mTOR gene or rapamycin treatment could suppress differentiation to Th17 cell (115). In contrast, rapamycin or diminution of mTOR activity promoted naive CD4+ T cells differentiating into Tregs (115). EPA/DHA and ω-6 PUFAs (particularly AA) had the opposite effects on mTORC1 signaling pathway. EPA/DHA reduced phosphorylation of ribosomal protein S6, indicative of inhibition of mTORC1 activity, whereas AA increased S6 protein phosphorylation levels by activating mTORC1. Moreover, rapamycin, the inhibitor of mTOR, completely blocked the AA-induced Th1 differentiation. Thus, the totally opposite impact of ω-6- and ω-3 PUFAs on the regulation of mTOR complexes serves a molecular switch to dictate the differentiation fate of CD4+ T cells following the treatment of PUFAs (80) (for a proposed working model, see Figure 1).
Interestingly, even in a chemically (Streptozotocin, STZ) induced diabetic model, the pancreatic enrichment of ω-3 PUFAs as a result of fat-1 expression could also prevent the development of diabetes (116). This dramatic effect was accompanied by the reduction of the expression of proinflammatory cytokine genes, the blockade of NF-κB activation, and a sharp suppression of proinflammatory PUFA-derived lipid mediators in the pancreas of the fat-1 mice (116). In contrast, the EPA-derived anti-inflammatory metabolites, lipoxin A4 (LXA4), and 18-hydroxy-5Z,8Z,11Z,14Z,16E-eicosapentaenoic acid (18-HEPE), were highly elevated in the STZ-treated fat-1 mice (116). Taken together, these clinical and animal studies have provided the hope that intervention with ω-3 PUFAs can be successfully applied to the prevention, and even therapeutic treatment of T1D. Additional studies are required to validate the effect of administration of ω-3 fatty acids on the progression of T1D.
Taken together, the reduction of ω-6 PUFAs and elevation of ω-3 PUFA intake are critical to the prevention and treatment of type 1 diabetes, which should help direct the future clinical intervention with EPA/DHA supplementation.
ω-3 PUFAs and Multiple Sclerosis
MS is a chronic autoimmune inflammatory disease of the brain and spinal cord in which immune cell infiltration promotes inflammation, demyelination, and neuroaxonal degeneration, leading to the damage of neuronal signaling (117, 118). At the initial stages of the disease, most patients experience reversible neurological dysfunction, which typically lasts several days or weeks. Over time, irreversible clinical, and cognitive deficits develop (119, 120).
ω-3 PUFAs have attracted a great deal of attention as the potential agents in the clinical management of MS owing to their anti-inflammatory, antithrombotic, antioxidant, and immunomodulatory functions (118, 121–123). Indeed, largely inspired by Swank's observation that a higher incidence of MS occurred in Norwegians with diets of higher levels of animal fats and dairy products than those with diets of higher amounts of fish, several clinical studies have been conducted with the focus on dietary supplements like vitamin D, polyunsaturated fatty acids or other specific diets (124). A Norwegian intervention study of the patients with newly diagnosed MS reported that a 2-year treatment regimen with 0.9 g/day supplement of long chain marine fatty acids and vitamins showed a significant reduction in the mean annual exacerbation rate and the mean expanded disability status scale (EDSS) as compared to the pre-study values (53) (Table 4). In a randomized, placebo-controlled clinical trial with EDSS score and inflammation as the primary outcomes, a significant improvement in EDSS compared with placebo was observed in the patients taking ω-3 fatty acid plus vitamin D supplements (54) (Table 4). In a multi-center incident case-control study (involving 267 cases and 517 controls) conducted in four regions of Australia, high intake of ω-3 PUFAs was strongly associated with a decreased risk of a first clinical diagnosis of central nervous system (CNS) demyelination (55) (Table 4). In a double-blind and randomized trial for the duration of 1 year, 27 relapsing-remitting MS (RRMS) patients were treated with the same doses of ω-3 PUFAs and olive oil (56) (Table 4). Patients were randomly divided into two groups: the ω-3 group with dietary fat intake restricted to <15% of the daily calorie intake and the olive oil group with dietary fat intake to <30%. The results showed no significant effect on relapse rate or clinical severity (EDSS) scores following the 12-month intervention. However, the patients with relapsing-remitting MS benefited from a low fat diet supplemented with ω-3 PUFAs as the mean change of relapse rate in the ω-3 group: −0.797 relapses/year (P = 0.021) vs. −0.69 (P = 0.044) in the olive oil control group (56) (Table 4). However, not all clinical studies yielded positive outcomes. Torkildsen et al. evaluated the effect of ω-3 PUFAs on MS disease severity in a randomized, double-blind, and placebo-controlled trial (57) (Table 4). They applied 1,350 mg of EPA plus 850 mg of DHA daily in the treatment group with placebo as the control group for 6 months. Magnetic resonance imaging (MRI) was applied to quantify the number of new T1-weighted gadolinium-enhancing lesions during the first 6 months. Relapse rate, disability progression, fatigue, and quality of life were measured after 9 and 24 months. There was no difference between the ω-3 PUFAs and placebo groups during the first 6 months in the number of new gadolinium-enhancing MRI lesions, as well as in disease activity after 6 months or 24 months (57) (Table 4). It is still unclear about the discrepancy of outcomes in different trials although the dosage, the source and concentrations of EPA/DHA agents, the duration of the trial, and the methods of evaluation all could have led to the different results at the end of the trial.
Some of the positive clinical observations mentioned above prompted a series of cellular and animal research studies about the effects of ω-3 PUFAs on MS development. As described above, several different groups (including our own) have reported that ω-3 PUFAs could inhibit the activation of NF-κB via increasing the expression of PPARs (24, 30, 125–128), which in turn reduces the production of inflammatory markers. In a combinatorial treatment with DHA and all-trans-retinoic acid (a bioactive derivative of vitamin A), the expression of IL-17 and RORγt gene, which is vital for the initiation and progression of MS, was significantly attenuated in the PBMCs isolated from the patients with relapsing-remitting multiple sclerosis (129). Addition of EPA and DHA in cultured cells significantly decreased the production and activity of matrix metalloproteinase-9 (MMP-9) and inhibited the migration of human T cell derived from the PBMCs from MS patients, which implied the reduction of infiltrating capability of T-cells (130).
Kong et al. first reported in 2011 that dietary ω-3 fatty acids could suppress EAE in an animal model (73). Their extensive work showed that pretreatment of bone marrow-derived dendritic cells (DC) with DHA could prevent LPS-induced DC maturation and weaken the stimulation of antigen-specific T cells (73). Consistent with these in vitro observations, the results from the animal model further demonstrated decreased numbers of IFNγ- and IL-17-producing CD4+ T cells in both spleen and CNS (73). In another independent study, the mice with myelin oligodendrocyte glycoprotein (MOG)-induced EAE showed a significant delay in onset and amelioration in severity by DHA-enriched diet (131). In such context, the female mice were fed 4 weeks of either the control diet, or the diet containing 0.3 or 1.0% DHA in either phospholipid (PL) form or triacylglycerol (TAG) form pre-EAE induction and for 4 weeks post-EAE induction. The TAG-form DHA appeared to be effective in lower daily scores in the early phase of EAE during day 9–16 while PL-form DHA was effective in decreasing daily scores in the final outcome during day 23–28 (131). Increasing EPA content in the diet appeared to have the benefit similar to that of DHA-enriched diet. Unoda et al. found that EPA-enriched diet significantly lowered clinical EAE scores, and remarkably inhibited the production of IFN-γ and IL-17 (132). Consistent with these findings in the nutritional intervention studies, modeling of MS with cuprizone in the fat-1 mice revealed that endogenous production of ω-3 PUFAs strongly promoted remyelination after a toxic injury to CNS oligodendrocytes via modulation of the immune system (133, 134). Interestingly, some of the protective effects were mediated at least in part through EPA-derived lipid metabolites, such as 18-HEPE by acting directly on oligodendrocytes and neurons (133, 134). Taken together, the findings coming out of the clinical investigations and animal models provided the hope of applying high dose of EPA/DHA agents in the treatment of MS.
Conclusion
The promising findings coming from the cumulative research work over the last decade solidified the role of ω-3 PUFAs as a potential candidate to prevent or even treat such autoimmune diseases as type 1 diabetes, RA, SLE, MS. Undoubtedly, many of the beneficial effects of ω-3 PUFAs can be traced back to their anti-inflammation actions; however, other mechanisms, such as the regulation of mTOR activity may also be in place. Although still facing some technical issues, particularly clinical evaluation of efficacy and safety, the use of high dosages of EPA/DHA or fat-1 gene therapy to generate endogenousω-3 PUFAs have a huge potential in the clinical treatment of these debilitating diseases.
Author Contributions
XB and XL wrote the manuscript. AZ oversaw the editing. XL, SW, ZZ, FL, and AZ revised the manuscript. All authors read and approved the final manuscript.
Funding
This work was supported by grants from the Guangdong Innovative Research Team Program (2016ZT06Y432 to AZ and FL), the National Natural Science Foundation of China (NSFC) (81630021 to AZ; 81372798 to FL; 81700690 to XB), the Special Grants of China Postdoctoral Science Fund (2018T110852 to XB), the Jiangsu Government Scholarship for Overseas Studies (to XL), and the National Key R&D Program of China (2018YFA0800603 to AZ).
Conflict of Interest
The authors declare that the research was conducted in the absence of any commercial or financial relationships that could be construed as a potential conflict of interest.
References
2. Holman RT. The slow discovery of the importance of omega 3 essential fatty acids in human health. J Nutr. (1998) 128 (2 Suppl):427S−33S. doi: 10.1093/jn/128.2.427S
3. Wall R, Ross RP, Fitzgerald GF, Stanton C. Fatty acids from fish: the anti-inflammatory potential of long-chain omega-3 fatty acids. Nutr Rev. (2010) 68:280–9. doi: 10.1111/j.1753-4887.2010.00287.x
4. Kang JX. The importance of omega-6/omega-3 fatty acid ratio in cell function. The gene transfer of omega-3 fatty acid desaturase. World Rev Nutr Diet. (2003) 92:23–36. doi: 10.1159/000073790
5. Aghdassi E, Ma DW, Morrison S, Hillyer LM, Clarke S, Gladman DD, et al. Alterations in circulating fatty acid composition in patients with systemic lupus erythematosus: a pilot study. JPEN J Parenter Enteral Nutr. (2011) 35:198–208. doi: 10.1177/0148607110386378
6. Jump DB. The biochemistry of n-3 polyunsaturated fatty acids. J Biol Chem. (2002) 277:8755–8. doi: 10.1074/jbc.R100062200
8. Calder PC, Yaqoob P. Understanding omega-3 polyunsaturated fatty acids. Postgrad Med. (2009) 121:148–57. doi: 10.3810/pgm.2009.11.2083
9. Abedi E, Sahari MA. Long-chain polyunsaturated fatty acid sources and evaluation of their nutritional and functional properties. Food Sci Nutr. (2014) 2:443–63. doi: 10.1002/fsn3.121
10. Huang YS, Smith RS, Redden PR, Cantrill RC, Horrobin DF. Modification of liver fatty acid metabolism in mice by n-3 and n-6 delta 6-desaturase substrates and products. Biochim Biophys Acta. (1991) 1082:319–27. doi: 10.1016/0005-2760(91)90208-Y
11. Yamazaki K, Fujikawa M, Hamazaki T, Yano S, Shono T. Comparison of the conversion rates of alpha-linolenic acid. (18:3. (n - 3)) and stearidonic acid. (18:4. (n - 3)) to longer polyunsaturated fatty acids in rats. Biochim Biophys Acta. (1992) 1123:18–26. doi: 10.1016/0005-2760(92)90166-S
12. Gregory MK, Gibson RA, Cook-Johnson RJ, Cleland LG, James MJ. Elongase reactions as control points in long-chain polyunsaturated fatty acid synthesis. PLoS ONE. (2011) 6:e29662. doi: 10.1371/journal.pone.0029662
13. Serhan CN, Clish CB, Brannon J, Colgan SP, Chiang N, Gronert K. Novel functional sets of lipid-derived mediators with antiinflammatory actions generated from omega-3 fatty acids via cyclooxygenase 2-nonsteroidal antiinflammatory drugs and transcellular processing. J Exp Med. (2000) 192:1197–204. doi: 10.1084/jem.192.8.1197
14. Serhan CN, Gotlinger K, Hong S, Lu Y, Siegelman J, Baer T, et al. Anti-inflammatory actions of neuroprotectin D1/protectin D1 and its natural stereoisomers: assignments of dihydroxy-containing docosatrienes. J Immunol. (2006) 176:1848–59. doi: 10.4049/jimmunol.176.3.1848
15. Hong S, Gronert K, Devchand PR, Moussignac RL, Serhan CN. Novel docosatrienes and 17S-resolvins generated from docosahexaenoic acid in murine brain, human blood, and glial cells - Autacoids in anti-inflammation. J Biol Chem. (2003) 278:14677–87. doi: 10.1074/jbc.M300218200
16. Schmitz G, Ecker J. The opposing effects of n-3 and n-6 fatty acids. Progress Lipid Res. (2008) 47:147–55. doi: 10.1016/j.plipres.2007.12.004
17. Serhan CN, Petasis NA. Resolvins and protectins in inflammation resolution. Chem Rev. (2011) 111:5922–43. doi: 10.1021/cr100396c
18. Neuhofer A, Zeyda M, Mascher D, Itariu BK, Murano I, Leitner L, et al. Impaired local production of proresolving lipid mediators in obesity and 17-HDHA as a potential treatment for obesity-associated inflammation. Diabetes. (2013) 62:1945–56. doi: 10.2337/db12-0828
19. Watson JE, Kim JS, Das A. Emerging class of omega-3 fatty acid endocannabinoids & their derivatives. Prostaglandins Other Lipid Mediat. (2019) 143:106337. doi: 10.1016/j.prostaglandins.2019.106337
20. Fritsche K. Fatty acids as modulators of the immune response. Annu Rev Nutr. (2006) 26:45–73. doi: 10.1146/annurev.nutr.25.050304.092610
21. Kang JX, Liu A. The role of the tissue omega-6/omega-3 fatty acid ratio in regulating tumor angiogenesis. Cancer Metastasis Rev. (2013) 32:201–10. doi: 10.1007/s10555-012-9401-9
22. Maskrey BH, Megson IL, Rossi AG, Whitfield PD. Emerging importance of omega-3 fatty acids in the innate immune response: molecular mechanisms and lipidomic strategies for their analysis. Molecul Nutr Food Res. (2013) 57:1390–400. doi: 10.1002/mnfr.201200723
23. Terry P, Wolk A, Vainio H, Weiderpass E. Fatty fish consumption lowers the risk of endometrial cancer: a nationwide case-control study in Sweden. Cancer Epidemiol Biomark Preven. (2002) 11:143–5.
24. Li J, Li FR, Wei D, Jia W, Kang JX, Stefanovic-Racic M, et al. Endogenous omega-3 polyunsaturated fatty acid production confers resistance to obesity, dyslipidemia, and diabetes in mice. Mol Endocrinol. (2014) 28:1316–28. doi: 10.1210/me.2014-1011
25. Zhang W, Wang H, Zhang H, Leak RK, Shi Y, Hu X, et al. Dietary supplementation with omega-3 polyunsaturated fatty acids robustly promotes neurovascular restorative dynamics and improves neurological functions after stroke. Exp Neurol. (2015) 272:170–80. doi: 10.1016/j.expneurol.2015.03.005
26. Boston PF, Bennett A, Horrobin DF, Bennett CN. Ethyl-EPA in Alzheimer's disease–a pilot study. Prostag Leukot Essential Fatty Acids. (2004) 71:341–6. doi: 10.1016/j.plefa.2004.07.001
27. Kang JX, Wang J, Wu L, Kang ZB. Transgenic mice: fat-1 mice convert n-6 to n-3 fatty acids. Nature. (2004) 427:504. doi: 10.1038/427504a
28. Kang JX. Fat-1 transgenic mice: a new model for omega-3 research. Prostag Leukotr Ess. (2007) 77:263–7. doi: 10.1016/j.plefa.2007.10.010
29. Hudert CA, Weylandt KH, Lu Y, Wang J, Hong S, Dignass A, et al. Transgenic mice rich in endogenous omega-3 fatty acids are protected from colitis. Proc Natl Acad Sci USA. (2006) 103:11276–81. doi: 10.1073/pnas.0601280103
30. Wei D, Li J, Shen M, Jia W, Chen N, Chen T, et al. Cellular production of n-3 PUFAs and reduction of n-6-to-n-3 ratios in the pancreatic beta-cells and islets enhance insulin secretion and confer protection against cytokine-induced cell death. Diabetes. (2010) 59:471–8. doi: 10.2337/db09-0284
31. Miles EA, Calder PC. Influence of marine n-3 polyunsaturated fatty acids on immune function and a systematic review of their effects on clinical outcomes in rheumatoid arthritis. Br J Nutr. (2012) 107(Suppl 2):S171–84. doi: 10.1017/S0007114512001560
32. de Medeiros MCS, Medeiros JCA, de Medeiros HJ, Leitão JCGdC, Knackfuss MI. Dietary intervention and health in patients with systemic lupus erythematosus: a systematic review of the evidence. Crit Rev Food Sci Nutri. 17:1–8. doi: 10.1080/10408398.2018.1463966
33. Virtanen SM. Dietary factors in the development of type 1 diabetes. Pediatr Diabet. (2016) 17:49–55. doi: 10.1111/pedi.12341
34. Mische LJ, Mowry EM. The evidence for dietary interventions and nutritional supplements as treatment options in multiple sclerosis: a review. Curr Treat Options Neurol. (2018) 20:8. doi: 10.1007/s11940-018-0494-5
35. Kremer JM, Bigauoette J, Michalek AV, Timchalk MA, Lininger L, Rynes RI, et al. Effects of manipulation of dietary fatty acids on clinical manifestations of rheumatoid arthritis. Lancet. (1985) 1:184–7. doi: 10.1016/S0140-6736(85)92024-0
36. Kremer JM, Lawrence DA, Jubiz W, DiGiacomo R, Rynes R, Bartholomew LE, et al. Dietary fish oil and olive oil supplementation in patients with rheumatoid arthritis. Clinical and immunologic effects. Arthr Rheumat. (1990) 33:810–20. doi: 10.1002/art.1780330607
37. Veselinovic M, Vasiljevic D, Vucic V, Arsic A, Petrovic S, Tomic-Lucic A, et al. Clinical benefits of n-3 PUFA and. (sic)-linolenic acid in patients with rheumatoid arthritis. Nutrients. (2017) 9:E325. doi: 10.3390/nu9040325
38. Beyer K, Lie SA, Kjellevold M, Dahl L, Brun JG, Bolstad AI. Marine omega-3, vitamin D levels, disease outcome and periodontal status in rheumatoid arthritis outpatients. Nutrition. (2018) 55–56:116–24. doi: 10.1016/j.nut.2018.03.054
39. van der Tempel H, Tulleken JE, Limburg PC, Muskiet FA, van Rijswijk MH. Effects of fish oil supplementation in rheumatoid arthritis. Ann Rheum Dis. (1990) 49:76–80. doi: 10.1136/ard.49.2.76
40. Lee YH, Bae SC, Song GG. Omega-3 polyunsaturated fatty acids and the treatment of rheumatoid arthritis: a meta-analysis. Arch Med Res. (2012) 43:356–62. doi: 10.1016/j.arcmed.2012.06.011
41. Espersen GT, Grunnet N, Lervang HH, Nielsen GL, Thomsen BS, Faarvang KL, et al. Decreased interleukin-1 beta levels in plasma from rheumatoid arthritis patients after dietary supplementation with n-3 polyunsaturated fatty acids. Clin Rheumatol. (1992) 11:393–5. doi: 10.1007/BF02207200
42. Clark WF, Parbtani A, Huff MW, Reid B, Holub BJ, Falardeau P. Omega-3 fatty acid dietary supplementation in systemic lupus erythematosus. Kidney Int. (1989) 36:653–60. doi: 10.1038/ki.1989.242
43. Westberg G, Tarkowski A. Effect of MaxEPA in patients with SLE. A double-blind, crossover study. Scand J Rheumatol. (1990) 19:137–43. doi: 10.3109/03009749009102117
44. Das UN. Beneficial effect of eicosapentaenoic and docosahexaenoic acids in the management of systemic lupus erythematosus and its relationship to the cytokine network. Prostag Leuk Essential Fatty Acids. (1994) 51:207–13. doi: 10.1016/0952-3278(94)90136-8
45. Duffy EM, Meenagh GK, McMillan SA, Strain JJ, Hannigan BM, Bell AL. The clinical effect of dietary supplementation with omega-3 fish oils and/or copper in systemic lupus erythematosus. J Rheumatol. (2004) 31:1551–6.
46. Elkan AC, Anania C, Gustafsson T, Jogestrand T, Hafstrom I, Frostegard J. Diet and fatty acid pattern among patients with SLE: associations with disease activity, blood lipids and atherosclerosis. Lupus. (2012) 21:1405–11. doi: 10.1177/0961203312458471
47. Arriens C, Hynan LS, Lerman RH, Karp DR, Mohan C. Placebo-controlled randomized clinical trial of fish oil's impact on fatigue, quality of life, and disease activity in Systemic Lupus Erythematosus. Nutr J. (2015) 14:82. doi: 10.1186/s12937-015-0068-2
48. Bello KJ, Fang H, Fazeli P, Bolad W, Corretti M, Magder LS, et al. Omega-3 in SLE: a double-blind, placebo-controlled randomized clinical trial of endothelial dysfunction and disease activity in systemic lupus erythematosus. Rheumatol Int. (2013) 33:2789–96. doi: 10.1007/s00296-013-2811-3
49. Stene LC, Joner G. Use of cod liver oil during the first year of life is associated with lower risk of childhood-onset type 1 diabetes: a large, population-based, case-control study. Am J Clin Nutr. (2003) 78:1128–34. doi: 10.1093/ajcn/78.6.1128
50. Norris JM, Yin X, Lamb MM, Barriga K, Seifert J, Hoffman M, et al. Omega-3 polyunsaturated fatty acid intake and islet autoimmunity in children at increased risk for type 1 diabetes. JAMA. (2007) 298:1420–8. doi: 10.1001/jama.298.12.1420
51. Cadario F, Savastio S, Ricotti R, Rizzo AM, Carrera D, Maiuri L, et al. Administration of vitamin D and high dose of omega 3 to sustain remission of type 1 diabetes. Eur Rev Med Pharmacol Sci. (2018) 22:512–5. doi: 10.26355/eurrev_201801_14203
52. Chase HP, Boulware D, Rodriguez H, Donaldson D, Chritton S, Rafkin-Mervis L, et al. Effect of docosahexaenoic acid supplementation on inflammatory cytokine levels in infants at high genetic risk for type 1 diabetes. Pediatr Diabet. (2015) 16:271–9. doi: 10.1111/pedi.12170
53. Nordvik I, Myhr KM, Nyland H, Bjerve KS. Effect of dietary advice and n-3 supplementation in newly diagnosed MS patients. Acta Neurol Scand. (2000) 102:143–9. doi: 10.1034/j.1600-0404.2000.102003143.x
54. Kouchaki E, Afarini M, Abolhassani J, Mirhosseini N, Bahmani F, Masoud SA, et al. High-dose omega-3 fatty acid plus vitamin D3 supplementation affects clinical symptoms and metabolic status of patients with multiple sclerosis: a randomized controlled clinical trial. J Nutr. (2018) 148:1380–6. doi: 10.1093/jn/nxy116
55. Hoare S, Lithander F, van der Mei I, Ponsonby AL, Lucas R, Grp AI. Higher intake of omega-3 polyunsaturated fatty acids is associated with a decreased risk of a first clinical diagnosis of central nervous system demyelination: results from the ausimmune study. Mult Scler J. (2016) 22:884–92. doi: 10.1177/1352458515604380
56. Weinstock-Guttman B, Baier M, Park Y, Feichter J, Lee-Kwen P, Gallagher E, et al. Low fat dietary intervention with omega-3 fatty acid supplementation in multiple sclerosis patients. Prostag Leuk Essential Fatty Acids. (2005) 73:397–404. doi: 10.1016/j.plefa.2005.05.024
57. Torkildsen O, Wergeland S, Bakke S, Beiske AG, Bjerve KS, Hovdal H, et al. omega-3 fatty acid treatment in multiple sclerosis. (OFAMS Study) a randomized, double-blind, placebo-controlled trial. Arch Neurol-Chicago. (2012) 69:1044–51. doi: 10.1001/archneurol.2012.283
58. Hoxha M. A systematic review on the role of eicosanoid pathways in rheumatoid arthritis. Adv Med Sci. (2018) 63:22–9. doi: 10.1016/j.advms.2017.06.004
59. McInnes IB, Schett G. Cytokines in the pathogenesis of rheumatoid arthritis. Nat Rev Immunol. (2007) 7:429–42. doi: 10.1038/nri2094
60. Tsokos GC. B cells, be gone — b-cell depletion in the treatment of rheumatoid arthritis. New Engl J Med. (2004) 350:2546–8. doi: 10.1056/NEJMp048114
61. Leslie CA, Gonnerman WA, Ullman MD, Hayes KC, Franzblau C, Cathcart ES. Dietary fish oil modulates macrophage fatty acids and decreases arthritis susceptibility in mice. J Exp Med. (1985) 162:1336–49. doi: 10.1084/jem.162.4.1336
62. Ierna M, Kerr A, Scales H, Berge K, Griinari M. Supplementation of diet with krill oil protects against experimental rheumatoid arthritis. BMC Musculoskelet Disord. (2010) 11:136. doi: 10.1186/1471-2474-11-136
63. Volker DH, FitzGerald PE, Garg ML. The eicosapentaenoic to docosahexaenoic acid ratio of diets affects the pathogenesis of arthritis in Lew/SSN rats. J Nutr. (2000) 130:559–65. doi: 10.1093/jn/130.3.559
64. Woo SJ, Lim K, Park SY, Jung MY, Lim HS, Jeon MG, et al. Endogenous conversion of n-6 to n-3 polyunsaturated fatty acids attenuates K/BxN serum-transfer arthritis in fat-1 mice. J Nutr Biochem. (2015) 26:713–20. doi: 10.1016/j.jnutbio.2015.01.011
65. Caughey GE, Mantzioris E, Gibson RA, Cleland LG, James MJ. The effect on human tumor necrosis factor alpha and interleukin 1 beta production of diets enriched in n-3 fatty acids from vegetable oil or fish oil. Am J Clin Nutr. (1996) 63:116–22. doi: 10.1093/ajcn/63.1.116
66. Finnegan YE, Minihane AM, Leigh-Firbank EC, Kew S, Meijer GW, Muggli R, et al. Plant- and marine-derived n-3 polyunsaturated fatty acids have differential effects on fasting and postprandial blood lipid concentrations and on the susceptibility of LDL to oxidative modification in moderately hyperlipidemic subjects. Am J Clin Nutr. (2003) 77:783–95. doi: 10.1093/ajcn/77.4.783
67. Miles EA, Banerjee T, Dooper MM, M'Rabet L, Graus YM, Calder PC. The influence of different combinations of gamma-linolenic acid, stearidonic acid and EPA on immune function in healthy young male subjects. Br J Nutr. (2004) 91:893–903. doi: 10.1079/BJN20041131
68. Kew S, Mesa MD, Tricon S, Buckley R, Minihane AM, Yaqoob P. Effects of oils rich in eicosapentaenoic and docosahexaenoic acids on immune cell composition and function in healthy humans. Am J Clin Nutr. (2004) 79:674–81. doi: 10.1093/ajcn/79.4.674
69. Rosu A, Margaritescu C, Stepan A, Musetescu A, Ene M. IL-17 patterns in synovium, serum and synovial fluid from treatment-naive, early rheumatoid arthritis patients. Rom J Morphol Embryol. (2012) 53:73–80.
70. Moon YM, Yoon BY, Her YM, Oh HJ, Lee JS, Kim KW, et al. IL-32 and IL-17 interact and have the potential to aggravate osteoclastogenesis in rheumatoid arthritis. Arthritis Res Ther. (2012) 14:R246. doi: 10.1186/ar4089
71. Qin S, Wen J, Bai XC, Chen TY, Zheng RC, Zhou GB, et al. Endogenous n-3 polyunsaturated fatty acids protect against imiquimod-induced psoriasis-like inflammation via the IL-17/IL-23 axis. Mol Med Rep. (2014) 9:2097–104. doi: 10.3892/mmr.2014.2136
72. Ye P, Li J, Wang S, Xie A, Sun W, Xia J. Eicosapentaenoic acid disrupts the balance between Tregs and IL-17+ T cells through PPARγ nuclear receptor activation and protects cardiac allografts. J Surg Res. (2012) 173:161–70. doi: 10.1016/j.jss.2010.08.052
73. Kong W, Yen JH, Ganea D. Docosahexaenoic acid prevents dendritic cell maturation, inhibits antigen-specific Th1/Th17 differentiation and suppresses experimental autoimmune encephalomyelitis. Brain Behav Immun. (2011) 25:872–82. doi: 10.1016/j.bbi.2010.09.012
74. Allen MJ, Fan YY, Monk JM, Hou TY, Barhoumi R, McMurray DN, et al. n-3 PUFAs reduce T-helper 17 cell differentiation by decreasing responsiveness to interleukin-6 in isolated mouse splenic CD4. (+) T cells. J Nutr. (2014) 144:1306–13. doi: 10.3945/jn.114.194407
75. Babcock TA, Novak T, Ong E, Jho DH, Helton WS, Espat NJ. Modulation of lipopolysaccharide-stimulated macrophage tumor necrosis factor-alpha production by omega-3 fatty acid is associated with differential cyclooxygenase-2 protein expression and is independent of interleukin-10. J Surg Res. (2002) 107:135–9. doi: 10.1006/jsre.2002.6498
76. Novak TE, Babcock TA, Jho DH, Helton WS, Espat NJ. NF-kappa B inhibition by omega−3 fatty acids modulates LPS-stimulated macrophage TNF-alpha transcription. Am J Physiol Lung Cell Mol Physiol. (2003) 284:L84–9. doi: 10.1152/ajplung.00077.2002
77. Zhao Y, Joshi-Barve S, Barve S, Chen LH. Eicosapentaenoic acid prevents LPS-induced TNF-alpha expression by preventing NF-kappaB activation. J Am Coll Nutr. (2004) 23:71–8. doi: 10.1080/07315724.2004.10719345
78. Trebble T, Arden NK, Stroud MA, Wootton SA, Burdge GC, Miles EA, et al. Inhibition of tumour necrosis factor-α and interleukin 6 production by mononuclear cells following dietary fish-oil supplementation in healthy men and response to antioxidant co-supplementation. Br J Nutr. (2003) 90:405–12. doi: 10.1079/BJN2003892
79. Pompos LJ, Fritsche KL. Antigen-driven murine CD4+ T lymphocyte proliferation and interleukin-2 production are diminished by dietary. (n-3) polyunsaturated fatty acids. J Nutr. (2002) 132:3293–300. doi: 10.1093/jn/132.11.3293
80. Bi X, Li F, Liu S, Jin Y, Zhang X, Yang T, et al. omega-3 polyunsaturated fatty acids ameliorate type 1 diabetes and autoimmunity. J Clin Invest. (2017) 127:1757–71. doi: 10.1172/JCI87388
81. Meydani SN, Endres S, Woods MM, Goldin BR, Soo C, Morrill-Labrode A, et al. Oral. (n-3) fatty acid supplementation suppresses cytokine production and lymphocyte proliferation: comparison between young and older women. J Nutr. (1991) 121:547–55. doi: 10.1093/jn/121.4.547
82. Soyland E, Nenseter MS, Braathen L, Drevon CA. Very long chain n-3 and n-6 polyunsaturated fatty acids inhibit proliferation of human T-lymphocytes in vitro. Eur J Clin Invest. (1993) 23:112–21. doi: 10.1111/j.1365-2362.1993.tb00750.x
83. Terada S, Takizawa M, Yamamoto S, Ezaki O, Itakura H, Akagawa KS. Suppressive mechanisms of EPA on human T cell proliferation. Microbiol Immunol. (2001) 45:473–81. doi: 10.1111/j.1348-0421.2001.tb02647.x
84. Mizota T, Fujita-Kambara C, Matsuya N, Hamasaki S, Fukudome T, Goto H, et al. Effect of dietary fatty acid composition on Th1/Th2 polarization in lymphocytes. JPEN J Parent Enteral Nutr. (2009) 33:390–6. doi: 10.1177/0148607108325252
85. Sierra S, Lara-Villoslada F, Comalada M, Olivares M, Xaus J. Dietary fish oil n-3 fatty acids increase regulatory cytokine production and exert anti-inflammatory effects in two murine models of inflammation. Lipids. (2006) 41:1115–25. doi: 10.1007/s11745-006-5061-2
86. Suzuki D, Furukawa K, Kimura F, Shimizu H, Yoshidome H, Ohtsuka M, et al. Effects of perioperative immunonutrition on cell-mediated immunity, T helper type 1. (Th1)/Th2 differentiation, and Th17 response after pancreaticoduodenectomy. Surgery. (2010) 148:573–81. doi: 10.1016/j.surg.2010.01.017
87. Khair-el-Din TA, Sicher SC, Vazquez MA, Wright WJ, Lu CY. Docosahexaenoic acid, a major constituent of fetal serum and fish oil diets, inhibits IFN gamma-induced Ia-expression by murine macrophages in vitro. J Immunol. (1995) 154:1296–306.
88. Hughes DA, Pinder AC. N-3 polyunsaturated fatty acids modulate the expression of functionally associated molecules on human monocytes and inhibit antigen-presentation in vitro. Clin Exp Immunol. (1997) 110:516–23. doi: 10.1046/j.1365-2249.1997.4351455.x
89. Hughes DA, Pinder AC, Piper Z, Johnson IT, Lund EK. Fish oil supplementation inhibits the expression of major histocompatibility complex class II molecules and adhesion molecules on human monocytes. Am J Clin Nutr. (1996) 63:267–72. doi: 10.1093/ajcn/63.2.267
90. Calder PC. Polyunsaturated fatty acids alter the rules of engagement. Future Lipidol. (2007) 2:27–30. doi: 10.2217/17460875.2.1.27
91. Hughes DA, Pinder AC. n-3 polyunsaturated fatty acids inhibit the antigen-presenting function of human monocytes. Am J Clin Nutr. (2000) 71 (Suppl 1):357S−60S. doi: 10.1093/ajcn/71.1.357s
92. Jolly CA, Muthukumar A, Avula CP, Troyer D, Fernandes G. Life span is prolonged in food-restricted autoimmune-prone. (NZB x NZW)F. (1) mice fed a diet enriched with. (n-3) fatty acids. J Nutr. (2001) 131:2753–60. doi: 10.1093/jn/131.10.2753
93. Yaniv G, Twig G, Shor DB, Furer A, Sherer Y, Mozes O, et al. A volcanic explosion of autoantibodies in systemic lupus erythematosus: a diversity of 180 different antibodies found in SLE patients. Autoimmun Rev. (2015) 14:75–9. doi: 10.1016/j.autrev.2014.10.003
94. Rose T, Dorner T. Drivers of the immunopathogenesis in systemic lupus erythematosus. Best Pract Res Clin Rheumatol. (2017) 31:321–33. doi: 10.1016/j.berh.2017.09.007
95. Navarini L, Bisogno T, Margiotta DPE, Piccoli A, Angeletti S, Laudisio A, et al. Role of the specialized proresolving mediator resolvin D1 in systemic lupus erythematosus: preliminary results. J Immunol Res. (2018). doi: 10.1155/2018/5264195
96. Fernandes G, Bysani C, Venkatraman JT, Tomar V, Zhao W. Increased TGF-beta and decreased oncogene expression by omega-3 fatty acids in the spleen delays onset of autoimmune disease in B/W mice. J Immunol. (1994) 152:5979–87.
97. Chandrasekar B, Troyer DA, Venkatraman JT, Fernandes G. Dietary omega-3 lipids delay the onset and progression of autoimmune lupus nephritis by inhibiting transforming growth factor beta mRNA and protein expression. J Autoimmun. (1995) 8:381–93. doi: 10.1006/jaut.1995.0030
98. Reifen R, Blank M, Afek A, Kopilowiz Y, Sklan D, Gershwin ME, et al. Dietary polyunsaturated fatty acids decrease anti-dsDNA and anti-cardiolipin antibodies production in idiotype induced mouse model of systemic lupus erythematosus. Lupus. (1998) 7:192–7. doi: 10.1191/096120398678919985
99. Halade GV, Williams PJ, Veigas JM, Barnes JL, Fernandes G. Concentrated fish oil. (Lovaza. (R)) extends lifespan and attenuates kidney disease in lupus-prone short-lived. (NZBxNZW)F1 mice. Exp Biol Med. (2013) 238:610–22. doi: 10.1177/1535370213489485
100. Pestka JJ, Vines LL, Bates MA, He K, Langohr I. Comparative effects of n-3, n-6 and n-9 unsaturated fatty acid-rich diet consumption on lupus nephritis, autoantibody production and CD4+ T cell-related gene responses in the autoimmune NZBWF1 mouse. PLoS ONE. (2014) 9:e100255. doi: 10.1371/journal.pone.0100255
101. Wallberg M, Cooke A. Immune mechanisms in type 1 diabetes. Trends Immunol. (2013) 34:583–91. doi: 10.1016/j.it.2013.08.005
102. Lehuen A, Diana J, Zaccone P, Cooke A. Immune cell crosstalk in type 1 diabetes. Nat Rev Immunol. (2010) 10:501–13. doi: 10.1038/nri2787
103. Kagohashi Y, Abiru N, Kobayashi M, Hashimoto M, Shido O, Otani H. Maternal dietary n-6/n-3 fatty acid ratio affects type 1 diabetes development in the offspring of non-obese diabetic mice. Congen Anom. (2010) 50:212–20. doi: 10.1111/j.1741-4520.2010.00296.x
104. Kagohashi Y, Otani H. Diet with a low n-6/n-3 essential fatty acid ratio when started immediately after the onset of overt diabetes prolongs survival of type 1 diabetes model NOD mice. Congen Anom. (2010) 50:226–31. doi: 10.1111/j.1741-4520.2010.00289.x
105. Schmid S, Koczwara K, Schwinghammer S, Lampasona V, Ziegler AG, Bonifacio E. Delayed exposure to wheat and barley proteins reduces diabetes incidence in non-obese diabetic mice. Clin Immunol. (2004) 111:108–18. doi: 10.1016/j.clim.2003.09.012
106. Winzell MS, Pacini G, Ahren B. Insulin secretion after dietary supplementation with conjugated linoleic acids and n-3 polyunsaturated fatty acids in normal and insulin-resistant mice. Am J Physiol Endocrinol Metabol. (2006) 290:E347–54. doi: 10.1152/ajpendo.00163.2005
107. Kato T, Shimano H, Yamamoto T, Ishikawa M, Kumadaki S, Matsuzaka T, et al. Palmitate impairs and eicosapentaenoate restores insulin secretion through regulation of SREBP-1c in pancreatic islets. Diabetes. (2008) 57:2382–92. doi: 10.2337/db06-1806
108. Patterson E, Wall R, Fitzgerald GF, Ross RP, Stanton C. Health implications of high dietary omega-6 polyunsaturated fatty acids. J Nutr Metabol. (2012) 2012:539426. doi: 10.1155/2012/539426
109. Oh da Y, Walenta E, Akiyama TE, Lagakos WS, Lackey D, Pessentheiner AR, et al. A Gpr120-selective agonist improves insulin resistance and chronic inflammation in obese mice. Nat Med. (2014) 20:942–7. doi: 10.1038/nm.3614
110. Chi H. Regulation and function of mTOR signalling in T cell fate decisions. Nat Rev Immunol. (2012) 12:325–38. doi: 10.1038/nri3198
111. Delgoffe GM, Pollizzi KN, Waickman AT, Heikamp E, Meyers DJ, Horton MR, et al. The kinase mTOR regulates the differentiation of helper T cells through the selective activation of signaling by mTORC1 and mTORC2. Nat Immunol. (2011) 12:295–303. doi: 10.1038/ni.2005
113. Powell JD, Pollizzi KN, Heikamp EB, Horton MR. Regulation of immune responses by mTOR. Ann Rev Immunol. (2012) 30:39–68. doi: 10.1146/annurev-immunol-020711-075024
114. Ichimura A, Hirasawa A, Poulain-Godefroy O, Bonnefond A, Hara T, Yengo L, et al. Dysfunction of lipid sensor GPR120 leads to obesity in both mouse and human. Nature. (2012) 483:350–4. doi: 10.1038/nature10798
115. Delgoffe GM, Kole TP, Zheng Y, Zarek PE, Matthews KL, Xiao B, et al. The mTOR kinase differentially regulates effector and regulatory T cell lineage commitment. Immunity. (2009) 30:832–44. doi: 10.1016/j.immuni.2009.04.014
116. Bellenger J, Bellenger S, Bataille A, Massey KA, Nicolaou A, Rialland M, et al. High pancreatic n-3 fatty acids prevent STZ-induced diabetes in fat-1 mice: inflammatory pathway inhibition. Diabetes. (2011) 60:1090–9. doi: 10.2337/db10-0901
117. Frischer JM, Bramow S, Dal-Bianco A, Lucchinetti CF, Rauschka H, Schmidbauer M, et al. The relation between inflammation and neurodegeneration in multiple sclerosis brains. Brain. (2009) 132(Pt 5):1175–89. doi: 10.1093/brain/awp070
118. Harbige LS, Sharief MK. Polyunsaturated fatty acids in the pathogenesis and treatment of multiple sclerosis. Br J Nutr. (2007) 98(Suppl 1):S46–53. doi: 10.1017/S0007114507833010
119. Lublin FD, Reingold SC, Cohen JA, Cutter GR, Sørensen PS, Thompson AJ, et al. Defining the clinical course of multiple sclerosis. Neurology. (2014) 83:278–86. doi: 10.1212/WNL.0000000000000560
120. Dendrou CA, Fugger L, Friese MA. Immunopathology of multiple sclerosis. Nat Rev Immunol. (2015) 15:545–58. doi: 10.1038/nri3871
121. Riccio P. The molecular basis of nutritional intervention in multiple sclerosis: a narrative review. Complement Ther Med. (2011) 19:228–37. doi: 10.1016/j.ctim.2011.06.006
122. Farooqui AA, Horrocks LA, Farooqui T. Modulation of inflammation in brain: a matter of fat. J Neurochem. (2007) 101:577–99. doi: 10.1111/j.1471-4159.2006.04371.x
123. Calder PC. n-3 polyunsaturated fatty acids, inflammation, and inflammatory diseases. Am J Clin Nutr. (2006) 83(Suppl 6):1505S−19S. doi: 10.1093/ajcn/83.6.1505S
124. Roy LSwank OL. Multiple sclerosis in rural norway — its geographic and occupational incidence in relation to nutrition. N Engl J Med. (1952) 246:721–8. doi: 10.1056/NEJM195205082461901
125. Rossi A, Kapahi P, Natoli G, Takahashi T, Chen Y, Karin M, et al. Anti-inflammatory cyclopentenone prostaglandins are direct inhibitors of I kappa B kinase. Nature. (2000) 403:103–8. doi: 10.1038/47520
126. Li H, Ruan XZ, Moorhead JF, Powis SH, Fernando R, Wheeler DC, et al. omega 3 Polyunsaturated fatty acids. (PUFA) inhibit NF-kappa B activity in LPS-Stimulated proximal tubular cells by PPAR γ activation. J Am Soc Nephrol. (2003) 14:85a–a.
127. Derecka K, Sheldrick EL, Wathes DC, Abayasekara DRE, Flint APF. A PPAR-independent pathway to PUFA-induced COX-2 expression. Mol Cell Endocrinol. (2008) 287:65–71. doi: 10.1016/j.mce.2008.02.015
128. Zuniga J, Cancino M, Medina F, Varela P, Vargas R, Tapia G, et al. N-3 PUFA supplementation triggers PPAR-α activation and PPAR-α/NF-κ B interaction: anti-inflammatory implications in liver ischemia-reperfusion injury. PLoS ONE. (2011) 6:e28502. doi: 10.1371/journal.pone.0028502
129. Mousavi Nasl-Khameneh A, Mirshafiey A, Naser Moghadasi A, Chahardoli R, Mahmoudi M, Parastouei K, et al. Combination treatment of docosahexaenoic acid. (DHA) and all-trans-retinoic acid. (ATRA) inhibit IL-17 and RORγt gene expression in PBMCs of patients with relapsing-remitting multiple sclerosis. Neurol Res. (2018) 40:11–7. doi: 10.1080/01616412.2017.1382800
130. Shinto L, Marracci G, Mohr DC, Bumgarner L, Murchison C, Senders A, et al. Omega-3 fatty acids for depression in multiple sclerosis: a randomized pilot study. PLoS ONE. (2016) 11:e0147195. doi: 10.1371/journal.pone.0147195
131. Adkins Y, Soulika AM, Mackey B, Kelley DS. Docosahexaenoic acid. (22:6n-3) ameliorated the onset and severity of experimental autoimmune encephalomyelitis in mice. Lipids. (2019) 54:13–23. doi: 10.1002/lipd.12130
132. Unoda K, Doi Y, Nakajima H, Yamane K, Hosokawa T, Ishida S, et al. Eicosapentaenoic acid. (EPA) induces peroxisome proliferator-activated receptors and ameliorates experimental autoimmune encephalomyelitis. J Neuroimmunol. (2013) 256:7–12. doi: 10.1016/j.jneuroim.2012.12.003
133. Siegert E, Paul F, Rothe M, Weylandt KH. The effect of omega-3 fatty acids on central nervous system remyelination in fat-1 mice. BMC Neurosci. (2017) 18:19. doi: 10.1186/s12868-016-0312-5
Keywords: ω-3 polyunsaturated fatty acids, autoimmune diseases, inflammation, eicosanoids, mTOR-the mammalian target of rapamycin
Citation: Li X, Bi X, Wang S, Zhang Z, Li F and Zhao AZ (2019) Therapeutic Potential of ω-3 Polyunsaturated Fatty Acids in Human Autoimmune Diseases. Front. Immunol. 10:2241. doi: 10.3389/fimmu.2019.02241
Received: 26 April 2019; Accepted: 04 September 2019;
Published: 27 September 2019.
Edited by:
Allen Jay Rosenspire, Wayne State University, United StatesReviewed by:
Philip Calder, University of Southampton, United KingdomMelissa Bates, Michigan Medicine, University of Michigan, United States
Copyright © 2019 Li, Bi, Wang, Zhang, Li and Zhao. This is an open-access article distributed under the terms of the Creative Commons Attribution License (CC BY). The use, distribution or reproduction in other forums is permitted, provided the original author(s) and the copyright owner(s) are credited and that the original publication in this journal is cited, in accordance with accepted academic practice. No use, distribution or reproduction is permitted which does not comply with these terms.
*Correspondence: Fanghong Li, ZmxpJiN4MDAwNDA7Z2R1dC5lZHUuY24=; Allan Z. Zhao, YXp6aGFvJiN4MDAwNDA7Z2R1dC5lZHUuY24=
†These authors have contributed equally to this work