- Division of Periodontology, Department of Developmental and Surgical Sciences, School of Dentistry, University of Minnesota, Minneapolis, MN, United States
Mouse models that combine specific loxP-flanked gene sequences with Cre recombinase expressed from cell-regulated promoters have become important tools to investigate gene function. Critically however, expression of Cre recombinase may not always be restricted to the target cell or tissue of interest due to promiscuous activity of the driving promoter. Expression of Cre recombinase and, by extension, excision of the loxP-flanked gene may occur in non-target cells and may not be readily apparent. Here we report on the fidelity of Cre recombinase expressed from the il17a or Foxp3 promoters by combining them with a constitutively expressed floxed-stopped tdTomato reporter gene. Foxp3-driven Cre recombinase in F1 mice induced tdTomato red fluorescent protein in Treg cells but also in a range of other immune cells. Frequency of tdTomato expression was variable but positively correlated (p < 0.0001) amongst lymphoid (B cells and CD8 T cells) and blood-resident myeloid cells (dendritic cells, monocytes, neutrophils) suggesting stochastic activity of the Foxp3 promoter rather than developmental regulation in common ancestral progenitors. Interestingly, frequency of tdTomato+ dendritic cells, monocytes and neutrophils did not correlate with the tdTomato+ fraction in eosinophils, indicating that activity of the Foxp3 promoter in eosinophils occurred after the split from a common multipotent progenitor. When these F1 mice were crossed to achieve homozygosity of the promoter and reporter gene, a novel visually red phenotype was observed segregating amongst littermates. The red coloration was widespread and prevalent in non-immune tissues. Thymocytes examined from these red mice showed that all four subsets of immature thymocytes (CD4− CD8−) based on differential expression of CD25 and CD44 were expressing tdTomato. Finally, we show evidence of Foxp3 Cre recombinase independent tdTomato expression, suggesting germ line transmission of an activated tdTomato reporter gene. Our data highlights potential issues with conclusions drawn from using specifically the B6.129(Cg)-Foxp3tm4(YFP/Cre)Ayr/J mice.
Introduction
The Cre-loxP system is a powerful genetic tool that allows for precise in vivo excision of DNA that is flanked by direct repeats of the loxP sequence through action of the Type 1 topoisomerase, Cre recombinase (1–4). The Cre-loxP system is particularly suited to mice and has been widely applied to targeting gene knockouts to specific tissues and cell types. Deletion of engineered loxP-flanked (floxed) gene sequences can be restricted to specific cells of interest through the use of mouse strains that express Cre recombinase from promoters only active in the target cell type. With the development of mice lines that carry constitutively expressed floxed-stopped reporter genes came the opportunity to characterize promoter activity in diverse tissue types and to allow specific immune cells to be tracked and temporal phenotypic changes to be monitored (5–8).
We are interested in examining plasticity in Th17 and Treg cells in the context of a mouse model of periodontitis, an alveolar bone destructive inflammatory disease. The model tests how chronic, long-term exposure to a pathogen associated with periodontitis in humans, reprograms cytokine production in Th17 cells. Plasticity of Th17 cells is well-documented (6, 9–11), and a late developmental switch to IFN-γ expression in Th17 cells has been implicated in the pathologies of a number of inflammatory autoimmune diseases (12–15). Similarly, Treg cells can also switch to IFN-γ-producing Th1-like cells (16) and even IL-17A-producing Th17-like cells (17) under certain inflammatory conditions [reviewed in (18, 19)]. Mouse reporter strains to track temporal changes in cytokine expression in Th17 and Treg cells were generated by crossing a well-characterized transgenic mouse that carries a constitutively expressed floxed-stopped tdTomato reporter gene (5) to mice expressing Cre recombinase under the control of either the il17a (6) or Foxp3 promoters (20), respectively. The mouse line expressing Cre recombinase from the il17a promoter has been used to investigate the requirement for transcription factors Tbet and RORγt in Th17 immunopathologies (21), factors required for Th17 differentiation (22, 23) and plasticity in a number of different disease contexts (7, 24, 25). The Foxp3 Cre recombinase mouse strain has been used to examine the role of IL-10 and IL-27R in Treg function (20, 26). It has also been used to examine the role of signaling molecules STAT3 and TRAF6, and transcriptional factor and regulator Tbet and Blimp1, respectively, in differentiation or plasticity in Treg cells (27–30).
A detailed analysis of mice strains engineered to express Cre recombinase from different promoters active in differentiated myeloid derived immune cells has revealed instances of promiscuous and unexpected promoter activity (31). Here, we present evidence that expands on an earlier report of promiscuous Foxp3 promoter activity (32). By breeding the Foxp3 promoter Cre recombinase mouse line to the floxed-stopped tdTomato reporter strain we not only found tdTomato expressed in target Treg cells, but also in other immune cells as well as non-immune cells. Our data underscores the potentially problematic interpretation of results obtained from in-vivo mouse models that exploit the Foxp3 promoter-Cre recombinase murine line. This problem may be exacerbated particularly when the in-vivo model is used to specifically to target abrogation of genes in Foxp3+ Treg cells.
Materials and Methods
Mouse Strains and Genotyping
All mouse experiments were reviewed and approved by the Institutional Animal Care and Use Committee of the University of Minnesota and performed on sex and age-matched (6–8 week) mice unless specified otherwise. The following mice strains, Il17atm1.1(iCre)Stck/J (6), B6.129(Cg)-Foxp3tm4(YFP/icre)Ayr/J (20), Foxp3tm9(EGFP/cre/ERT2)Ayr/J (33), and B6.Cg-Gt(ROSA)26Sortm14(CAG−tdTomato)Hze/J (5) were purchased from Jackson Laboratory (Bar Harbor, ME). F1 reporter mouse strain IL17aCre+/− tdTomato+/− was generated by crossing Il17atm1.1(iCre)Stck/J to B6.Cg-Gt(ROSA)26Sortm14(CAG−tdTomato)Hze/J mice. F1 reporter mouse strain Foxp3YFP/Cre+/− tdTomato+/− was generated by crossing B6.129(Cg)-Foxp3tm4(YFP/icre)Ayr/J to B6.Cg-Gt(ROSA)26Sortm14(CAG−tdTomato)Hze/J mice. In F1 reporter mice when either the il17a or the Foxp3 promoter is active, cells, and all subsequent daughter cells will permanently express tdTomato through the action of Cre recombinase. When required F1 mice were crossed and genotypes of F2 and derived F3 mice were determined using DNA obtained from tail snips and PCR protocols tailored to the specific transgene (Jackson Laboratory). To generate a tamoxifen-inducible Cre recombinase mouse we crossed Foxp3tm9 (EGFP/cre/ERT2)Ayr/J to B6.Cg-Gt(ROSA)26Sortm14(CAG−tdTomato)Hze/J. Experimental mice were either Foxp3GFP/Cre/ERT2+/+ tdTomato+/+ females or Foxp3GFP/Cre/ERT2+ tdTomato+/+ hemizygous males. All mice were housed in microisolator cages under specific-pathogen free conditions with food and water ad libitum in compliance with the Association for Assessment and Accreditation of Laboratory Animal Care at the University of Minnesota.
Mating and Administration of Tamoxifen in Foxp3GFP/Cre/ERT2+/+ tdTomato+/+ Females
Sibling 8-week-old female Foxp3GFP/Cre/ERT2+/+ tdTomato+/+ mice were placed in a cage with one Foxp3GFP/Cre/ERT2+ tdTomato+/+ hemizygous male overnight. The following morning females were checked for the presence of a copulation plug. Presence of a copulation plug was used to indicate embryonic day 0 (E0) of gestation. Successful implantation was verified by monitoring female weight gain. In this strain of female mouse the activation of the Foxp3 promoter drives the expression of Cre recombinase/Estrogen Receptor 2 (ERT2) fusion protein. Binding of estrogen or estrogen analogs like Tamoxifen to ERT2 lets the Cre/ERT2 complex into the nucleus allowing the endonuclease Cre to excise the flox-stop-flox upstream to the tdTomato which in turns is driven by the very strong ROSA-26 promoter. On E12 and E13 of gestation, 8 mg of Tamoxifen (Sigma-Aldrich, Milwaukee, WI) suspended in 200 μl olive oil (Ward's Science, Rochester, NY) was delivered via oral gavage into the stomach as previously described (33). In these animals, removal of the floxed stop codon and expression of tdTomato red fluorescent protein is controlled temporally by administration of tamoxifen. Tamoxifen is capable of crossing the placental barrier and has been utilized to induce fetal expression of reporter genes (34, 35). However, tamoxifen treatment during pregnancy has been shown to interfere with normal labor and delivery, necessitating the use of fetal mice in this study (35).
Harvest of Fetal Mouse Tissues
Female mice were euthanized on E20 with CO2 followed by cervical dislocation. Afterwards females were disinfected externally with 70% ethanol prior to opening the abdominal cavity. Each uterine chain was rinsed three times with sterile PBS to remove maternal blood contamination prior to separation of fetoplacental units. Each fetoplacental unit was dissected into individual wells of a 12-well plate. After opening of the amniotic sac (fetal membranes), placentas were immediately separated from fetuses and fetuses were rinsed in PBS again to remove any traces of maternal blood contamination as previously described (36). All fetuses were decapitated prior to tissue collection to ensure humane euthanasia. Lungs and spleen were collected from each fetal unit and processed separately.
Analysis of Cells From Cervical Lymph Nodes and Fetal Lung and Spleen by Flow Cytometry
Single-cell suspensions were isolated from cervical lymph nodes (CLN) and fetal spleens by standard techniques (37). Cells were stained with viability dye Zombie Aqua (BioLegend, San Diego, CA) and Fc receptors blocked using anti CD16/CD32 antibody (eBioscience; clone 93). Cells were next stained with anti-mouse CD3 (BioLegend; clone 17A2), CD4 (eBioscience; clone RM4-5), CD8 (eBioscience; clone 53-6.7) and B220 (eBioscience; clone RA3-6B2) fluorochrome-conjugated mAbs to identify B cells, CD4 T cells and CD8 T cells. Cell phenotypes were sorted on an LSR II flow cytometer (BD Biosciences, San Jose, CA) and fluorescence emissions analyzed with FlowJo software (Tree Star, Ashland, OR). For analysis of lung cells, lungs were minced, treated with 2 mg/ml collagenase D and 1 mg/ml DNAse for 30 min at 37°C followed by a further 10 min with 5 nM EDTA to aid cell dissociation. Minced lung fragments were mashed over nylex membrane, and washed and filtered to produce a single cell suspension. The entire cell suspension was stained with Zombie Aqua, Fc receptors blocked using anti CD16/CD32 antibody then stained with anti-mouse CD45 (BioLegend; clone 30-F11), CD31 (BioLegend; clone 390) and CD326 (BioLegend; clone G8.8) fluorochrome-conjugated mAbs to identify epithelial (CD45−, CD326+, CD31−), endothelial (CD45−, CD326−, CD31+) and immune cells (CD45+, CD326−, CD31−). Stained cells were analyzed as above.
Identification of Immune Cells in Blood by Flow Cytometry
Blood samples from Foxp3YFP/Cre+/− tdTomato+/− and IL17aCre+/− tdTomato+/− reporter mice were prepared for flow cytometry as previously described (38). Zombie Aqua stained, FC receptor blocked blood cells were stained with anti-mouse CD11b (clone M1/70), F4/80 (clone BM8), Ly-6G (clone 1A8), B220 (clone RA3-6B2), and I-A/I-E (clone M5/114.15.2) fluorochrome conjugated mAbs from BioLegend. Cells were acquired on an LSR II flow cytometer (BD Biosciences, San Jose, CA) and fluorescence emissions analyzed with FlowJo software (Tree Star, Ashland, OR).
Phenotypic Analysis of Thymocytes
Thymi were dissected from 4-week-old mice and single-cell suspensions stained with Zombie Aqua and FC receptors blocked as described earlier. Thymocytes were stained with anti-mouse CD3, CD4, CD8, CD44 (BioLegend; clone IM7), and CD25 (BioLegend; clone 3C7) flurochrome-conjugated mAbs. Cell phenotypes were sorted on an LSR II flow cytometer and mature and immature thymocyte subsets identified using FlowJo software.
Confocal Microscopy
Cervical lymph nodes (CLN) were embedded in O.C.T medium (Sakura Finetek, Torrance, CA) and 10 μm frozen cryostat sections cut. Acetone fixed sections were re-hydrated with PBS, blocked with 5% (v/v) rat serum in PBS, and incubated for 30 min at room temperature with 10 μg/mL anti-mouse B220-Alexa Fluor 488 (eBioscience; clone RA3-6B2) mAb in rat serum supplemented PBS. After serial washes with PBS-Tween20 and PBS, DAPI (Life Technologies, Carlsbad, CA) stained sections were imaged at 40X using a LSM 700 Zeiss confocal laser scanning microscope and specific signals quantified using ZEN software (Carl Zeiss Microscopy GmbH, Jena, Germany).
Statistical Analysis
Data was analyzed and plotted using Prism 7 software (GraphPad Software, San Diego, CA) and displayed as individual data points with means ± SEM. Correlation was determined from computed Pearson correlation coefficients.
Results
Cre Expression in Foxp3YFP/Cre+/− tdTomato+/− Mice Results in tdTomato Activation in B Cells and CD8 T Cells
Flow cytometry was used to test the fidelity and robustness of tdTomato expression in cells obtained from the CLN of 6–8 week-old F1 female IL17aCre+/− tdTomato+/− (Figure 1A) or Foxp3YFP/Cre+/− tdTomato+/− mice (Figure 1B). To our surprise we found expression of tdTomato in a small but variable fraction of B cells and CD8 T cells in 10 out of the 11 Foxp3YFP/Cre+/− tdTomato+/− mice we analyzed (Figures 1Bd,e, respectively). In comparison, expression of tdTomato in B cells and CD8 T cells was effectively zero in all the IL17aCre+/− tdTomato+/− mice we tested (Figures 1Aa,b, respectively). The percentage of tdTomato expressing CD4 T cells, presumably memory Th17 cells recirculating in CLN of IL17aCre+/− tdTomato+/− mice (Figure 1C). This Th17 finding is consistent with what observed when staining CD4 T cells for IL-17A in CLN of wild-type mice at homeostasis (37). In contrast, the percentage of tdTomato+ CD4 T cells we found in Foxp3YFP/Cre+/− tdTomato+/− mice was higher than expected (Figure 1D) based on our observations of Foxp3 expression in CD4 T cells isolated from CLN of similarly aged specific-pathogen free mice (37).
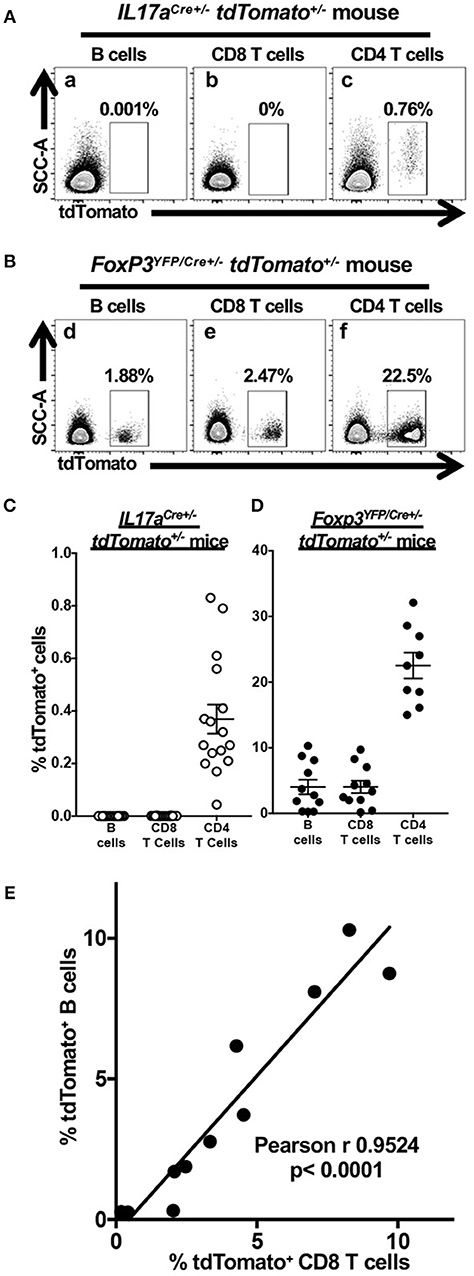
Figure 1. Cre expression in Foxp3YFP/Cre+/− tdTomato+/− mice results in tdTomato accumulation in non-target lymphoid cells. Single cell suspensions obtained from CLN of 6–8 week-old IL17aCre+/− tdTomato+/− or Foxp3YFP/Cre+/− tdTomato+/− female mice were stained with Zombie Aqua and incubated with anti-mouse CD3, CD4, CD8, and B220 mAbs to identify live B cells and CD4 and CD8 T cells by flow cytometry. Representative flow cytometry dot plots gated on live (Zombie Aqualow) B cells, CD4 T cells or CD8 T cells showing expression of tdTomato in (A) IL17aCre+/− tdTomato+/− or (B) Foxp3YFP/Cre+/− tdTomato+/− female mice. The percentage of cells falling within a given tdTomato+ gate is given. Summary data of tdTomato expression in three lymphoid populations from (C) IL17aCre+/− tdTomato+/− mice or (D) Foxp3YFP/Cre+/− tdTomato+/−. Means and SEMs are plotted along with individual data points. (E) The percentage of CD8 T cells expressing tdTomato (x axis) is positively correlated to tdTomato-expressing B cells (y axis) only in Foxp3YFP/Cre+/− tdTomato+/− mice. Pearson's correlation test was used to detect the relationship between the two cell frequencies. The regression line is plotted. N = 11 mice.
Unexpected expression of tdTomato in B cells and CD8 T cells in Foxp3YFP/Cre+/− tdTomato+/− mice prompted us to examine whether the Foxp3 promoter is independently active in these two lymphoid cell types or it became active in an ancestral lineage progenitor. We found that the percentage of tdTomato+ CD8 T cells and B cells is positively correlated in individual Foxp3YFP/Cre+/− tdTomato+/− mice (Figure 1E, p < 0.0001). A positive correlation suggests that a single event is responsible for the activation of tdTomato expression in these cells and that this event occurred in some, but not all, of the multipotent progenitor cells shared by the B cell and CD8 T cell lineage. Variation in the percentage of cells affected indicates that these events are stochastic in nature rather than developmentally programed.
tdTomato Expression in Myeloid Cells Isolated From Blood of Foxp3YFP/Cre+/− tdTomato+/− Mice Indicates Ancestral Foxp3 Promoter Activity
The observation that the tdTomato reporter gene appeared to be activated in multipotent progenitor cells leading to the B and T cell lineages in Foxp3YFP/Cre+/− tdTomato+/− mice led us to question if tdTomato expression is also activated in myeloid immune cells. Here we chose to sample blood. We consistently found expression of tdTomato in subpopulations of neutrophils, dendritic cells, eosinophils and monocytes obtained from Foxp3YFP/Cre+/− tdTomato+/− mice (Figure 2A), in addition to B cells as expected from our results above. These cells are all non-target cell types. Myeloid cell types in IL17aCre+/− tdTomato+/− mice did not express tdTomato, although we did observe significant expression of tdTomato in γδ T cells (data not shown), consistent with reported robust expression of IL-17A in these cells (24, 25).
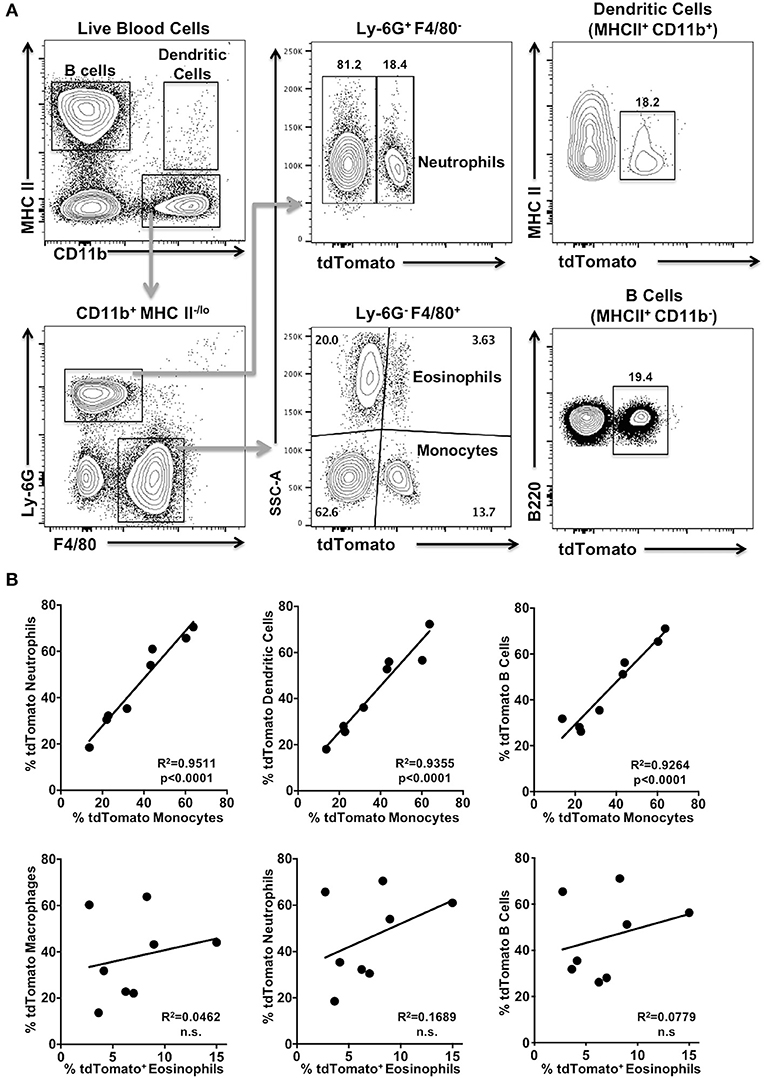
Figure 2. tdTomato is expressed in myeloid cells isolated from blood of Foxp3YFP/Cre+/− tdTomato+/− mice. Blood from 6 to 8 week old Foxp3YFP/Cre−/+ tdTomato+/− female mice was harvested and red blood cells lysed prior to analysis. (A) Cells were stained with Zombie Aqua and rat anti-mouse B220, I-A/I-E, CD11b, Ly-6G, and F4/80 mAbs to identify live B cells, monocytes, neutrophils, dendritic cells, and eosinophils by flow cytometry. Representative flow cytometry plots of live cells obtained from a blood sample are shown. Numbers are indicative of the percentage of cells in a given gated population. (B) Pearson's correlation test was used to detect the relationship between the frequency of two given tdTomato+ cell populations within a mouse. The regression line is plotted and significance given. Not significant (n.s). N = 8 mice.
Next we determined if frequencies of tdTomato+ cells in these four myeloid populations were correlated with each other and with the tdTomato+ B cell fraction in blood samples from Foxp3YFP/Cre+/− tdTomato+/− mice. We found significant correlation between the frequency of tdTomato+ monocytes and the tdTomato+ fraction we detected in both dendritic cells and neutrophils (Figure 2B; p < 0.0001), as well as B cells. Interestingly, the frequency of tdTomato+ eosinophils was not correlated with the corresponding tdTomato+ populations in dendritic cells, neutrophils, monocytes or B cells (Figure 2B; and data not shown).
YFP Expression in tdTomato+ Cells Is Restricted to CD4 T Cells in Foxp3YFP/Cre+/− tdTomato+/− Mice
Our data indicated that the Foxp3 promoter is active or has been active in multipotent progenitor cells other than those leading directly to Treg ontogeny. These results prompted us to determine if the Foxp3 promoter is currently active in the non-Treg tdTomato+ cells. To address this question, we exploited the fact that the Foxp3 promoter also drives expression of the YFP reporter in Foxp3YFP/Cre+/− tdTomato+/− mice. We reasoned that only cells with an active Foxp3 promoter would also be positive for YFP. YFP expression in CD4 T cells in the CLN and oral mucosa were almost exclusively tdTomato+ (Figure 3A). We found no evidence of YFP expression driven by Foxp3 promoter activity in tdTomato+ neutrophils, B cells or CD8 T cells. This data further supports our contention that the Foxp3 promoter is only transiently active in multipotent progenitor cells that lead to neutrophils, B cells and CD8 T cells and other immune cells. Interestingly, the majority of tdTomato+ CD4 T cells were no longer expressing YFP (Figure 3B). Since all mice were kept in specific pathogen-free conditions, it is highly unlikely that all tdTomato+ CD4 T cells are former Treg cells that have ceased Foxp3 promoter activity. Presumably, these tdTomato+ CD4 T cells derive from lymphoid progenitors that have transiently activated the Foxp3 promoter, expressed Cre recombinase and in doing so have activated the tdTomato reporter.
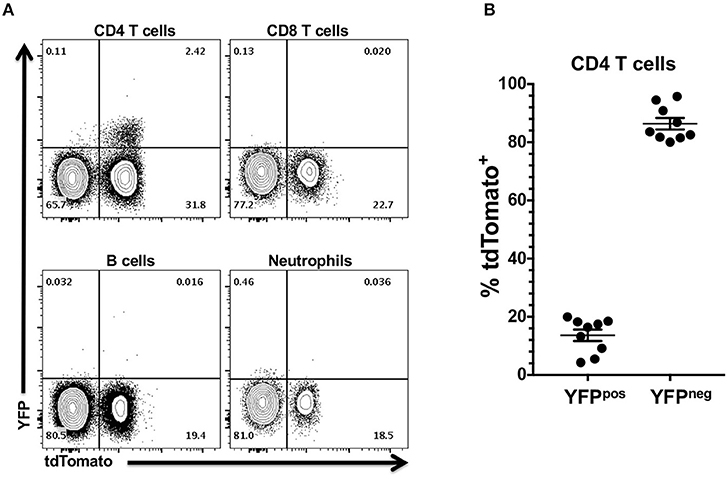
Figure 3. YFP expression in tdTomato+ cells from Foxp3YFP/Cre+/− tdTomato+/− mice is restricted to the CD4 T cell compartment. CLN and pooled oral mucosa of 6–8 week old Foxp3YFP/Cre+/− tdTomato+/− female mice were stained with rat anti-mouse CD3, CD4, CD8, and B220 mAbs (CLN) or rat anti-mouse CD45, I-A/I-E, CD11b, Ly-6G, and F4/80 mAbs (oral mucosa). All cells were stained with Zombie Aqua to discriminate live from dead cells. (A) Representative flow cytometry plots showing expression of YFP in CD4 T cell, CD8 T cell, B cell from CLN, and in neutrophils from three pooled oral mucosa samples. Numbers are indicative of the percentage of cells in a given gated population. (B) Summary plot data showing percentage of YFP+ or YFP− CD4 T cells. Individual data points are shown along with means and SEMs. N = 9 mice.
Stochastic Activity of Foxp3 Promoter Revealed by Variable Expression of tdTomato in CD4 T Cells Isolated From Spleens of Fetal Foxp3GFP/Cre/ERT2+/+ tdTomato+/+ Mice
Foxp3GFP/Cre/ERT2+/+ tdTomato+/+ mouse allows activation of tdTomato in cells that only have an active Foxp3 promoter at the time of tamoxifen exposure, thereby avoiding the confounding effect of transient and historical Foxp3 activity in multipotent progenitor cells. Moreover, this reporter mouse allows us to test for temporal stochastic activity of Foxp3 in a range of tissues. Tamoxifen readily crosses the placenta. However, preliminary experiments showed that tamoxifen administrated to pregnant females prevented term birth. To circumvent this issue, we analyzed tdTomato expression in tissues of fetal mice. Published expression data indicated that the Foxp3 gene may be active by embryonic day 12 (E12). Foxp3 expression in the central nervous system was been reported on E11.5 through E18, and well as the E14.5 limb and liver (39, 40). Expression in the hemolymphoid system, particularly the developing thymus, as well as alimentary system by E15.5 has been reported (41). Other groups however, have reported that Foxp3 activity at E15.5 only in the reproductive tract (42). This may speak either to the variable nature of Foxp3 expression or differences in analysis. Given these premises, we opted to administer tamoxifen to two pregnant sibling females at E12 and E13 and then harvested lungs and spleens from E20 fetal mice and CLN from the does (Figure 4A). Given that the half-life of Tamoxifen is 5–7 days, this would introduce a sufficient concentration into the systemic circulation to induce red fluorescent protein expression at E14.5-15.5 in the fetus. Foxp3 protein has not been detected in fetal mouse lungs at E14.5, so any red expression in the lung should be limited to transiting immune cells (43). In lung harvested from fetal mice, we found no evidence of tdTomato expression in the epithelial (CD45−, CD326+, CD31−) or endothelial compartments (CD45−, CD326+, CD31−) nor in the relatively abundant CD45+ cell fraction (data not shown). However, we observed expression of tdTomato in some CD4 T cells isolated from the fetal spleen and from the CLN of each doe (Figure 4B). The frequency of tdTomato+ CD4 T cells was highly variable within fetal cohorts ranging from 0 to 25.9% in one cohort and 0.63 to 16.6% in the other, but was very similar in the cervical lymph node samples from the two sibling does (Figure 4C). We also observed a small population of cervical lymph node-resident tdTomato+ CD8 T cells in the does consistent with CD8 Treg cells (44–48), but these cells were absent in all fetal spleens (Figure 4C). tdTomato+ expression was also absent within the larger B cell population of all fetal spleens (data not shown).
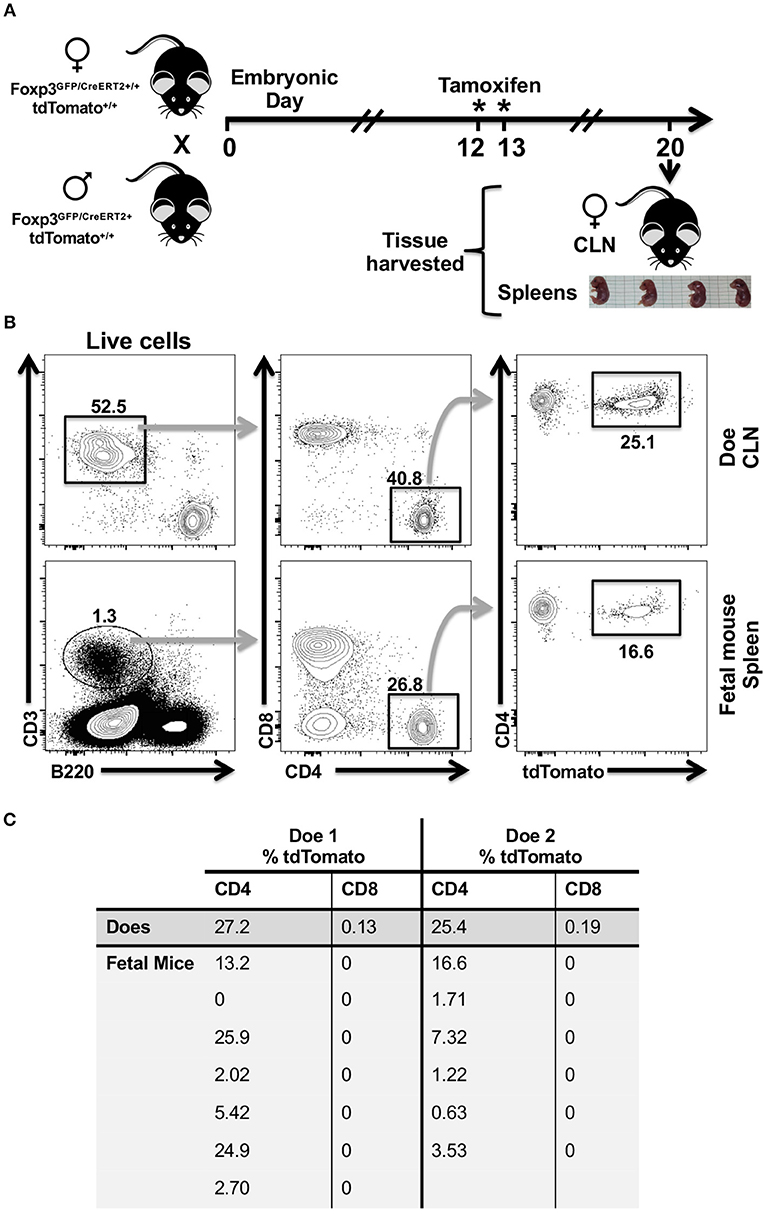
Figure 4. CD4 T cells recovered from the spleens of fetal mice cohorts show variability in tdTomato+ frequency in a tamoxifen-inducible Foxp3-Cre model. (A) Cartoon showing experimental timeline and design. Eight-week old sibling female Foxp3GFP/Cre/ERT2+/+ tdTomato+/+ mice were mated to the same hemizygous Foxp3GFP/Cre/ERT2+ tdTomato+/+ male mouse. Females (Does) that had evidence of a copulation plug on embryonic day 0 and that had gained weight by E12 compared to copulation plug minus siblings were orally administered tamoxifen on E12 and E13. (B) Single cell suspensions were obtained from CLN of does and fetal mice spleens, stained with Zombie Aqua and rat anti-mouse mAbs to identify CD4 and CD8 T cells by flow cytometry. Representative flow cytometry plots of CLN and a single fetal mouse spleen are shown. Numbers indicate the percentage of cells within a given gate. (C) Summary table showing percentage of tdTomato+ CD4 and CD8 T cells identified in the spleens of fetal mice and in the CLN of does. *Day of tamioxifen administration.
Widespread Expression of tdTomato in Homozygous Foxp3YFP/Cre+/+ tdTomato+/+ Mice
During the process of generating homozygous mouse lines, we observed that crosses involving F1 Foxp3YFP/Cre+/− tdTomato+/− mice generated a novel “red” phenotype that was clearly segregating amongst F2 littermates. This phenotype was not observed in our prior F1 experimental mice. This red phenotype typically correlated with a double homozygous Foxp3YFP/Cre+/+ tdTomato+/+ genotype in female pups or a Foxp3YFP/Cre+ tdTomato+/+ genotype in male pups. Note here that the Foxp3 transgene is X-linked. This red phenotype was not seen in any of the double homozygous IL17aCre+/+ tdTomato+/+ pups. The appearance of this red coloration was striking and seemed widespread in non-immune tissue. To examine this further we dissected female mice that were genotypically Foxp3YFP/Cre+/+ tdTomato+/+ (Figure 5A) or IL17aCre+/+ tdTomato+/+ (Figure 5B). In addition to skin cells, the red coloration was clearly visible in cells that comprise muscle, salivary glands and the capsule of CLN suggesting widespread expression of tdTomato in non-immune cells. We reasoned that a cervical lymph node would give a clear picture of tdTomato expression in immune cells as well as non-immune cells that comprise the capsule, cortex and medullary stroma. Using confocal microscopy CLN sections stained with DAPI and anti-B220, it was obvious that tdTomato is strongly expressed in the capsule and T cell zones and to a lesser extent in the B cell zone of CLN obtained from a red mouse (Figure 5C). In contrast, only a few tdTomato+ cells were seen in lymph nodes taken from an IL17aCre+/+ tdTomato+/+ mouse (Figure 5D). These cells are presumably Th17 cells and were primarily located along the fringes of the B cell zone.
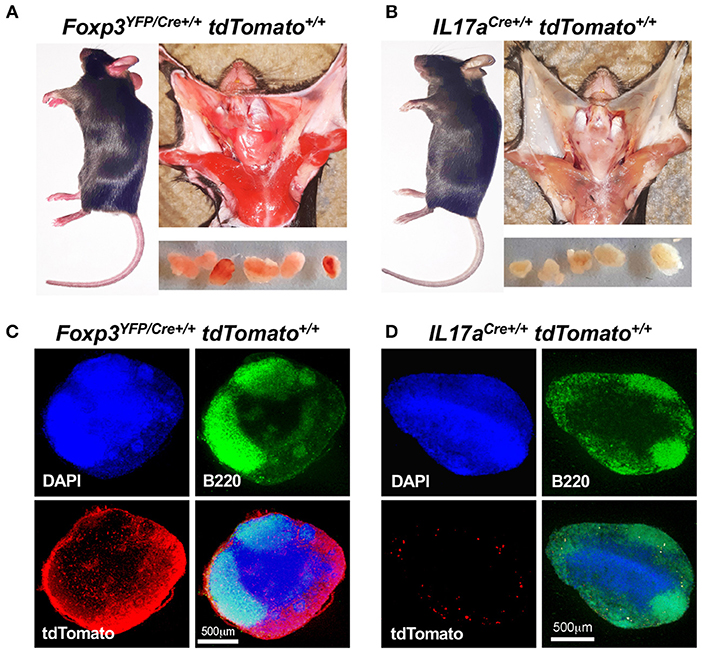
Figure 5. Foxp3YFP/Cre+/+ tdTomato+/+ mice express tdTomato in non-immune tissues. Visual comparison of Foxp3YFP/Cre+/+ tdTomato+/+ (A) and IL17aCre+/+ tdTomato+/+ mice (B) showing “red” coloration in tissues of the Foxp3YFP/Cre+/+ tdTomato+/+ mouse. Dissected CLN are shown in the lower panels of each montage. Frozen sections from CLN of Foxp3YFP/Cre+/+ tdTomato+/+ (C) and IL17aCre+/+ tdTomato+/+ mice (D) were stained with DAPI and rat anti-mouse B220 mAb conjugated to FITC and images captured at 40 X using a confocal microscope. Red fluorescence was captured in the tdTomato channel. Merged images, with a scale bar (500 μm), are shown in the lower right quadrant of each photograph.
Activation of the Foxp3 Promoter Occurs Prior to Thymocyte Selection in Red Foxp3YFP/Cre+/+ tdTomato+/+ Female Mice
Widespread expression of tdTomato in red Foxp3YFP/Cre+/+ tdTomato+/+ mice together with our data that suggested transient activity of the Foxp3 promoter in immune progenitor cells, prompted us to examine thymocytes for expression of tdTomato. Thymocytes were prepared from the thymi of four red 4-week-old female Foxp3YFP/Cre+/+ tdTomato+/+ mice and a single age-matched IL17aCre+/+ tdTomato+/+ female mouse to serve as a control. Live mature and immature thymocytes were identified by flow cytometry. Classic immature double negative (DN: CD4− CD8−) or double positive (DP: CD4+ CD8+) thymocytes as well as mature CD4+ and CD8+ thymocytes were identified (Figure 6A). tdTomato expression in each of these thymocyte groups was compared in the equivalent populations obtained from the IL17aCre+/+ tdTomato+/+ mouse (gray histogram) and Foxp3YFP/Cre+/+ tdTomato+/+ mice (white histograms; Figure 6B). This analysis clearly showed that tdTomato was expressed in all four of the identified thymocyte populations. DN cells are considered early progenitors, but they themselves can be further subdivided into DN1 (CD25− CD44+), DN2 (CD25+ CD44+), DN3 (CD25+ CD44−), and DN4 (CD25− CD44−) populations based on the expression and sequential loss of cell surface markers CD25 and CD44 (Figure 6C) (49). We decided to also look for tdTomato expression in these thymocyte populations. Our analysis revealed tdTomato expression in the earliest of these progenitor thymocytes, DN4 (CD25− CD44−), indicating that activation of the tdTomato reporter gene occurred very early in the stem cell lineage that leads to thymocytes (Figure 6D).
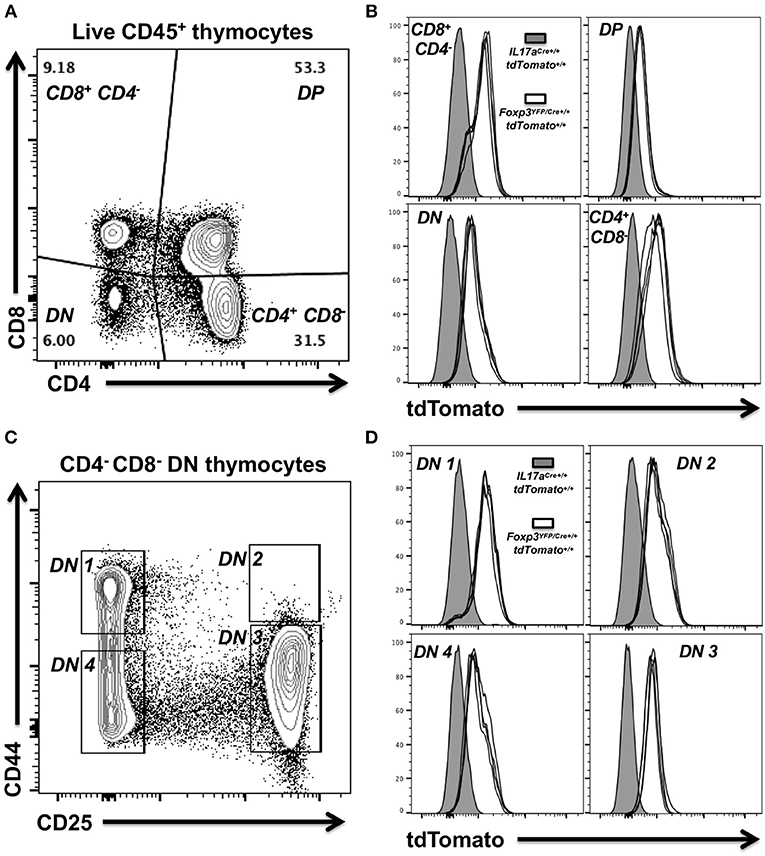
Figure 6. Expression of tdTomato occurs early during thymocyte selection in Foxp3YFP/Cre+/+ dtTomato+/+ homozygous female mice. Thymocytes were prepared from thymi isolated from 4 week-old female Foxp3YFP/Cre+/+ tdTomato+/+or IL17aCre+/+ tdTomato+/+ mice. Live mature and immature thymocytes were identified using Zombie Aqua, stained with a panel of rat anti-mouse mAbs and analyzed by flow cytometry. (A) Representative flow cytometry plot showing gating strategy used to identify classic immature double negative (DN—CD4− CD8−) or double positive (DP—CD4+ CD8+) thymocytes and mature CD4 and CD8 T cells. Numbers shown indicate the percentage of cells in a given gated population. (B) Summary plot data from four female Foxp3YFP/Cre+/+ tdTomato+/+ mice showing tdTomato expression in the four thymocyte populations identified. Thymocytes obtained from a single 4 week-old female IL17aCre+/+ tdTomato+/+ mouse were used as baseline of no tdTomato. (C) Representative flow cytometry plot showing gating strategy used to identify DN1 (CD25− CD44+), DN2 (CD25+ CD44+), DN3 (CD25+ CD44−), or DN4 (CD25− CD44−) thymocyte progenitor populations amongst DN cells (CD4− CD8−). (D) Summary plot data from four female Foxp3YFP/Cre+/+ tdTomato+/+ mice and the IL17aCre+/+ tdTomato+/+ control.
Expression of tdTomato in Foxp3YFP/Cre− tdTomato+/+ Male Mice
To determine if the red phenotype is associated with a particular genotype, we used a simple binary visual scoring system to score F2 siblings and later generations as red or “non-red” and then used PCR to identify their genotype. As expected from our earlier data, all female mice that were double homozygous Foxp3YFP/cre+/+ tdTomato+/+ had the visual red phenotype (Table 1). While the majority of Foxp3YFP/cre+ tdTomato+/+ males were red, unexpectedly some non-red males of this genotype were also observed. Foxp3YFP/cre+ tdTomato+/− heterozygous males were equally split between red and non-red phenotypes. In females, the Foxp3YFP/cre+/+ tdTomato+/− genotype yielded predominantly non-red mice whereas the Foxp3YFP/cre+/− tdTomato+/+ genotype gave predominantly red mice. Intriguingly, we identified two F3 brothers that were visually red that after repeated genotyping showed the presence of only the wild-type Foxp3 allele and not the Foxp3YFP/cre transgene (data not shown). The mother was Foxp3YFP/cre+/− tdTomato+/+ and the father was Foxp3YFP/cre+ tdTomato+/−, both having a red phenotype. This result suggests that the tdTomato gene received by these two F3 male siblings already had the stop codon excised by activity of Foxp3YFP/cre in germline cell progenitors in one of the parents, thereby making its expression independent of the presence of the Foxp3YFP/cre transgene.
Discussion
We developed fate-tracking reporter mouse strains to examine plasticity in Th17 and Treg cells in the context of a murine model of chronic periodontitis. Both these cell types have been reported to undergo cytokine reprogramming during conditions of heightened chronic inflammation and a shifting cytokine milieu that develop during a number of CD4 T cell-mediated autoimmune diseases (6, 7, 13, 19, 50, 51). In a preliminary set of experiments to determine the specificity and fitness of the fate-tracking reporter mice, we observed unexpected tdTomato expression in non-target lymphoid and myeloid cells within Foxp3YFP/Cre+/− tdTomato+/− mice. Translation of the tdTomato gene construct is tightly controlled in B6.Cg-Gt(ROSA)26Sortm14(CAG−tdTomato)Hze/J mice by an inframe loxP-flanked stop codon (5). Moreover, we did not detect tdTomato in these cells in IL17aCre+/− tdTomato+/− mice ruling out the possibility that non-target cell expression in Foxp3YFP/Cre+/− tdTomato+/− mice is due to leaky translation of the tdTomato transgene. Some CD8 T cells, however, have been reported to act as immuno-regulatory CD8 Treg cells expressing Foxp3 in a number of murine models of disease (44–48), in bone homeostasis (52) and at low background levels (20). Therefore, detecting expression of tdTomato in CD8 T cells in both the Foxp3YFP/Cre+/− tdTomato+/− and Foxp3GFP/Cre/ERT2+/+ tdTomato+/+ mice is consistent with these observations, even in homeostatic specific pathogen-free mice. It has been reported that CD8 Treg cells do not express CD28 or have low expression (46, 53) and they appear to differentiate in the thymus of mice (53). We did not determine CD28 expression on tdTomato+ CD8 T cells. However, we consider it unlikely that these are CD8 Treg cells as our analysis of B cells and CD8 T cells revealed that expression of tdTomato appeared to be stochastically determined in Foxp3YFP/Cre+/− tdTomato+/− mice, ruling out a developmentally regulated program of CD8 Treg cell polarization. Moreover, the frequencies of tdTomato+ CD8 T cells in Foxp3YFP/Cre+/− tdTomato+/− mice were at least 5–10-fold higher than YFP+ Foxp3+ CD8 T cells reported in the original mouse strain (20). Furthermore, the frequency of tdTomato+ CD8 T cells we observed in the CLN from our Foxp3GFP/Cre/ERT2+/+ tdTomato+/+ females, a model that avoids the issue of tdTomato reporter activation due to transient stochastic Foxp3 driven Cre expression, was consistent with reports of CD8 Treg cell frequency (20).
We chose the B6.129(Cg)-Foxp3tm4(YFP/Cre)Ayr/J mouse strain to develop a model whereby we could track the expression of pro-inflammatory cytokines IL-17A and IFN-γ in ex-CD4 Treg cells over the course of a chronic disease using tdTomato expression as a marker of Treg differentiation. However, we found that tdTomato was activated in a broad range of CD4 T cells. Between 80 and 95% of tdTomato+ CD4 T cells were not expressing YFP, which by design is the readout of an active Foxp3 promoter in these mice. We consider it highly unlikely that these YFP negative tdTomato+ CD4 T cells could all be exTregs. A more likely scenario is that these cells comprise a combination of naïve or recirculating memory or effector CD4 T cells where transient expression of Cre recombinase has activated the tdTomato reporter gene. This makes such a mouse model highly problematic for tracking authentic ex-Treg cells. For example, in this model it is more likely that YFP negative tdTomato+ CD4 T cells expressing IL-17A are simply Th17 cells and not ex-CD4 Treg cells expressing IL-17A de novo (17–19).
tdTomato expression in Foxp3YFP/Cre+/− tdTomato+/− mice revealed evidence of the stochastic nature of Foxp3 activity and that this activity occurred in multipotent progenitor cells of both lymphoid and myeloid lineages. Interestingly, lack of correlation between tdTomato+ frequency in the eosinophil population with other myeloid cells examined (i.e., monocytes and neutrophils), suggests that in the eosinophil precursor lineage, Foxp3 is activated stochastically at a different frequency to other hemopoietic stem cell-derived cells. We speculate that such differences between eosinophils and monocytes, neutrophils, B cells, and dendritic cells may be related to hemopoietic stem cell microenvironments within the bone marrow or fetal liver (54, 55).
Variability in the frequency of tdTomato+ cells within populations of CD8 T cells, B cells and monocytes for example is highly suggestive of random Foxp3 promoter activity outside of the normal developmental program. Our analysis of tdTomato expression in fetal cohorts from Foxp3GFP/Cre/ERT2+/+ tdTomato+/+ does underscored this conclusion. If Foxp3 promoter activity, and therefore Cre recombinase expression, was developmentally regulated then we would expect to see similar tdTomato+ frequencies in fetal cohorts given that each fetus was at the same embryonic age when given tamoxifen and when tissues were harvested. The fact that we observed high variation in the frequency of tdTomato+ CD4 T cells within a fetal cohort speaks to the random nature of Foxp3 activity at least in these CD4 T cells. Interestingly, unlike for Foxp3YFP/Cre+/− tdTomato+/− mice, in Foxp3GFP/Cre/ERT2+/+ tdTomato+/+ mice we did not observe tdTomato expression in CD8 T cells and B cells from spleen or non-immune cells like epithelial and endothelial cells from fetal lung. This observation likely reflects differences in when the Foxp3 promoter is transiently and/or stochastically activated in embryonic tissue. For example, transient and/or stochastic Foxp3 promoter activity in multipotent progenitor cells that lead to B and T cell lineages may occur prior to or well-after embryonic day 12 and 13 or when tamoxifen is metabolized.
Both Cre recombinase and the tdTomato transgene construct can be considered dominant traits. Interestingly, we observed a novel striking red phenotype that segregated amongst littermates resulting from F1 Foxp3YFP/Cre+/−tdTomato+/− crosses. This red phenotype primarily was found in mice that carried two copies of each of the transgenes. This novel red phenotype could be attributed to widespread expression of tdTomato beyond the target Treg cell type. In an analysis of immature thymocytes from visually “red” mice, we found that the earliest of the thymocyte progenitors expressed tdTomato suggesting early, albeit transient, expression of the Foxp3 promoter had occurred even earlier in the lineage. Our data contrasts with an earlier report where B6.129(Cg)-Foxp3tm4(YFP/Cre)Ayr/J mouse strain was crossed to R26RFP or R26YFP mice (32). These authors did not report issues with expression of RFP or YFP outside of Treg cells, although it appears that homozygous mice were not tested. It is also not clear which R26RFP reporter mouse they used. Differences between their work and ours may also reflect the differential accessibility of different floxed alleles by Cre recombinase (3, 4). Nonetheless, our work points to potential issues with the interpretation of data generated from crosses involving the B6.129(Cg)-Foxp3tm4(YFP/Cre)Ayr/J mouse strain (20, 26–30), particularly if deletion of floxed genes was not monitored in non-target cells and other experimentation, such as adoptive transfer, was not used to confirm the results (27, 56). For example, IL-10 targeted for deletion in Treg cells (20), is produced by a wide range of immune cells and our data suggests that the IL-10 gene could also have been disrupted in those cells, which could have led to a “heightened” loss of IL-10 effect and one that would not be solely attributed to Treg cells. Furthermore, using this mouse strain to target deletion of STAT3 or Tbet in Treg cells would also have likely affected Th17 and Th1 cells, respectively, which calls into question some of the data particularly as it relates to diseases where Th17 or Th1 cells may also play a contributory role (28, 30).
We also reveal one further potential issue of the B6.129(Cg)-Foxp3tm4(YFP/Cre)Ayr/J mouse strain. We showed that it was possible for the red phenotype to segregate independently of the Foxp3YFP/Cre transgene. Presumably, excision of the floxed stop signal had occurred early in germline progenitors in one of the parents and that an activated tdTomato gene could now segregate independently of the Foxp3YFP/Cre transgene. It would, therefore, be problematic if negative controls are selected on the basis of the absence of the Foxp3YFP/Cre transgene in littermates that are otherwise homozygous for the floxed target gene. Our data indicates that some of these negative controls may in fact carry the floxed gene deletion and it would be prudent to test for this potential scenario.
In summary, our data shows that the use of the B6.129(Cg)-Foxp3tm4(YFP/Cre)Ayr/J mouse strain to generate mutations based on floxed genes targeted to Treg cells requires special consideration of the potential to disrupt these genes in non-target cell types. Additional experimentation would be prudent to confirm results obtained in vivo in a whole mouse. Transcription factors, such as Foxp3, may be problematic in forming the basis of a Cre recombinase mouse models, as they are often not tissue or cell specific but have pleiotropic or transient activities. Better models are based on tightly regulated cell specific markers to cell types. The development of the Foxp3 cre-ert2 mouse strain (33), which allows Cre recombinase to be active on nuclear DNA only upon the administration of tamoxifen, would likely mitigate against most of the issues of transient expression from the Foxp3 promoter that our paper has highlighted.
Data Availability
The datasets generated for this study are available on request to the corresponding author.
Ethics Statement
This study was carried out in compliance with the Association for Assessment and Accreditation of Laboratory Animal Care at the University of Minnesota. All mouse experiments were reviewed and approved by the Institutional Animal Care and Use Committee of the University of Minnesota.
Author Contributions
MC and PB-E designed the experiments and interpreted the data. PB-E drafted the manuscript. All authors performed the experiments and edited and reviewed the manuscript.
Funding
This research was supported by NIH grants R03 DE025882 to PB-E and R21 DE026209A to MC, and was also supported in part by the Schaffer Chair for Periodontal Research at the University of Minnesota.
Conflict of Interest Statement
The authors declare that the research was conducted in the absence of any commercial or financial relationships that could be construed as a potential conflict of interest.
Acknowledgments
We acknowledge the University of Minnesota UFCR for flow cytometry resources.
References
1. Ray MK, Fagan SP, Brunicardi FC. The Cre-loxP system: a versatile tool for targeting genes in a cell- and stage-specific manner. Cell Transplant. (2000) 9:805–15. doi: 10.1177/096368970000900607
2. Nagy A. Cre recombinase: the universal reagent for genome tailoring. Genesis. (2000) 26:99–109. doi: 10.1002/(SICI)1526-968X(200002)26:2<99::AID-GENE1>3.0.CO;2-B
3. García-Otín AL, Guillou F. Mammalian genome targeting using site-specific recombinases. Front Biosci. (2006) 11:1108–36. doi: 10.2741/1867
4. Bouabe H, Okkenhaug K. Gene targeting in mice: a review. Methods Mol Biol. (2013) 1064:315–36. doi: 10.1007/978-1-62703-601-6_23
5. Madisen L, Zwingman TA, Sunkin SM, Oh SW, Zariwala HA, Gu H, et al. A robust and high-throughput Cre reporting and characterization system for the whole mouse brain. Nat Neurosci. (2010) 13:133–40. doi: 10.1038/nn.2467
6. Hirota K, Duarte JH, Veldhoen M, Hornsby E, Li Y, Cua DJ, et al. Fate mapping of IL-17-producing T cells in inflammatory responses. Nat Immunol. (2011) 12:255–63. doi: 10.1038/ni.1993
7. Hirota K, Turner JE, Villa M, Duarte JH, Demengeot J, Steinmetz OM, et al. Plasticity of Th17 cells in Peyer's patches is responsible for the induction of T cell-dependent IgA responses. Nat Immunol. (2013) 14:372–9. doi: 10.1038/ni.2552
8. Hasenberg A, Hasenberg M, Männ L, Neumann F, Borkenstein L, Stecher M, et al. Catchup: a mouse model for imaging-based tracking and modulation of neutrophil granulocytes. Nat Methods. (2015) 12:445–52. doi: 10.1038/nmeth.3322
9. Shi G, Cox CA, Vistica BP, Tan C, Wawrousek EF, Gery I. Phenotype switching by inflammation-inducing polarized Th17 cells, but not by Th1 cells. J Immunol. (2008) 181:7205–13. doi: 10.4049/jimmunol.181.10.7205
10. Lee YK, Turner H, Maynard CL, Oliver JR, Chen D, Elson CO, et al. Late developmental plasticity in the T helper 17 lineage. Immunity. (2009) 30:92–107. doi: 10.1016/j.immuni.2008.11.005
11. Zielinski CE, Mele F, Aschenbrenner D, Jarrossay D, Ronchi F, Gattorno M, et al. Pathogen-induced human TH17 cells produce IFN-gamma or IL-10 and are regulated by IL-1beta. Nature. (2012) 484:514–8. doi: 10.1038/nature10957
12. Martin-Orozco N, Chung Y, Chang SH, Wang YH, Dong C. Th17 cells promote pancreatic inflammation but only induce diabetes efficiently in lymphopenic hosts after conversion into Th1 cells. Euro J Immunol. (2009) 39:216–24. doi: 10.1002/eji.200838475
13. Nistala K, Adams S, Cambrook H, Ursu S, Olivito B, de Jager W, et al. Th17 plasticity in human autoimmune arthritis is driven by the inflammatory environment. Proc Natl Acad Sci USA. (2010) 107:14751–6. doi: 10.1073/pnas.1003852107
14. Feng T, Qin H, Wang L, Benveniste EN, Elson CO, Cong Y. Th17 cells induce colitis and promote Th1 cell responses through IL-17 induction of innate IL-12 and IL-23 production. J Immunol. (2011) 186:6313–8. doi: 10.4049/jimmunol.1001454
15. Morrison PJ, Bending D, Fouser LA, Wright JF, Stockinger B, Cooke A, et al. Th17-cell plasticity in Helicobacter hepaticus-induced intestinal inflammation. Mucosal Immunol. (2013) 6:1143–56. doi: 10.1038/mi.2013.11
16. Zeng H, Yang K, Cloer C, Neale G, Vogel P, Chi H. mTORC1 couples immune signals and metabolic programming to establish T(reg)-cell function. Nature. (2013) 499:485–90. doi: 10.1038/nature12297
17. Okui T, Aoki Y, Ito H, Honda T, Yamazaki K. The presence of IL-17+/FOXP3+ double-positive cells in periodontitis. J Dental Res. (2012) 91:574–9. doi: 10.1177/0022034512446341
18. Sawant DV, Vignali DA. Once a Treg, always a Treg? Immunol Rev. (2014) 259:173–91. doi: 10.1111/imr.12173
19. Zhou X, Bailey-Bucktrout SL, Jeker LT, Penaranda C, Martinez-Llordella M, Ashby M, et al. Instability of the transcription factor Foxp3 leads to the generation of pathogenic memory T cells in vivo. Nat Immunol. (2009) 10:1000–7. doi: 10.1038/ni.1774
20. Rubtsov YP, Rasmussen JP, Chi EY, Fontenot J, Castelli L, Ye X, et al. Regulatory T cell-derived interleukin-10 limits inflammation at environmental interfaces. Immunity. (2008) 28:546–58. doi: 10.1016/j.immuni.2008.02.017
21. Brucklacher-Waldert V, Ferreira C, Innocentin S, Kamdar S, Withers DR, Kullberg MC, et al. Tbet or continued RORγt expression is not required for Th17-associated immunopathology. J Immunol. (2016) 196:4893–904. doi: 10.4049/jimmunol.1600137
22. Kim HS, Jang SW, Lee W, Kim K, Sohn H, Hwang SS, et al. PTEN drives Th17 cell differentiation by preventing IL-2 production. J Exp Med. (2017) 214:3381–98. doi: 10.1084/jem.20170523
23. Carr TM, Wheaton JD, Houtz GM, Ciofani M. JunB promotes Th17 cell identity and restrains alternative CD4. Nat Commun. (2017) 8:301. doi: 10.1038/s41467-017-00380-3
24. Conti HR, Peterson AC, Brane L, Huppler AR, Hernández-Santos N, Whibley N, et al. Oral-resident natural Th17 cells and γδ T cells control opportunistic Candida albicans infections. J Exp Med. (2014) 211:2075–84. doi: 10.1084/jem.20130877
25. Papotto PH, Gonçalves-Sousa N, Schmolka N, Iseppon A, Mensurado S, Stockinger B, et al. IL-23 drives differentiation of peripheral γδ17 T cells from adult bone marrow-derived precursors. EMBO Rep. (2017) 18:1957–67. doi: 10.15252/embr.201744200
26. Do J, Kim D, Kim S, Valentin-Torres A, Dvorina N, Jang E, et al. Treg-specific IL-27Rα deletion uncovers a key role for IL-27 in Treg function to control autoimmunity. Proc Natl Acad Sci USA. (2017) 114:10190–5. doi: 10.1073/pnas.1703100114
27. Muto G, Kotani H, Kondo T, Morita R, Tsuruta S, Kobayashi T, et al. TRAF6 is essential for maintenance of regulatory T cells that suppress Th2 type autoimmunity. PLoS ONE. (2013) 8:e74639. doi: 10.1371/journal.pone.0074639
28. Kluger MA, Melderis S, Nosko A, Goerke B, Luig M, Meyer MC, et al. Treg17 cells are programmed by Stat3 to suppress Th17 responses in systemic lupus. Kidney Int. (2016) 89:158–66. doi: 10.1038/ki.2015.296
29. Bankoti R, Ogawa C, Nguyen T, Emadi L, Couse M, Salehi S, et al. Differential regulation of effector and regulatory T cell function by Blimp1. Sci Rep. (2017) 7:12078. doi: 10.1038/s41598-017-12171-3
30. Nosko A, Kluger MA, Diefenhardt P, Melderis S, Wegscheid C, Tiegs G, et al. T-bet enhances regulatory T cell fitness and directs control of Th1 responses in crescentic GN. J Am Soc Nephrol. (2017) 28:185–96. doi: 10.1681/ASN.2015070820
31. Abram CL, Roberge GL, Hu Y, Lowell CA. Comparative analysis of the efficiency and specificity of myeloid-Cre deleting strains using ROSA-EYFP reporter mice. J Immunol Methods. (2014) 408:89–100. doi: 10.1016/j.jim.2014.05.009
32. Franckaert D, Dooley J, Roos E, Floess S, Huehn J, Luche H, et al. Promiscuous Foxp3-cre activity reveals a differential requirement for CD28 in Foxp3+ and Foxp3− T cells. Immunol Cell Biol. (2015) 93:417–23. doi: 10.1038/icb.2014.108
33. Rubtsov YP, Niec RE, Josefowicz S, Li L, Darce J, Mathis D, et al. Stability of the regulatory T cell lineage in vivo. Science. (2010) 329:1667–71. doi: 10.1126/science.1191996
34. Nakamura E, Nguyen MT, Mackem S. Kinetics of tamoxifen-regulated Cre activity in mice using a cartilage-specific CreER(T) to assay temporal activity windows along the proximodistal limb skeleton. Dev Dyn. (2006) 235:2603–12. doi: 10.1002/dvdy.20892
35. Lizen B, Claus M, Jeannotte L, Rijli FM, Gofflot F. Perinatal induction of Cre recombination with tamoxifen. Transgenic Res. (2015) 24:1065–77. doi: 10.1007/s11248-015-9905-5
36. Fischer LA, Bittner-Eddy PD, Costalonga M. Fetal weight outcomes in C57BL/6J and C57BL/6NCrl mice after oral colonization with P. gingivalis. Infect Immun. (2019). doi: 10.1128/iai.00280-19
37. Bittner-Eddy PD, Fischer LA, Kaplan DH, Thieu K, Costalonga M. Mucosal langerhans cells promote differentiation of Th17 cells in a murine model of periodontitis but are not required for Porphyromonas gingivalis-driven alveolar bone destruction. J Immunol. (2016) 197:1435–46. doi: 10.4049/jimmunol.1502693
38. Bittner-Eddy PD, Fischer LA, Tu AA, Allman DA, Costalonga M. Discriminating between interstitial and circulating leukocytes in tissues of the murine oral mucosa avoiding nasal-associated lymphoid tissue contamination. Front Immunol. (2017) 8:1398. doi: 10.3389/fimmu.2017.01398
39. Yue F, Cheng Y, Breschi A, Vierstra J, Wu W, Ryba T, et al. A comparative encyclopedia of DNA elements in the mouse genome. Nature. (2014) 515:355–64. doi: 10.1038/nature13992
40. Magdaleno S, Jensen P, Brumwell CL, Seal A, Lehman K, Asbury A, et al. BGEM: an in situ hybridization database of gene expression in the embryonic and adult mouse nervous system. PLoS Biol. (2006) 4:e86. doi: 10.1371/journal.pbio.0040086
41. Chung YC, Tsai YJ, Shiu TY, Sun YY, Wang PF, Chen CL. Screening large numbers of expression patterns of transcription factors in late stages of the mouse thymus. Gene Expr Patterns. (2011) 11:84–92. doi: 10.1016/j.gep.2010.09.007
42. McMahon AP, Aronow BJ, Davidson DR, Davies JA, Gaido KW, Grimmond S, et al. GUDMAP: the genitourinary developmental molecular anatomy project. J Am Soc Nephrol. (2008) 19:667–71. doi: 10.1681/asn.2007101078
43. Visel A, Thaller C, Eichele G. GenePaint.org: an atlas of gene expression patterns in the mouse embryo. Nucleic Acids Res. (2004) 32:D552–6. doi: 10.1093/nar/gkh029
44. Han YK, Jin Y, Miao YB, Shi T, Lin XP. CD8. Inflammation. (2018) 41:1791–803. doi: 10.1007/s10753-018-0822-7
45. Zou Q, Wu B, Xue J, Fan X, Feng C, Geng S, et al. CD8+ Treg cells suppress CD8+ T cell-responses by IL-10-dependent mechanism during H5N1 influenza virus infection. Eur J Immunol. (2014) 44:103–14. doi: 10.1002/eji.201343583
46. Ben-David H, Sharabi A, Dayan M, Sela M, Mozes E. The role of CD8+CD28 regulatory cells in suppressing myasthenia gravis-associated responses by a dual altered peptide ligand. Proc Natl Acad Sci USA. (2007) 104:17459–64. doi: 10.1073/pnas.0708577104
47. Iamsawat S, Daenthanasanmak A, Voss JH, Nguyen H, Bastian D, Liu C, et al. Stabilization of Foxp3 by targeting JAK2 enhances efficacy of CD8 induced regulatory T cells in the prevention of graft-versus-host disease. J Immunol. (2018) 201:2812–23. doi: 10.4049/jimmunol.1800793
48. Nakagawa T, Tsuruoka M, Ogura H, Okuyama Y, Arima Y, Hirano T, et al. IL-6 positively regulates Foxp3+CD8+ T cells in vivo. Int Immunol. (2010) 22:129–39. doi: 10.1093/intimm/dxp119
49. Godfrey DI, Kennedy J, Suda T, Zlotnik A. A developmental pathway involving four phenotypically and functionally distinct subsets of CD3-CD4-CD8- triple-negative adult mouse thymocytes defined by CD44 and CD25 expression. J Immunol. (1993) 150:4244–52.
50. Ueno A, Jijon H, Chan R, Ford K, Hirota C, Kaplan GG, et al. Increased prevalence of circulating novel IL-17 secreting Foxp3 expressing CD4+ T cells and defective suppressive function of circulating Foxp3+ regulatory cells support plasticity between Th17 and regulatory T cells in inflammatory bowel disease patients. Inflamm Bowel Dis. (2013) 19:2522–34. doi: 10.1097/MIB.0b013e3182a85709
51. Hovhannisyan Z, Treatman J, Littman DR, Mayer L. Characterization of interleukin-17-producing regulatory T cells in inflamed intestinal mucosa from patients with inflammatory bowel diseases. Gastroenterology. (2011) 140:957–65. doi: 10.1053/j.gastro.2010.12.002
52. Buchwald ZS, Kiesel JR, Yang C, DiPaolo R, Novack DV, Aurora R. Osteoclast-induced Foxp3+ CD8 T-cells limit bone loss in mice. Bone. (2013) 56:163–73. doi: 10.1016/j.bone.2013.05.024
53. Vuddamalay Y, Attia M, Vicente R, Pomié C, Enault G, Leobon B, et al. Mouse and human CD8+ CD28low regulatory T lymphocytes differentiate in the thymus. Immunology. (2016) 148:187–96. doi: 10.1111/imm.12600
54. Morrison SJ, Hemmati HD, Wandycz AM, Weissman IL. The purification and characterization of fetal liver hematopoietic stem cells. Proc Natl Acad Sci USA. (1995) 92:10302–6. doi: 10.1073/pnas.92.22.10302
55. Coskun S, Hirschi KK. Establishment and regulation of the HSC niche: roles of osteoblastic and vascular compartments. Birth Defects Res C Embryo Today. (2010) 90:229–42. doi: 10.1002/bdrc.20194
Keywords: Foxp3, Treg cells, Th17 cells, Cre recombinase, fate-tracking
Citation: Bittner-Eddy PD, Fischer LA and Costalonga M (2019) Cre-loxP Reporter Mouse Reveals Stochastic Activity of the Foxp3 Promoter. Front. Immunol. 10:2228. doi: 10.3389/fimmu.2019.02228
Received: 11 April 2019; Accepted: 03 September 2019;
Published: 20 September 2019.
Edited by:
Wanjun Chen, National Institutes of Health (NIH), United StatesReviewed by:
Baojun Zhang, Xi'an Jiaotong University, ChinaXing Chang, Westlake Institute for Advanced Study (WIAS), China
Copyright © 2019 Bittner-Eddy, Fischer and Costalonga. This is an open-access article distributed under the terms of the Creative Commons Attribution License (CC BY). The use, distribution or reproduction in other forums is permitted, provided the original author(s) and the copyright owner(s) are credited and that the original publication in this journal is cited, in accordance with accepted academic practice. No use, distribution or reproduction is permitted which does not comply with these terms.
*Correspondence: Massimo Costalonga, Y29zdGEwMDJAdW1uLmVkdQ==