- 1Programa de Pós-Graduação em Imunologia Básica e Aplicada, Instituto de Ciências Biológicas, Universidade Federal do Amazonas (UFAM), Manaus, Brazil
- 2Programa de Pós-Graduação em Biociência e Biotecnologia, Faculdade de Ciências Farmacêuticas de Ribeirão Preto, Universidade de São Paulo (USP), Ribeirão Preto, Brazil
- 3Departamento de Análises Clínicas, Toxicológicas e Bromatológicas, Faculdade de Ciências Farmacêuticas de Ribeirão Preto, Universidade de São Paulo (USP), Ribeirão Preto, Brazil
- 4Departamento de Ensino e Pesquisa, Fundação Hospitalar de Hematologia e Hemoterapia do Amazonas (HEMOAM), Manaus, Brazil
- 5Programa de Pós-Graduação em Medicina Tropical, Universidade do Estado do Amazonas (UEA), Manaus, Brazil
- 6Instituto de Pesquisa Clínica Carlos Borborema, Fundação de Medicina Tropical Doutor Heitor Vieira Dourado (FMT-HVD), Manaus, Brazil
- 7Programa de Pós-Graduação em Ciências Aplicadas à Hematologia, Universidade do Estado do Amazonas (UEA), Manaus, Brazil
- 8Programa de Pós-Graduação da Bioquímica, Faculdade de Medicina de Ribeirão Preto, Universidade de São Paulo (USP), Ribeirão Preto, Brazil
- 9Departamento de Química, Faculdade de Filosofia, Ciências e Letras de Ribeirão Preto, Universidade de São Paulo (USP), Ribeirão Preto, Brazil
- 10Instituto de Pesquisas Leônidas & Maria Deane, FIOCRUZ-Amazônia, Manaus, Brazil
The participation of cytokines and chemokines in Plasmodium vivax malaria (Pv-malaria) activates the immune response and thus causes the production of several inflammatory mediators. This process is already well-established, but little is known about eicosanoids in malaria physiopathology, especially in regards to inflammation and immunity. Malaria is an acute febrile syndrome similar to any other less important infectious disease and people may self-medicate with any anti-inflammatory drugs in order to cease the recurrent symptoms of the disease. Based on this information, the study describes the eicosanoid profile and its possible influence on the production of cytokines and chemokines in P. vivax infections. In addition, we investigated the influence of self-medication with anti-inflammatory drugs in this immune profile. Twenty-three patients were included in the study, with or without self-medication by anti-inflammatory drugs prior to diagnosis. A total 12 individuals were selected for the control group. Eicosanoid profiles were quantified by HPLC-MS/MS, and cytokines and chemokines by flow cytometry and ELISA. The Pv-malaria infection significantly reduces the production of several lipid mediators, and its action is increased by self-medication. We observed that the eicosanoids we found derive from the lipoxygenase and cyclooxygenase pathways, and present positive and negative correlations with chemokines and cytokines in the follow-up of patients. Our data suggest that self-medication may interfere in the immunological characteristics in P. vivax infection and may modify the follow-up of the disease.
Introduction
Malaria is one of the oldest infectious diseases on earth, and often leads to severe disease or even death (1). Caused by the Apicomplexa protozoans from Plasmodium genus, five species infect and cause disease in humans (Plasmodium falciparum, P. knowlesi, P. malariae, P. ovale, and P. vivax) (2–4). Their transmission occurs via the bite of the female mosquito of the Anopheles genus (5). Approximately 216 million cases of malaria were diagnosed worldwide in 2016, with 445,000 deaths. In Brazil, 175,000 cases were reported in 2017. The Amazon region contributes to nearly 99% of the malaria notifications and Plasmodium vivax is responsible for most diagnosed cases (6–8).
The onset of the immune response occurs when the mosquito inoculates the sporozoites in the skin, which may be phagocytosed by dendritic cells and macrophages and presented to the lymphocytes (9). Hemolysis of red blood cells infected with Plasmodium is the main pathogenic mechanism observed during infection. Parasites release endotoxins known as hemozoin linked to parasitic DNA that bind to the Toll-like Receptor 9 (TLR-9) and activate the host immune response and the production of several inflammatory mediators against these pathogens (10, 11).
Eicosanoids are lipid mediators of local signaling, derived from arachidonic acid (AA), which participate in homeostatic and inflammatory processes (12, 13). Their production occurs when AA fuels enter three main metabolic pathways: the cyclooxygenases (COX-1 and COX-2) pathway that produces prostaglandins (PGs) and thromboxanes (TXs); the lipoxygenases (5-LO, 12-LO, and 15-LO) pathway producing the leukotrienes (LTs), lipoxins (LPs), hepoxylines and hydroxyethylacetaenoic acids (HETEs); and the cytochrome P450 (CYP450) pathway that produces epoxyleicatriatrienoic acids (EETs), dihydroxyyeatriatrienoic acids (DiHETEs), and HETEs (14). The production of eicosanoids has already been documented in patients infected with P. falciparum, and studies suggest that low levels of plasma PGE2 are associated with severe disease (15). Moreover, high concentrations of LTB4 in mice infected with Plasmodium berghei were detrimental to the pathogenesis of cerebral malaria (16).
Previous studies have shown alterations in phospholipase A2 (PLA2) activity, which is involved in the glycerophospholipid metabolism, during P. vivax malaria episodes in human and in erythrocytes infected with P. falciparum (17). More recently, alterations in glycerophospholipid and glycosphingolipid metabolism was also observed in P. vivax malaria (18). Furthermore, metabolomic data suggest that the host response to P. vivax infection might be affected by metabolites involved in the degradation of heme and metabolism of several lipids, including oleic acid and omega-carboxytrinor-leukotriene B4 (19).
The Amazon region comprises the largest and most biodiverse part of the world's tropical forests. The Amazon region comprises the largest and most biodiverse part of the world's tropical forest. It is an endemic area for tropical and infectious diseases with similar clinical spectrum and severity, such fever, headache, and myalgia, favoring the maintenance of the self-medicate culture (20, 21). Because of this, self-medication is a common practice in Brazil and involves the use of medicinal plants and medicines without a prescription. The aim of this research was to describe the profile of lipid mediators and its possible influence on the production of cytokines and chemokines in patients with P. vivax malaria (Pv-malaria), both in the phase acute and convalescent stage. The data from this research suggest that self-medication influences the production of eicosanoids in patients infected with P. vivax and results in a greater interaction between the studied inflammatory mediators.
Materials and Methods
Ethics Statement
The study was approved by the Research Ethics Committee at Fundação de Medicina Tropical Dr. Heitor Vieira Dourado (CEP/FMT-HVD process n°.1.358.078/15). All participants read and signed the written informed consent form, in accordance with the Declaration of Helsinki and Resolution 466/12 of the National Health Board for research involving human subjects.
Healthy Individuals and Patients
Twenty-three patients were included in this study. They were diagnosed with non-severe acute malaria caused by P. vivax via a thick blood smear and the diagnosis was confirmed by the 18S rRNA gene using the 7500 Fast qPCR System (Applied Biosystems, Foster, CA, USA) as described previously (22–24) at FMT-HVD, which is located in the city of Manaus, Western Brazilian Amazon. Of these 23, 14 patients reported self-medication with anti-inflammatory drugs prior to diagnosis (SM), and 9 patients stated that they did not take any type of medication until the time of confirmation of the disease (NSM). 12 patients returned to the hospital 28 days (D28) after admission. This period is known as the convalescent stage after-treatment (AT) and is characterized when the individual is not sick, but has not yet fully recovered. This is known as the transition phase between disease and total cure. A total of 12 individuals were selected for the control group, and comprised of healthy donors who lived in the same city and had no previous history of malaria. Exclusion criteria included: patients under 18 years of age, pregnant women, people belonging to the indigenous population, patients with co-infected or already receiving treatment for malaria. All study participants underwent serological screening recommended by all Brazilian blood banks and this was carried out at the Fundação Hospitalar de Hematologia e Hemoterapia do Amazonas (HEMOAM) serology laboratory for the purpose of exclusion. The clinical and demographic data were acquired using a standardized questionnaire.
Collection, Processing and Transport of Biological Samples
Whole blood samples from healthy subjects and patients were collected in tubes containing EDTA (BD Vacutainer® EDTA K2 Franklin Lakes, New Jersey, USA) and tubes containing Sodium Heparin (Labor Import® São Paulo, Brazil). Samples were then stored in isolated boxes for biological material and transported to the laboratory, where samples collected with EDTA were centrifuged in a refrigerated centrifuge (5702R, Eppendorf, Hamburg, DEU) for 5 min at 1,900 g. The obtained plasma was separated and placed in cryotubes and stored in a freezer at −80°C for further quantification of cytokines and chemokines.
Tubes containing sodium heparin were stimulated with 20 μM Thapsigargine (SIGMA-ALDRICH Life Science) for 20 min in a water bath at 37°C under agitation. After this period, the reaction was blocked in an ice bath, and the stimulated whole blood was centrifuged at 400 g for 20 min at 4°C. The acquired plasma (300 μL) was aliquoted into cryotubes and immediately frozen at −80°C (25). These frozen aliquots were transported on dry ice to the Laboratory of Inflammation and Immunology of Parasitoses (LIIP), located at the School of Pharmaceutical Sciences of Ribeirão Preto (FCFRP-USP) and later submitted to high performance liquid chromatography-mass spectrometry (HPLC-MS/MS).
All samples were stored and discarded after the experiments, obeying the norms of Good Clinical and Laboratorial Practices (GCLP).
Preparation of Plasma Samples for HPLC-MS/MS
The extraction of the eicosanoids in the plasma samples was performed by the solid phase extraction (SPE) method. In summary, the 12-epi-LTB4-d4 analyte acting as internal standard (PI) was added to the plasma samples, then these samples were denatured overnight with 1.5 mL of an ice-cold methanol/acetonitrile (1–1, v-v). The denatured proteins were removed by centrifugation at 400 g for 20 min at 4°C and the supernatants obtained (~1.2 ml) were diluted in 14 ml of ultra-pure water to decrease the methanol/acetonitrile content to 15% between organic phase and aqueous phase. Purification of the eicosanoids was performed by a Waters® Extraction Manifold and Sep-Pak C18 cartridges, 500 mg sorbent, 2.8 ml, HyperSep™ (Thermo Scientific, USA). Samples were transferred to pre-activated cartridges with 2 mL of methanol and 2 mL of 0.1% (v-v) water/acetic acid solution. After elution of the plasma samples, 2 mL of water −0.1% acetic acid (v-v) was added to the cartridge to elute hydrophilic polar compounds and, finally, the eicosanoids adhered on C18 silica were eluted with 2 mL of methanol/0.1% acetic acid (v-v) solution. The solvent was removed under reduced pressure at speedvac, and 100 μL of methanol was added to the extract obtained and transferred to HPLC-MS/MS (25).
Qualitative and Quantitative Analysis of Eicosanoids by HPLC-MS/MS
For this type of analysis, an Acquity™ UPLC (Waters®) system was used consisting of a quaternary pump and automatic injector coupled to the Xevo TQ-S mass spectrometer with ESI ionization source equipped with Orthogonal Z-spray (Waters®). The chromatographic analyses were performed on an Ascentis EXPRESS C18 column (100 × 4.6 mm, 2.7 μm) using a flow rate of 0.6 mL/min at 25°C. Elution was done using a binary gradient system consisting of water: acetonitrile: acetic acid (69.98–30-0.02, v-v-v) (Step A) and acetonitrile: isopropanol (70–30. v-v) (Stage B) with 0% B from 0 to 2 min, increasing to 15% B at 2 min, 20% B at 5 min, 35% B at 8 min, 40% B at 11 min, 100% B at 15 min and remained in this proportion up to 19 min. From this time up to 30 min, the gradient returned to the initial ratio for column conditioning. The ionization source operated in negative mode and by Multiple Reaction Monitoring (MRM). To optimize the MRM conditions, a standard solution of each eicosanoid at 100 ng/mL diluted in methanol/water/ammonium acetate (71-30-0.1, v-v-v) was infused into the MS at 10 μL/min and fragmented by dissociation induced by collision with argon in order to obtain the spectrum of the ion product of each analyte. After selection of the MRM transitions, the cone and collision energies were standardized through the direct infusion of the standards to obtain adequate sensitivity. The linearity was evaluated through a calibration curve, in triplicate, of plasma samples enriched with standard solutions of LTB4, 6-trans-LTB4, LTD4, 11-trans-LTD4, LTE4, PGD2, PGE2, 20-OH- PGE2, 15-keto-PGE2, 15-deoxy-Δ12,14-PGJ2, PGJ2,5-HETE, 12-HETE, 15-HETE, 20-HETE, (+) 11,12-DHET, 15-DHET, 5-Oxo-ETE, 11,12-EET, 5 (S), 6 (R) -DiHETE, LXA4, and TXB2 (Cayman Chemical) and extracted by the method described in the above item, of 5.0; 7.5; 15.0; 30.0; 50.0, and 75.0 ng/ml. A linearity curve was also constructed for LTB4, 5-HETE, 12-HETE, and TXB2 (Cayman Chemical) contemplating the following concentrations: 75.0; 150.0; 300.0; 450.0; 800.0, and 1000.0 ng/ml. The calibration graph was constructed by plotting the plasma concentration values of each eicosanoid on the abscissa axis and the ratio between the area of the peaks obtained for each mediator and the area of the PI on the axis of the ordinates. Data analysis was done via linear regression using the least squares method, where the area/concentration relation is expressed by the equation of the line (y = ax + b), where is the angular coefficient and b is the linear coefficient of the line. The correlation coefficient (r) was also calculated from the experimental points, which is the parameter that estimates the linearity of the calibration curve. Masslynx 4.1 software (Micromass, Manchester, UK) was used for data acquisition and processing.
Quantification of Plasma Concentrations of Circulating Cytokines and Chemokines
The concentrations of chemokines (CXCL-8, CCL-2, CCL-5, CXCL-9, and CXCL-10) and circulating cytokines (IL-2, IL-4, IL-6, IL-10. TNF, IFN-γ, and IL-17A) through the CBA (Cytometric Bead Array) BD™ Human Chemokine Kit and CBA (Cytometric Bead Array) BD™ Human Th1, Th2, Th17 Cytokine kit, respectively. IL-1β and IL-5 cytokines were quantified by ELISA (BD OptEIA II Human Kit, BD Biosciences Pharmingen, USA), following the manufacturer's instructions.
Statistical Analyses
Clinical, epidemiological, laboratory, lipid mediator, chemokine, and cytokine concentrations were tabulated and stored using Microsoft Excel® Software (2010 version for Windows). The descriptive analyses were presented in tables and graphs of frequency distribution, elaborated in GraphPad Prism 5.0 software (San Diego, CA, USA), taking into account that the data have a non-parametric distribution. The comparative analyses between the groups were performed using the Mann-Whitney (NSM vs. HD, SM vs. HD, NSM vs. SM) and Wilcoxon test (NSM and SM vs. AT), with significant values being set at p < 0.05. From analysis of the Spearman correlation between the biomarkers, the networks analysis and demonstration of the complex interactions between chemokine, cytokines, and lipid mediators were performed in all groups of this study using Cytoscape software 3.0.4 (Cytoscape Consortium San Diego, CA, USA). The thickness of the lines was adjusted to portray the strength and whether the type of correlations was positive or negative.
Results
Demographic, Parasitological, and Baseline Clinical Characteristics of the Study Population
All subjects in this study reside in an area of intense malaria transmission. Although they are in contact with P. vivax malaria (Pv-malaria) transmission vectors, 28% of the recruited individuals were primo-infected. The median age between the control and Pv-malaria groups was similar (33 and 39 years [p = 0.144], respectively), with male subjects predominating (58 and 74% [p = 0.450], respectively). The average parasite load was 127,574 parasites/μL, and was similar among patients who did not Self-Medicate (NSM) and those who Self-Medicated (SM) (p = 0.361). Furthermore, median of the number of infections was 2 episodes, without any difference between the Pv-malaria subgroups (p = 0.303). Table 1 summarizes the demographic, parasitological, and clinical characteristics.
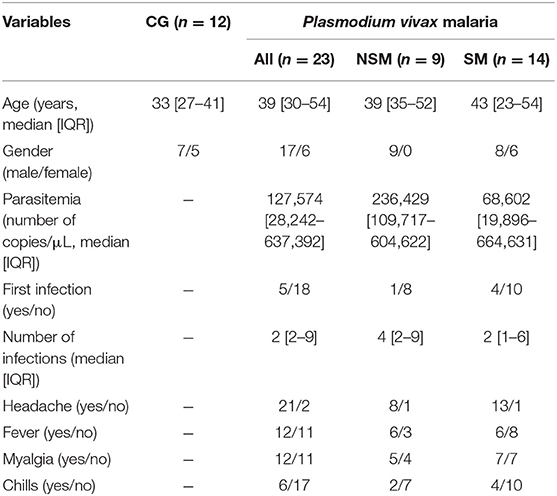
Table 1. Demographic, parasitological, and baseline clinical characteristics of Control Group (CG) and Pv-malaria patients that did Not Self-Medicate (NSM) and Self-Medicated (SM).
Hematological and Biochemical Parameters of Pv-Malaria Patients That Did Not Self-Medicate (NSM) and Self-Medicated (SM)
Table 2 summarizes the hematological and biochemical parameters which were evaluated in the subgroups of Pv-malaria and control group (CG). No significant differences were observed among patients than did not Self-Medicate (NSM) and Self-Medicated (SM), except for differences in hematocrit and uric acid concentrations. Furthermore, the CG had significantly higher/lower concentrations and numbers of hematocrit, red cell distribution width, white blood cells, eosinophil, lymphocyte, monocyte, mean platelet volume, platelet, total cholesterol, HDL, LDL, uric acid and total, direct and indirect bilirubin when compared to NSM and SM groups.
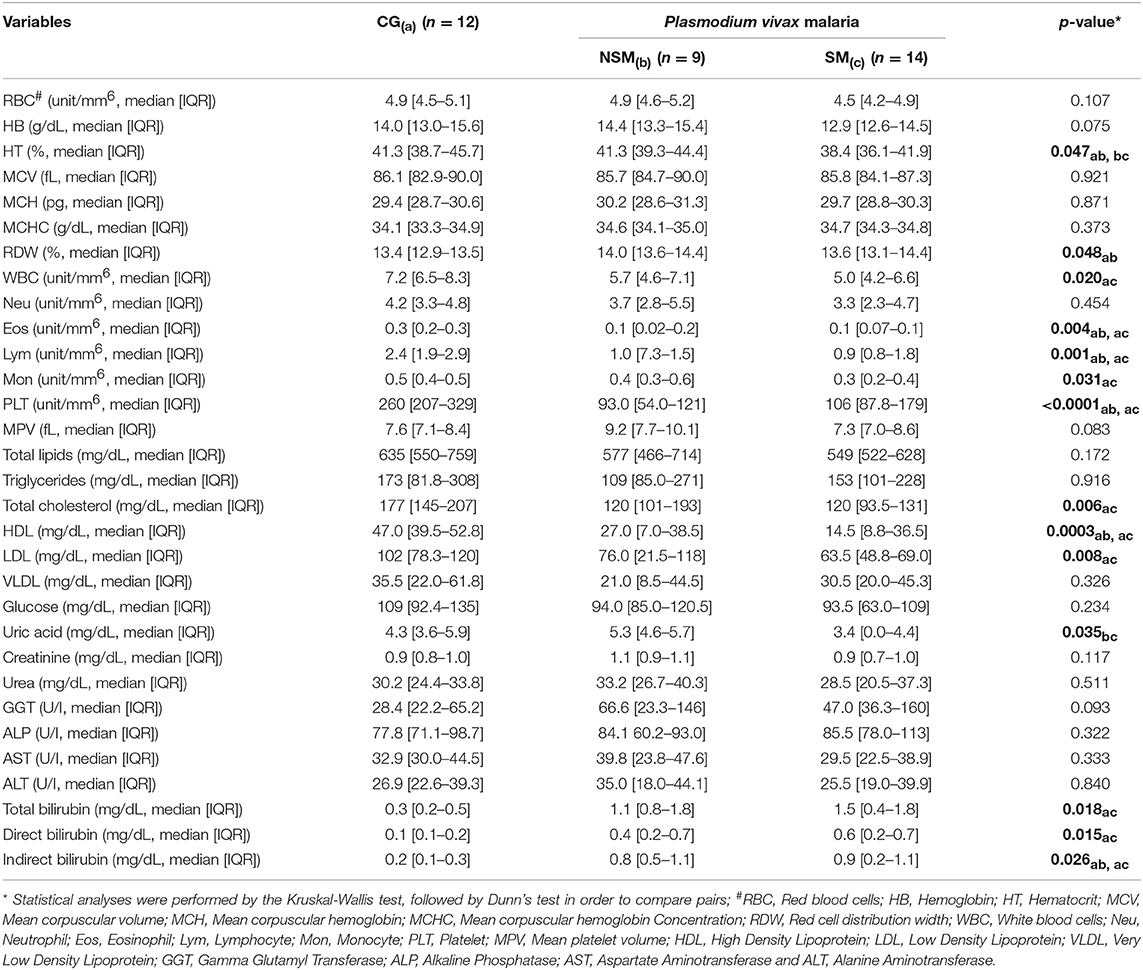
Table 2. Hematological and Biochemical parameters of Control Group (CG) and Pv-malaria patients that did not Self-Medicate (NSM) and Self-Medicated (SM).
Plasmatic Profile of Eicosanoids in P. vivax Malaria
Analysis of the plasmatic profile of eicosanoids after whole blood stimulation indicated that P. vivax infection may influence the serum concentrations of these biomolecules (Figure 1). The evaluation of these lipid mediators demonstrated a significant decrease of LTE4, PGE2, 5-HETE, 12-HETE, 5-Oxo-ETE, and TXB2 (Figures 1C,F,I,J,L,M) in patients with Pv-malaria. In addition, increased levels of LTD4, PGD2, 15-keto-PGE2, 20-OH-PGE2, and 15-HETE were observed in these individuals (Figures 1B,E,G,H,K). We observed significantly lower concentrations of LTB4, 6-trans-LTB4, and 5-HETE when comparing the prior (Pv-malaria) and convalescent stage after-treatment (AT) patients (Figures 1A,D,I), with the increase of 12-HETE and TXB2 (Figures 1J,M).
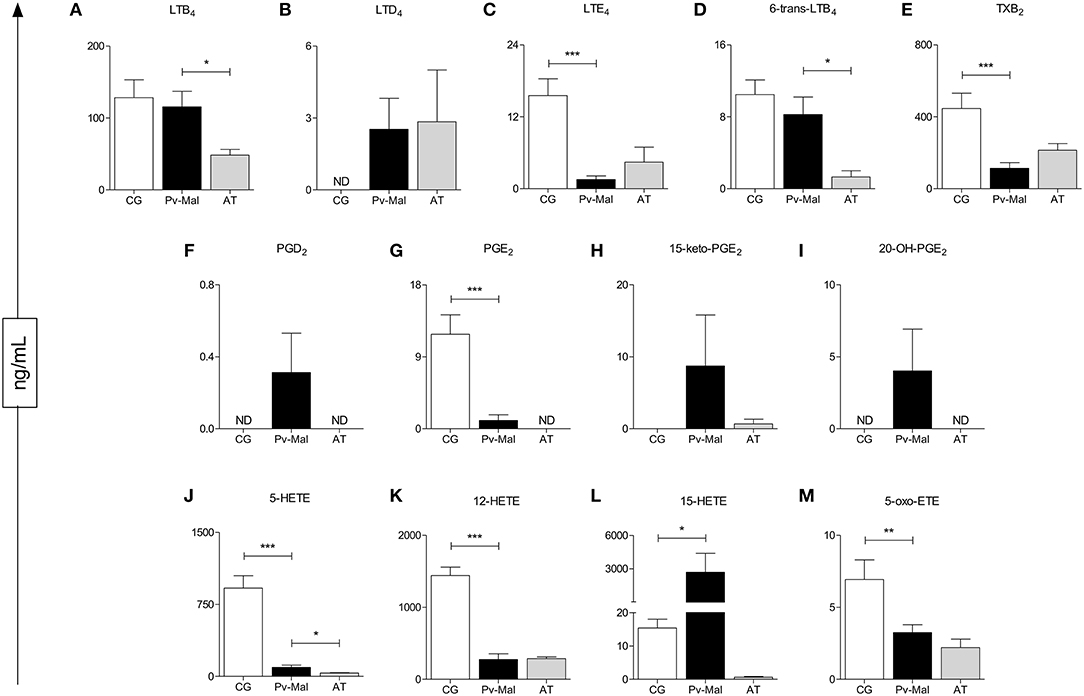
Figure 1. Identification and quantification of eicosanoids after stimulation with Thapsigargine, from control group (white bar), Pv-malaria patients (black bar) and patients who returned 28 days after treatment, understood as the period of convalescence (gray bar). *p < 0.05, **p < 0.01, ***p < 0.0001 between groups studied. ND = Concentration not detected. The quantifications of these lipid mediators were measured using high performance liquid chromatography-mass spectrometry (HPLC-MS).
Influence of Self-Medication on the Circulating Concentration of Eicosanoids in Pv-Malaria
To understand the influence of self-medication on eicosanoid levels in Pv-malaria, we separated the patients into two groups: No Self-Medication (NSM) and Self-Medication (SM) Pv-malaria patients (Figure 2). A significant reduction of LTB4, LTE4, 6-trans-LTB4, 5-HETE, and 5-Oxo-ETE (Figures 2A,C,D,I,L) was observed in SM Pv-malaria patients. In addition, the eicosanoids LTD4, PGD2, PGE2, 20-OH-PGE2, and 15-HETE (Figures 2B,E,F,H,K) were not detected in these individuals. 12-HETE and TXB2 were not significantly different between groups (Figures 2J,M).
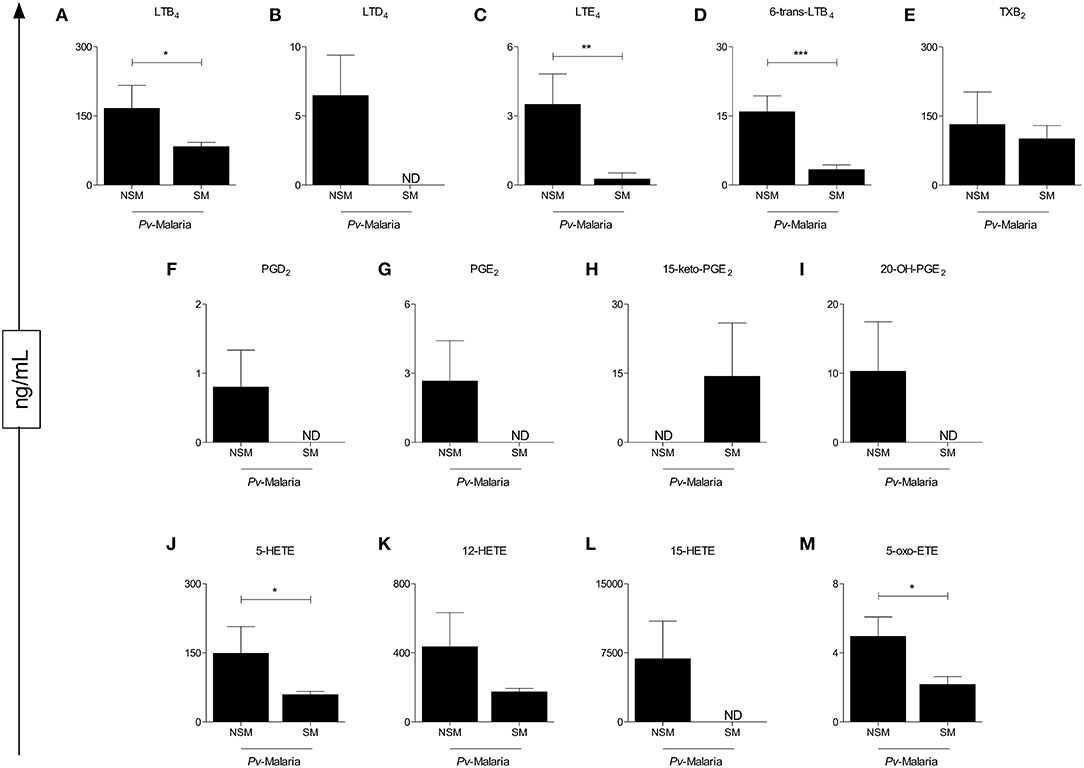
Figure 2. Analysis of the eicosanoid concentrations of Pv-malaria patients at the time of diagnosis who did not Self-Medicate (NSM) and Self-Medicated (SM). *p < 0.05, **p < 0.01, ***p < 0.0001 between groups studied. ND = Concentration not detected. The quantifications of these lipid mediators were measured using high performance liquid chromatography-mass spectrometry (HPLC-MS).
Plasmatic Profile of Chemokines and Cytokines in Pv-Malaria and the Influence of Self-Medication in These Patients
Our data demonstrated that patients with P. vivax malaria presented chemokine and cytokine storms during infection (Figure 3). A significant increase of CXCL-8, CCL-2, CXCL-9, CXCL-10. IL-6, IL-10. IFN-γ, IL-17A, and IL-5 (Figures 3A–C,E,F,I,K,L,N) were observed in these patients. Although this phenomenon is observed at the end of the convalescence stage after treatment, the circulating levels reestablish themselves and reach the concentrations of the control group (Figure 3). To further characterize these immunological markers, chemokine levels and plasma cytokines were analyzed in NSM and SM patients. It is observed that the use of the drugs positively influenced the production of CCL-2, CCL-5, and IL-5 (Figures 3B,D,N).
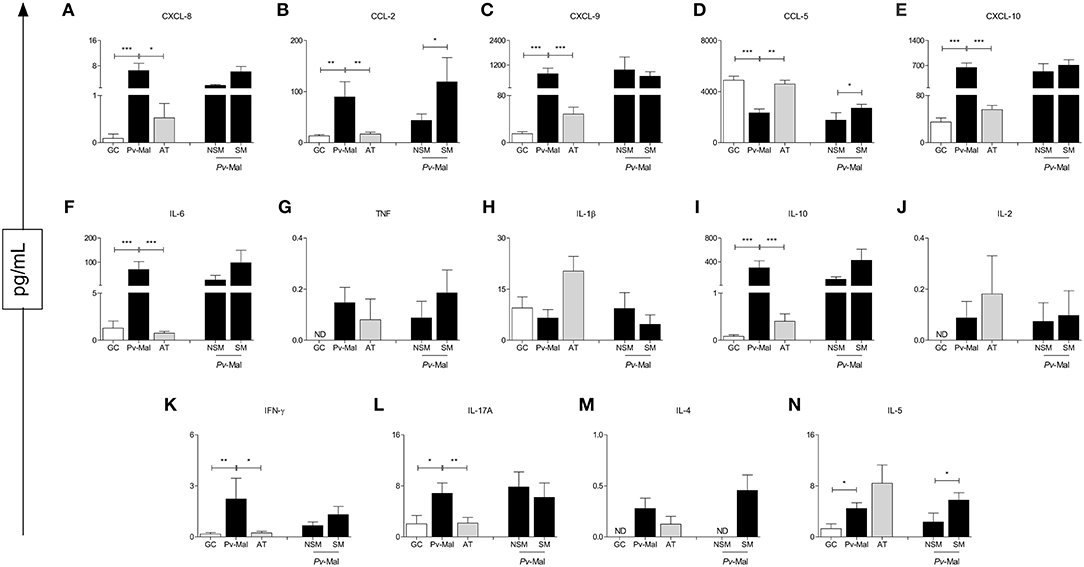
Figure 3. Concentration of chemokines and cytokines in control group (white bar), Pv-malaria patients (black bar) and patients who returned 28 days after treatment, understood as the period of convalescence (gray bar). In addition, analysis of the chemokines and cytokines concentrations of Pv-malaria patients at the time of diagnosis who did not Self-Medicate (NSM) and Self-Medicated (SM). *p < 0.05, **p < 0.01, ***p < 0.0001 between groups studied. ND = Concentration not detected. The quantifications of these chemokines and cytokines were measured using Cytometric Bead Array (CBA) and ELISA.
Plasmodium vivax Malaria and Self-Medication (SM) Change the Interaction of Eicosanoids, Chemokines, and Cytokines During Infection
To evaluate the relationship between levels of eicosanoids, chemokines, and cytokines during P. vivax infection, a series of correlation analyses were performed (Figure 4). When comparing the analyzed interactions between molecules in the Pv-malaria and control group, there was an intense increase in relations, especially the chemokine (CXCL-8, CCL-2 and CXCL-10) and cytokines (IL-6, IL- 10. IFN-γ, IL-4, and IL-5) (Figure 4B). In addition, the number of interactions between the analyzed eicosanoids also increased during P. vivax infection, but this was not observed in the control group (Figure 4A). This phenomenon remains even in the convalescent stage after-treatment (Figure 4C). Finally, we performed the analysis of the correlation indices by categorizing patients with Pv-malaria in two different groups (NSM and SM) and observed that the interactions were influenced in individuals who self-medicated. Decreased eicosanoid ratios and increased correlations with chemokines and cytokines were noted (Figure 4E).
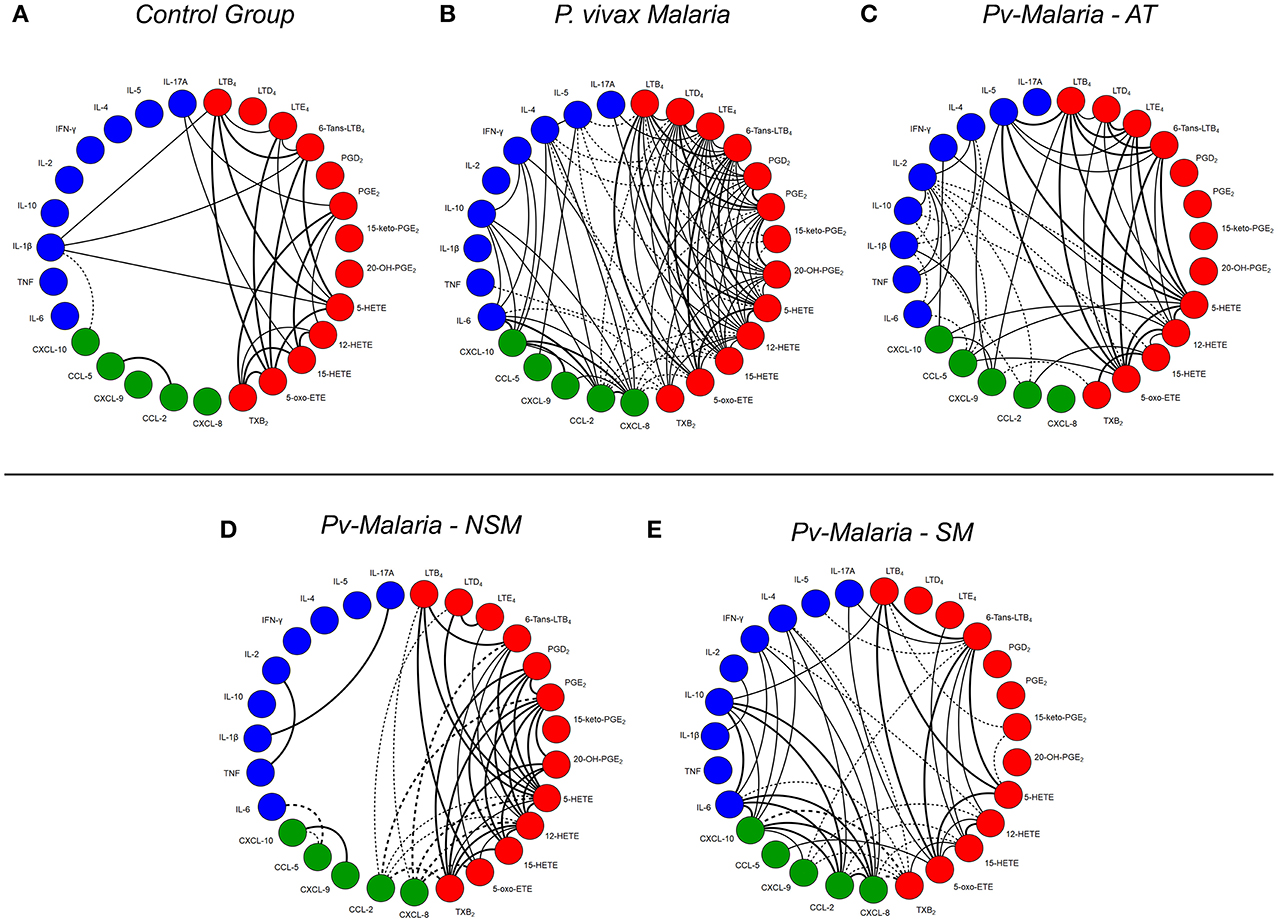
Figure 4. A network of interaction between eicosanoids, chemokines and cytokines in control group, Pv-malaria patients, individuals who returned 28 days after treatment, understood as the period of convalescence and Pv-malaria patients at the time of diagnosis who did not Self-Medication (NSM) and Self-Medicated (SM). Each connecting line is a significant correlation between a pair of markers. Continuous lines represent positive correlations, while dashed lines represent negative correlations (p < 0.05). The degree of significance is represented by the thickness of the line. Correlations were obtained through the Spearman test; the value of r and p were used to classify the connections as weak (r ≤ 0.35, p < 0.05), moderate (r = 0.36–0.67, p < 0.01) or strong (r ≥ 0.68, p < 0.0001). The absence of the line indicates the non-existence of the relationship.
Discussion
In this study, we characterize the eicosanoid profile of patients with Pv-malaria during the acute phase and convalescent stage after-treatment. Moreover, we report the influence of self-medication on this profile. These findings are particularly relevant in order to better understand the interaction between inflammatory and lipid mediators during the cure of malaria-vivax infection. We demonstrate that Pv-malaria infection significantly affects the levels of metabolites derived from LO or COX pathways. Levels of these lipid mediators are tightly correlated with plasma levels of cytokines and chemokines that are related to clinical characteristics of the patients. Moreover, our data suggest that self-medication (SM) contributes to changes in the correlations between eicosanoids, chemokines, and cytokines during infection, which may aid in parasitic clearance and/or symptom alleviation. To our knowledge, this is the first study to comprehensively profile eicosanoid production and their associations with cytokines and chemokines during infections with P. vivax.
Plasmodium vivax infection induces inflammatory and regulatory processes that are designed to contain the parasite multiplication, while maintaining the integrity of tissues and organs (26–28). Although most patients with P. vivax malaria exhibit mild clinical symptoms, severe cases are reported and have been associated with elevated levels of TNF-α, IFN-γ and low levels of PGs, TXBs, and IL-10 (29–33). Our data further extend these findings by demonstrating reduced a production of PGE2 even during mild cases of P. vivax infection. PGE2 suppresses type I interferon responses, while P. vivax infection promotes robust transcriptional interferon signatures, which are associated with linoleate metabolism (34) and a pathway that influences arachidonic acid biosynthesis. Furthermore, TXB2, an inactive product derived from TXA2, was also reduced during P. vivax infection and might reflect the degree of thrombocytopenia in these patients (35). This is further supported because treatment recovered platelet counts, while only levels of TXB2 increased during convalescence.
Treatment down-regulated the lypoxygenase pathways, reducing the production of LTB4, 6-trans-LTB4, 5-HETE, and 12-HETE. Except for the last lipid mediator which is exclusively produced by action of 12-LO, since formation of the other eicosanoids involves reactions catalyzed by 5-LO. All these metabolites were negatively correlated with the Th2 cytokine profiles, IL-4 and/or IL-5. After-treatment changed these correlations, whereby IL-5 became positively correlated with LTB4, 6-trans-LTB4 and 5-HETE. IL-5 signaling has been shown to promote 5-LO activating protein (FLAP) expression and 5-LO activation and nucleus translocation (36). Self-medication reduced the production of these mediators, whose 6-trans-LTB4 was also negatively correlated with IL-5. These data highlight an underlying association between Th2 cytokine profiles and LOX products that can be relevant to the treatment of P. vivax malaria. A recent study has demonstrated that a major product of LTB4 catabolism by neutrophils, omega-carboxy-tinor-LTB4, is significantly associated with P. vivax parasite burden, which suggests that the production of eicosanoids might also be affected by clinical parameters such as the degree of parasitemia (19).
We observed little association between the production of eicosanoids and circulating cytokines in the control group, but, in particular, IL-1β was positively correlated with levels of LTB4, 6-trans-LTB4, and 5-HETE. This is interesting because depending on the context, LTB4 exerts a regulatory role over IL-1β production (37, 38). This suggests that 5-LO activity might also be important in order to limit IL-1β release during homeostatic state, of which levels increased during convalescence, when 5-LO metabolites were also reduced. After P. vivax infection, there were no significant correlations with IL-1β, which did not recover after treatment. However, further sub-classification of patients demonstrated that IL-1β positively correlated with IL-17 during infection of NSM patients but not SM patients, although both groups exhibited similar levels of these cytokines. This is consistent with the role of IL-1β for the differentiation and polarization of naïve T lymphocytes into Th17 cells (39), whereas lack of correlation after self-medication suggests a disruption in this process. Levels of IFN-γ and the related chemokine, CXCL-10. increased after P. vivax infection. IFN-γ did not correlate with lipid mediators during acute infection for all patients, but self-medication changed this profile, showed inverse correlations between IFN-γ and 12-HETE in the interaction network between immunological molecules and lipid mediators (Figure 4). During convalescence, significant correlations were also observed between IFN-γ, CXCL-10, and 12-HETE, suggesting a potential cross-talk between these mediators.
The local population is very well-informed about malaria and knows the need for diagnosing malaria at the onset of the disease. However, the study has limitations since eicosanoids, chemokines, and cytokines were not evaluated at the onset of infection and/or symptoms. Furthermore, the small sample size does not allow intra-comparison of the eicosanoids, chemokines, and cytokines with clinical manifestations of Pv-malaria, including in asymptomatic and severe malaria.
Conclusion
In summary, P. vivax infection triggers production of immune and lipid mediators, generally influencing the increase of these molecules in the acute stage of the disease. Our data also suggest that after antimalarial treatment, the serum levels of these molecules are reestablished. Furthermore, self-medicated individuals had a significant impact on the production of lipid mediators, chemokines and cytokines, altering the interaction dynamics between these molecules in Pv-malaria infection. Finally, we believe that self-medication deserves more attention during disease follow-up, since most of the drugs consumed are exempt from prescription, but aren't exempt from risk.
Data Availability
The raw data supporting the conclusions of this manuscript will be made available by the authors, without undue reservation, to any qualified researcher.
Ethics Statement
All protocols and consent forms were approved by the Research Ethics Committee at the FMT-HVD (CEP/FMT-HVD process #1.358.078/2015). Patients were treated according to recommendations of the Brazilian Health Ministry.
Author Contributions
PA-F, AT, AC, LG, LF, and AM designed and performed the experiments, analyzed data, and wrote the manuscript. PA-F and AC analyzed data. AFGM, TC, FZ, LG, and LM performed the experiments. CS analyzed the data and revised the manuscript. PA-F, WM, ML, LF, and AM conceived and supervised the project, designed the experiments, interpreted the data, wrote and revised the manuscript. All authors read and approved the final manuscript.
Funding
Financial support was provided by grants from Fundação de Amparo à Pesquisa do Estado do Amazonas (FAPEAM), Conselho Nacional de Desenvolvimento Científico e Tecnológico (CNPq) and Coordenação de Aperfeiçoamento de Pessoal de Nível Superior (CAPES). PA-F, FZ, and TC have fellowship from CAPES and FAPEAM (Ph. D. and Masters student). AC has a fellowship from CAPES (PNPD/CAPES program). ML and LF are level 1 research fellows from CNPq. AM and WM are level 2 research fellows from CNPq. The funders had no role in study design, data collection and analysis, decision to publish, or preparation of the manuscript.
Conflict of Interest Statement
The authors declare that the research was conducted in the absence of any commercial or financial relationships that could be construed as a potential conflict of interest.
References
1. Cowman AF, Healer J, Marapana D, Marsh K. Malaria: biology and disease. Cell. (2016) 167:610–24. doi: 10.1016/j.cell.2016.07.055
2. Ministério da Saúde (BR). Secretaria de Vigilância em Saúde. Departamento de Vigilância Epidemiológica. Ações de controle da Malária: manual para profissionais de Saúde na Atenção Básica. Brasília: Ministério da Saúde (Série, A. Normas e manuais técnicos) (2006).
3. Cox-Singh J, Davis TM, Lee KS, Shamsul SS, Matusop A, Ratnam S, et al. Plasmodium knowlesi malaria in humans is widely distributed and potentially life threatening. Clin Infect Dis. (2008) 46:165–71. doi: 10.1086/524888
4. Crawley J, Chu C, Mtove G, Nosten F. Malaria in children. Lancet. (2010) 375:1468–81. doi: 10.1016/S0140–6736(10)60447–3
5. The beast in the mosquito: the correspondence of Ronald Ross and Patrick Manson. Clio Med. (1998) 51:1–528.
7. Ministério da Saúde (BR). Secretaria de vigilância em Saúde. Situação epidemiológica da malária no Brasil, 2012 e 2013. Brasília: Boletim Epidemiológico (2015).
8. Brazilian Ministry of Health. Secretaria de Vigilância em Saúde. Brasília: Boletim Epidemiológico (2017)
9. Sinnis P, Zavala F. The skin: where malaria infection and the host immune response begin. Semin Immunopathol. (2012) 34:787–92. doi: 10.1007/s00281–012-0345–5
10. Good MF, Xu H, Wykes M, Engwerda CR. Development and regulation of cell-mediated immune responses to the blood stages of malaria: implications for vaccine research. Annu Rev Immunol, 23, 69–99 (2005) doi: 10.1146/annurev.immunol.23.021704.115638
11. Parroche P, Lauw FN, Goutagny N, Latz E, Monks BG, Visintin A, et al. Malaria hemozoin is immunologically inert but radically enhances innate responses by presenting malaria DNA to Toll-like receptor 9. Proc Natl Acad Sci USA. (2007) 104:1919–24. doi: 10.1073/pnas.0608745104
12. Funk CD. Prostaglandins and leukotrienes: advances in eicosanoid biology. Science. (2001) 294:1871–5. doi: 10.1126/science.294.5548.1871
13. Buczynski MW, Dumlao DS, Dennis EA. Thematic review series: proteomics. an integrated omics analysis of eicosanoid biology. J Lipid Res. (2009) 50:1015–38. doi: 10.1194/jlr.R900004-JLR200
14. Tam VC. Lipidomic profiling of bioactive lipids by mass spectrometry during microbial infections. Semin Immunol. (2013) 25:240–8. doi: 10.1016/j.smim.2013.08.006
15. Perkins DJ, Kremsner PG, Weinberg JB. Inverse relationship of plasma prostaglandin E2 and blood mononuclear cell cyclooxygenase-2 with disease severity in children with Plasmodium falciparum malaria. J Infect Dis. (2001) 183:113–8. doi: 10.1086/317660
16. Xiao L, Patterson PS, Yang C, Lal AA. Role of eicosanoids in the pathogenesis of murine cerebral malaria. Am J Trop Med Hyg. (1999) 60:668–73. doi: 10.4269/ajtmh.1999.60.668
17. Zidovetzki R, Sherman IW, Cardenas M, Borchardt DB. Chloroquine stabilization of phospholipid membranes against diacylglycerol-induced perturbation. Biochem Pharmacol. (1993) 45:183–9. doi: 10.1016/0006–2952(93)90391–9
18. Uppal K, Salinas JL, Monteiro WM, Val F, Cordy RJ, Liu K, et al. Plasma metabolomics reveals membrane lipids, aspartate/asparagine and nucleotide metabolism pathway differences associated with chloroquine resistance in Plasmodium vivax malaria. PLoS ONE. (2017) 12:e0182819. doi: 10.1371/journal.pone.0182819
19. Gardinassi LG, Cordy RJ, Lacerda MV G, Salinas JL, Monteiro WM, Melo GC, et al. Metabolome-wide association study of peripheral parasitemia in Plasmodium vivax malaria. Int J Med Microbiol. (2017) 307:533–41. doi: 10.1016/j.ijmm.2017.09.002
20. Valdir Veiga. Study of the medicinal plants consumption in the Middle-North Region of the Rio de Janeiro State: acceptance by health professionals, way of use of the population. Brazil J Pharmacog. (2008) 18:308–13. doi: 10.1590/S0102-695X2008000200027
21. Arrais PS, Fernandes ME, Pizzol TD, Ramos LR, Mengue SS, Luiza VL, et al. Prevalence of self-medication in Brazil and associated factors. Rev Saude Publica. (2016) 50(Suppl. 2):13. doi: 10.1590/s1518–8787.2016050006117
22. Rosanas-Urgell A, Mueller D, Betuela I, Barnadas C, Iga J, Zimmerman PA, et al. Comparison of diagnostic methods for the detection and quantification of the four sympatric Plasmodium species in field samples from Papua New Guinea. Malar J. (2010) 9:361. doi: 10.1186/1475–2875-9–361
23. Wampfler R, Mwingira F, Javati S, Robinson L, Betuela I, Siba P, et al. Strategies for detection of Plasmodium species gametocytes. PLoS ONE. (2013) 8:e76316. doi: 10.1371/journal.pone.0076316
24. Costa AG, Ramasawmy R, Ibiapina HN S, Sampaio VS, Xábregas LA, Brasil LW, et al. Association of TLR variants with susceptibility to Plasmodium vivax malaria and parasitemia in the Amazon region of Brazil. PLoS ONE. (2017) 12:e0183840. doi: 10.1371/journal.pone.0183840
25. Galvão AF, Petta T, Flamand N, Bollela VR, Silva CL, Jarduli LR, et al. Plasma eicosanoid profiles determined by high-performance liquid chromatography coupled with tandem mass spectrometry in stimulated peripheral blood from healthy individuals and sickle cell anemia patients in treatment. Anal Bioanal Chem. (2016) 408:3613–23. doi: 10.1007/s00216–016-9445–8
26. da Costa AG, Antonelli LR, Costa PA, Pimentel JP, Garcia NP, Tarragô AM, et al. The robust and modulated biomarker network elicited by the Plasmodium vivax infection is mainly mediated by the IL-6/IL-10 axis and is associated with the parasite load. J Immunol Res. (2014) 2014:318250. doi: 10.1155/2014/318250
27. Costa AG, Ramasawmy R, Val FFA, Ibiapina HNS, Oliveira AC, Tarragô AM, et al. Polymorphisms in TLRs influence circulating cytokines production in Plasmodium vivax malaria: TLR polymorphisms influence cytokine productions in malaria-vivax. Cytokine. (2018) 110:374–80. doi: 10.1016/j.cyto.2018.04.008
28. Mendonça VR, Queiroz AT, Lopes FM, Andrade BB, Barral-Netto M. Networking the host immune response in Plasmodium vivax malaria. Malar J. (2013) 12l:69. doi: 10.1186/1475–2875-12–69
29. Anyona SB, Kempaiah P, Raballah E, Davenport GC, Were T, Konah SN, et al. Reduced systemic bicyclo-prostaglandin-E2 and cyclooxygenase-2 gene expression are associated with inefficient erythropoiesis and enhanced uptake of monocytic hemozoin in children with severe malarial anemia. Am J Hematol. (2012) 87:782–9. doi: 10.1002/ajh.23253
30. Wilson NO, Jain V, Roberts CE, Lucchi N, Joel PK, Singh MP, et al. CXCL4 and CXCL10 predict risk of fatal cerebral malaria. Dis Markers. (2011) 30:39–49. doi: 10.1155/2011/828256
31. Andrade BB, Reis-Filho A, Souza-Neto SM, Clarêncio J, Camargo LM, Barral A, et al. Severe Plasmodium vivax malaria exhibits marked inflammatory imbalance. Malar J. (2010) 9:13. doi: 10.1186/1475–2875-9–13
32. Daniel-Ribeiro CT, Lacerda MV, Oliveira-Ferreira J. [Plasmodium vivax malaria in the Brazilian Amazon, some aspects of its epidemiology, clinical spectrum and naturally induced immune responses]. Bull Soc Pathol Exot. (2008) 101:243–8.
33. Obata T, Nakano Y, Looareesuwan S, Ohtomo H, Aikawa M. Prostaglandin spectrum in falciparum malaria patients. Int Congress Series. (2002) 1233:475–8. doi: 10.1016/S0531–5131(02)00244–3
34. Gardinassi LG, Arévalo-Herrera M, Herrera S, Cordy RJ, Tran V, Smith MR, et al. Integrative metabolomics and transcriptomics signatures of clinical tolerance to Plasmodium vivax reveal activation of innate cell immunity and T cell signaling. Redox Biol. (2018) 17:158–70. doi: 10.1016/j.redox.2018.04.011
35. Coelho HC, Lopes SC, Pimentel JP, Nogueira PA, Costa FT, Siqueira AM, et al. Thrombocytopenia in Plasmodium vivax malaria is related to platelets phagocytosis. PLoS ONE. (2013) 8:e63410. doi: 10.1371/journal.pone.0063410
36. Cowburn AS, Holgate ST, Sampson AP. IL-5 increases expression of 5-lipoxygenase-activating protein and translocates 5-lipoxygenase to the nucleus in human blood eosinophils. J Immunol. (1999) 163:456–65.
37. Zoccal KF, Gardinassi LG, Sorgi CA, Meirelles AF G, Bordon KC F, Glezer I, et al. CD36 shunts eicosanoid metabolism to repress CD14 licensed interleukin-1β release and inflammation. Front Immunol. (2018) 9:890. doi: 10.3389/fimmu.2018.00890
38. Zoccal KF, Ferreira GZ, Prado MKB, Gardinassi LG, Sampaio SV, Faccioli LH. LTB4and PGE2 modulate the release of MIP-1α and IL-1β by cells stimulated with Bothrops snake venoms. Toxicon. (2018) 150:289–96. doi: 10.1016/j.toxicon.2018.06.066
Keywords: eicosanoids, Plasmodium vivax, inflammatory mediators, cytokines, self-medication
Citation: Abreu-Filho PG, Tarragô AM, Costa AG, Monteiro WM, Meielles AFG, Costa TCC, Silva JS, Zambuzi FA, Gardinassi LG, Moraes LAB, Lacerda MVG, Sorgi CA, Faccioli LH and Malheiro A (2019) Plasma Eicosanoid Profile in Plasmodium vivax Malaria: Clinical Analysis and Impacts of Self-Medication. Front. Immunol. 10:2141. doi: 10.3389/fimmu.2019.02141
Received: 12 February 2019; Accepted: 27 August 2019;
Published: 18 September 2019.
Edited by:
Wanderley De Souza, Federal University of Rio de Janeiro, BrazilReviewed by:
Sharvan Sehrawat, Indian Institute of Science Education and Research Mohali, IndiaPatricia Talamás-Rohana, Center for Research and Advanced Studies (CINVESTAV), Mexico
Copyright © 2019 Abreu-Filho, Tarragô, Costa, Monteiro, Meielles, Costa, Silva, Zambuzi, Gardinassi, Moraes, Lacerda, Sorgi, Faccioli and Malheiro. This is an open-access article distributed under the terms of the Creative Commons Attribution License (CC BY). The use, distribution or reproduction in other forums is permitted, provided the original author(s) and the copyright owner(s) are credited and that the original publication in this journal is cited, in accordance with accepted academic practice. No use, distribution or reproduction is permitted which does not comply with these terms.
*Correspondence: Adriana Malheiro, malheiroadriana@yahoo.com.br