- 1Clinical Science & Immunology Program, H. Lee Moffitt Cancer Center, Tampa, FL, United States
- 2Department of Breast Oncology, Tianjin Medical University Cancer Institute & Hospital, National Clinical Research Center for Cancer, Tianjin, China
- 3Perelman School of Medicine, University of Pennsylvania, Philadelphia, PA, United States
- 4Department of Breast Oncology, H. Lee Moffitt Cancer Center, Tampa, FL, United States
Patients with metastatic HER2 breast cancer (MBC) often become resistant to HER 2 targeted therapy and have recurrence of disease. The Panacea trial suggested that HER2 MBC patients were more likely to respond to checkpoint therapy if TIL were present or if tumor expressed PD-L1. We assessed whether type I polarized dendritic cells (DC1) could improve checkpoint therapy in a preclinical model of HER2+ breast cancer. TUBO bearing mice were vaccinated with either MHC class I or class II HER2 peptide pulsed DC1 (class I or class II HER2-DC1) concurrently or sequentially with administration of anti-PD-1 or anti-PDL1. Infiltration of tumors by immune cells, induction of anti-HER2 immunity and response to therapy was evaluated. Class I or class II HER2-DC1 vaccinated mice generated anti-HER2 CD8 or CD4+ T cell immune responses and demonstrated delayed tumor growth. Combining both MHC class I and II HER2-pulsed DC1 did not further result in inhibition of tumor growth or enhanced survival compared to individual administration. Interestingly class II HER2-DC1 led to both increased CD4 and CD8 T cells in the tumor microenvironment while class I peptides typically resulted in only increased CD8 T cells. Anti-PD-1 but not anti-PD-L1 administered sequentially with class I or class II HER2-DC1 vaccine could improve the efficacy of HER2-DC1 vaccine as measured by tumor growth, survival, infiltration of tumors by T cells and increase in systemic anti-HER2 immune responses. Depletion of CD4+ T cells abrogated the anti-tumor efficacy of combination therapy with class II HER2-DC1 and anti-PD-1, suggesting that tumor regression was CD4 dependent. Since class II HER2-DC1 was as effective as class I, we combined class II HER2-DC1 vaccine with anti-rat neu antibodies and anti-PD-1 therapy. Combination therapy demonstrated further delay in tumor growth, and enhanced survival compared to control mice. In summary, Class II HER2-DC1 drives both a CD4 and CD8 T cell tumor infiltration that leads to increased survival, and in combination with anti-HER2 therapy and checkpoint blockade can improve survival in preclinical models of HER2 positive breast cancer and warrants exploration in patients with HER2 MBC.
Introduction
Breast cancer is the most commonly diagnosed tumor and a major cause of cancer death among women (1). A subset of breast cancers present expression/amplification of the HER2 protein/oncogene which correlates with increased recurrence rates and poor survival (2–4). HER2-targeted treatments have led to meaningful improvement in clinical outcomes for patients with breast tumors driven by HER2. For example, the HER2-targeted antibodies, trastuzumab and pertuzumab, combined with docetaxel improved the median overall survival (mOS) of patients with HER2-positive (HER2+) metastatic breast cancer (MBC) to 56.5 months compared to 20.3 months for patients receiving chemotherapy alone (5, 6). In the second line setting, treatment with the antibody drug conjugate ado-trastuzumab (T-DM1) improved the mOS in patients with trastuzumab-resistant HER2+ MBC from 15.9–25.9 to 22.7–29.9 months when compared to chemotherapy or to treatment with small tyrosine kinase inhibitor (lapatinib) (7). Taken together these data support the clinical validity of the HER2 antigen as a valid predictive biomarker of clinical benefit for treatment with HER2-targeted therapies even after disease progression with approved targeted agents. Notwithstanding the recent advances, HER2+ MBC will eventually acquire resistance to HER2-targeted therapies and disease progression will ensue. Therefore, alternative or other combinatorial approaches are needed to overcome resistance to HER2-targeted treatment and improve clinical outcomes.
The presence of tumor infiltrating lymphocyte (TIL) in HER2+ breast cancer is consistently associated with improved prognosis and better survival (8–10). Trastuzumab treatment of breast cancer patients with the presence of TIL have improved survival and complete response to neoadjuvant therapy (9–12). HER2 antibody treatment has been reported in preclinical studies to induce adaptive and innate immune responses and to increase infiltration of immune cells into the tumor microenvironment (13).
Several co-inhibitory immune checkpoint signals such as programmed death 1 (PD-1) receptor/PD-ligand 1 (PD-L1) have been shown to inhibit anti-tumor immune responses (14, 15). Binding of PD-L1 to its receptor PD-1 on the surface of T cells can induce TIL exhaustion and evade anti-tumor immunity (16). Preclinical studies combining antibodies against PD-1 improved the immune-mediated effects of anti-HER2 monoclonal antibody therapy (17). These data provide a strong rationale for the use of immune checkpoint inhibitors as a combinatorial approach in HER2+ breast cancer. The phase 1b/2 KEYNOTE-014/PANACEA trial evaluated the efficacy of pembrolizumab (anti-PD-1 antibody) in combination with trastuzumab in HER2+ MBC patients that progressed after previous HER2 targeted therapies. Fifteen percent of the patients that were PD-L1 positive achieved an overall response and no overall response was observed in the PD-L1 negative cohort (18). Another study in a phase 1 trial evaluated trastuzumab in combination with durvalumab (anti-PD-L1) in metastatic HER2+ breast cancer patients and no impact on objective responses was observed and all the patients enrolled in the trials had lower than 1% PD-L1 expression (19). Overall, these studies suggest that checkpoint inhibitors combined with anti-HER2 therapy have minimal impact. Developing strategies that can increase T cell infiltration in tumors may improve the efficacy of these therapies.
Vaccine strategies using dendritic cells to activate the immune system and generate Th1 immune responses have been extensively studied (20, 21). It has been well-documented that Th1 cytokine, IFN-γ can induce PD-L1 expression on tumor cells (22). In preclinical models of various cancer types with increased levels of immune checkpoint molecules expression on TIL, immune checkpoint blockade in combination with vaccine strategies has shown a superior response compared to monotherapy (23, 24). The role of CD8+ T cells to generate anti-tumor immunity in HER2+ breast cancers has been shown in various clinical trials (25) and has had minimal clinical impact (26, 27). However, the role and prognostic value of CD4+ T cells has not been extensively studied in breast cancer. Previous findings from our lab have shown that anti-HER2 CD4+ T helper cell (Th1) immunity plays a crucial role in cancer therapy and peripheral loss of the Th1 response correlates with poor treatment response and prognosis (28). Administration of class II HER2 peptide-pulsed Type I polarized dendritic cell (DC1) vaccine induced a strong anti-HER2 immune response with pathologic complete response rate (pCR) in HER2+ DCIS patients (29–31). Very little is known about the role of anti-HER2 CD4+ Th1 immune responses in combination with immune checkpoint therapy. Based on these preliminary findings, we hypothesized that HER2 peptide pulsed DC1 vaccine could prime an anti-HER2 response and generate anti-HER2 Th1 immune responses leading to the conversion of “cold” to “hot” tumors and thus improve the efficiency of immune checkpoint antibody therapy. The goal of this study was to investigate the anti-tumor efficacy of HER2 peptide pulsed DC1 vaccine in combination with PD-1/PD-L1 blockade and HER2 targeted therapy in a preclinical model of HER2+ breast cancer.
Materials and Methods
Animals
This study was carried out in strict accordance with the recommendations in the Guide for the Care and Use of Laboratory Animals of the National Institutes of Health. The protocol was reviewed and approved by the Institutional Animal Care and Use Committee at the University of South Florida (#A4100-01). Mice were euthanized by CO2 inhalation according to the American Veterinary Medical Association Guidelines. Mice were observed daily and euthanized if a solitary subcutaneous tumor exceeded the end point. All efforts were made to minimize suffering. Female Balb/C mice (6–8 weeks old) were purchased from Charles river. Mice were housed at the Animal Research Facility of the H. Lee Moffitt Cancer Center and Research Institute.
Tumor Cell Lines
TUBO breast cancer cell line (kind gift from Dr. Wei Zen Wei, Wayne State University) was cloned from a spontaneous mammary tumor in BALB/c mice transgenic for the rat Her-2/neu gene (BALB-neuT) (32) and was maintained by serial in vitro passages in complete medium (CM). Complete media consisted of RPMI 1640 (Fisher Scientific, Cat. No. MT-10-040-CM) supplemented with 10% heat-inactivated FBS (Fisher Scientific, Cat. No. MT35010CV), 0.1 mM nonessential amino acids (Fisher Scientific, Cat. No. 25025CI), 1 mM sodium pyruvate (Fisher Scientific, Cat. No. 25000CI), 2 mM fresh L-glutamine (Fisher Scientific, Cat. No. 25005CI), 100 mg/ml streptomycin and 100 U/mL penicillin (Fisher Scientific, Cat. No. MT-30-002-CI), 50 mg/mL gentamicin (Gibco, Cat. No. 15750060), 0.5 mg/mL fungizone (Gibco, Cat. No. 15290018) (all purchased from Life Technologies, Rockville, MD), and 0.05 mM 2-ME (Gibco, Cat. No. 21985023).
DC Generation
Bone marrow (BM) cells were harvested from femurs and tibias of Balb/C mice as described previously (33). Briefly, BM cells were flushed into a cell suspension in RPMI 1640, and RBCs were lysed using ACK lysing buffer. Cells were cultured with rFLT3L (VWR Peprotech, Cat. No. 10778-670) at 25 ng/mL and rmIL-6 (R&D Systems, Cat. No. 406-ML-025) at 30 ng/mL in T75 flasks and incubated for 6 days at 37°C and 5% CO2. The BM cells were then harvested, washed with RPMI 1640 and cultured with 50 ng/mL of rmGM-CSF (R&D Systems, Cat. No. 415-ML-050) and 10 ng/mL of rmIL-4 (R&D Systems, Cat. No. 404-ML-050) overnight, followed by DC1 maturation for 6–8 hours (h) with DC1 polarizing signals: CPG/ODN1826 (InVivoGen, Cat. No. tlrl-1826), a TLR 9 agonist at 10 ng/mL and lipopolysaccharide (LPS) (Millipore Sigma, Cat. No. L4391), a TLR-4 agonist at 20 ng/mL as described previously (33). When used for vaccination, DC1 cells were pulsed with multi-epitope peptides from the rat HER2/neu (rHER2/neu) oncogene at the concentration of 10 μg/ml of each peptide individually overnight; p5 (ELAAWCRWGFLLALLPPGIAG), p435 (IRGRILHDGAYSLTLQGLGIH), and p1209 (SPPHPSPAFSPAFDNLYYWDQ) and were pooled for class II HER2-DC1 vaccine studies (34). DC1 were pulsed with class I rat HER2/neu peptide p66 (TYVPANASL) for class I HER2-DC1 vaccine studies (35). All the peptides were synthesized from Bachem Americas, Inc. DC maturation was confirmed in a subset of samples at 24 h post addition of LPS and CPG by FACS analysis of cell surface markers, MHC class II (I Ad), CD80, CD86, and CD40 (FITC anti-mouse I-Ad (Clone 39-10-8, Biolegend, Cat. No. 115006); PE anti-mouse CD80 (Clone 16-10A1, Biolegend, Cat. No. 104708) anti-mouse CD40; PE anti-mouse CD86 (Clone GL-1, Biolegend, Cat. No. 105008); PE anti-mouse CD40 (Clone 3/23, Biolegend, Cat. No. 124610). IL-12 (p70) secretion by DC1 in culture supernatants was measured by standard IL-12 (p70) ELISA from R& D systems (Cat. No. M1270).
Monoclonal Antibodies
The monoclonal antibodies anti-PD-1 (clone RMP1-14, Cat. No. BE0146) and anti-PDL-1 (clone 10F.9G2, Cat. No. BE0101) were purchased from BioXCell (West Lebanon, NH). InVivoMAb rat IgG2a isotype (BioXCell, Cat. No. BE0089) was used as control. Anti-HER2 mouse monoclonal antibody 7.9.5 was a kind gift from Dr. Mark Greene, University of Pennsylvania and clone 7.16.4 was purchased from BioXCell (Cat. No. BE0277).
Immunofluorescence Staining for HER2
TUBO cells were grown to 80% confluence on sterile round glass coverslips in a six well tissue culture plate. Cells were washed three times with PBS and fixed in 4% (wt/vol) paraformaldehyde (Fisher Scientific, Cat. No. 50-980-487) for 15 min. Next, cells were permeabilized with 0.2% Triton X (Sigma Aldrich, Cat. No. T8787) for 10 min and washed three times with PBS. After washing, cells attached to cover slips were incubated with 5% (wt/vol) bovine serum albumin (BSA) (Fisher Scientific, Cat. No. BP1605) in PBS for 1 h at room temperature. Cells were then incubated with monoclonal primary anti-HER2/ErbB2 antibody (Cell Signaling Technology, Beverly, MA, Cat. No. 2165) over night at 4°C followed by incubation with Alexa Fluor 594-conjugated anti-rabbit secondary antibody (Cell Signaling Technology, Beverly, MA, Cat. No. 8889) for 1 h at room temperature in the dark. After being washed with PBS three times, coverslips were mounted using VECTASHIELD Antifade Mounting Medium with DAPI (Vector Laboratories, Cat. No. H-1200). The stained coverslips were examined and imaged using a Zeiss Apotome.2 fluorescence microscope (Carl Zeiss Inc., Thornwood, NY).
Western Blot Analysis
Total protein was isolated from TUBO and 4T1 cells for Western blot analysis. Briefly, cells were lysed with 1X RIPA buffer (EMD Millipore™, Cat. No. 20-188) containing protease inhibitor (Millipore Sigma, Cat. No. P8340) and phosphatase inhibitor (ThermoScientific Pierce, Cat. No. A32957), for 20 min at 4°C. The cell lysate was centrifuged at 15,000 rpm for 20 min and the supernatant containing total protein was collected and stored at −80°C until further use. Protein concentration was measured by Bradford protein assay (Bio-Rad, Hercules, CA, Cta. No. 5000006). For Western blotting, 20 μg of each protein sample was resolved in a 4–12% SDS-PAGE and then transferred to a polyvinylidene difluoride (PVDF) membrane (Millipore Sigma, Cat. No. IPVH00010) using eBlot® L1 wet transfer system (GenScript, Piscataway, NJ). Membranes were blocked with 5% bovine serum albumin (BSA)/TBS-T for 1 h at room temperature, followed by overnight incubation with primary monoclonal anti-HER2/ErbB2 antibody (Cell Signaling Technology®, Cat. No. 2165) (1:1,000 dilution in 5% BSA/TBS-T) at 4°C. The next day, membranes were washed three times, 10 min each wash with TBS-T, and was probed with goat anti-rabbit IgG (H + L)-HRP conjugated secondary antibody (Cell Signaling Technology®, Cat. No. 7074; 1:5,000 dilution in 5% non-fat dry milk/TBS-T) for 1 h at room temperature and detected using the ECL western blotting detection system (Thermo Scientific Pierce, Cat. No. 32106). β-actin was used as endogenous control for all Western blot data analyses.
In vivo Treatments
A total of 2.5 × 105 TUBO cells were injected subcutaneously (s.c.) in female Balb/C mice. Seven days later when tumors were palpable, mice were treated with six doses of class I or class II HER2-DC1 vaccine subcutaneously either once, twice or three times weekly. For combination therapy, mice received either class I or class II HER2-DC1 vaccine (1 × 106 cells/mouse/subcutaneous injection/ 100 μl) with 150 μg of monoclonal antibody (isotype control or anti-PD-1 or anti-PD-L1) intraperitoneally twice a week concurrently or DC1 vaccination given first, followed by checkpoint antibodies. Mice continued to receive checkpoint antibody treatment twice a week until the tumor reached a size of 2 cm in diameter. Tumor size was measured and recorded every 2–3 days. Six mice per group were used and each experiment was performed three times. For functional analysis, mice were euthanatized at day 28 after tumor injection. Tumors and splenocytes were harvested for in vitro assays.
CD4 T Cells Depletion
Anti-CD4 antibody (InVivoMab clone GK1.5 purchased from BioXCell, Cat. No. BE0003-1) was used to deplete CD4 T cells in the experimental mice. Three days before the TUBO injection, Balb/c mice were administered intraperitoneally with 300 μg of anti-CD4 antibody and continued with two injections per week until the end point. When tumors were palpable around days 7–10, mice were treated with multi-epitope class II HER2-DC1 vaccine subcutaneously twice a week. Another group of TUBO bearing mice without CD4 depletion received class II HER2-DC1 vaccine twice a week for total of six doses. Mice treated with or without CD4 depleting antibody were randomized into two groups to receive a follow up treatment with anti-PD1 antibody twice a week until the end point. Tumor size was measured and recorded twice a week.
HER2 Blockade in Combination With Class II HER2-DC1 and Anti-PD-1 Therapy
For in vivo treatments of HER2 targeted therapy in combination with HER2-DC1 vaccine and anti-PD-1 antibody, Balb/C mice were injected with 3 × 104 TUBO cells/50 μl in mammary fat pad per mouse. On day 12 after TUBO cells injection and when tumors were palpable, mice were randomized in four groups: (1) untreated, (2) anti-PD-1 therapy, (3) class II HER2-DC1 vaccine, and (4) combination therapy with anti-HER2, anti-PD-1, and HER2-DC1. For combination treatments, mice received anti-HER2 antibodies (clone 7.16.4 and 7.6.5) (50 μg/clone/mouse) on day 12. One week after anti-HER2 antibody treatment, mice received HER2-DC1 vaccine subcutaneously twice a week concurrently with combined 7.16.4 and 7.6.5 antibodies given once a week for 3 weeks. Upon completion of the combination treatment, follow up with anti-PD-1 antibody was given twice a week until the end point. Tumor growth was monitored twice a week and tumor volume was calculated following the formula: (L x W2)/2 = mm3.
Flow Cytometry
On day 28 after tumor injection, spleens and/or tumors were harvested under sterile conditions. Single-cell suspensions were prepared, and red blood cells were lysed using ACK lysis buffer. Tumor cell suspensions were prepared from solid tumors by enzymatic digestion in HBSS (Fisher Scientific, Cat. No. MT-21-022-CM) containing 1 mg/ml collagenase (Cat. No. C9891 and C-5138), 0.1 mg/ml DNase I (Cat. No. DN25), and 2.5 U/ml of hyaluronidase (Cat. No. H-6254-1G) (all purchased from Millipore Sigma) with constant stirring for 2 h at room temperature as described previously (36). For analysis of immune cell populations, 1 × 106 cells (tumor digest suspension) were incubated for 30 min with Live/ Dead Zombie near IR (Biolegend, Cat. No. 423106) for 30 min in 1X PBS at room temperature in dark. After washing cells with 1X PBS, cells were stained with anti-mouse CD3 Alexa 488 (Clone 17A2, Biolegend, Cat. No. 100210), anti-mouse CD4 BV805 (Clone GK1.5, BD Biosciences, Cat. No. 564922), and anti-mouse CD8 pacific Blue (Clone 53–6.7, BD Bioscience, Cat No. 558106) and anti-mouse PD-1 BV605 (Clone 29F.1.A12, Biolegend, Cat. No. 135220) for 20 min on ice in staining buffer for surface expression analysis, according to the manufacturer's instructions (all antibodies were purchased from BD Biosciences). Samples were analyzed using an LSRII (BD Biosciences) cytometer and FACS data was analyzed using FlowJo software (Tree Star).
Functional Assays
To examine antigen specificity following HER2-DC1 vaccination in TUBO bearing mice, 2 × 106 splenocytes from control and treatment groups were cultured with 2 μg/ml of class I (p66) peptide, control peptide, or no peptide (complete media only) or multi-epitope class II rat HER2/neu peptides (p5, p435, p1209) individually for 3–4 days. Culture supernatants were collected to measure IFN-γ secretion using a standard quantikine IFN-γ ELISA (R&D systems, Cat. No. SMIF00) according to manufacturer's recommendations.
Statistical Analysis
The Mann–Whitney test (unpaired) or the Student's t-test was used to compare results between two treatment groups. All statistical analyses of data were performed using GraphPad Prism software. Statistical significance was achieved at p < 0.05.
Results
DC1 From Balb/C Mice Secrete IL-12 and Express CD80, CD86, and CD40
To examine the phenotype and the maturation status of DC generation from bone marrow of Balb/C mice, DC1 were collected following maturation and stained for the expression of cell surface markers, class II (I-Ad), CD80, CD86, and CD40 and data was acquired on flow cytometer as described in the Materials and Methods section. Functional status of DC1 was measured by IL-12 production 24 h after addition of final maturation signals. Culture supernatants from immature DC (iDC) were used as control. Flow gating strategy is shown in Figure 1A. Cells were gated on live population followed by gating on MHC class II (IAd) positive cells for CD80, CD86, and CD40 expression. Addition of CPG and LPS resulted in a higher percentage of DC maturation surface markers CD80, CD86, and CD40 (Figure 1A) with higher levels of IL-12 production compared to iDC (Figure 1B, p < 0.001). To examine the anti-tumor efficacy of DC1 vaccine in a HER2+ breast tumor model, we utilized the TUBO cell line which was derived from a spontaneous mammary tumor in Balb/c mice transgenic for the rat Her-2/neu gene (BALB-neuT). As shown in Figure 1C, we confirmed the surface expression of HER2 on TUBO cells by immunofluorescence. HER2 protein expression was also confirmed by western blot, along with 4T1, a triple negative cell line (negative for ER, PR, and HER2), as negative control (Figure 1D). Overall, our data suggests that DC1 we generated from bone marrow of Balb/C mice secrete high levels of IL-12, and express mature DC phenotype.
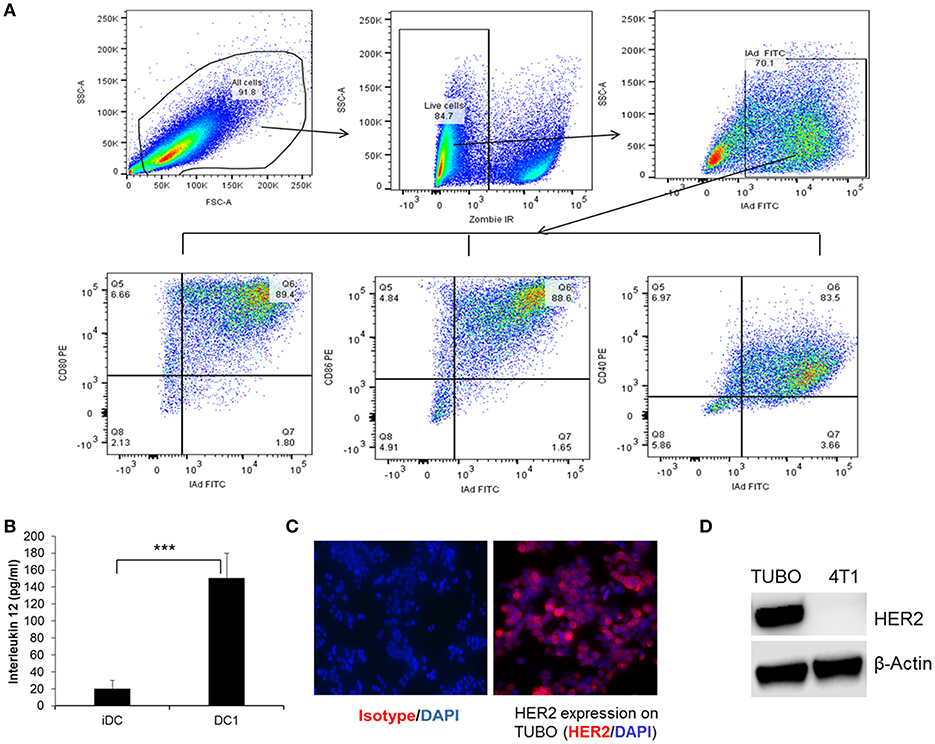
Figure 1. Immunophenotyping and function of type I polarized DC1 from Balb/C mice. (A) DC collected after maturation with CpG and LPS were stained for MHC class II (IAd), CD80, CD86, and CD40 and data were acquired on LSRII flow cytometer and analyzed by FlowJo software. Flow gating strategy and representative flow dot plot of DC1 staining for MHC class II, CD80, CD86, and CD40. (B) Culture supernatants were collected before and after DC maturation and measured for IL-12 secretion using standard ELISA. (C) HER2 expression on TUBO cells using immunofluorescence staining. (D) Western blot analysis of HER2 protein expression on tumor cells. P-values were determined by Student t-test. ***p < 0.001.
Class I or Class II HER2 Peptide- DC1 Vaccine Delay Tumor Growth and Induce Anti-HER2 Th1 Immune Response With Increased T Cell Infiltration in TUBO Bearing Mice
To examine the anti-tumor efficacy of DC1 vaccine, we utilized the HER2 positive TUBO model. Balb/C mice were injected with TUBO cells (2.5 × 105 cells/mouse/s.c.,) on day 0. Starting on day 7 when tumors were palpable, TUBO bearing mice were treated with either class I HER2 pulsed DC1 vaccine (Class I HER2-DC1, 1 × 106 DC1/mouse/100 μl /s.c.,) or class II HER2 peptide pulsed DC1 (Class II HER2-DC1). Treatment groups included TUBO bearing mice with no treatment, HER2-DC1 vaccine given once a week, twice or three times a week for total of up to six injections. TUBO bearing mice receiving class I or class II pulsed HER2-DC1 vaccine showed significantly delayed tumor growth compared to control mice (Figures 2A,B; p < 0.001) irrespective of whether HER2-DC1 vaccine was given once, twice or three times weekly. However, TUBO bearing mice receiving HER2-DC1 vaccine twice or three times a week had reduced tumor burden compared to the mice receiving weekly dose of class I or class II HER2-DC1 vaccine (Figures 2A,B). Although there was a significant delay in tumor growth in mice receiving HER2-DC1 vaccine given three times a week, as shown in Figure 2A, toxicity was observed (weight loss, hunched and sudden death) in this group. There was none observed in mice receiving once or twice weekly class I HER2-DC1 vaccine.
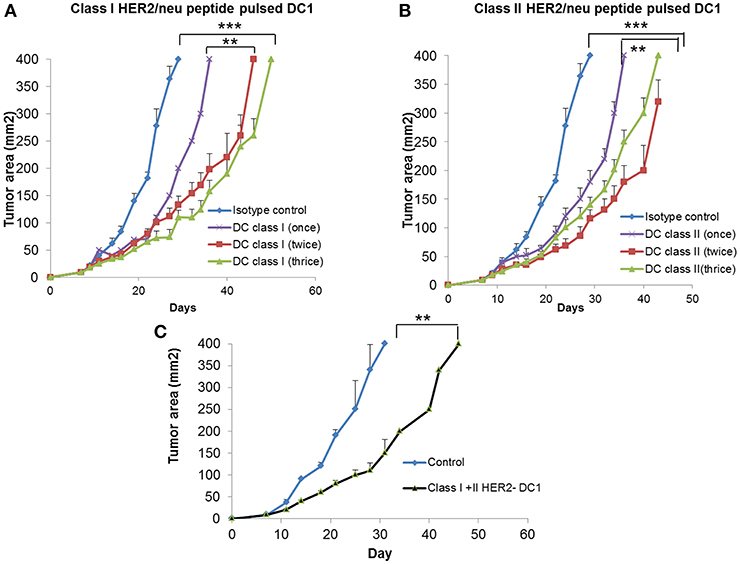
Figure 2. Vaccination with DC1 pulsed with class I or class II HER2 peptides delays tumor growth in TUBO bearing mice. (A) DC1 was generated as described in methods section and pulsed with class I rHER2 peptide p66 (class I HER2-DC1). Balb/C mice were injected with 2.5 × 105 TUBO cells subcutaneously on the right flank on day 0. When tumors were palpable on day 7, mice received class I HER2-DC1 vaccine subcutaneously on the left flank once, twice or three times a week for a total of six doses. N = 8 mice/group was used for these studies. Tumor area was measured 2–3 days a week. (B) Balb/C mice received tumor cells and HER2-DC1 vaccine as described above. DC1 were pulsed with 10 μg/ml of each peptides from the rat HER2 (rHER2) oncogene; p5 (ELAAWCRWGFLLALLPPGIAG), p435 (IRGRILHDGAYSLTLQGLGIH), and p1209 (SPPHPSPAFSPAFDNLYYWDQ) and p66 (TYVPANASL). (C) Balb/C mice received tumor cells as described above and received both class I and class II pulsed HER2-DC1 vaccine. Data shown are the representative from three independent experiments and are shown as mean number ± SEM ***p < 0.001, **p < 0.01 using Student t-test.
Since we observed anti-tumor effects of class I and class II HER2-DC1 vaccines shown in Figures 2A,B, we examined whether combining both class I and class II pulsed HER2-DC1 vaccine could have a synergistic effect in delaying tumor growth. As shown in Figure 2C, combined class I and class II pulsed HER2-DC1 vaccine given twice a week significantly delayed tumor growth in TUBO bearing mice but there was no additive benefit in reducing tumor burden (Figure 2C) compared to class I or class II HER2-DC1 alone as shown in Figures 2A,B.
HER2-DC1 Vaccine Generates Anti-HER2 Th1 Immune Responses in TUBO Bearing Mice
Next, we evaluated whether vaccination with HER2-DC1 could generate strong anti-HER2 Th1 immune responses in TUBO bearing mice. Spleens were harvested 1 week after the last DC1 vaccination and splenocytes were cultured with class I or class II peptides as described in the Materials and Methods section. Re-stimulation of splenocytes from class I HER2-DC1 vaccinated mice with p66 (class I) peptide had significantly increased IFN-γ secretion compared to splenocytes from untreated mice (Figure 3A, p < 0.001). Similarly, re-stimulation of splenocytes from the class II HER2 peptide pulsed DC1 group had higher levels of IFN-γ production in response to HER2 peptides, p5, p1209, and p435 peptides (Figure 3B, p < 0.001). These findings suggest that class I and class II HER2-DC1 vaccine can generate anti-HER2 CD8+ and CD4+ Th1 specific immune responses and can delay tumor growth in HER2+ TUBO bearing mice. We next examined whether vaccination with HER2-DC1 vaccine could improve T cell infiltration within the tumor. Tumors were excised from control and treatment groups and single cell suspensions were prepared and stained for cell surface markers (Live/Dead Zombie near IR, CD3, CD4, and CD8 gated on live cells) as described in Materials and Methods section. The flow gating strategy to identify CD4+ and CD8+ tumor infiltrating lymphocytes is shown in Figure 3C. Class I (p66) HER2-DC1 vaccine in TUBO bearing mice led to a significant increase in tumor infiltrating CD8+ T cells but not CD4+ T cells, while class II HER2-DC1 vaccine significantly increased both CD4 and CD8+ T cell infiltration compared to untreated controls (Figures 3D,E, p < 0.001). Figure 3D represents the T cell infiltration per milligram of tumor and Figure 3E shows percent of T cells of all live cells from tumor digest suspension. This data suggests that HER2-DC1 vaccine enhances T cell infiltration within the tumor in TUBO bearing mice.
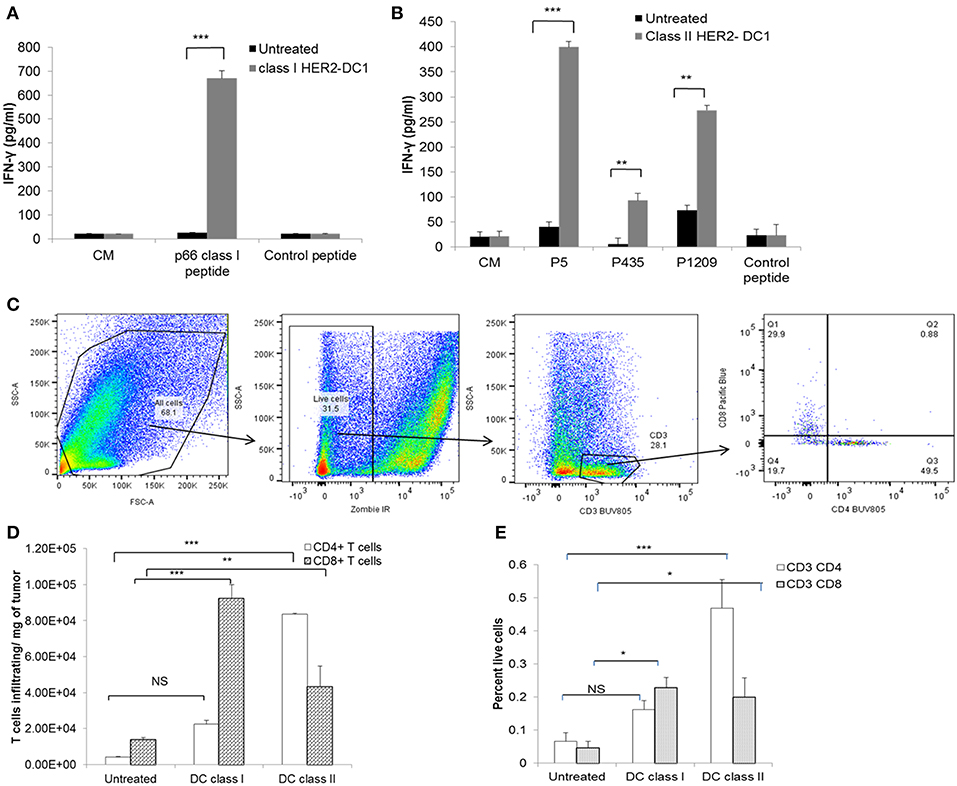
Figure 3. Vaccination with HER2-DC1 enhances anti-HER2 Th1 immune response and increases T cell infiltration in TUBO bearing mice. (A) Splenocytes from class I HER2-DC1 vaccinated mice were re-stimulated with p66 rHER2 peptide. Culture supernatants were collected after 3 days and IFN-γ secretion was measured by standard IFN-γ ELISA. (B) Splenocytes from class II HER2-DC1 vaccinated mice were re-stimulated with (class II) rat HER2 peptides (rHER2); p5 (ELAAWCRWGFLLALLPPGIAG), p435 (IRGRILHDGAYSLTLQGLGIH), and p1209 (SPPHPSPAFSPAFDNLYYWDQ) individually and IFN-γ secretion was measured by standard IFN-γ ELISA. (C) Flow gating strategy and analysis of T cell infiltration in tumors by flow cytometry. Tumors were harvested on day 30 and processed as described in Materials and Methods section. Single cell suspension was stained for live/dead near IR, CD3, CD4, and CD8. Data were acquired on an LSRII flow cytometer and analyzed by FlowJo software. (D) Bar graphs represent T cell infiltration gated on live cells per mg of tumor and (E) percent T cells of all live cells within the tumor single cell suspension;. ***p < 0.001, **p < 0.01, *p < 0.05 using Student t-test.
TIL From HER2-DC1 Vaccinated Mice Express Higher Levels of PD-1 Checkpoint Receptor
Inhibitory receptors such as PD-1 expressed on T cells and their ligands such as PD-L1 expressed on tumor cells have been shown to contribute to immune mediated suppression. We investigated whether HER2-DC1 has any effect in modulating the expression of PD-L1 on tumor cells and PD-1 receptor expression on TIL. Tumors were harvested from experimental mice, a single cell suspension was prepared and flow staining was performed as described in Materials and Methods section. As shown in Figure 4A, PD-L1 expression was observed in tumors from untreated mice and HER2-DC1 vaccinated tumor-bearing mice. We did not observe any difference in the expression levels of PD-L1 between tumors from control and HER2-DC1 vaccinated mice. We evaluated the expression of PD-1 on tumor infiltrating lymphocytes. Flow gating strategy is shown in Figure 4B. Cells were gated on live population followed by gating on CD3 positive cells. PD-1 expression on CD4 and CD8 cells was analyzed on samples by gating on Fluorescent minus one (FMO) controls. We observed increased PD-1 expression on CD8+ T cells infiltrating within the tumor following HER2-DC1 vaccination compared to control mice (Figure 4C; p < 0.05). In contrast to CD8+ T cells, there was only a modest but not statistically significant increase in PD-1 expression on CD4+ T cells within the tumor following HER2-DC1 vaccination compared to untreated control (Figure 4C). This data suggests that blockade of immune checkpoints in combination with HER2-DC1 vaccine may improve the anti-tumor immune responses in the preclinical model of HER2 positive TUBO breast cancer.
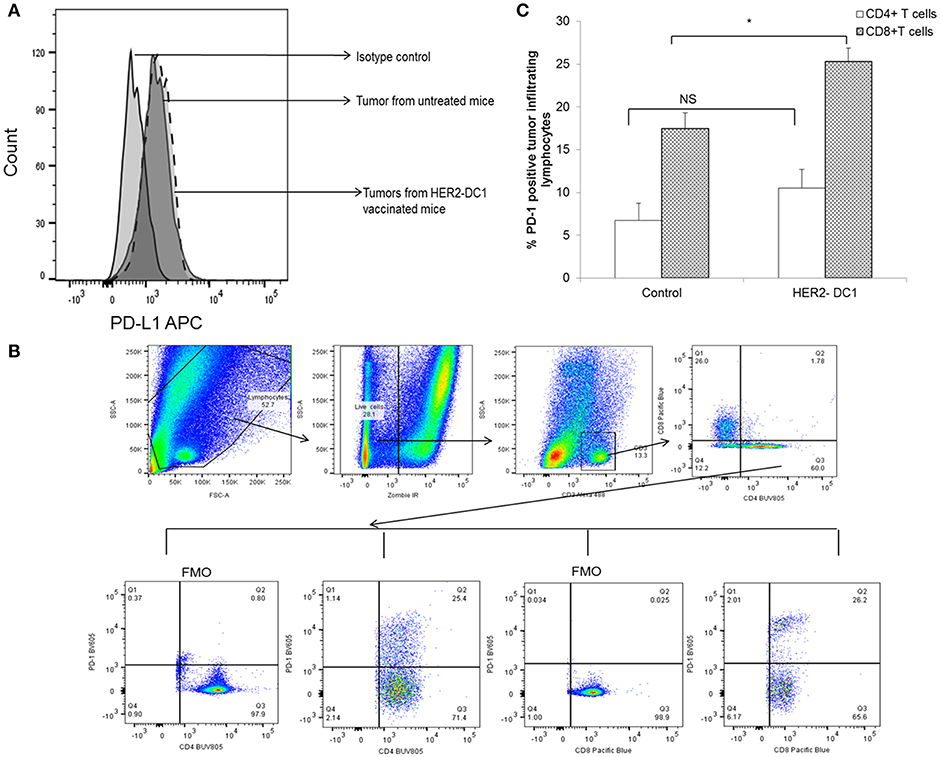
Figure 4. PD-1 expression on TIL and PD-L1 expression on tumors of TUBO bearing mice. (A) Tumors were harvested 1 week after the last HER2-DC1 treatment and single suspensions were prepared as described in Materials and Methods section. Single cell tumor digest suspensions were stained for PD-L1 expression and data were acquired on a LSR-II and analyzed using Flowjo software. (B) Flow gating strategy of PD-1 expression on tumor infiltrating T cells. (C) Tumors were harvested as described in Materials and Methods section and flow staining was performed. Bar graphs show the percent PD-1 expression on CD4 and CD8+ TIL *p < 0.05, NS, Not significant using Student t-test.
Class I HER2-DC1 Vaccine in Combination PD-1/PD-L1 Blockade
The presence of TILs has been associated with a favorable prognosis in HER2+ breast cancer and to potentially predict responders to immune checkpoint blockade (37, 38). Targeting the PD-1 pathway with pembrolizumab in combination with trastuzumab has shown efficacy in HER2 positive trastuzumab resistant patients. The overall response was encouraging in PD-L1 positive cohort. However, there was no overall response in the PD-L1 negative cohort (18). Since we observed increased PD-1 expression on TILs and PD-L1 expression on tumors cells following HER2-DC1 vaccination in TUBO bearing mice, we investigated whether blockade of immune checkpoints, PD-1 or PD-L1 in combination with HER2-DC1 vaccine would enhance the anti-tumor immune response in TUBO bearing mice. Balb/C mice were injected with TUBO cells on day 0. On day 7, when tumors were palpable, two different treatment regimens were followed to examine the efficacy of combination therapy. One group of mice received checkpoint monoclonal antibodies (anti-PD-1 or anti-PD-L1) in combination with class I HER2-DC1 concurrently. Another group of mice received class I HER2-DC1 vaccine twice a week and at the completion of sixth HER2-DC1 vaccine, mice received anti-PD-1 or anti-PD-L1 antibody therapy twice a week until the end point. In addition other treatment groups received either HER2-DC1 alone, anti-PD-1, or anti-PD-L1 antibody as monotherapy. Control mice received isotype control antibody as described in Materials and Methods section. As shown in Figure 5A, TUBO- bearing mice that received Class I (p66) HER2 peptide pulsed DC1 concurrently with intraperitoneal injection of anti-PD-1 or anti-PD-L1 monoclonal antibodies had no significant delay in the tumor growth compared to mice treated with class I (p66) HER2-DC1 alone. However, TUBO bearing mice that received Class I (p66) HER2 peptide pulsed DC1 vaccine followed by treatment with anti-PD-1 monoclonal checkpoint antibodies had a significant delay in tumor growth compared to the mice that received DC1 or checkpoint antibodies alone (Figure 5B). Importantly, TUBO bearing mice that received Class I (p66) HER2 peptide pulsed DC1 in combination with anti-PD-1 antibody had significant delay in tumor growth and doubled the survival rate in TUBO bearing mice, compared to mice that received single treatment or no treatment (Figures 5B,C, p < 0.01). However, sequential combination of Class I (p66) HER2-DC1 with anti-PD-L1 did not have an impact on delaying tumor growth or survival benefit compared to DC1 alone (Figures 5B,C).
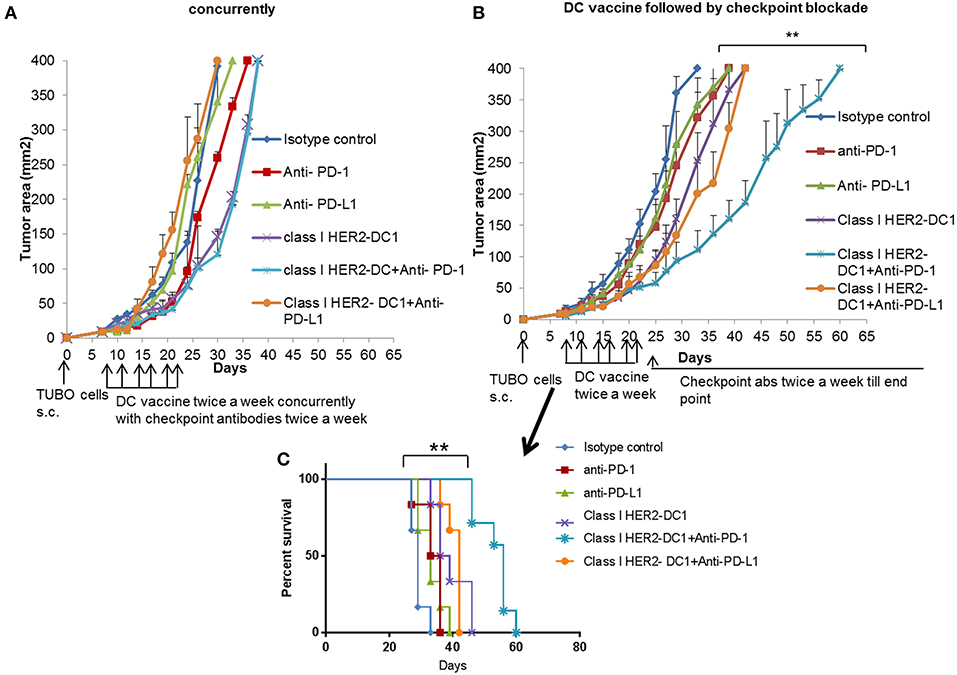
Figure 5. Optimal scheduling of checkpoint antibodies is critical in improving the efficacy of class I HER2-DC1 vaccine in TUBO bearing mice. (A) Balb/C mice were injected with 2.5 × 105 TUBO cells subcutaneously on the right flank on day 0. When tumors were palpable on day 7, mice received class I HER2-DC1 vaccine subcutaneously on the left flank twice a week for a total of six doses concurrently with intraperitoneal injection of 150 μg/mouse/200 μl of anti-PD-1 or anti-PD-L1 antibody. (B) Balb/C mice were injected with 2.5 × 105 TUBO cells subcutaneously on the right flank on day 0. When tumors were palpable on day 7, mice received class I HER2-DC1 vaccine (1 × 106 DC1/mouse/100 μl) subcutaneously on the left flank twice a week for a total of six doses. Anti-PD-1 or anti-PD-L1 antibody therapy (150 mg/200 ml/mouse/intraperitoneally) twice a week was started after the last injection of HER2-DC1 and continued until the end point. N = 8 mice/group was used for these studies and the line graph shown is the representative of triplicate experiments. (C) Survival curve. Data shown are the representative from three independent experiments and are shown as the mean number ± SEM. P-values were determined by unpaired student t-test (A,B) or a log-rank test (C). **p < 0.01.
Class II HER2 Peptides Pulsed DC1 in Combination With Anti-PD-1 Antibody Therapy
The role of CD8+ T cells in improving immune checkpoint blockade has been shown previously (39, 40). However, to the best of our knowledge, the role of CD4+ helper T cells in facilitating and mediating anti-tumor immune responses in combination with checkpoint blockade has not been studied. To address this, we evaluated therapeutic efficacy of class II HER2-DC1 vaccine in combination with anti-PD-1 antibody therapy. Balb/C mice were injected with TUBO cells on day 0. On day 7, when tumors were palpable, mice received class II HER2-DC1 twice a week for 3 weeks followed by anti-PD-1 antibody therapy twice a week until the end point. Addition of anti-PD1 antibody delayed tumor growth in class II HER2-DC1 vaccinated mice compared to HER2-DC1 alone with survival rate tripled (Figures 6A,B). We also evaluated anti-tumor efficacy of class II HER2-DC1 vaccine in combination with anti-PD-L1 antibodies. We did not observe any additional therapeutic benefit of class II HER2-DC1 vaccine when combined with anti-PD-L1 antibody (Data not shown). These results suggest that checkpoint inhibitors given concurrently with HER2- DC1 vaccine do not have any additive benefit, while administration of anti-PD-1 antibody following generation of anti-HER2 Th1 immune response has an impact on both tumor growth and survival. Overall, these findings suggest that optimal scheduling of immune checkpoints is critical in enhancing the efficacy of HER2-DC1 vaccine.
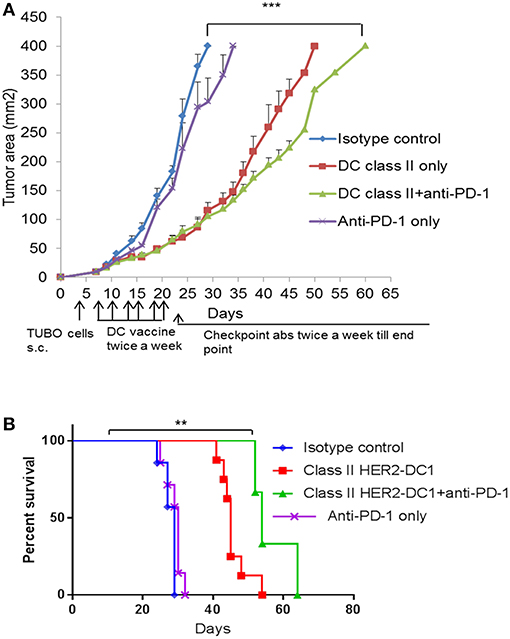
Figure 6. Anti-tumor efficacy of class II HER2 peptides pulsed DC1 in combination with anti-PD-1 antibody therapy. (A) Balb/C mice were injected with 2.5 × 105 TUBO cells subcutaneously on the right flank on day 0. When tumors were palpable on day 7, mice received class II HER2 peptides pulsed DC1 vaccine (1 × 106 DC1/mouse/100 μl) subcutaneously on the left flank twice a week for a total of six doses followed by intraperitoneal injection of 150 μg/mouse/200 μl of anti-PD-1 antibody twice a week until the end point. (B) Survival curve. Data shown are representative from three independent experiments and are shown as the mean number ± SEM. P-values were determined by unpaired student t-test (A) or a log-rank test (B). ***p < 0.001, **p < 0.01.
Class I and Class II HER2-DC1 Vaccine in Combination With Anti-PD-1 Antibody Therapy Improves T Cell Infiltration, Function, and Specificity
The effect of PD-1 blockade in combination with HER2-DC1 on T cell infiltration, function and specificity was examined. Balb/C mice were injected with TUBO cells on day 0. On day 7, when tumors were palpable, mice received class I or class II HER2-DC1 twice a week for 3 weeks followed by anti-PD-1 antibody therapy. Spleens and tumors were collected from experimental mice on day 35 to examine the T cell infiltration, function and antigen specificity as described in Materials and Methods section and figure legends. Administration of anti-PD-1 antibody in combination with class I HER2-DC1 vaccination increased CD8+ T cell infiltration in tumors (per milligram of tumor) compared to tumor bearing mice that received class I HER2-DC1 only (Figure 7A, p < 0.01). We did not observe any changes in CD4+ T cell infiltration per milligram of tumor in mice that received class I HER2-DC1 alone or in combination with anti-PD-1 antibody therapy. Administration of class II HER2-DC1 in combination with anti-PD-1 antibody therapy significantly increased both CD4 and CD8+ T cell infiltration per milligram of tumor (p < 0.01) compared to HER2-DC1 group alone and untreated controls as shown in Figure 7B. Data shown in Figure 7C represent the percent T cells of all live cells from tumor digest suspension.
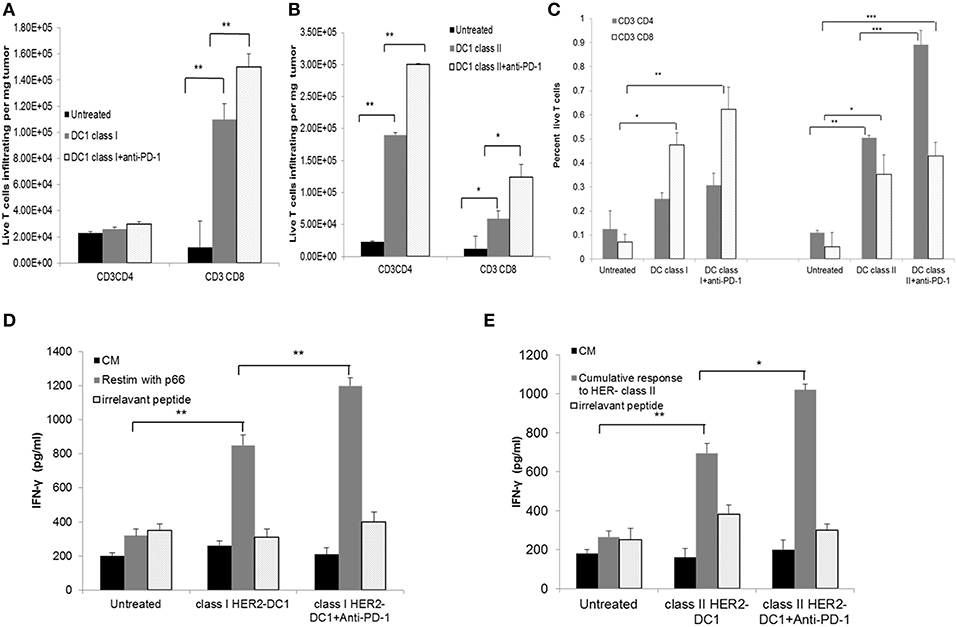
Figure 7. HER2-DC1 vaccine in combination with anti-PD-1 antibody therapy improves T cell infiltration, function, and specificity. (A,B) Tumors were collected and single cell suspensions were prepared and stained for CD3, CD4, and CD8 as described in methods. Bar graph shows T cell infiltration per mg of tumor. (C) Percent T cells of all live cells within the tumor single cell suspension. (D,E) Splenocytes were re-stimulated with class I, class II HER2 peptides or irrelevant OT-I or OT-II peptides. Culture supernatants were collected after 72 h and IFN-γ was measured by standard ELISA. P-values were determined by unpaired student t-test ***p < 0.001, **p < 0.01, *p < 0.05.
Splenocytes from mice that received treatments were re-stimulated with p66 class I HER2 peptide. Irrelevant OVA peptides served as negative controls and did not show any non-specific reactivity. Higher levels of IFN-γ production were observed in class I HER2-DC1 in combination with anti-PD-1 therapy compared to class I HER2-DC1 alone group (Figure 7D, p < 0.01). Similar results were observed in mice that received class II HER2-DC1 vaccine when re-stimulated with relevant peptides. Cumulative response to p5, p1209, and p435 peptides is shown in Figure 7E. There was a slight trend toward increased IFN-γ levels between the class II HER2-DC1 alone group and the combination of class II HER2-DC1 and anti-PD-1 antibody treated group in response to re-stimulation with peptides. This data suggest that addition of anti-PD-1 antibody therapy with HER2-DC1 vaccine significantly increases T cell infiltration, enhances tumor specificity and function.
Effect of Combination Therapy With Class II HER2-DC1 and PD-1 Blockade Is Mediated by CD4+ T Cells
Our findings suggest that TUBO bearing mice treated with class II HER2-DC1 vaccine can drive both CD4 and CD8+ T cell infiltration. To determine the role of CD4+ T cells in mediating tumor delay in TUBO tumors, mice were treated with CD4 depleting antibody on day −3 followed by injection of TUBO cells on day 0. When tumors were palpable, mice received either class II HER2-DC1 alone for 6 weeks or followed by anti-PD-1 antibody twice a week until the end point. Mice that received combination treatment with class II HER2-DC1 and anti-PD-1 antibodies in the absence of CD4 T cell depleting antibodies served as positive control. Control mice received isotype antibody control. As shown in Figure 8A, treatment with combination class II HER2-DC1 and anti-PD-1 antibody significantly delayed tumor growth compared to single treatments alone (p < 0.03) while combination therapy of class II HER2-DC1 with anti-PD-1 antibody further delayed tumor growth and survival (Figures 8A,B; p < 0.002). Depletion of CD4+ T cells led not only to the loss of anti-tumor effects mediated by the combination therapy with class II HER2-DC1 and anti-PD-1 but also to more rapid tumor growth and diminished survival. Nevertheless, class II HER2-DC1 vaccine failed to delay tumor growth in the absence of CD4+ T cells. Similar results were also observed when class II HER2-DC1 vaccine was combined with anti-PD1 antibody in the absence of CD4+ T cells (Figure 8A). This data strongly support a critical role of class II HER2-DC1 vaccine in mediating CD4+ Th1 immune responses.
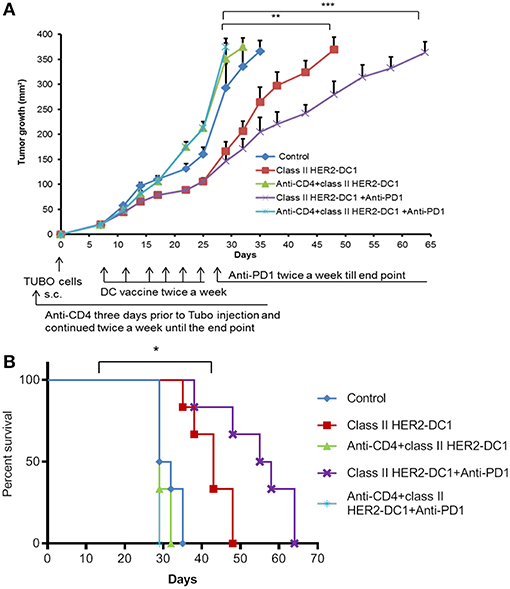
Figure 8. Depletion of CD4+ T cells abrogates the anti-tumor efficacy of HER2-DC1 vaccine and anti-PD1 antibody therapy. (A) Balb/c mice were injected s.c. with TUBO cells and treated with class II HER2-DC1 vaccine (1 × 106 DC1/mouse/100 μl, twice a week for 3 weeks) with or without anti-PD1 antibody (150 mg/200 ml/mouse/intraperitoneally twice a week until the end point). CD4 depleting antibody (clone GK1.5) was administered 3 days prior to TUBO induction and continued twice a week until the end point. Tumor growth was monitored periodically. (B) Survival curve. Data shown are representative from three independent experiments and are shown as the mean number ± SEM. P-values were determined by unpaired student t-test (A) or a log-rank test (B). ***p < 0.001, **p < 0.01, *p < 0.05.
Synergistic Effect of HER2 Targeted Therapy in Combination With Class II HER2-DC1 Vaccine and PD-1 Blockade Delays Tumor Growth and Enhance Survival Rate
In a PANACEA trial, Pembrolizumab plus trastuzumab showed a clinical benefit in patients with PD-L1-positive, trastuzumab-resistant, advanced, HER2+ breast cancer, and in patients with increased TIL. However, the trial did not show any clinical benefit in the PD-L1 negative cohort (18). This trial suggests the importance of immune mechanism in trastuzumab resistance populations, PD-L1 expression on tumors, and TIL infiltration. Since we observed increased TIL infiltration following HER-2 DC1 vaccination and higher levels of PD-1 expression on TIL, we investigated whether combination with anti-HER2 antibodies, class II HER2-DC1 vaccine, and anti-PD-1 therapy have an impact on tumor burden in the TUBO breast cancer model. TUBO cells (30,000 cells) were injected in the mammary fat pad on day 0 and when tumors were palpable, mice received anti-HER2 antibody once a week for 3 weeks or in combination with class II HER2-DC1 given twice a week for 3 weeks. Upon completion of combination treatment, anti-PD-1 antibody was given twice a week until the end point as described in Materials and Methods section. The treatment schema is outlined in Figure 9A. As shown in Figure 9B, blockade of HER2 overexpression using anti-rat neu antibodies (clones 7.16.4 and 7.9.5) significantly reduced tumor burden when combined with class II HER2-DC1 and anti-PD-1 antibody (Figure 9; p < 0.001). The class II HER2-DC1 vaccine treatment alone significantly delayed tumor growth compared to TUBO bearing control mice and doubled the survival rate. We observed no significant effect in delaying tumor growth in TUBO bearing mice treated with anti-PD-1 antibody alone while anti-rat neu antibody had minimal effect in delaying tumor growth. The combination of class II HER2-DC1 alone with anti-rat neu antibodies 7.16.4 and 7.6.5 did not have any significant delay in tumor growth compared to class II HER2-DC1 alone. Interestingly, the addition of anti-PD-1 antibody with monoclonal anti-rat neu antibodies 7.16.4 and 7.6.5 and class II HER2-DC1 vaccine not only significantly delayed the tumor growth but also enhanced and quadrupled the survival rate from control mice (Figures 9B,C). This data suggest that addition of HER2 targeted therapy to the combination of HER2-DC1 vaccine and anti-PD-1 antibody reduces tumor burden and improves survival rate in an orthotopic HER2 positive breast cancer model.
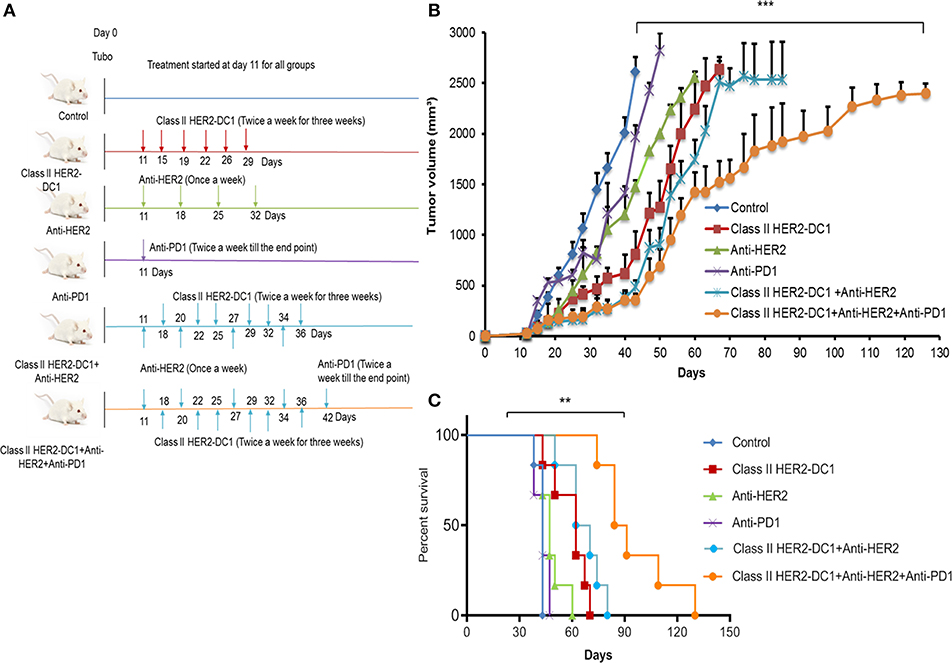
Figure 9. HER2 targeted therapy in combination with HER2-DC1 vaccine and anti-PD-1 therapy delays tumor growth and improves survival. (A) The treatment schema. (B) Balb/C mice were injected with 3 × 104 TUBO cells into the mammary fat pad to create orthotopic primary tumors. TUBO bearing mice with orthotopic primary tumor were treated with class II HER2 peptide pulsed DC1 vaccine (1 × 106 DC1/mouse/100 μl, twice a week for 3 weeks), anti-PD-1 antibody (two times per week) or the combination of HER2 targeted monoclonal antibodies 7.16.4 and 7.6.5 (once in a week) and class II HER2 peptide pulsed DC1 vaccine followed up with anti-PD-1 antibody twice a week. (C) Survival curve. Data shown are representative from three independent experiments and are shown as the mean number ± SEM. P-values were determined by unpaired student t-test (B) or a log-rank test (C). ***p < 0.001, **p < 0.01.
Discussion
HER2 overexpression/amplification accounts for about 25% of breast cancers and is associated with aggressive disease and poor clinical prognosis. As previously discussed, the humanized monoclonal antibodies (trastuzumab and pertuzumab) directed against HER2 in combination with chemotherapy have been demonstrated to be clinically effective for the treatment of patients with HER2+ MBC (41). Other therapeutic options for these patients include combinations of Lapatinib (an oral tyrosine kinase inhibitor), T-DM1, and chemotherapies (42). It should be emphasized however that patients with HER2+ MBC will eventually face disease progression while receiving treatment with currently approved HER2-targeted therapies. Development of new treatments is an obvious unmet clinical need not only for patients with de novo MBC but also for the subset of patients with HER2+ resectable breast cancer who will have MBC recurrence despite multimodality treatment (43, 44). Therefore, effective therapy to overcome resistance and improve the clinical response in metastatic patients is warranted.
In this study, for the first time we describe the anti-tumor efficacy of MHC class I HER2 peptide p66 and class II HER2 peptides (p5, p435, and p1209) pulsed DC1 vaccine alone or in combination with immune checkpoint blockade and HER2-targeted therapy in a preclinical model of HER2 over expressing breast cancer. Vaccination with class I HER2-DC1 or class II HER2-DC1 generated anti-HER2 Th1 immune responses and delayed tumor growth. In contrast, there was no enhanced anti-tumor efficacy in mice vaccinated with both class I and class II HER2 peptide pulsed DC1 compared to mice vaccinated with class I or class II HER2-DC1 alone. While class I HER2-DC1 enhanced only CD8+ T cell infiltration but not CD4+ T cells, class II HER2-DC1 vaccination induced both CD4 and CD8+ T cell infiltration, suggesting that vaccination with class II HER2-DC1 may be sufficient in generating anti-tumor immunity and HER2-specific immune responses. Re-stimulation of splenocytes from vaccinated mice with class II HER2 peptides led to increased IFN-γ production, suggesting the critical role of the anti-HER2 CD4+ Th1 immune response in mediating reduction in tumor burden with enhanced survival benefit. IFN-γ is recognized as a key cytokine in mediating CD4+ and CD8+ Th1 immune responses (45, 46). We have previously shown that TLR-4 activated DC1 inhibit CD4+CD25+FoxP3+ regulatory T cells and converts them in to Th1 like effector cells. These Th1 like effector cells co-expressed T-bet and increased IFN-γ production. This suggests the critical role of DC1 in mediating CD4+ Th1 immune responses (47). A recent study from our lab reported that IFN-γ eliminates HER2 expressing breast cancer cells through JAK-STAT-1 dependent induction of senescence and apoptosis (48). Our preclinical findings corroborate the clinical trial data showing that treatment of HER2 positive invasive breast cancer patients with HER2 peptide-pulsed DC1 vaccine resulted in successful restoration of anti-HER2 Th1 immune response with improved pCR (28, 31, 49).
Various clinical findings have shown that HER2 expressing breast cancer cells in the tumor microenvironment utilize the PD-1/PD-L1 dominant immune checkpoint pathway to down regulate anti-tumor immune cells function and evade immune cells mediated tumor eradication (50, 51). Importantly, PD-L1, a ligand for PD-1 is constitutively expressed on HER2 overexpressing breast cancer (52). Despite the reports that monoclonal antibodies that directly inhibit PD-1 and PD-L1 have been a successful treatment options for advanced melanoma, non-small cell lung carcinoma (NSCLC), and some patients with high mutational burden like HNPCC colorectal cancer, limited success rate was noted in breast cancer clinical trials (53). The combination of trastuzumab and pembrolizumab in HER2 resistant advanced breast cancer patients had modest clinical benefit only in the PD-L1 positive cohort (18) or in those with at least some TIL infiltration and no benefit was seen in the PD-L1 negative cohort.
Our preclinical findings suggest that both class I and class II HER2-DC1 increased infiltration of TIL which express high levels of PD-1 receptor. Class I HER2-DC1 vaccine given sequentially in combination with anti-PD-1 antibody therapy had an impact in reducing tumor burden with improved survival benefit. In contrast, HER2-DC1 vaccine and anti-PD-1 antibody given concurrently did not generate any synergistic effect. These results corroborate with a recent study indicating that in MMTV-PyMT mammary cancer model, concurrent treatment with anti-PD1 antibody and anti-OX-40 diminished the therapeutic efficacy. However, sequential treatment with anti-PD-1 and anti-OX-40 resulted in improved therapeutic efficacy and was associated with worse outcomes and increased T cell apoptosis (54). This supports our data suggesting that sequence and timing of checkpoint blockade is critical for combinatorial strategies. The optimal impact of checkpoint antibody treatment on tumor growth and resistance also likely depends in part on the tumor burden. A recent study demonstrated the anti-tumor efficacy of anti-CTLA-4 and anti-PD-1 in the low tumor burden state in pre-clinical melanoma model as well as in melanoma patients. Their data suggest that in the low tumor burden setting, combination therapy induced higher levels of IFN-γ receptor on activated tumor specific T cells which were more susceptible to apoptosis than naïve T cells. In this setting, combination therapy induced deletion of tumor-specific T cells and altered the T cell repertoire compared to the high tumor burden setting. The authors suggest that there is a less exhausted immune status in the low tumor burden state. In addition, they suggest that in the setting of high tumor burden, duration of antigen exposure and antigen loads could alter or reprogram the exhaustion status of T cell profile (55). We believe that in our model, one possible reason for the difference in response to checkpoint therapy could be that mice receiving concurrent HER2-DC1 and anti-PD-1 antibody treatment in the low tumor burden setting made anti-PD-1 therapy less effective. While in the mice receiving sequential treatment, where anti-PD-1 therapy was given in a setting of higher tumor burden, treatment was more effective. Altogether our results indicate that the effect of checkpoint blockade largely depends on optimal scheduling to be successful with other immunotherapeutic strategies.
Class II HER2-DC1, when combined with anti-PD-1 antibody, quadrupled the survival rate with increased anti-HER2 CD4+ Th1 immune response and increased CD4+ and CD8+ T cells infiltration within the tumor. Interestingly, depletion of CD4+ T cells completely abrogated the anti-tumor efficacy of the class II HER2-DC1 alone or in combination with anti-PD-1 therapy suggesting the crucial role of CD4+ T cells. A recent study indicates that NSCLC patients with highly dysfunctional CD4 immunity had no objective response to PD-1/PD-L1 blockade therapy. However, in patients with non-dysfunctional CD4 responses there was a response rate of about 50% to PD-1/PD-L1 blockade therapy. More importantly, CD8 immunity was recovered only in patients with functional CD4 immunity (56). These data support our findings that boosting the anti-HER2 CD4+ Th1 immune responses prior to immune checkpoint blockade will be beneficial in breast cancer patients.
In contrast, no effect on delaying tumor growth was observed when class I or class II HER2-DC1 vaccine was combined with anti-PD-L1 antibody. In the clinical setting, Avelumab, a PD-L1- antibody, has been shown to have only modest responses in breast cancer subtypes in the JAVELIN study (57). It is well-known that IFN-γ upregulates PD-L1 expression (45). Despite the fact that HER2+ TUBO cells expressed higher levels of PD-L1, we were unable to observe synergy with anti-PD-L1 antibody and HER2-DC1 vaccine. Further studies are warranted on the optimal dosing and scheduling of anti-PD-L1 antibody and to validate its efficacy in combination with HER2-DC1 vaccine.
Our data suggests that addition of anti-HER2 antibodies further enhanced the efficacy of class II HER2-DC1 vaccine in combination with anti-PD-1 antibody, with a prolonged survival advantage. In a HER2 overexpressing and trastuzumab resistant preclinical breast cancer model, targeted treatment with ado-trastuzumab emtansine (T-DM1) in combination with anti-PD1 antibody or anti-CTLA-4 antibody showed enhanced anti-tumor efficacy, T cells trafficking in to the tumor and Th1 cell polarization (58). Preclinical studies combining IFN-γ and anti-HER2 antibody have been shown to induce a synergistic effect in reducing HER2 expressing orthotopic mammary tumor growth in vivo (14). Taken together, this study highlights the critical role of CD4+ T cells and the use of class II HER2-DC1 vaccine in combination with immune checkpoint blockade and HER2 targeted therapy in facilitating and mediating anti-HER2 Th1 immune responses. This combinatorial approach could be directly translated to clinical settings.
Data Availability
The datasets generated for this study are available on request to the corresponding author.
Author Contributions
KK and BC conceived and designed the experiments and supervised the work. KK, CS, GR, AB, YJ, HZ, and LL performed the experiments. KK, CS, GR, and BC analyzed data and contributed to data analysis. KK, BC, and RC wrote the manuscript. GR, CS, DW, APB, SA, AB, LL, HZ, MG, RC, and YJ edited the manuscript.
Funding
This work has been supported by Pennies in action and Henle foundation grant to BC. This work was also supported by breast cancer research foundation to MIG. This work was supported in part by the Flow Cytometry, Analytic Microscopy Core Facility, and animal Core Facility at the H. Lee Moffitt Cancer Center & Research Institute; an NCI designated Comprehensive Cancer Center (P30-CA076292). The funders had no role in study design, data collection and analysis, decision to publish, or preparation of the manuscript.
Conflict of Interest Statement
The authors declare that the research was conducted in the absence of any commercial or financial relationships that could be construed as a potential conflict of interest.
Abbreviations
BM, Bone marrow; BSA, bovine serum albumin; Class I HER2-DC1, Class I HER2 pulsed DC1 vaccine; Class II HER2-DC1, Class II HER2 peptide pulsed DC1; DAPI, 4′6-diamindino-2-phenylindole; DC1, Type I polarized dendritic cells; FMO, Fluorescent minus one; iDC, Immature DC; LPS, lipopolysaccharide; MBC, Metastatic HER2 breast cancer; mOS, Median overall survival; NSCLC, Non- small cell lung carcinoma; PD-1, Programmed death 1 receptor; PD-L1, Programmed cell death 1 ligand 1; PVDF, Polyvinylidene difluoride; rHER2, rat HER2 oncogene/peptide; s.c., Subcutaneously; T-DM1, Ado-trastuzumab/ado-trastuzumab emtansine; Th1, T helper cell; TIL, Tumor infiltrating lymphocyte.
References
1. Siegel RL, Miller KD, Jemal A. Cancer statistics, 2017. CA Cancer J Clin. (2017) 67:7–30. doi: 10.3322/caac.21387
2. Wolff M, Hammond EH, Allison KH, Harvey BE, Mangu PB, Bartlett JMS, et al. Human epidermal growth factor receptor 2 testing in breast cancer: American Society of Clinical Oncology/College of American Pathologists Clinical Practice Guideline Focused Update. J Clin Oncol. (2018) 36:2105–22. doi: 10.1200/JCO.2018.77.8738
3. Hammond ME, Hayes DF, Wolff AC, Mangu PB, Temin S. American society of clinical oncology/college of American pathologists guideline recommendations for immunohistochemical testing of estrogen and progesterone receptors in breast cancer. J Oncol Pract. (2010) 6:195–7. doi: 10.1200/JOP.777003
4. Burstein HJ. The distinctive nature of HER2-positive breast cancers. N Engl J Med. (2005) 353:1652–4. doi: 10.1056/NEJMp058197
5. Slamon J, Leyland-Jones B, Shak S, Fuchs H, Paton V, Bajamonde A, et al. Use of chemotherapy plus a monoclonal antibody against HER2 for metastatic breast cancer that overexpresses HER2. N Engl J Med. (2001) 344:783–92. doi: 10.1056/NEJM200103153441101
6. Swain SM, Baselga J, Kim SB, Ro J, Semiglazov V, Campone M, et al. Pertuzumab, trastuzumab, and docetaxel in HER2-positive metastatic breast cancer. N Engl J Med. (2015) 372:724–34. doi: 10.1056/NEJMoa1413513
7. Dieras V, Miles D, Verma S, Pegram M, Welslau M, Baselga J, et al. Trastuzumab emtansine versus capecitabine plus lapatinib in patients with previously treated HER2-positive advanced breast cancer (EMILIA): a descriptive analysis of final overall survival results from a randomised, open-label, phase 3 trial. Lancet Oncol. (2017) 18:732–42. doi: 10.1016/S1470-2045(17)30312-1
8. Perez EA, Ballman KV, Tenner KS, Thompson EA, Badve SS, Bailey H, et al. Association of stromal tumor-infiltrating lymphocytes with recurrence-free survival in the N9831 adjuvant trial in patients with early-stage HER2-positive breast cancer. JAMA Oncol. (2016) 2:56–64. doi: 10.1001/jamaoncol.2015.3239
9. Loi S, Sirtaine N, Piette F, Salgado R, Viale G, Van Eenoo F, et al. Prognostic and predictive value of tumor-infiltrating lymphocytes in a phase III randomized adjuvant breast cancer trial in node-positive breast cancer comparing the addition of docetaxel to doxorubicin with doxorubicin-based chemotherapy: BIG 02-98. J Clin Oncol. (2013) 31:860–7. doi: 10.1200/JCO.2011.41.0902
10. Ingold Heppner B, Untch M, Denkert C, Pfitzner BM, Lederer B, Schmitt W, et al. Tumor-infiltrating lymphocytes: a predictive and prognostic biomarker in neoadjuvant-treated HER2-positive breast cancer. Clin Cancer Res. (2016) 22:5747–54. doi: 10.1158/1078-0432.CCR-15-2338
11. Mahmoud SM, Paish EC, Powe DG, MacMillan RD, Grainge MJ, Lee AH, et al. Tumor-infiltrating CD8+ lymphocytes predict clinical outcome in breast cancer. J Clin Oncol. (2011) 29:1949–55. doi: 10.1200/JCO.2010.30.5037
12. West NR, Milne K, Truong PT, Macpherson N, Nelson BH, Watson PH. Tumor-infiltrating lymphocytes predict response to anthracycline-based chemotherapy in estrogen receptor-negative breast cancer. Breast Cancer Res. (2011) 13:R126. doi: 10.1186/bcr3072
13. Park S, Jiang Z, Mortenson ED, Deng L, Radkevich-Brown O, Yang X, et al. The therapeutic effect of anti-HER2/neu antibody depends on both innate and adaptive immunity. Cancer Cell. (2010) 18:160–70. doi: 10.1016/j.ccr.2010.06.014
14. Nagai Y, Tsuchiya H, Runkle EA, Young PD, Ji MQ, Norton L, et al. Disabling of the erbB pathway followed by IFN-γ modifies phenotype and enhances genotoxic eradication of breast tumors. Cell Rep. (2015) 12:2049–59. doi: 10.1016/j.celrep.2015.08.044
15. Ostrand-Rosenberg S, Horn LA, Haile ST. The programmed death-1 immune-suppressive pathway: barrier to antitumor immunity. J Immunol. (2014) 193:3835–41. doi: 10.4049/jimmunol.1401572
16. Zhao Y, Harrison DL, Song Y, Ji J, Huang J, Hui E. Antigen-presenting cell-intrinsic PD-1 neutralizes PD-L1 in cis to attenuate PD-1 signaling in T cells. Cell Rep. (2018) 24:379–90e6. doi: 10.1016/j.celrep.2018.06.054
17. Stagg J, Loi S, Divisekera U, Ngiow SF, Duret H, Yagita H, et al. Smyth: Anti-ErbB-2 mAb therapy requires type I and II interferons and synergizes with anti-PD-1 or anti-CD137 mAb therapy. Proc Natl Acad Sci USA. (2011) 108:7142–7. doi: 10.1073/pnas.1016569108
18. Loi S, Giobbie-Hurder A, Gombos A, Bachelot T, Hui R, Curigliano G, et al. Pembrolizumab plus trastuzumab in trastuzumab-resistant, advanced, HER2-positive breast cancer (PANACEA): a single-arm, multicentre, phase 1b-2 trial. Lancet Oncol. (2019) 20:371–82. doi: 10.1016/S1470-2045(18)30812-X
19. Chia SKL, Bedard PL, Hilton J, Amir E, Gelmon KA, Anne Goodwin R, et al. A phase I study of a PD-L1 antibody (Durvalumab) in combination with trastuzumab in HER-2 positive metastatic breast cancer (MBC) progressing on prior anti HER-2 therapies (CCTG IND.229)[NCT02649686]. J Clin Oncol. (2018) 36(15_Suppl.):1029. doi: 10.1200/JCO.2018.36.15_suppl.1029
20. Datta J, Terhune JH, Lowenfeld L, Cintolo JA, Xu S, Roses RE, et al. Optimizing dendritic cell-based approaches for cancer immunotherapy. Yale J Biol Med. (2014) 87:491–518.
21. Cintolo JA, Datta J, Mathew SJ, Czerniecki BJ. Dendritic cell-based vaccines: barriers and opportunities. Future Oncol. (2012) 8:1273–99. doi: 10.2217/fon.12.125
22. Abiko K, Matsumura N, Hamanishi J, Horikawa N, Murakami R, Yamaguchi K, et al. IFN-γ from lymphocytes induces PD-L1 expression and promotes progression of ovarian cancer. Br J Cancer. (2015) 112:1501–9. doi: 10.1038/bjc.2015.101
23. Rice E, Latchman YE, Balint JP, Lee JH, Gabitzsch ES, Jones FR. An HPV-E6/E7 immunotherapy plus PD-1 checkpoint inhibition results in tumor regression and reduction in PD-L1 expression. Cancer Gene Ther. (2015) 22:454–62. doi: 10.1038/cgt.2015.40
24. Antonios JP, Soto H, Everson RG, Orpilla J, Moughon D, Shin N, et al. PD-1 blockade enhances the vaccination-induced immune response in glioma. JCI Insight. (2016) 1:e87059. doi: 10.1172/jci.insight.87059
25. Fisk B, Blevins TL, Wharton JT, Ioannides CG. Identification of an immunodominant peptide of HER-2/neu protooncogene recognized by ovarian tumor-specific cytotoxic T lymphocyte lines. J Exp Med. (1995) 181:2109–17. doi: 10.1084/jem.181.6.2109
26. Murray JL, Gillogly ME, Przepiorka D, Brewer H, Ibrahim NK, Booser DJ, et al. Toxicity, immunogenicity, and induction of E75-specific tumor-lytic CTLs by HER-2 peptide E75 (369-377) combined with granulocyte macrophage colony-stimulating factor in HLA-A2+ patients with metastatic breast and ovarian cancer. Clin Cancer Res. (2002) 8:3407–18.
27. Zaks TZ, Rosenberg SA. Immunization with a peptide epitope (p369-377) from HER-2/neu leads to peptide-specific cytotoxic T lymphocytes that fail to recognize HER-2/neu+ tumors. Cancer Res. (1998) 58:4902–8.
28. Datta J, Rosemblit C, Berk E, Showalter L, Namjoshi P, Mick R, et al. Progressive loss of anti-HER2 CD4(+) T-helper type 1 response in breast tumorigenesis and the potential for immune restoration. Oncoimmunology. (2015) 4:e1022301. doi: 10.1080/2162402X.2015.1022301
29. De La Cruz LM, Nocera NF, Czerniecki BJ. Restoring anti-oncodriver Th1 responses with dendritic cell vaccines in HER2/neu-positive breast cancer: progress and potential. Immunotherapy. (2016) 8:1219–32. doi: 10.2217/imt-2016-0052
30. Lowenfeld L, Zaheer S, Oechsle C, Fracol M, Datta J, Xu S, et al. Addition of anti-estrogen therapy to anti-HER2 dendritic cell vaccination improves regional nodal immune response and pathologic complete response rate in patients with ER(pos)/HER2(pos) early breast cancer. Oncoimmunology. (2017) 6:e1207032. doi: 10.1080/2162402X.2016.1207032
31. Sharma A, Koldovsky U, Xu S, Mick R, Roses R, Fitzpatrick E, et al. HER-2 pulsed dendritic cell vaccine can eliminate HER-2 expression and impact ductal carcinoma in situ. Cancer. (2012) 118:4354–62. doi: 10.1002/cncr.26734
32. Rovero S, Amici A, Di Carlo E, Bei R, Nanni P, Quaglino E, et al. DNA vaccination against rat her-2/Neu p185 more effectively inhibits carcinogenesis than transplantable carcinomas in transgenic BALB/c mice. J Immunol. (2000) 165:5133–42. doi: 10.4049/jimmunol.165.9.5133
33. Cintolo JA, Datta J, Xu S, Gupta M, Somasundaram R, Czerniecki BJ. Type I-polarized BRAF-pulsed dendritic cells induce antigen-specific CD8+ T cells that impact BRAF-mutant murine melanoma. Melanoma Res. (2016) 26:1–11. doi: 10.1097/CMR.0000000000000203
34. Jalali SA, Sankian M, Tavakkol-Afshari J, Jaafari MR. Induction of tumor-specific immunity by multi-epitope rat HER2/neu-derived peptides encapsulated in LPD Nanoparticles. Nanomedicine. (2012) 8:692–701. doi: 10.1016/j.nano.2011.09.010
35. Pouyanfard S, Bamdad T, Hashemi H, Bandehpour M, Kazemi B. Induction of protective anti-CTL epitope responses against HER-2-positive breast cancer based on multivalent T7 phage nanoparticles. PLoS ONE. (2012) 7:e49539. doi: 10.1371/journal.pone.0049539
36. Kodumudi KN, Siegel J, Weber AM, Scott E, Sarnaik AA, Pilon-Thomas S. Immune checkpoint blockade to improve tumor infiltrating lymphocytes for adoptive cell therapy. PLoS ONE. (2016) 11:e0153053. doi: 10.1371/journal.pone.0153053
37. Salgado R, Denkert C, Demaria S, Sirtaine N, Klauschen F, Pruneri G, et al. The evaluation of tumor-infiltrating lymphocytes (TILs) in breast cancer: recommendations by an International TILs Working Group 2014. Ann Oncol. (2015) 26:259–71. doi: 10.1093/annonc/mdu450
38. Denkert C, Wienert S, Poterie A, Loibl S, Budczies J, Badve S, et al. Standardized evaluation of tumor-infiltrating lymphocytes in breast cancer: results of the ring studies of the international immuno-oncology biomarker working group. Mod Pathol. (2016) 29:1155–64. doi: 10.1038/modpathol.2016.109
39. McArthur HL, Diab A, Page DB, Yuan J, Solomon SB, Sacchini V, et al. A pilot study of preoperative single-dose ipilimumab and/or cryoablation in women with early-stage breast cancer with comprehensive immune profiling. Clin Cancer Res. (2016) 22:5729–37. doi: 10.1158/1078-0432.CCR-16-0190
40. Ali HR, Provenzano E, Dawson SJ, Blows FM, Liu B, Shah M, et al. Association between CD8+ T-cell infiltration and breast cancer survival in 12,439 patients. Ann Oncol. (2014) 25:1536–43. doi: 10.1093/annonc/mdu191
41. Gingras I, Gebhart G, de Azambuja E, Piccart-Gebhart M. HER2-positive breast cancer is lost in translation: time for patient-centered research. Nat Rev Clin Oncol. (2017) 14:669–81. doi: 10.1038/nrclinonc.2017.96
42. Pernas S, Tolaney SM. HER2-positive breast cancer: new therapeutic frontiers and overcoming resistance. Ther Adv Med Oncol. (2019) 11:1758835919833519. doi: 10.1177/1758835919833519
43. de Azambuja E, Holmes AP, Piccart-Gebhart M, Holmes E, Di Cosimo S, Swaby RF, et al. Lapatinib with trastuzumab for HER2-positive early breast cancer (NeoALTTO): survival outcomes of a randomised, open-label, multicentre, phase 3 trial and their association with pathological complete response. Lancet Oncol. (2014) 15:1137–46. doi: 10.1016/S1470-2045(14)70320-1
44. von Minckwitz G, Procter M, de Azambuja E, Zardavas D, Benyunes M, Viale G, et al. Adjuvant pertuzumab and trastuzumab in early HER2-positive breast cancer. N Engl J Med. (2017) 377:122–31. doi: 10.1056/NEJMoa1703643
45. Mandai M, Hamanishi J, Abiko K, Matsumura N, Baba T, Konishi I. Dual faces of IFNγ in cancer progression: a role of PD-L1 induction in the determination of pro- and antitumor immunity. Clin Cancer Res. (2016) 22:2329–34. doi: 10.1158/1078-0432.CCR-16-0224
46. Zhang H, Lam L, Nagai Y, Zhu Z, Chen X, Ji MQ, et al. A targeted immunotherapy approach for HER2/neu transformed tumors by coupling an engineered effector domain with interferon-gamma. Oncoimmunology. (2018) 7:e1300739. doi: 10.1080/2162402X.2017.1300739
47. Amiri-Kordestani L, Blumenthal GM, Xu QC, Zhang L, Tang SW, Ha L, et al. FDA approval: ado-trastuzumab emtansine for the treatment of patients with HER2-positive metastatic breast cancer. Clin Cancer Res. (2014) 20:4436–41. doi: 10.1158/1078-0432.CCR-14-0012
48. Rosemblit C, Datta J, Lowenfeld L, Xu S, Basu A, Kodumudi K, et al. Oncodriver inhibition and CD4(+) Th1 cytokines cooperate through Stat1 activation to induce tumor senescence and apoptosis in HER2+ and triple negative breast cancer: implications for combining immune and targeted therapies. Oncotarget. (2018) 9:23058–77. doi: 10.18632/oncotarget.25208
49. Datta J, Xu S, Rosemblit C, Smith JB, Cintolo JA, Powell DJ Jr, et al. CD4(+) T-helper type 1 cytokines and trastuzumab facilitate CD8(+) T-cell targeting of HER2/neu-expressing cancers. Cancer Immunol Res. (2015) 3:455–63. doi: 10.1158/2326-6066.CIR-14-0208
50. Meng X, Huang Z, Teng F, Xing L, Yu J. Predictive biomarkers in PD-1/PD-L1 checkpoint blockade immunotherapy. Cancer Treat Rev. (2015) 41:868–76. doi: 10.1016/j.ctrv.2015.11.001
51. Topalian SL, Taube JM, Anders RA, Pardoll DM. Mechanism-driven biomarkers to guide immune checkpoint blockade in cancer therapy. Nat Rev Cancer. (2016) 16:275–87. doi: 10.1038/nrc.2016.36
52. Wimberly H, Brown JR, Schalper K, Haack H, Silver MR, Nixon C, et al. PD-L1 expression correlates with tumor-infiltrating lymphocytes and response to neoadjuvant chemotherapy in breast cancer. Cancer Immunol Res. (2015) 3:326–32. doi: 10.1158/2326-6066.CIR-14-0133
53. Savas P, Salgado R, Denkert C, Sotiriou C, Darcy PK, Smyth MJ, et al. Clinical relevance of host immunity in breast cancer: from TILs to the clinic. Nat Rev Clin Oncol. (2016) 13:228–41. doi: 10.1038/nrclinonc.2015.215
54. Messenheimer DJ, Jensen SM, Afentoulis ME, Wegmann KW, Feng Z, Friedman DJ, et al. Timing of PD-1 blockade is critical to effective combination immunotherapy with anti-OX40. Clin Cancer Res. (2017) 23:6165–77. doi: 10.1158/1078-0432.CCR-16-2677
55. Pai CS, Huang JT, Lu X, Simons DM, Park C, Chang A, et al. Clonal deletion of tumor-specific T cells by interferon-γ confers therapeutic resistance to combination immune checkpoint blockade. Immunity. (2019) 50:477–92.e8. doi: 10.1016/j.immuni.2019.01.006
56. Zuazo M, Arasanz H, Fernandez-Hinojal G, Garcia-Granda MJ, Gato M, Bocanegra A, et al. Functional systemic CD4 immunity is a differential factor for clinical responses to PD-L1/PD-1 blockade therapy in lung cancer. bioRxiv. (2019) 508739. doi: 10.1101/508739
57. Dirix Y, Takacs I, Jerusalem G, Nikolinakos P, Arkenau HT, Forero-Torres A, et al. Avelumab, an anti-PD-L1 antibody, in patients with locally advanced or metastatic breast cancer: a phase 1b JAVELIN Solid Tumor study. Breast Cancer Res Treat. (2018) 167:671–86. doi: 10.1007/s10549-017-4537-5
Keywords: breast cancer, dendritic cells, PD-1, PD-L1, HER2, immune checkpoints, CD4 T cells, Th1
Citation: Kodumudi KN, Ramamoorthi G, Snyder C, Basu A, Jia Y, Awshah S, Beyer AP, Wiener D, Lam L, Zhang H, Greene MI, Costa RLB and Czerniecki BJ (2019) Sequential Anti-PD1 Therapy Following Dendritic Cell Vaccination Improves Survival in a HER2 Mammary Carcinoma Model and Identifies a Critical Role for CD4 T Cells in Mediating the Response. Front. Immunol. 10:1939. doi: 10.3389/fimmu.2019.01939
Received: 21 May 2019; Accepted: 31 July 2019;
Published: 14 August 2019.
Edited by:
Riccardo Dolcetti, University of Queensland, AustraliaReviewed by:
Alexandre Corthay, Oslo University Hospital, NorwayMaría Marcela Barrio, Fundación Cáncer, Argentina
Copyright © 2019 Kodumudi, Ramamoorthi, Snyder, Basu, Jia, Awshah, Beyer, Wiener, Lam, Zhang, Greene, Costa and Czerniecki. This is an open-access article distributed under the terms of the Creative Commons Attribution License (CC BY). The use, distribution or reproduction in other forums is permitted, provided the original author(s) and the copyright owner(s) are credited and that the original publication in this journal is cited, in accordance with accepted academic practice. No use, distribution or reproduction is permitted which does not comply with these terms.
*Correspondence: Brian J. Czerniecki, YnJpYW4uY3plcm5pZWNraSYjeDAwMDQwO21vZmZpdHQub3Jn