- 1Transplant Institute, Beth Israel Deaconess Medical Center, Harvard Medical School, Boston, MA, United States
- 2Center for Virology and Vaccines Research, Beth Israel Deaconess Medical Center, Harvard Medical School, Boston, MA, United States
- 3Viracor-Eurofins, Lee's Summit, MO, United States
- 4Division of Infectious Diseases, Beth Israel Deaconess Medical Center, Harvard Medical School, Boston, MA, United States
Background: A previously proposed immune risk profile (IRP), based on T cell phenotype and CMV serotype, is associated with mortality in the elderly and increased infections post-kidney transplant. To evaluate if NK cells contribute to the IRP and if the IRP can be predicted by a clinical T cell functional assays, we conducted a cross sectional study in renal transplant candidates to determine the incidence of IRP and its association with specific NK cell characteristics and ImmuKnow® value.
Material and Methods: Sixty five subjects were enrolled in 5 cohorts designated by age and dialysis status. We determined T and NK cell phenotypes by flow cytometry and analyzed multiple factors contributing to IRP.
Results: We identified 14 IRP+ [CMV seropositivity and CD4/CD8 ratio < 1 or being in the highest quintile of CD8+ senescent (28CD–/CD57+) T cells] individuals equally divided amongst the cohorts. Multivariable linear regression revealed a distinct IRP+ group. Age and dialysis status did not predict immune senescence in kidney transplant candidates. NK cell features alone could discriminate IRP– and IRP+ patients, suggesting that NK cells significantly contribute to the overall immune status in kidney transplant candidates and that a combined T and NK cell phenotyping can provide a more detailed IRP definition. ImmuKnow® value was negatively correlated to age and significantly lower in IRP+ patients and predicts IRP when used alone or in combination with NK cell features.
Conclusion: NK cells contribute to overall immune senescence in kidney transplant candidates.
Introduction
The number of elderly end stage renal disease (ESRD) patients in the United States continues to grow (1), as does the number of elderly patients listed for kidney transplant (2, 3). Several studies have shown impaired T-cell function in both ESRD (4–6) and elderly individuals (7, 8) and that these conditions compound one another (9, 10). Elderly recipients appear to have a lower risk of allograft rejection; however, they are at an increased risk for infectious complications after transplant. Lowering of immune suppressions to treat infections can often precipitate rejection episodes with detrimental outcomes (11–13), highlighting the fine line that must be walked in immune suppressing such patients.
Changes in T-cell phenotype and function known as T-cell senescence have been associated with mortality in the elderly (14), increased risk of infection (15, 16), and cardiovascular disease (17, 18). While some studies also associated T- cell senescence with a decreased risk for acute kidney rejection post-transplant (19–21), others have found differentiated T cells as biomarkers for acute rejection in a subgroup of patients (22) and long-term graft dysfuction in another group (23). Senescence is characterized by phenotypic changes including low CD4/CD8 ratio, reduced naïve and increased differentiated memory T cells (24), and the accumulation of terminally differentiated and highly cytotoxic CD28–/CD57+ T cells (25), as well as functional deficits including decreased responsiveness to stimulation and reduced proliferative capacity (9). Through chronic antigenic exposure, cytomegalovirus (CMV) appears to be a major driver of this process (26), however, age (8, 27) and chronic kidney disease (CKD) (28–30) have also been associated with similar changes, which are not reversed by kidney transplantation (31). An immune risk profile (IRP) has been proposed which has been associated with increased infectious complications post-transplant and mortality in the elderly (32–34). The IRP has been variably defined to include many of the above findings of immune senescence.
Natural killer (NK) cells represent early effector cells of the innate immune system and serve as the first line of defense against nascent neoplasms or viral infections. Similar to T cells, NK cells are affected by CMV, age (35) and the uremic state. Yet there is scarce evidence that age or CKD directly alters NK cell phenotype or compromises NK cell function. Healthy elderly present slightly enhanced proportions of NK cells, mostly driven by an increase in the more mature cytotoxic CD56dim NK cell subset (36–41), while frequencies of the immature CD56bright NK cell subset decrease with age (42). Functionally, diminished NK cell cytotoxicity has been reported in the elderly (39), and expression of the inhibitory receptor KLRG1 on mature CD56dim NK cells increases with age (43). CD57 expression also serves as a marker of terminal differentiation in NK cells (44–46). Proportions of CD57+ NK cells are enhanced in the elderly. However, the direct effects of aging on NK cells are difficult to discriminate from those mediated by CMV infection, which significantly reshapes the NK cell repertoire. In particular, CMV replication promotes the expansion of NK cells expressing the activating NKG2C receptor (47–51). CD57 is frequently co-expressed on the NKG2C+ NK cell subset, and surface expression of both receptors has been associated with antigen-experienced and mature NK cells (51). Accordingly, CD57 and NKG2C are co-expressed on a significant portion of the memory-like NK cell subset lacking the FcR intracellular gamma signaling chain (FcγRΔg NK cells) and endowed with significantly enhanced antibody-dependent effector functions (52–54). Expression of other cell surface receptors on NK cells, such as CD27, has been proposed to mark functionally distinct subsets of NK cells, yet whether their expression reflects specific stages of human NK cell maturation remains unclear (55, 56). Furthermore, to date, little is known of NK cell contributions to IRP. Previous studies focusing on alterations of NK cells in patients with CKD report conflicting results, yet recent data strongly suggest a decrease in overall NK cell numbers in IRP+ (15) or dialyzed transplant candidates (57). However, phenotypic and functional characteristics of an optimal aged NK cell population associated with low viral reactivation and graft rejection remain unclear.
Lastly, determining IRP in a transplant candidate has the potential to better guide immune suppression after transplant. There are currently no clinical tests available to measure IRP. IRP has been previously determined based on a composite of serology and T cell phenotyping assays. We sought to determine if ImmuKnow®- an FDA approved clinical test for measuring overall T cell function in transplant recipients—can be used as a single clinical test that correlates closely to IRP to identify pre transplant patients at greater risk for infectious complications post-transplant.
Hypothesis/Objective
While lymphocyte immune senescence has been described in ESRD, CKD, and post-transplant populations, it must be noted that patients listed for kidney transplant, especially elderly listed patients, represent a highly select population. We hypothesized that in addition to NK cells significantly contribute to immune senescence, in addition to T lymphocytes. We therefore sought to evaluate whether distinct NK cell signatures might be used in combination with T cell profiles, chronologic age, and other clinical information as markers of immune senescence, and to test whether T-cell based IRP definitions also correlate with distinct NK cell signatures.
Materials and Methods
Patients
This study was approved by Beth Israel Deaconess Medical Center Institutional Review Board. At our institution, 65 years older are the ages when kidney transplant candidates are considered as elderly candidates. In total, 59 subjects were recruited into five groups: (1) Healthy controls, (2) <65 years of age with CKD not on dialysis, (3) ≥65 years of age with CKD not on dialysis, (4) <65 years of age on dialysis, (5) ≥65 years of age on dialysis. Healthy controls were defined as individuals aged >18 years old with an estimated glomerular filtration rate (eGFR) >60 and no albuminuria. CKD patients were defined as adults listed for kidney transplant, but not yet requiring renal replacement therapy. Dialysis patients were defined as adults listed for kidney transplant and currently requiring renal replacement therapy. Patients with active infections, known systemic autoimmune conditions, those who have previously received immunosuppressant therapy, chemotherapy or a prior transplant were excluded. Patients were approached at their Transplant clinic visits and consented. Blood was collected from each subject. Healthy controls were approached at their Pre surgical visits for their elective surgery procedures. The IRP was defined as CMV seropositivity and CD4/CD8 ratio <1 (58) or being in the highest quintile of CD8+ senescent (CD28–/CD57+) T cells (14).
Lymphocyte Phenotyping
A 100 μL aliquot of fresh whole blood was stained using a master mix of fluorescently-conjugated antibodies targeting the following human markers: CD3 (BD Biosciences SP34.2), CD20 (BD Biosciences L27), CD4 (BD Biosciences L200), CD8 (BD Biosciences RPA-T8), CD45RO (Beckman Coulter UHCL1), CD45RA (BD Biosciences 5H9), CD197/CCR7 (BD Biosciences 150503), CD28 (ThermoFisher 28.2), and CD57 (BD Biosciences NK-1). Following a 20 min/4°C staining incubation, automated red blood cell lysis was performed on the stained samples using a TQ-Prep™ Workstation (Beckman Coulter). Samples were then twice washed with 1X Dulbecco's Phosphate-Buffered Saline (Gibco), and subsequently fixed for cytometric analysis in 1.5% formaldehyde. Multicolor flow cytometry was then performed on the samples using a LSRII flow cytometer (BD Biosciences), and results were analyzed using FlowJo software (Treestar).
Specific cell populations were gated according to the following strategy: T-cells were identified using forward vs. side scatter, excluded of doublets, and further gated on CD3+ cells using a CD20 vs. CD3 plot to exclude B-cells. To gate CD3+/CD4+ and CD3+/CD8+ subpopulations most accurately, a Boolean gating strategy was designed directly off the CD3+ parent population. The effector cell subpopulation was defined for the CD3+/CD4+ and CD3+/CD8+ populations using Boolean gating for the CD3+/CD197+/CD45RA-/CD45RO+ cells. In a similar manner, the effector memory (EM), central memory (CM), and naive subpopulations of the CD3+/CD4+ and CD3+/CD8+ populations were defined using Boolean gating for the CD3+/197CD–/CD45RA–/CD45RO+, CD3+/CD197+/CD45RA–/CD45RO+, and CD3+/CD197+/CD45RA+/CD45RO– cells, respectively. Finally, senescent cells were defined for each group by applying Boolean gating for CD3+/28CD–/CD57+ cells to all subpopulations defined above.
To analyze NK cells, a 100 μL aliquot of fresh whole blood was stained using a master mix of fluorescently-conjugated antibodies. NK cells were defined as lymphocytes that were 3CD– (BioLegend UCHT1), 14CD– (BioLegend M5E2), and 19CD– (BioLegend HIB19). NK cell subpopulations were then identified based on their expression of CD56 (BioLegend HCD56) and/or CD16 (Pharmingen 3G8), namely bulk NK cells including CD56dim (3CD–CD56+CD16+), CD56bright (3CD–CD56+16CD–), and CD56neg (3CD−56CD–CD16+). Additional antibodies targeting the following human markers were used to assess their expression in NK cells: CD25 (eBioscience BC96), NKG2C (R&D Systems 134591), KLRG1 (BioLegend 2F1), CD2+ (Pharmingen RPA2.10), CD57 (BioLegend HCD57), CD27 (BioLegend 0323), FcεRIγ- (Millipore Sigma). Samples were fixed and analyzed using a LSRII flow cytometer (BD Biosciences), and data analyzed using FlowJo software 7.6.5 (Treestar).
Flow Cytometric Analysis of NK Cell Function
To quantify NK cell function, thawed peripheral blood mononuclear cells (PBMC)s were incubated at 1 × 106 cells/mL in RPMI-1640 supplemented with 10% fetal bovine serum, 2 mM L-glutamine, 100 mg/mL streptomycin, 100 U/mL penicillin, and 1 ng/mL rhIL-15 without stimulus or in the presence of 2 μg/mL CMV pp65 peptide pool (NIH AIDS reagents), 2 μg/mL BK virus capsid protein VP1 peptide pool (15 mer overlapping by 11, JPT), a combination of 50 ng/mL rhIL-12+100 ng/mL rhIL-18, HLA-I-deficient K562 or 721.221 cells at effector-to-target ratio of 10:1. To measure NK-cell mediated antibody-dependent cellular cytotoxicity (ADCC) function, p815 cells (a mouse leukemic cell line) were co-cultured with 1 mg/ml p815-specific antibody (Abcam) for 1 h and then washed twice. PBMCs were then stimulated with p815 cells or p815 cells plus p815-specific antibodies at an effector-to-target ratio of 10:1. PBMCs were stimulated for 6 h in the presence of 5 uL/mL CD107a-BV786 antibody (H4A3, BD Biosciences) and 1 μL/mL GolgiStop (monensin; BD Biosciences) prior to surface staining using the following anti-human monoclonal antibodies from BD Biosciences: CD3 (SP34-2), CD14 (M5E2), CD19 (HIB19), CD56 (B159), CD16 (3G8), fixed, permeabilized (BD Biosciences), and finally stained for intracellular interferon using IFN-γ-FITC (B27; BD Biosciences). Multiparameter flow cytometric analysis was performed on an LSRII instrument (BD Biosciences).
ImmuKnow® Assay
The ImmuKnow® assay was performed by Viracor-Eurofin laboratory (Lee's Summit, MO) in accordance with published protocol (59). This assay determines total ATP released from CD4+ T cells with ex vivo mitogen stimulation by phytohemaglutinin-L. The assay has been approved by the United States Food and Drug Administration as a measurement total CD4+ T cell response in transplant recipients.
Data Analysis
Data analysis was performed using STATA statistical software, version 14 (College Station, Texas). Continuous data was analyzed first utilizing the 5 original patient cohorts as predictors utilizing a Kruskal-Wallis test. Association between categorical variables was measured via Fisher's Exact test. In order to evaluate the contributions of each individual parameter on the outcome variables, univariable regression screen was performed. Any significant variables were placed into a multivariable linear regression. A partial least square discriminant analysis (PLSDA) using the mixomics R package (http://mixomics.org/methods/pls-da/) was performed to determine which features contribute to IRP. PLS-DA uses covariance to identify linear combinations of independent or latent variables that best differentiate between the different groups. Each variable is assigned a score, which can be visualized in the latent variable space (score plots). Latent variable loadings (loadings plots) can then be used to identify biomarker profiles associated with different groups. The prediction power of each set of variables was assessed using area under the curve (AUC) implemented in the mixomics package (60).
Results
A portion of these data were presented at the American Transplant Congress in 2018 (61).
Patients
Patient clinical characteristics are compared in Table 1. There were no significant differences between the study groups except for their age and the group with <65 and on dialysis had a large proportion of patients with diabetes as the cause of their CKD. We analyzed the CKD stages for the two groups not on dialysis. Within each group about half was in stage IV and half in stage V. There was no significant difference between the two groups. As expected the estimated glomeruli filtration rate (eGFR) were significantly lower in the two groups on dialysis.
Immune Risk Profile
Based on the definition of IRP, as CMV seropositivity and CD4/CD8 ratio <1 (58) or being in the highest quintile of CD8+ senescent (28CD–/CD57+) T cells (14), weidentified 14 individuals as having the IRP (IRP+). The 14 individuals were equally divided amongst the 5 original study groups (P = 1.0). Of the 40 CMV seropositive patients, 14 were found to be IRP+ (35%). Of the patients in the highest quintile of CD8+ senescent cells, 0 were found to be CMV negative, which is significantly different from the other quintiles (P = 0.003). Caucasians were less likely to be IRP+ than black/AA (0.36; 0.15–0.58) or Asian (0.42; 0.09–0.75). No other factors including CKD/dialysis status, dialysis modality or age were associated. Diabetes was included due to its near significance on the univariable logistic regression. It should be noted that whites were significantly less likely to be CMV positive with only 17/39 (44%) being CMV positive compared to 16/19 (84%) blacks/AA and 5/6 (83.3%) Asians (P = 0.003) consistent with previous population descriptions (62). Racial disparities in IRP status persisted when comparing only CMV+ Caucasians to black/AA (0.35; 0.02–0.68), but did not persist for Asian patients. In order to test if any of the other patients features except those that were used to determine IRP (CMV status, CD4 and CD8, and CD8+ senescent (28CD–/CD57+) cells, could predict the IRP status, we performed a PLS-DA analysis using the IRP status as the outcome and all factors except those that were used to determine IRP, as predictors. This model was able to predict IRP with an accuracy of 1 and a p-value = 0.0004 (Figure 1) suggesting in addition to CMV status, CD4 and CD8, and CD8+ senescent (28CD–/CD57+) cells, other factors contribute significantly in defining IRP.
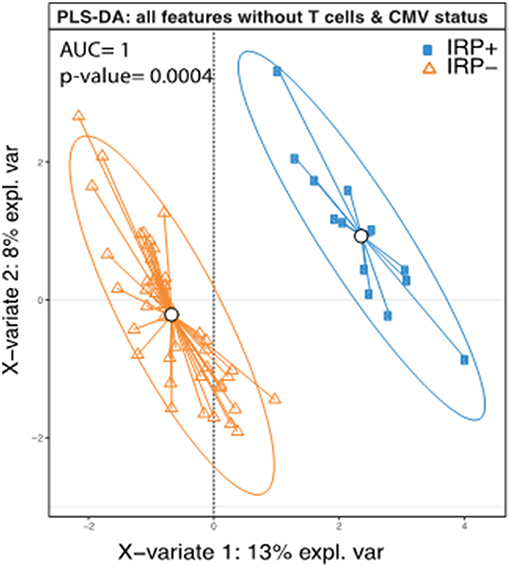
Figure 1. Complete separation of IRP+ and IRP– groups indicate contributions from both clinical and immune factors aside from those used to define IRP. PLS-DA plot of IRP+ and IRP– patients using the collected immune data except those that were used to determine IRP (CMV status, CD4 and CD8, and CD8+ senescent (28CD–/CD57+) cells. Each dot on the plot represents a subject, where blue represents IRP+ samples and orange represents IRP– samples. Confidence ellipses for each group were plotted to highlight the strength of the discrimination (confidence level set to 95%). Groups centroids were represented in the center of each group with circles.
Analysis of T Cell Phenotypes
On multivariable analysis, age and IRP+ but not CMV+/IRP– status were found to affect frequency of CD4+. The percent of CD4+ T cells with a naïve phenotype decreased in both IRP+ and dialysis patients (Table 2A). Age did not affect the proportion of CD4+ naïve cells. The proportions of CD4+ CM cells remained unaffected by any of the variables. IRP+ status is strongly associated with an increased proportion of CD4+ EM cells. The proportion of CD4+ effector cells were not influenced by CMV status, but IRP is associated with an increase of these cells and CKD associated with a decrease. The proportion of CD4+ T cells which are 28CD–/CD57+ indicating senescence did not differ between the 5 cohorts (Table 2B). They were substantially and only increased in IRP+ patients and were not affected by other factors. CD4+ naïve and CM senescent cells are very rare and seen essentially only in IRP+ patients. CD4+ EM and effector senescent cells are more common and significantly increased in IRP+ and CMV+/IRP– patients, but are not affected by age, CKD or dialysis status.
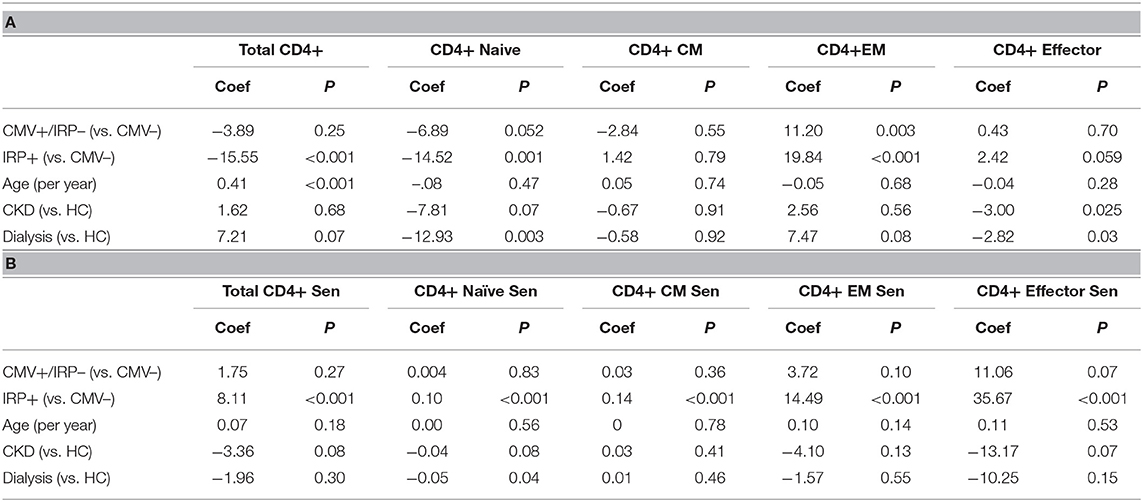
Table 2. Multivariable analysis of factors associated with CD4+ T cell phenotypes (A) and senescence markers (B).
On multivariable analysis, IRP+ but not CMV+/IRP– was associated with an increase in CD8+ T-cells while age was associated with a decline (Table 3A). CD8+ naïve cells were reduced in both the CMV+/IRP– and IRP+ groups and were unaffected by age, CKD and dialysis status. CD8+ CM cells were decreased in IRP+ patients. CD8+ EM were not affected by any variable. CD8+ TEMRA cells were expanded in both CMV+/IRP– and IRP+ groups. The proportion of CD8+ T cells which are 28CD–/57+ indicating senescence was increased only in the IRP+ group, and slightly decreased with age (Table 3B). The CMV–/IRP+ group was not different from the CMV– group. CD8+ senescent cells were unaffected by CKD or dialysis status. CD8+ naïve senescent cells were rare and only decreased in IRP+ patients. CD8+ CM naïve senescent cells are also rare and slightly increased with age. CD8+ EM and TEMRA cells were markedly increased in IRP+ patients and not in CMV+/IRP– patients and were not affected by the other variables.
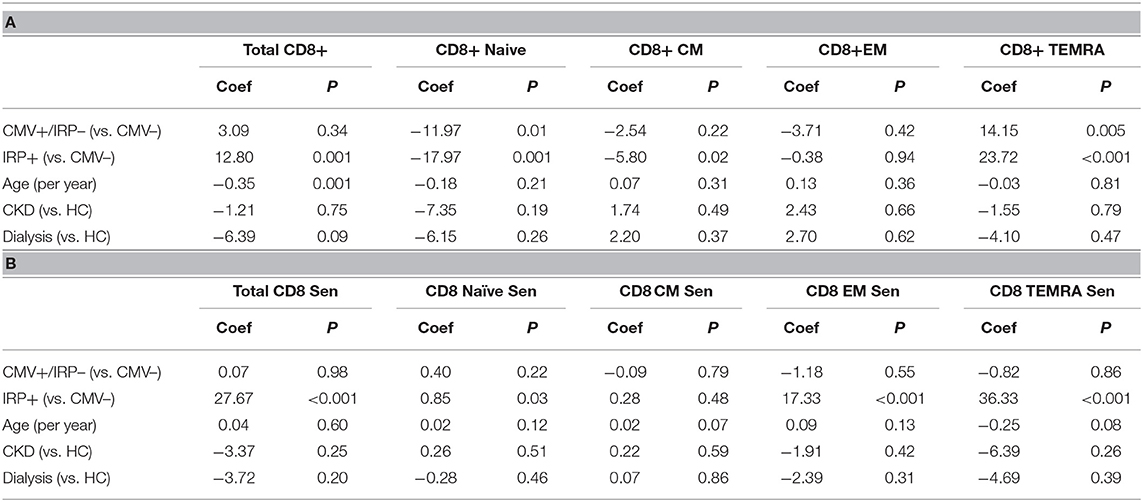
Table 3. Multivariable analysis of factors associated with CD8+ T cell phenotypes (A) and senescence markers (B).
Proportions, Phenotype, and Function of NK Cells
We next evaluated if enhanced proportions of NK cells with phenotypic features reflecting terminal differentiation are associated with CMV serostatus, age, kidney disease, and dialysis in our cohort of renal transplant candidates. To do so, we determined frequencies of NK cells expressing markers of NK cell activation (CD25), as well as those indicative of differentiation (CD57, KLRG1, NKG2C, CD2, and CD27) or adaptive features (FcεRIγ) (Tables 4A,B). Multivariable analysis demonstrates that age is associated with an increase in NK cells, which is not affected by IRP or CKD status. There were no differences in CD2, CD25, or CD57 expression. CMV+/IRP– and IRP+ status both tended toward an increase in CD27+ CD56bright NK cells, though this did not reach significance. Memory-like FcγRΔg NK cells were significantly increased in CKD but not in dialysis patients, and were not significantly affected by IRP status or age. KLRG1+ NK cells were significantly reduced in IRP+ patients but were unaffected by the other factors. NKG2C+ NK cells were significantly increased in dialysis patients but not affected by the other factors. To examine if race affected NK cell phenotypes in our cohort, we performed analysis of data from the Caucasian subjects only and did not show additional significant difference.
To further determine if NK cell profiles can discriminate IRP+ and IRP– patients and can be used in combination with ImmuKnow® values as a surrogate predictor of IRP, we performed a PLSDA analysis using NK cell phenotypic and functional data alone and in combination with ImmuKnow® values. Our multivariate analysis revealed that NK cell features, including proportions of bulk NK cells, and percentages of NK cells expressing KLRG1, CD27, CD57, and NKG2C, predicted IRP with an accuracy of 0.78 and a p-value of 0.002 (Figure 2A). When using ImmuKnow® alone we achieved an accuracy of 0.68 and p-value of 0.04 (Figure 2B). Interestingly, by combining the ImmuKnow® variable and NK cell features, we enhanced the prediction power of our model by reaching an accuracy of 0.83 and a p-value of 0.0002 (Figure 2C). This analysis showed that combining NK features and ImmuKnow® segregated IRP+ and IRP– groups where 18% of the variability between these two groups was explained by the first component and 11% by the second component of the PLSDA (Figure 2D). Several NK features were significant on both components and associated with either IRP+ or IRP– (Figures 2E,F).
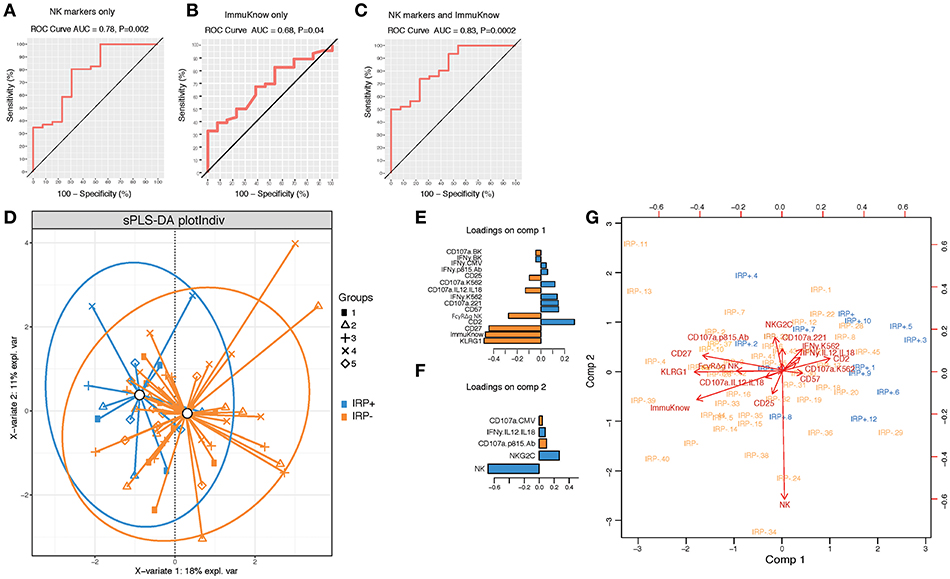
Figure 2. Multivariate analysis using partial least-square discriminant analysis (PLS-DA) shows NK activation markers and ImmuKnow® variable segregate IRP– and IRP+ subjects. (A–C) Receiver operating characteristic (ROC) curves for NK markers alone (A), ImmuKnow® only (and NK markers + ImmuKnow® (C). Receiver operating characteristic (ROC) curve of PLS-DA model predicting IRP outcome. Receiver operating characteristic (ROC) curve showing the accuracy of the PLS-DA model in predicting IRP+ vs. IRP– outcomes using NK markers alone (A), ImmuKnow® alone (B), or NK markers with ImmuKnow® combined (C). The true positive rate (sensitivity) is plotted in function of the false positive rate (100-specificity). The area under the ROC curve (AUC) measures how well the model distinguishes IRP+ from IRP– subjects for each analysis. (D) Individual PLS-DA plot of IRP+ and IRP– patients using NK markers and ImmuKnow® as predictors and IRP status as outcome. Each dot on the plot represents a subject, where blue represents IRP+ samples and orange represents IRP– samples. Confidence ellipses for each group are plotted to highlight the strength of the discrimination (confidence level set to 95%). Groups centroids were represented in green circles. (E,F) Loading weights of each feature selected on the first the second component of the multivariate model. Loading plots represent the top features selected on the first (E) and the second (F) component of the PLS-DA model using tune sPLS-DA with color indicating the class with a maximal mean expression value for each feature. (G) Features correlation circle plots. Correlation circle plots display the correlation between measured features and latent components. Each variable coordinate is defined as the Pearson correlation between the original data and latent components 1 and 2. The contribution of each feature to each component is represented by features proximity to the large circle of radius 1. This plot shows also the correlation between features (clusters of features). The cosine angle between any two points represent the correlation (negative, positive, or null) between two features. A threshold of 0.5 is set to remove weaker correlations and to plot only features with major importance. Strongly correlated variables are projected in the same direction from the origin. The distance from the origin is correlated to the strength of the association.
Overall, this analysis showed that NK cell signatures contributed significantly to the IRP status where KLRG1, FcγRΔg NK cells, and CD27 were associated with the IRP– status and CD2, CD57, NKG2C, and proportions of NK cells were associated with the IRP+ status (Figure 2G). Furthermore, NK cell function against BK or CMV peptide pools also contributed to the NK cell-driven differentiation of IRP, with the IRP+ status being associated with IFN-γ production and the IRP– status with degranulation, as measured by CD107a upregulation, in response to those viral antigens (see Supplementary Material).
T Cell Function Measured by ImmuKnow® Assay
The ImmuKnow® assay was not significantly different across the 5 study groups (P = 0.08). In order to evaluate the role of CMV positivity as well as the IRP, we performed further analysis comparing CMV+ patients who did not display the IRP (CMV+/IRP–) and IRP+ to CMV– patients (Table 5). A multivariable analysis demonstrated that increased age (−4.70; P = 0.005) was significantly associated with decreased ImmuKnow® value (Table 5). Dialysis patients tended to have a higher ImmuKnow® value (111.40; p = 0.08), and CMV seropositivity was associated with lower ImmuKnow® value (−74.21, p = 0.16), though these did not reach significance. Other demographic and clinical factors, including CKD not requiring dialysis, were not associated with ImmuKnow® values.
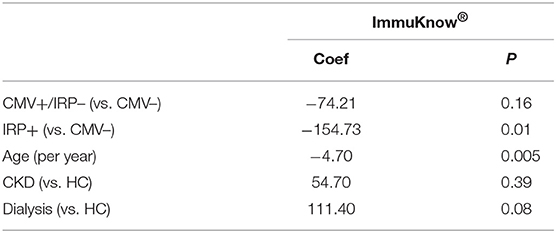
Table 5. Multivariable analysis showed ImmuKnow® results were significantly associated with age and IRP.
Discussion
In this study, we sought to determine if NK cells contribute to immune senescence in renal transplant candidates. We have demonstrated that CMV serostatus, age, and dialysis all contribute toward phenotypic compositions of the T cell compartment. We have also shown that not all patients who are CMV seropositive display an IRP. These patients are in some ways phenotypically similar to CMV negative patients and in others display an intermediate phenotype between CMV– and IRP+ patients. Thus, while CMV latency seems necessary to produce the IRP, it is not sufficient.
While CMV drives senescence, the IRP is concentrated in only a few individuals with the most exaggerated T-cell phenotypic changes. The proportion of T cells which are CD4+ is markedly decreased and the proportion which are CD8+ is increased, leading to a decreased CD4/CD8 ratio. Naïve and CM cells in both CD4 and CD8 lineages are reduced while there is an increase in more differentiated EM and TEMRA cells, consistent with prior descriptions of IRP (24). CD4+/28CD–/CD57+ senescent T cells, which are associated with unstable atherosclerotic plaques (63, 64) and clinical coronary atherosclerosis (17, 18, 65–67) are seen essentially only in IRP+ patients.
CD8+ senescent T cells, which are associated with a reduced likelihood of allograft rejection (20, 21) are markedly increased in IRP patients, as it is part of the definition of IRP, but more importantly, are not increased in CMV+/IRP– patients when compared to CMV– patients. This suggests that recipient CMV seropositivity in of itself is not an adequate marker of T cell senescence. Other contributing factors to CD8+ T cell senescence could include exposure to other latent viral infections such as herpes simplex viruses and human polyomaviruses, and immune modulation by T regulatory cells (68).
In this study, we included a panel of antibodies to specifically characterize NK cell subpopulations in kidney transplant candidates. As previously reported, we found decreased proportions of NK cells in our cohort of patients, although the difference with healthy controls did not reach statistical significance. Unexpectedly, we found that proportions of NK cells expressing markers previously associated with CMV latency were not increased in CMV–seropositive patients compared to seronegative subjects, suggesting that in this cohort of renal transplant candidates, other factors than CMV might significantly influence the differentiation status of NK cells. For instance, dialysis was associated with a marked increase in NKG2C positive NK cells, and further investigations are warranted to determine if this is a direct effect of dialysis, and if dialysis can modulate the ability of NK cells to control viral infections. Similarly, CKD but not CMV seropositivity was associated with augmented proportions of the memory-like FcγRΔg NK cell subset, suggesting that other viral infections or clinical factors might drive the generation of this NK cell subset. Importantly, the IRP+ status could be discriminated based on NK cell expression of the terminal maturation marker CD57 and of the adaptive markers NKG2C and CD2 (69–72). This observation suggests that in patients currently defined as positive for IRP, NK cells are characterized by a terminally differentiated and CMV–driven phenotype.
We examined the contributions of NK cells to immune senescence, and, to our knowledge, provide the first report of distinct NK cell signatures associated with IRP status, dialysis or kidney disease in kidney transplant candidates. Whether the NK cell features identified in this study can predict the IRP status and are associated with reduced NK cell-mediated control of viral infections will need to be confirmed in additional cohorts. These observations highlight the importance of considering additional factors than those currently used to determine the overall immune status of those patients. A thorough evaluation of immune senescence will need to include additional facets of the immune system, such as B cells and antibodies (73), macrophages, and activation and exhaustion markers of the immune cells. Future studies compiling these additional immune features are needed to build IRP and guide precise personalized transplant immune suppression.
We had hypothesized that natural age is not predictive of immune senescence and that immunological age would be a better predictor. Our data showed that age is associated with a relative expansion of the CD4+ T cell subset and decrease in the CD8+ T cell subset, but did not seem to significantly alter CD3+ T cell subsets nor senescence markers. It was, however, associated with a decrease in ImmuKnow® value. Thus, in this highly select population, chronological age does not predict T cell senescence, though is associated with reduced T cell function as measured via the ImmuKnow® assay.
Dialysis was associated with a substantial decline in CD4+ naïve T cells which seems to be exacerbated by the IRP. However, CKD and dialysis status does not seem to significantly alter the CD8+ T cell pool nor significantly affect T cell senescence. Dialysis status was also associated with a non-significant increase in ImmuKnow® value, which is at odds with the known decrease in proliferative capacity in such patients, but may instead reflect the aberrant state of T cell activation (74).
We evaluated the potential of using ImmuKnow® as a clinical tool to identify subjects with IRP+, who may be at increased risk for acquiring infection post-transplant. ImmuKnow® value was significantly reduced in IRP+ patients, but not in CMV+/IRP– patients, consistent with the reduced T cell function seen in immune senescence. This may indicate that CMV infection affects CD8+ T cells more than CD4+ T cells. ImmuKnow® measures total CD4+ T cell function by quantifying ATP release, which does not take into account CD8+ T cells, including the TEMRA cells associated with immune senescence. In elderly kidney transplant candidates, ImmuKnow® could potentially be used to identify those who are IRP+, as demonstrated by our data. However, the wide variability in ImmuKnow values may mean that a larger cohort is needed for further determination.
There are several limitations in this study. We prospectively consented and enrolled 65 subjects, the number of subjects in each group was small. Given the heterogeneous nature of human subject studies, a larger sample size may be needed to better account for these differences. We had consented subjects consecutively in pre kidney transplant clinics and in the outpatient elective surgery testing center without gender preference. This resulted in an imbalance of more female in the healthy control group as compared to the male dominance in the other groups. NK cell activity and thymic function differs between the two genders (75, 76). This could have attributed bias to our results.
Conclusion
Immune senescence is associated with increased risk of infection and decreased risk of rejection post kidney transplant. CMV seems to drive T cell senescence in some, but not all patients who have latent infection. The subset of patients with exaggerated T cell phenotypic changes of senescence called the IRP are not easily identified by age, CKD or dialysis status or other clinical and demographic factors. The ImmuKnow® assay can be used to identify kidney transplant candidates with IRP. NK cell features are affected by CKD and dialysis status more so than age or CMV latency and may help explain the susceptibility of such patients to infections and malignancies. NK cells also contribute to immune senescence, and NK cell signatures combined with ImmuKnow® assay have the potential to predict IRP in the study population.
Traditional risk factors for a senescent or aged immune system correlate well with actual phenotypic changes of senescence associated with increased risk of infection, cardiovascular disease, death and lower risks of allograft rejection. In order to assess senescence, T cell phenotyping appears to be necessary but not sufficient. Phenotype and function of additional components of the immune system such as NK cells, potentially in combination with the Immuknow® assay, could be used to accurately define immune senescence.
Data Availability
The raw data supporting the conclusions of this manuscript will be made available by the authors, without undue reservation, to any qualified researcher.
Author Contributions
DD, MA, KM, SK, SJ, and CT contributed conception and design of the study. DD, KM, JG, EG, CH, and SM enrolled subjects into the study, collected primary data, and performed the immunology experiments. MA performed the statistical analysis. DD wrote the first draft of the manuscript. MA, KM, JG, EG, CH, SM, SK, SJ, and CT wrote sections of the manuscript. All authors contributed to manuscript revision and read and approved the submitted version.
Funding
This project was funded in part by Roche Organ Transplantation Research Grant, NIH NINDS K02 NS097146 (CT), NIH NIAID R01 AI116363 (SJ). ImmuKnow® assays were performed Viracor-Eurofins.
Conflict of Interest Statement
The authors declare that the research was conducted in the absence of any commercial or financial relationships that could be construed as a potential conflict of interest.
Supplementary Material
The Supplementary Material for this article can be found online at: https://www.frontiersin.org/articles/10.3389/fimmu.2019.01890/full#supplementary-material
References
1. Foley RN, Collins AJ. End-stage renal disease in the United States: an update from the United States Renal Data System. J Am Soc Nephrol. (2007) 18:2644–8. doi: 10.1681/ASN.2007020220
2. Danovitch GM, Cohen DJ, Weir MR, Stock PG, Bennett WM, Christensen LL, et al. Current status of kidney and pancreas transplantation in the United States, 1994–2003. Am J Transplant. (2005) 5:904–15. doi: 10.1111/j.1600-6135.2005.00835.x
3. Schaeffner ES, Rose C, Gill JS. Access to kidney transplantation among the elderly in the United States: a glass half full, not half empty. Clin J Am Soc Nephrol. (2010) 5:2109–14. doi: 10.2215/CJN.03490410
4. Girndt M, Sester M, Sester U, Kaul H, Kohler H. Molecular aspects of T- and B-cell function in uremia. Kidney Int Suppl. (2001) 78:S206–11. doi: 10.1046/j.1523-1755.59.s78.6.x
5. Matsumoto Y, Shinzato T, Amano I, Takai I, Kimura Y, Morita H, et al. Relationship between susceptibility to apoptosis and Fas expression in peripheral blood T cells from uremic patients: a possible mechanism for lymphopenia in chronic renal failure. Biochem Biophys Res Commun. (1995) 215:98–105. doi: 10.1006/bbrc.1995.2438
6. Moser B, Roth G, Brunner M, Lilaj T, Deicher R, Wolner E, et al. Aberrant T cell activation and heightened apoptotic turnover in end-stage renal failure patients: a comparative evaluation between non-dialysis, haemodialysis, and peritoneal dialysis. Biochem Biophys Res Commun. (2003) 308:581–5. doi: 10.1016/S0006-291X(03)01389-5
7. Naylor K, Li G, Vallejo AN, Lee WW, Koetz K, Bryl E, et al. The influence of age on T cell generation and TCR diversity. J Immunol. (2005) 174:7446–52. doi: 10.4049/jimmunol.174.11.7446
8. Globerson A, Effros RB. Ageing of lymphocytes and lymphocytes in the aged. Immunol Today. (2000) 21:515–21. doi: 10.1016/S0167-5699(00)01714-X
9. Litjens NH, de Wit EA, Betjes MG. Differential effects of age, cytomegalovirus-seropositivity and end-stage renal disease (ESRD) on circulating T lymphocyte subsets. Immun Ageing. (2011) 8:2. doi: 10.1186/1742-4933-8-2
10. Huang L, Langerak AW, Baan CC, Litjens NH, Betjes MG. Latency for cytomegalovirus impacts T cell ageing significantly in elderly end-stage renal disease patients. Clin Exp Immunol. (2016) 186:239–48. doi: 10.1111/cei.12846
11. Pirsch JD, Stratta RJ, Armbrust MJ, D'Alessandro AM, Sollinger HW, Kalayoglu M, et al. Cadaveric renal transplantation with cyclosporine in patients more than 60 years of age. Transplantation. (1989) 47:259–61. doi: 10.1097/00007890-198902000-00012
12. Tullius SG, Milford E. Kidney allocation and the aging immune response. N Engl J Med. (2011) 364:1369–70. doi: 10.1056/NEJMc1103007
13. de Fijter JW. The impact of age on rejection in kidney transplantation. Drugs Aging. (2005) 22:433–49. doi: 10.2165/00002512-200522050-00007
14. Wikby A, Johansson B, Olsson J, Lofgren S, Nilsson BO, Ferguson F. Expansions of peripheral blood CD8 T-lymphocyte subpopulations and an association with cytomegalovirus seropositivity in the elderly: the Swedish NONA immune study. Exp Gerontol. (2002) 37:445–53. doi: 10.1016/S0531-5565(01)00212-1
15. Crepin T, Gaiffe E, Courivaud C, Roubiou C, Laheurte C, Moulin B, et al. Pre-transplant end-stage renal disease-related immune risk profile in kidney transplant recipients predicts post-transplant infections. Transpl Infect Dis. (2016) 18:415–22. doi: 10.1111/tid.12534
16. DeWolfe D, Gandhi J, Mackenzie MR, Broge TA Jr, Bord E, Babwah A, et al. Pre-transplant immune factors may be associated with BK polyomavirus reactivation in kidney transplant recipients. PLoS ONE. (2017) 12:e0177339. doi: 10.1371/journal.pone.0177339
17. Alber HF, Duftner C, Wanitschek M, Dorler J, Schirmer M, Suessenbacher A, et al. Neopterin, CD4+CD28– lymphocytes and the extent and severity of coronary artery disease. Int J Cardiol. (2009) 135:27–35. doi: 10.1016/j.ijcard.2008.03.010
18. Liuzzo G, Goronzy JJ, Yang H, Kopecky SL, Holmes DR, Frye RL, et al. Monoclonal T-cell proliferation and plaque instability in acute coronary syndromes. Circulation. (2000) 101:2883–8. doi: 10.1161/01.CIR.101.25.2883
19. Betjes MG, Meijers RW, de Wit EA, Weimar W, Litjens NH. Terminally differentiated CD8+ Temra cells are associated with the risk for acute kidney allograft rejection. Transplantation. (2012) 94:63–9. doi: 10.1097/TP.0b013e31825306ff
20. Trzonkowski P, Debska-Slizien A, Jankowska M, Wardowska A, Carvalho-Gaspar M, Hak L, et al. Immunosenescence increases the rate of acceptance of kidney allotransplants in elderly recipients through exhaustion of CD4+ T-cells. Mech Ageing Dev. (2010) 131:96–104. doi: 10.1016/j.mad.2009.12.006
21. Dedeoglu B, Meijers RW, Klepper M, Hesselink DA, Baan CC, Litjens NH, et al. Loss of CD28 on peripheral T cells decreases the risk for early acute rejection after kidney transplantation. PLoS ONE. (2016) 11:e0150826. doi: 10.1371/journal.pone.0150826
22. Crepin T, Carron C, Roubiou C, Gaugler B, Gaiffe E, Simula-Faivre D, et al. ATG-induced accelerated immune senescence: clinical implications in renal transplant recipients. Am J Transplant. (2015) 15:1028–38. doi: 10.1111/ajt.13092
23. Yap M, Boeffard F, Clave E, Pallier A, Danger R, Giral M, et al. Expansion of highly differentiated cytotoxic terminally differentiated effector memory CD8+ T cells in a subset of clinically stable kidney transplant recipients: a potential marker for late graft dysfunction. J Am Soc Nephrol. (2014) 25:1856–68. doi: 10.1681/ASN.2013080848
24. Chidrawar S, Khan N, Wei W, McLarnon A, Smith N, Nayak L, et al. Cytomegalovirus-seropositivity has a profound influence on the magnitude of major lymphoid subsets within healthy individuals. Clin Exp Immunol. (2009) 155:423–32. doi: 10.1111/j.1365-2249.2008.03785.x
25. Appay V, van Lier RA, Sallusto F, Roederer M. Phenotype and function of human T lymphocyte subsets: consensus and issues. Cytometry A. (2008) 73:975–83. doi: 10.1002/cyto.a.20643
26. Derhovanessian E, Maier AB, Hahnel K, Beck R, de Craen AJ, Slagboom EP, et al. Infection with cytomegalovirus but not herpes simplex virus induces the accumulation of late-differentiated CD4+ and CD8+ T-cells in humans. J Gen Virol. (2011) 92:2746–56. doi: 10.1099/vir.0.036004-0
27. Czesnikiewicz-Guzik M, Lee WW, Cui D, Hiruma Y, Lamar DL, Yang ZZ, et al. T cell subset-specific susceptibility to aging. Clin Immunol. (2008) 127:107–18. doi: 10.1016/j.clim.2007.12.002
28. Betjes MG, Meijers RW, Litjens NH. Loss of renal function causes premature aging of the immune system. Blood Purif. (2013) 36:173–8. doi: 10.1159/000356084
29. Litjens NH, van Druningen CJ, Betjes MG. Progressive loss of renal function is associated with activation and depletion of naive T lymphocytes. Clin Immunol. (2006) 118:83–91. doi: 10.1016/j.clim.2005.09.007
30. Meijers RW, Litjens NH, de Wit EA, Langerak AW, van der Spek A, Baan CC, et al. Uremia causes premature ageing of the T cell compartment in end-stage renal disease patients. Immun Ageing. (2012) 9:19. doi: 10.1186/1742-4933-9-19
31. Meijers RW, Litjens NH, de Wit EA, Langerak AW, Baan CC, Betjes MG. Uremia-associated immunological aging is stably imprinted in the T-cell system and not reversed by kidney transplantation. Transpl Int. (2014) 27:1272–84. doi: 10.1111/tri.12416
32. Ferguson FG, Wikby A, Maxson P, Olsson J, Johansson B. Immune parameters in a longitudinal study of a very old population of Swedish people: a comparison between survivors and nonsurvivors. J Gerontol A Biol Sci Med Sci. (1995) 50:B378–82. doi: 10.1093/gerona/50A.6.B378
33. Wikby A, Maxson P, Olsson J, Johansson B, Ferguson FG. Changes in CD8 and CD4 lymphocyte subsets, T cell proliferation responses and non-survival in the very old: the Swedish longitudinal OCTO-immune study. Mech Ageing Dev. (1998) 102:187–98. doi: 10.1016/S0047-6374(97)00151-6
34. Olsson J, Wikby A, Johansson B, Lofgren S, Nilsson BO, Ferguson FG. Age-related change in peripheral blood T-lymphocyte subpopulations and cytomegalovirus infection in the very old: the Swedish longitudinal OCTO immune study. Mech Ageing Dev. (2000) 121:187–201. doi: 10.1016/S0047-6374(00)00210-4
35. Borrego F, Alonso MC, Galiani MD, Carracedo J, Ramirez R, Ostos B, et al. NK phenotypic markers and IL2 response in NK cells from elderly people. Exp Gerontol. (1999) 34:253–65. doi: 10.1016/S0531-5565(98)00076-X
36. Almeida-Oliveira A, Smith-Carvalho M, Porto LC, Cardoso-Oliveira J, Ribeiro Ados S, Falcao RR, et al. Age-related changes in natural killer cell receptors from childhood through old age. Hum Immunol. (2011) 72:319–29. doi: 10.1016/j.humimm.2011.01.009
37. Campos C, Pera A, Lopez-Fernandez I, Alonso C, Tarazona R, Solana R. Proinflammatory status influences NK cells subsets in the elderly. Immunol Lett. (2014) 162:298–302. doi: 10.1016/j.imlet.2014.06.015
38. Hazeldine J, Hampson P, Lord JM. Reduced release and binding of perforin at the immunological synapse underlies the age-related decline in natural killer cell cytotoxicity. Aging Cell. (2012) 11:751–9. doi: 10.1111/j.1474-9726.2012.00839.x
39. Le Garff-Tavernier M, Beziat V, Decocq J, Siguret V, Gandjbakhch F, Pautas E, et al. Human NK cells display major phenotypic and functional changes over the life span. Aging Cell. (2010) 9:527–35. doi: 10.1111/j.1474-9726.2010.00584.x
40. Lutz CT, Karapetyan A, Al-Attar A, Shelton BJ, Holt KJ, Tucker JH, et al. Human NK cells proliferate and die in vivo more rapidly than T cells in healthy young and elderly adults. J Immunol. (2011) 186:4590–8. doi: 10.4049/jimmunol.1002732
41. Lutz CT, Moore MB, Bradley S, Shelton BJ, Lutgendorf SK. Reciprocal age related change in natural killer cell receptors for MHC class I. Mech Ageing Dev. (2005) 126:722–31. doi: 10.1016/j.mad.2005.01.004
42. Chidrawar SM, Khan N, Chan YL, Nayak L, Moss PA. Ageing is associated with a decline in peripheral blood CD56bright NK cells. Immun Ageing. (2006) 3:10. doi: 10.1186/1742-4933-3-10
43. Muller-Durovic B, Lanna A, Covre LP, Mills RS, Henson SM, Akbar AN. Killer cell lectin-like receptor G1 inhibits NK cell function through activation of adenosine 5'-monophosphate-activated protein kinase. J Immunol. (2016) 197:2891–9. doi: 10.4049/jimmunol.1600590
44. Bjorkstrom NK, Riese P, Heuts F, Andersson S, Fauriat C, Ivarsson MA, et al. Expression patterns of NKG2A, KIR, and CD57 define a process of CD56dim NK-cell differentiation uncoupled from NK-cell education. Blood. (2010) 116:3853–64. doi: 10.1182/blood-2010-04-281675
45. Phillips JH, Lanier LL. A model for the differentiation of human natural killer cells. Studies on the in vitro activation of Leu-11+ granular lymphocytes with a natural killer-sensitive tumor cell, K562. J Exp Med. (1985) 161:1464–82. doi: 10.1084/jem.161.6.1464
46. Lopez-Verges S, Milush JM, Pandey S, York VA, Arakawa-Hoyt J, Pircher H, et al. CD57 defines a functionally distinct population of mature NK cells in the human CD56dimCD16+ NK-cell subset. Blood. (2010) 116:3865–74. doi: 10.1182/blood-2010-04-282301
47. Della Chiesa M, Falco M, Bertaina A, Muccio L, Alicata C, Frassoni F, et al. Human cytomegalovirus infection promotes rapid maturation of NK cells expressing activating killer Ig-like receptor in patients transplanted with NKG2C-/- umbilical cord blood. J Immunol. (2014) 192:1471–9. doi: 10.4049/jimmunol.1302053
48. Foley B, Cooley S, Verneris MR, Pitt M, Curtsinger J, Luo X, et al. Cytomegalovirus reactivation after allogeneic transplantation promotes a lasting increase in educated NKG2C+ natural killer cells with potent function. Blood. (2012) 119:2665–74. doi: 10.1182/blood-2011-10-386995
49. Guma M, Angulo A, Vilches C, Gomez-Lozano N, Malats N, Lopez-Botet M. Imprint of human cytomegalovirus infection on the NK cell receptor repertoire. Blood. (2004) 104:3664–71. doi: 10.1182/blood-2004-05-2058
50. Guma M, Budt M, Saez A, Brckalo T, Hengel H, Angulo A, et al. Expansion of CD94/NKG2C+ NK cells in response to human cytomegalovirus-infected fibroblasts. Blood. (2006) 107:3624–31. doi: 10.1182/blood-2005-09-3682
51. Lopez-Verges S, Milush JM, Schwartz BS, Pando MJ, Jarjoura J, York VA, et al. Expansion of a unique CD57(+)NKG2Chi natural killer cell subset during acute human cytomegalovirus infection. Proc Natl Acad Sci USA. (2011) 108:14725–32. doi: 10.1073/pnas.1110900108
52. Lee J, Zhang T, Hwang I, Kim A, Nitschke L, Kim M, et al. Epigenetic modification and antibody-dependent expansion of memory-like NK cells in human cytomegalovirus-infected individuals. Immunity. (2015) 42:431–42. doi: 10.1016/j.immuni.2015.02.013
53. Schlums H, Cichocki F, Tesi B, Theorell J, Beziat V, Holmes TD, et al. Cytomegalovirus infection drives adaptive epigenetic diversification of NK cells with altered signaling and effector function. Immunity. (2015) 42:443–56. doi: 10.1016/j.immuni.2015.02.008
54. Zhang T, Scott JM, Hwang I, Kim S. Cutting edge: antibody-dependent memory-like NK cells distinguished by FcRgamma deficiency. J Immunol. (2013) 190:1402–6. doi: 10.4049/jimmunol.1203034
55. Fu B, Wang F, Sun R, Ling B, Tian Z, Wei H. CD11b and CD27 reflect distinct population and functional specialization in human natural killer cells. Immunology. (2011) 133:350–9. doi: 10.1111/j.1365-2567.2011.03446.x
56. Vossen MT, Matmati M, Hertoghs KM, Baars PA, Gent MR, Leclercq G, et al. CD27 defines phenotypically and functionally different human NK cell subsets. J Immunol. (2008) 180:3739–45. doi: 10.4049/jimmunol.180.6.3739
57. Vacher-Coponat H, Brunet C, Lyonnet L, Bonnet E, Loundou A, Sampol J, et al. Natural killer cell alterations correlate with loss of renal function and dialysis duration in uraemic patients. Nephrol Dial Transplant. (2008) 23:1406–14. doi: 10.1093/ndt/gfm596
58. Strindhall J, Skog M, Ernerudh J, Bengner M, Lofgren S, Matussek A, et al. The inverted CD4/CD8 ratio and associated parameters in 66-year-old individuals: the Swedish HEXA immune study. Age. (2013) 35:985–91. doi: 10.1007/s11357-012-9400-3
59. Zeevi A, Lunz J. Cylex ImmuKnow cell function assay. Methods Mol Biol. (2013) 1034:343–51. doi: 10.1007/978-1-62703-493-7_21
60. Rohart F, Gautier B, Singh A, Le Cao KA. mixOmics: An R package for 'omics feature selection and multiple data integration. PLoS Comput Biol. (2017) 13:e1005752. doi: 10.1371/journal.pcbi.1005752
61. DeWolfe D, McGann K, Ghofrani J, Geiger E, Helzer C, Kleiboeker S, et al. Multiple factors contribute to an expanded immune risk profile in pre renal transplant candidates. Am J Transplant. (2018) 18 (suppl. 4):483. doi: 10.1111/ajt.14918
62. Betjes MG, Litjens NH, Zietse R. Seropositivity for cytomegalovirus in patients with end-stage renal disease is strongly associated with atherosclerotic disease. Nephrol Dial Transplant. (2007) 22:3298–303. doi: 10.1093/ndt/gfm348
63. Liuzzo G, Biasucci LM, Trotta G, Brugaletta S, Pinnelli M, Digianuario G, et al. Unusual CD4+CD28null T lymphocytes and recurrence of acute coronary events. J Am Coll Cardiol. (2007) 50:1450–8. doi: 10.1016/j.jacc.2007.06.040
64. Liuzzo G, Kopecky SL, Frye RL, O'Fallon WM, Maseri A, Goronzy JJ, et al. Perturbation of the T-cell repertoire in patients with unstable angina. Circulation. (1999) 100:2135–9. doi: 10.1161/01.CIR.100.21.2135
65. Sun Z, Ye H, Tang B, Shen X, Wu X, Zhong H, et al. Prevalence of circulating CD4+CD28null T cells is associated with early atherosclerotic damage in patients with end-stage renal disease undergoing hemodialysis. Hum Immunol. (2013) 74:6–13. doi: 10.1016/j.humimm.2012.08.001
66. Teo FH, de Oliveira RT, Mamoni RL, Ferreira MC, Nadruz W Jr, Coelho OR, et al. Characterization of CD4+CD28null T cells in patients with coronary artery disease and individuals with risk factors for atherosclerosis. Cell Immunol. (2013) 281:11–9. doi: 10.1016/j.cellimm.2013.01.007
67. Broadley I, Pera A, Morrow G, Davies KA, Kern F. Expansions of cytotoxic CD4+CD28– T cells drive excess cardiovascular mortality in rheumatoid arthritis and other chronic inflammatory conditions and are triggered by CMV infection. Front Immunol. (2017) 8:195. doi: 10.3389/fimmu.2017.00195
68. Bellon M, Nicot C. Telomere dynamics in immune senescence and exhaustion triggered by chronic viral infection. Viruses. (2017) 9:E289. doi: 10.3390/v9100289
69. Wagner JA, Fehniger TA. Human adaptive natural killer cells: beyond NKG2C. Trends Immunol. (2016) 37:351–3. doi: 10.1016/j.it.2016.05.001
70. Kared H, Martelli S, Tan SW, Simoni Y, Chong ML, Yap SH, et al. Adaptive NKG2C(+)CD57(+) natural killer cell and Tim-3 expression during viral infections. Front Immunol. (2018) 9:686. doi: 10.3389/fimmu.2018.00686
71. Redondo-Pachon D, Crespo M, Yelamos J, Muntasell A, Perez-Saez MJ, Perez-Fernandez S, et al. Adaptive NKG2C+ NK cell response and the risk of cytomegalovirus infection in kidney transplant recipients. J Immunol. (2017) 198:94–101. doi: 10.4049/jimmunol.1601236
72. Pupuleku A, Costa-Garcia M, Farre D, Hengel H, Angulo A, Muntasell A, et al. Elusive role of the CD94/NKG2C NK cell receptor in the response to cytomegalovirus: novel experimental observations in a reporter cell system. Front Immunol. (2017) 8:1317. doi: 10.3389/fimmu.2017.01317
73. de Bourcy CF, Angel CJ, Vollmers C, Dekker CL, Davis MM, Quake SR. Phylogenetic analysis of the human antibody repertoire reveals quantitative signatures of immune senescence and aging. Proc Natl Acad Sci USA. (2017) 114:1105–10. doi: 10.1073/pnas.1617959114
74. Ankersmit HJ, Deicher R, Moser B, Teufel I, Roth G, Gerlitz S, et al. Impaired T cell proliferation, increased soluble death-inducing receptors and activation-induced T cell death in patients undergoing haemodialysis. Clin Exp Immunol. (2001) 125:142–8. doi: 10.1046/j.1365-2249.2001.01590.x
75. Chaudhry MS, Velardi E, Dudakov JA, van den Brink MR. Thymus: the next (re)generation. Immunol Rev. (2016) 271:56–71. doi: 10.1111/imr.12418
Keywords: NK cells, immune risk profile, BK virus, kidney transplant, immune senescence, end stage renal disease, dialysis
Citation: DeWolfe D, Aid M, McGann K, Ghofrani J, Geiger E, Helzer C, Malik S, Kleiboeker S, Jost S and Tan CS (2019) NK Cells Contribute to the Immune Risk Profile in Kidney Transplant Candidates. Front. Immunol. 10:1890. doi: 10.3389/fimmu.2019.01890
Received: 08 March 2019; Accepted: 26 July 2019;
Published: 23 August 2019.
Edited by:
Ulrike Koehl, Hannover Medical School, GermanyReviewed by:
Christophe Picard, Établissement Français du Sang (EFS), FrancePhilippe Saas, INSERM U1098 Interactions Hôte-Greffon-Tumeur and Ingénierie Cellulaire et Génique, France
Copyright © 2019 DeWolfe, Aid, McGann, Ghofrani, Geiger, Helzer, Malik, Kleiboeker, Jost and Tan. This is an open-access article distributed under the terms of the Creative Commons Attribution License (CC BY). The use, distribution or reproduction in other forums is permitted, provided the original author(s) and the copyright owner(s) are credited and that the original publication in this journal is cited, in accordance with accepted academic practice. No use, distribution or reproduction is permitted which does not comply with these terms.
*Correspondence: Chen Sabrina Tan, Y3RhbkBiaWRtYy5oYXJ2YXJkLmVkdQ==
†Present Address: David DeWolfe and Kevin McGann, University of Rochester, Medical Center, New York, NY, United States
‡Co-first authors