- 1Conjoint Internal Medicine Laboratory, Chemical Pathology, Pathology Queensland, Brisbane, QLD, Australia
- 2Kidney Health Service, Royal Brisbane and Women's Hospital, Brisbane, QLD, Australia
- 3Princess Alexandra Hospital, Brisbane, QLD, Australia
- 4Medical School, University of Queensland, Brisbane, QLD, Australia
- 5Institute of Health and Biomedical Innovation, Queensland University of Technology, Brisbane, QLD, Australia
- 6School of Biomedical Sciences, Queensland University of Technology, Brisbane, QLD, Australia
Background: Human natural killer (NK) cells are key functional players in kidney transplant rejection. However, the respective contributions of the two functionally distinct human NK cell subsets (CD56bright cytokine-producing vs. CD56dim cytotoxic effector) in episodes of allograft rejection remain uncertain, with current immunohistochemical methods unable to differentiate these discrete populations. We report the outcomes of an innovative multi-color flow cytometric-based approach to unequivocally define and evaluate NK cell subsets in human kidney allograft rejection.
Methods: We extracted renal lymphocytes from human kidney transplant biopsies. NK cell subsets were identified, enumerated, and phenotyped by multi-color flow cytometry. Dissociation supernatants were harvested and levels of soluble proteins were determined using a multiplex bead-based assay. Results were correlated with the histopathological patterns in biopsies—no rejection, borderline cellular rejection, T cell-mediated rejection (TCMR), and antibody-mediated rejection (AMR).
Results: Absolute numbers of only CD56bright NK cells were significantly elevated in TCMR biopsies. In contrast, both CD56bright and CD56dim NK cell numbers were significantly increased in biopsies with histopathological evidence of AMR. Notably, expression of the activation marker CD69 was only significantly elevated on CD56dim NK cells in AMR biopsies compared with no rejection biopsies, indicative of a pathogenic phenotype for this cytotoxic NK cell subset. In line with this, we detected significantly elevated levels of cytotoxic effector molecules (perforin, granzyme A, and granulysin) in the dissociation supernatants of biopsies with a histopathological pattern of AMR.
Conclusions: Our results indicate that human NK cell subsets are differentially recruited and activated during distinct types of rejection, suggestive of specialized functional roles.
Introduction
Kidney transplantation is the gold standard treatment for end stage kidney disease, with superior quality of life and patient survival compared to dialysis. Despite advances in kidney transplantation techniques and immunosuppression therapy, immunological rejection continues to account for loss of graft function and eventually graft loss (1). Immune-mediated allograft rejection is classified histopathologically into two types: T cell-mediated rejection (TCMR) and antibody-mediated rejection (AMR) (2, 3). TCMR is characterized by tubulointerstitial inflammation mediated by host alloreactive lymphocytes targeting donor human leukocyte antigen (HLA) molecules in the graft, whilst AMR is a process of microvascular inflammation (glomerulitis, peritubular capillaritis) driven by donor-specific antibodies (DSA) interacting with the allograft endothelium (4–6). Most immuno-biological studies of kidney allograft rejection have focused on conventional T (CD4+ or CD8+) and B cells. Less is known about the roles of innate lymphocytes in the different patterns of immune-mediated allograft rejection.
Natural killer (NK) cells are innate lymphocytes that have an immune surveillance function under homeostatic conditions, but can be rapidly recruited to sites of inflammation under pathological conditions. NK cells are activated by a combination of inhibitory and activating signals orchestrated through cell surface receptors and/or cytokine stimulation. Once activated, NK cells display immediate effector function through the production of pro-inflammatory cytokines and via cytotoxic activity (7).
Human NK cells are classically defined as CD3−/CD56+/CD335 (NKp46)+ mononuclear cells. They are subcategorised based on expression levels of CD56 (neural cell adhesion molecule) into low-density (CD56dim) and high density (CD56bright) subsets. The two NK cell subsets differ in phenotypic and functional properties. CD56dim NK cells express high levels of CD16 (low affinity receptor for IgG, FcγRIII) (8) and are considered functionally to be cytotoxic effector cells (9). Upon activation, CD56dim NK cells produce cytotoxic granules containing perforin/granulysin that, when released, create pores in the cell membranes of targeted cells and granzymes that induce apoptosis (9). In contrast, CD56bright NK cells express high levels of CD56, are CD16−/low and mediate immune responses by secreting pro-inflammatory cytokines [e.g., interferon (IFN)-γ] (9).
NK cells are emerging as powerful drivers in immune-mediated kidney allograft rejection. Their functional role has been established in mouse models of kidney allograft injury (10–12). Initial investigations in humans, focused primarily on peripheral blood NK cells from kidney transplant recipients and transcriptomic studies of allograft biopsies, provide compelling evidence supporting a role for NK cells in kidney allograft injury (13–18). Indeed, immunohistochemical (IHC)-based studies report significant associations between human NK cells and both TCMR (19, 20) and AMR (18, 21, 22). However, these IHC-based studies are limited to single antigen (CD56, CD16, or NKp46) labeling to identify human NK cells, a technical approach that cannot unequivocally define this innate lymphocyte population nor differentiate between the distinct NK cell subsets. Thus, to date, the discrete roles of kidney NK cell subsets in different types of human allograft rejection have not been reported.
These technical limitations can be addressed using multi-parameter staining methodologies that accurately identify, enumerate and phenotype human NK cells, in particular, NK cell subsets, in kidney allograft biopsies. In this study, we extend our previously published multi-color flow cytometry-based approach to provide, for the first time, a comprehensive mapping of human NK cell subsets in kidney allograft rejection, ascribing specialized roles during the two patterns of immune-mediated rejection (TCMR vs. AMR).
Methods
Study Design
Kidney transplant recipients (n = 56) were biopsied at the Royal Brisbane and Women's Hospital or Princess Alexandra Hospital between 2015 and 2018. All biopsies were undertaken for clinical indications. Written informed consent for participation in the study was obtained. The Royal Brisbane and Women's Hospital Human Research Ethics Committee (2006/072) and the Princess Alexandra Hospital Ethics Committee (HREC/16/QPAH/214) approved the study.
Kidney Tissue Specimens
Fresh biopsy specimens were taken with either an 18-gauge or 16-gauge biopsy needle (Biopsybell, Mirandola, Italy) and immediately divided for (i) tissue dissociation (1–5 mm of a core biopsy specimen); and (ii) assessment of allograft rejection by specialist renal histopathologists blinded to experimental results. The biopsies were examined for rejection in the pathology departments of participating hospitals.
Samples were graded according to the Banff-classification (23). According to these criteria, biopsies were then grouped into: no evidence of rejection (no rejection), borderline cellular rejection (borderline), TCMR alone, or biopsies with an indication of AMR. Samples that arrived for processing >12 h post collection were excluded. Biopsies that had other diagnoses such as BK nephropathy, recurrent patterns of glomerulonephritis like IgA nephropathy and additional pathology (e.g., amyloidosis) were also excluded from the study.
Tissue Dissociation for Flow Cytometric Analysis
Allograft biopsy specimens excess to clinical diagnostic need were digested within 12 h of collection using our published protocol (24). In brief, kidney cortical tissue was digested with 1 mg/ml collagenase P (Roche, Mannheim, Germany) in the presence of 20 mg/ml DNase I (Roche) for 15 min. Following centrifugation, supernatant was collected for assessment of soluble cytotoxic effector proteins. Tissue was further digested with 10 mg/ml trypsin + 4 mg/ml ethylenediamine tetraacetic acid (EDTA) (Life Technologies, Grand Island, NY) for 10 min.
Flow Cytometry
Single cell suspensions were initially stained with LIVE/DEAD Fixable Near-IR Dead Cell Stain Kit (Life Technologies) to exclude non-viable cells. Cells were then incubated with Human TruStain FcX Blocking Solution (Biolegend, San Diego, CA) at room temperature for 5 min and then stained on ice for 30 min with combinations of test- (0.25 μg per antibody) (Table 1) or isotype-matched control antibodies in cold fluorescence-activated cell sorter buffer (0.5% bovine serum albumin [Sigma-Aldrich, St. Louis, MO] and 0.02% sodium azide [Sigma-Aldrich] in phosphate buffered saline).
Flow-Count Fluorospheres (Beckman Coulter, Brea, CA) were used for direct determination of absolute counts following the manufacturer's recommendations. Briefly, target cell concentrations (cells/μl) were calculated as the total number of target cells counted/total number of fluorospheres counted X Flow-Count Fluorosphere concentration. This value was then multiplied by the total sample volume to obtain absolute counts for each target cell population. Total cell counts were then normalized to cell numbers per cubic centimeter of tissue, in which the volume of renal tissue was calculated as πr2 × length of biopsy tissue, where the radius (r) of a 16-gauge biopsy specimen is 0.8 mm and 18-gauge biopsy specimen is 0.6 mm. Cell acquisition was performed on an LSR Fortessa (BD Biosciences, San Jose, CA) and data analyzed with FlowJo software (TreeStar, Ashland, OR) to identify immune cell populations as presented in Figure 1.
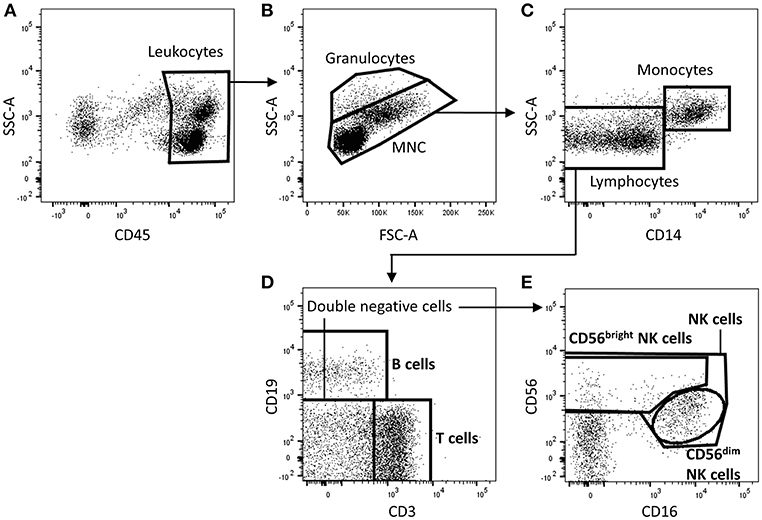
Figure 1. Identification of T cells, B cells, and natural killer (NK) cell subsets in human kidney tissue. Gating strategy used to identify T cells (CD3+), B cells (CD19+), total NK cells (CD3− CD19− CD56+ lymphocytes), and NK cell subpopulations (CD56dim and CD56bright NK cells) in human kidney transplant tissue. Single, live, CD45+ mononuclear cells (MNC) and granulocytes are gated on a forward-scatter (FSC)/side-scatter (SSC) plot (A,B). Total lymphocytes are distinguished from granulocytes and monocytes based on low SSC and absent CD14 expression (C). Total lymphocytes are further separated into T cells or B cells by their expression of CD3 and CD19 respectively (D). NK subpopulations, CD56bright and CD56dim NK cells, are identified based on CD56 intensity and CD16 expression (E). Representative flow cytometric data from 1 of 10 individual antibody-mediated rejection (AMR) renal biopsy specimens are shown. An identical gating strategy was used for no rejection, borderline rejection and T cell-mediated rejection (TCMR) biopsies. MNC, mononuclear cells; FSC-A, forward-scatter area; SSC-A, side-scatter area.
Quantification of Cytokines by Multi-analyte Flow Cytometric Immunoassay
Cytotoxic effector proteins in tissue dissociation supernatants were quantified by the LEGENDplex™ Multi-analyte flow assay kit (human CD8/NK panel) according to the manufacturer's instructions (Biolegend, San Diego, USA). Data acquisition was performed on an LSR Fortessa (BD Biosciences, San Jose, CA). Standard curve and concentrations were calculated with BioLegend's LEGENDplex™ Data Analysis Software (Biolegend, San Diego, USA). Cytokine values were normalized to pg per cm3 of tissue.
Statistics
All statistical tests were performed using Prism 7.0 analysis software (GraphPad Software, La Jolla, CA). Multiple comparisons were performed using a Kruskal-Wallis test with Dunn's post-test. A Mann-Whitney u-test was used for comparisons of non-parametric data from two groups. P < 0.05 were considered statistically significant.
Results
Human Population Demographics
As reported in Table 2, the mean age of the 56 patients in the study was 52.2 ± 14.5 years (range 20–80 years), with 66.1% (37/56) male. The majority of patients (85.7%; 48/56) undergoing biopsy had not undergone previous transplantation, suggesting a reasonably unsensitised population. Cadaveric transplants accounted for 92.8% (52/56) of all biopsies, with donor after brain death (DBD) being the most common type of cadaveric graft biopsied. The median HLA matching was 4/6. All but one patient underwent basiliximab, mycophenolic acid, tacrolimus, and prednisolone based induction therapy, with the remaining patient receiving thymoglobulin induction. The majority of patients (55.4%; 31/56) were classed as low immune risk at transplantation with undetectable calculated Panel Reactive Antibodies (cPRA 0%), whilst highly sensitized patients with cPRA >95% accounted for only 3.6% (2/56) of total patients. The majority of patients (66.1%; 37/56) underwent biopsy within the first 3 months of transplantation. Mean creatinine at time of biopsy was 219.1 ± 106.7 μmol/L (n = 41), with 15 patients who were haemodialysis-dependent at time of biopsy due to delayed graft function or kidney injury excluded from mean creatinine calculations.
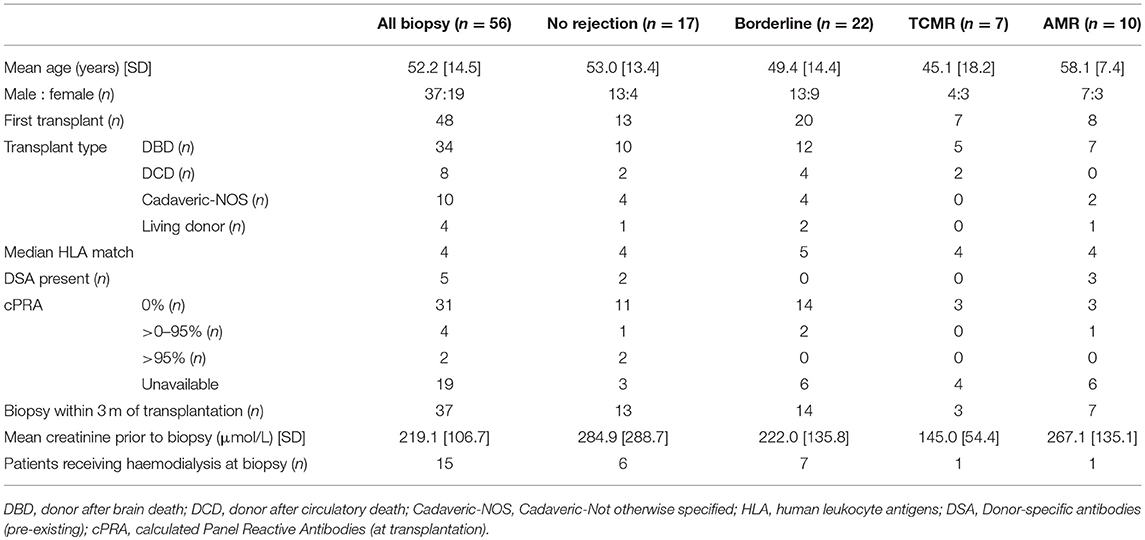
Table 2. Demographic and clinical characteristics of human kidney transplant patients in study cohort.
The transplant biopsy specimens were categorized based upon histopathological examination by renal histopathologists blinded to experimental results. Samples were graded according to the Banff-classification (23). According to these criteria, the 56 biopsies sorted into groups without histopathological evidence of rejection (no rejection; n = 17; 13 males/4 females; mean age of 53.0 ± 13.4 years), borderline cellular rejection (borderline; n = 22; 13 males/9 females; mean age of 49.4 ± 14.4 years), T cell-mediated rejection (TCMR; n = 7; 4 males/3 females; mean age of 45.1 ± 18.2 years) and antibody-mediated rejection (AMR; n = 10; 7 males/3 females; mean age of 58.1 ± 7.4 years). Demographic and clinical characteristics of these cohorts are given in Table 2.
Identification of NK Cell Subsets in Human Allograft Kidney Tissue
Human allograft kidney tissue was enzymatically digested to obtain single cells for flow cytometric analysis. Briefly CD45+ leukocytes separated into granulocytes (with higher side scatter) and mononuclear cells (Figures 1A,B). The mononuclear cells were further divided into CD14+ monocyte and CD14− lymphocyte populations (Figure 1C). Lymphocytes were then delineated into CD3+ T cells, CD19+ B cells, and CD3− CD19− double-negative cells (Figure 1D). Within this double-negative population, total NK cells were identified as CD56+ cells, with CD56bright CD16−/low and CD56dim CD16+ NK cell subsets defined for the first time in human kidney allograft tissue (Figure 1E). Importantly, these surface molecules used to identify NK cells subsets in kidney allograft tissue were resistant to proteolytic cleavage as confirmed by enzymatic digestion (with collagenase P and trypsin-EDTA) of peripheral blood mononuclear cells (data not shown).
Significantly Increased Numbers of Total NK Cells in Allograft Biopsy Specimens With TCMR and AMR
In order to profile the major lymphocyte populations in human kidney allograft tissue, we enumerated total T (CD3+) cells, B (CD19+) cells, and NK (CD3− CD19− CD56+) cells in patient biopsies. Biopsies were stratified based on the histopathological pattern of rejection, classified as: unsuspicious biopsy (no rejection), borderline cellular rejection, TCMR or AMR.
To establish the independent association between lymphocyte numbers and the severity of cellular rejection as graded using Banff criteria, we firstly performed a subgroup analysis in which patients with a histopathological pattern of AMR were excluded. For this analysis, biopsies were stratified based on the severity of cellular rejection into borderline rejection and TCMR rejection (grades I–II). Quantification using Flow-Count Fluorospheres showed numbers of total T cells, B cells, and NK cells to be significantly increased in biopsy specimens with a histopathological pattern of TCMR compared with biopsy specimens with no evidence of rejection (Figures 2A–C; p < 0.05).
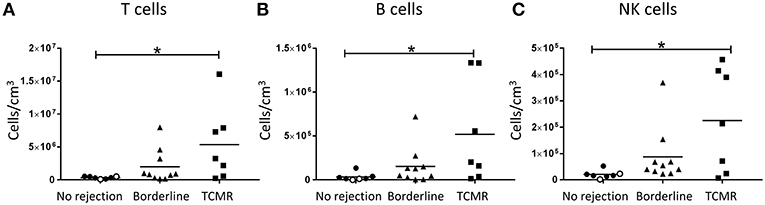
Figure 2. Significantly increased T cells, B cells, and NK cells in TCMR. Absolute numbers of total (A) T cells, (B) B cells, and (C) NK cells in kidney transplant biopsy tissue with histopathological diagnosis of no rejection (n = 7), borderline cellular rejection (n = 10), and T cell-mediated rejection (TCMR; grades I–II; n = 7). Values for individual donors are presented; donors with DSA are identified using open symbols; bars represent means. *P < 0.05, Kruskal-Wallis test with Dunn's post-test.
To independently assess lymphocyte numbers in association with AMR, a second subgroup analysis was performed in which patients with cellular rejection alone were excluded. Total T cell and NK cell numbers were significantly increased in biopsies with a histopathological pattern of AMR compared with no rejection (Figure 3A; p < 0.01 and Figure 3C; p < 0.001, respectively), whilst no statistical difference was observed for total B cells (Figure 3B). Collectively, these results associate human NK cells with both TCMR and AMR.
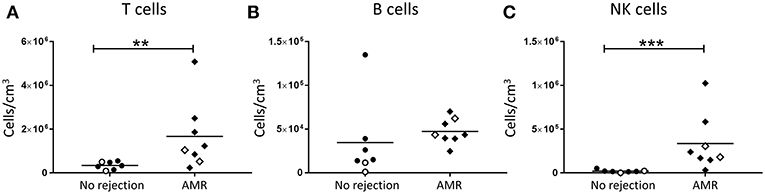
Figure 3. Significantly increased T cells and NK cells in AMR. Absolute numbers of total (A) T cells, (B) B cells, and (C) NK cells in kidney transplant biopsy tissue with histopathological diagnosis of no rejection (n = 7) and with indication of AMR (AMR; n = 8). Values for individual donors are presented; donors with DSA are identified using open symbols; bars represent means. **P < 0.01, ***P <0.001, Mann-Whitney test.
Significantly Increased Numbers of CD56bright NK Cells in Allograft Biopsy Specimens With TCMR
We next assessed human kidney NK cells at a subset level. Firstly, we examined the absolute numbers of CD56bright and CD56dim NK cell subsets in allograft biopsies with cellular rejection alone (borderline/TCMR). Notably, only the CD56bright NK cells were significantly elevated in the TCMR group compared with biopsies with no evidence of rejection (P < 0.01; Figures 4A,B). We also examined the phenotypes of the human kidney NK cell subsets in cellular rejection. Although not reaching statistical significance, expression levels of activation marker CD69 were elevated on CD56bright NK cells in the borderline/TCMR group compared with non-rejecting biopsies (Figure 4C), whilst CD69 expression levels on CD56dim NK cells were comparable between the two groups (Figure 4D).
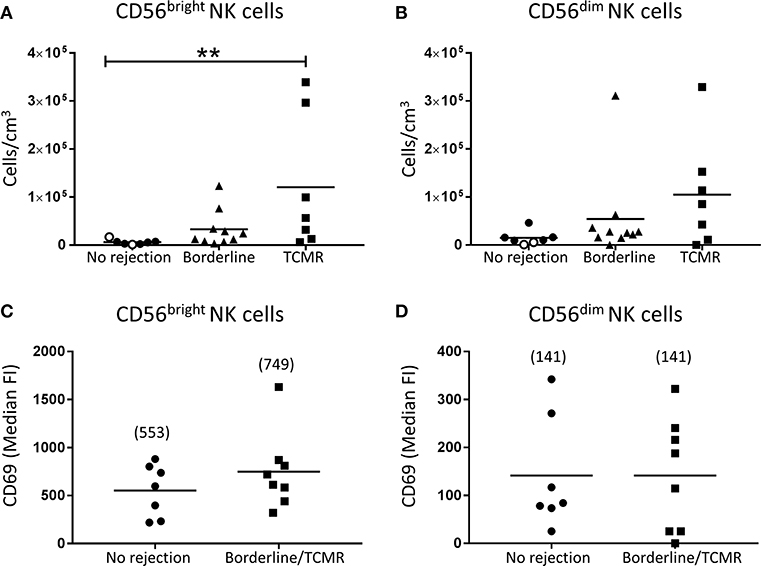
Figure 4. Significantly increased CD56bright NK cells in TCMR. (A,B) Absolute numbers of total (A) CD56bright NK cells and (B) CD56dim NK cells in kidney transplant biopsy tissue with histopathological diagnosis of no rejection (n = 7), borderline cellular rejection (n = 10) and T cell-mediated rejection (TCMR; grades I–II; n = 7). Values for individual donors are presented; donors with DSA are identified using open symbols; bars represent means. (C,D) Surface expression of CD69 on (C) CD56bright NK cells and (D) CD56dim NK cells in kidney transplant biopsy tissue with histopathological diagnosis of no rejection (n = 7) and borderline or T cell-mediated rejection (n = 8). Median fluorescence intensity (median FI) values for individual donors are presented; bars represent means, with mean values presented in parentheses. **P < 0.01, Kruskal-Wallis test with Dunn's post-test.
CD56dim NK Cells With an Activated Phenotype Associated With AMR
In order to investigate the role of human NK cell subsets in humoral rejection, we examined the absolute numbers of CD56bright and CD56dim NK cells in allograft biopsies with a histopathological pattern of AMR. Interestingly, both CD56bright (p < 0.001; Figure 5A) and CD56dim NK cell subsets (p < 0.01; Figure 5B) were significantly increased in the AMR group compared with non-rejecting biopsies.
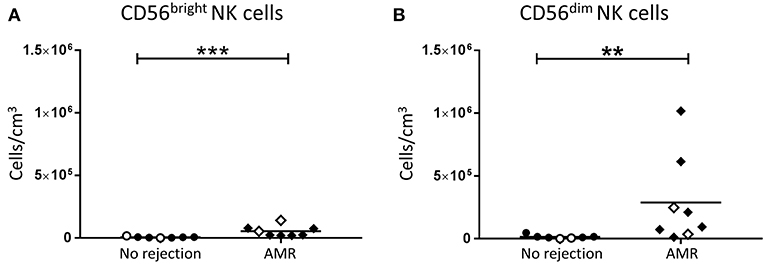
Figure 5. Significantly increased CD56bright NK cells and CD56dim NK cells in AMR. Absolute numbers of total (A) CD56bright NK cells and (B) CD56dim NK cells in kidney transplant biopsy tissue with histopathological diagnosis of no rejection (n = 7) and with indication of AMR (AMR; n = 8). Values for individual donors are presented; donors with DSA are identified using open symbols; bars represent means. **P < 0.01, ***P < 0.001, Mann-Whitney test.
Expression levels of activation markers on NK cell subsets were again assessed. Whilst CD69 expression levels on CD56bright NK cells were similar between the AMR and no rejection groups (Figure 6A), expression of CD69 on CD56dim NK cells was significantly elevated in AMR biopsies (p < 0.05; Figure 6B), suggestive of an activated phenotype. Human CD56dim NK cells are reported to downregulate CD16 expression and upregulate HLA-DR upon activation (16). Consistent with an activated phenotype, we observed trends toward lower CD16 expression (Figure 6C) and higher HLA-DR (Figure 6D) on CD56dim NK cells from biopsies with evidence of AMR; however, these did not reach statistical significance. Collectively, these results indicate that CD56dim NK cells shift toward a more activated state in the AMR micro-environment.
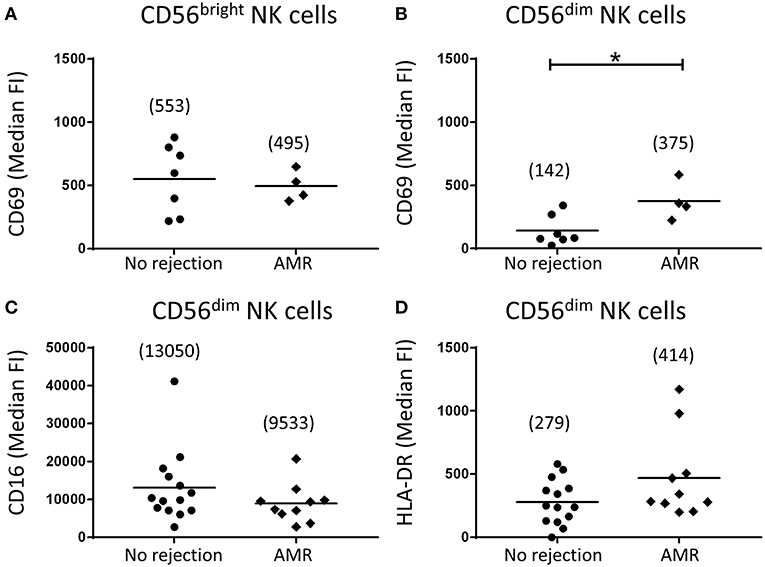
Figure 6. Human CD56dim natural killer (NK) cells in AMR biopsies display an activated phenotype. (A,B) Surface expression of CD69 on CD56bright NK cells (A) and CD56dim NK cells (B) in biopsy specimens without (no rejection; n = 7) and with AMR (n = 4). (C,D) Surface expression of (C) CD16 and (D) HLA-DR on CD56dim NK cells in biopsy specimens without (no rejection; n = 14) and with AMR (n = 10). Median fluorescence intensity (median FI) values for individual donors are shown; bars represent means, with mean values presented in parentheses. *P < 0.05, Mann-Whitney test.
Significantly Elevated Levels of Cytotoxic Effector Molecules in AMR Biopsies
The primary function of activated CD56dim NK cells is cytotoxicity through the release of effector molecules perforin, granulysin, and granzymes. Thus, the supernatants from dissociated allograft biopsies were analyzed for levels of these cytotoxic effector molecules. Consistent with a putative role for activated CD56dim NK cells in AMR, we observed significantly elevated levels of perforin (p < 0.01; Figure 7A), granulysin (p < 0.05; Figure 7B), and granzyme A (p < 0.01; Figure 7C) in biopsies with a histopathological pattern of AMR compared with non-rejecting biopsies.
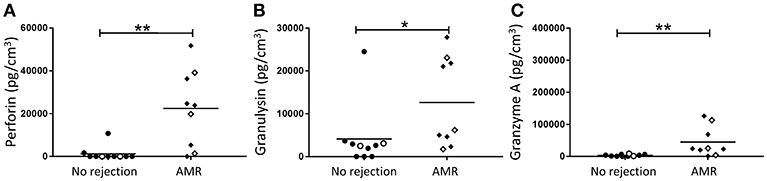
Figure 7. Human kidney tissue from patients with an indication of AMR have an elevated cytotoxic effector molecule profile. Expression of (A) Perforin, (B) Granulysin, and (C) Granzyme A in transplant biopsy specimens without (no rejection; n = 10) and with AMR (n = 9). Concentrations of effector molecules normalized to cubic centimeters of kidney tissue for individual donors are shown; donors with DSA are identified using open symbols; bars represent means. *P < 0.05, **P < 0.01, Mann-Whitney test.
Discussion
Current treatment modalities offer non-specific therapeutic targets in the treatment of TCMR and especially AMR, and while patients can be risk stratified using donor and recipient immunological (cytotoxic and flow cross-match, HLA mismatching, DSAs, and previous recipient sensitization) and non-immunological (ischemia-reperfusion injury, warm and cold ischemic time) parameters, no biomarker exists to predict which patients will develop renal allograft rejection. To more accurately define the local drivers of immune-mediated allograft rejection, we report the use of a multi-color flow cytometric approach to analyse immune cell populations in human renal allograft tissue. Muczynski et al. used this technique to examine the relative proportions (not absolute counts) of immune cell populations in kidney allograft rejection (25). Here, we report, for the first time, the use of this methodology to examine absolute numbers of discrete lymphocyte populations (including NK cell subsets) in different patterns of immune-mediated rejection (TCMR, AMR).
To focus in on putative drivers of grades of cellular rejection, we performed a subgroup analysis in which AMR biopsies were excluded and remaining biopsies stratified into borderline cellular rejection and TCMR (grades I–II). Using this approach, we observed significantly elevated numbers of total NK cells in TCMR biopsies, consistent with previous transcriptomic and IHC-based studies of cellular rejection. An early transcriptomic investigation of kidney allograft biopsies reported associations between high NK cell transcript expression and histological patterns of TCMR (14). More recently, IHC-based studies showed significant associations between human NK cells and TCMR (19, 20, 22). In agreement with our findings, dos Santos et al. reported significantly increased numbers of CD56+ cells associated with histopathological manifestations of interstitial inflammation and tubulitis; both hallmark features of TCMR (20, 22). Another study reported a positive correlation between the number of CD56+ cells and the severity of TCMR (19). However, these IHC-based evaluations have identified NK cells based on the expression of a single marker (e.g., CD56) and thus, none of these studies are able to irrefutably exclude the interference of CD3+ CD56+ NK-like T cells or evaluate NK cell subsets.
Through the use of multi-parameter flow cytometry, our group has extended these earlier investigations to unequivocally identify and characterize human CD56bright and CD56dim NK cell subsets in kidney allograft tissue. Indeed, in TCMR biopsies, we observed significantly elevated numbers of only the CD56bright NK cell subset (and not CD56dim NK cells). CD56bright NK cells function to trigger pathological immune responses through the production of pro-inflammatory cytokines (e.g., IFN-γ, TNF-α) and chemokines (e.g., monokine induced by gamma interferon; MIG) (9, 26, 27). It is thus tempting to speculate that CD56bright NK cells play a specialized functional role in TCMR pathology by secreting these pro-inflammatory molecules that, in turn: (1) enhance recruitment of alloreactive T cells (28, 29) and (2) upregulate HLA alloantigens (e.g., MHC class I and II) on target donor kidney cells to make them more susceptible to cytotoxic killing (26).
AMR remains one of the major barriers to graft survival in kidney transplant patients. Diagnoses of AMR center on the detection of DSA, complement deposition and the presence of inflammatory effector cells in the peritubular capillaries and glomeruli (30). Thus, we next identified and examined the role of effector NK cells in AMR pathology. We observed significantly elevated numbers of total NK cells in biopsies with a histopathological pattern of AMR, in line with earlier studies of humoral rejection. Indeed, previous investigations using single marker immunostaining (CD56 or NKp46) reported elevated NK cell numbers associated with peritubular capillaritis (13, 19), glomerulitis (22), microcirculatory inflammation, and peritubular C4d deposition (18), associating NK cells with microcirculatory injury. Furthermore, elevated numbers of CD56+ cells have been shown to correlate with AMR and poor graft survival (19). Differential gene expression analyses of allograft biopsies have also demonstrated NK cell-related transcriptomic signatures in biopsies from patients with DSA and microcirculatory damage (14, 18).
We extended the work of these studies by examining human NK cells in AMR biopsies at a subset level. We report here that numbers of both CD56bright and CD56dim NK cells were elevated in biopsies with a histological pattern of AMR. However, only CD56dim NK cells uniquely displayed significantly elevated CD69 levels in biopsy-proven AMR, suggesting this subset specifically is driven to an activated phenotype within the AMR micro-environment. Our results are in line with a recent study by Hoffman et al. showing that NK cells in the peripheral blood of kidney transplant recipients display an activated phenotype (16). In particular, this study reported the presence of activated circulating CD56dim NK cells in kidney transplant recipients, characterized by up-regulated CD69 and HLA-DR, as well as reduced expression of CD16 (16). Similarly, the kidney CD56dim NK cells in our AMR biopsies displayed elevated HLA-DR and reduced CD16 expression levels, although these did not reach statistical significance. Given that only this cytotoxic subset was both elevated in number and displayed an activated phenotype in AMR biopsies, our findings specifically implicate the CD16-expressing CD56dim NK cell subset in the pathogenesis of AMR.
Antibody-mediated activation of NK cells via triggering of CD16 on their cell surface has been proposed to drive antibody-dependent cellular cytotoxicity (ADCC) and thus, allograft rejection (21). Indeed, gene transcript profiling of AMR biopsies has provided initial evidence for NK cell activation and signaling via engagement of Fc receptor CD16 with IgG DSA (17). Critically, in our AMR biopsies, CD56dim NK cells express high levels of cell surface CD16, making them capable of engaging with DSA bound to allograft endothelial cells and, in turn, releasing cytotoxic granules (containing perforin, granulysin, and granzyme A) that can trigger targeted allograft apoptosis. In line with this concept, we found significantly elevated levels of perforin, granulysin, and granzyme A in biopsies with AMR. We speculate that CD56dim NK cells contribute to the burden of cytotoxic molecules observed in biopsies with AMR. In support of our hypothesis, a recent study of the molecular signatures in human AMR biopsies strongly associated endothelial injury and cytotoxic molecules characteristic of CD56dim NK cells [e.g., granulysin (GNLY) and FGFBP2] with chronic AMR (17). In conjunction with these earlier reports, our collective findings in AMR biopsies strongly support an ADCC-mediated pathogenic function for the human CD56dim NK cell subset in humoral rejection.
Previous studies have reported reduced numbers of CD56dim NK cells in the peripheral blood of kidney transplant recipients with indicative AMR (15, 31). In one study, the presence of DSA was associated with reduced numbers of CD56dim NK cells in peripheral blood of kidney transplant recipients compared to healthy donors (31). A second, larger cohort confirmed this finding, reporting patients with anti-HLA DSA had lower proportions and absolute numbers of peripheral blood CD56dim NK cells compared to patients without HLA antibodies (15). In the context of our hypothesis, a reduction in circulating CD56dim NK cells might reflect homing of this cytotoxic subset into the rejecting allograft.
It is problematic as to whether human NK cell functions are adequately inhibited by current immunosuppression regimes. An in vitro study of peripheral blood NK cells from kidney transplant recipients demonstrated that immunosuppression did not affect the capacity of NK cells to respond to stimulation. In this study, circulating NK cells from recipients receiving immunosuppression secreted equivalent levels of IFN-γ, perforin, and granzyme A in response to stimulation with HLA class I-negative K562 cells compared with NK cells from healthy individuals (16). The inability of current immunosuppression regimes to downregulate activated NK cell functions represents a therapeutic opportunity. Novel treatments with the specificity to target either activated NK cells and/or their effector functions warrant testing.
In addition to our novel human NK cell subset data, our present study also examined the absolute numbers of other lymphocyte populations (e.g., B lymphocytes) in different patterns of immune-mediated rejection. Notably, we demonstrated significant increases in absolute numbers of B lymphocytes in TCMR biopsies (Figure 2B), but not in AMR biopsies (Figure 3B). Although an unexpected result given the role of B lymphocyte lineage cells (plasma cells) in antibody production, these findings are in fact consistent with previous reports of B lymphocyte infiltration significantly associating with TCMR, but not with AMR (32, 33). These analogous findings from these earlier IHC-based studies further confirm the efficiency and integrity of our multi-color flow cytometric approach.
In summary, we report the first comprehensive characterization of discrete kidney NK cell subsets in human allograft rejection. Our data provide the first evidence that human kidney NK cells may have subset-specific functional roles in the pathobiology of TCMR vs. AMR. Further evaluation of the kidney NK cell compartment in allograft models will test its utility in high precision tissue-based diagnostics. In addition, this will foster therapeutic approaches that specifically target the recruitment (e.g., chemokine receptors) or triggering (e.g., activating receptors) of discrete NK cell subsets dependent on the pathological conditions.
Data Availability
All datasets generated for this study are included in the manuscript.
Ethics Statement
Written informed consent for participation in the study was obtained. The Royal Brisbane and Women's Hospital Human Research Ethics Committee (2006/072) and the Princess Alexandra Hospital Ethics Committee (HREC/16/QPAH/214) approved the study.
Author Contributions
Each author has participated sufficiently in the work to take public responsibility for the content. KK, RF, SH, GJ, JU, RW, AK, and HH conceived and designed the study. KK, SH, MH, KG, ES, BL, XW, and AK carried out experiments and analyzed the data. KK, SH, AK, and HH drafted the paper. All authors revised and approved the final version of the manuscript.
Funding
The work was funded by Pathology Queensland, a Royal Brisbane and Women's Hospital (RBWH) Research Grant, the Kidney Research Foundation and National Health and Medical Research Council (NHMRC) Project Grants (GNT1099222 and GNT1161319). KG was supported by an Australian Government Research Training Program (RTP) Scholarship. BL was supported by a Pathology Queensland – Study, Education and Research Committee (SERC) PhD Scholarship.
Conflict of Interest Statement
The authors declare that the research was conducted in the absence of any commercial or financial relationships that could be construed as a potential conflict of interest.
Acknowledgments
The authors would like to thank the tissue donors and clinicians, particularly renal histopathologists (Queensland Health), Dr. Leo Francis, Dr. Kim Oliver, Dr. Bibiana Tie, and Dr. Anne Stewart for assessment of rejection in kidney biopsies.
References
1. Kloc M, Ghobrial RM. Chronic allograft rejection: a significant hurdle to transplant success. Burns Trauma. (2014) 2:3–10. doi: 10.4103/2321-3868.121646
2. Racusen LC, Solez K, Colvin RB, Bonsib SM, Castro MC, Cavallo T, et al. The Banff 97 working classification of renal allograft pathology. Kidney Int. (1999) 55:713–23. doi: 10.1046/j.1523-1755.1999.00299.x
3. Solez K, Racusen LC. The Banff classification revisited. Kidney Int. (2013) 83:201–6. doi: 10.1038/ki.2012.395
4. Halloran PF, Wadgymar A, Ritchie S, Falk J, Solez K, Srinivasa NS. The significance of the anti-class I antibody response. I. Clinical and pathologic features of anti-class I-mediated rejection. Transplantation. (1990) 49:85–91. doi: 10.1097/00007890-199001000-00019
5. Trpkov K, Campbell P, Pazderka F, Cockfield S, Solez K, Halloran PF. Pathologic features of acute renal allograft rejection associated with donor-specific antibody, analysis using the Banff grading schema. Transplantation. (1996) 61:1586–92. doi: 10.1097/00007890-199606150-00007
6. Halloran PF. T cell-mediated rejection of kidney transplants: a personal viewpoint. Am J Transplant. (2010) 10:1126–34. doi: 10.1111/j.1600-6143.2010.03053.x
7. Spits H, Di Santo JP. The expanding family of innate lymphoid cells: regulators and effectors of immunity and tissue remodeling. Nat Immunol. (2011) 12:21–7. doi: 10.1038/ni.1962
8. Angelo LS, Banerjee PP, Monaco-Shawver L, Rosen JB, Makedonas G, Forbes LR, et al. Practical NK cell phenotyping and variability in healthy adults. Immunol Res. (2015) 62:341–56. doi: 10.1007/s12026-015-8664-y
9. Jacobs R, Hintzen G, Kemper A, Beul K, Kempf S, Behrens G, et al. CD56bright cells differ in their KIR repertoire and cytotoxic features from CD56dim NK cells. Eur J Immunol. (2001) 31:3121–6. doi: 10.1002/1521-4141(2001010)31:10<3121::AID-IMMU3121>3.0.CO;2-4
10. Zhang Z-X, Huang X, Jiang J, Lau A, Yin Z, Liu W, et al. Natural killer cells mediate long-term kidney allograft injury. Transplantation. (2015) 99:916–24. doi: 10.1097/TP.0000000000000665
11. Kohei N, Tanaka T, Tanabe K, Masumori N, Dvorina N, Valujskikh A, et al. Natural killer cells play a critical role in mediating inflammation and graft failure during antibody-mediated rejection of kidney allografts. Kidney Int. (2016) 89:1293–306. doi: 10.1016/j.kint.2016.02.030
12. Yagisawa T, Tanaka T, Miyairi S, Tanabe K, Dvorina N, Yokoyama WM, et al. In the absence of natural killer cell activation donor-specific antibody mediates chronic, but not acute, kidney allograft rejection. Kidney Int. (2019) 95:350–62. doi: 10.1016/j.kint.2018.08.041
13. Hidalgo L, Sis B, Sellares J, Campbell P, Mengel M, Einecke G, et al. NK cell transcripts and NK cells in kidney biopsies from patients with donor-specific antibodies: evidence for NK cell involvement in antibody-mediated rejection. Am J Transplant. (2010) 10:1812–22. doi: 10.1111/j.1600-6143.2010.03201.x
14. Hidalgo L, Sellares J, Sis B, Mengel M, Chang J, Halloran P. Interpreting NK cell transcripts versus T cell transcripts in renal transplant biopsies. Am J Transplant. (2012) 12:1180–91. doi: 10.1111/j.1600-6143.2011.03970.x
15. Crespo M, Yelamos J, Redondo D, Muntasell A, Perez-Saéz M, López-Montañés M, et al. Circulating NK-cell subsets in renal allograft recipients with anti-HLA donor-specific antibodies. Am J Transplant. (2015) 15:806–14. doi: 10.1111/ajt.13010
16. Hoffmann U, Neudörfl C, Daemen K, Keil J, Stevanovic-Meyer M, Lehner F, et al. NK cells of kidney transplant recipients display an activated phenotype that is influenced by immunosuppression and pathological staging. PLoS ONE. (2015) 10:e0132484. doi: 10.1371/journal.pone.0132484
17. Venner J, Hidalgo L, Famulski K, Chang J, Halloran P. The molecular landscape of antibody-mediated kidney transplant rejection: evidence for NK involvement through CD16a Fc receptors. Am J Transplant. (2015) 15:1336–48. doi: 10.1111/ajt.13115
18. Yazdani S, Callemeyn J, Gazut S, Lerut E, de Loor H, Wevers M, et al. Natural killer cell infiltration is discriminative for antibody-mediated rejection and predicts outcome after kidney transplantation. Kidney Int. (2018) 95:188–98. doi: 10.1016/j.kint.2018.08.027
19. Shin S, Kim YH, Cho YM, Park Y, Han S, Choi BH, et al. Interpreting CD56+ and CD163+ infiltrates in early versus late renal transplant biopsies. Am J Nephrol. (2015) 41:362–9. doi: 10.1159/000430473
20. dos Santos DC, Campos EF, Saraiva Câmara NO, David DSR, Malheiros DMAC. Compartment-specific expression of natural killer cell markers in renal transplantation: immune profile in acute rejection. Transpl Int. (2016) 29:443–52. doi: 10.1111/tri.12726
21. Hirohashi T, Chase C, Della Pelle P, Sebastian D, Alessandrini A, Madsen J, et al. A novel pathway of chronic allograft rejection mediated by NK cells and alloantibody. Am J Transplant. (2012) 12:313–21. doi: 10.1111/j.1600-6143.2011.03836.x
22. dos Santos DC, Saraiva Camara NO, David DSR, Malheiros DMAC. Expression patterns of CD56+ and CD16+ cells in renal transplant biopsies with acute rejection: associations with microcirculation injuries and graft survival. Nephrology. (2017) 22:993–1001. doi: 10.1111/nep.12897
23. Haas M, Sis B, Racusen LC, Solez K, Glotz D, Colvin RB, et al. Banff 2013 meeting report: inclusion of C4d-negative antibody-mediated rejection and antibody-associated arterial lesions. Am J Transplant. (2014) 14:272–83. doi: 10.1111/ajt.12590
24. Kildey K, Law BMP, Muczynski KA, Wilkinson R, Healy H, Kassianos AJ. Identification and quantitation of leukocyte populations in human kidney tissue by multi-parameter flow cytometry. Bioprotocol. (2018) 8:e2980. doi: 10.21769/BioProtoc.2980
25. Muczynski KA, Leca N, Anderson AE, Kieran N, Anderson SK. Multicolor flow cytometry and cytokine analysis provides enhanced information on kidney transplant biopsies. Kidney Int Rep. (2018) 3:956–69. doi: 10.1016/j.ekir.2018.02.012
26. Hadad U, Martinez O, Krams SM. NK cells after transplantation: friend or foe. Immunol Res. (2014) 58:259–67. doi: 10.1007/s12026-014-8493-4
27. Law BM, Wilkinson R, Wang X, Kildey K, Lindner M, Rist MJ, et al. Interferon-γ production by tubulointerstitial human CD56bright natural killer cells contributes to renal fibrosis and chronic kidney disease progression. Kidney Int. (2017) 92:79–88. doi: 10.1016/j.kint.2017.02.006
28. Hancock WW, Gao W, Faia KL, Csizmadia V. Chemokines and their receptors in allograft rejection. Curr Opin Immunol. (2000) 12:511–6. doi: 10.1016/S0952-7915(00)00130-8
29. Martín-Fontecha A, Thomsen LL, Brett S, Gerard C, Lipp M, Lanzavecchia A, et al. Induced recruitment of NK cells to lymph nodes provides IFN-γ for T H 1 priming. Nat Immunol. (2004) 5:1260. doi: 10.1038/ni1138
30. Racusen LC, Colvin RB, Solez K, Mihatsch MJ, Halloran PF, Campbell PM, et al. Antibody-mediated rejection criteria–an addition to the Banff′ 97 classification of renal allograft rejection. Am J Transplant. (2003) 3:708–14. doi: 10.1034/j.1600-6143.2003.00072.x
31. Neudoerfl C, Mueller BJ, Blume C, Daemen K, Stevanovic-Meyer M, Keil J, et al. The peripheral NK cell repertoire after kidney transplantation is modulated by different immunosuppressive drugs. Front Immunol. (2013) 4:46. doi: 10.3389/fimmu.2013.00046
32. Tsai EW, Rianthavorn P, Gjertson DW, Wallace WD, Reed EF, Ettenger RB. CD20+ lymphocytes in renal allografts are associated with poor graft survival in pediatric patients. Transplantation. (2006) 82:1769–73. doi: 10.1097/01.tp.0000250572.46679.45
Keywords: natural killer cells, innate lymphocytes, kidney allograft rejection, T cell mediated rejection, antibody-mediated rejection
Citation: Kildey K, Francis RS, Hultin S, Harfield M, Giuliani K, Law BMP, Wang X, See EJ, John G, Ungerer J, Wilkinson R, Kassianos AJ and Healy H (2019) Specialized Roles of Human Natural Killer Cell Subsets in Kidney Transplant Rejection. Front. Immunol. 10:1877. doi: 10.3389/fimmu.2019.01877
Received: 17 April 2019; Accepted: 24 July 2019;
Published: 07 August 2019.
Edited by:
Guseppe Grandaliano, University of Foggia, ItalyReviewed by:
Jakob Nilsson, University Hospital Zürich, SwitzerlandChristophe Picard, Établissement Français du Sang (EFS), France
Copyright © 2019 Kildey, Francis, Hultin, Harfield, Giuliani, Law, Wang, See, John, Ungerer, Wilkinson, Kassianos and Healy. This is an open-access article distributed under the terms of the Creative Commons Attribution License (CC BY). The use, distribution or reproduction in other forums is permitted, provided the original author(s) and the copyright owner(s) are credited and that the original publication in this journal is cited, in accordance with accepted academic practice. No use, distribution or reproduction is permitted which does not comply with these terms.
*Correspondence: Helen Healy, aGVsZW4uaGVhbHkmI3gwMDA0MDtoZWFsdGgucWxkLmdvdi5hdQ==
†These authors have contributed equally to this work