- Department of Neurology, The Third Affiliated Hospital of Sun Yat-sen University, Guangzhou, China
Gut microbiota has been proposed as an important environmental factor which can intervene and modulate central nervous system autoimmunity. Here, we altered the composition of gut flora with Clostridium butyricum and norfloxacin in experimental autoimmune encephalomyelitis (EAE), an animal model of multiple sclerosis. We found that appropriate C. butyricum (5.0 × 106 CFU/mL intragastrically daily, staring at weaning period of age) and norfloxacin (5 mg/kg intragastrically daily, 1 week prior to EAE induction) treatment could both ameliorate EAE although there are obvious differences in gut microbiota composition between these two interventions. C. butyricum increased while norfloxacin decreased the abundance and diversity of the gut microbiota in EAE mice, and both of the treatments decreased firmicutes/bacteroidetes ratio. In the genus level, C. butyricum treatment increased the abundance of Prevotella while Akkermansia and Allobaculum increased in norfloxacin treatment. Moreover, both interventions reduced Desulfovibroneceae and Ruminococcus species. Although there was discrepancy in the gut microbiota composition with the two interventions, C. butyricum and norfloxacin treatment both reduced Th17 response and increased Treg response in the gastrointestinal tract and extra-gastrointestinal organ systems in EAE mice. And the reduced activity of p38 mitogen-activated kinase and c-Jun N-terminal kinase signaling in spinal cord could be observed in the two interventions. The results suggested that manipulation of gut microbiota interventions should take factors such as timing, duration, and dosage into consideration. The discrepancy in the gut microbiota composition and the similar protective T cells response of C. butyricum and norfloxacin implies that achieving intestinal microecology balance by promoting and/or inhibiting the gut microbiota contribute to the well-being of immune response in EAE mice.
Introduction
Multiple sclerosis (MS) is a chronic autoimmune inflammatory demyelinating disease of the central nervous system (CNS) and its incidence continues to increase worldwide (1). The pathogenesis of MS is complex and therapeutic effects are not satisfying. Besides genetic factors, environmental factors also play an important role in pathogenesis of MS. Among them, gut microbiota can make a major contribution to the disease both in susceptibility and protection (2–4). Thus, the discovery of MS-related pathogenic and/or protective gut microbiota organisms or their products would provide novel opportunities for diagnosis and therapy of the disease. The latter would mainly rely on appropriate probiotic and/or antibiotic treatments, dietary modification, and fecal microbial transplantation.
Probiotics are live bacteria that can exert beneficial effects on the host when administered in appropriate amounts. Clostridium butyricum (C. butyricum) is a butyric acid-producing bacterium which has been widely used for improving gastrointestinal function as probiotics. It has been demonstrated to ameliorate experimental colitis and asthmatic in mice by reversing the imbalance of Th1/Th2 through IL-10 dependent mechanism (5, 6). C. butyricum also can protect against autoimmune diabetes in mice by modulating Treg/Th17 differentiation and generate a less pro-inflammatory immunological microenvironment in the gut (7). Moreover, it has been used for treatment of human gastrointestinal disease in clinical practice (8, 9). Rare reports was investigated the effects of C. butyricum on experimental autoimmune encephalomyelitis (EAE), a classical animal model of MS.
Antibiotics can easily affect the components of the gut microbiota and alleviate EAE (10, 11). The study of antibiotics on EAE did not focus on gut microbiota, but on other EAE pathogenesis-related components (12). Norfloxacin is a fluoroquinolone antibiotic which has pleiotropic effects beyond its bactericidal effect (13–15). It exerts immunomodulatory effects via maintaining low endotoxin levels and stimulating the production of IL-10 in experimental cirrhosis mice (13, 14). Moreover, norfloxacin inhibits lipopolysaccharide-induced pro-inflammatory cytokine production such as tumor necrosis factor-α (TNF-α) and interleukin-1β (IL-1β), then modifies inflammatory responses (15). However, gut microbiota intervention with norfloxacin on EAE has never been examined.
In the present study, C. butyricum and norfloxacin were selected as gut microbiota interventions on EAE. We found that appropriate C. butyricum and norfloxacin can ameliorate EAE although there are obviously different changes in gut microbiota composition between the two interventions. The benefit of these two interventions is associated with reduced Th17 response and increased Treg response in the gastrointestinal (GI) tract and extra-GI organ systems in EAE mice. And the reduced activity of p38 p38 mitogen-activated kinase (MARK) and c-Jun N-terminal kinase (JNK) signaling may contribute to the molecular mechanisms of these benefit effects.
Materials and Methods
Mice and Reagents
C57BL/6J WT female mice were purchased from Guangdong Medical Laboratory Animal Center (Guangzhou, China). All mice were maintained under specific pathogen free conditions at South China Agricultural University (Guangzhou, China). The mice were allowed to acclimatize to the laboratory for 1 week prior to beginning of the study. All experiments were performed using female mice in accordance with guidelines for animal care, created by according to the National Institutes of Health Guide for Care and Use of Laboratory Animals and approved by the Bioethics Committee of South China Agricultural University (Approval ID: 2018-D006). C. butyricum powder (GDBIO1501, GDBIO-TECH Biotechnology) was stored at −20°C. Norfloxacin were purchased from Ji Lin SUNFUNG MEDCINE Co., Ltd, and MOG35-55 peptide (MEVGWYRSPFSRVVHLYRNGK) was synthesized by CL. BioScientific CO., LTD (Xi'an, China). Amino acid sequences were confirmed by aminoacid analysis and mass spectroscopy. The purity of the peptide was >95%. Mycobacterium tuberculosis H37RA was purchased from Difco (Detroit, MI). Pertussis toxin (PTX) was purchased from Alexis Corp (San Diego, CA). FITC- or PC-5.5-conjugated anti-mouse CD4, PC-7A-conjugated anti-mouse IFN-γ (interferon-gamma), PE-conjugated anti-mouse IL-17A, PE-conjugated anti-mouse Foxp3 were purchased from eBioscience (San Diego, CA, USA). P38 MAPK, p-p38 MAPK, extracellular signal-regulated kinase (ERK) 1/2, p-ERK 1/2, JNK, p-SAPK/JNK were purchased from Cell Signaling Technology (USA).
Dose-Finding Experiments and Treatment of Mice
Mice were randomly assigned to four groups: control mice, PBS-treated EAE mice, C. butyricum-treated EAE mice, and norfloxacin-treated EAE mice. The concentrations of C. butyricum and norfloxacin were chosen on the basis of previous in vivo data and our preliminary dose-finding experiment. Because 3–4 weeks (weaning period) of age, is an ideal time for probiotic intervention (7, 16), 5.0 × 105, 5.0 × 106, and 5.0 × 107 CFU/mL concentrations of C. butyricum in PBS (200 μL) was intragastrical administration 3 weeks before EAE induction, and 5.0 × 106 CFU/mL concentration of C. butyricum was selected as the optimal dosage. One week prior to EAE induction, mice received norfloxacin in PBS 5 mg/kg intragastrically daily (14, 17).
Induction and Assessment of EAE
EAE was induced by the procedure which had been described previously (18, 19). Briefly, 6–8 week female mice received a subcutaneous injection in the flanks with 300 μg of MOG35-55 peptide per animal emulsified in CFA containing 500 ug of Mycobacterium tuberculosis H37RA. Immediately thereafter, and again 48 h later, the mice received an intraperitoneal (i.p.) injection of 300 ng of PTX in 100 μL of phosphate buffered saline (PBS). An additional injection of MOG35-55 peptide in CFA was delivered 7 days later. The animals were examined daily for disability. Clinical assessment of EAE scores was performed daily on a scale of 0–5 as instructed (19).
Histology Evaluation
After 21 days post immunization, different treated mice (n = 6) were fixed by heart perfusion with 4% (w/v) paraformaldehyde, and the lumbosacral spinal cords were obtained and embedded in paraffin. Paraffin sections were stained with hematoxylin and eosin and solochrome cyanin impregnation for evaluating inflammatory infiltration and demyelination, respectively. The scale evaluated for inflammation was as follows (20): 0, no inflammatory cells; 1, a few scattered inflammatory cells; 2, organization of inflammatory infiltrates around blood vessels; 3, extensive perivascular cuffing with extension into adjacent parenchyma, or parenchymal infiltration without obvious cuffing. Demyelination in the spinal cord was scored as previously described (21, 22): 1, traces of subpial demyelination; 2, marked subpial and perivascular demyelination; 3, confluent perivascular or subpial demyelination; 4, massive perivascular and subpial demyelination involving one half of the spinal cord with presence of cellular infiltrates into CNS parenchyma; 5, extensive perivascular and subpial demyelination involving the whole cord section with presence of cellular infiltrates into CNS parenchyma.
16S rRNA Gene Sequencing
Fresh extruded stools were collected before EAE treatment and immediately positioned in carbon dioxide ice for the gut microbial analysis. Feces DNA was extracted using QuickGene DNA tissue kit from Kurabo Company (Neyagawa, Japan) and sent for PCR amplification and sequencing of the V3 and V4 region of bacterial 16S rRNA genes using the Illumina MiSeq technology at BGI Co. (Shenzhen, China).
For alpha diversity analysis, we rarified the OTU to several metrics, including curves of OTU rank, rarefaction and Shannon, and calculated indexes of Shannon, Chao1, Simpson, and ACE. For beta diversity analysis, principal component analysis (PCA) was performed using QIIME (23). The LDA effect size (LEfSe) analysis was performed for the quantitative analysis of biomarkers among each group (24). Briefly, LEfSe analysis, LDA threshold of >4, used the non-parametric factorial Kruskal-Wallis (KW) sum-rank test and then used the (unpaired) Wilcoxon rank-sum test to identify the most differently abundant taxa.
Intracellular Cytokine and Intra-Nuclear Foxp3 Staining
For intracellular cytokine staining, lymphocytes isolated from designated organs 21 days after immunization were stimulated, fixed and permeabilized as instructed (25), followed by fluorescent-conjugated intracellular cytokine antibody staining. Intra-nuclear fork-head box p3 (Foxp3) was stained using the Foxp3 Staining Buffer Set (eBioscience, San Diego, CA, USA).
Western Blot
To investigate the protein expression of p38 MAPK, p-p38 MAPK, ERK1/2, p-ERK1/2, JNK, p-JNK in the spinal cord of control mice, PBS-treated EAE mice, C. butyricum-treated EAE mice, and norfloxacin-treated EAE mice, we performed Western blot analysis. Samples of the spinal cord from differently treated mice were loaded on 10% gradient sodium dodecyl sulfate-polyacrylamide gels (20 mg protein per lane). Proteins were transferred onto PVDF membrane (Bio-Rad). The membranes were blocked by 5% non-fat milk. Afterward, the membranes were incubated with p38 MAPK (1:1,000), p-p38 MAPK (1:1,000), ERK1/2 (1:1,000), p-ERK1/2 (1:1,000), JNK (1:1,000), p-JNK (1:1,000) overnight, respectively. After three times, washes with TBST buffer, the membrane was incubated with anti-mouse-HRP and goat anti-rabbit-HRP for 30 min, respectively. The experiment was repeated in triplicate and β-actin was used as internal control.
Statistical Analysis
Data were expressed as mean ± s.d., except for the clinical EAE score, which was expressed as mean ± s.e.m. Differences between two groups were analyzed by a two-tailed Student's t-test. ANOVA was used to compare difference of data from more than two groups, and the non-parametric data. Statistically significant data are indicated by asterisks (*P < 0.05, **P < 0.01, ***P < 0.001, and ****P < 0.0001). Experiments were repeated three time.
Results
Gut Microbiota Interventions With C. butyricum and Norfloxacin Ameliorated EAE
In an attempt to study the effects of the gut intervention on EAE, C.butyricum and norfloxacin treatment were used. We found that 5.0 × 105 CFU/mL C. butyricum treatment could not produce protective effects on EAE mice. Both 5.0 × 106 and 5.0 × 107 CFU/mL C. butyricum could suppress the severity of EAE while there were no significant differences between these two groups. Therefore, 5.0 × 106 CFU/mL was selected as the optimal dose in the following experiments. Results showed that both treatments significantly ameliorated the disease severity, as evidenced by reduced disease score (Figure 1A). As for neuropathology, both treatments could decrease lymphocyte infiltration and plaques of demyelination in lumber spinal cord (Figures 1B–E). These results indicated that gut microbiota interventions with C. butyricum and norfloxacin both could ameliorate clinical severity and neuropathology of EAE mice. And we did not find any obvious adverse effects of C. butyricum or norfloxacin at the chosen doses.
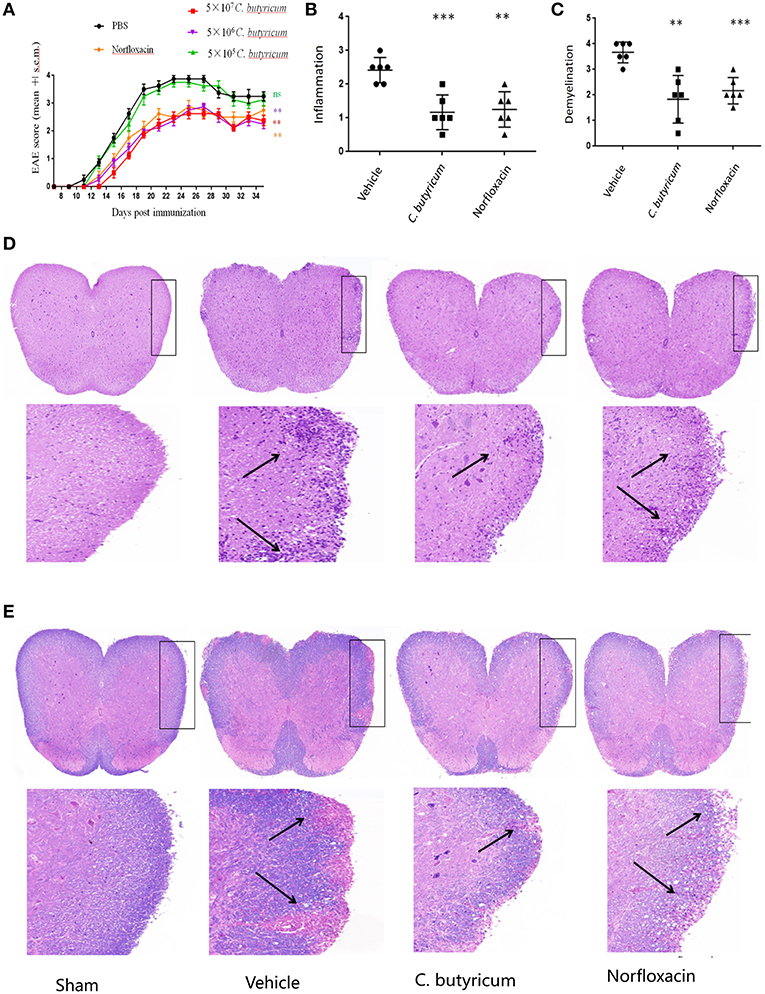
Figure 1. C.butyricum and norfloxacin treatment both ameliorated clinical severity and neuropathology of EAE mice. (A) Clinical score was assessed daily and shown (n = 8). (B–E) At 21 days post immunization, lumbosacral spinal cords were isolated and performed H&E (n = 6) (D) or Solochrome cyanin impregnation (E) staining for assessment of histopathology. Representative sections (D,E) and statistical analysis (B,C) data are shown. The infiltration of lymphocytes and demyelination are highlighted by arrow. Experiments were repeated three times with similar results. Statistically significant data are indicated by asterisks (**P < 0.01 and ***P < 0.001).
Gut Microbiota Interventions With C. butyricum and Norfloxacin Reconstituted the Composition of Intestinal Flora in EAE Mice
In order to investigate the gut microbial profile under C. butyricum and norfloxacin treatment, we analyzed the fecal bacteria from EAE mice by sequencing the bacterial 16s rRNA V3+V4 region. Based on 97% similarity level, all of the effective reads were clustered into operational taxonomic units (OTUs). The intestinal microbiota structural changes were then analyzed using unsupervised multivariate statistical methods PCA. Genus species phylogeny tree revealed the relationship between intestinal flora compositions in mice (Figure 2A). As shown in Figures 2B–J, three groups presented a distinct clustering of microbiota composition and norfloxacin decreased the number of OTUs while C. butyricum increased the numbers of OTUs. The observed species (Figure 2D), as well as the indexes of Chao1 (Figure 2E), ACE (Figure 2F), Shannon (Figure 2G), and Simpson (Figure 2H) were calculated. Consistent with the number of OTUs, norfloxacin treatment significantly reduced both the abundance and diversity while C. butyricum treatment increased the abundance and diversity of the intestinal microbiota.
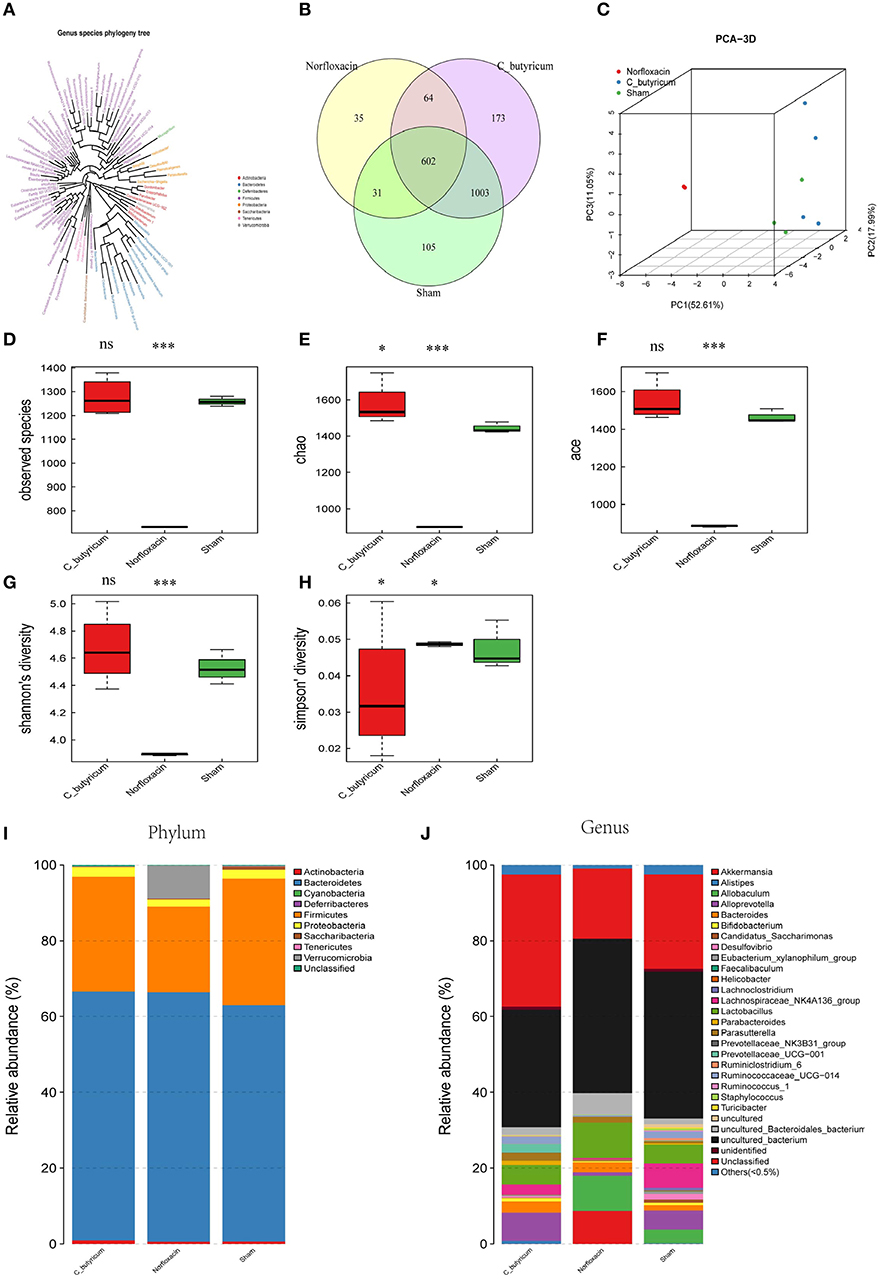
Figure 2. Responses of the diversity, richness, and structure of the gut microbiota to sham, C. butyricum, and norfloxacin treatment in EAE mice. (A) Genus species phylogeny tree of all three groups. (B) Venn diagram of each group and the number of differences between species are shown. (C) Unweighted Unifrac principal coordinates analysis plots 3D of each sample and the distance of each group are shown. Each sample was collected from three mice (n = 3). (D–H) shows the Observed species index, Shannon index, Chao index, Simpson index, and ACE index of each group. (I–J) Relative abundances of the gut microbiota at phylum level and genus level. Statistically significant data are indicated by asterisks (*P < 0.05 and ***P < 0.001). ns, not significant.
As shown in Figures 2I,J, the phylum level and genus level analysis demonstrated that both C. butyricum and norfloxacin administration significantly increased the relative abundance of Bacteroidetes and decreased the relative abundance of Firmicutes. Furthermore, norfloxacin notably increased the abundance of Verrucomicrobia. LEfSe and LDA score were employed to identify the differential distribution of microbiota among three groups (Figure 3). We found that C. butyricum induced a trend of a large increase in Prevotella while norfloxacin administration induced a trend of a large increase in Akkermansia and Allobaculum. Both C. butyricum and norfloxacin treatment can reduce the abundance of Desulfovibroneceae and Ruminococcus. Collectively, these results revealed the reconstitution of the gut microbiota composition in EAE mice when treated by C. butyricum or norfloxacin.
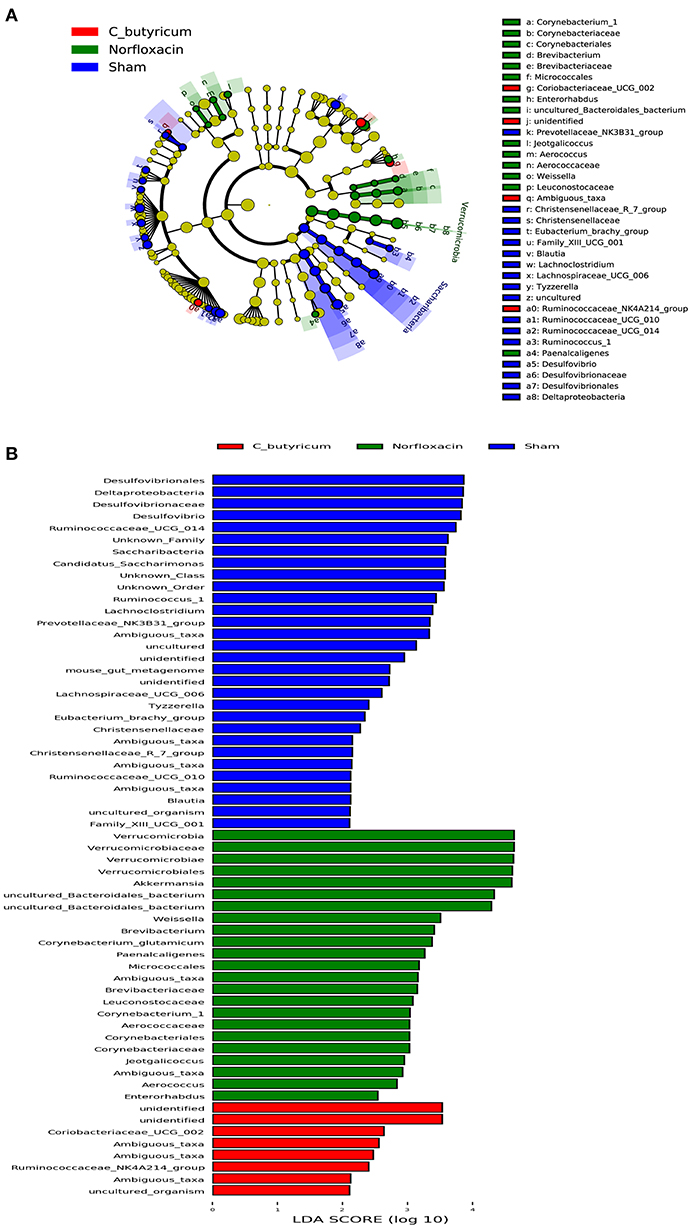
Figure 3. Identification of differential microbes in response to C.butyricum and norfloxacin treatment in mice based on the linear discriminant analysis (LDA) and effect size (LEfSe) pipeline. (A) Cladogram using LEfSe method indicated the phylogenetic distribution of gut microbiota associated with mice in three groups. (B) LDA scores showed the significant bacterial differences in three groups.
Gut Microbiota Interventions With C. butyricum and Norfloxacin Suppressed Th17 Cells Response and Increased Treg Response in EAE Mice
EAE is caused by aberrant T-cell responses to myelin self-peptides (26). And alterations of gut microbiota are involved in the changed T cells response in autoimmune diseases in the gastrointestinal (GI) tract and extra-GI organ systems (27). We further detected the levels of Th17 (CD4+ IL-17A+) and Th1 (CD4+ IFN-γ+) in the CNS, inguinal lymph nodes (LN), spleen, small intestine, and colon (Figure 4). And CD4+ Foxp3+ Treg cells in the LN, spleen, colon and small intestine were also analyzed in EAE mice (Figure 5). Results showed that C. butyricum reduced Th17 cells in all above-mentioned regions while norfloxacin reduced Th17 cells in CNS, LN, colon, and spleen except small intestine (Figures 4A–D). When it comes to Th1 cells, only the proportion of IFN-γ producing CD4+ T cells in the spleen from the C. butyricum group was reduced (Figures 4A–D). And no significant differences were noted in the percentages of Th1 cells in norfloxacin treatment in EAE mice (Figures 4A–D). We also found no statistical differences in IL-17A+ IFN-γ+ positive CD4 T cells in the treated groups (data not shown). Moreover, Treg cells decreased in the above regions while treatment with C. butyricum and norfloxacin promoted the Treg differentiation in EAE mice (Figures 5A,B).
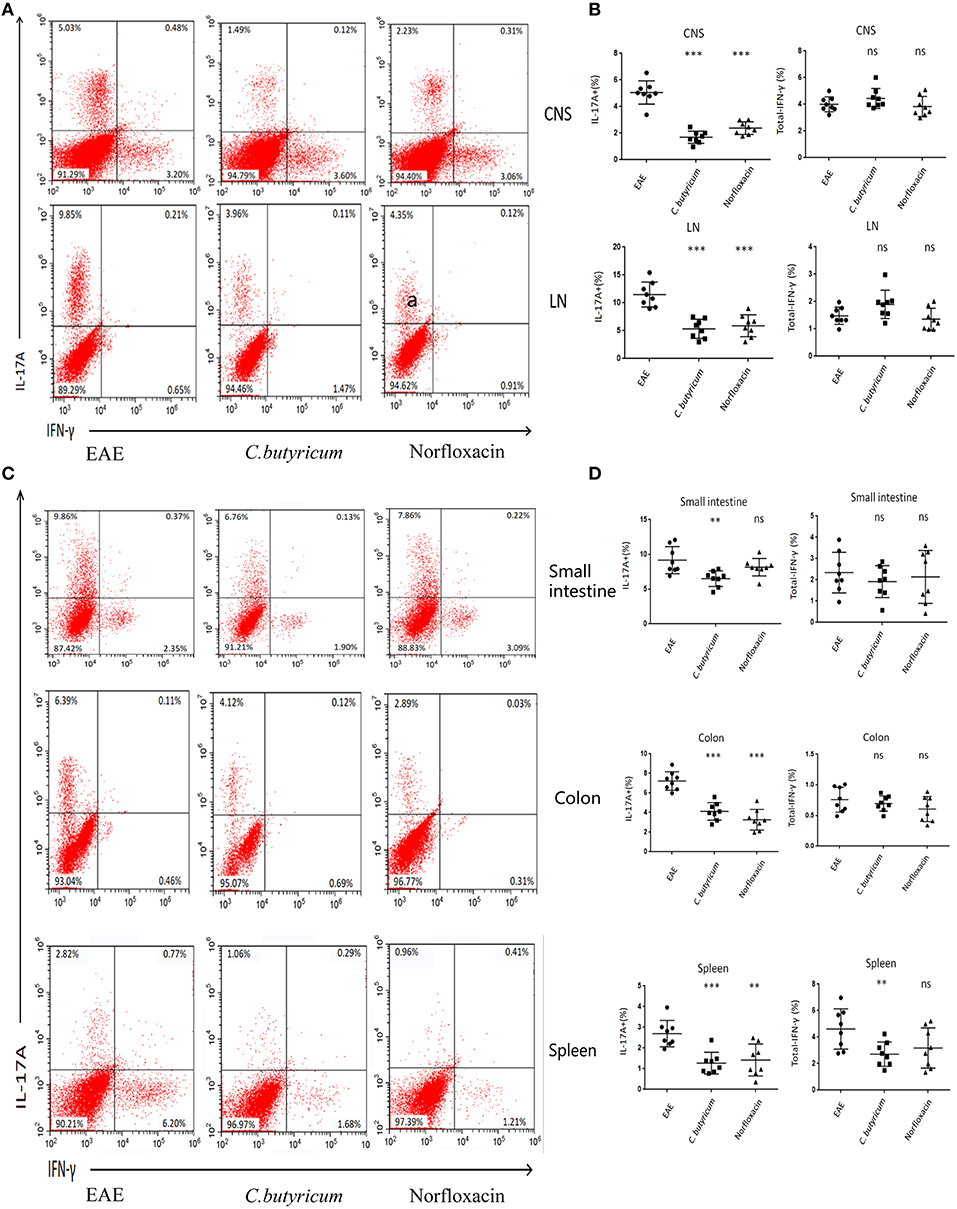
Figure 4. Gut microbiota intervention diminish Th17 responses in EAE. Lymphocytes from CNS, LN, small intestine, colon, and spleen were isolated 21 days post immunization and used for assessment of different CD4 T cell subsets. (A) Representative staining of different CD4 T cell subsets in CNS and LN, gated on TCRβ+ CD4+. (B) Statistical analysis of the percentages of IL-17 and IFN-γ in (A). Representative staining (D) and statistical analysis (C) of IL-17 and IFN-γ in CD4 T cells isolated from small intestine, colon, and spleen of mice, gated on TCRβ+ CD4+. Statistically significant data are indicated by asterisks (**P < 0.01 and ***P < 0.001). ns, not significant.
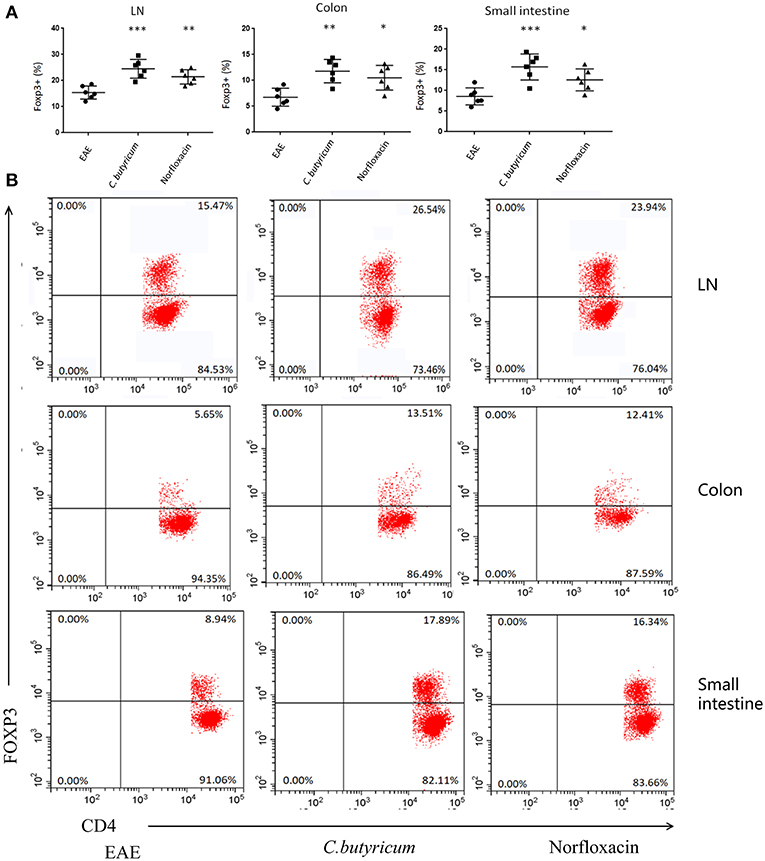
Figure 5. Gut microbiota intervention expanded the Treg cells in EAE. (B) Representative staining of different CD4 T cell subsets in inguinal LN, colon and small intestine, gated on TCRβ+ CD4+. Statistical analysis of data in (A). Statistically significant data are indicated by asterisks (*P < 0.05, **P < 0.01, and ***P < 0.001).
Gut Microbiota Interventions With C. butyricum and Norfloxacin Suppressed the MAPK Pathway in EAE Mice
T cell activation depends upon phosphorylation of MAPKs, which plays a critical role in the regulation of immune responses. MAPKs signaling, including p38 MAPK, ERK1/2, and JNKs, plays important roles in Th17 cell differentiation, which is a central player in MS and EAE (28, 29). We evaluated the expressions of MAPK signaling in the lumbar spinal cord of different groups by Western blot. We found that the level of phosphorylation of p38 MAPK, ERK1/2 and JNK was significantly increased in the lumbar spinal cord of EAE mice. Both C. butyricum and norfloxacin treatment suppressed the elevation of p38 MAPK and JNK, whereas the phosphorylation of ERK1/2 was not affected in the EAE mice (Figure 6).
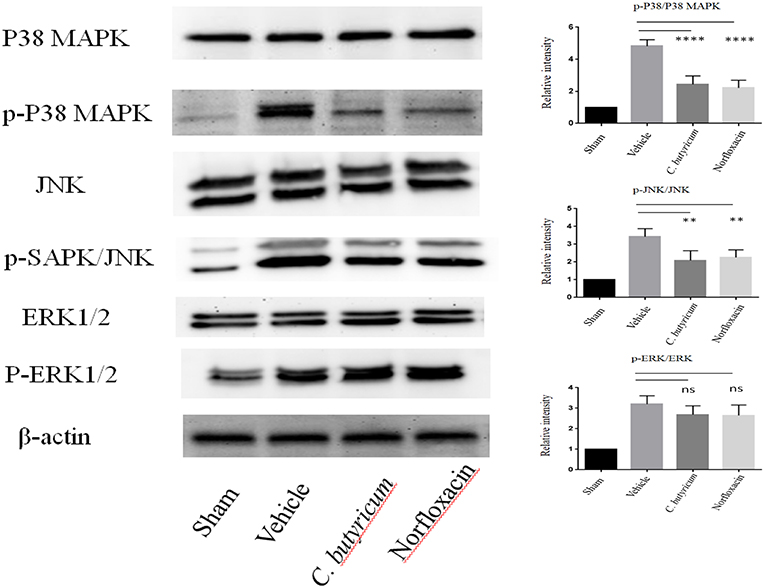
Figure 6. Gut microbiota intervention suppressed the phosphorylation of p38 MAPK and JNK, but not ERK1/2 in the lumbar spinal cord of EAE mice. The expressions of p38 MAPK, JNK, ERK1/2 and their phosphorylation expression were analyzed by Western blot. Statistically significant data are indicated by asterisks (**P < 0.01 and ****P < 0.0001). ns, not significant.
Discussion
Gut microbiota, particularly bacterial community has been proposed as an important environmental factor which can intervene and modulate CNS autoimmunity. Here, we demonstrated that altering gut flora by C. butyricum and norfloxacin could alleviate EAE in C57 BL/6 mice. In this experiment, the timing and duration of the two interventions were different. C. butyricum (5.0 × 106 CFU/mL) treatment started at 3–4 weeks aged mice because weaning period in C57 BL/6 mice is important for the development of its immune system (7, 16, 30). Early life antibiotics intervention can predispose an individual to develop CNS autoimmunity diseases in latter life, thus norfloxacin (5 mg/kg intragastrically/daily) treatment started 1 week prior to EAE induction (31, 32). We found that both interventions could ameliorate EAE while the alteration of gut microbiota composition is different. C. butyricum treatment increased the abundance and diversity of the gut microbiota in EAE mice. The results are consisted with previous reports that C. butyricum could regulate gut homeostasis and improve gastrointestinal function as probiotics. Also, it reconfirmed that weaning period of age is an ideal time for probiotic intervention (7, 16, 30). Norfloxacin treatment significantly reduced both the abundance and diversity of the gut microbiota in EAE mice. The reduced abundance and diversity of gut microbiota in different situation could produce discrepancy results after norfloxacin treatment (31, 32). It was reported that norfloxacin administration for 2 weeks could suppress gut flora thus enhance glucose tolerance in diabetes mice and show beneficial effects for improving glycemic control (31), while norfloxacin treatment for the same time duration induced gut drug resistance gene expression and restricted the growth of collembolans (32). In our experiment, 5 mg/kg/d norfloxacin treatment for 1 week before EAE immunization produced beneficial effects on EAE mice. The results remind us that manipulating gut microbiota interventions on EAE should consider the timing, dosage and duration, which may consequently lead to divergent outcomes.
In the phylum level analysis, supply of C. butyricum and norfloxacin both can significantly increase the abundance of Bacteroidetes and decrease the richness of firmicutes in EAE mice. Firmicutes and Bacteroidetes are the two major members of gut bacteria at the phylum level and play an important role in modulating host inflammation and immune status (33). And the elevated firmicutes/bacteroidetes ratio (F/B ratio) can describe pro-inflammatory environment and immunological imbalance characteristic of autoimmune disorders (34, 35). It was reported that bacteroidetes can regulate intestinal epithelium function and reduce inflammation (34, 35). Some of them produce short chain fatty acids (SCFAs) including acetate, propionate and butyrate. SCFAs were reported to promote production of anti-inflammatory cytokines transforming growth factor-β (TGFβ) and IL-10, then activate Treg cells and modulate inflammatory and immune responses in host (36). Firmicutes are thought to have important and core roles in the host's metabolism (37). It could lead to the increased production of metabolic endotoxins like lipopolysaccharides, which was able to enter the blood streams and caused the chronic inflammation (38). We found that decreased F/B ratio in C. butyricum and norfloxacin treated EAE mice. This may be one of the important factors that contribute to the alleviation of EAE. Moreover, norfloxacin treatment increased abundance of Verrucomicrobia which is usually used as probiotics for its capacity of reducing inflammatory immune responses (25).
When it comes to the genus level analysis, we found that treatment with C. butyricum was associated with increased relative abundance of Prevotella, while genus Akkermansia and Allobaculum increased in norfloxacin treated EAE mice. In MS patients, Prevotella was decreased without treatment but increased after treated with disease-modifying drugs such as interferon-β (IFN-β) and glatiramer acetate (GA) (3). Prevotella could also metabolize phytoestrogens into beneficial metabolites and promote the generation of butyrate (one of SCFAs) which has anti-inflammatory and immune-modulating effects (39–41). Akkermansia and Allobaculum are mucin-degrading bacteria and increased in norfloxacin treated EAE mice. Whether the increase of Akkermansia could exhibit beneficial activities by mucin degradation is controversial (42–47). It was reported that Akkermansia have immunoregulatory effects on converting mucin to SCFAs, and attenuating inflammation in adipose tissue through induction of Foxp3 regulatory T cells, and suppression of IL-6 and IL-1β (42, 43). And Akkermansia also plays a reverse role in degrading the mucus layer in proinflammation function (44, 45). The discrepancy suggests that whether Akkermansia produce pro- or anti-inflammatory effects depending on the immune status of the host. Allobaculum was reported to be inversely correlated with dietary-induced inflammation markers, including leptin and IL-22 (46, 47). Furthermore, both C. butyricum and norfloxacin treatment reduced Desulfovibroneceae and Ruminococcus species which are reported to be the IL-17 provocating bacteria (48, 49).
Moreover, we found although both C. butyricum and norfloxacin could decrease F/B ratio and alleviate EAE, the genus level analysis of gut microbiota was obviously different. This suggested that the overall change and outcome of intervention of gut microbiota is intricate. Each strain of bacteria may have good or bad or no effect on EAE, and the balance of the all the bacteria may determine the overall effect of gut microbiota. The final outcome of an intervention may depend on whether it could promote the good bacteria and/or inhibit bad bacteria to achieve a balanced and beneficial community in EAE mice. In different situation, a balanced community of gut microbiota may show inter-individual differences, so “good” or “bad” bacteria may be a shift status at different condition. And this can explain why the specific bacterial strains have distinct effects in different hosts as well as the same treatment may produce divergent outcomes in different status in autoimmune diseases.
Gut microbiota can modulate the immune response in a variety of ways, such as affecting antigen presentation and regulating the production of cytokines and the function of lymphocytes (50). We further investigated the effects of C. butyricum and norfloxacin treatment on T cell responses which play an important role in regulation and propagation of encephalitogenic immune damage (51). We found that C. butyricum treatment reduced CNS-, LN-, small intestine-, colon-, and spleen- infiltration of pro-inflammatory Th17 cells, and increased the percentages of Tregs in LN, colon, and small intestine. The above mentioned gut microbiota alternation may be responsible for this effect. As Th17 cells are considered as the main population of pathogenic T cells driving EAE while Treg cells are of paramount importance for suppressing inflammatory immune responses in EAE (25, 51, 52), the change of T cells response in our study account for the reduced clinical and neuropathology score in EAE mice in our experiment. The results are consistent with a series of researches in which beneficial effects of gut microbes intervention with probiotics on EAE were achieved through generation of Tregs (53–55). No significant differences were observed in the IFN-γ producing Th1 cells in CNS, LN, small intestine and colon. The results suggested that the Th1 response in these locations may not be affected by C. butyricum treatment in this experiment. Interestingly, C. butyricum decreased the frequency of Th1 cells in spleen. Since spleen is an important peripheral immune organ in EAE, reduced Th1 in spleen may attenuate the severity of EAE. Further studies to explore the relationships of C. butyricum, the gut microbes and the Th1 response are highly wanted.
Norfloxacin displayed reduced CNS-, LN-, colon-, and spleen- infiltration of pro-inflammatory Th17 cells and increased the percentages of Tregs were observed in LN, colon, and small intestine. Our results are consistent with previous studies which found that the protective effect of antibiotic treatment in EAE is related with a regulation of the abnormal in T cell responses including increasing secretion of Treg cells (10, 56). Besides the reduced F/B ratio and Desulfovibroneceae and Ruminococcus species, increased the Akkermansia and Allobaculum may also contribute to the beneficial effects. However, the Th17 of small intestine was not decreased in EAE mice. This result may be partly due to that Th17 cells mostly differentiate in small-intestinal lamina propria (57–59). And it may have better resistance capacity to norfloxacin treatment. No significant differences were noted in the percentages of Th1 cells in norfloxacin treatment. Our results verified the extra-anti-infective effects of norfloxacin on hosts through the modulation of gut microbiota and immune response in EAE mice. And this is consistent with previous reports that norfloxacin could enhance a regulatory T cell-mediated inflammatory control in cirrhosis by maintaining low IL-2 and IFN-γ levels and stimulating IL-10 production (13, 14).
The molecular mechanisms behind the protective effect of C. butyricum and norfloxacin may be associated with MAPK signaling. We found that the p38 MAPK and JNK, but not ERK1/2, were not activated by C. butyricum and norfloxacin administration. p38 MAPK-mediated signaling, a well-characterized integrator of environmental stressors (60), regulates numerous cellular events associated with the inflammatory response, cell proliferation and cell survival and induction of Th17 cell differentiation (60, 61). Additionally, the coupling of both the p38 and JNK-MAPK pathways to T cell receptor signaling might allow for lineage-specific signals in T cell differentiation (62). Collectively, these researches suggest that alterations in MAPK signaling may be associated with changes in the microbiota.
In conclusion, interventions with C. butyricum and norfloxacin influenced the composition of gut microbiota and, consequently, modulated the immune response in EAE mice. These findings support the idea that gut microbiota modulation has the potential to the future treatment of MS. The results also remind us that factors such as timing, duration and dosage must be carefully considered in probiotics and antibiotic-associated manipulation of the gut microbiota. The “good bacteria” are not always beneficial and “bad bacteria” are not always bad for hosts at any conditions. Recovery from dysbiosis by gut microbiota intervention on MS patients should consider the cross-talk between gut bacteria and immune status of the host. This study contributes to the pool of knowledge regarding the complex relationship between gut microbiota and CNS autoimmunity.
Data Availability
The raw data supporting the conclusions of this manuscript will be made available by the authors, without undue reservation, to any qualified researcher.
Ethics Statement
This study was carried out in accordance with the recommendations of guidelines for animal care, created by according to the National Institutes of Health Guide for Care and Use of Laboratory Animals and approved by the Bioethics Committee of South China Agricultural University (Approval ID: 2018-D006). The protocol was approved by the Bioethics Committee of South China Agricultural University.
Author Contributions
XC designed the research and charge correspondence. HC, XiM, ZC, XL, LS, and LM performed the experiments. HC, YL, XiM, and XC wrote the manuscript. All the authors read and approved the final manuscript.
Funding
This work was supported by grants (to XC) from the Israel Science Foundation (ISF) and the National Natural Science Foundation of China (NSFC) joint programme (813111290).
Conflict of Interest Statement
The authors declare that the research was conducted in the absence of any commercial or financial relationships that could be construed as a potential conflict of interest.
References
1. Koch-Henriksen N, Sorensen PS. The changing demographic pattern of multiple sclerosis epidemiology. Lancet Neurol. (2010) 9:520–32. doi: 10.1016/S1474-4422(10)70064-8
2. Chen J, Chia N, Kalari KR, Yao JZ, Novotna M, Paz Soldan MM, et al. Multiple sclerosis patients have a distinct gut microbiota compared to healthy controls. Sci Rep. (2016) 6:28484. doi: 10.1038/srep28484
3. Jangi S, Gandhi R, Cox LM, Li N, von Glehn F. Alterations of the human gut microbiome in multiple sclerosis. Nat Commun. (2016) 7:12015. doi: 10.1038/ncomms12015
4. Rothhammer V, Quintana FJ. Environmental control of autoimmune inflammation in the central nervous system. Curr Opin Immunol. (2016) 43:46–53. doi: 10.1016/j.coi.2016.09.002
5. Hayashi A, Sato T, Kamada N, Mikami Y, Matsuoka K, Hisamatsu T, et al. A single strain of Clostridium butyricum induces intestinal IL-10-producing macrophages to suppress acute experimental colitis in mice. Cell Host Microbe. (2013) 13:711–22. doi: 10.1016/j.chom.2013.05.013
6. Juan Z, Zhao-Ling S, Ming-Hua Z, Chun W, Hai-Xia W, Meng-Yun L, et al. Oral administration of Clostridium butyricum CGMCC0313-1 reduces ovalbumin-induced allergic airway inflammation in mice. Respirology. (2017) 22:898–904. doi: 10.1111/resp.12985
7. Jia L, Shan K, Pan L-L, Feng N, Lv Z, Sun Y, et al. Clostridium butyricum CGMCC0313.1 protects against autoimmune diabetes by modulating intestinal immune homeostasis and inducing pancreatic regulatory T cells. Front Immunol. (2017) 8:1345. doi: 10.3389/fimmu.2017.01345
8. Seki H, Shiohara M, Matsumura T, Miyagawa N, Tanaka M, Komiyama A, et al. Prevention of antibiotic-associated diarrhea in children by Clostridium butyricum MIYAIRI. Pediatr Int. (2003) 45:86–90. doi: 10.1046/j.1442-200X.2003.01671.x
9. Shimbo I, Yamaguchi T, Odaka T, Nakajima K, Koide A, Koyama H, et al. Effect of Clostridium butyricum on fecal flora in Helicobacter pylori eradication therapy. World J Gastroenterol. (2005) 11:7520–4. doi: 10.3748/wjg.v11.i47.7520
10. Yokote H, Miyake S, Croxford JL, Oki S, Mizusawa H. NK T cell-dependent amelioration of a mouse model of multiple sclerosis by altering gut flora. Am J Pathol. (2008) 173:1714–23. doi: 10.2353/ajpath.2008.080622
11. Ochoa-Repáraz J, Mielcarz DW, Ditrio LE, Burroughs AR, Foureau DM, Haque-Begum S, et al. Role of gut commensal microflora in the development of experimental autoimmune encephalomyelitis. J Immunol. (2009) 183:6041–50. doi: 10.4049/jimmunol.0900747
12. van den Hoogen WJ, Laman JD, t Hart BA. Modulation of multiple sclerosis and its animal model experimental autoimmune encephalomyelitis by food and gut microbiota. Front Immunol. (2017) 8:1081. doi: 10.3389/fimmu.2017.01081
13. Juanola O, Gómez-Hurtado I, Zapater P, Moratalla A, Caparrós E, Piñero P, et al. Selective intestinal decontamination with norfloxacin enhances a regulatory T cell-mediated inflammatory control mechanism in cirrhosis. Liver Int. (2016) 36:1811–20. doi: 10.1111/liv.13172
14. Gómez-Hurtado I, Moratalla A, Moya-Pérez Á, Peiró G, Zapater P, González-Navajas JM, et al. Role of interleukin 10 in norfloxacin prevention of luminal free endotoxin translocation in mice with cirrhosis. J Hepatol. (2014) 61:799–808. doi: 10.1016/j.jhep.2014.05.031
15. Ogino H, Fujii M, Ono M, Maezawa K, Hori S, Kizu J. In vivo and in vitro effects of fluoroquinolones on lipopolysaccharide-induced pro-inflammatory cytokine production. J Infect Chemother. (2009) 15:168–73. doi: 10.1007/s10156-009-0680-1
16. Atarashi K, Tanoue T, Shima T, Imaoka A, Kuwahara T, Momose Y, et al. Induction of colonic regulatory T cells by indigenous Clostridium species. Science. (2011) 331:337–41. doi: 10.1126/science.1198469
17. Gómez-Hurtado I, Gimenez P, García I, Zapater P, Francés R, González-Navajas JM, et al. Norfloxacin is more effective than Rifaximin in avoiding bacterial translocation in an animal model of cirrhosis. Liver Int. (2018) 38: 295–302. doi: 10.1111/liv.13551
18. Jiang Y, Zou Y, Chen S, Zhu C, Wu A, Liu Y, et al. The anti-inflammatory effect of donepezil on experimental autoimmune encephalomyelitis in C57 BL/6 mice. Neuropharmacology. (2013) 73:415–24. doi: 10.1016/j.neuropharm.2013.06.023
19. Stromnes IM, Goverman JM. Active induction of experimental allergic encephalomyelitis. Nat Protoc. (2006) 1:1810–9. doi: 10.1038/nprot.2006.285
20. O'Neill EJ, Day MJ, Wraith DC. IL-10 is essential for disease protection following intranasal peptide administration in the C57BL/6 model of EAE. J Neuroimmunol. (2006) 178:1–8. doi: 10.1016/j.jneuroim.2006.05.030
21. Kuerten S, Kostova-Bales DA, Frenzel LP, Tigno JT, Tary-Lehmann M, Angelov DN, et al. MP4- and MOG:35-55-induced EAE in C57BL/6 mice differentially targets brain, spinal cord and cerebellum. J Neuroimmunol. (2007) 189:31–40. doi: 10.1016/j.jneuroim.2007.06.016
22. Zappia E, Casazza S, Pedemonte E, Benvenuto F, Bonanni I, Gerdoni E, et al. Mesenchymal stem cells ameliorate experimental autoimmune encephalomyelitis inducing T-cell anergy. Blood. (2005) 106:1755–61. doi: 10.1182/blood-2005-04-1496
23. Caporaso JG, Kuczynski J, Stombaugh J, Bittinger K, Bushman FD, Costello EK, et al. QIIME allows analysis of high-throughput community sequencing data. Nat Methods. (2010) 7:335–6. doi: 10.1038/nmeth.f.303
24. Segata N, Izard J, Waldron L, Gevers D, Miropolsky L, Garrett WS, et al. Metagenomic biomarker discovery and explanation. Genome Biol. (2011) 12:60. doi: 10.1186/gb-2011-12-6-r60
25. Cignarella F, Cantoni C, Ghezzi L, Salter A, Dorsett Y, Chen L, et al. Intermittent fasting confers protection in CNS autoimmunity by altering the gut microbiota. Cell Metab. (2018) 27:1222–35. doi: 10.1016/j.cmet.2018.05.006
26. Stinissen P, Raus J, Zhang J. Autoimmune pathogenesis of multiple sclerosis: role of autoreactive T lymphocytes and new immunotherapeutic strategies. Crit Rev Immunol. (1997) 17:33–75. doi: 10.1615/CritRevImmunol.v17.i1.20
27. Atarashi K, Honda K. Microbiota in autoimmunity and tolerance. Curr Opin Immunol. (2011) 23:761–8. doi: 10.1016/j.coi.2011.11.002
28. Raman M, Chen W, Cobb MH. Differential regulation and properties of MAPKs. Oncogene. (2007) 26: 3100–12. doi: 10.1038/sj.onc.1210392
29. Dong C, Davis RJ, Flavell RA. MAP kinases in the immune response. Annu Rev Immunol. (2002) 20:55–72. doi: 10.1146/annurev.immunol.20.091301.131133
30. Kanai T, Mikami Y, Hayashi A. A breakthrough in probiotics: Clostridium butyricum regulates gut homeostasis and anti-inflammatory response in inflammatory bowel disease. J Gastroenterol. (2015) 50:928–39. doi: 10.1007/s00535-015-1084-x
31. Membrez M, Blancher F, Jaquet M, Bibiloni R, Cani PD, Burcelin RG, et al. Gut microbiota modulation with norfloxacin and ampicillin enhances glucose tolerance in mice. FASEB J. (2008) 22:2416–26. doi: 10.1096/fj.07-102723
32. Zhu D, An XL, Chen QL, Yang XR, Christie P, Ke X, et al. Antibiotics disturb the microbiome and increase the incidence of resistance genes in the gut of a common soil collembolan. Environ Sci Technol. (2018) 52:3081–90. doi: 10.1021/acs.est.8b02825
33. Chang CJ, Lin CS, Lu CC, Martel J, Ko YF, Ojcius DM, et al. Ganoderma lucidum reduces obesity in mice by modulating the composition of the gut microbiota. Nat Commun. (2015) 6:7489. doi: 10.1038/ncomms8489
34. Ley RE, Turnbaugh PJ, Klein S, Gordon JI. Microbial ecology: human gut microbes associated with obesity. Nature. (2006) 444:1022–3. doi: 10.1038/4441022a
35. Ley RE, Bäckhed F, Turnbaugh P, Lozupone CA, Knight RD, Gordon JI. Obesity alters gut microbial ecology. Proc Natl Acad Sci USA. (2005) 102:11070–5. doi: 10.1073/pnas.0504978102
36. Smith PM, Howitt MR, Panikov N, Michaud M, Gallini CA, Bohlooly-Y M, et al. The microbial metabolites, short-chain fatty acids, regulate colonic Treg cell homeostasis. Science. (2013) 341:569–73. doi: 10.1126/science.1241165
37. Ley RE, Lozupone CA, Hamady M, Knight R, Gordon JI. Worlds within worlds: evolution of the vertebrate gut microbiota. Nat Rev Microbiol. (2008) 6:776–88. doi: 10.1038/nrmicro1978
38. Poppleton DI, Duchateau M, Hourdel V, Matondo M, Flechsler J, Klingl A, et al. Outer membrane proteome of veillonella parvula: a diderm firmicute of the human microbiome. Front Microbiol. (2017) 8:1215. doi: 10.3389/fmicb.2017.01215
39. Schogor AL, Huws SA, Santos GT, Scollan ND, Hauck BD, Winters AL, et al. Ruminal Prevotella spp. may play an important role in the conversion of plant lignans into human health beneficial antioxidants. PLoS ONE. (2014) 9:e87949. doi: 10.1371/journal.pone.0087949
40. Wu GD, Chen J, Hoffmann C, Bittinger K, Chen YY, Keilbaugh SA, et al. Linking long-term dietary patterns with gut microbial enterotypes. Science. (2011) 334: 105–8. doi: 10.1126/science.1208344
41. Mangalam A, Shahi SK, Luckey D, Karau M, Marietta E, Luo N, et al. Human gut-derived commensal bacteria suppress central nervous system inflammatory and demyelinating disease. Cell Rep. (2017) 20:1269–77. doi: 10.1016/j.celrep.2017.07.031
42. Anhe FF, Roy D, Pilon G, Dudonne S, Matamoros S, Varin TV, et al. A polyphenolrich cranberry extract protects from diet-induced obesity, insulin resistance and intestinal inflammation in association with increased Akkermansia spp. Population in the gut microbiota of mice. Gut. (2015) 64:872–83. doi: 10.1136/gutjnl-2014-307142
43. Everard A, Belzer C, Geurts L, Ouwerkerk JP, Druart C, Bindels LB, et al. Cross-talk between Akkermansia muciniphila and intestinal epithelium controls dietinduced obesity. Proc Natl Acad Sci USA. (2013) 110:9066–71. doi: 10.1073/pnas.1219451110
44. Derrien M, Van Baarlen P, Hooiveld G, Norin E, Müller M, de Vos WM. Modulation of mucosal immune response, tolerance, and proliferation in mice colonized by the mucin-degrader Akkermansia muciniphila. Front Microbiol. (2011) 2:166. doi: 10.3389/fmicb.2011.00166
45. Ganesh BP, Klopfleisch R, Loh G, Blaut M. Commensal Akkermansia muciniphila exacerbates gut inflammation in Salmonella Typhimurium-infected gnotobiotic mice. PLoS ONE. (2013) 8:e74963. doi: 10.1371/journal.pone.0074963
46. Ravussin Y, Koren O, Spor A, LeDuc C, Gutman R, Stombaugh J, et al. Responses of gut microbiota to diet composition and weight loss in lean and obese mice. Obesity. (2012) 20: 738–47. doi: 10.1038/oby.2011.111
47. Zenewicz LA, Yin X, Wang G, Elinav E, Hao L, Zhao L, et al. IL-22 deficiency alters colonic microbiota to be transmissible and colitogenic. J Immunol. (2013) 190: 5306–12. doi: 10.4049/jimmunol.1300016
48. Chassaing B, Koren O, Carvalho FA, Ley RE, Gewirtz AT. AIEC pathobiont instigates chronic colitis in susceptible hosts by altering microbiota composition. Gut. (2014) 63:1069–80. doi: 10.1136/gutjnl-2013-304909
49. Chassaing B, Ley RE, Gewirtz AT. Intestinal epithelial cell toll-like receptor 5 regulates the intestinal microbiota to prevent low-grade inflammation and metabolic syndrome in mice. Gastroenterology. (2014) 147:1363–77. doi: 10.1053/j.gastro.2014.08.033
50. Chu F, Shi M, Lang Y, Shen D, Jin T, Zhu J, et al. Gut microbiota in multiple sclerosis and experimental autoimmune encephalomyelitis: current applications and future perspectives. Mediators Inflamm. (2018) 2018:8168717. doi: 10.1155/2018/8168717
51. Stanisavljević S, Dinić M, Jevtić B, Dedović N, Momčilović M, Dokić J, et al. Gut microbiota confers resistance of albino oxford rats to the induction of experimental autoimmune encephalomyelitis. Front Immunol. (2018) 9:942. doi: 10.3389/fimmu.2018.00942
52. Singh RP, Hasan S, Sharma S, Nagra S, Yamaguchi DT, Wong DT, et al. Th17 cells in inflammation and autoimmunity. Autoimmun Rev. (2014) 13:1174–81. doi: 10.1016/j.autrev.2014.08.019
53. Ochoa Repáraz J, Mielcarz DW, Wang Y, Begum-Haque S, Dasgupta S, Kasper DL, et al. A polysaccharide from the human commensal Bacteroides fragilis protects against CNS demyelinating disease. Mucosal Immunol. (2010) 3:487–95. doi: 10.1038/mi.2010.29
54. Ochoa-Repáraz J, Mielcarz DW, Ditrio LE, Burroughs AR, Begum-Haque S, Dasgupta S, et al. Central nervous system demyelinating disease protection by the human commensal Bacteroides fragilis depends on polysaccharide A expression. J Immunol. (2010) 185:4101–8. doi: 10.4049/jimmunol.1001443
55. Wang Y, Begum-Haque S, Telesford KM, Ochoa-Repáraz J, Christy M, Kasper EJ, et al. A commensal bacterial product elicits and modulates migratory capacity of CD39(+) CD4 T regulatory subsets in the suppression of neuroinflamma-tion. Gut Microbes. (2014) 5:552–61. doi: 10.4161/gmic.29797
56. Ochoa-Repáraz J, Rynda A, Ascón MA, Yang X, Kochetkova I, Riccardi C, et al. IL-13 production by regulatory T cells protects against experimental autoimmune encephalomyelitis independently of autoantigen. J Immunol. (2008) 181:954–68. doi: 10.4049/jimmunol.181.2.954
57. Ivanov II, Frutos Rde L, Manel N, Yoshinaga K, Rifkin DB, Sartor RB, et al. Specific microbiota direct the differentiation of IL-17-producing T-helper cells in the mucosa of the small intestine. Cell Host Microbe. (2008) 4:337–49. doi: 10.1016/j.chom.2008.09.009
58. Farkas AM, Panea C, Goto Y, Nakato G, Galan-Diez M, Narushima S, et al. Induction of Th17 cells by segmented filamentous bacteria in the murine intestine. J Immunol Methods. (2015) 421:104–11. doi: 10.1016/j.jim.2015.03.020
59. Haghikia A, Jörg S, Duscha A, Berg J, Manzel A, Waschbisch A, et al. Dietary fatty acids directly impact central nervous system autoimmunity via the small intestine. Immunity. (2015) 43:817–29. doi: 10.1016/j.immuni.2015.09.007
60. Min S, Li L, Zhang M, Zhang Y, Liang X, Xie Y, et al. TGF-β-associated miR-27a inhibits dendritic cell-mediated differentiation of Th1 and Th17 cells by TAB3, p38 MAPK, MAP2K4 and MAP2K7. Genes Immun. (2012) 13:621–31. doi: 10.1038/gene.2012.45
61. Kleinewietfeld M, Manzel A, Titze J, Kvakan H, Yosef N, Linker RA, et al. Sodium chloride drives autoimmune disease by the induction of pathogenic TH17 cells. Nature. (2013) 496:518–22. doi: 10.1038/nature11868
Keywords: Clostridium butyricum, norfloxacin, Th17/Treg, gut microbiota, EAE, multiple sclerosis
Citation: Chen H, Ma X, Liu Y, Ma L, Chen Z, Lin X, Si L, Ma X and Chen X (2019) Gut Microbiota Interventions With Clostridium butyricum and Norfloxacin Modulate Immune Response in Experimental Autoimmune Encephalomyelitis Mice. Front. Immunol. 10:1662. doi: 10.3389/fimmu.2019.01662
Received: 08 March 2019; Accepted: 03 July 2019;
Published: 23 July 2019.
Edited by:
Pamela Ann McCombe, University of Queensland, AustraliaReviewed by:
Clara Ballerini, University of Florence, ItalyIain Comerford, University of Adelaide, Australia
Copyright © 2019 Chen, Ma, Liu, Ma, Chen, Lin, Si, Ma and Chen. This is an open-access article distributed under the terms of the Creative Commons Attribution License (CC BY). The use, distribution or reproduction in other forums is permitted, provided the original author(s) and the copyright owner(s) are credited and that the original publication in this journal is cited, in accordance with accepted academic practice. No use, distribution or reproduction is permitted which does not comply with these terms.
*Correspondence: Xiaohong Chen, eGlhb2hvbmdjaGVuenNzeSYjeDAwMDQwO2FsaXl1bi5jb20=
†These authors have contributed equally to this work as co-first authors