- 1Institute for Immunology and Informatics, University of Rhode Island, Providence, RI, United States
- 2Department of Medicine, University of Minnesota Medical School, Minneapolis, MN, United States
- 3Department of Virology, Armed Forces Research Institute of Medical Sciences, Bangkok, Thailand
- 4Viral Diseases Branch, Walter Reed Army Institute of Research, Silver Spring, MD, United States
- 5Department of Microbiology and Immunology, State University of New York-Upstate Medical University, Syracuse, NY, United States
Prior exposure to dengue virus (DENV) has a profound impact on the outcome of infection, which varies according to the interval between infections. Antibodies secreted by B cells and cytokines secreted by T cells are thought to contribute both to protective immunity against DENV and the pathogenesis of dengue disease. We analyzed peripheral blood mononuclear cells (PBMC) collected from Thai children over a 5-year prospective cohort study to define the dynamics of DENV-specific memory B and T cell responses and the impact of symptomatic or subclinical DENV infections. To measure B cell responses, PBMC were stimulated with IL-2 plus R848 and culture supernatants were tested for DENV-binding antibodies by ELISA. To measure T cell responses, PBMC were stimulated in dual-color ELISPOT assays with overlapping peptide pools of structural and non-structural proteins from the four DENV types. B cell responses were low to one or more DENV types prior to symptomatic infection and increased with reactivity to all four types after infection. Subjects who had a subclinical infection or who did not experience a DENV infection during the study period showed strong memory B cell responses to all four DENV types. T cell responses to DENV peptides demonstrated a cytokine hierarchy of IFN-γ > IL-2 > IFN-γ/IL-2. T cell responses were low or absent prior to secondary infections. The trends in T cell responses to DENV peptides over 3 year post-infection were highly variable, but subjects who had experienced a secondary DENV1 infection showed higher cytokine responses compared to subjects who had experienced a secondary DENV2 or subclinical infection. The longitudinal nature of our study demonstrates persistent memory B cell responses over years and a lasting but variable impact of secondary DENV infection on DENV-specific T cell responses.
Introduction
Immune memory is the hallmark of the adaptive immune response. The generation and persistence of memory B and T cells are key to protection against a subsequent infection with a pathogen and are the principles behind effective vaccination. Most of our understanding of durable adaptive immune responses to viruses comes from mouse models where pathogens are administered in germ-free environments under controlled conditions. Studies in mice, however, do not recapitulate the exposure in humans to multiple pathogens or even different strains of the same pathogen over time.
Dengue is the most important arthropod-borne viral disease caused by infection with any of the four dengue virus serotypes (DENV1-4) (1, 2). Most infections are subclinical but in some cases lead to clinical symptoms ranging from mild febrile illness (dengue fever, DF) to the life-threatening dengue hemorrhagic fever/dengue shock syndrome (DHF/DSS) (3). A primary infection with any of the four DENV serotypes elicits long-lasting homotypic immunity (4, 5), although there are a few reported cases of homotypic reinfections (6–9). In endemic areas, periodic inapparent exposures to DENV may boost this DENV-specific immunity (10–12). However, infection with a different DENV serotype (heterotypic secondary infection) is associated with an increased risk for DHF/DSS (4, 5, 13). Both antibodies and T cells are hypothesized to contribute to this increased risk of severe disease through a variety of mechanisms, although other data point to their ability to protect against severe DENV disease (14–20). Furthermore, evidence for a lower risk of severe disease during third or fourth DENV infections suggests that the cumulative effects of cross-reactive memory immune responses are beneficial (21–23).
The degree to which B and T cell immune responses remain stable or fluctuate over time after DENV infection may influence the balance between protective and detrimental effects of DENV-specific immune responses, but remains relatively poorly characterized. In a prospective cohort study conducted in Kamphaeng Phet province in north-central Thailand, we collected peripheral blood mononuclear cells (PBMC) over a 5-year period from children who were followed for the occurrence of DENV infections. These samples provided the opportunity to analyze the dynamics, magnitude, and durability of memory B and T cell responses to DENV over an extended period. We found that both B and T cell responses were low before symptomatic infection and increased after infection, albeit with different kinetics. In contrast, PBMC from subjects who did not experience a DENV infection during the 5-year study period had higher antibody titers at baseline and maintained broadly reactive memory B cell responses over the 5-year period. Our data demonstrate persistent memory B cell responses over years and a lasting but variable impact of secondary DENV infection on DENV-specific T cell responses.
Materials and Methods
Study Subjects
Subjects were selected from a 5-year prospective cohort study in Thailand that has been described elsewhere (24). In brief, blood samples from schoolchildren were collected during January of every year from 1998 to 2002. PBMC were cryopreserved in liquid nitrogen until used. In addition, sera were collected in June, August, and November of each year. Surveillance for school absences and febrile illnesses was conducted between June and November each year; acute and convalescent blood samples were collected from children with fever or history of fever. Symptomatic DENV infection was identified by febrile illness with laboratory confirmation of acute DENV infection defined by virus isolation and/or reverse transcriptase polymerase chain reaction (RT-PCR) in acute serum samples or seroconversion between acute and convalescent serum samples by IgM/IgG ELISA or hemagglutination inhibition (HAI) assay. Subclinical infection was defined by the absence of symptoms and ≥4-fold increase in HAI antibody titer from June–August or August–November. Acute primary or secondary DENV infection were distinguished by IgM/IgG ratio and HAI titers as previously described (24, 25). Subjects who consistently had no detectable antibody or stable (<4-fold change) antibody titer by HAI assay over the 5-year period were defined as having had no DENV infection during the study. Written informed consent was obtained from each subject or his/her parent or guardian. The study protocol was approved by the Institutional Review Board of the Thailand Ministry of Public Health, the Human Use Review and Regulatory Agency of the Office of the U.S Army Surgeon General and the Institutional Review Board of the University of Massachusetts School of Medicine.
Detection of Memory B Cell-Derived Total IgG, DENV-Specific IgG, and DENV-Specific IgG Subclasses
Approximately 1–2 ×106 PBMC were stimulated with 2.5 μg/ml of R848 (Invitrogen, San Diego, CA, USA) and 1,000 U/ml of IL-2 (Peprotech, Rocky Hill, NJ, USA) in a 48 well plate for 7 days at 37°C and 5% CO2. Culture supernatants were tested for total IgG by Human IgG ELISA Quantitation Set according to the manufacturer's instructions (No. E80-104, Bethyl Laboratories, Inc., Montgomery, TX, USA). Total IgG levels in the supernatants from stimulated PBMC ranged from 4.21 to 32.73 μg/ml (mean 16.92 μg/ml). Samples with a concentration below the limit of detection (1.56 μg/ml) were excluded. DENV-specific IgG and the four IgG subclasses (IgG1-4) were detected in the supernatant by ELISA. Briefly, 96 well plates were coated overnight with 10 ng/well of DENV1-4 virus-like particles (VLPs) (Native Antigen Company). The plates were blocked for 90 min with 1% BSA. The diluted culture supernatant was added to the wells for 60 min. Plates were washed and goat anti-human IgG coupled to HRP (A80-104P; Bethyl Laboratories Inc., Montgomery, TX, USA) was added for total DENV-specific IgG detection. HRP conjugated secondary antibodies for IgG subclasses detection include goat anti-human IgG1 FC (9054-05), IgG1 hinge (9052-05), IgG2 FC (9060-05), IgG3 hinge (9210-05), and IgG4 FC (9200-05); all were from Southern Biotechnology Associates, Birmingham, AL, USA. The assay was developed with TMB substrate (34021; Thermo Scientific, MA, USA), stopped with 1M HCL, and read at 450 nm.
Peptide Pools
To evaluate memory T cell responses, we used four peptide pools spanning the prM and E proteins from DENV1-4, four peptide pools spanning NS1, NS3, and NS5 proteins (NSA pools) from DENV1-4, and one peptide pool spanning NS2a/b, NS4a/b, and C protein from DENV2 (NSB pool). Peptide pools contained peptides that ranged in length from 12 to 20 amino acids (aa), in overlap from 10 to 14 aa. The total number of peptides per pool ranged from 88 to 323 aa (Table S1). Peptides were obtained from NIH Biodefense & Emerging Infections Research Resources Repository (BEI Resources, Bethesda, MD, USA) and Peptide technologies (JPT, Acton, MA, USA). Further details of the peptides are available at http://www.beiresources.org/.
Ex vivo Dual Color IL-2 and IFN-γ Enzymatic ELISPOT Assay
The ELISPOT assay was performed according to the manufacturer's instructions (CTL, Cleveland, OH, USA). Cryopreserved PBMC were thawed and plated at a density of 1–2 × 105 cells/well. Peptide pools were added at a final concentration of 2 μg/ml/peptide. As a positive control, PBMC were incubated with anti-CD3 and anti-CD28 antibodies at final concentrations of 1 and 0.1 μg/ml, respectively. As a negative control, PBMC were incubated with medium. PBMC were stimulated for 45 h at 37°C with 5% CO2. As a positive control, every plate had PBMC from a well-characterized DENV-immune subject tested with the same conditions. The number of spots per well was determined using an automated ELISPOT reader (S5UV analyzer, CTL, Cleveland, OH, USA) with the double color software. Determinations from duplicate wells were averaged. Data were analyzed by subtracting the mean number of spots in the wells with cells and medium-only from the mean counts of spots in wells with cells and antigen and expressed as spot-forming cells (SFC) per 106 PBMC. If the response to anti-CD3/CD28 antibodies was below 500 IFN-γ SFC per million PBMC, the sample was excluded.
Statistical Analysis
Statistical analysis was performed using GraphPad Prism software V 8.00 (GraphPad Software Inc., La Jolla, CA). The non-parametric Mann-Whitney U or Wilcoxon signed rank test was used to compare two groups as appropriate. Statistical significance was set at P < 0.05.
Results
Characteristics of the Study Population
PBMC from 27 subjects were selected for study based on available data from 5-years of follow-up (Table S2). Sixteen subjects were selected for B cell assays: six who had symptomatic DENV infection, four who had a subclinical infection and six subjects with no DENV infection. Twenty-five subjects were selected for T cell assays: 13 who had a symptomatic secondary DENV infection (six with DENV1, six with DENV2, and one without a serotype determined), six who had a subclinical infection, and six with no DENV infection. For the symptomatic and subclinical infection groups, we selected subjects who experienced an infection in the first or second year of the study to allow us to test PBMC collected 1–2 years before and 3–4 years after secondary DENV infection. HAI antibody titers to the four DENV serotypes at baseline (start of the study) were higher in the subclinical and no DENV infection groups compared to the symptomatic DENV1 and DENV2 groups (p < 0.05) (Figure S1).
Memory B Cell Responses to DENV
To study the dynamics, magnitude, and durability of the memory B cell (MBC) responses to DENV, PBMC from 16 subjects were stimulated with IL-2 plus R848. Dengue-specific IgG antibodies were assessed in supernatants of stimulated PBMC by ELISA using VLPs from each of the four DENV serotypes (Figures 1A–C). A total of 74 PBMC samples were analyzed. DENV-specific MBC responses were low to the infecting serotype prior to secondary DENV infection in hospitalized subjects 7, 13, 14, and 15 while they were high to all four serotypes in two subjects who were not hospitalized (Figure 1A, Figure S2A). For some subjects, MBC responses were low to one or more additional DENV serotypes. MBC responses increased in the first year after infection, with cross-reactivity to all four serotypes suggesting an expansion of DENV-cross-reactive B cells (Figure 1A, Figure S2). The increased MBC response was maintained in PBMC from two of four hospitalized individuals with symptomatic infection (subjects 13 and 14) for at least 3–4 years after infection (Figure 1A). In contrast, MBC responses to all four DENV serotypes were high and persisted over the 5 years in 2 of 4 subjects with subclinical infection and 5 of 6 subjects studied who did not experience a DENV infection over the 5-year period of observation (Figures 1B,C). Serial dilution of supernatants did not reveal preferential binding to a specific DENV serotype (Figure 1D, Figure S2). DENV-specific antibodies detected in MBC culture supernatants were predominantly of the IgG1 subclass (Figures 1E,F). IgG subclass-specific responses did not differ between subjects with symptomatic vs. no DENV infection.
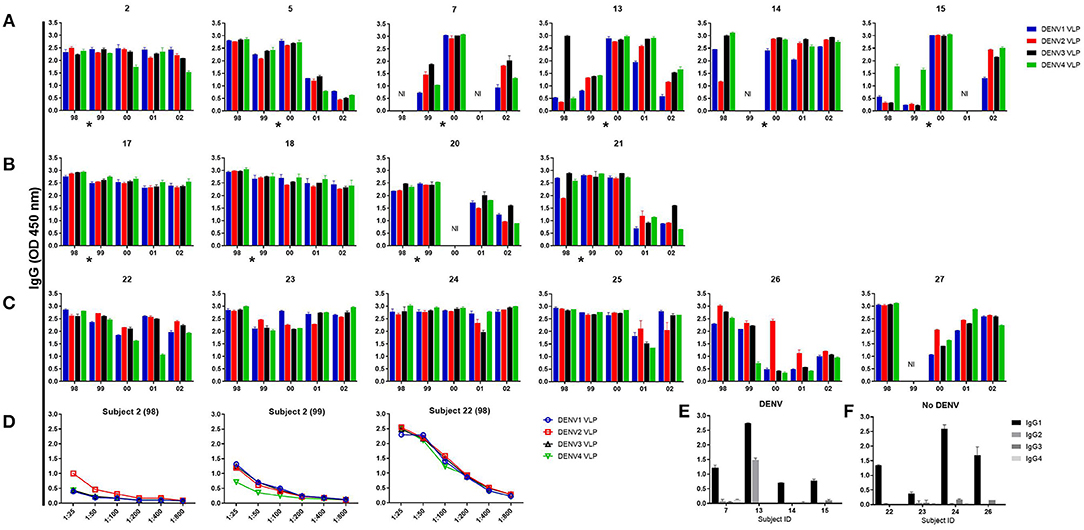
Figure 1. Memory B cell responses to DENV. Peripheral blood mononuclear cells from 6 subjects with symptomatic secondary DENV infection (subjects 2 and 5 were not hospitalized, subjects 7, 13, 14, and 15 were hospitalized) (A), 4 subjects with subclinical infection (B), and 6 subjects with no DENV infection (C) were cultured with R848 plus IL-2 for 7 days and supernatants were tested for DENV-specific IgG Abs. Representative data of supernatant dilution from a subject with symptomatic and no DENV infection (D). DENV-specific IgG isotypes in supernatants from the year 2000 in 4 subjects with DENV and 4 subjects with no DENV, respectively (E,F). Bars represent mean with standard error of the mean of the OD values. The asterisk indicates the approximate time when DENV infection occurred. NI, not included.
Memory T Cell Responses to DENV
To assess the dynamics, magnitude, and durability of the memory T cell responses to DENV, 106 PBMC samples from 25 subjects were stimulated with peptide pools of structural and non-structural proteins. DENV-specific memory T cells producing IFN-γ, IL-2, or IFN-γ/IL-2 were determined by dual color ELISPOT. Because of the limited number of cells available, PBMC from subjects who experienced a symptomatic DENV1 or DENV2 infection were stimulated with DENV1 or DENV2 prM/E peptides, respectively, in addition to all non-structural peptide pools. PBMC from subjects with a subclinical or no DENV infection were stimulated with prM/E peptides from all four DENV serotypes in addition to the non-structural peptide pools (Table S1).
Overall, T cell responses to DENV peptide pools demonstrated a hierarchy of cytokine production of IFN-γ > IL-2 > IFN-γ/IL-2 in all subjects (Figure S3). DENV-specific IFN-γ responses were low or absent prior to symptomatic DENV infection. Responses increased after infection in most of the subjects (Figure 2, Figure S4). However, the peak frequency of DENV-specific IFN-γ-producing memory T cells was not uniformly detected in the first sample collected after infection. Overall, the difference in magnitude of DENV-specific IFN-γ responses before vs. after infection did not reach statistical significance. DENV-specific T cells were somewhat more stable over the 5-year period in subjects who did not experience a DENV infection (Figure 2).
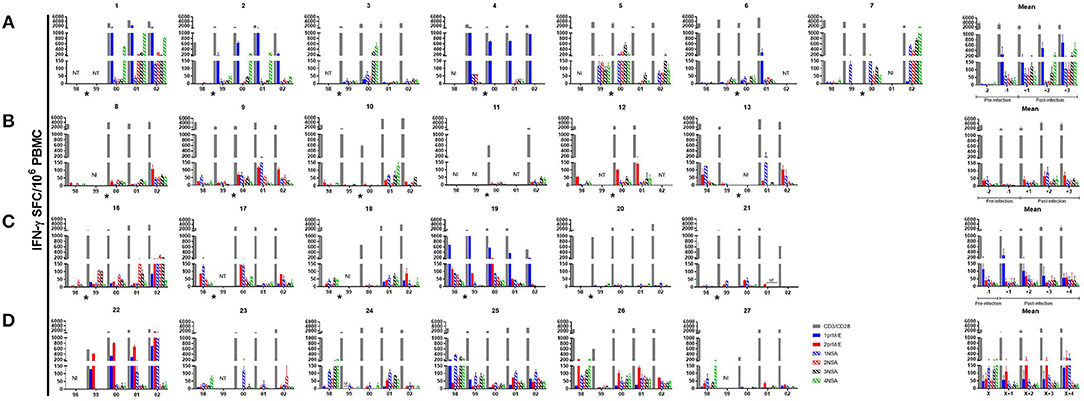
Figure 2. IFN-γ ELISPOT responses in individual subjects. DENV peptide-specific interferon γ (IFN-γ) spot-forming cells (SFC) were determined by dual color ELISPOT in PBMC collected at the indicated time points. PBMC were collected at the beginning of each year. Subjects who experienced a (A) DENV1 infection (B) DENV2 infection (C) subclinical dengue infection and (D) no dengue infection over the 5-year period. Bars represent mean with standard error of the mean for duplicate wells. The asterisk indicates the approximate time when DENV infection occurred. NT, not tested; NI, not included.
DENV-specific IFN-γ responses in PBMC collected in the first year of the study (baseline responses) reflect immune responses potentially predictive of outcome. There were no significant differences in baseline DENV-specific IFN-γ responses between subjects who subsequently had a symptomatic infection vs. those who had a subclinical infection. However, baseline responses tended to be higher in subjects who did not experience a DENV infection compared to the other groups (symptomatic or subclinical DENV infection; Figure S5).
DENV-specific IFN-γ responses in PBMC collected in later years of the study assess the effects of secondary DENV infection on memory T cell responses. There was a trend toward higher IFN-γ responses following symptomatic DENV1 infection compared to the other groups (symptomatic DENV2, subclinical or no DENV infection), but this did not reach statistical significance (Figure 2, Figure S6). As expected, PBMC collected post-infection showed IFN-γ responses to more than one DENV serotype (Figure 2).
Discussion
We conducted a longitudinal analysis of memory B and T cell responses to DENV over a 5-year period in primary schoolchildren living in a dengue-endemic area. Subjects were chosen to elucidate the impacts of incident DENV infections, both clinical and subclinical, on DENV-specific immunological memory, to follow changes in DENV-specific immunological memory over 3+ years after infection, and to compare findings in subjects who had symptomatic, subclinical, or no DENV infections during the study period. Other studies have measured memory B and T cell responses to DENV, however, these have largely been cross-sectional studies of individuals following natural DENV infection or vaccination (19, 26–33). The longitudinal nature of our study, availability of PBMC samples both before and after DENV infection, and the inclusion of groups with different DENV experience led to several novel observations.
Our assay of memory B cell responses to DENV used ELISA to evaluate binding to virions by antibodies secreted from circulating memory B cells after in vitro stimulation. Responses were detected in PBMC collected prior to an incident DENV infection. This finding is consistent with the known high baseline seroprevalence in this population and supports the secondary nature of most infections in our cohort even when titers of HAI and neutralizing antibody were undetectable (15, 34, 35). These samples also showed lower responses to one or more serotype(s), which in each case included the serotype that caused the next infection. This finding suggests that assays of memory B cells might help to assess susceptibility to infection among DENV-experienced individuals, which has been difficult to define based on serum assays (14, 36). The high responses to all four serotypes in study subjects who did not experience a DENV infection may therefore reflect protective immunity in those subjects (37).
Following an incident DENV infection, memory B cell responses increased in magnitude and breadth, consistent with a model wherein serotype-cross-reactive memory B cells are preferentially expanded by sequential DENV infections (32, 33, 38). However, while memory B cell responses increased in the earliest blood sample collected after infection, there was substantial heterogeneity in the durability of this increase between study subjects and, for each subject, across viral serotypes. Human memory B cells have been detected decades after infection or vaccination, but are less durable for some vaccines and pathogens (39–44). Our data imply an important role of subject-specific and cell-intrinsic factors in the durability of DENV-specific memory B cells (44). Further studies are needed to define these factors and their relationship to susceptibility or resistance to subsequent DENV infection or disease.
We measured memory T cell responses to DENV using dual IFN-γ/IL-2 ELISPOT assays and stimulation with overlapping peptide pools. In contrast to memory B cell responses, we did not detect memory T cell responses in all subjects. Whereas, some studies of adult volunteers after natural DENV infection or administration of live virus vaccine candidates have reported DENV-specific memory T cell responses in nearly all subjects (26, 45, 46), our results are in line with other cross-sectional studies of dengue-endemic populations (15, 16, 19, 27, 29, 47–50). Other factors that may contribute to lower memory T cell responses in our study include the young age of our subjects, the number of prior exposures to DENV, and other endemic infections (51, 52). Memory T cell responses were generally lower in those subjects who experienced a symptomatic DENV infection, as we observed for memory B cells. There have been very few prospective studies of DENV-specific memory T cell responses in PBMC collected prior to secondary DENV infection (15, 49, 53). An inverse relationship was previously found in our cohort between DENV-specific T cell responses measured by intracellular staining for IFN-γ or IL-2 and symptomatic DENV infection (15). Others reported an inverse correlation between DENV-specific IFN-γ ELISPOT responses and HLA class I-related susceptibility to dengue (16).
Memory T cell responses to DENV were higher in the PBMC collected after an incident DENV infection, but these responses were quite variable over a 3-year period, and responses were not consistently highest in the samples collected closest in time to the infection. Previous studies have either been cross-sectional in nature (26, 27, 29, 30) or covered a short time period (46, 47, 54), and therefore are not directly comparable to our findings. It is possible that measurement of other T cell functions would have reflected different trends in cell frequency (55–57). Given ongoing DENV transmission in the study area throughout the period of observation, it is also possible that some subjects experienced additional exposures to DENV that modulated T cell frequency but were not detected as significant increases in antibody titer.
Our findings must be interpreted in the context of several limitations of our study design. The quantity of PBMC available from the small blood volumes collected from young children was a major consideration for assay design. As a result, we could not test all antigen specificities or effector functions of memory B and T lymphocytes. Antibody responses to NS1 (58), T cell responses to other structural and non-structural antigens (59, 60), and multiparameter single-cell analyses (46, 61) would be of interest and might modify some of our conclusions. We used pools containing a large number of overlapping peptides in T cell ELISPOT assays, which has been reported to be suboptimal for detection of responses (19, 46, 62–64). We studied memory T and B cell responses in PBMC; lymphocyte repertoires in blood and tissue have been shown to differ in some infections, such as influenza (65). As DENV causes systemic infection, the most relevant tissues have not been defined, and most other studies of the immune repertoire have utilized PBMC. The narrow criteria we used for selection of cohort subjects limited the number available for these studies and reduced the statistical power for comparisons between groups. Our cohort study design along with these criteria also restricted the age range, host genetics, and geographic distribution of study subjects, which is likely to have limited the diversity of prior DENV infection history (66). Comparable studies in other populations will be needed to determine the generalizability of our findings.
Overall, this longitudinal analysis of memory B and T cell responses to DENV and the impacts of intervening symptomatic and subclinical DENV infections supports the paradigm of selective expansion of DENV-specific immunological memory by sequential exposures, but reveals additional heterogeneity between and within individual subjects than could be ascertained from cross-sectional studies. This heterogeneity should be considered in the interpretation of data from cohort studies of natural DENV infection and clinical trials of dengue vaccines. We suggest that further studies are warranted to evaluate the associations with susceptibility vs. resistance to DENV infection and disease.
Ethics Statement
Written informed consent was obtained from each subject or his/her parent or guardian. The study protocol was approved by the Institutional Review Board of the Thailand Ministry of Public Health, the Human Use Review and Regulatory Agency of the Office of the U.S Army Surgeon General and the Institutional Review Board of the University of Massachusetts School of Medicine.
Author Contributions
LS-V, AS, AM, and AR conceived and designed the experiments and wrote the manuscript text. LS-V, SK, and MS performed experiments and prepared figures. LS-V conducted statistical analyses. AS, KA, DE, and TE supervised the clinical study, subject enrollment, and collection of clinical data and blood samples. AS, KA, and DE contributed to the analysis of clinical, virologic, and serologic data. JC contributed to the analysis of immunological data. All authors contributed to the final manuscript and agree with the results and conclusions.
Funding
This work was supported by NIH grant P01AI034533 and the U.S. Military Infectious Diseases Research Program, and core facilities supported by NIH grant P20 GM104317.
Disclaimer
Material has been reviewed by the Walter Reed Army Institute of Research. There is no objection to its presentation and/or publication. The opinions or assertions contained herein are the private views of the author, and are not to be construed as official, or as reflecting true views of the Department of the Army or the Department of Defense. The investigators have adhered to the policies for protection of human subjects as prescribed in AR 70–25. The opinions expressed are those of the authors and do not represent official positions of the National Institutes of Health.
Conflict of Interest Statement
AR has received compensation as a consultant to Sanofi Pasteur, Takeda, and Merck.
The remaining authors declare that the research was conducted in the absence of any commercial or financial relationships that could be construed as a potential conflict of interest.
Acknowledgments
We are especially grateful to the children and parents of Kamphaeng Phet for their participation. We would like to thank the donors who generously provided PBMC for use in our studies.
Supplementary Material
The Supplementary Material for this article can be found online at: https://www.frontiersin.org/articles/10.3389/fimmu.2019.01359/full#supplementary-material
References
1. Bhatt S, Gething PW, Brady OJ, Messina JP, Farlow AW, Moyes CL, et al. The global distribution and burden of dengue. Nature. (2013) 496:504–7. doi: 10.1038/nature12060
2. Brady OJ, Gething PW, Bhatt S, Messina JP, Brownstein JS, Hoen AG, et al. Refining the global spatial limits of dengue virus transmission by evidence-based consensus. PLoS Negl Trop Dis. (2012) 6:e1760. doi: 10.1371/journal.pntd.0001760
3. Guzman MG, Kouri G. Dengue and dengue hemorrhagic fever in the Americas: lessons and challenges. J Clin Virol. (2003) 27:1–13. doi: 10.1016/S1386-6532(03)00010-6
4. Sabin AB. Research on dengue during World War II. Am J Trop Med Hyg. (1952) 1:30–50. doi: 10.4269/ajtmh.1952.1.30
5. Halstead SB. Observations related to pathogensis of dengue hemorrhagic fever. VI. Hypotheses and discussion. Yale J Biol Med. (1970) 42:350–62.
6. Waggoner JJ, Balmaseda A, Gresh L, Sahoo MK, Montoya M, Wang C, et al. Homotypic dengue virus reinfections in nicaraguan children. J Infect Dis. (2016) 214:986–93. doi: 10.1093/infdis/jiw099
7. Forshey BM, Reiner RC, Olkowski S, Morrison AC, Espinoza A, Long KC, et al. Incomplete protection against dengue virus type 2 re-infection in Peru. PLoS Negl Trop Dis. (2016) 10:e0004398. doi: 10.1371/journal.pntd.0004398
8. Sharp TM, Hunsperger E, Munoz-Jordan JL, Margolis HS, Tomashek KM. Sequential episodes of dengue–Puerto Rico, 2005–2010. Am J Trop Med Hyg. (2014) 91:235–9. doi: 10.4269/ajtmh.13-0742
9. Endy TP, Nisalak A, Chunsuttitwat S, Vaughn DW, Green S, Ennis FA, et al. Relationship of preexisting dengue virus (DV) neutralizing antibody levels to viremia and severity of disease in a prospective cohort study of DV infection in Thailand. J Infect Dis. (2004) 189:990–1000. doi: 10.1086/382280
10. Clapham HE, Rodriguez-Barraquer I, Azman AS, Althouse BM, Salje H, Gibbons RV, et al. Dengue virus (DENV) neutralizing antibody kinetics in children after symptomatic primary and postprimary DENV infection. J Infect Dis. (2016) 213:1428–35. doi: 10.1093/infdis/jiv759
11. Katzelnick LC, Montoya M, Gresh L, Balmaseda A, Harris E. Neutralizing antibody titers against dengue virus correlate with protection from symptomatic infection in a longitudinal cohort. Proc Natl Acad Sci USA. (2016) 113:728–33. doi: 10.1073/pnas.1522136113
12. Bernardo L, Izquierdo A, Prado I, Rosario D, Alvarez M, Santana E, et al. Primary and secondary infections of Macaca fascicularis monkeys with Asian and American genotypes of dengue virus 2. Clin Vaccine Immunol. (2008) 15:439–46. doi: 10.1128/CVI.00208-07
13. Guzman MG, Alvarez M, Halstead SB. Secondary infection as a risk factor for dengue hemorrhagic fever/dengue shock syndrome: an historical perspective and role of antibody-dependent enhancement of infection. Arch Virol. (2013) 158:1445–59. doi: 10.1007/s00705-013-1645-3
14. Katzelnick LC, Gresh L, Halloran ME, Mercado JC, Kuan G, Gordon A, et al. Antibody-dependent enhancement of severe dengue disease in humans. Science. (2017) 358:929–32. doi: 10.1126/science.aan6836
15. Hatch S, Endy TP, Thomas S, Mathew A, Potts J, Pazoles P, et al. Intracellular cytokine production by dengue virus-specific T cells correlates with subclinical secondary infection. J Infect Dis. (2011) 203:1282–91. doi: 10.1093/infdis/jir012
16. Weiskopf D, Angelo MA, de Azeredo EL, Sidney J, Greenbaum JA, Fernando AN, et al. Comprehensive analysis of dengue virus-specific responses supports an HLA-linked protective role for CD8+ T cells. Proc Natl Acad Sci USA. (2013) 110:E2046–53. doi: 10.1073/pnas.1305227110
17. Weiskopf D, Bangs DJ, Sidney J, Kolla RV, De Silva AD, de Silva AM, et al. Dengue virus infection elicits highly polarized CX3CR1+ cytotoxic CD4+ T cells associated with protective immunity. Proc Natl Acad Sci USA. (2015) 112:E4256–63. doi: 10.1073/pnas.1505956112
18. Rivino L, Kumaran EA, Thein TL, Too CT, Gan VC, Hanson BJ, et al. Virus-specific T lymphocytes home to the skin during natural dengue infection. Sci Transl Med. (2015) 7:278ra35. doi: 10.1126/scitranslmed.aaa0526
19. Wijeratne DT, Fernando S, Gomes L, Jeewandara C, Ginneliya A, Samarasekara S, et al. Quantification of dengue virus specific T cell responses and correlation with viral load and clinical disease severity in acute dengue infection. PLoS Negl Trop Dis. (2018) 12:e0006540. doi: 10.1371/journal.pntd.0006540
20. Katzelnick LC, Harris E. Immune correlates of protection for dengue: state of the art and research agenda. Vaccine. (2017) 35:4659–69. doi: 10.1016/j.vaccine.2017.07.045
21. Gibbons RV, Kalanarooj S, Jarman RG, Nisalak A, Vaughn DW, Endy TP, et al. Analysis of repeat hospital admissions for dengue to estimate the frequency of third or fourth dengue infections resulting in admissions and dengue hemorrhagic fever, and serotype sequences. Am J Trop Med Hyg. (2007) 77:910–3. doi: 10.4269/ajtmh.2007.77.910
22. Olkowski S, Forshey BM, Morrison AC, Rocha C, Vilcarromero S, Halsey ES, et al. Reduced risk of disease during postsecondary dengue virus infections. J Infect Dis. (2013) 208:1026–33. doi: 10.1093/infdis/jit273
23. Montoya M, Gresh L, Mercado JC, Williams KL, Vargas MJ, Gutierrez G, et al. Symptomatic versus inapparent outcome in repeat dengue virus infections is influenced by the time interval between infections and study year. PLoS Negl Trop Dis. (2013) 7:e2357. doi: 10.1371/journal.pntd.0002357
24. Endy TP, Chunsuttiwat S, Nisalak A, Libraty DH, Green S, Rothman AL, et al. Epidemiology of inapparent and symptomatic acute dengue virus infection: a prospective study of primary school children in Kamphaeng Phet, Thailand. Am J Epidemiol. (2002) 156:40–51. doi: 10.1093/aje/kwf005
25. Innis BL, Nisalak A, Nimmannitya S, Kusalerdchariya S, Chongswasdi V, Suntayakorn S, et al. An enzyme-linked immunosorbent assay to characterize dengue infections where dengue and Japanese encephalitis co-circulate. Am J Trop Med Hyg. (1989) 40:418–27. doi: 10.4269/ajtmh.1989.40.418
26. Mangada MM, Rothman AL. Altered cytokine responses of dengue-specific CD4+ T cells to heterologous serotypes. J Immunol. (2005) 175:2676–83. doi: 10.4049/jimmunol.175.4.2676
27. Duangchinda T, Dejnirattisai W, Vasanawathana S, Limpitikul W, Tangthawornchaikul N, Malasit P, et al. Immunodominant T-cell responses to dengue virus NS3 are associated with DHF. Proc Natl Acad Sci USA. (2010) 107:16922–7. doi: 10.1073/pnas.1010867107
28. Dung NT, Duyen HT, Thuy NT, Ngoc TV, Chau NV, Hien TT, et al. Timing of CD8+ T cell responses in relation to commencement of capillary leakage in children with dengue. J Immunol. (2010) 184:7281–7. doi: 10.4049/jimmunol.0903262
29. Malavige GN, Huang LC, Salimi M, Gomes L, Jayaratne SD, Ogg GS. Cellular and cytokine correlates of severe dengue infection. PLoS ONE. (2012) 7:e50387. doi: 10.1371/journal.pone.0050387
30. Jeewandara C, Adikari TN, Gomes L, Fernando S, Fernando RH, Perera MK, et al. Functionality of dengue virus specific memory T cell responses in individuals who were hospitalized or who had mild or subclinical dengue infection. PLoS Negl Trop Dis. (2015) 9:e0003673. doi: 10.1371/journal.pntd.0003673
31. Velumani S, Toh YX, Balasingam S, Archuleta S, Leo YS, Gan VC, et al. Low antibody titers 5 years after vaccination with the CYD-TDV dengue vaccine in both pre-immune and naive vaccinees. Hum Vaccin Immunother. (2016) 12:1265–73. doi: 10.1080/21645515.2015.1126012
32. Zompi S, Montoya M, Pohl MO, Balmaseda A, Harris E. Dominant cross-reactive B cell response during secondary acute dengue virus infection in humans. PLoS Negl Trop Dis. (2012) 6:e1568. doi: 10.1371/journal.pntd.0001568
33. Mathew A, West K, Kalayanarooj S, Gibbons RV, Srikiatkhachorn A, Green S, et al. B-cell responses during primary and secondary dengue virus infections in humans. J Infect Dis. (2011) 204:1514–22. doi: 10.1093/infdis/jir607
34. Salje H, Cummings DAT, Rodriguez-Barraquer I, Katzelnick LC, Lessler J, Klungthong C, et al. Reconstruction of antibody dynamics and infection histories to evaluate dengue risk. Nature. (2018) 557:719–23. doi: 10.1038/s41586-018-0157-4
35. Endy TP, Anderson KB, Nisalak A, Yoon IK, Green S, Rothman AL, et al. Determinants of inapparent and symptomatic dengue infection in a prospective study of primary school children in Kamphaeng Phet, Thailand. PLoS Negl Trop Dis. (2011) 5:e975. doi: 10.1371/journal.pntd.0000975
36. Moodie Z, Juraska M, Huang Y, Zhuang Y, Fong Y, Carpp LN, et al. Neutralizing antibody correlates analysis of tetravalent dengue vaccine efficacy trials in Asia and Latin America. J Infect Dis. (2018) 217:742–53. doi: 10.1093/infdis/jix609
37. Buddhari D, Aldstadt J, Endy TP, Srikiatkhachorn A, Thaisomboonsuk B, Klungthong C, et al. Dengue virus neutralizing antibody levels associated with protection from infection in thai cluster studies. PLoS Negl Trop Dis. (2014) 8:e3230. doi: 10.1371/journal.pntd.0003230
38. Priyamvada L, Cho A, Onlamoon N, Zheng NY, Huang M, Kovalenkov Y, et al. B Cell Responses during secondary dengue virus infection are dominated by highly cross-reactive, memory-derived plasmablasts. J Virol. (2016) 90:5574–85. doi: 10.1128/JVI.03203-15
39. Crotty S, Felgner P, Davies H, Glidewell J, Villarreal L, Ahmed R. Cutting edge: long-term B cell memory in humans after smallpox vaccination. J Immunol. (2003) 171:4969–73. doi: 10.4049/jimmunol.171.10.4969
40. Smith SA, Zhou Y, Olivarez NP, Broadwater AH, de Silva AM, Crowe JEJr. Persistence of circulating memory B cell clones with potential for dengue virus disease enhancement for decades following infection. J Virol. (2012) 86:2665–75. doi: 10.1128/JVI.06335-11
41. Crotty S, Aubert RD, Glidewell J, Ahmed R. Tracking human antigen-specific memory B cells: a sensitive and generalized ELISPOT system. J Immunol Methods. (2004) 286:111–22. doi: 10.1016/j.jim.2003.12.015
42. Hammarlund E, Lewis MW, Hansen SG, Strelow LI, Nelson JA, Sexton GJ, et al. Duration of antiviral immunity after smallpox vaccination. Nat Med. (2003) 9:1131–7. doi: 10.1038/nm917
43. Pinna D, Corti D, Jarrossay D, Sallusto F, Lanzavecchia A. Clonal dissection of the human memory B-cell repertoire following infection and vaccination. Eur J Immunol. (2009) 39:1260–70. doi: 10.1002/eji.200839129
44. Amanna IJ, Carlson NE, Slifka MK. Duration of humoral immunity to common viral and vaccine antigens. N Engl J Med. (2007) 357:1903–15. doi: 10.1056/NEJMoa066092
45. Bashyam HS, Green S, Rothman AL. Dengue virus-reactive CD8+ T cells display quantitative and qualitative differences in their response to variant epitopes of heterologous viral serotypes. J Immunol. (2006) 176:2817–24. doi: 10.4049/jimmunol.176.5.2817
46. Chu H, George SL, Stinchcomb DT, Osorio JE, Partidos CD. CD8+ T-cell responses in flavivirus-naive individuals following immunization with a live-attenuated tetravalent dengue vaccine candidate. J Infect Dis. (2015) 212:1618–28. doi: 10.1093/infdis/jiv258
47. Simmons CP, Dong T, Chau NV, Dung NT, Chau TN, Thao le TT, et al. Early T-cell responses to dengue virus epitopes in Vietnamese adults with secondary dengue virus infections. J Virol. (2005) 79:5665–75. doi: 10.1128/JVI.79.9.5665-5675.2005
48. Grifoni A, Angelo MA, Lopez B, O'Rourke PH, Sidney J, Cerpas C, et al. Global assessment of dengue virus-specific CD4(+) T cell responses in dengue-endemic areas. Front Immunol. (2017) 8:1309. doi: 10.3389/fimmu.2017.01309
49. Mangada MM, Endy TP, Nisalak A, Chunsuttiwat S, Vaughn DW, Libraty DH, et al. Dengue-specific T cell responses in peripheral blood mononuclear cells obtained prior to secondary dengue virus infections in Thai schoolchildren. J Infect Dis. (2002) 185:1697–703. doi: 10.1086/340822
50. Appanna R, Huat TL, See LL, Tan PL, Vadivelu J, Devi S. Cross-reactive T-cell responses to the nonstructural regions of dengue viruses among dengue fever and dengue hemorrhagic fever patients in Malaysia. Clin Vaccine Immunol. (2007) 14:969–77. doi: 10.1128/CVI.00069-07
51. Anderson KB, Gibbons RV, Thomas SJ, Rothman AL, Nisalak A, Berkelman RL, et al. Preexisting Japanese encephalitis virus neutralizing antibodies and increased symptomatic dengue illness in a school-based cohort in Thailand. PLoS Negl Trop Dis. (2011) 5:e1311. doi: 10.1371/journal.pntd.0001311
52. Mathew A, Townsley E, Ennis FA. Elucidating the role of T cells in protection against and pathogenesis of dengue virus infections. Future Microbiol. (2014) 9:411–25. doi: 10.2217/fmb.13.171
53. Friberg H, Beaumier CM, Park S, Pazoles P, Endy TP, Mathew A, et al. Protective versus pathologic pre-exposure cytokine profiles in dengue virus infection. PLoS Negl Trop Dis. (2018) 12:e0006975. doi: 10.1371/journal.pntd.0006975
54. Haltaufderhyde K, Srikiatkhachorn A, Green S, Macareo L, Park S, Kalayanarooj S, et al. Activation of peripheral T follicular helper cells during acute dengue virus infection. J Infect Dis. (2018) 218:1675–85. doi: 10.1093/infdis/jiy360
55. Calarota SA, Baldanti F. Enumeration and characterization of human memory T cells by enzyme-linked immunospot assays. Clin Dev Immunol. (2013) 2013:637649. doi: 10.1155/2013/637649
56. Jeewandara C, Ogg GS, Malavige GN. Cultured ELISpot assay to investigate dengue virus specific T-cell responses. Methods Mol Biol. (2018) 1808:165–71. doi: 10.1007/978-1-4939-8567-8_14
57. Malyguine AM, Strobl S, Dunham K, Shurin MR, Sayers TJ. ELISPOT assay for monitoring cytotoxic T lymphocytes (CTL) activity in cancer vaccine clinical trials. Cells. (2012) 1:111–26. doi: 10.3390/cells1020111
58. Jayathilaka D, Gomes L, Jeewandara C, Jayarathna GSB, Herath D, Perera PA, et al. Role of NS1 antibodies in the pathogenesis of acute secondary dengue infection. Nat Commun. (2018) 9:5242. doi: 10.1038/s41467-018-07667-z
59. Rivino L, Kumaran EA, Jovanovic V, Nadua K, Teo EW, Pang SW, et al. Differential targeting of viral components by CD4+ versus CD8+ T lymphocytes in dengue virus infection. J Virol. (2013) 87:2693–706. doi: 10.1128/JVI.02675-12
60. Weiskopf D, Cerpas C, Angelo MA, Bangs DJ, Sidney J, Paul S, et al. Human CD8+ T-cell responses against the 4 dengue virus serotypes are associated with distinct patterns of protein targets. J Infect Dis. (2015) 212:1743–51. doi: 10.1093/infdis/jiv289
61. Carlin AF, Wen J, Vizcarra EA, McCauley M, Chaillon A, Akrami K, et al. A longitudinal systems immunologic investigation of acute Zika virus infection in an individual infected while traveling to Caracas, Venezuela. PLoS Negl Trop Dis. (2018) 12:e0007053. doi: 10.1371/journal.pntd.0007053
62. Kiecker F, Streitz M, Ay B, Cherepnev G, Volk HD, Volkmer-Engert R, et al. Analysis of antigen-specific T-cell responses with synthetic peptides–what kind of peptide for which purpose? Hum Immunol. (2004) 65:523–36. doi: 10.1016/j.humimm.2004.02.017
63. Suneetha PV, Schlaphoff V, Wang C, Stegmann KA, Fytili P, Sarin SK, et al. Effect of peptide pools on effector functions of antigen-specific CD8+ T cells. J Immunol Methods. (2009) 342:33–48. doi: 10.1016/j.jim.2008.11.020
64. Zhang W, Moldovan I, Targoni OS, Subbramanian RA, Lehmann PV. How much of virus-specific CD8 T cell reactivity is detected with a peptide pool when compared to individual peptides? Viruses. (2012) 4:2636–49. doi: 10.3390/v4112636
65. Koutsakos M, Wheatley AK, Loh L, Clemens EB, Sant S, Nussing S, et al. Circulating TFH cells, serological memory, and tissue compartmentalization shape human influenza-specific B cell immunity. Sci Transl Med. (2018) 10:eaan8405. doi: 10.1126/scitranslmed.aan8405
Keywords: dengue, T cells, cytokines, IFN-γ, IL-2, B cells, antibodies, ELISPOT
Citation: Sánchez-Vargas LA, Kounlavouth S, Smith ML, Anderson KB, Srikiatkhachorn A, Ellison DW, Currier JR, Endy TP, Mathew A and Rothman AL (2019) Longitudinal Analysis of Memory B and T Cell Responses to Dengue Virus in a 5-Year Prospective Cohort Study in Thailand. Front. Immunol. 10:1359. doi: 10.3389/fimmu.2019.01359
Received: 02 February 2019; Accepted: 29 May 2019;
Published: 13 June 2019.
Edited by:
Gathsaurie Neelika Malavige, University of Sri Jayewardenepura, Sri LankaReviewed by:
Kristina De Paris (Abel), University of North Carolina at Chapel Hill, United StatesScott B. Halstead, Uniformed Services University of the Health Sciences, United States
Copyright © 2019 Sánchez-Vargas, Kounlavouth, Smith, Anderson, Srikiatkhachorn, Ellison, Currier, Endy, Mathew and Rothman. This is an open-access article distributed under the terms of the Creative Commons Attribution License (CC BY). The use, distribution or reproduction in other forums is permitted, provided the original author(s) and the copyright owner(s) are credited and that the original publication in this journal is cited, in accordance with accepted academic practice. No use, distribution or reproduction is permitted which does not comply with these terms.
*Correspondence: Alan L. Rothman, YWxhbl9yb3RobWFuQHVyaS5lZHU=