- 1Department of Comparative Biomedicine and Food Science (BCA), University of Padova, Padova, Italy
- 2Department of Animal Medicine, Productions and Health, University of Padova, Padova, Italy
The term “acute phase response” (APR) is referred to a nonspecific and complex reaction of an organism that occurs shortly after any tissue damage, such as infection, trauma, neoplasia, inflammation, and stress. The APR can be identified and monitored with some laboratory tests, such as the concentration of several plasma proteins, the acute phase proteins (APPs). The APPs are components of the non-specific innate immune response, and their plasma concentration is proportional to the severity and/or the extent of tissue damage. The evaluation of health status of marine mammals is difficult because the classical clinical signs of illness used for human and domestic animals are difficult to recognize and understand. For this reason, in the past years, several efforts were done to identify laboratory markers of disease in these animals. The APPs have demonstrated their role as early markers of inflammation in veterinary medicine, thus several APPs were tested in marine mammals, such as C-reactive protein (CRP), serum amyloid-A (SAA), and Haptoglobin (Hp). However, the difficulty to extrapolate the knowledge about APPs in one species to another, the lack of specie-specific reagents, the absence of data about negative APPs have hampered their extent use in marine mammals. Herein, the state of art of APPs in marine mammals is reviewed, with particular attention to pre-analytical and analytical factors that should be taken into account in validation and interpretation of APPs assays. Moreover, the current application, potential utility and the future developments of APPs in marine mammals is highlighted and discussed.
Introduction
The mammalian immune system includes innate or nonspecific immunity as well as adaptive or specific immunity. The responses of these two different pathways are distinct, but highly interconnected. The first reaction of an organism to different pathological conditions is an innate, non-specific response (1), a more conserved response during evolution which aim is the immediate reaction against pathological stimuli (2). After the initial recognition of pathogens or tissue damages by the tissue-resident macrophages, which express the pattern recognition receptors (PRRs), a variety of different inflammatory mediators are produced by leukocytes, endothelial cells, tissue cells or are derived from plasma proteins. These mediators include different chemokine, cytokine, vasoactive amines and products of the arachidonic acid: their primary effect is to elicit inflammatory response locally and to recruit leukocytes and plasma proteins in the site of injury (3).
At the same time, many of these inflammatory mediators influence the function of many tissues and organs throughout all the organisms, inducing the acute phase response.
The activated macrophages on the site of injury are also responsible of the activation of the adaptive immune response, a more slowly, but more focused response. The expression of major histocompatibility complex (MHC) class II antigens, combined with foreign antigen, by tissue macrophages leads to the antigen-dependent activation of T-cells and start the precise immune response against that specific target. T-cells can differentiate in different kinds of T-helper (Th) lymphocytes with the expression of different cytokine pattern. Th1 cells coordinate the interaction between innate and cell-mediated adaptive response by means of different cytokines, among them IFN-γ and IL-2. Differently, Th2 cells, which produce IL-4 and IL-5, promote humoral immune response, the differentiation of B-cell into plasma cells, but have also an anti-inflammatory activity by producing IL-10 (4).
The term “acute phase response” (APR) refers to a nonspecific and complex reaction that occurs shortly after tissue damage due to several conditions such as infection, trauma, neoplasia, inflammation, and stress (5). The reaction of APR includes systemic consequences, such as fever, leucocytosis, increased release of several hormones and drastic rearrangement of plasma protein synthesis (6). These changes include a decrease of plasma low and high density lipoproteins-bound cholesterol, an increase of ACTH and glucocorticoids, the activation of complement and coagulation system, a decrease of calcium, zinc, iron, vitamin A, and α-tocopherol serum levels. All these changes in blood plasma composition induced by APR are beneficial to the organism because they prevent microbial growth and help restore of homeostasis (1). Moreover, a considerable change in concentration of several plasma proteins, the acute phase proteins (APPs), is evident few hours after an inflammatory stimulus (7).
APPs are a group of blood proteins that change in concentration in animals subjected to external or internal challenges, such as infection, inflammation, trauma or stress, proportionally to the severity of the disorder and/or the extent of tissue damage (8). They are synthesized primarily by hepatocytes stimulated by cytokines or endogenous glucocorticoids (9, 10). APPs are classified based on the direction of change: synthesis of positive APPs is upregulated with a resulting increase in blood concentration, and synthesis of negative APPs is downregulated with a resulting decrease in blood concentration (11). Positive APPs are further classified as major, moderate or minor based on the magnitude of increase: major APPs showed an increase of 10- to 100-fold, moderate APPs showed an increase of 2- to 10-fold, and minor APPs have only a slight increase (5). Also the rate of the concentration increase varies between major, moderate, and minor positive APPs: major APPs usually have a marked increase within 48 h after a pathogen stimulus and a subsequent rapid decline after the cessation of stimulus due to their short half-life (12). Moderate and minor APPs usually have a slower increase, but also a slower decline to normal value and so they usually increase during chronic inflammatory process (5). However, the APPs response varies between species: the major APP in dogs and men is C-reactive protein (CRP) (13), in cats is α1–acid glycoprotein (AGP) (9), in ruminants is Haptoglobin (Hp) (14); in horse is Serum Amyloid A (SAA) (15) and in pigs are Hp, SAA, and major acute phase protein (MAP) (10).
Recently, in veterinary medicine, studies on the role of APPs as markers of infectious, inflammatory and neoplastic diseases have proliferated (16) and at least 40 different plasma proteins have been identified as APPs (8). Their use as marker of homeostasis perturbation provides some advantages compared with traditional parameters like the white blood cell (WBC) counts. Compared to WBC count, the diagnostic sensitivity of APPs is higher and the change in concentration is faster (17). Moreover, their stability in serum/plasma is high, so it is possible to measure APPs in frozen samples (18). One limitation of the APPs is the poor diagnostic specificity, for this reason they cannot be used as primary diagnostic test for a specific disease, but they were successfully used to detect subclinical diseases and to monitor clinical evolution and to assess the response to treatment (5). Additionally, the combined measurement of several APPs provides more information than the evaluation of a single protein: in the “APP value index,” proposed by Gruys et al. (1), sensitivity and specificity are improved by combining response of both positive and negative major and minor APPs.
Marine mammals are a group of around 130 mammalian species which depend on water environment for most of their needs. They include 3 orders: Carnivora (pinnipeds, otters, and polar bear), Cetacea (dolphins, whale, and porpoise) and Sirenia (manatee and dugogons). Marine mammals are differently adapted to life in water, with some species, which are fully aquatic (cetaceans and sirenia), and others that spend part of their life on land (pinniped and polar bears) (19). From an immunological point of view, aquatic adaptation caused few differences in distribution and function of immune system between marine and terrestrial mammals (20). However, nowadays the marine mammal' immune system is deeply exposed to environmental pollution because they are a long-lived animals placed at the top of food chain, thus they are exposed to a progressive bioaccumulation of fat-soluble pollutants, such as PCBs, which affect both innate and adaptive immune function (21). For these reasons, increasing knowledge in cellular and humoral immune response is continuously required to understand their immune system and in particular its relationship with infectious pathologies and the environmental pollution (22). Furthermore, marine mammals live also in controlled environment like aquaria, rehabilitation facilities and research center where health assessment is fundamental to evaluate the correct management of animals and to monitor the response to therapy during rehabilitation. From this perspective, the availability of new markers to asses immune functions is fundamental both for medical care and research purpose (20).
Acute Phase Reaction and Acute Phase Protein in Marine Mammals
Innate immune response represent the first line of response against pathological stimuli, it's very fast and it's based primarily on effector cells (e.g., mast cells, macrophages, neutrophils) and antimicrobial substances (e.g., complement, reactive oxygen, and nitrogen species). On the other hand, adaptive immune system is antigen-specific, it takes more days to be effective and it's based on different T-cells response and on B-lymphocytes which are responsible of humoral response mediated by the different subclass of immunoglobulin (IgG, IgM, IgA) (20). Several assays were proposed to evaluate both immune response in marine mammals, generally based on isolated leucocytes with the aim to evaluate the leukocytes response against in vitro stimuli (23).
To assess the response pattern of cetaceans' cellular innate immune system, the phagocytosis and the generation of reactive oxygen species of polymorphonuclear leukocytes were investigated. In particular, in vitro ingestion of latex beads and hydrogen peroxide production have been evaluated in beluga whales (Delphinapterus leucas) and in bottlenose dolphins (Tursiops truncatus) (24, 25) whereas phagocytosis and respiratory burst assay, using whole blood from bottlenose dolphins, were used to assess the antimicrobial activity (26). In addition, the investigation of APR by analyzing the cytokine expression gives important information on the functionality of lymphoid cells. The production of specie-specific antibodies allows the development of immunological assays for the quantification of cytokine expression useful to investigate the inflammatory response in whales and dolphins. The coding regions of IL-2, IL-1β, IL-6, and TNF-α gene of the beluga whale have been sequenced, and a cytokine-specific rabbit antisera have been produced (27–29). In harbor porpoise (Phocoena phocoena), the quantification of mRNA of IL-1β, IL-2, IL-4, IL-6, IL-10, and TNF-α have been performed by RT-PCR (30), and the increase of IL-10 was seen in harbor porpoises suffering from long lasting infectious (31). Also in bottlenose dolphins, pacific white-sided dolphins (Lagenorhynchus obliquidens), and beluga whales, IL-2, IL-4, IL-10, IL-12, IL-13, IL-18, TNF-α, TGF-β, and interferon (IFN)-γ quantification was performed using RT-PCR (32). An IL-2 receptor expression assay and an IL-6 ELISA were developed in bottlenose dolphins and killer whale (Orcinus Orca), respectively (33, 34).
Similarly, both innate and the cell-mediated response were studied in pinnipeds. To better understand the innate response, phagocytic activity of isolated peripheral blood leukocytes was evaluated in harbor seal (Phoca vitulina), gray seal (Halichoerus grypus), and harp seal (Phoca groenlandica) pups, in harbor seal female during lactation and in harbor seal pups admitted to rescue center (35, 36). The authors found an age-related variation in both pups and adults: phagocytosis increased with age in gray and harbor seal pups, while in female harbor seals decreased from sub-adult to adulthood. At the same time, pups after rehabilitation showed a decreased phagocytic activity, probably due to the decreased stimulation of innate response after therapy. Also cytokine response was evaluate in harbor seal. Pro-inflammatory cytokine mRNA (IL-1β, IL-6, IL-8, and IL-12) in pups in a rehabilitation center were higher at admission whilst IL-4 was higher before the release (37), demonstrating the recovery from inflammation. Recently, a multiplex canine cytokine assay was validate in harbor, gray and harp seal to measure proteins levels in cell culture supernatant of peripheral blood mononuclear cells (PBMC) (38).
However, all these techniques are not generally applicable in a clinical setting in which the primary goal is a sensitive diagnostic tool with a rapid turnaround, even if give us important information on factors affecting cetaceans' immune system. For this reason, in the past years, several efforts were made to identify laboratory markers of disease in these animals. First parameters tested were WBC and erythrocyte sedimentation rate (39). However, even if they are inexpensive and rapid, they lack specificity and sensitivity. Moreover, changes in WBC occur after several hours after inflammatory stimuli. Thus, efforts were directed to identify inflammation at earlier stage (40). To examine the humoral response, species-specific antibodies against IgG were produced and used to evaluate serum IgG levels in killer whale by radial immunodiffusion assay (41) and by competitive ELISA in bottlenose dolphins (42, 43). The determination of IgG baseline values in free-ranging and in managed dolphins revealed higher levels of immunoglobulin in the first group with several values over the accurate range of the assay, probably due to the higher parasitic load in free-ranging dolphins (43).
Serum total protein, albumin, globulin and albumin:globulin ratio (A:G) are undoubtedly among the most measured markers in basic health assessment in domestic animals as well as in marine mammals. Serum protein electrophoresis is also broadly applied in veterinary medicine and it has the advantage to produce an accurate measurement of albumin and the visualization of globulin fractions (44). The interpretation of total proteins values and electrophoretic pattern of serum proteins is receiving increased attention also in marine mammals in which a typical pathologic pattern could be identified in inflammatory diseases (40). Reference intervals for these markers are available for free-ranging bottlenose dolphins (45) and, compared to these, recently data on managed dolphins showed slightly lower values of TP, α-globulins, and γ-globulins and higher albumin and albumin/globulins ratio (46).
It's interesting to note that Hp, α1-antitrypsin, α1-antichymotripsin, and α2-macroglobulin migrate in the α-globulins fraction, while the IgG and CRP migrate in the γ-globulins fraction. Albumin acts as a negative acute phase protein since the synthesis of this protein is decreased during an inflammation (47). Thus, the lower concentration of positive APPs associated to a higher concentration of albumin and the consequent higher albumin/globulins ratio could reflect lower antigenic stimuli in managed population compared to the free-ranging populations (36). Serum total protein analysis were used to assess health status in several cetaceans species such as pantropical spotted dolphins (Stenella attenuata) (48), beluga (49), minke whales (Balaenoptera acutorostrata) (50) and killer whales (51) as well as in other marine mammals, like harbor seals (Phoca vitulina) (52) and walruses (Odobenus rosmarus) (53). In all these species, serum total protein analysis was demonstrated to be one of the most used and commonly accepted marker of inflammation.
However, specific APPs have demonstrated their superior role as early markers of inflammation, so based on the results obtained in humans and companion animals, several positive APPs were tested in marine mammals (Table 1). Published works had the primary aims to evaluate the feasibility of the assays to measure the APPs, to validate the antibody-based assay and to determine the RIs. In bottlenose dolphins three APPs (CRP, SAA, and Hp) were tested, even if not always complete validation studies were performed (54, 61). For these APPs, the authors established the RIs in free-ranging and managed dolphins using automated assays (54) and they found significantly lower SAA and higher Hp levels in free ranging animals. The only clinical significance of these alteration was a higher ability to detect chronic inflammation for Hp. Regarding Hp, Segawa and colleagues validated commercially available Hp-ELISA and Hp-hemoglobin binding assay in bottlenose dolphins with ‘’acceptable” intra- and inter-assay imprecision (CV: 3.3% healthy dolphins and 3.5% inflamed dolphins; CV: 10.4% healthy dolphins and 21.7% inflamed dolphins) and demonstrated that Hp levels in the serum increase under inflammatory conditions (62).
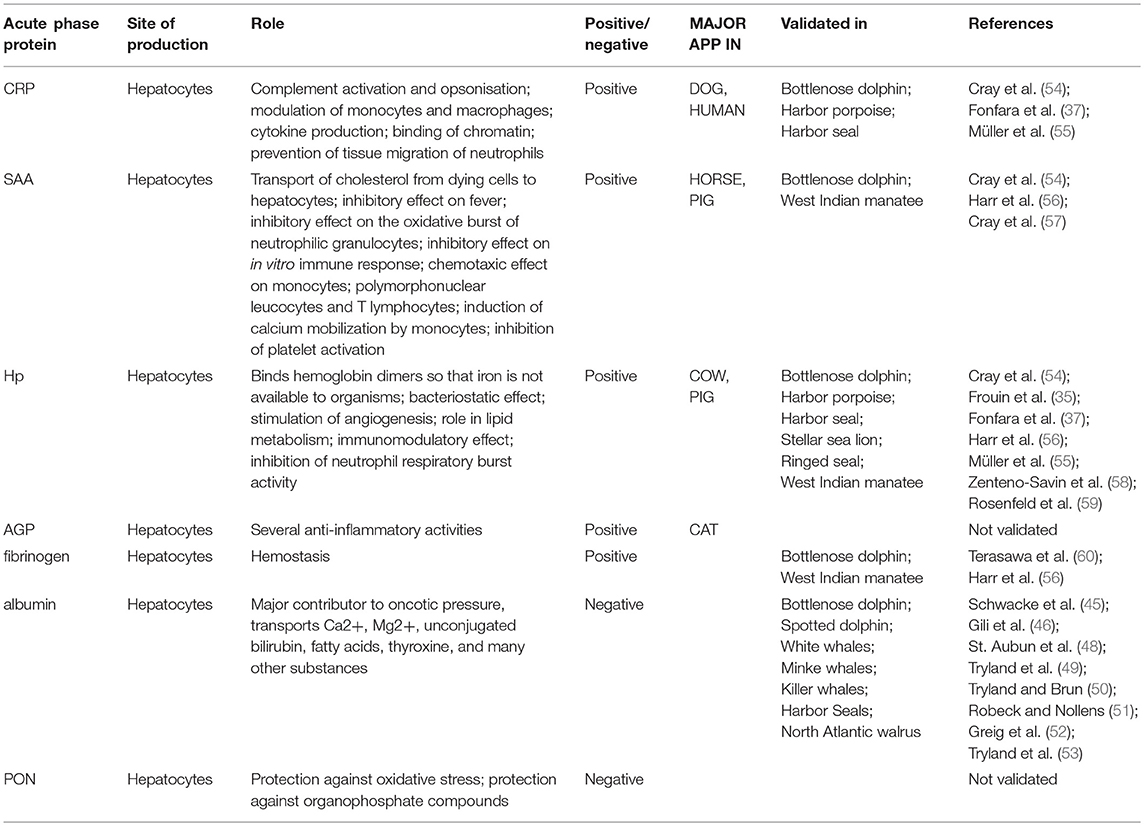
Table 1. Acute phase proteins: site of production, role and marine mammal species in which they are validated.
Positive APPs were tested also in Florida manatees (Trichechus manatus latirostris) to define the more accurate marker of inflammation. Five different APPs were tested: AGP, CRP, Hp, fibrinogen, and SAA. SAA showed the highest diagnostic sensitivity and specificity (90% for both sensitivity and specificity) in the detection of inflammatory diseases, the diagnostic specificity of Hp and fibrinogen were 93 and 95%, respectively, while their diagnostic sensitivity were 60 and 40%, respectively, (56). When used in stranded manatee suffering from cold stress and trauma, SAA showed 93% of sensitivity and 98% of specificity in detecting diseased animals (57). By contrast, the Abs used for the determination of AGP and CRP did not cross-react in this species (56).
In harbor seal, an Ab anti-CRP and a competitive immunoassay was produced (63), but Hp is probably the APP most used in pinnipeds. A multispecies assay based on hemoglobin binding capacity was used to demonstrate as Hp is a sensitive marker of the health vs. disease status in harbor seal (64). In seal pups admitted in a rescue center. Hp, total protein, IgG and globulin values correlated positively, but Hp levels increased during the hospitalization, probably reflecting age-related changes (35). Hp is considered a health marker also in Steller sea lions (Eumetopias jubatus): significantly higher levels of Hp were found in declining population compared to more stable ones (58). However, also genetic differences between distant and isolated population of wild animals could be the causes of this difference, not only a pathological condition.
If some data on marine mammals positive APPs are available in literature, quite surprising are the lack of data available on negative APPs. For these reasons, the possibility to evaluate the usefulness of an “APP value index” is still far from being applied.
Acute Phase Protein in Marine Mammals: Challenges and Future Developments
The availability of sensitive markers of inflammation both for free-ranging and managed marine mammals is nowadays considered fundamental to evaluate the health status and, in rehabilitation setting, to monitor the response to therapy and to define the prognosis. As serum markers, the APPs have several advantages: they have longer stability compared to other blood component such as WBC; they can be performed on frozen serum, thus the samples can be shipped to references laboratories; some assays can be automated to obtain results in an excellent turnaround time.
However, is important to consider that the knowledge about APPs in one species cannot be readily generalized to another species, in which healthy levels, response to inflammation or infection, and prognostic significance may be different (65). Moreover, the evolution of marine mammals and their adaptation throughout the millennia to an aquatic environment had led to a different physiology and metabolism compared to terrestrial mammals. Thus, the understanding of the genetic, phenotypical and biochemical properties of marine mammals APPs are essential prior to using them as a new biomarker.
An example of how the biochemical properties influence the analytic method is paroxonase-1 (PON1), a HDL-bound esterase which protects against organophosphate compounds, acts as negative APP and as oxidative stress marker. PON1 is usually assessed by enzymatic method and, based on the different PON1 functions, several substrates have been identified to evaluate serum PON1 activities. Nevertheless, both in humans as in some terrestrial mammals, PON1 gene polymorphisms highly influence the enzymatic activity toward different substrate: the single-nucleotide polymorphisms (SNPs) Leu55Met and Gln192Arg increase the paraoxonase activity (66) in humans and different PON1 genotypes influence activities toward paraoxon and phenyl-acetate in rabbit (67). Also in cows, some SNPs in the promotor region of PON1 gene are associated with serum PON1 activity (68). Recently, a phylogenetic study on convergent functional losses across marine mammals, has identified a PON1 functional loss in marine mammals, probably related to their different lipid metabolism and fatty acid oxidation due to adaptation to the marine environment and a high concentration of ω-3 fatty acids on their diet. As a consequence, in several marine mammals species paroxonase activity is very low, while enzymatic activity against other PON1 substrates is still present, such as arylesterase activity (69). For these reasons, the use of classical enzymatic assays is hampered in these animals and further studies are needed to elucidate the role of PON1 as possible negative APP, oxidative stress marker and the consequences of its inability to detoxify organophosphates compounds.
From an analytical point of view, another challenge in the evaluation of APPs in marine mammals is the need of species-specific assays, especially for the immunological assay, such as ELISA or immunoturbidimetry. This means the development of a de-novo method, often a time-consuming and expensive approach, or the validation of a commercial available assay used in other species (65). The latter approach is surely the most used in veterinary medicine, in which some human assays were validated for dogs, cats and horses (62). However, even if some APPs appeared highly conserved among species, an accurate validation of antibody cross reactivity is needed as well as species specific standards and control material (54). Among positive APPs, SAA is the most used across different species: it appeared as the most conserved APP in mammals even if some difference in circulating isoforms were reported (61) and it's considered a major APP in all the mammals in which it was investigated (65). Some commercial SAA assays showed good results also in marine mammals, such as bottlenose dolphin, manatee and striped dolphin (Stenella ceoreloualba) (54, 56, 70) and its use as diagnostic and prognostic marker appears nowadays the most promising.
To obtain accurate data, all the pre-analytical factors that could influence the results should be taken into consideration. The effect of storage, temperature and different anticoagulant had to be evaluated in a correct validation process as well as the interference of hemolysis and lipemia, as done in other species (5).
The application of a novel biomarker required a full evaluation of all the analytical performances and the clinical value. This process is usually divided in 4 steps: the assessment of analytical features (precision, accuracy, detection limits), the overlap performance (the ability to detect difference between healthy and diseased animals), the assessment of diagnostic capacity (sensitivity, specificity, accuracy, positive, and negative predictive values) and, at the end, the evaluation of the outcome of the new methods (which is the advantage of the test and its influence in the patient management) (65). In veterinary medicine, the validation studies do not always follow all these steps, mainly due to the lack of resources or technical limitation (44, 65). Also in marine mammals, the majority of studies had performed only some steps (44, 54, 56, 61, 62). This is mainly due to the limitation in species-specific reagents, the number of samples from animal with known health status and, last but not least, the capability to generate appropriate reference intervals, hampered the possibility to perform complete validation studies.
Population-based reference intervals derived from an appropriate group of reference individuals are usually required for diagnostic purpose (71). However, a number of biological factors have to be taken in consideration to select the appropriate reference population. Surely, age, sex and pregnancy could be used for partitioning (45, 72), but in marine mammals greater attention should be given to the difference between wild and managed animals. Serum protein electrophoresis values obtained in managed bottlenose dolphins showed lower total proteins and higher albumin levels compared to reference intervals derived from free-ranging (46) while 9 wild-caught manatees, apparently clinically healthy, had SAA level above reference limit (56). These data could indicate a trend to an inflammatory status or the presence of subclinical inflammation in free-ranging animals which are more exposed to immunological stimuli. In any case, this highlights the need to define appropriate reference intervals for animals living in different environment to have an accurate toll for the evaluation of clinical condition.
Compared to human and companion animals, the use of APPs in marine mammals is just getting started. The increasing need of knowledge on immune system and its response against infectious diseases or chemical pollutants and the request of more sensitive inflammation markers have increased the effort of researchers to study the APR and APPs. Even if APPs are considered a sensitive, but non-specific marker of inflammation, some studies revealed that, in some infectious diseases, APPs showed a specific behavior and biochemical features. One example is the modification of the glycan moiety of AGP in feline infectious peritonitis, FIV and FeLV, influencing the host-pathogens interaction and the immune response (73–75). Currently, some of the greatest threats for wild marine mammals is pathogens, like Morbillivirus, Herpesvirus, Brucella ceti, and Toxoplasma gondii (76): the evaluation of APR and APPs patterns during these infectious diseases could lead to the identification of a distinctive response of the immune system and increase the understanding of host-pathogen interaction.
Secondly, for managed or rescued animals, the forthcoming needs are the increase of automated assays, the standardization of procedures across laboratories and the discovery of new markers, for example negative APPs, to generate an APP index also in marine mammals. These new tools will certainly increase the diagnostic and prognostic skills for health assessment and, especially for stranded animals, the development of new “health status” markers will provide valuable resources in evaluating the response to treatment and rehabilitation prior to the release into the wild.
Author Contributions
MG and FB analyzed the literature review, designed, and wrote the review.
Conflict of Interest Statement
The authors declare that the research was conducted in the absence of any commercial or financial relationships that could be construed as a potential conflict of interest.
Acknowledgments
The literature review presented in the manuscript is partially included in Ph.D. thesis of Bonsembiante (77), supported by a Ph.D. Grant from the University of Padova.
References
1. Gruys E, Toussaint MJM, Niewold TA, Koopmans SJ, Van Dijk E, Meloen RH. Monitoring health by values of acute phase proteins. Acta Histochem. (2006) 108:229–32. doi: 10.1016/j.acthis.2006.03.009
2. Van Dyke TE, Kornman KS. Inflammation and factors that may regulate inflammatory response. J Periodontol. (2008) 79:1503–07. doi: 10.1902/jop.2008.080239
3. Medzhitov R. Origin and physiological roles of inflammation. Nature. (2008) 454:428–35. doi: 10.1038/nature07201
4. Libby P. Inflammatory mechanisms: the molecular basis of inflammation and disease. Nutr Rev. (2007) 65:140–6. doi: 10.1111/j.1753-4887.2007.tb00352.x
5. Cerón JJ, Eckersall PD, Martínez-Subiela S. Acute phase proteins in dogs and cats: current knowledge and future perspectives. Vet Clin Pathol. (2005) 34:85–99. doi: 10.1111/j.1939-165X.2005.tb00019.x
6. Koj A. Initiation of acute phase response and synthesis of cytokines. Biochim Biophys Acta Mol Basis Dis. (1996) 1317:84–94. doi: 10.1016/S0925-4439(96)00048-8
7. Eckersall PD, Bell R. Acute phase proteins: biomarkers of infection and inflammation in veterinary medicine. Vet J. (2010) 185:23–7. doi: 10.1016/j.tvjl.2010.04.009
8. Murata H, Shimada N, Yoshioka M. Current research on acute phase proteins in veterinary diagnosis: an overview. Vet J. (2004) 168:28–40 doi: 10.1016/S1090-0233(03)00119-9
9. Paltrinieri S. The feline acute phase reaction. Vet J. (2008) 177:26–35. doi: 10.1016/j.tvjl.2007.06.005
10. Petersen HH, Nielsen JP, Heegaard PMH. Application of acute phase protein measurements in veterinary clinical chemistry. Vet Res. (2004) 35:163–87. doi: 10.1051/vetres:2004002
11. Schrödl W, Büchler R, Wendler S, Reinhold P, Muckova P, Reindl J, et al. Acute phase proteins as promising biomarkers: perspectives and limitations for human and veterinary medicine. Proteomics Clin Appl. (2016) 10:1077–92. doi: 10.1002/prca.201600028
12. Johnson HL, Chiou CC, Cho CT. Applications of acute phase reactants in infectious diseases. J Microbiol Immunol Infect. (1999) 32:73–82.
13. Eckersall PD, Conner JG, Harvie J. An immunoturbidimetric assay for canine C-reactive protein. Vet Res Commun. (1991) 15:17–24. doi: 10.1007/BF00497786
14. Conner JG, Eckersall PD, Wiseman A, Aitchison TC, Douglas TA. Bovine acute phase response following turpentine injection. Res Vet Sci. (1988) 44:82–8. doi: 10.1016/0034-5288(88)90018-5
15. Pepys MB, Baltz ML, Tennent GA, Kent J, Ousey J, Rossdale PD. Serum amyloid A protein (SAA) in horses: objective measurement of the acute phase response. Equine Vet J. (1989) 21:106–9. doi: 10.1111/j.2042-3306.1989.tb02108.x
16. Eckersall PD, Schmidt EM. The final hurdles for acute phase protein analysis in small animal practice. J Small Anim Pract. (2014) 55:1–3. doi: 10.1111/jsap.12168
17. Kjelgaard-Hansen M, Mikkelsen LF, Kristensen AT, Jensen AL. Study on biological variability of five acute-phase reactants in dogs. Comp Clin Path. (2003) 12:69–74. doi: 10.1007/s00580-003-0477-z
18. Solter PF, Hoffmann WE, Hungerford LL, Siegel JP, St Denis SH, Dorner JL. Haptoglobin and ceruloplasmin as determinants of inflammation in dogs. Am J Vet Res. (1991) 52:1738–42.
19. Berta A, Sumich JL, Kovacs KM (Eds). Chapter 1 – Introduction. In: Mar Mammals: Evolutionary Biology. 3rd ed. Academic Press (2015) p. 1–14.
20. Levin M. Marine mammal immunology. In: Dierauf LA, Gulland FMD, Whitman KL, editors. CRC Handbook of Marine Mammal Medicine. 3rd ed. Boca Raton, FL: CRC Press (2018). p. 209–30.
21. Desforges JP, Sonne C, Levin M, Siebert U, De Guise S, Dietz R. Immunotoxic effects of environmental pollutants in marine mammals. Environ Int. (2016) 86:126–39. doi: 10.1016/j.envint.2015.10.007
22. Di Guardo G, Centelleghe C, Mazzariol S. Cetacean host-pathogen interaction(s): critical knowledge gaps. Front Immunol. (2018) 9:2815. doi: 10.3389/fimmu.2018.02815
23. Beineke A, Siebert U, Wohlsein P, Baumgärtner W. Immunology of whales and dolphins. Vet Immunol Immunopathol. (2010) 133:81–94. doi: 10.1016/j.vetimm.2009.06.019
24. Noda K, Aoki M, Akiyoshi H, Asaki H, Shimada T, Ohashi F. Evaluation of the polymorphonuclear cell functions of bottlenose dolphins. J Vet Med Sci. (2003) 65:727–9. doi: 10.1292/jvms.65.727
25. De Guise S, Bernier J, Dufresne MM, Martineau D, Beland P, Fournier M. Immune functions in beluga whales (Delphinapterus leucas): evaluation of mitogen-induced blastic transformation of lymphocytes from peripheral blood, spleen and thymus. Vet Immunol Immunopathol. (1996) 50:117–26. doi: 10.1016/0165-2427(95)05490-1
26. Keogh MJ, Spoon T, Ridgway SH, Jensen E, Van Bonn W, Romano TA. Simultaneous measurement of phagocytosis and respiratory burst of leukocytes in whole blood from bottlenose dolphins (Tursiops truncatus) utilizing flow cytometry. Vet Immunol Immunopathol. (2011) 144:468–75 doi: 10.1016/j.vetimm.2011.08.017
27. Denis F, Archambault D. Molecular cloning and characterization of beluga whale (Delphinapterus leucas) interleukin-1beta and tumor necrosis factor-alpha. Can J Vet Res. (2001) 65:233–40.
28. St-Laurent G, Archambault D. Molecular cloning, phylogenetic analysis and expression of beluga whale (Delphinapterus leucas) interleukin 6. Vet Immunol Immunopathol. (2000) 73:31–44. doi: 10.1016/S0165-2427(99)00150-6
29. St-Laurent G, Béliveau C, Archambault D. Molecular cloning and phylogenetic analysis of beluga whale (Delphinapterus leucas) and grey seal (Halichoerus grypus) interleukin 2. Vet Immunol Immunopathol. (1999) 67:385–94. doi: 10.1016/S0165-2427(99)00009-4
30. Beineke A, Siebert U, van Elk N, Baumgärtner W. Development of a lymphocyte-transformation-assay for peripheral blood lymphocytes of the harbor porpoise and detection of cytokines using the reverse-transcription polymerase chain reaction. Vet Immunol Immunopathol. (2004) 98:59–68. doi: 10.1016/j.vetimm.2003.10.002
31. Beineke A, Siebert U, Müller G, Baumgärtner W. Increased blood interleukin-10 mRNA levels in diseased free-ranging harbor porpoises (Phocoena phocoena). Vet Immunol Immunopathol. (2007) 115:100–6. doi: 10.1016/j.vetimm.2006.09.006
32. Sitt T, Bowen L, Blanchard MT, Smith BR, Gershwin LJ, Byrne BA, et al. Quantitation of leukocyte gene expression in cetaceans. Dev Comp Immunol. (2008) 32:1253–9. doi: 10.1016/j.dci.2008.05.001
33. Erickson KL, DiMolfetto-Landen L, Wells RS, Reidarson T, Stott JL, Ferrick DA. Development of an interleukin-2 receptor expression assay and its use in evaluation of cellular immune responses in bottlenose dolphin (Tursiops truncatus). J Wildl Dis. (1995) 31:142–9. doi: 10.7589/0090-3558-31.2.142
34. Funke C, King DP, McBain JF, Adelung D, Stott JL. Expression and functional characterization of killer whale (Orcinus orca) interleukin-6 (IL-6) and development of a competitive immunoassay. Vet Immunol Immunopathol. (2003) 93:69–79. doi: 10.1016/S0165-2427(03)00055-2
35. Frouin H, Haulena M, Akhurst LM, Raverty SA, Ross PS. Immune status and function in harbor seal pups during the course of rehabilitation. Vet Immunol Immunopathol. (2013) 155:98–109. doi: 10.1016/j.vetimm.2013.06.011
36. Frouin H, Lebeuf M, Hammill M, Fournier M. Phagocytosis in pup and adult harbour, grey and harp seals. Vet Immunol Immunopathol. (2010) 134:160–8. doi: 10.1016/j.vetimm.2009.08.017
37. Fonfara S, Kakuschke A, Rosenberger T, Siebert U, Prange A. Cytokine and acute phase protein expression in blood samples of harbour seal pups. Mar Biol. (2008) 155:337–45. doi: 10.1007/s00227-008-1031-y
38. Levin M, Romano T, Matassa K, De Guise S. Validation of a commercial canine assay kit to measure pinniped cytokines. Vet Immunol Immunopathol. (2014) 160:90–6. doi: 10.1016/j.vetimm.2014.04.001
39. Bossart GD, Reidarson TH, Dierauf LA, Duffield DA. Clinical Pathology. In: Dierauf LA, Gulland FMD, editors. CRC Handbook of Marine Mammal Medicine. 2nd ed. Boca Raton, Fl: CRC Press (2001). p. 383–436.
40. King DP, Aldrige BM, Kennedy-Stoskopf S, Stott JL. Immunology. In: Dierauf LA, Gulland FMD, editors. CRC Handbook of Marine Mammal Medicine. 2nd ed. Boca Raton, Fl: CRC Press (2001). p. 237–52.
41. Taylor BC, Brotheridge RM, Jessup DA, Stott JL. Measurement of serum immunoglobulin concentration in killer whales and sea otters by radial immunodiffusion. Vet Immunol Immunopathol. (2002) 89:187–95. doi: 10.1016/S0165-2427(02)00208-8
42. Nollens HH, Green LG, Duke D, Walsh MT, Chittick B, Gearhart S, et al. Development and validation of monoclonal and polyclonal antibodies for the detection of immunoglobulin G of bottlenose dolphins (Tursiops truncatus). J Vet Diagn Invest. (2007) 19:465–70. doi: 10.1177/104063870701900502
43. Ruiz CL, Nollens HH, Venn-Watson S, Green LG, Wells RS, Walsh MT, et al. Baseline circulating immunoglobulin G levels in managed collection and free-ranging bottlenose dolphins (Tursiops truncatus). Dev Comp Immunol. (2009) 33:449–55. doi: 10.1016/j.dci.2008.09.002
44. Cray C, Zaias J, Altman NH. Acute phase response in animals: a review. Comp Med. (2009) 59:517–26.
45. Schwacke LH, Hall AJ, Townsend FI, Wells RS, Hansen LJ, Hohn AA, et al. Hematologic and serum biochemical reference intervals for free-ranging common bottlenose dolphins (Tursiops truncatus) and variation in the distributions of clinicopathologic values related to geographic sampling site. Am J Vet Res. (2009) 70:973–85. doi: 10.2460/ajvr.70.8.973
46. Gili C, Bonsembiante F, Bonanni R, Giordano A, Ledda S, Beffagna G, et al. Detection of hereditary bisalbuminemia in bottlenose dolphins (Tursiops truncatus, Montagu 1821): comparison between capillary zone and agarose gel electrophoresis. BMC Vet Res. (2016) 12:172. doi: 10.1186/s12917-016-0801-x
47. Stockham SL, Scott MA. Fundamentals of Veterinary Clinical Pathology. Amse, IA: Blackwell Pub (2008) p. 376.
48. St. Aubin DJ, Forney KA, Chivers SJ, Scott MD, Danil K, Romano TA, et al. Hematological, serum, and plasma chemical constituents in pantropical spotted dolphins (Stenella attenuata) following chase, encirclement, and tagging. Mar Mammal Sci. (2013) 29:14–35. doi: 10.1111/j.1748-7692.2011.00536.x.
49. Tryland M, Thoresen SI, Kovacs KM, Lydersen C. Serum chemistry of free-ranging white whales (Delphinapterus leucas) in Svalbard. Vet Clin Pathol. (2006) 35:199–203. doi: 10.1111/j.1939-165X.2006.tb00114.x
50. Tryland M, Brun E. Serum chemistry of the minke whale from the northeastern Atlantic. J Wildl Dis. (2001) 37:332–41. doi: 10.7589/0090-3558-37.2.332
51. Robeck TR, Nollens HH. Hematological and serum biochemical analytes reflect physiological challenges during gestation and lactation in killer whales (Orcinus orca). Zoo Biol. (2013) 32:497–509. doi: 10.1002/zoo.21083
52. Greig DJ, Gulland FMD, Rios CA, Hall AJ. Hematology and serum chemistry in stranded and wild-caught harbor seals in central california: reference intervals, predictors of survival, and parameters affecting blood variables. J Wildl Dis. (2010) 46:1172–84. doi: 10.7589/0090-3558-46.4.1172
53. Tryland M, Lydersen C, Kovacs KM, Thoresen SI. Serum chemistry reference values in free-ranging North Atlantic male walruses (Odobenus rosmarus rosmarus) from the Svalbard archipelago. Vet Clin Pathol. (2009) 38:501–6. doi: 10.1111/j.1939-165X.2009.00154.x
54. Cray C, Arheart KL, Hunt M, Clauss T, Leppert LL, Roberts K, et al. Acute phase protein quantitation in serum samples from healthy Atlantic bottlenose dolphins (Tursiops truncatus). J Vet Diagn Invest. (2013) 25:107–11. doi: 10.1177/1040638712467986
55. Müller S, Lehnert K, Seibel H, Driver J, Ronnenberg K, Teilmann J, et al. Evaluation of immune and stress status in harbour porpoises (Phocoena phocoena): can hormones and mRNA expression levels serve as indicators to assess stress? BMC Vet Res. (2013) 9:145. doi: 10.1186/1746-6148-9-145
56. Harr K, Harvey J, Bonde R, Murphy D, Lowe M, Menchaca M, et al. Comparison of methods used to diagnose generalized inflammatory disease in manatees (Trichechus manatus latirostris). J Zoo Wildl Med. (2006) 37:151–9. doi: 10.1638/05-023.1
57. Cray C, Rodriguez M, Dickey M, Brewer LB, Arheart KL. Assessement of serum amyloid a levels in the rehabilitation setting in the florida manatee (Trichechus manatus latirostris). J Zoo Wildl Med. (2013) 44:911–7. doi: 10.1638/2012-0270R.1
58. Zenteno-Savin T, Castellini MA, Rea LD, Fadely BS. Plasma haptoglobin levels in threatened Alaskan pinniped populations. J Wildl Dis. (1997) 33:64–71. doi: 10.7589/0090-3558-33.1.64
59. Rosenfeld H, Lassen S, Prange A. Characterization of haptoglobin in the blood plasma of harbor seals (Phoca vitulina). J Proteome Res. (2009) 8:2923–32. doi: 10.1021/pr900035s
60. Terasawa F, Arai T, Tokura T, Ohshita I. Fibrinogen concentrations in captive bottlenose dolphins during pregnancy. J Vet Med Sci. (2008) 70:1277–9. doi: 10.1292/jvms.70.1277
61. Segawa T, Otsuka T, Itou T, Suzuki M, Karatani N, Sakai T. Characterization of the circulating serum amyloid A in bottlenose dolphins. Vet Immunol Immunopathol. (2013) 152:218–24. doi: 10.1016/j.vetimm.2012.12.006
62. Segawa T, Amatsuji H, Suzuki K, Suzuki M, Yanagisawa M, Itou T, et al. Molecular characterization and validation of commercially available methods for haptoglobin measurement in bottlenose dolphin. Results Immunol. (2013) 3:57–63. doi: 10.1016/j.rinim.2013.05.003
63. Funke C, King DP, Brotheridge RM, Adelung D, Stott JL. Harbor seal (Phoca vitulina) C-reactive protein (C-RP): purification, characterization of specific monoclonal antibodies and development of an immuno-assay to measure serum C-RP concentrations. Vet Immunol Immunopathol. (1997) 59:151–62. doi: 10.1016/S0165-2427(97)00059-7
64. Kakuschke A, Erbsloeh H-B, Griesel S, Prange A. Acute phase protein haptoglobin in blood plasma samples of harbour seals (Phoca vitulina) of the Wadden Sea and of the isle Helgoland. Comp Biochem Physiol B Biochem Mol Biol. (2010) 155:67–71. doi: 10.1016/j.cbpb.2009.10.002
65. Kjelgaard-Hansen M, Jacobsen S. Assay validation and diagnostic applications of major acute-phase protein testing in companion animals. Clin Lab Med. (2011) 31:51–70. doi: 10.1016/j.cll.2010.10.002
66. Mackness M, Mackness B. Human paraoxonase-1 (PON1): gene structure and expression, promiscuous activities and multiple physiological roles. Gene. (2015) 567:12–21. doi: 10.1016/j.gene.2015.04.088
67. Watson CE, Draganov DI, Billecke SS, Bisgaier CL, La Du BN. Rabbits possess a serum paraoxonase polymorphism similar to the human Q192R. Pharmacogenetics. (2001) 11:123–34. doi: 10.1097/00008571-200103000-00003
68. Silveira PA, Schwegler E, Montagner P, Krause AR, Acosta DA, Halfen J, et al. Characterization of single nucleotide polymorphisms in the promoter region of the bovine paraoxonase 1 (PON1) gene affecting serum enzyme activity in dairy cows. Vet J. (2015) 205:101–3. doi: 10.1016/j.tvjl.2015.04.028
69. Meyer WK, Jamison J, Richter R, Woods SE, Partha R, Kowalczyk A, et al. Ancient convergent losses of Paraoxonase 1 yield potential risks for modern marine mammals. Science. (2018) 361:591–4. doi: 10.1126/science.aap7714
70. Bonsembiante F, Centelleghe C, Rossi G, Giglio S, Madeo E, Gelain ME, et al. Clinico-pathological findings in a striped dolphin (Stenella coeruleoalba) affected by rhabdomyolysis and myoglobinuric nephrosis (capture myopathy). J Vet Med Sci. (2017) 79:1013–8. doi: 10.1292/jvms.17-0023
71. Geffré A, Friedrichs K, Harr K, Concordet D, Trumel C, Braun JP. Reference values: a review. Vet Clin Pathol. (2009) 38:288–98. doi: 10.1111/j.1939-165X.2009.00179.x
72. Venn-Watson S, Jensen ED, Ridgway SH. Effects of age and sex on clinicopathologic reference ranges in a healthy managed Atlantic bottlenose dolphin population. J Am Vet Med Assoc. (2007) 231:596–601. doi: 10.2460/javma.231.4.596
73. Paltrinieri S, Gelain ME, Ceciliani F, Ribera AM, Battilani M. Association between faecal shedding of feline coronavirus and serum alpha1-acid glycoprotein sialylation. J Feline Med Surg. (2008) 10:514–8. doi: 10.1016/j.jfms.2008.04.004
74. Rossi G, Capitani L, Ceciliani F, Restelli L, Paltrinieri S. Hyposialylated α1-acid glycoprotein inhibits phagocytosis of feline neutrophils. Res Vet Sci. (2013) 95:465–71. doi: 10.1016/j.rvsc.2013.04.026
75. Pocacqua V, Provasi E, Paltrinieri S, Gelain E, Comunian C, Ceciliani F. Glycan moiety modifications of feline alpha1-acid glycoprotein in retrovirus (FIV, FeLV) affected cats. Vet Immunol Immunopathol. (2005) 107:17–26. doi: 10.1016/j.vetimm.2005.02.021
76. Bressem M Van, Van Bressem M-F, Raga JA, Di Guardo G, Jepson PD, Duignan PJ, et al. Emerging infectious diseases in cetaceans worldwide and the possible role of environmental stressors. Dis Aquat Organ. (2009) 86:143–57. doi: 10.3354/dao02101
Keywords: marine mammals, immune system, acute phase reaction, acute phase proteins, serum proteins
Citation: Gelain ME and Bonsembiante F (2019) Acute Phase Proteins in Marine Mammals: State of Art, Perspectives and Challenges. Front. Immunol. 10:1220. doi: 10.3389/fimmu.2019.01220
Received: 29 November 2018; Accepted: 13 May 2019;
Published: 29 May 2019.
Edited by:
Mike Criscitiello, Texas A & M University, United StatesReviewed by:
Annalisa Pinsino, Istituto di Biomedicina e di Immunologia Molecolare Alberto Monroy (IBIM), ItalyCara Lisa Field, Marine Mammal Center, United States
Copyright © 2019 Gelain and Bonsembiante. This is an open-access article distributed under the terms of the Creative Commons Attribution License (CC BY). The use, distribution or reproduction in other forums is permitted, provided the original author(s) and the copyright owner(s) are credited and that the original publication in this journal is cited, in accordance with accepted academic practice. No use, distribution or reproduction is permitted which does not comply with these terms.
*Correspondence: Maria Elena Gelain, bWFyaWFlbGVuYS5nZWxhaW5AdW5pcGQuaXQ=