- Department of Molecular Microbiology and Immunology, Saint Louis University School of Medicine, St. Louis, MO, United States
CD5—a Bridge Between Thymic Selection and Peripheral Differentiation of Treg Cells
CD5 is a cysteine-rich scavenger-like receptor expressed by B-1a and T cells that is generally thought to be a negative regulator of antigen-specific receptor signaling (1). In developing thymocytes, expression of CD5 correlates with T cell receptor (TCR) signal strength, resulting in high CD5 expression by thymocytes that strongly interact with self-peptide: major histocompatibility complexes (MHC) (2). While the mechanisms that mediate the ability of CD5 to dampen TCR signaling are still partially unclear, it has been shown that negative regulators, including SHP-1, Ras-GAP, c-Cbl and casein kinase 2 (CK2), are recruited to the cytoplasmic domain of CD5 (1, 3). Further, CD5 has been shown to interact with TCR signaling molecules such as PI3K, Fyn, Lck, and ZAP-70 (1, 4, 5). Other functions of CD5 in mature peripheral T cells influence their survival, anergy, and T helper 17 (Th17) cell differentiation. These functions of CD5 rely on regulation of the activation of mechanistic target of rapamycin (mTOR) as well as crucial interactions between CD5 and CK2 (3, 6, 7). Although ligation of CD5 can modify its functions, the cell-autonomous functions of CD5 in T cells are independent of CD5 engagement of its extracellular domain by a specific ligand (8). In contrast, such cell-autonomous functions of CD5 depend on its specific level of expression. In the thymus, negative regulation of TCR signaling by CD5 allows for strongly self-reactive thymocytes to escape deletion during thymic selection, therefore extending the range of naïve T cells cross-reactive against various pathogens (2, 9–14). These T cells retain high expression of CD5 upon their migration to the periphery, so the expression of CD5 in T cells can be used as a marker of TCR signal strength (1). Further, a specific high or low expression of CD5 may also indirectly mark some T cells differing in intrinsic changes in TCR signaling pre-determined by TCR interactions in the thymus (14, 15). Overall, the increased expression of CD5 in CD4+ T cells may serve as an indicator of self-reactivity in the polyclonal T cell repertoire and such CD5hi T cells present greater risks for autoimmune responses (11–14).
Additional specific mechanisms are therefore needed to mitigate the risk of autoimmune responses by self-reactive CD5hi T cells that are released into the periphery. Our previous results elucidated a CD5-dependent mechanism, separate from its role in regulation of TCR signaling, that facilitates the formation of peripheral regulatory T (pTreg) cells from CD5hi T cells (16, 17). This mechanism is also consistent with the previously proposed idea of precursors for pTreg cells that are present among peripheral T cells (18, 19). In contrast to the CD5-dependent process of pTreg cell conversion, the development of tTreg cells in thymus is independent of CD5 functions (20, 21). We found that CD5 promotes induction of Foxp3 expression and conversion into pTreg cells by opposing in CD5hi T cells the activation of mTOR mediated by effector differentiating cytokines such as interleukin-4 (IL-4), IL-6, and interferon-γ (IFN-γ) that can be constantly produced by small numbers of effector T cells present even under physiological steady state conditions (16, 17). Similarly in vitro, the functions of CD5 prevent effector differentiating cytokines from blocking the TGF-β mediated induction of iTreg cells (17, 22). This effect on mTOR activation is likely mediated through CD5 interference with PI3K signaling, and an inhibition of either PI3K or mTOR leads to a restored conversion of Treg cells in T cells lacking CD5 functions (17). This is consistent with the established roles of PI3K and mTOR in the inhibition of Treg cell differentiation (23–25). While it is still unclear how CD5 inhibits PI3K/AKT/mTOR signaling in pTreg cells, CK2 may be involved in this process as it has been shown to modulate activation of this pathway in other contexts (26, 27). Further, it remains unclear to what extent the initially formed CD5hi T cells can resist subsequent differentiation into effector T cells under acute pro-inflammatory conditions. Future research may clarify this.
Regulation of Peripheral CD5 Expression by Dendritic Cells
The formation of pTreg cells is crucial for peripheral tolerance and prevention of specific autoimmunity (28, 29). However, as discussed above, the expression of CD5 among a polyclonal T cell repertoire represents a spectrum resulting in different expression of CD5 in T cells of various antigenic specificities (11–14). Therefore, the designation of T cells leaving the thymus as “CD5hi” is in fact relative. Also, despite a lower affinity for the peptides represented in thymus, some of the developing CD5lo T cells can still include clones highly responsive to peripheral self-antigens (30). Therefore, the increased expression of CD5 induced in the thymus cannot sufficiently direct a conversion of antigen-specific pTreg cells. Further, a model that relies on pTreg cell conversion being pre-programmed in the thymus is not easily reconciled with the well-established instructive roles of peripheral dendritic cells (DCs) to govern pTreg cell conversion in response to specific antigens and other signals perceived by such T cells and DCs in the periphery (28). However, in addition to the mechanisms regulating CD5 expression previously identified in the thymus, peripheral T cells can also increase their CD5 expression in response to pro-tolerogenic stimulation (31, 32). Such upregulation of CD5 expression in peripheral self-reactive T cells may therefore act to further promote a conversion into pTreg cells. We recently clarified the mechanisms governing induction of CD5 expression in peripheral T cells. Results from our laboratory have elucidated crucial roles for the immunoregulatory axis dependent on the immunoglobulin superfamily member B and T lymphocyte associated (BTLA) expressed on some DCs (BTLAhi) that signals through herpesvirus entry mediator (HVEM) in T cells to up regulate the expression of CD5 and instruct the conversion of pTreg cells under physiological conditions that include multiple pro-effector and pro-tolerogenic cytokines (17, 22) and (Figure 1).
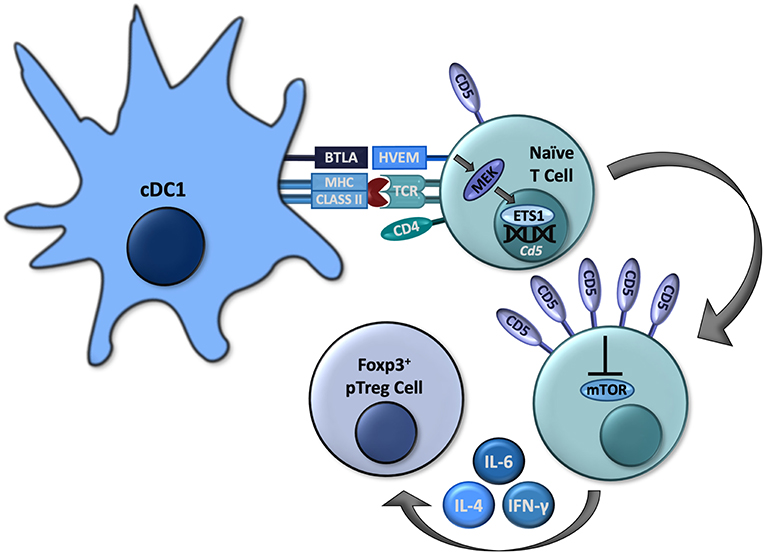
Figure 1. Some cDC1s express BTLA, which can signal through HVEM in naïve CD4+ T cells to activate MEK and subsequently ETS1 to increase expression of Cd5. High CD5 expression allows these to cells to convert to Foxp3+ pTreg cells by interfering with mTOR activation, even in the presence of effector-differentiating cytokines such as IL-4, IL-6, and IFN-γ.
DCs are antigen presenting cells (APCs) that are critical for the initiation and regulation of T cell responses to foreign and self-antigens. The outcomes of antigen-specific interactions between DCs and T cells are governed by immunomodulatory molecules expressed by each cell type (33). Both human and murine DCs consist of two main populations, conventional (myeloid) (cDC) and plasmacytoid (pDC), both of which develop from progenitors in the bone marrow that differentiate into various subsets present throughout multiple tissues (34–36). The cDC population can be further divided into the cDC1 and cDC2 subsets, defined by the transcription factors required for their development. The cDC1 subset, which requires the transcription factors Irf8, Id2, and Batf3 for development, can be distinguished by expression of XCR1 and BTLA. In contrast, the cDC2 subset depends on the transcription factor Irf4 for development and is distinguished by expression of CD172a (SIRPα) (22, 34, 35). In the absence of specific acute pro-inflammatory stimuli (steady state), DCs generally promote T cell tolerance that crucially relies on the induction of pTreg cells (29, 37, 38). Although the developmental designation of DC subsets does not strictly overlap with their distinct immune functions, the specific subsets are characterized by a degree of functional specialization. Whereas, cDC2s can preferentially promote Th2, Th17, and follicular helper T cell differentiation, cDC1s have crucial roles for the cross-priming of CD8+ T cells, priming of Th1 cells, and induction of CD4+CD25+Foxp3+ pTreg cells (28, 33, 34, 39).
BTLA and HVEM Instructed Regulation of CD5 Expression
In addition to cDC1s, BTLA is also expressed in T cells, B cells, macrophages, NK cells, and NKT cells (22, 40–42). BTLA contains three immunoreceptor tyrosine-based inhibition motifs (ITIMs) which, upon phosphorylation, recruit Src homology domain 2 (SH2)-containing protein tyrosine phosphatases, SHP-1 and SHP-2, which exert a variety of inhibitory effects within various lymphocytes (40, 41). However, in addition to the above-described intrinsic signaling mediated by its intracellular domains, BTLA also serves as a ligand for HVEM, a tumor necrosis factor receptor (TNFR) superfamily member primarily expressed in naïve CD4+ and CD8+ T cells, which downregulate its expression following activation (41). HVEM contains four extracellular cysteine-rich domains (CRDs) that mediate its specific binding to BTLA or other ligands such as LIGHT in different conformations (41). Following ligation by LIGHT, HVEM activates PI3K leading to immunogenic lymphocyte activation (43). Upon binding to BTLA, HVEM can signal through TNF receptor associated factor 2 (TRAF2) to induce phosphorylation of STAT3, resulting in NF-kB activation as well as other pro-survival signals (44, 45). These interactions between BTLA and HVEM can also modulate a variety of immunological processes, including CD8+ T cell survival and memory formation, Treg cell functionality, and DC homeostasis (41, 42, 45, 46). We recently established specific roles for BTLA and HVEM in governing a conversion of pTreg cells through the modulation of CD5 expression in T cells (22). We found that in antigen-specific T cells activated by BTLA-expressing cDC1s, HVEM-mediated signals lead to an increased phosphorylation of mitogen-activated protein kinase (MAPK) kinase (MEK) and expression of ETS1, resulting in increased transcription of Cd5 and the corresponding increased surface expression in CD5hi T cells (22). While other signals might contribute to regulation of CD5 expression, BTLA-HVEM signaling is indispensable for the upregulation of CD5 in T cells activated by DCs in vivo under normal physiological conditions (22). The subsequent conversion of such DC-induced CD5hi T cells into pTreg cells is then enhanced because CD5hi T cells become resistant to the specific mTOR-dependent signals mediated by effector differentiating cytokines as discussed above (Figure 1).
Conclusions and Future Directions
The regulation of immune tolerance by DCs through the induction of pTreg cells is mediated by various mechanisms utilized by specific subsets of DCs (28, 33). The BTLA-HVEM-CD5 signaling axis is critically important for the ability of cDC1s to promote differentiation of pTreg cells that have crucial functions in blocking an autoimmune process (22). Despite the finding that BTLA-HVEM signaling upregulates CD5 expression in T cells, the mechanisms utilized by CD5 to modulate functions of mTOR and to promote the differentiation of pTreg cells still need to be fully clarified. Particularly, determining the possible roles of the established interactions between CD5 and CK2 could help to elucidate this process. Further, CD5 may govern relevant molecular functions in the newly formed pTreg cells whose responses to cytokine-mediated stimulation depend on specific transcription factors (22, 38). Obtaining a deeper mechanistic understanding of the signaling mechanisms by which DCs control T cell responses, such as the BTLA-HVEM-CD5 axis described here, is necessary for the development of new immunotherapies for the treatment of cancer, autoimmunity, and infection.
Author Contributions
All authors listed have made a substantial, direct and intellectual contribution to the work, and approved it for publication.
Funding
This work was supported in part by grants from the National Institute of Allergy and Infectious Diseases of the National Institutes of Health (R01AI113903) (to DH). This publication is solely the responsibility of the authors and does not necessarily represent the official view of the National Institutes of Health.
Conflict of Interest Statement
The authors declare that the research was conducted in the absence of any commercial or financial relationships that could be construed as a potential conflict of interest.
References
1. Soldevila G, Raman C, Lozano F. The immunomodulatory properties of the CD5 lymphocyte receptor in health and disease. Curr Opin Immunol. (2011) 23:310–8. doi: 10.1016/j.coi.2011.03.003
2. Azzam HS, Grinberg A, Lui K, Shen H, Shores EW, Love PE. CD5 expression is developmentally regulated by T cell receptor (TCR) signals and TCR avidity. J Exp Med. (1998) 188:2301–11. doi: 10.1084/jem.188.12.2301
3. Axtell RC, Xu L, Barnum SR, Raman C. CD5-CK2 binding/activation-deficient mice are resistant to experimental autoimmune encephalomyelitis: protection is associated with diminished populations of IL-17-expressing T cells in the central nervous system. J Immunol. (2006) 177:8542–9. doi: 10.4049/jimmunol.177.12.8542
4. Gary-Gouy H, Lang V, Sarun S, Boumsell L, Bismuth G. In vivo association of CD5 with tyrosine-phosphorylated ZAP-70 and p21 phospho-zeta molecules in human CD3+ thymocytes. J Immunol. (1997) 159:3739–47.
5. Mier-Aguilar CA, Vega-Baray B, Burgueno-Bucio E, Lozano F, Garcia-Zepeda EA, Raman C, et al. Functional requirement of tyrosine residue 429 within CD5 cytoplasmic domain for regulation of T cell activation and survival. Biochem Biophys Res Commun. (2015) 466:381–7. doi: 10.1016/j.bbrc.2015.09.033
6. Sestero CM, McGuire DJ, De Sarno P, Brantley EC, Soldevila G, Axtell RC, et al. CD5-dependent CK2 activation pathway regulates threshold for T cell anergy. J Immunol. (2012) 189:2918–30. doi: 10.4049/jimmunol.1200065
7. McGuire DJ, Rowse AL, Li H, Peng BJ, Sestero CM, Cashman KS, et al. CD5 enhances Th17-cell differentiation by regulating IFN-gamma response and RORgammat localization. Eur J Immunol. (2014) 44:1137–42. doi: 10.1002/eji.201343998
8. Bhandoola A, Bosselut R, Yu Q, Cowan ML, Feigenbaum L, Love PE, et al. CD5-mediated inhibition of TCR signaling during intrathymic selection and development does not require the CD5 extracellular domain. Eur J Immunol. (2002) 32:1811–7. doi: 10.1002/1521-4141(200206)32:6<1811::AID-IMMU1811>3.0.CO
9. Tarakhovsky A, Kanner SB, Hombach J, Ledbetter JA, Muller W, Killeen N, et al. A role for CD5 in TCR-mediated signal transduction and thymocyte selection. Science. (1995) 269:535–7. doi: 10.1126/science.7542801
10. Kassiotis G, Zamoyska R, Stockinger B. Involvement of avidity for major histocompatibility complex in homeostasis of naive and memory T cells. J Exp Med. (2003) 197:1007–16. doi: 10.1084/jem.20021812
11. Stritesky GL, Jameson SC, Hogquist KA. Selection of self-reactive T cells in the thymus. Annu Rev Immunol. (2012) 30:95–114. doi: 10.1146/annurev-immunol-020711-075035
12. Mandl JN, Monteiro JP, Vrisekoop N, Germain RN. T cell-positive selection uses self-ligand binding strength to optimize repertoire recognition of foreign antigens. Immunity. (2013) 38:263–74. doi: 10.1016/j.immuni.2012.09.011
13. Klein L, Kyewski B, Allen PM, Hogquist KA. Positive and negative selection of the T cell repertoire: what thymocytes see (and don't see). Nat Rev Immunol. (2014) 14:377–91. doi: 10.1038/nri3667
14. Persaud SP, Parker CR, Lo WL, Weber KS, Allen PM. Intrinsic CD4+ T cell sensitivity and response to a pathogen are set and sustained by avidity for thymic and peripheral complexes of self peptide and MHC. Nat Immunol. (2014) 15:266–74. doi: 10.1038/ni.2822
15. Fulton RB, Hamilton SE, Xing Y, Best JA, Goldrath AW, Hogquist KA, et al. The TCR's sensitivity to self peptide-MHC dictates the ability of naive CD8(+) T cells to respond to foreign antigens. Nat Immunol. (2015) 16:107–17. doi: 10.1038/ni.3043
16. Henderson JG, Hawiger D. Regulation of extrathymic Treg cell conversion by CD5. Oncotarget. (2015) 6:26554–5. doi: 10.18632/oncotarget.5809
17. Henderson JG, Opejin A, Jones A, Gross C, Hawiger D. CD5 instructs extrathymic regulatory T cell development in response to self and tolerizing antigens. Immunity. (2015) 42:471–83. doi: 10.1016/j.immuni.2015.02.010
18. Schallenberg S, Tsai PY, Riewaldt J, Kretschmer K. Identification of an immediate Foxp3(-) precursor to Foxp3(+) regulatory T cells in peripheral lymphoid organs of nonmanipulated mice. J Exp Med. (2010) 207:1393–407. doi: 10.1084/jem.20100045
19. Zhao C, Davies JD. A peripheral CD4+ T cell precursor for naive, memory, and regulatory T cells. J Exp Med. (2010) 207:2883–94. doi: 10.1084/jem.20100598
20. Ordonez-Rueda D, Lozano F, Sarukhan A, Raman C, Garcia-Zepeda EA, Soldevila G. Increased numbers of thymic and peripheral CD4+ CD25+Foxp3+ cells in the absence of CD5 signaling. Eur J Immunol. (2009) 39:2233–47. doi: 10.1002/eji.200839053
21. Engel M, Sidwell T, Vasanthakumar A, Grigoriadis G, Banerjee A. Thymic regulatory T cell development: role of signalling pathways and transcription factors. Clin Dev Immunol. (2013) 8:617595. doi: 10.1155/2013/617595
22. Jones A, Bourque J, Kuehm L, Opejin A, Teague RM, Gross C, et al. Immunomodulatory functions of BTLA and HVEM govern induction of extrathymic regulatory T cells and tolerance by dendritic cells. Immunity. (2016) 45:1066–77. doi: 10.1016/j.immuni.2016.10.008
23. Haxhinasto S, Mathis D, Benoist C. The AKT-mTOR axis regulates de novo differentiation of CD4+Foxp3+ cells. J Exp Med. (2008) 205:565–74. doi: 10.1084/jem.20071477
24. Kang J, Huddleston SJ, Fraser JM, Khoruts A. De novo induction of antigen-specific CD4+CD25+Foxp3+ regulatory T cells in vivo following systemic antigen administration accompanied by blockade of mTOR. J Leukoc Biol. (2008) 83:1230–9. doi: 10.1189/jlb.1207851
25. Sauer S, Bruno L, Hertweck A, Finlay D, Leleu M, Spivakov M, et al. T cell receptor signaling controls Foxp3 expression via PI3K, Akt, and mTOR. Proc Natl Acad Sci USA. (2008) 105:7797–802. doi: 10.1073/pnas.0800928105
26. Park JH, Kim JJ, Bae Y-S. Involvement of PI3K-AKT-mTOR pathway in protein kinase CKII inhibition-mediated senescence in human colon cancer cells. Biochem Biophys Res Commun. (2013) 433:420–5. doi: 10.1016/j.bbrc.2013.02.108
27. Gibson SA, Yang W, Yan Z, Qin H, Benveniste EN. CK2 controls Th17 and regulatory T cell differentiation through inhibition of foxO1. J Immunol. (2018) 201:383. doi: 10.4049/jimmunol.1701592
28. Iberg CA, Jones A, Hawiger D. Dendritic cells as inducers of peripheral tolerance. Trends Immunol. (2017) 38:793–804. doi: 10.1016/j.it.2017.07.007
29. Jones A, Hawiger D. Peripherally induced regulatory T cells: recruited protectors of the central nervous system against autoimmune neuroinflammation. Front Immunol. (2017) 8:532. doi: 10.3389/fimmu.2017.00532
30. Richards DM, Ruggiero E, Hofer AC, Sefrin JP, Schmidt M, von Kalle C, et al. The contained self-reactive peripheral T cell repertoire: size, diversity, and cellular composition. J Immunol. (2015) 195:2067–79. doi: 10.4049/jimmunol.1500880
31. Stamou P, de Jersey J, Carmignac D, Mamalaki C, Kioussis D, Stockinger B. Chronic exposure to low levels of antigen in the periphery causes reversible functional impairment correlating with changes in CD5 levels in monoclonal CD8 T cells. J Immunol. (2003) 171:1278–84. doi: 10.4049/jimmunol.171.3.1278
32. Hawiger D, Masilamani RF, Bettelli E, Kuchroo VK, Nussenzweig MC. Immunological unresponsiveness characterized by increased expression of CD5 on peripheral T cells induced by dendritic cells in vivo. Immunity. (2004) 20:695–705. doi: 10.1016/j.immuni.2004.05.002
33. Bourque J, Hawiger D. Immunomodulatory bonds of the partnership between dendritic cells and T cells. Crit Rev Immunol. (2018) 38, 379–401. doi: 10.1615/CritRevImmunol.2018026790
34. Durai V, Murphy KM. Functions of murine dendritic cells. Immunity. (2016) 45:719–36. doi: 10.1016/j.immuni.2016.10.010
35. Guilliams M, Dutertre CA, Scott CL, McGovern N, Sichien D, Chakarov S, et al. Unsupervised high-dimensional analysis aligns dendritic cells across tissues and species. Immunity. (2016) 45:669–84. doi: 10.1016/j.immuni.2016.08.015
36. Collin M, Bigley V. Human dendritic cell subsets: an update. Immunology. (2018) 154:3–20. doi: 10.1111/imm.12888
37. Kretschmer K, Apostolou I, Hawiger D, Khazaie K, Nussenzweig MC, von Boehmer H. Inducing and expanding regulatory T cell populations by foreign antigen. Nature Immunol. (2005) 6:1219–27. doi: 10.1038/ni1265
38. Jones A, Opejin A, Henderson JG, Gross C, Jain R, Epstein JA, et al. Peripherally induced tolerance depends on peripheral regulatory T cells that require hopx to inhibit intrinsic IL-2 expression. J Immunol. (2015) 195:1489–97. doi: 10.4049/jimmunol.1500174
39. Krishnaswamy JK, Alsén S, Yrlid U, Eisenbarth SC, Williams A. Determination of T follicular helper cell fate by dendritic cells. Front Immunol. (2018) 9:2169. doi: 10.3389/fimmu.2018.02169
40. Watanabe N, Gavrieli M, Sedy JR, Yang J, Fallarino F, Loftin SK, et al. BTLA is a lymphocyte inhibitory receptor with similarities to CTLA-4 and PD-1. Nat Immunol. (2003) 4:670–9. doi: 10.1038/ni944
41. Murphy TL, Murphy KM. Slow down and survive: enigmatic immunoregulation by BTLA and HVEM. Annu Rev Immunol. (2010) 28:389–411. doi: 10.1146/annurev-immunol-030409-101202
42. Steinberg MW, Huang Y, Wang-Zhu Y, Ware CF, Cheroutre H, Kronenberg M. BTLA interaction with HVEM expressed on CD8(+) T cells promotes survival and memory generation in response to a bacterial infection. PLoS ONE 8:e77992. doi: 10.1371/journal.pone.0077992
43. So T, Croft M. Regulation of PI-3-kinase and Akt signaling in T lymphocytes and other cells by TNFR family molecules. Front Immunol. (2013) 4:139. doi: 10.3389/fimmu.2013.00139
44. Shui JW, Steinberg MW, Kronenberg M. Regulation of inflammation, autoimmunity, and infection immunity by HVEM-BTLA signaling. J Leukoc Biol. (2011) 89:517–23. doi: 10.1189/jlb.0910528
45. Shui JW, Larange A, Kim G, Vela JL, Zahner S, Cheroutre H, et al. HVEM signalling at mucosal barriers provides host defence against pathogenic bacteria. Nature. (2012) 488:222–5. doi: 10.1038/nature11242
Keywords: BTLA, HVEM, CD5, pTreg cells, dendritic cells
Citation: Bourque J and Hawiger D (2019) The BTLA–HVEM–CD5 Immunoregulatory Axis–An Instructive Mechanism Governing pTreg Cell Differentiation. Front. Immunol. 10:1163. doi: 10.3389/fimmu.2019.01163
Received: 18 March 2019; Accepted: 08 May 2019;
Published: 22 May 2019.
Edited by:
David William Scott, Uniformed Services University of the Health Sciences, United StatesReviewed by:
Subbarao Bondada, University of Kentucky, United StatesMichael Croft, La Jolla Institute for Immunology (LJI), United States
Copyright © 2019 Bourque and Hawiger. This is an open-access article distributed under the terms of the Creative Commons Attribution License (CC BY). The use, distribution or reproduction in other forums is permitted, provided the original author(s) and the copyright owner(s) are credited and that the original publication in this journal is cited, in accordance with accepted academic practice. No use, distribution or reproduction is permitted which does not comply with these terms.
*Correspondence: Daniel Hawiger, ZGFuaWVsLmhhd2lnZXImI3gwMDA0MDtoZWFsdGguc2x1LmVkdQ==