- 1Millennium Institute on Immunology and Immunotherapy, Departamento de Genética Molecular y Microbiología, Facultad de Ciencias Biológicas, Pontificia Universidad Católica de Chile, Santiago, Chile
- 2Millennium Institute on Immunology and Immunotherapy, Departamento de Ciencias Biológicas, Facultad de Ciencias de la Vida, Universidad Andrés Bello, Santiago, Chile
- 3División de Pediatría, Unidad de Enfermedades Respiratorias Pediátricas, Facultad de Medicina, Pontificia Universidad Católica de Chile, Santiago, Chile
The human respiratory syncytial virus (hRSV) is one of the most important causes of upper and lower respiratory tract infections in children and the main cause of bronchiolitis worldwide. Disease manifestations caused by hRSV may vary from mild to severe, occasionally requiring admission and hospitalization in intensive care units. Despite the high morbidity rates associated to bronchiolitis, treatment options against hRSV are limited and there are no current vaccination strategies to prevent infection. Importantly, the early identification of high-risk patients can help improve disease management and prevent complications associated with hRSV infection. Recently, the characterization of pro- and anti-inflammatory cytokine patterns produced during hRSV-related inflammatory processes has allowed the identification of potential prognosis biomarkers. A suitable biomarker should allow predicting the severity of the infection in a simple and opportune manner and should ideally be obtained from non-invasive samples. Among the cytokines associated with hRSV disease severity, IL-8, interferon-alpha (IFN-alpha), and IL-6, as well as the Th2-type cytokines thymic stromal lymphopoietin (TSLP), IL-3, and IL-33 have been highlighted as molecules with prognostic value in hRSV infections. In this review, we discuss current studies that describe molecules produced by patients during hRSV infection and their potential as biomarkers to anticipate the severity of the disease caused by this virus.
Introduction
The human respiratory syncytial virus (hRSV) is a viral agent predominantly involved in acute lower respiratory tract infections (LRTIs), frequently associated to bronchiolitis and pneumonia in children and infants (1, 2). HRSV is responsible for approimately 60% of all LRTIs in children under 5 years old and causes more than 80% of the reported cases in infants (3, 4). At the age of 2 years, almost all children have been infected with hRSV at least once, and disease severity among these children may vary from mild to severe manifestations, sometimes requiring hospitalization with oxygen administration or admission into intensive care units (5, 6). Moreover, hRSV infection may cause exacerbated airway diseases and has been associated with recurrent wheezing and asthma development (7, 8).
Several attempts to reduce the impact of hRSV-LRTI in health-care have been made. The first vaccine trial for hRSV was based on a formalin-inactivated hRSV formulation (FI-hRSV) in the 1960's, but this formulation was unable to generate an effective immune response and conversely produced an exacerbated disease in children after hRSV infection (9). Since this first failed attempt, several other vaccination strategies have been addressed, ranging from live-attenuated viral approaches to recombinant proteins, as well as recombinant organisms using both, viral and bacterial vectors as immunoadjuvants (10). It is important to highlight a growing number of clinical vaccine trials in the last decades aiming to identify a protective approach (phase I and II, ClinicalTrials.gov 2017: Identifier: NCT03213405 and 2018 Identifier: NCT03636906) (2, 11). However, despite the significant progress achieved in this field, until now there are no commercially available vaccines against hRSV (12).
Regarding hRSV disease management in high-risk groups, prophylaxis based on neutralizing monoclonal antibodies has been implemented to prevent severe manifestations associated to hRSV-LRTI (13–15). Palivizumab and Motavizumab are two humanized monoclonal antibodies generated against the hRSV fusion protein F that have shown efficacy in preventing hRSV infection and the capacity to decrease the rate of hospitalization of hRSV-infected infants (16). However, only Palivizumab has been licensed to be used as a therapy against hRSV severe infections associated with bronchiolitis and pneumonia. Yet, it is unable to induce long-lasting protection in those treated and the costs associated to its use make difficult the implementation of this strategy as a first treatment option (14). Despite the existence of the neutralizing antibodies described above as prophylactic and therapeutic strategies, these approaches do not work as vaccines. Hence, to date there is no successful and affordable strategy available to control hRSV outbreaks, which represent an important public health problem worldwide (17, 18).
Therefore, strategies to prevent complications derived from hRSV infection and improve disease management are needed. Based on this premise, early diagnosis, and prediction of disease severity has raised considerable interest in researchers and the search for biological biomarkers to predict disease severity during hRSV infection. In this review we discuss the latest studies available in PubMed on potential prognosis biomarkers and revise the feasibility of including them during routine hRSV diagnosis.
Characteristics and Pathogenesis of HRSV
HRSV is an enveloped, negative, single-stranded RNA virus belonging to the Pneumoviridae family (19, 20). The genome of hRSV has 10 genes encoding 11 proteins required for the replicative cycle of hRSV in infected cells (21, 22), as well as for the modulation of the host immune response (23). Two hRSV subtypes have been identified, A and B, with the subtype A mostly associated to outbreaks during winter in countries with temperate climates (24, 25). hRSV is transmitted by direct contact or aerosol particles and once in the airways it replicates in mucosal epithelial cells, starting in the upper respiratory tract and then continuing to the lower respiratory tract (26). When hRSV arrives to the lower respiratory tract, viral antigen recognition by innate immune cells induce an inflammatory response, a process that is the result of complex interactions between the pathogen and host factors (27, 28). Lung inflammation is likely the result of a non-effective activation of the innate immunity by hRSV infection, mainly leading to Th2 and/or Th17 immune responses that generate mucus overproduction in the airways and enhance the inflammatory immune response in this tissue, leading to lung immunopathology (29, 30). After airway epithelial cells (AECs) recognize hRSV components (e.g. F protein and virus-related nucleic acids) through Toll-like receptors (i.e., TLR3 or 4) (Figure 1A) and retinoic-acid inducible gene I (RIG-I) receptors, signaling pathways activate transcription factors, such as interferon-regulatory factor 3 (IRF-3), and nuclear factor κB (NF-κB) (Figure 1A). In turn, these proteins promote the transcription of several anti-viral genes and soluble molecules (30, 31). In response to hRSV infection, AECs produce proinflammatory molecules such as type-I and type-III interferons (IFN) (31, 32). IFNs bind to IFN receptors (e.g., IFNAR) located on the surface of target cells and activate signaling pathways via Signal Transducer and Activator of Transcription 1 (STAT-1) and STAT-2 transcription factors. Ideally, STAT will bind to IFN-regulatory factors for a complete promotion in the transcription of interferon-stimulated genes (ISGs). Concomitantly, pro-inflammatory cytokines such as IL-6, tumor necrosis factor alpha (TNF-α) and chemokines (e.g., CXCL8, CCL3, CCL2, and CCL5) are induced and secreted to the extracellular medium. Importantly, some of these molecules (i.e., CCL2 and CCL5) will promote the recruitment of leukocytes (i.e., monocytes and neutrophils), dendritic cells, macrophages, natural killer cells, and CD4+ T cells to the site of infection (31, 32).
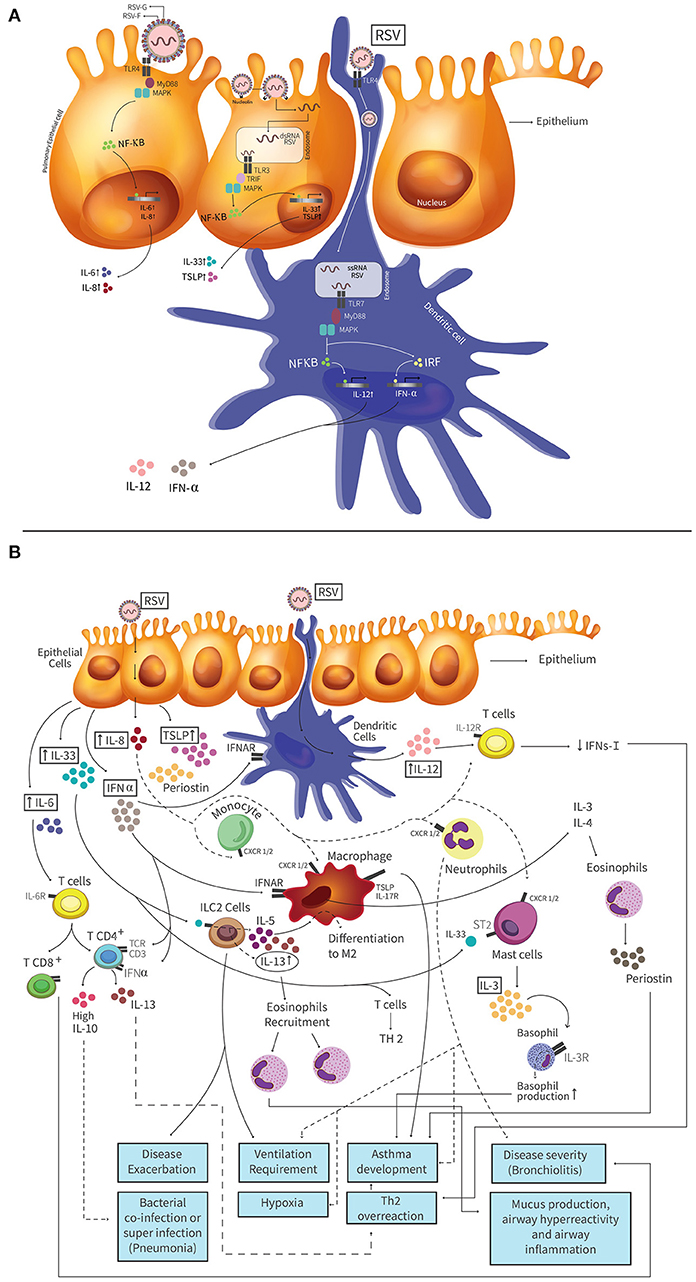
Figure 1. Pathogenesis of hRSV and molecules with a biomarker potential induced in the airways during hRSV infection. (A) HRSV attaches to airway epithelial cells and this binding is mediated by the interaction between the fusion (F) or glycoprotein (G) protein of hRSV. Toll-like receptor 4 (TLR4) is expressed on AECs and it is involved in the hRSV entry. When hRSV F protein binds to TLR4, this triggers a cascade of signaling, where the protein myeloid differentiation primary response 88 (MyD88) is activated. The activation of MyD88 leads to activation of mitogen-activated protein kinase (MAPK), and the NF-kB transcription factor. Activated NF-kB translocates to the nucleus and promotes the production of Th1 cytokines (like as TNF-α, IL-6, and IL-8). Nucleolin is a protein located on the cell surface that is also involved in the entry process of hRSV, which generates a fusion between host cell membrane and the virus. This fusion allows the entry of the viral genetic material to the cell, and the binding of dsRNA to TLR3. TLR3 triggers a cascade of signaling by the TIR-domain-containing adapter-inducing interferon-β (TRIF), MAPKs and NF-kB transcription factor. This signaling pathway promotes the IL-33 and TSLP production. HRSV also can infect Dendritic Cells (DCs) and the virus mediates its entry by TLR4 receptor, present on the surface of the DC. DCs are then infected and the genetic material of the virus enters the cell. dsRNA binds TLR7 receptor, present in the endosome produced by the fusion, which one TLR3 triggers a cascade of signaling by the MyD88 protein, MAPKs and NF-kB transcription factor or interferon-regulatory factor (IRF). Those signaling pathways promote the IL-12 and IFN-α production, respectively. (B) Infected AECs secrete several cytokines and chemokines that have been described as potential biomarkers. High IL-33 levels are produced by AECs and cells expressing ST2 receptor, such as ILC2s, respond to IL-33 through the production of IL-5 and IL-13, which promote the recruitment of eosinophils that generate disease exacerbation and is associated to ventilation requirement. The mast cells also express the ST2 receptor and when IL-33 binds to these receptors the production of IL-3 is promoted. AECs produce high levels of IL-8, promoting the recruitment neutrophils to the infection site, that could generate a degree of hypoxia, ventilation requirement and asthma development. TSLP production is mediate by AECs. This cytokine is recognized by the receptor TSLPR, which is expressed by macrophages, generating an exacerbation of the disease and asthma. Periostin is produced by AECs or eosinophils. This protein increases the expression of inflammatory mediators. Deposits of periostin in the lung is associated with increased severity of asthma. IL-6 is produced by AECs and promotes a Th2 response. This cytokine is involved in the promotion of naïve differentiation to CD4+ and CD8+ T cell. CD4+ T cells trigger the IL-13 production and Th2 overreaction response. CD8+ T cells increase the disease severity. IFN-α is produced by pDCs and AECs. At late times of infection, high levels of this cytokine produce high IL-10 levels by T cells. IL-12 is produced by pDCs and promotes the differentiation of naive T cells into Th1 cells and induces weak IFN-γ-production by T cells. This low IFN-γ-production generate a Th2 overreaction response. IL-3 promotes basophil and eosinophil production, triggering inflammatory and allergic diseases as asthma. IL-13 is produced by ILC2 cells and CD4+ T cells, among other. High IL-13 levels result in a Th2 overreaction response and the recruitment of eosinophils that generate exacerbated mucus production, airway hyperreactivity and inflammation. Different lines (dotted and solid) were used to facilitate understanding of the figure and the different signaling pathways involved.
Effective clearance of the hRSV requires a balanced Th1 and Th2 adaptive immune response, which promotes IFN-γ production by cytotoxic CD8+ T cells (27, 33). However, during hRSV infection a weak type-I IFN response is elicited in the host, whereby viral replication is effective in infected cells and a pro-inflammatory Th2-response is generated (34) (Figures 1A,B). Because hRSV infection does not produce an effective memory response that confers protective immunity to subsequent viral exposure, re-infections are very frequent which lead to hyperreactivity, recurrent wheezing and an increased susceptibility of developing asthma (35).
Clinical Manifestations of HRSV Infection
Clinical manifestations of LRTI caused by hRSV might vary depending on the individual's co-morbidity, age or sex, air pollution exposure, parental asthma history or previous infections, among others (2, 36). HRSV-LRTI might be accompanied by nasal congestion, rhinorrhea, cough, wheezing and shortness of breath (36, 37), with an increased risk of subsequent wheezing episodes that can last for several years after acute infection. Indeed, pathology induced in the airways by respiratory viruses is characterized by alterations in the respiratory epithelium, which stimulates the production of pro-inflammatory cytokines and chemokines that promote the infiltration of immune cells into the lungs (38, 39). In some cases, this response might become exacerbated and bring temporary or lifetime changes in the lungs, leading to the recurrent wheezing episodes and asthma (3, 40). Although most viral infections induce a transient airway hyperresponsiveness (41, 42), those with a history of atopy or asthma might display enhanced virus-related inflammation with significant airway obstruction leading to a more severe disease (43, 44). Therefore, the identification of hRSV-infected patients susceptible to develop more severe diseases would be important for performing better clinical decisions.
Diagnosis of HRSV Infection
Early clinical diagnosis of hRSV infection could help to improve the care management of patients with respiratory infections and anticipate severe outcomes, according to the clinical predisposing factors, such as age. Currently, the available methods for hRSV diagnosis include tests that are based on molecular, virologic, or immunologic diagnostic.
Nowadays, the most used methods for hRSV diagnosis are based on direct immunofluorescence (DIF), reverse transcriptase-PCR (RT-PCR), immunochromatographic assay (CIA) and enzyme immune-assay (EIA). Other more complex methodologies that have been used more frequently in the last years are based in the detection of multiple analytes in high-complexity multiplex assays (such as Luminex or Affimetrix), as these approaches are faster than viral culture (11, 45, 46). Some molecular assays, such as RT-PCR and Luminex have high diagnostic sensitivity as compared to cell culture technique, but only RT-PCR is used as reference technique (47). Although RT-PCR is the fastest, its implementation is expensive as compared to DIF, EIA, or CIA assays. However, while the latter are low cost and fast, their sensitivity is lower than that of RT-PCR or Luminex and, in some cases (i.e., DIF), the interpretation of the results is somewhat subjective and requires technical skills, time, and expertise (47).
Immunologic diagnosis of hRSV is based on the characterization of cellular and cytokine/chemokine profiles (48, 49). In this case, flow cytometry is the main technique used to identify the cell types present in the bronchoalveolar lavage fluid (BALF) and peripheric blood samples of patients with hRSV infection. Cells recruited to the lungs include neutrophils, dendritic cells, T and B cells, alveolar macrophages and monocytes (10, 35). Clinical studies with hRSV infected children have shown an increased amount of neutrophils (CD11b+, CD18+, and CD54+) (50), alveolar macrophages (expressing TNF-α) (51), monocytes (CD69+) (52) and B cells (53) infiltrating the infected airways. Contrarily, the presence of T cells (CD4+ and CD8+) and plasmacytoid dendritic cells (DCs, HLA-DR+, CD123+/CD11c−) significantly decrease in peripheral blood of infected children, as compared to healthy children control groups (54, 55). Besides the characterization of the cells infiltrating the airways, the cytokines/chemokine profile observed in the infected tissue is also informative. The main cytokines evaluated in the BALFs of hRSV infected individuals are mainly IL-2, IL-12, IFN-γ, IL-8, IL-6, and TNF-α (35). Importantly, all these cytokines can be evaluated by flow cytometry, ELISA, RT-PCR, or Luminex (56–58).
The types of samples used to detect hRSV or immune-related markers can be nasal washes, nasopharyngeal aspirates (NPA), nasopharyngeal swabs, BALFs, serum and peripheral blood (11, 45, 46). However, cytokines as biomarkers should be assessed at the site of infection (upper and lower respiratory tract) and to a lower extent in peripheric blood. The role of the above-mentioned cytokines during infection is discussed below in the following sections.
Severity Prognosis in HRSV Infection
Among the patients diagnosed with LRTI, a significant number of hRSV-infected children treated as outpatients will require additional medical attention due to respiratory complications. Furthermore, a significant percentage of diagnosed patients will display recurring wheezing episodes and other complications in the following months after the first LRTI episode (59, 60). It is worth mentioning that these patients can not be identified early after infection due to a lack of accurate tools for predicting disease severity. Furthermore, at present there is no consensus on predicting the outcome of patients with LRTI caused by hRSV, which represents a problem for disease management due to the rapid evolution of the disease in which mechanical ventilation might be unexpectedly required in the course of 24 hours or less (61). Currently, methods that are based on clinical parameters used by physicians are widely accepted to support clinical decisions (62). However, these parameters may be somewhat subjective and are not accurate enough to perform a precise categorization or prognosis of disease severity (63, 64). To address this problem, biomarkers within samples of patients might contribute to a better diagnosis and could help physicians take more accurate decisions, increasing the possibility of obtaining better outcomes (4, 65). In line with this notion, in the last years several research groups have focused on identifying an accurate method for determining the severity and progression of LRTI by hRSV (62, 66–68). Below, we describe diverse parameters and soluble molecules currently used to assess disease severity in hRSV-infected patients (Table 1).
Clinical Score as a Biomarker Related to Disease Severity
The use of prediction models to calculate the risk of severe outcome in LRTI in children has been previously implemented based on the clinical characteristics of patients, radiological findings, and laboratory results (77). In the last 10 years, remarkable progress in diagnostics has been achieved thanks to the availability of transcriptional profiles that have allowed establishing fingerprints related to disease progression and severity caused by hRSV infection (78). Among the available methods based on transcriptomic approaches, the “molecular distance to health” (MDTH) has shown to be a promising diagnostic tool for respiratory tract infections (68, 79). The MDTH is a tool designed to measure alterations in the transcriptional profile of immune cells (i.e., neutrophils, cytotoxic cells, and T-cells) obtained from patients (80). Data is obtained from the test as a single score that is compared with a basal score from healthy controls. Importantly, MDTH scores performed during the first days of hRSV infection have been able to predict disease severity in terms of hospitalization days and intensive care requirements (78).
Microbial Factors as Severity Biomarkers
It is well known that the higher microbial load at the site of infection, the greater the possibility to cause tissue damage, which is related to worse prognosis. Based on this premise, several research groups have tried to demonstrate a direct relationship between viral loads and the severity of the disease (81), but the conclusions are somewhat controversial. Different studies have shown a direct correlation between the increase of viral loads with more severe clinical manifestations (81–83). In fact, these studies showed that high hRSV viral loads at day 3 are significantly associated with requirement for intensive care and respiratory failure (84). In contrast, studies, such as (69, 85) and Piedra et al. have reported the opposite, where high hRSV loads at the beginning of the infection correlate with protective immune response and less severe disease progression (86). These findings raise the discussion about the role of viral loads in disease progression and the possibility of considering this factor as a potential biomarker to determine disease severity in hRSV-LRTI, as viral loads could be leading the host immune response to the virus.
Soluble Proteins as Biomarkers for Disease Severity
In the last few years, the analysis of protein expression patterns has become one of the most explored fields in diagnosis. The samples used to obtain the protein expression patterns range from blood to nasopharyngeal samples, with both suggesting helpful insights into the identification of molecules related to the severity of the infection. For example, increased levels of serum transaminases, aminotransferases and antidiuretic hormones have been related to severe cases of hRSV bronchiolitis (70, 71). Furthermore, increased levels of lactate dehydrogenase (LDH) in nasopharyngeal samples has also shown to have a predictive value of 88% in determining the severity of the disease in young children with bronchiolitis (72, 73). Another molecule proposed as a disease severity biomarker is mucin 5AC (MUC5AC), a highly glycosylated protein present in the airway mucus (74). This protein has been reported to be detected in nasal aspirates obtained from hRSV-infected children and its presence and concentration is correlated to disease severity caused by hRSV (75). Taken together, several soluble molecules show a correlation with the severity of hRSV-related disease and can be easily detected in samples that are simple to obtain, and thus may be used as biomarkers of disease severity related to infections caused by hRSV.
Pro-inflammatory Cytokines as Biomarkers for Disease Severity in hRSV Infections
During hRSV infection, the host innate immune response generated against the virus can be unbalanced and ultimately detrimental to the host. Non-optimal responses against the virus are Th2-like responses with the generation of cytokines, which in turn can recruit numerous pro-inflammatory immune cells (35, 56). Furthermore, several studies have reported an increase in the levels of Th2-like cytokines in different types of samples (BALF, serum, blood, plasma, nasopharyngeal, or aspirate washes), which can be correlated with disease severity in children. Such cytokines, which could be used as prognosis biomarkers are IL-33, IL-8, TSLP, IL-6, periostin, and IFN-α. Those biomarkers could predict hRSV disease severity in children (Table 2). Other cytokines, such as IL-12, IL-3, and IL-13 could also be potential biomarkers, although more clinical studies are required (Table 3). Next, we will explain further how some of these cytokines could be useful to predict the severity of hRSV infection.
Interleukin-33 (IL-33)
IL-33 is constitutively expressed by endothelial and epithelial cells. The main function of this cytokine is the initiation and development of the innate and adaptive Th2 type immune response (103). Cells expressing the ST2 receptor respond to IL-33, including mast cells, eosinophils, and basophils, among others (104). Type-2 innate lymphoid cells (ILC2s) are also targeted by IL-33 to produce Th2-type cytokines (IL-6, IL-8, IL-5, IL-13), which in turn promote a Th2 response with eosinophil recruitment, generating an exacerbated disease (105) (Figure 1B). Recent studies with mice in which IL-33 was neutralized during hRSV infection, showed that severe pathology was not induced and that mice treated with IL-33 during hRSV infection quickly developed the disease, resulting in more severe clinical outcome (35, 88). Interestingly, Saravia et al. measured IL-33 levels in NPA and showed a link with ventilation requirement in infants hospitalized by bronchiolitis caused by hRSV (87). In 2015, Bertrand et al. performed a study in children with bronchiolitis caused by hRSV and detected high levels of IL-33 expression levels in NPA in patients with a family history of atopy (66). García-García et al. measured IL-33 levels from NPA in children infected with hRSV, associating bronchiolitis with high levels of this cytokine. Furthermore, both studies describe that IL-33 cytokine is elevated when coinfection occurred (88). Taken together, these results indicate that IL-33 could be a good biomarker to determine the severity and prognosis during bronchiolitis caused by hRSV.
Interleukin-8 (IL-8)
IL-8 has a mayor chemotactic role, and is mainly produced by monocytes, endothelial cells, macrophages, and T cells (106, 107). IL-8 binds to G protein-coupled receptors CXCR1 and CXCR2 expressed by cells that include monocytes, neutrophils, endothelial cells, macrophages, and T cells, among others (108, 109) (Figure 1B). During an infection with hRSV, McNamara et al. found that the concentration of IL-8 remains elevated during the disease, even when the number and recruitment of neutrophils ultimately decreased (110). Elevated IL-8 levels (in nasopharyngeal samples) have been widely correlated with disease severity caused by hRSV infection, including the risk of mechanical ventilation (4, 90). In 2013, Díaz et al. found high IL-8 levels in NPA in children with severe hRSV bronchiolitis as compared to controls and patients with mild disease manifestations. More specifically, they observed an increase in IL-8 in a group of patients with severe disease (111), which may suggest that higher levels of this cytokine relate to higher severity of hRSV infection. Tabarani et al. identified in nasopharyngeal washes increased levels of IL-6, IL-8, and TNF-α associated to hRSV disease severity in young children (89). In this study, the authors associated the severity of disease with the age of the individuals, chronic diseases and elevated concentrations of IL-8, as well as other molecules (89). In another study, which was performed in children with severe hRSV infection, Brand et al. assessed the levels of IL-8 in plasma and NPA and found an increase in IL-8 in the plasma of children with severe disease, as compared to children with mild or moderate disease (48). In 2015, Díaz et al. performed another study in children with bronchiolitis caused by hRSV and Rhinovirus (RV). This study showed higher IL-8 levels in NPA of children infected with both, hRSV and RV than children infected with RV alone, which was associated with more days requiring O2 treatment (92). Based on this study, it can be suggested that high IL-8 levels in children infected hRSV will act as a good predictor for determining the days that requiring O2 treatment. In 2016, Huang et al. performed a clinical study that included 96 patients with asthma-chronic obstructive pulmonary disease (COPD) and 35 healthy controls. Their results showed an increment of IL-8 and other cytokine levels that were related to the severity of airway diseases. The researchers suggest that IL-8 could be a potential marker for the evaluation of asthma and COPD (91). There are not new clinical studies that correlate high levels of this cytokine with the disease severity.
Thymic Stromal Lymphopoietin (TSLP)
TSLP is expressed by several cell types, but mainly by epithelial cells and keratinocytes (112, 113). Two isoforms have been described for this cytokine: a long and a short form of TSLP (114). The short isoform is constitutively expressed in several tissues, particularly in those that are highly sensitive to inflammation. Importantly, the long isoform of TSLP has been widely correlated with exacerbated immune responses and the establishment of allergic and asthma in patients with atopic dermatitis (95) (Figure 1B). Asthma may result as a consequence of different factors in children. However, a possible association with viral infections has gained increased attention of researchers in the last decade (88). At present, there is increasing evidence suggesting an association between TSLP elicited upon infection with hRSV or RV and the development of asthma (88, 94). However, it still remains to be elucidated whether asthma favors severe viral disease or if asthma is the result of severe disease elicited during respiratory infection. Lee et al. reported that viral antigen recognition triggers a signaling cascade involving the NF-κB nuclear factor and retinoic acid induced gene 1 (RIG-1) (115). The activation of this cascade resulted in TSLP production and a strong Th2 response, contributing to the pathophysiology observed in severe bronchiolitis, which eventually in some cases progressed to asthma (115). Later, García-García et al. showed an association between TSLP, together with periostin and IL-33, with disease severity in the infection of the respiratory tract of children. This study showed a correlation between increased levels of TSLP with hRSV bronchiolitis and coinfections with rhinovirus, as well as with severe disease and intensive care unit (ICU) admission (88).
Interleukin-6 (IL-6)
IL-6 is a soluble mediator that can be produced by macrophages and epithelial cells (116). After its synthesis, IL-6 moves to the liver through the bloodstream and generates a pleiotropic effect over immunity and inflammation (117). This cytokine is involved in the promotion of the differentiation of naïve CD4+ and CD8+ T cells and is an important link between innate and acquired immunity (117) (Figure 1B). In 2013, Tabarani et al. evaluated the levels of IL-6 in nasopharyngeal wash samples from children with LRTI and hRSV. Interestingly, they found a correlation between the magnitude of the clinical manifestations elicited by hRSV infection and high levels of IL-6 amongst other inflammatory mediators (CCL2, TNF-α, CXCL8, IL-10) (89). On the other hand, Brown et al. have suggested that high levels of IL-6 in the plasma could indicate a higher probability of infant hospitalization and severe bronchiolitis caused by hRSV (4). In 2016, Lu et al. also detected high levels of IL-6 in NPA of patients with hRSV and this was correlated with higher hRSV disease severity (100). Increased levels of IL-6 and other cytokines have also been found in nasal lavage fluids of children with LRTI, particularly those which needed O2 treatment (35, 118). Other studies performed in children with bronchiolitis caused by hRSV infection showed that high IL-6 levels in nasal samples and BALF correlated with the need for ventilation and with a higher degree of hypoxia (35, 92). In this study, the authors suggested that IL-6 and other cytokines assessed could be reliable biomarkers to determine the severity of hRSV infection.
Periostin
Periostin is a protein that is expressed at basal levels in almost all human tissues (119). Its expression is also found in the respiratory epithelium and is elevated levels in asthmatic children (120). This protein is produced by eosinophils in response to IL-4 an IL-13 signaling (121) (Figure 1B). The role of periostin is related to the generation of allergic inflammation and the development of a Th2 phenotype, among others (120) (Figure 1B). Periostin has been associated with asthma severity and increased levels of periostin have been found in the serum of children with exacerbated manifestations of asthma (122). Lopez-Guisa and colleagues evaluated periostin levels in bronchial and nasal cells from asthmatic, non-asthmatic, atopic, and healthy children and found a significant increase in periostin levels in asthmatic children (3.7 times), as compared to the other groups (96). These results were confirmed in studies that showed a correlation between high levels of periostin in the serum with persistent or uncontrolled asthma in children (97, 98). In fact, clinical manifestations of asthma are considered to be very similar to bronchiolitis symptoms (123). These findings suggest that asthma could be a sequel of severe bronchiolitis in children (123). García-García et al. showed in NPAs that increased concentrations of periostin were associated with more severe hRSV infection, as compared to healthy children (88, 93). More recently, periostin levels were associated with the severity of viral bronchiolitis, as children with severe pulmonary hypertension had high levels of this protein as compared to children with mild pulmonary hypertension (8,887 ± 1,582 pg/ml vs. 5,016 ± 1,017 pg/ml) (99). These results indicate that periostin could be another good biomarker for the prognosis of hRSV infection and particularly bronchiolitis.
Interferon Alpha (IFN-α)
IFNs are a large family of pleiotropic cytokines. Particularly, IFN-α and IFN-β are type-I interferon family members produced by epithelial cells and most of immune cells (124). To exert its biological action, type-I IFNs binds to the type-I IFN receptor (IFNAR1/2) (125), which triggers the expression of pro-inflammatory molecules and antiviral genes, such as those involved in the degradation of viral RNA (126). Importantly, the recognition of the hRSV non-structural protein 1 (NS1) has been correlated with impaired IFN-α function, particularly through the induction of the miRNA miR-29a, which inhibits the expression of the IFN-α receptor in infected cells (101). These studies suggest that low levels of IFN-α could be related to the severity of hRSV infection and hence could be used as a biomarker. However, other studies based on the transcriptional profile of blood samples and nasopharyngeal swabs, report contrasting results, indicating that type-I interferons, particularly IFN-α/β are increased in hRSV bronchiolitis and correlate with severe illness and recurrent wheezing (89, 102). These studies suggest that interferon signaling pathways may serve as important biomarkers associated to hRSV loads and severity (102). Resolving the discrepancies found among different studies analyzing the role of IFN-α in hRSV disease severity will require further investigations that ideally relate transcriptional findings with protein levels in blood and nasopharyngeal samples.
Other Potencial Pro-Inflammatory Cytokines as Biomarkers for Severity Caused by HRSV
Besides the cytokines described above as potential biomarkers for hRSV severity (4), recent studies have preliminarily pointed out other pro-inflammatory cytokines that show positive correlations with hRSV severity and are potential prognosis biomarkers for respiratory diseases (Table 3). Some of these cytokines are described below.
Interleukin-12 (IL-12)
IL-12 is produced in response to viral or bacterial infections by DCs and other antigen-presenting cells and is involved in promoting naïve T cell differentiation into Th1 T cells (127) (Figure 1B). Bertrand et al. have shown that nasal and lung samples display increased levels of IL-12 in LRTI patients. Furthermore, they showed for first time that high levels of IL-12p40 (in BALF) and other cytokine could be correlated with recurrent wheezing and the development of asthma in infants with bronchiolitis caused by hRSV infection (35, 66).
Interleukin-3 (IL-3)
IL-3 is mainly expressed by mast cells and activated T cells located in the airways (128). This cytokine induces an increase in basophil and eosinophil production (129) (Figure 1B) and is involved in the pathogenesis of asthma (128). In 2015, Bertrand et al. described for the first-time the presence of high levels of IL-3 in BALF and NPA obtained from children < 9 months with acute bronchiolitis caused by hRSV. Furthermore, the authors found a correlation between high levels of IL-3 with episodes of recurring wheezing and the development of asthma in the future (66). Lu et al. also found high levels of IL-3 in NPA in children with bronchiolitis caused by hRSV and an increased risk of asthma, which was associated with higher disease severity (100). The results of this study suggest that IL-3 could be involved in the development of chronic airway inflammatory diseases and that it could be used to predict clinical outcomes in hRSV-LRTI. Consistently, the authors suggested that IL-3 could be eventually used to predict the clinical outcome of patients.
Interleukin-13 (IL-13)
In the lungs, IL-13 is the mediator of eosinophilic inflammation, mucosal secretion, and bronchial hyper reactivity (130). It has been observed that IL-13 is elevated in COPD, as well as in asthma and other lung diseases (131). Importantly, IL-13 is produced in response to IL-33 signaling and is released from various cells, including alveolar macrophages, basophils, mast cells, eosinophils, ILC2 and CD4+ T cells (132) (Figure 1B). In 2015, Saravia et al. linked high levels of IL-13 and IL-33 with the requirement for ventilation in infants hospitalized with bronchiolitis caused by hRSV (87). Consistently, in an animal model of hRSV (BALB/c mice), an up-regulation of IL-13 has been reported, which results in the recruitment of eosinophils to the airways that generates exacerbated mucus production, lung hyperreactivity and airway inflammation (132). A more recent study performed in 2016 evaluated IL-13 levels in the blood of children being treated for respiratory symptoms following severe hRSV bronchiolitis and found that IL-13 could be used as a clinical asthma diagnosis marker (100).
Concluding Remarks
Biomarkers for classifying the severity of respiratory tract infections have become a global need due to the lack of effective strategies to decrease the impact of such diseases and the need for improving the management of patients and their potential outcomes. Most efforts point to the development of highly sensitive, rapid, and low-cost techniques that allow predicting in an accurate way the prognosis of patients with respiratory infections. Nowadays, an important number of molecules have been identified which could help asses disease severity, however their specificity and sensitivity remain challenging and are not strong enough yet to accurately predict disease outcome and become a canonic biomarker for predicting LRTI severity associated to hRSV. Hence, more studies are needed to establish the pro-inflammatory cytokine and cytokine expression patterns that are related to disease development during the different stages of hRSV infection. Ideally, particular pro-inflammatory cytokine profiles will ultimately allow determining early on during infection the severity of disease caused by hRSV.
Author Contributions
YV, LG, and LN are responsible for the writing of this review article. PG, CR, and PB are responsible for reviewing the article and SB is the leading investigator and assisted in the organization and revision of the manuscript.
Funding
This review was supported by grant numbers 1170964, 1190864 and 1161525 from the National Fund for Scientific and Technological Development (FONDECYT) program of the Ministry of Education of Chile; Grant 13CTI-21526 from the INNOVA-CORFO program of the Chilean Ministry of Economy and Millennium Institute on Immunology and Immunotherapy, grant P09/016-F.
Conflict of Interest Statement
The authors declare that the research was conducted in the absence of any commercial or financial relationships that could be construed as a potential conflict of interest.
Acknowledgments
We thank the Pediatric Division and School of Medicine from Pontificia Universidad Católica de Chile for the help and the clínical samples. We are grateful to Trinidad Celis (Pontificia Universidad Católica de Chile) who generated the graphic art.
Abbreviations
hRSV, Human respiratory syncytial virus; LRTI, Lower Respiratory Tract Infection; TSLP, Thymic Stromal Lymphopoietin; IL, Interleukin; IFN, Interferon; BALF, Bronchoalveolar lavage fluid; NPA, Nasopharyngeal aspirate; TLR, Toll-like receptors; AECs, Airway epithelial cells; NF-κB, Nuclear factor κB.
References
1. Simões EA. Treatment and prevention of respiratory syncytial virus lower respiratory tract infection: long-term effects on respiratory outcomes. Am J Respir Crit Care Med. (2001) 163:S14–7. doi: 10.1164/ajrccm.163.supplement_1.2011112
2. Mazur NI, Bont L, Cohen AL, Cohen C, Von Gottberg A, Groome MJ, et al. Severity of respiratory syncytial virus lower respiratory tract infection with viral coinfection in HIV-uninfected children. Clin Infect Dis. (2016) 64:443–50. doi: 10.1093/cid/ciw756
3. Zhong Q, Feng H, Lu Q, Liu X, Zhao Q, Du Y, et al. Recurrent wheezing in neonatal pneumonia is associated with combined infection with Respiratory Syncytial Virus and Staphylococcus aureus or Klebsiella pneumoniae. Sci Rep. (2018) 8:995. doi: 10.1038/s41598-018-19386-y
4. Brown PM, Schneeberger DL, Piedimonte G. Biomarkers of respiratory syncytial virus (RSV) infection: specific neutrophil and cytokine levels provide increased accuracy in predicting disease severity. Paediat Respir Rev. (2015) 16:232–40. doi: 10.1016/j.prrv.2015.05.005
5. Glezen WP, Taber LH, Frank AL, Kasel JA. Risk of primary infection and reinfection with respiratory syncytial virus. Am J Dis Children. (1986) 140:543–6. doi: 10.1001/archpedi.1986.02140200053026
6. Smyth RL, Peter JM, Openshaw. Bronchiolitis. Lancet. (2006) 368:312–322. doi: 10.1016/S0140-6736(06)69077-6
7. Matsumoto K, Inoue H. Viral infections in asthma and COPD. Respir Investig. (2014) 52:92–100. doi: 10.1016/j.resinv.2013.08.005
8. Kokturk N, Bozdayi G, Yilmaz S, Dogan B, Gulbahar O, Rota S, et al. Detection of adenovirus and respiratory syncytial virus in patients with chronic obstructive pulmonary disease: exacerbation versus stable condition. Mol. Med. Rep.(2015) 12:3039–46. doi: 10.3892/mmr.2015.3681
9. Kim HW, Canchola JG, Brandt CD, Pyles G, Chanock RM, Jensen K, et al. Respiratory syncytial virus disease in infants despite prior administration of antigenic inactivated vaccine. Am. J. Epidemiol. (1969) 89:422–34. doi: 10.1093/oxfordjournals.aje.a120955
10. Rey-Jurado E, Soto J, Gálvez N, Kalergis AM. A safe and efficient BCG vectored vaccine to prevent the disease caused by the human respiratory syncytial virus. Hum Vacc Immunotherapeutics. (2017) 13:2092–7. doi: 10.1080/21645515.2017.1334026
11. Griffiths C, Drews SJ, Marchant DJ. Respiratory syncytial virus: infection, detection, and new options for prevention and treatment. Clin. Microbiol. Rev. (2017) 30:277–319. doi: 10.1128/CMR.00010-16
12. Mazur NI, Higgins D, Nunes MC, Melero JA, Langedijk AC, Horsley N, et al. The respiratory syncytial virus vaccine landscape: lessons from the graveyard and promising candidates. Lancet Infect. Dis. (2018) 18:e295–311. doi: 10.1016/S1473-3099(18)30292-5
13. Touch SM, Spitzer AR. Palivizumab, a humanized respiratory syncytial virus monoclonal antibody, reduces hospitalization from respiratory syncytial virus infection in high-risk infants. Clin. Pediatr. (Phila). (1999) 38:556.
14. Resch B. Product review on the monoclonal antibody palivizumab for prevention of respiratory syncytial virus infection. Hum Vacc Immunotherapeutics. (2017) 13:2138–49. doi: 10.1080/21645515.2017.1337614
15. Simões EA, Bont L, Manzoni P, Fauroux B, Paes B, Figueras-Aloy J, et al. Past, present and future approaches to the prevention and treatment of respiratory syncytial virus infection in children. Infect Dis Ther. (2018) 7:87–120. doi: 10.1007/s40121-018-0188-z
16. O'Brien KL, Chandran A, Weatherholtz R, Jafri HS, Griffin MP, Bellamy T, et al. Efficacy of motavizumab for the prevention of respiratory syncytial virus disease in healthy Native American infants: a phase 3 randomised double-blind placebo-controlled trial. Lancet Infect. Dis. (2015) 15:1398–408. doi: 10.1016/S1473-3099(15)00247-9
17. World Health Organization. Meeting of the strategic advisory group of experts on immunization, april 2014—conclusions and recommendations. Weekly Epidemiol Record. (2014) 89:221–236.
18. WHO. WHO Informal Consultation on Surveillance of Respiratory Syncytial Virus on the WHO Global Influenza Surveillance and Response System (GISRS) Platform. Geneva, Switzerland. (2016) p. 25–27.
19. Chanock R, Roizman B, Myers R. Recovery from infants with respiratory illness of a virus related to chimpanzee coryza agent (CCA). Am J Epidemiol. (1957) 66:281–90. doi: 10.1093/oxfordjournals.aje.a119901
20. Muñoz-Durango N, Pizarro-Ortega MS, Rey-Jurado E, Díaz FE, Bueno SM, Kalergis AM. Patterns of antibody response during natural RSV infection: insights for the development of new antibody-based therapies. Exp Opin Investig Drugs. (2018) 27:721–31. doi: 10.1080/13543784.2018.1511699
21. Grosfeld H, Hill MG, Collins PL. RNA replication by respiratory syncytial virus (RSV) is directed by the N, P, and L proteins; transcription also occurs under these conditions but requires RSV superinfection for efficient synthesis of full-length mRNA. J Virol. (1995) 69:5677–86.
22. González PJ, Carreno LM, Bueno SA, Riedel CA, Kalergis AM. Understanding respiratory syncytial virus infection to improve treatment and immunity. Curr Mol Med. (2013) 13:1122–39. doi: 10.2174/1566524011313070007
23. Vandini S, Calamelli E, Faldella G, Lanari M. Immune and inflammatory response in bronchiolitis due to respiratory Syncytial Virus and Rhinovirus infections in infants. Paediatr Respir Rev. (2017) 24:60–4. doi: 10.1016/j.prrv.2016.11.006
24. Sullender WM. Respiratory syncytial virus genetic and antigenic diversity. Clin Microbiol Rev. (2000) 13:1–15. doi: 10.1128/CMR.13.1.1
25. Nolan T, Borja-Tabora C, Lopez P, Weckx L, Ulloa-Gutierrez R, Lazcano-Ponce E, et al. Prevalence and incidence of respiratory syncytial virus and other respiratory viral infections in children aged 6 months to 10 years with influenza-like illness enrolled in a randomized trial. Clin Infect Dis. (2015) 60:e80–9. doi: 10.1093/cid/civ065
26. Eiland LS. Respiratory syncytial virus: diagnosis, treatment and prevention. J Pediatric Pharmacol Therape. (2009) 14:75–85. doi: 10.5863/1551-6776-14.2.75
27. Bueno SM, González PA, Cautivo KM, Mora JE, Leiva ED, Tobar HE, et al. Protective T cell immunity against respiratory syncytial virus is efficiently induced by recombinant BCG. Proc Natl Acad Sci USA. (2008) 105:20822–7. doi: 10.1073/pnas.0806244105
28. Taleb SA, Al Thani AA, Al Ansari K, Yassine HM. Human respiratory syncytial virus: pathogenesis, immune responses, and current vaccine approaches. Eur J Clin Microbiol Infect Dis. (2018) 37:1817–27. doi: 10.1007/s10096-018-3289-4
29. Kristjansson S, Bjarnarson SP, Wennergren G, Palsdottir AH, Arnadottir T, Haraldsson A, et al. Respiratory syncytial virus and other respiratory viruses during the first 3 months of life promote a local TH2-like response. J Allergy Clin Immunol. (2005) 116:805–11. doi: 10.1016/j.jaci.2005.07.012
30. Feng S, Zeng D, Zheng J, Zhao D. microRNAs: mediators and therapeutic targets to airway hyper reactivity after respiratory syncytial virus infection. Front Microbiol. (2018) 9:02177. doi: 10.3389/fmicb.2018.02177
31. Lay MK, Bueno SM, Gálvez N, Riedel CA, Kalergis AM. New insights on the viral and host factors contributing to the airway pathogenesis caused by the respiratory syncytial virus. Crit Rev Microbiol. (2016) 42:800–12. doi: 10.3109/1040841X.2015.1055711
32. Jartti T, Smits HH, Bonnelykke K, Cavkaytar O, Elenius V, Konradsen JR, et al. Bronchiolitis needs a revisit: distinguishing between virus entities and their treatments. Allergy. (2018) 74:40–52. doi: 10.1111/all.13624
33. Schmidt ME, Varga SM. The CD8 T cell response to respiratory virus infections. Front Immunol. (2018) 9:678. doi: 10.3389/fimmu.2018.00678
34. Aoyagi M, Shimojo N, Sekine K, Nishimuta T, Kohno Y. Respiratory syncytial virus infection suppresses IFN-γ production of γδ T cells. Clin Exp Immunol. (2003) 131:312–7. doi: 10.1046/j.1365-2249.2003.02062.x
35. Russell CD, Unger SA, Walton M, Schwarze J. The human immune response to respiratory syncytial virus infection. Clin Microbiol Rev. (2017) 30:481–502. doi: 10.1128/CMR.00090-16
36. Wright M, Piedimonte G. Respiratory syncytial virus prevention and therapy: past, present, and future. Pediatr Pulmonol. (2011) 46:324–47. doi: 10.1002/ppul.21377
37. Mohapatra SS, Boyapalle S. Epidemiologic, experimental, and clinical links between respiratory syncytial virus infection and asthma. Clin Microbiol Rev. (2008) 21:495–504. doi: 10.1128/CMR.00054-07
38. Giuffrida MJ, Valero N, Mosquera J, Alvarez de Mon M, Chacín B, Espina LM, et al. Increased cytokine/chemokines in serum from asthmatic and non-asthmatic patients with viral respiratory infection. Influenza Other Respi Viruses. (2014) 8:116–22. doi: 10.1111/irv.12155
39. Newton AH, Cardani A, Braciale TJ. The host immune response in respiratory virus infection: balancing virus clearance and immunopathology. In: Hussell T, Grabiec AM, editors. Seminars in Immunopathology. Berlin: Springer (2016) p. 471–82.
40. Jartti T, Gern JE. Role of viral infections in the development and exacerbation of asthma in children. J Allergy Clin Immunol. (2017) 140:895–906. doi: 10.1016/j.jaci.2017.08.003
41. Bønnelykke K, Vissing NH, Sevelsted A, Johnston SL, Bisgaard H. Association between respiratory infections in early life and later asthma is independent of virus type. J Allergy Clin Immunol. (2015) 136:81–6. doi: 10.1016/j.jaci.2015.02.024
42. Tekkanat KK, Maassab HF, Cho DS, Lai JJ, John A, Berlin A, et al. IL-13-induced airway hyperreactivity during respiratory syncytial virus infection is STAT6 dependent. J Immunol. (2018) 166:3542–8. doi: 10.4049/jimmunol.166.5.3542
43. Wu P, Hartert TV. Evidence for a causal relationship between respiratory syncytial virus infection and asthma. Exp Rev Anti Infect Ther. (2011) 9:731–45. doi: 10.1586/eri.11.92
44. Knudson CJ, Varga SM. The relationship between respiratory syncytial virus and asthma. Vet Pathol. (2015) 52:97–106. doi: 10.1177/0300985814520639
45. Chartrand C, Tremblay N, Renaud C, Papenburg J. Diagnostic accuracy of rapid antigen detection tests for respiratory syncytial virus infection: systematic review and meta-analysis. J Clin Microbiol. (2015) 53:3738–49. doi: 10.1128/JCM.01816-15
46. González LA, Vázquez Y, Mora JE, Palavecino CE, Bertrand P, Ferrés M, et al. Evaluation of monoclonal antibodies that detect conserved proteins from Respiratory Syncytial Virus, Metapneumovirus and Adenovirus in human samples. J Virol Methods. (2018) 254:51–64. doi: 10.1016/j.jviromet.2018.01.011
47. Aslanzadeh J, Zheng X, Li H, Tetreault J, Ratkiewicz I, Meng S, et al. Prospective evaluation of rapid antigen tests for diagnosis of respiratory syncytial virus and human metapneumovirus infections. J Clin Microbiol. (2008) 46:1682–5. doi: 10.1128/JCM.00008-08
48. Brand HK, Ferwerda G, Preijers F, de Groot R, Neeleman C, Staal FJ, et al. CD4+ T-cell counts and interleukin-8 and CCL-5 plasma concentrations discriminate disease severity in children with RSV infection. Pediatr Res. (2013) 73:187. doi: 10.1038/pr.2012.163
49. Raiden S, Sananez I, Remes-Lenicov F, Pandolfi J, Romero C, De Lillo L, et al. Respiratory syncytial virus (RSV) infects CD4+ T cells: frequency of circulating CD4+ RSV+ T cells as a marker of disease severity in young children. J Infect Dis. (2017) 215:1049–58. doi: 10.1093/infdis/jix070
50. Everard ML, Swarbrick A, Wrightham M, McIntyre J, Dunkley C, James PD, et al. Analysis of cells obtained by bronchial lavage of infants with respiratory syncytial virus infection. Arch Dis Child. (1994) 71:428–32. doi: 10.1136/adc.71.5.428
51. Midulla F, Villani A, Panuska JR, Dab I, Kolls JK, Merolla R, et al. Respiratory syncytial virus lung infection in infants: immunoregulatory role of infected alveolar macrophages. J Infect Dis. (1993) 168:1515–9. doi: 10.1093/infdis/168.6.1515
52. Johnson JE, Gonzales RA, Olson SJ, Wright PF, Graham BS. The histopathology of fatal untreated human respiratory syncytial virus infection. Modern Pathol. (2007) 20:108. doi: 10.1038/modpathol.3800725
53. Román M, Calhoun WJ, Hinton KL, Avendaño LF, Simon V, Escobar AM, et al. Respiratory syncytial virus infection in infants is associated with predominant Th-2-like response. Am J Respir Crit Care Med. (1997) 156:190–5. doi: 10.1164/ajrccm.156.1.9611050
54. O'Donnell DR, Carrington D. Peripheral blood lymphopenia and neutrophilia in children with severe respiratory syncytial virus disease. Pediatr Pulmonol. (2002) 34:128–30. doi: 10.1002/ppul.10140
55. Weng K, Zhang J, Mei X, Wu A, Zhang B, Cai M, et al. Lower number of plasmacytoid dendritic cells in peripheral blood of children with bronchiolitis following respiratory syncytial virus infection. Influenza Other Respi Viruses. (2014) 8:469–73. doi: 10.1111/irv.12242
56. Ugonna K, Douros K, Bingle CD, Everard ML. Cytokine responses in primary and secondary respiratory syncytial virus infections. Pediatr Res. (2016) 79:946. doi: 10.1038/pr.2016.29
57. Da Eun Roh SHP, Choi HJ, Kim YH. Comparison of cytokine expression profiles in infants with a rhinovirus induced lower respiratory tract infection with or without wheezing: a comparison with respiratory syncytial virus. Korean J Pediatr. (2017) 60:296. doi: 10.3345/kjp.2017.60.9.296
58. Fan R, Wen B, Liu W, Zhang J, Liu C, Fan C, et al. Altered regulatory cytokine profiles in cases of pediatric respiratory syncytial virus infection. Cytokine. (2018) 103:57–62. doi: 10.1016/j.cyto.2017.12.028
59. Valkonen H, Waris M, Ruohola A, Ruuskanen O, Heikkinen T. Recurrent wheezing after respiratory syncytial virus or non-respiratory syncytial virus bronchiolitis in infancy: a 3-year follow-up. Allergy. (2009) 64:1359–65. doi: 10.1111/j.1398-9995.2009.02022.x
60. Blanken MO, Rovers MM, Molenaar JM, Winkler-Seinstra PL, Meijer A, Kimpen JL, et al. Respiratory syncytial virus and recurrent wheeze in healthy preterm infants. N Engl J Med. (2013) 368:1791–9. doi: 10.1056/NEJMoa1211917
61. Bradley JS, Byington CL, Shah SS, Alverson B, Carter ER, Harrison C, et al. The management of community-acquired pneumonia in infants and children older than 3 months of age: clinical practice guidelines by the pediatric infectious diseases society and the infectious diseases society of America. Clin Infect Dis. (2011) 53:e25–76. doi: 10.1093/cid/cir625
62. Caserta MT, Qiu X, Tesini B, Wang L, Murphy A, Corbett A, et al. Development of a global respiratory severity score for respiratory syncytial virus infection in infants. J Infect Dis. (2016) 215:750–6. doi: 10.1093/infdis/jiw624
63. Marlais M, Evans J, Abrahamson E. Clinical predictors of admission in infants with acute bronchiolitis. Arch Dis Child. (2011) 96:648–52. doi: 10.1136/adc.2010.201079
64. Houben ML, Bont L, Wilbrink B, Belderbos ME, Kimpen JL, Visser GH, et al. Clinical prediction rule for RSV bronchiolitis in healthy newborns: prognostic birth cohort study. Pediatrics. (2011) 127:35–41. doi: 10.1542/peds.2010-0581
65. Brand HK, Ahout IML, De Ridder D, van Diepen A, Li Y, Zaalberg M, et al. Olfactomedin 4 serves as a marker for disease severity in pediatric respiratory syncytial virus (RSV) infection. PLoS ONE. (2015) 10:e0131927. doi: 10.1371/journal.pone.0131927
66. Bertrand P, Lay MK, Piedimonte G, Brockmann PE, Palavecino CE, Hernández J, et al. Elevated IL-3 and IL-12p40 levels in the lower airway of infants with RSV-induced bronchiolitis correlate with recurrent wheezing. Cytokine. (2015) 76:417–23. doi: 10.1016/j.cyto.2015.07.017
67. Van den Kieboom CH, Ahout IM, Zomer A, Brand KH, de Groot R, Ferwerda G, et al. Nasopharyngeal gene expression, a novel approach to study the course of respiratory syncytial virus infection. Eur Respir J. (2015) 45:718–25. doi: 10.1183/09031936.00085614
68. Jong VL, Ahout IM, van den Ham HJ, Jans J, Zaaraoui-Boutahar F, Zomer A, et al. Transcriptome assists prognosis of disease severity in respiratory syncytial virus infected infants. Sci Rep. (2016) 6:36603. doi: 10.1038/srep36603
69. Luchsinger V, Ampuero S, Palomino MA, Chnaiderman J, Levican J, Gaggero A, et al. Comparison of virological profiles of respiratory syncytial virus and rhinovirus in acute lower tract respiratory infections in very young Chilean infants, according to their clinical outcome. J Clin Virol. (2014) 61:138–44. doi: 10.1016/j.jcv.2014.06.004
70. Eisenhut M. Extrapulmonary manifestations of severe respiratory syncytial virus infection–a systematic review. Crit Care. (2006) 10:R107. doi: 10.1186/cc4984
71. Thorburn K, Fulton C, King C, Ramaneswaran D, Alammar A, McNamara PS. Transaminase levels reflect disease severity in children ventilated for respiratory syncytial virus (RSV) bronchiolitis. Sci Rep. (2018) 8:1803. doi: 10.1038/s41598-018-20292-6
72. Laham FR, Trott AA, Bennett BL, Kozinetz CA, Jewell AM, Garofalo RP, et al. LDH concentration in nasal-wash fluid as a biochemical predictor of bronchiolitis severity. Pediatrics. (2010) 125:e225–33. doi: 10.1542/peds.2009-0411
73. Mehta R, Scheffler M, Tapia L, Aideyan L, Patel KD, Jewell AM, et al. Lactate dehydrogenase and caspase activity in nasopharyngeal secretions are predictors of bronchiolitis severity. Influenza Other Respi Viruses. (2014) 8:617–25. doi: 10.1111/irv.12276
74. Kesimer M, Ford AA, Ceppe A, Radicioni G, Cao R, Davis CW, et al. Airway mucin concentration as a marker of chronic bronchitis. N Engl J Med. (2017) 377:911–22. doi: 10.1056/NEJMoa1701632
75. Rajan D, O'Keefe EL, Travers C, McCracken C, Geoghegan S, Caballero MT, et al. MUC5AC levels associated with respiratory syncytial virus disease severity. Clin Infect Dis. (2018) 67:1441–4. doi: 10.1093/cid/ciy340
76. Barrios J, Ai X. Neurotrophins in asthma. Curr Allergy Asthma Rep. (2018) 18:10. doi: 10.1007/s11882-018-0765-y
77. Williams DJ, Zhu Y, Grijalva CG, Self WH, Harrell FE, Reed C, et al. Predicting severe pneumonia outcomes in children. Pediatrics. (2016) 138:e20161019. doi: 10.1542/peds.2016-1019
78. Mejias A, Dimo B, Suarez NM, Garcia C, Suarez-Arrabal MC, Jartti T, et al. Whole blood gene expression profiles to assess pathogenesis and disease severity in infants with respiratory syncytial virus infection. PLoS Med. (2013) 10:e1001549. doi: 10.1371/journal.pmed.1001549
79. Wallihan RG, Suarez NM, Cohen DM, Marcon M, Moore-Clingenpeel M, Mejias A, et al. Molecular distance to health transcriptional score and disease severity in children hospitalized with community-acquired pneumonia. Front Cell Infect Microbiol. (2018) 8:00382. doi: 10.3389/fcimb.2018.00382
80. Pankla R, Buddhisa S, Berry M, Blankenship DM, Bancroft GJ, Banchereau J, et al. Genomic transcriptional profiling identifies a candidate blood biomarker signature for the diagnosis of septicemic melioidosis. Genome Biol. (2009) 10:R127. doi: 10.1186/gb-2009-10-11-r127
81. Garcia-Mauriño C, Moore-Clingenpeel M, Thomas J, Mertz S, Cohen DM, Ramilo O, et al. Viral load dynamics and clinical disease severity in infants with respiratory syncytial virus infection. J Infect Dis. (2018) 219:1207–15. doi: 10.1093/infdis/jiy655
82. DeVincenzo JP, Wilkinson T, Vaishnaw A, Cehelsky J, Meyers R, Nochur S, et al. Viral load drives disease in humans experimentally infected with respiratory syncytial virus. Am J Respir Crit Care Med. (2010) 182:1305–14. doi: 10.1164/rccm.201002-0221OC
83. Kadji FMN, Okamoto M, Furuse Y, Tamaki R, Suzuki A, Lirio I, et al. Differences in viral load among human respiratory syncytial virus genotypes in hospitalized children with severe acute respiratory infections in the Philippines. Virol J. (2016) 13:113. doi: 10.1186/s12985-016-0565-8
84. El Saleeby CM, Bush AJ, Harrison LM, Aitken JA, DeVincenzo JP. Respiratory syncytial virus load, viral dynamics, and disease severity in previously healthy naturally infected children. J Infect Dis. (2011) 204:996–1002. doi: 10.1093/infdis/jir494
85. Houben ML, Coenjaerts FEJ, Rossen JWA, Belderbos ME, Hofland RW, Kimpen JLL, et al. Disease severity and viral load are correlated in infants with primary respiratory syncytial virus infection in the community. J Med Virol. (2010) 82:1266–71. doi: 10.1002/jmv.21771
86. Piedra FA, Mei M, Avadhanula V, Mehta R, Aideyan L, Garofalo RP, et al. The interdependencies of viral load, the innate immune response, and clinical outcome in children presenting to the emergency department with respiratory syncytial virus-associated bronchiolitis. PLoS ONE. (2017) 12:e0172953. doi: 10.1371/journal.pone.0172953
87. Saravia J, You D, Shrestha B, Jaligama S, Siefker D, Lee GI, et al. Respiratory syncytial virus disease is mediated by age-variable IL-33. PLoS Pathog. (2015) 11:e1005217. doi: 10.1371/journal.ppat.1005217
88. García-García ML, Calvo C, Moreira A, Cañas JA, Pozo F, Sastre B, et al. Thymic stromal lymphopoietin, IL-33, and periostin in hospitalized infants with viral bronchiolitis. Medicine. (2017) 96:e6787. doi: 10.1097/MD.0000000000006787
89. Tabarani CM, Bonville CA, Suryadevara M, Branigan P, Wang D, Huang D, et al. Novel inflammatory markers, clinical risk factors, and virus type associated with severe respiratory syncytial virus infection. Pediatr Infect Dis J. (2013) 32:e437. doi: 10.1097/INF.0b013e3182a14407
90. Neto EA, Durigon TS, Oliveira-Junior MC, dos Santos Dias A, MacKenzie B, Constancio FS, et al. New serum and nasal immunological biomarkers in pediatric infection caused by respiratory syncytial virus. Eur Respi J. (2016) 48:PA1277. doi: 10.1183/13993003.congress-2016.PA1277
91. Huang AX, Lu LW, Liu WJ, Huang M. Plasma inflammatory cytokine IL-4, IL-8, IL-10, and TNF-α levels correlate with pulmonary function in patients with asthma-chronic obstructive pulmonary disease (COPD) overlap syndrome. Med Sci Monitor. (2016) 22:2800. doi: 10.12659/MSM.896458
92. Díaz PV, Valdivia G, Gaggero AA, Bono MR, Zepeda G, Rivas M, et al. Pro-inflammatory cytokines in nasopharyngeal aspirate from hospitalized children with respiratory syncytial virus infection with or without rhinovirus bronchiolitis and use of the cytokines as predictors of illness severity. Medicine. (2015) 94:39. doi: 10.1097/MD.0000000000001512
93. Moreira A, García ML, Calvo C, Quevedo S, Pozo F, Casas I, et al. TSLP and periostin in infants with viral bronchiolitis. Eur Respi J. (2016) 48:OA4987. doi: 10.1183/13993003.congress-2016.OA4987
94. Han M, Rajput C, Hong JY, Lei J, Hinde JL, Wu Q, et al. The innate cytokines IL-25, IL-33, and TSLP cooperate in the induction of type 2 innate lymphoid cell expansion and mucous metaplasia in rhinovirus-infected immature mice. J Immunol. (2017) 199:1308–18. doi: 10.4049/jimmunol.1700216
95. Varricchi G, Pecoraro A, Marone G, Criscuolo G, Spadaro G, Genovese A, et al. Thymic stromal lymphopoietin isoforms, inflammatory disorders and cancer. Front Immunol. (2018) 9:1595. doi: 10.3389/fimmu.2018.01595
96. Lopez-Guisa JM, Powers C, File D, Cochrane E, Jimenez N, Debley JS. Airway epithelial cells from asthmatic children differentially express proremodeling factors. J Allergy Clin Immunol. (2012) 129:990–7. doi: 10.1016/j.jaci.2011.11.035
97. Inoue T, Akashi K, Watanabe M, Ikeda Y, Ashizuka S, Motoki T, et al. Periostin as a biomarker for the diagnosis of pediatric asthma. Pediat Allergy Immunol. (2016) 27:521–6. doi: 10.1111/pai.12575
98. Habernau AM, Del VPA, Rodríguez FV, Bobadilla PG. Role of Periostin in Uncontrolled Asthma in Children (DADO study). J Invest Allergol Clin Immunol. (2017) 27:291–8. doi: 10.18176/jiaci.0144
99. Vu LD, Ward C, Devincenzo J, Philip R, Cormier SA, Kimura D. Elevated periostin and nt-probnp in tracheal aspirates were associated with pulmonary hypertension in mechanically ventilated children with rsv bronchiolitis. In: D25. Advances in Rare Lung and Pulmonary Vascular Disease in Children. American Thoracic Society (2018). p. A6313
100. Lu S, Hartert TV, Everard ML, Giezek H, Nelsen L, Mehta A, et al. Predictors of asthma following severe respiratory syncytial virus (RSV) bronchiolitis in early childhood. Pediatr Pulmonol. (2016) 51:1382–92. doi: 10.1002/ppul.23461
101. Zhang Y, Yang L, Wang H, Zhang G, Sun X. Respiratory syncytial virus non-structural protein 1 facilitates virus replication through miR-29a-mediated inhibition of interferon-α receptor. Biochem Biophys Res Commun. (2016) 478:1436–41. doi: 10.1016/j.bbrc.2016.08.142
102. Do LAH, Pellet J, van Doorn HR, Tran AT, Nguyen BH, Tran TTL, et al. Host transcription profile in nasal epithelium and whole blood of hospitalized children under 2 years of age with respiratory syncytial virus infection. J Infect Dis. (2017) 217:134–46. doi: 10.1093/infdis/jix519
103. Drake LY, Kita H. IL-33: biological properties, functions, and roles in airway disease. Immunol Rev. (2017) 278:173–84. doi: 10.1111/imr.12552
104. Peine M, Marek RM, Löhning M. IL-33 in T cell differentiation, function, and immune homeostasis. Trends Immunol. (2016) 37:321–33. doi: 10.1016/j.it.2016.03.007
105. Halim TY, Steer CA, Mathä L, Gold MJ, Martinez-Gonzalez I, McNagny KM, et al. Group 2 innate lymphoid cells are critical for the initiation of adaptive T helper 2 cell-mediated allergic lung inflammation. Immunity. (2014) 40:425–35. doi: 10.1016/j.immuni.2014.01.011
106. Baggiolini M, Clark-Lewis I. Interleukin-8, a chemotactic and inflammatory cytokine. FEBS Lett. (1992) 307:97–101. doi: 10.1016/0014-5793(92)80909-Z
107. Russo RC, Garcia CC, Teixeira MM, Amaral FA. The CXCL8/IL-8 chemokine family and its receptors in inflammatory diseases. Exp Rev Clin Immunol. (2014) 10:593–619. doi: 10.1586/1744666X.2014.894886
108. Hess C, Means TK, Autissier P, Woodberry T, Altfeld M, Addo MM, et al. IL-8 responsiveness defines a subset of CD8 T cells poised to kill. Blood. (2004) 104:3463–71. doi: 10.1182/blood-2004-03-1067
109. Katoh H, Wang D, Daikoku T, Sun H, Dey SK, DuBois RN. CXCR2-expressing myeloid-derived suppressor cells are essential to promote colitis-associated tumorigenesis. Cancer Cell. (2013) 24:631–44. doi: 10.1016/j.ccr.2013.10.009
110. McNamara PS, Ritson P, Selby A, Hart CA, Smyth RL. Bronchoalveolar lavage cellularity in infants with severe respiratory syncytial virus bronchiolitis. Arch Dis Child. (2003) 88:922–6. doi: 10.1136/adc.88.10.922
111. Díaz PV, Gaggero AA, Pinto RA, Mamani R, Uasapud PA, Bono MR. Levels of inflammatory cytokines and plasma cortisol in respiratory syncytial virus bronchiolitis. Rev Med Chil. (2013) 141:574–81. doi: 10.4067/S0034-98872013000500004
112. Reche PA, Soumelis V, Gorman DM, Clifford T, Liu MR, Travis M, et al. Human thymic stromal lymphopoietin preferentially stimulates myeloid cells. J Immunol. (2001) 167:336–43. doi: 10.4049/jimmunol.167.1.336
113. Soumelis V, Reche PA, Kanzler H, Yuan W, Edward G, Homey B, et al. Human epithelial cells trigger dendritic cell–mediated allergic inflammation by producing TSLP. Nat Immunol. (2002) 3:673. doi: 10.1038/ni805
114. Harada M, Hirota T, Jodo AI, Doi S, Kameda M, Fujita K, et al. Functional analysis of the thymic stromal lymphopoietin variants in human bronchial epithelial cells. Am J Respir Cell Mol Biol. (2009) 40:368–74. doi: 10.1165/rcmb.2008-0041OC
115. Lee HC, Headley MB, Loo YM, Berlin A, Gale M Jr., Debley JS, et al. Thymic stromal lymphopoietin is induced by respiratory syncytial virus–infected airway epithelial cells and promotes a type 2 response to infection. J Allergy Clin Immunol. (2012) 130:1187–96. doi: 10.1016/j.jaci.2012.07.031
116. Jones SA, Jenkins BJ. Recent insights into targeting the IL-6 cytokine family in inflammatory diseases and cancer. Nat Rev Immunol. (2018) 18:773–89. doi: 10.1038/s41577-018-0066-7
117. Tanaka T, Narazaki M, Kishimoto T. IL-6 in inflammation, immunity, and disease. Cold Spring Harb Perspect Biol. (2014) 6:a016295. doi: 10.1101/cshperspect.a016295
118. Vieira RA, Diniz EMDA, Ceccon MEJR. Correlation between inflammatory mediators in the nasopharyngeal secretion and in the serum of children with lower respiratory tract infection caused by respiratory syncytial virus and disease severity. J Brasileiro de Pneumol. (2010) 36:59–66. doi: 10.1590/S1806-37132010000100011
119. Nuzzo PV, Buzzatti G, Ricci F, Rubagotti A, Argellati F, Zinoli L, et al. Periostin: a novel prognostic and therapeutic target for genitourinary cancer? Clin Genitourin Cancer. (2014) 12:301–11. doi: 10.1016/j.clgc.2014.02.005
120. Federico MJ, Hoch HE, Anderson WC, Spahn JD, Szefler SJ. Asthma Management for Children: risk identification and Prevention. Adv Pediatr. (2016) 63:103–26. doi: 10.1016/j.yapd.2016.04.010
121. Gour N, Wills-Karp M. IL-4 and IL-13 signaling in allergic airway disease. Cytokine. (2015) 75:68–78. doi: 10.1016/j.cyto.2015.05.014
122. Parulekar AD, Diamant Z, Hanania NA. Role of T2 inflammation biomarkers in severe asthma. Curr Opin Pulm Med. (2016) 22:59–68. doi: 10.1097/MCP.0000000000000231
123. Sigurs N, Aljassim F, Kjellman B, Robinson PD, Sigurbergsson F, Bjarnason R, et al. Asthma and allergy patterns over 18 years after severe RSV bronchiolitis in the first year of life. Thorax. (2010) 65:1045–52. doi: 10.1136/thx.2009.121582
124. Ivashkiv LB, Donlin LT. Regulation of type I interferon responses. Nat Rev Immunol. (2014) 14:36. doi: 10.1038/nri3581
125. Ng CT, Mendoza JL, Garcia KC, Oldstone MB. Alpha and beta type 1 interferon signaling: passage for diverse biologic outcomes. Cell. (2016) 164:349–52. doi: 10.1016/j.cell.2015.12.027
126. Chen K, Liu J, Cao X. Regulation of type I interferon signaling in immunity and inflammation: a comprehensive review. J Autoimmun. (2017) 83:1–11. doi: 10.1016/j.jaut.2017.03.008
127. Gentile DA, Skoner DP. Effect of respiratory syncytial virus infection during early infancy on the ontogeny of cytokine immune responses. In Allergy and Asthma Proceedings. OceanSide Publications. (2002). p. 399.
128. Murphy JM, Young IG. IL-3, IL-5, and GM-CSF signaling crystal structure of the human beta-common receptor. Vitam Horm. (2006) 74:1–30. doi: 10.1016/S0083-6729(06)74001-8
129. Broughton SE, Dhagat U, Hercus TR, Nero TL, Grimbaldeston MA, Bonder CS, et al. The GM–CSF/IL-3/IL-5 cytokine receptor family: from ligand recognition to initiation of signaling. Immunol Rev. (2012) 250:277–302. doi: 10.1111/j.1600-065X.2012.01164.x
130. Wynn TA. IL-13 effector functions. Annu Rev Immunol. (2003) 21:425–56. doi: 10.1146/annurev.immunol.21.120601.141142
131. Ingram JL, Kraft M. IL-13 in asthma and allergic disease: asthma phenotypes and targeted therapies. J Allergy Clin Immunol. (2012) 130:829–42. doi: 10.1016/j.jaci.2012.06.034
Keywords: biomarker, cytokines, LRTI, hRSV, severity, prognosis
Citation: Vázquez Y, González L, Noguera L, González PA, Riedel CA, Bertrand P and Bueno SM (2019) Cytokines in the Respiratory Airway as Biomarkers of Severity and Prognosis for Respiratory Syncytial Virus Infection: An Update. Front. Immunol. 10:1154. doi: 10.3389/fimmu.2019.01154
Received: 15 December 2018; Accepted: 07 May 2019;
Published: 04 June 2019.
Edited by:
Steven Varga, The University of Iowa, United StatesReviewed by:
Suresh Pallikkuth, University of Miami, United StatesJun Chang, Ewha Womans University, South Korea
Copyright © 2019 Vázquez, González, Noguera, González, Riedel, Bertrand and Bueno. This is an open-access article distributed under the terms of the Creative Commons Attribution License (CC BY). The use, distribution or reproduction in other forums is permitted, provided the original author(s) and the copyright owner(s) are credited and that the original publication in this journal is cited, in accordance with accepted academic practice. No use, distribution or reproduction is permitted which does not comply with these terms.
*Correspondence: Susan M. Bueno, c2J1ZW5vQGJpby5wdWMuY2w=