- 1Department of Pharmacology, Faculty of Pharmaceutical Sciences, Doshisha Women's College of Liberal Arts, Kyoto, Japan
- 2Laboratory of Immunology, Faculty of Pharmacy, Osaka Ohtani University, Osaka, Japan
- 3Department of Pharmacology, Faculty of Pharmacy, Keio University, Tokyo, Japan
- 4Department of Molecular Pharmacology, Kitasato University School of Pharmaceutical Sciences, Tokyo, Japan
It is now apparent that immune cells express a functional cholinergic system and that α7 nicotinic acetylcholine receptors (α7 nAChRs) are involved in regulating T cell differentiation and the synthesis of antigen-specific antibodies and proinflammatory cytokines. Here, we investigated the specific function α7 nAChRs on T cells and antigen presenting cells (APCs) by testing the effect of GTS-21, a selective α7 nAChR agonist, on differentiation of CD4+ T cells from ovalbumin (OVA)-specific TCR transgenic DO11.10 mice activated with OVA or OVA peptide323−339 (OVAp). GTS-21 suppressed OVA-induced antigen processing-dependent development of CD4+ regulatory T cells (Tregs) and effector T cells (Th1, Th2, and Th17). By contrast, GTS-21 up-regulated OVAp-induced antigen processing-independent development of CD4+ Tregs and effector T cells. GTS-21 also suppressed production of IL-2, IFN-γ, IL-4, IL-17, and IL-6 during OVA-induced activation but, with the exception IL-2, enhanced their production during OVAp-induced activation. In addition, during antigen-nonspecific, APC-independent anti-CD3/CD28 antibody-induced CD4+ polyclonal T cell activation in the presence of respective polarizing cytokines, GTS-21 promoted development of all lineages, which indicates that GTS-21 also acts via α7 nAChRs on T cells. These results suggest 1) that α7 nAChRs on APCs suppress CD4+ T cell activation by interfering with antigen presentation through inhibition of antigen processing; 2) that α7 nAChRs on CD4+ T cells up-regulate development of Tregs and effector T cells; and that α7 nAChR agonists and antagonists could be potentially useful agents for immune response modulation and enhancement.
Introduction
Human peripheral blood T cells and leukemic T cell lines contain substantial amounts of acetylcholine (ACh) and express the mRNA and protein for the ACh-synthesizing enzyme choline acetyltransferase (ChAT) (1–5). Similarly, expression of ChAT mRNA and/or protein has also been detected in rat and mouse T cells (4–10). Among T cell subpopulations, human and rat CD4+ T cells exhibit prominent ChAT mRNA expression and contain higher amounts of ACh than do CD8+ T cells (6, 11). Other immune cells, including B cells (6), dendritic cells (DCs) (4, 5, 12–14) and macrophages (4, 5, 14), also express ChAT mRNA and/or contain ACh. Together, these findings indicate that immune cells have the ability to synthesize ACh via ChAT.
T and B cells, DCs and macrophages all express various subtypes of muscarinic and nicotinic ACh receptors (mAChRs and nAChRs, respectively) (1–5, 15). All five mAChR subtypes (M1-M5) are expressed to some degree in most immune cells (1–5, 15). Because of the wide variety of nAChR subtypes, available data on the expression of nAChR subtypes in immune cells are not consistent (1–5, 15). That said, immune cells most commonly express mRNAs for the α2, α5, α6, α7, α9, and α10 subunits (4, 5, 12, 16–18). Furthermore, Qian et al. (18) showed that immunological activation of T cells can modify the pattern and intensity of mAChR and nAChR expression.
Among the nAChR subtypes, the role of α7 nAChR in the regulation of immune function has drawn attention in part because stimulating α7 nAChRs on macrophages suppresses the synthesis and release of tumor necrosis factor (TNF)-α, thereby protecting mice from lethal endotoxin shock induced by lipopolysaccharide (19). α7 nAChR gene-deficient (α7-KO) mice immunized with ovalbumin (OVA) exhibit significantly higher serum antigen-specific IgG1 concentrations than wild-type (WT) C57BL/6J mice, and, in the presence of OVA, OVA-immunized splenic cells from α7-KO mice produce greater amounts of TNF-α, interferon (IFN)-γ, and IL-6 than do those from the WT mice (20). Furthermore, α7 nAChRs enhance B cell survival in bone marrow (21) but suppress mature B cell proliferation activated via CD40-mediated pathways (22), which suggests signaling via α7 nAChRs in B cells plays a role in regulating antibody (Ab) production (23). Stimulation of α7 nAChRs using nicotine suppresses differentiation into Th1 and Th17 cells but enhances differentiation into Th2 cells of naïve CD4+ T cells non-specifically activated with anti-CD3/CD28 Abs (24, 25). On the other hand, Galitovskiy et al. (26) showed that nicotine acts via α7 nAChR-mediated pathways to increase the percentage of colonic regulatory T cells (Tregs) while reducing Th17 cells in oxazolone colitis, and that nicotine increases numbers of Tregs among CD4+ CD62L+ T cells non-specifically activated using anti-CD3/CD28 Abs. These findings suggest α7 nAChR signaling may modulate immune function through regulation of such T cell activities as differentiation and cytokine production.
In the present study, we endeavored to clarify the roles of α7 nAChRs expressed on T cells and antigen presenting cells (APCs) during regulation of CD4+ T cell differentiation. The effects of 3-[(2,4-dimethoxy)benzylidene]-anabaseine (GTS-21), a selective partial α7 nAChR agonist (27), on antigen-specific, antigen processing-dependent; antigen-specific, antigen processing-independent; and antigen-nonspecific, APC-independent CD4+ T cell differentiation were studied in spleen cells from OVA-specific T cell receptor (TCR) transgenic DO11.10 mice (28) and α7-KO mice. Our findings demonstrate that α7 nAChR signaling in APCs suppresses CD4+ T cell development by interfering with antigen presentation through inhibition of antigen processing, and that α7 nAChR signaling in CD4+ T cells up-regulates differentiation and proliferation into both Tregs and effector T cells.
Materials and Methods
Animals
OVA-specific TCR transgenic DO11.10 (H-2d) mice on a BALB/c background, and α7 nAChR-deficient (α7-KO) (H-2b) mice on a C57BL/6J background were purchased from The Jackson laboratory. C57BL/6J (H-2b) mice were from Japan SLC.
CD4+ T Cell Culture and Differentiation
Spleen cells were prepared from mice (3–6 months old) as described previously (29) and cultured in RPMI 1640 supplemented with 10% fetal bovine serum (FBS), 50 μM 2-mercaptoethanol, 100 units/ml penicillin and 100 μg/ml streptomycin at 37°C under a humidified atmosphere with 5% CO2.
To examine the effects of the selective partial α7 nAChR agonist GTS-21 on CD4+ T cell differentiation into Tregs and effector T cells (Th1, Th2, and Th17), spleen cells (4 × 106 cells) were cultured for 5 days in 24-well plates in the presence of 20 μg/ml OVA with and without GTS-21 (3–30 μM).
To examine the effects of GTS-21 on antigen processing-independent differentiation, CD4+ T cell differentiation was activated with 200 ng/ml OVA peptide323−339 (OVAp) for 5 days in the absence or presence of GTS-21 (3–30 μM) under the same experimental conditions described above.
To determine the role of α7 nAChRs expressed on CD4+ T cells in the regulation of CD4+ T cell differentiation into Tregs and effector T cells, naïve CD4+ T cells were isolated from spleen cells from DO11.10, α7-KO, and control WT C57BL/6J mice using a naïve CD4+ T cell isolation kit (130-104-453, Miltenyi Biotec) according to the manufacturer's instructions. The isolated CD4+ cells were cultured in 96-well plates (1 × 105 cells) coated with anti-CD3 Ab (145-2C11, 5 μg/ml) in the presence of anti-CD28 Ab (37.51, 1 μg/ml), GTS-21 (3–30 μM), and the respective cytokines and Abs required for induction of each subset of effector T cell (Table 1) (30).
Flow Cytometry
For detection of Tregs, spleen cells were stained using FITC-conjugated anti-CD4 Ab (RM4.5, Thermo Fisher Scientific) and PE-conjugated anti-CD25 Ab (PC61.5, Thermo Fisher Scientific). After fixation and permeabilization using BD Cytofix/Cytoperm solution (BD Biosciences), the cells were further stained with APC-conjugated anti-FoxP3 (3G3, Thermo Fisher Scientific). The cells were then washed with Hanks' balanced salt solution (HBSS) supplemented with 0.1% bovine serum albumin (BSA) and 0.1% NaN3 and subjected to flow cytometry (CytoFLEX, Beckman Coulter). A gate was set on the lymphocytes using characteristic forward scatter (FSC) and side scatter (SSC) parameters. Isotype-matched FITC, PE and APC-conjugated mouse IgG1 Abs were used as controls. The acquired data was analyzed using CytExpert (Beckman Coulter).
For detection of effector T cells (Th1, Th2, and Th17 cells), spleen cells were stimulated for 1 h with 50 ng/ml phorbol 12-myristate 13-acetate and 1 μg/ml ionomycin and then with GolgiStop (BD Biosciences) for 4 h. The cells were then collected, washed with HBSS supplemented with 0.1% BSA and 0.1% NaN3 and stained with FITC-conjugated anti-CD4 Ab for 20 min at 4°C. The stained cells were fixed and permeabilized using BD Cytofix/Cytoperm solution and further stained with PE-conjugated anti-IFN-γ Ab (XMG1.2, Thermo Fisher Scientific) (Th1), APC-conjugated anti-IL-4 Ab (11B11, Thermo Fisher Scientific) (Th2), or anti-IL-17A Ab (eBio17B7, Thermo Fisher Scientific) (Th17) for 20 min at 4°C. After washing, the prepared cells were subjected to flow cytometry.
For cell proliferation assay, spleen cells prepared from DO11.10 mice were stained with 5 μM carboxyfluorescein succinimidyl ester (CFSE) cell proliferation reagent (Nacalai tesque) in PBS for 10 min, and cultured for 5 days under the same experimental conditions as described above with OVA or OVAp in the presence or absence of 30 μM GTS-21 and then stained with APC-conjugated anti-CD4 Ab. After washing, the prepared cells were subjected to flow cytometry.
For detection of accessory surface molecules in APCs, spleen cells prepared from DO11.10 mice were incubated for 16 h in 24-well plates (4 × 106 cells) in the presence of 20 μg/ml OVA with and without GTS-21 (30 μM). To detect CD40, CD80 and MHC class II expression, spleen cells were incubated with anti-CD16/CD32 Abs (2.4G2, BD Biosciences) and then stained using FITC-conjugated anti-CD40 Ab (3/23, Biolegend), anti-CD80 Ab (16-10A1, Biolegend), or anti-MHC class II Ab (I-A/I-E, M5/114.15.2, Biolegend) along with PE-conjugated anti-CD11b (M1/70, Thermo Fisher Scientific) and APC-conjugated anti-CD11c Abs (N418, Thermo Fisher Scientific). After washing, the prepared cells were subjected to flow cytometry.
For cell viability assays, DO11.10 spleen cells prepared as described above were cultured for 5 days in 24-well plates (4 × 106 cells) in the presence of 20 μg/ml OVA or 200 ng/ml OVAp, with or without GTS-21 (30 μM). At the end of the culture, the viability (7AAD exclusion) of the CD4+ T cells and the CD11b+ and CD11c+ cells was determined. Briefly, spleen cells were stained with APC-conjugated anti-CD4, PE-conjugated anti-CD11b and APC-conjugated anti-CD11c Abs, after which they were counterstained with 7AAD (0.25 μg/ml) and subjected to flow cytometry.
Enzyme-Linked Immunosorbent Assay (ELISA)
Levels of IL-2, IFN-γ, IL-4, IL-6, and IL-17 in culture supernatants were quantified using a sandwich ELISA. The following pairs of capture and biotinylated detection rat anti-mouse mAbs were used: for IFN-γ, anti-IFN-γ (P4-6A2, Biolegend) and biotin-conjugated anti-IFN-γ (XMG1.2, Biolegend) Abs; for IL-2, anti-IL-2 (JES6-1A12, BD Biolegend) and biotin-conjugated anti-IL-2 (JES6-5H4, BD Biolegend) Abs; for IL-4, anti-IL-4 (11B11, Biolegend) and biotin-conjugated anti-IL-4 (BVD6-24G2, Biolegend) Abs; for IL-6, anti-IL-6 (MP5-20F3, BD Biosciences) and biotin-conjugated anti-IL-6 (MP5-32C11, BD Biosciences); for IL-17, anti-IL-17 Ab (TC11-18H10, BD biosciences) and biotin-conjugated anti-IL-17Ab (TC11-8H4, BD Biosciences). Capture Abs (2 μg/ml) were coated onto 96-well plates. After blocking with 0.5% BSA in PBS containing 0.05% Tween 20, the diluted samples and recombinant protein standards were incubated for 1 h at room temperature. Plates were then incubated with biotin-conjugated detection Abs (1 μg/ml) for 1 h at 37°C and reacted with streptavidin-conjugated horseradish peroxidase, followed by o-phenylenediamine. The reaction was terminated by addition of 0.5 M H2SO4. The absorbance at 490 nm was then measured, and a graph was created by analyzing three samples.
Detection of OVA Uptake Into APCs
Spleen cells (4 × 106 cells) were incubated for 4 h with FITC-labeled OVA (OVA-FITC) (50 μg/ml, Thermo Fisher Scientific) in the presence or absence of GTS-21 (30 μM). To observe OVA-FITC uptake using confocal microscopy, the cells were plated onto poly-d-lysine-coated glass-bottom dishes, fixed with 4% paraformaldehyde for 20 min at 4°C, and permeabilized and blocked with Blocking One (Nacalai tesque) containing 0.5% Triton X-100 for 1 h at room temperature. The cells were then incubated with PE-conjugated anti-CD11b and APC-conjugated anti-CD11c Abs for 20 min at 4°C. Nuclei were stained with DAPI (300 nM) for 10 min at room temperature. Cells were imaged using a confocal microscope (Zeiss LSM 800 Meta, Carl Zeiss, Inc., Germany) equipped with an oil-immersion objective (40 × , NA = 1.3). Fluorescence images were processed using ImageJ 1.37a (National Institutes of Health). For flow cytometric analysis, spleen cells were stained with PE-conjugated anti-CD11b and APC-conjugated anti-CD11c Abs for 20 min at 4°C and subjected to flow cytometry.
Statistical Analysis
Data are presented as means ± S.E.M. All experiments were repeated at least three times. Statistical analysis was performed using SigmaPlot (Systat Software Inc.). Differences between two groups were evaluated using Student's t-test, and between three or more groups using one- and two-way analysis of variance (ANOVA) with post hoc Dunnett's or Tukey's test, respectively. Values of P < 0.05 were considered significant.
Results
Effect of GTS-21 on Antigen-Specific CD4+ T Cell Differentiation Induced by OVA
To activate CD4+ T cells and induce differentiation, OVA must be endocytosed into APCs, processed to OVAp, and bound to MHC class II molecules before presentation to CD4+ T cells. As shown in Figure 1, OVA (20 μg/ml) induced CD4+ T cell development into Tregs (CD4+CD25+FoxP3+) and Th1 (CD4+IFN-γ+), Th2 (CD4+IL-4+), and Th17 (CD4+IL-17+) cells (Figure 1B; Control (C) vs. OVA). GTS-21 dose-dependently suppressed OVA-induced CD4+ T cell development into all the lineages (Figures 1A,B). GTS-21 significantly suppressed OVA-induced CD4+ T cell proliferation (Figures 1C,D). OVA also increased the synthesis of IL-2, IFN-γ, IL-4, IL-17, and IL-6 in DO11.10 spleen cells (Figure 1E, Control (C) vs. GTS-21 at 0 μM), and these effects too were dose-dependently suppressed by GTS-21 (Figure 1), which is consistent with its suppression of OVA-induced CD4+ T cell development (Figures 1A,B).
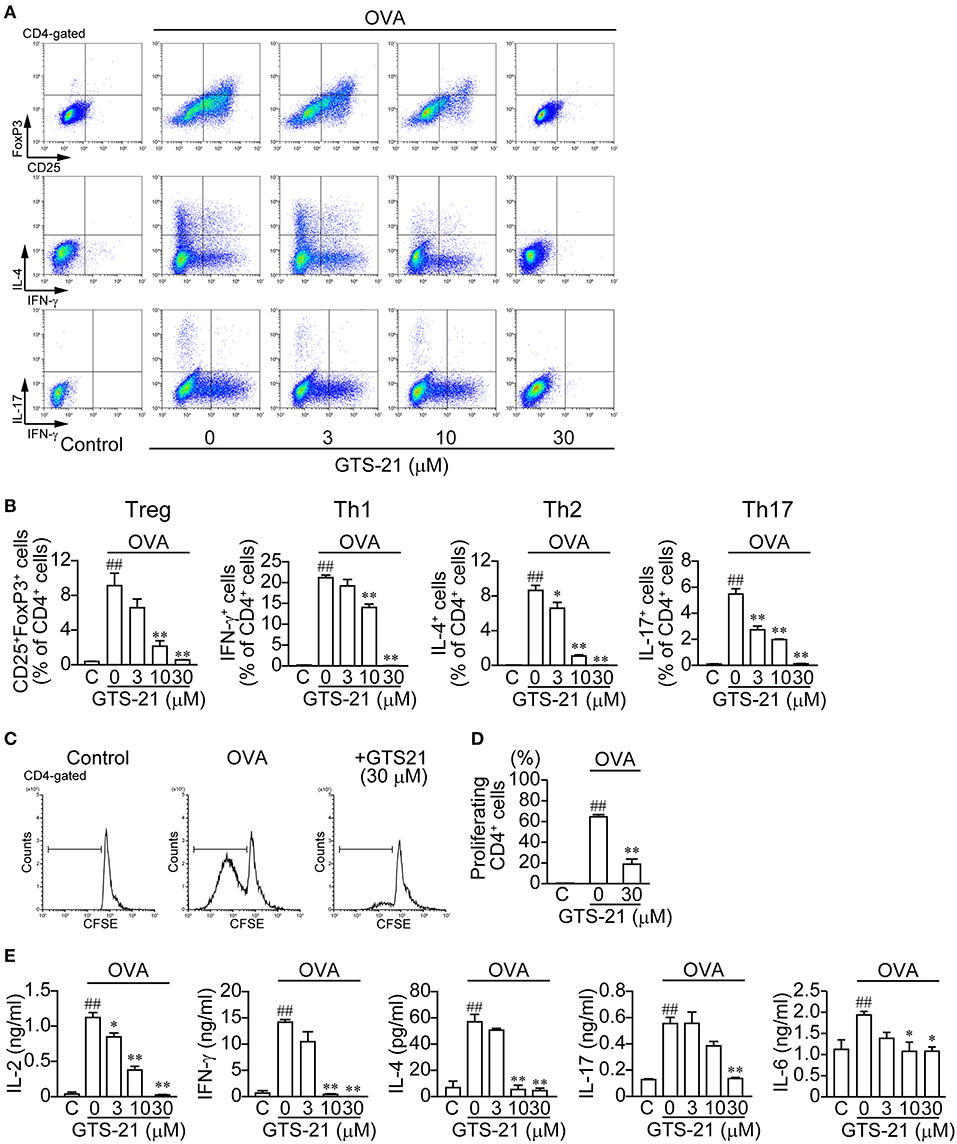
Figure 1. GTS-21 suppresses CD4+ T cell differentiation in OVA-activated DO.11.10 spleen cells. The cells were cultured with OVA and the indicated concentrations of GTS-21 for 5 days. Cultured cells were examined for surface expression of CD4, CD25 and intracellular expression of FoxP3, IL-4, IL-17, IFN-γ. Plots are gated on CD4+ T cells. (A) Representative flow cytometric plots for CD4+CD25+FoxP3+ T cells (Tregs), CD4+IFN-γ+ T cells (Th1), CD4+IL-4+ T cells (Th2), and CD4+IL-17+ T cells (Th17) at the indicated concentrations of GTS-21. (B) Corresponding percentages of OVA-activated Tregs and Th1, Th2, and Th17 cells of CD4+ T cells detected at the indicated concentrations of GTS-21. The bars represent means ± SEM for at least three samples. Note that GTS-21 suppressed OVA-activated differentiation. C, control (without OVA). ##P < 0.01 vs. C. *P < 0.05, **P < 0.01 vs. GTS-21 at 0 μM. (C) Representative flow cytometric histograms for CFSE-labeled CD4+ cells after 5-day incubation with OVA with or without GTS-21. (D) Corresponding percentages of proliferating CD4+ cells. The bars represent means ± SEM for at least three samples. C, control (without OVA). ##P < 0.01 vs. C. **P < 0.01 vs. GTS-21 at 0 μM. (E) Effect of GTS-21 on Th cytokine synthesis. Levels of IL-2, IFN-γ, IL-4, IL-17, and IL-6 in the conditioned media were determined using ELISAs after culture for 5 days. The bars represent means ± SEM for at least three samples. Note that GTS-21 suppressed OVA-activated Th cytokine synthesis. C, control (without OVA). ##P < 0.01 vs. C. *P < 0.05, **P < 0.01 vs. GTS-21 at 0 μM.
Effect of GTS-21 on Antigen-Specific CD4+ T Cell Differentiation Induced by OVAp
In contrast to OVA, which must be taken up by APCs and processed, OVAp binds directly to MHC class II molecules on the surface of APCs to activate CD4+ T cell differentiation. OVAp (200 ng/ml) activated CD4+ T cell development into Tregs (CD4+CD25+FoxP3+) and Th1 (CD4+IFN-γ+), Th2 (CD4+IL-4+) and Th17 (CD4+IL-17+) cells (Figure 2). GTS-21 dose-dependently up-regulated OVAp-activated CD4+ T cell development into all the lineages (Figure 2). This suggests GTS-21 suppresses OVA-activated CD4+ T cell development by impairing APC endocytosis and/or antigen processing. GTS-21 significantly but slightly suppressed OVAp-induced CD4+ T cell proliferation (Figures 2C,D). OVAp also increased the synthesis of IL-2, IFN-γ, IL-4, IL-17, and IL-6 in DO11.10 spleen cells (Figure 2E, Control (C) vs. GTS-21 at 0 μM) and, with the exception of IL-2 production, those effects were enhanced by GTS-21 (Figure 2E), which reflected well its effects on OVAp-induced T cell development (Figures 2A,B). That IL-2 production was not affected by GTS-21 suggests α7 nAChRs on CD4+ T cells are not involved in IL-2 synthesis.
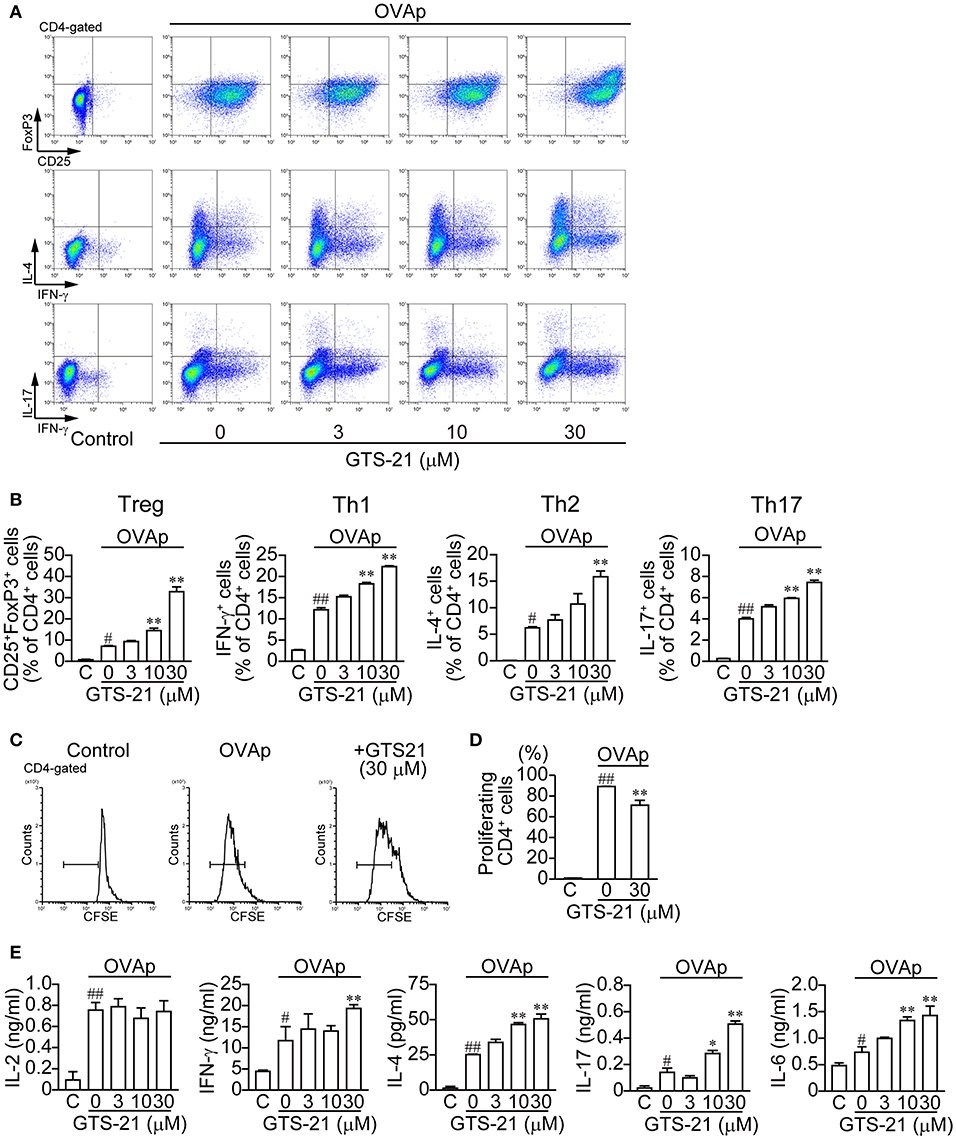
Figure 2. GTS-21 enhances CD4+ T cell differentiation in OVAp-activated DO.11.10 spleen cells. The cells were cultured with OVAp and the indicated concentrations of GTS-21 for 5 days. Cultured cells were examined for surface expression of CD4, CD25 and intracellular expression of FoxP3, IL-4, IL-17, IFN-γ. Plots are gated on CD4+ T cells. (A) Representative flow cytometric plots for Tregs, Th1, Th2, and Th17 at the indicated concentrations of GTS-21. Gates were used to calculate the percentages of positive cells. (B) Corresponding percentages of OVAp-activated Tregs and Th1, Th2, and Th17 cells of CD4+ T cells in the presence of the indicated concentrations of GTS-21. Note that GTS-21 up-regulated OVAp-activated differentiation into all lineages. The bars represent means ± SEM for at least three samples. C, control (without OVAp). #P < 0.05, ##P < 0.01 vs. C. *P < 0.05, **P < 0.01 vs. GTS-21 at 0 μM. (C) Representative flow cytometric histograms for CFSE-labeled CD4+ cells after 5-day incubation with OVAp with or without GTS-21. (D) Corresponding percentages of proliferating CD4+ cells. The bars represent means ± SEM for at least three samples. C, control (without OVA). ##P < 0.01 vs. C. **P < 0.01 vs. GTS-21 at 0 μM. (E) Effect of GTS-21 on Th cytokine synthesis. Levels of IL-2, IFN-γ, IL-4, IL-17, and IL-6 in the conditioned media were determined using ELISAs after culture for 5 days. The bars represent means ± SEM for at least three samples. Note that GTS-21 enhanced OVAp-activated synthesis of Th cytokines, except IL-2. C, control (without OVA). ##P < 0.01 vs. C. *P < 0.05, **P < 0.01 vs. GTS-21 at 0 μM.
These findings shown in Figures 1, 2 suggest that GTS-21 does not affect the polarizing cytokine synthesis necessary for development of Tregs and effector T cells by spleen cells, but that GTS-21 inhibits OVA processing to OVAp in APCs, which leads to suppression of CD4+ T cell development.
Effect of GTS-21 on OVA Endocytosis Into APCs and MHC Class II, CD40, and CD80 Expression
APCs, such as macrophages (CD11b+) and dendritic cells (DCs, CD11c+), are responsible for antigen presentation to CD4+T cells (31). Whether GTS-21 affects the endocytosis of OVA into the CD11b+ and CD11c+ cells was investigated 4 h after addition of OVA-FITC to the cultures. Both CD11b+ and CD11c+ cells endocytosed OVA-FITC, and GTS-21 (30 μM) did not affect the fluorescent signal from these cells (Figure 3A). Moreover, flow-cytometric analysis revealed that GTS-21 did not affect the number of fluorescence-positive CD11b+ and CD11c+ cells or the mean fluorescence intensity (MFI) from CD11b+ and CD11c+ cells, indicating that α7 nAChRs are not involved in regulating OVA endocytosis into APCs (Figures 3B,C). GTS-21 also did not affect the expression of MHC class II, CD40 or CD80 molecules (Figure 3D) as well as their MFIs, indicating that α7 nAChRs are not involved in regulation of expression of these molecules on APCs (Figure 3E). These results suggest that GTS-21 impairs antigen processing after the endocytosis. On the other hand, GTS-21 did not affect the viability of CD4+ T or CD11c+ cells in the presence of OVA or OVPp, though it slightly decreased the viability of CD11b+ cells under both conditions, suggesting a minor role for CD11b+ cells as APCs for OVA antigen (Figure 3F).
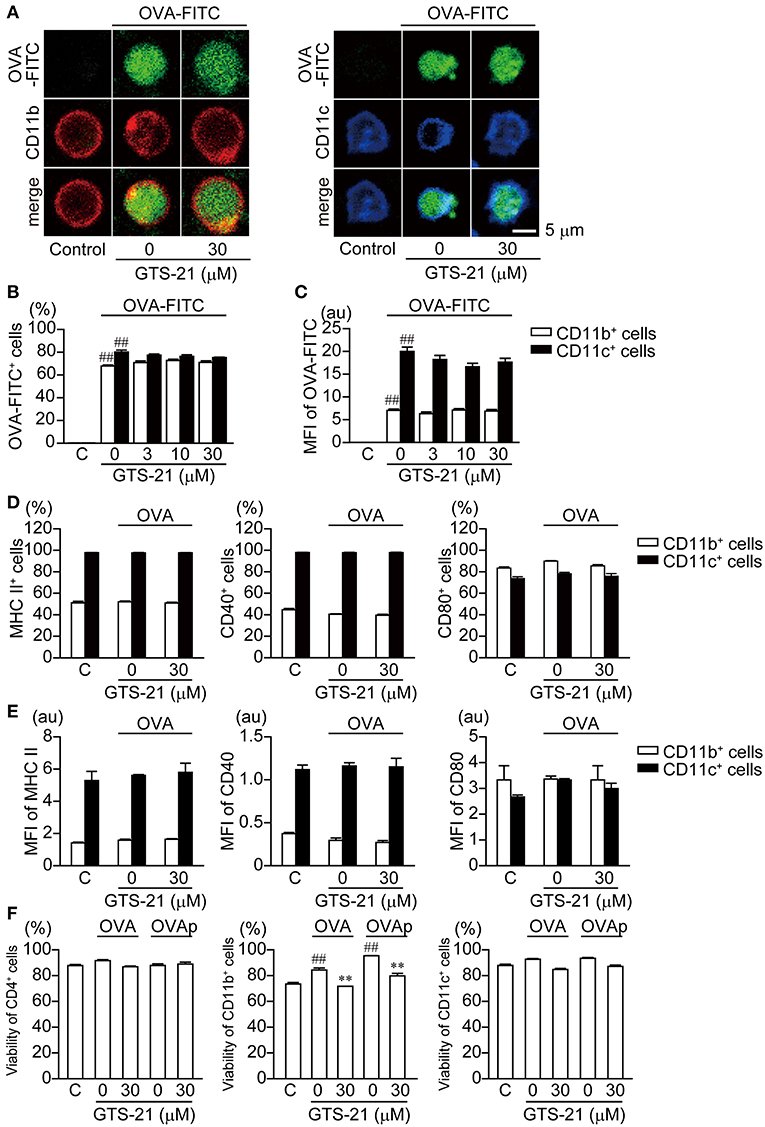
Figure 3. Effects of GTS-21 on FITC-OVA endocytosis; expression of MHC class II, CD40, and CD80 molecules in DO.11.10 CD11b+ and CD11c+ cells; and the viability of DO.11.10 spleen cells. (A) Representative micrographs showing FITC-OVA endocytosis in CD11b+ and CD11c+ cells. The spleen cells were cultured with FITC-OVA (50 μg/ml) on poly-d-lysine-coated glass-bottom dishes in the presence or absence of GTS-21 for 4 h. (B) Flow cytometric analysis of OVA-FITC uptake into CD11b+ and CD11c+. Graphs show the percentages of OVA-FITC+CD11b+ (left) or CD11c+ (right) cells in the presence of the indicated concentrations of GTS-21. The bars represent means ± SEM for at least three samples. C, control (without OVA-FITC). ##P < 0.01 vs. C. Note that GTS-21 did not affect FITC-OVA endocytosis in APCs. (C) Mean fluorescence intensity (MFI) of the gated positive population for each of the respective OVA-FITC+CD11b+ (left) or CD11c+ (right) markers. (D) Percentages of CD11b+ and CD11c+ cells showing surface expression of MHC class II, CD40, and CD80. DO.11.10 spleen cells were cultured for 16 h with OVA in the presence or absence of GTS-21 (30 μM). (E) MFI of MHC II, CD40 and CD80 in CD11b+ (left) or CD11c+ (right) markers. Note that GTS-21 did not affect surface expression of MHC class II, CD40 or CD80 in APCs. (F) Viability (7AAD exclusion) of CD4+ T cells, and CD11b+ and CD11c+ cells. C, control (without OVA). ##P < 0.01 vs. C. **P < 0.01 vs. GTS-21 at 0 μM. Note that GTS-21 slightly decreased the viability of CD11b+ cells but had no significant effect on the viability of CD4+ T cells or CD11c+ cells.
Effect of GTS-21 on Antigen-Non-Specific, APC-Independent CD4+ T Cell Differentiation
We next determined the roles of α7 nAChRs expressed on DO11.10 CD4+ T cells in the regulation of polyclonal CD4+ T cell differentiation activated with anti-CD3/CD28 Abs in the presence and absence of GTS-21. To induce naïve CD4+ T cell differentiation into Tregs and effector T cells (Th1, Th2, and Th17), culture media were supplemented with the appropriate cytokines and Abs, as described in the Materials and Methods (Table 1). GTS-21 dose-dependently up-regulated naïve CD4+ T cell differentiation into Tregs and effector T cells, and their proliferation (Figure 4A). These effects are in line with those observed in OVAp-activated spleen cells from DO11.10 mice (Figure 2), which is consistent with the idea that α7 nAChRs expressed on CD4+ T cells are involved in promoting CD4+ T cell development into all the lineages.
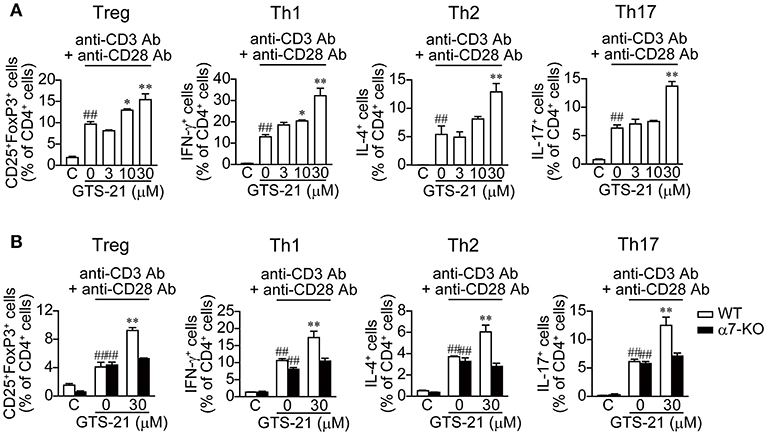
Figure 4. GTS-21 enhances APC-independent CD4+ T cell differentiation induced by anti-CD3/CD28 Abs. (A) Naïve CD4+ T cells isolated from the spleens of DO11.10 mice using a naïve CD4+ T cell isolation kit (130-104-453, Miltenyi Biotec) were stimulated with anti-CD3/CD28 and cultured for 5 days under Treg, Th1, Th2, and Th17 polarizing conditions (Table 1) in the presence of the indicated concentrations of GTS-21. (B) Naïve CD4+ T cells isolated from the spleens of WT or α7-KO mice were stimulated with anti-CD3/CD28 Abs and cultured for 5 days under Treg, Th1, Th2, and Th17 polarizing conditions (Table 1) in the absence or presence of 30 μM GTS-21. Note that GTS-21 enhanced T cell differentiation only in CD4+ cells from the WT mice. The bars represent means ± SEM for at least three samples. C, control (without OVA). ##P < 0.01 vs. C. *P < 0.05, **P < 0.01 vs. GTS-21 at 0 μM.
Moreover, our results obtained using naïve CD4+ T cells from α7-KO and WT mice confirmed the involvement of α7 nAChRs on naïve CD4+ T cells in the promotion of T cell differentiation and proliferation. GTS-21 (30 μM) enhanced anti-CD3/CD28 Ab-activated differentiation and proliferation of polyclonal WT naïve CD4+ T cells into Tregs and effector T cells, but did not affect α7-KO CD4+ T cell differentiation (Figure 4B). These results confirm that the action of GTS-21 on α7 nAChRs expressed on CD4+ T cells up-regulates the cells' differentiation and proliferation.
Discussion
T cell activation via TCR/CD3-mediated pathways up-regulates expression of cholinergic elements such as ChAT, mAChRs and nAChRs within T cells (1–5, 18, 32, 33). Secreted lymphocyte antigen-6/urokinase-type plasminogen activator receptor-related peptide (SLURP)-1, an allosteric α7 nAChR ligand (34, 35) that has been detected in CD205+ DCs in the human tonsil, up-regulates ChAT mRNA expression and ACh synthesis in T cells (36). This suggests ACh released from T cells and from DCs along with SLURP-1 during immune responses such as antigen presentation contributes to the regulation of immune function via α7 nAChR-mediated pathways.
DO11.10 CD4+ T cell differentiation is triggered by TCR recognition of OVA peptide326−339 (OVAp) presented on MHC class II in APCs (28). Thus, OVA activates CD4+ T cell differentiation only after it has been endocytosed into APCs; cleaved to OVAp by endosomal and lysosomal enzymes such as γ-interferon-inducible lysosomal thiol reductase (37, 38) and the cathepsins (39); and then translocated to the cell surface as part of an OVAp-MHC class II complex (see Supplementary Figure 1). By contrast, OVAp can directly bind to MHC class II on the surface of APCs and activate CD4+ T cells differentiation with no further processing (40, 41).
In the present study, GTS-21 suppressed OVA-activated DO.11.10 CD4+ T cell development into Tregs and effector T cells (Th1, Th2 and Th17) (Figure 1) and also suppressed the cytokine synthesis related to CD4+ T cell development (Figure 1E). The importance of α7 nAChRs expressed on APCs, including macrophages, to the regulation of immune function is further supported by the finding that galantamine, an acetylcholinesterase inhibitor with positive allosteric modulator activity toward α7 nAChR (42), suppressed release of IgG, IL-4, and IL-6 during an ex vivo antigen challenge in spleen cells from immunized mice (43). GTS-21 did not affect endocytosis of OVA or expression of MHC class II molecules by APCs (Figures 3A–D). Although GTS-21 (30 μM) slightly decreased the viability of CD11b+ cells in the presence of antigens, it did not affect the viability of CD4+ T cells or CD11c+ cells determined with 7AAD (Figure 3E). No apparent cytotoxicity of GTS-21, even at 50 μM, was demonstrated by Sitapara et al. (44), who reported that by inhibiting the release of nuclear HMGB1, GTS-21 at 25–50 μM restored hyperoxia-compromised particle phagocytic activity in murine macrophage-like RAW 364.7 cells. Furthermore, in the present study, GTS-21 suppressed OVA-induced APC-dependent and antigen processing-dependent CD4+ T cell development (Figure 1) but promoted OVAp-induced APC-dependent and antigen processing-independent CD4+ T cell development at the same concentrations (Figure 2). Taken together, the findings suggest GTS-21 suppresses CD4+ T cell development by pharmacologically inhibiting OVA antigen processing via α7 nAChRs expressed on APCs, not by eliciting functional impairments ascribable to its cytotoxicity in CD4+ T cells or dendritic cells (Supplementary Figures 1, 2).
GTS-21-induced up-regulation of CD4+ T cell development into Tregs and effector T cells in both OVAp-activated DO.11.10 CD4+ T cells and anti-CD3/CD28 Abs-activated CD4+ T cells (Figures 2, 4) suggests α7 nAChRs on CD4+ T cells play role in the up-regulation of T cell differentiation and proliferation. That idea is consistent with our present (Figure 4B) and earlier observations that GTS-21 up-regulates anti-CD3/CD28 Abs-activated CD4+ T cell development into Tregs in WT C57BL/6J spleen cells but not α7-KO spleen cells (4). The results of cell proliferation assay with CSFE suggest the possibility that GTS-21 rather up-regulates differentiation than proliferation (Figure 2C).
The T-cell activator phytohemagglutinin (PHA) up-regulates mRNA expression of IL-2 and ChAT, an enzyme catalyzing ACh synthesis. PHA most likely elicits this response by activating PKC and mitogen-activated protein kinase pathways via TCR/CD3-mediated pathways (45). Recently, Mashimo et al. (29) confirmed that ACh released from activated T cells via TCR/CD3-mediated pathways induces Ca2+ signaling and enhances IL-2 release leading to up-regulation of T cell proliferation, and that both IL-2 production and T cell proliferation are suppressed by mecamylamine, a non-specific nAChR antagonist. However, involvement of α7 nAChRs expressed on T cells in the induction of Ca2+ signaling and enhancement of IL-2 release is unlikely because those receptors are insensitive to methyllycaconitine and α-bungarotoxin, two specific antagonists that block α7 nAChRs expressed on neurons (46). This suggests nAChRs other than α7 nAChR contribute to the induction of Ca2+ signaling and enhanced IL-2 release in TCR/CD3-activated T cells. The induction of IL-2 production in the presence of OVA or OVAp observed in the present study (Figure 2D) can be attributed to naïve T cell activation via TCR/CD3-mediated pathways as a result of antigen presentation triggering ACh synthesis (1–5). Thus, GTS-21 suppressed OVA-induced IL-2 production by interfering with antigen processing, which led to inhibition of antigen presentation but did not affect OVAp-induced IL-2 production, because GTS-21 does not affect OVAp presentation.
The detailed mechanisms involved in the promotion of CD4+ T cell development into Tregs and effector T cells via α7 nAChR-mediated pathways are still unclear. Stein and Singer (47) suggested that IL-6 could replace the requirement for APC-derived co-stimulatory signals for primary CD4+ Th cell proliferation. GTS-21-induced increases in IL-6 production during OVAp-activation, suggesting IL-6 is involved in enhancing CD4+ T cell development (Figure 2). This in turn suggests GTS-21 may affect CD4+ T cell development by enhancing IL-6 synthesis in CD4+ T cells and APCs during the early stages of differentiation. In fact, Eto et al (48) reported that optimal T cell differentiation requires IL-6 along with IL-21. IL-6 signaling in T cells is transduced through the Janus kinase (JAK) family of proteins, culminating in signal transducer and activator of transcription 3 (STAT3) activation (49, 50). STAT3 is a critical positive regulator of T cell differentiation and functions in several CD4+ T cell subsets, including Th2 and Th17 cells and Tregs (51–56). In non-neuronal cells such as macrophages (57) and keratinocytes (58, 59), stimulation of α7 nAChRs by agonists such as GTS-21 and SLURP-1 activates the catalytic intracellular domain of the receptor, leading to recruitment and phosphorylation of JAK2 and subsequent activation of STAT3. This suggests that in addition to promoting IL-6 synthesis, GTS-21 binding to α7 nAChRs on CD4+ T cells promotes their development into Tregs and effecter T cells through activation of the JAK2/STAT3 signaling cascade.
Our observation that GTS-21 suppressed antigen-specific, antigen processing-dependent CD4+ T cell development while promoting antigen-specific, antigen processing-independent CD4+ T cell development suggests α7 nAChR agonists or antagonists could potentially be useful in the treatment of autoimmune diseases or cancers. Studies of the effects of adoptive transfer of antigen-specific Tregs have demonstrated their contribution to the protection and recovery of an animal model of autoimmune encephalomyelitis (60). However, the difficulty in achieving adequate numbers of antigen-specific Tregs for adoptive transfer is a major limitation of its clinical application. The promotion of antigen-specific, antigen processing-independent CD4+ T cell development into Tregs by GTS-21 suggests the possibility that culturing peripheral blood mononuclear cells containing CD4+ T cells and APCs in the presence of specific antigenic epitopes and an α7 nAChR agonist would enhance CD4+ T cells development into antigen-specific Tregs, making them available in greater numbers. Moreover, because GTS-21 promotes antigen-specific, antigen processing-independent CD4+ T cell development into effector T cells, it may be possible to obtain sufficient numbers of the relevant subset of effector T cells for the purpose of immune enhancement.
In summary, the results of the present study revealed that activation of α7 nAChRs on APCs suppresses antigen-specific, antigen processing-dependent CD4+ T cell development by suppressing antigen processing. By contrast, activation of α7 nAChRs expressed on CD4+ T cells up-regulates antigen-specific, antigen processing-independent CD4+ T cell development into Tregs and effector T cells, most likely via activation of JAK2/STAT pathways. α7 nAChRs expressed by immune cells are thus crucially involved in the regulation of both innate and adoptive immunity.
Ethics Statement
This study was carried out in accordance with the recommendations of the Ethical Committees of Doshisha Women's College of Liberal Arts. The protocol was approved by the Ethical Committees of Doshisha Women's College of Liberal Arts (Nos. Y15012, Y15028, Y16002, Y17010, Y17024, Y18001, Y18010).
Author Contributions
KK, TF, MM, and SO were involved in study design, interpretation of the results, and writing and revising the manuscript. MM, MK, YYM, MXM, TF, ST, and HO performed experiments. YM and HM provided experimental materials and were involved in revising the manuscript. KK, TF, MM, MK, YYM, MXM, ST, YM, HM, SO, and HO reviewed and approved the final version of the manuscript.
Funding
This work was supported in part by Grants-in-Aid for Scientific Research (15K07969 and 24590120) from the Ministry of Education, Science, Sports and Culture (C) of Japan (KK, TF, MM) and funding from the Smoking Research Foundation (KK, TF, MM, SO, HM).
Conflict of Interest Statement
The authors declare that the research was conducted in the absence of any commercial or financial relationships that could be construed as a potential conflict of interest.
Supplementary Material
The Supplementary Material for this article can be found online at: https://www.frontiersin.org/articles/10.3389/fimmu.2019.01102/full#supplementary-material
Supplementary Figure 1. Schematic drawing of OVA-, OVAp-, and anti-CD3/CD28 Abs-activated CD4+ T cell differentiation into Tregs and effector T cells (Th1, Th2, and Th17). (A) OVA-activated CD4+ T cell differentiation of DO11.10 spleen cells. OVA endocytosed in antigen presenting cells (APCs) is processed within endosomes by lysosomal enzymes such as γ-interferon-inducible lysosomal thiol reductase (GILT) and cathepsins to OVAp. After binding to MHC class II molecules (MHC II), OVAp-MHC II complex is translocated to the surface of APCs. Recognition of OVAp presented on MHC II by TCR on DO11.10 CD4+ T cells generates “Signal 1” and triggers a series of activation processes leading to differentiation. Along with Signal 1, the interaction with CD80/CD86 co-stimulatory molecules via CD28 (“Signal 2”) enhances the differentiation. Thus, Signals 1 and 2 promote CD4+ T cell differentiation into Tregs and effector T cells. (B) OVA-activated differentiation of DO11.10 spleen cells in the presence of GTS-21. GTS-21 suppresses OVA-activated CD4+ T cell differentiation (Figure 1), most likely by suppressing lysosomal enzyme expression, and thus antigen processing, via stimulation of α7 nAChRs on APCs. This inhibition of antigen processing suppresses antigen presentation and, thus, Signal 1, which triggers CD4+ T cell differentiation. (C) OVAp-activated diffrentiation of DO11.10 spleen cells in the presence of GTS-21. OVAp activated CD4+ T cell development into Tregs and Th1, Th2, and Th17 (Figure 2, GTS-21 at 0 μM). OVAp directly binds to MHC II on the surface of APCs and is then recognized by TCRs on DO11.10 CD4+ T cells, leading to generation of Signals 1 and 2. (D) Anti-CD3/CD28 Abs-activated differentiation of DO11.10 naïve CD4+ T cells in the presence of polarizing cytokines and GTS-21. Anti-CD3/CD28 Abs activates naïve CD4+ T cell differentiation into Tregs and Th1, Th2, and Th17 by binding to CD3 and CD28, which leads to generation of Signals 1 and 2 (Figure 4; GTS-21 at 0 μM). GTS-21 enhances both OVAp- and Anti-CD3/CD28 Abs-activated differentiation and proliferation of Tregs and Th1, Th2, and Th17 by stimulating CD4+ T cell α7 nAChRs (Figure 4; GTS-21 at 30 μM).
Supplementary Figure 2. Contribution of α7 nAChRs expressed on APCs (CD11b+ and CD11c + cells) to the regulation of CD4+ T cell development. To define the role for α7 nAChRs expressed on APCs, naïve CD4+ T cells isolated from OVA-specific TCR transgenic OT-II (H-2b) mice were co-cultured for 5 days with APCs isolated from control WT C57BL/6J or α7-KO mice in the presence of 20 μg/ml OVA with and without GTS-21 (30 μM). (A) Representative flow cytometric plots for CD4+CD25+FoxP3+ T cells (Tregs). (B) Corresponding percentages of OVA-activated Tregs in the presence or absence of GTS-21. Note that OVA induced development into Tregs in both the WT and α7-KO samples, and that GTS-21 suppressed OVA-activated development only in the WT samples. These results suggest that activation of α7 nAChRs expressed on APCs down-regulates antigen presentation, which is a common stimulus for induction of naïve CD4+ T cell activation. Thus, GTS-21 also appears to suppress OVA-activated naïve CD4+ T cell development into effector T cells via α7 nAChRs on APCs. The bars represent means ± SEM for at least three samples. C, control (without OVA).##P < 0.01 vs. C. **P < 0.01 vs. GTS-21 at 0 μM.
References
1. Kawashima K, Fujii T. Extraneuronal cholinergic system in lymphocytes. Pharmacol Ther. (2000) 86:29s−48. doi: 10.1016/S0163-7258(99)00071-6
2. Kawashima K, Fujii T. The lymphocytic cholinergic system and its contribution to the regulation of immune activity. Life Sci. (2003) 74:675–96. doi: 10.1016/j.lfs.2003.09.037
3. Kawashima K, Fujii T. Expression of non-neuronal acetylcholine in lymphocytes and its contribution to the regulation of immune function. Front Biosci. (2004) 9:2063–85. doi: 10.2741/1390
4. Fujii T, Mashimo M, Moriwaki Y, Misawa H, Ono S, Horiguchi K, et al. Expression and function of the cholinergic system in immune cells. Front Immunol. (2017) 8:1085. doi: 10.3389/fimmu.2017.01085
5. Fujii T, Mashimo M, Moriwaki Y, Misawa H, Ono S, Horiguchi K, et al. Physiological functions of the cholinergic system in immune cells. J Pharmacol Sci. (2017) 134:1–21. doi: 10.1016/j.jphs.2017.05.002
6. Rinner I, Kawashima K, Schauenstein K. Rat lymphocytes produce and secrete acetylcholine in dependence of differentiation and activation. J Neuroimmunol. (1998) 81:31–7. doi: 10.1016/S0165-5728(97)00155-0
7. Tallini YN, Shui B, Greene KS, Deng KY, Doran R, Fisher PJ, et al. BAC transgenic mice express enhanced green fluorescent protein in central and peripheral cholinergic neurons. Physiol Genomics. (2006) 27:391–7. doi: 10.1152/physiolgenomics.00092.2006
8. Rosas-Ballina M, Olofsson PS, Ochani M, Valdes-Ferrer SI, Levine YA, Reardon C, et al. Acetylcholine-synthesizing T cells relay neural signals in a vagus nerve circuit. Science. (2011) 334:98–101. doi: 10.1126/science.1209985
9. Gautron L, Rutkowski JM, Burton MD, Wei W, Wan Y, Elmquist JK. Neuronal and nonneuronal cholinergic structures in the mouse gastrointestinal tract and spleen. J Comp Neurol. (2013) 521:3741–67. doi: 10.1002/cne.23376
10. Hoover DB. Cholinergic modulation of the immune system presents new approaches for treating inflammation. Pharmacol Ther. (2017) 179:1–16. doi: 10.1016/j.pharmthera.2017.05.002
11. Fujii T, Watanabe Y, Fujimoto K, Kawashima K. Expression of acetylcholine in lymphocytes and modulation of an independent lymphocytic cholinergic activity by immunological stimulation. Biog Amine. (2003) 17:373–86. doi: 10.1163/15693910260698320
12. Kawashima K, Yoshikawa K, Fujii YX, Moriwaki Y, Misawa H. Expression and function of genes encoding cholinergic components in murine immune cells. Life Sci. (2007) 80:2314–9. doi: 10.1016/j.lfs.2007.02.036
13. Matsueda H, Kawano M, Takagi R, Koshimizu K, Matsushita S. Acetylcholine stored in dendritic cells plays a role in the differentiation of naïve CD4+ T cells into a Th1 phenotype Clin Exp Neuroimmunol. (2018) 9:115–23. doi: 10.1111/cen3.12427
14. Reardon C, Duncan GS, Brustle A, Brenner D, Tusche MW, Olofsson PS, et al. Lymphocyte-derived ACh regulates local innate but not adaptive immunity. Proc Natl Acad Sci USA. (2013) 110:1410–5. doi: 10.1073/pnas.1221655110
15. Sato KZ, Fujii T, Watanabe Y, Yamada S, Ando T, Kazuko F, et al. Diversity of mRNA expression for muscarinic acetylcholine receptor subtypes and neuronal nicotinic acetylcholine receptor subunits in human mononuclear leukocytes and leukemic cell lines. Neurosci Lett. (1999) 266:17–20. doi: 10.1016/S0304-3940(99)00259-1
16. Kawashima K, Fujii T, Moriwaki Y, Misawa H. Critical roles of acetylcholine and the muscarinic and nicotinic acetylcholine receptors in the regulation of immune function. Life Sci. (2012) 91(21-22):1027–32. doi: 10.1016/j.lfs.2012.05.006
17. Kawashima K, Fujii T, Moriwaki Y, Misawa H, Horiguchi K. Non-neuronal cholinergic system in regulation of immune function with a focus on alpha7 nAChRs. Int Immunopharmacol. (2015) 29:127–34. doi: 10.1016/j.intimp.2015.04.015
18. Qian J, Galitovskiy V, Chernyavsky AI, Marchenko S, Grando SA. Plasticity of the murine spleen T-cell cholinergic receptors and their role in in vitro differentiation of naive CD4 T cells toward the Th1, Th2 and Th17 lineages. Genes Immun. (2011) 12:222–30. doi: 10.1038/gene.2010.72
19. Wang H, Yu M, Ochani M, Amella CA, Tanovic M, Susarla S, et al. Nicotinic acetylcholine receptor alpha7 subunit is an essential regulator of inflammation. Nature. (2003) 421:384–8. doi: 10.1038/nature01339
20. Fujii YX, Fujigaya H, Moriwaki Y, Misawa H, Kasahara T, Grando SA, et al. Enhanced serum antigen-specific IgG1 and proinflammatory cytokine production in nicotinic acetylcholine receptor alpha7 subunit gene knockout mice. J Neuroimmunol. (2007) 189:69–74. doi: 10.1016/j.jneuroim.2007.07.003
21. Skok M, Grailhe R, Agenes F, Changeux JP. The role of nicotinic acetylcholine receptors in lymphocyte development. J Neuroimmunol. (2006) 171:86–98. doi: 10.1016/j.jneuroim.2005.09.011
22. Koval L, Lykhmus O, Zhmak M, Khruschov A, Tsetlin V, Magrini E, et al. Differential involvement of alpha4beta2, alpha7 and alpha9alpha10 nicotinic acetylcholine receptors in B lymphocyte activation in vitro. Int J Biochem Cell Biol. (2011) 43:516–24. doi: 10.1016/j.biocel.2010.12.003
23. Koval L, Kalashnyk O, Lykhmus O, Skok M. alpha7 nicotinic acetylcholine receptors are involved in suppression of the antibody immune response. J Neuroimmunol. (2018) 318:8–14. doi: 10.1016/j.jneuroim.2018.01.012
24. Nizri E, Brenner T. Modulation of inflammatory pathways by the immune cholinergic system. Amino Acids. (2013) 45:73–85. doi: 10.1007/s00726-011-1192-8
25. Nizri E, Irony-Tur-Sinai M, Lory O, Orr-Urtreger A, Lavi E, Brenner T. Activation of the cholinergic anti-inflammatory system by nicotine attenuates neuroinflammation via suppression of Th1 and Th17 responses. J Immunol. (2009) 183:6681–8. doi: 10.4049/jimmunol.0902212
26. Galitovskiy V, Qian J, Chernyavsky AI, Marchenko S, Gindi V, Edwards RA, et al. Cytokine-induced alterations of alpha7 nicotinic receptor in colonic CD4 T cells mediate dichotomous response to nicotine in murine models of Th1/Th17- versus Th2-mediated colitis. J Immunol. (2011) 187:2677–87. doi: 10.4049/jimmunol.1002711
27. Meyer EM, Kuryatov A, Gerzanich V, Lindstrom J, Papke RL. Analysis of 3-(4-hydroxy, 2-Methoxybenzylidene)anabaseine selectivity and activity at human and rat alpha-7 nicotinic receptors. J Pharmacol Exp Ther. (1998) 287:918–25.
28. Murphy KM, Heimberger AB, Loh DY. Induction by antigen of intrathymic apoptosis of CD4+CD8+TCRlo thymocytes in vivo. Science. (1990) 250:1720–3.
29. Mashimo M, Iwasaki Y, Inoue S, Saito S, Kawashima K, Fujii T. Acetylcholine released from T cells regulates intracellular Ca2+, IL-2 secretion and T cell proliferation through nicotinic acetylcholine receptor. Life Sci. (2017) 172:13–8. doi: 10.1016/j.lfs.2016.12.015
30. Flaherty S, Reynolds JM. Mouse Naive CD4+ T cell isolation and in vitro differentiation into T Cell Subsets. J Vis Exp. (2015) 98:e52739. doi: 10.3791/52739
31. Poulter LW. Antigen presenting cells in situ: their identification and involvement in immunopathology. Clin Exp Immunol. (1983) 53:513–20.
32. Fujii T, Takada-Takatori Y, Kawashima K. Regulatory mechanisms of acetylcholine synthesis and release by T cells. Life Sci. (2012) 91:981–5. doi: 10.1016/j.lfs.2012.04.031
33. Fujii T, Tsuchiya T, Yamada S, Fujimoto K, Suzuki T, Kasahara T, et al. Localization and synthesis of acetylcholine in human leukemic T cell lines. J Neurosci Res. (1996) 44:66–72. doi: 10.1002/(SICI)1097-4547(19960401)44:1<66::AID-JNR9>3.0.CO;2-G
34. Arredondo J, Chernyavsky AI, Webber RJ, Grando SA. Biological effects of SLURP-1 on human keratinocytes. J Invest Dermatol. (2005) 125:1236–41. doi: 10.1111/j.0022-202X.2005.23973.x
35. Chimienti F, Hogg RC, Plantard L, Lehmann C, Brakch N, Fischer J, et al. Identification of SLURP-1 as an epidermal neuromodulator explains the clinical phenotype of Mal de Meleda. Hum Mol Genet. (2003) 12:3017–24. doi: 10.1093/hmg/ddg320
36. Fujii T, Horiguchi K, Sunaga H, Moriwaki Y, Misawa H, Kasahara T, et al. SLURP-1, an endogenous alpha7 nicotinic acetylcholine receptor allosteric ligand, is expressed in CD205(+) dendritic cells in human tonsils and potentiates lymphocytic cholinergic activity. J Neuroimmunol. (2014) 267:43–9. doi: 10.1016/j.jneuroim.2013.12.003
37. Lackman RL, Jamieson AM, Griffith JM, Geuze H, Cresswell P. Innate immune recognition triggers secretion of lysosomal enzymes by macrophages. Traffic. (2007) 8:1179–89. doi: 10.1111/j.1600-0854.2007.00600.x
38. Barjaktarević I, Rahman A, Radoja S, Bogunović B, Vollmer A, Vukmanović S, et al. Inhibitory role of IFN-gamma-inducible lysosomal thiol reductase in T cell activation. J Immunol. (2006) 177:4369–75. doi: 10.4049/jimmunol.177.7.4369
39. Pilzner C, Bühling F, Reinheckel T, Chwieralski C, Rathinasamy A, Lauenstein HD, et al. Allergic airway inflammation in mice deficient for the antigen-processing protease cathepsin E. Int Arch Allergy Immunol. (2012) 159:367–83. doi: 10.1159/000338288
40. Burster T, Giffon T, Dahl ME, Bjorck P, Bogyo M, Weber E, et al. Influenza A virus elevates active cathepsin B in primary murine DC. Int Immunol. (2007) 19:645–55. doi: 10.1093/intimm/dxm030
41. Villadangos JA, Ploegh HL. Proteolysis in MHC class II antigen presentation: who's in charge? Immunity. (2000) 12:233–9. doi: 10.1016/S1074-7613(00)80176-4
42. Lopes C, Pereira EF, Wu HQ, Purushottamachar P, Njar V, Schwarcz R, et al. Competitive antagonism between the nicotinic allosteric potentiating ligand galantamine and kynurenic acid at alpha7* nicotinic receptors. J Pharmacol Exp Ther. (2007) 322:48–58. doi: 10.1124/jpet.107.123109
43. Hanes WM, Olofsson PS, Kwan K, Hudson LK, Chavan SS, Pavlov VA, et al. Galantamine attenuates type 1 diabetes and inhibits anti-insulin antibodies in nonobese diabetic mice. Mol Med. (2015) 21:702–8. doi: 10.2119/molmed.2015.00142
44. Sitapara RA, Antoine DJ, Sharma L, Patel VS, Ashby CR Jr, Gorasiya S, et al. The alpha7 nicotinic acetylcholine receptor agonist GTS-21 improves bacterial clearance in mice by restoring hyperoxia-compromised macrophage function. Mol Med. (2014) 20:238–47. doi: 10.2119/molmed.2013.00086
45. Fujii T, Yamada S, Watanabe Y, Misawa H, Tajima S, Fujimoto K, et al. Induction of choline acetyltransferase mRNA in human mononuclear leukocytes stimulated by phytohemagglutinin, a T-cell activator. J Neuroimmunol. (1998) 82:101–7. doi: 10.1016/S0165-5728(97)00195-1
46. Razani-Boroujerdi S, Boyd RT, Davila-Garcia MI, Nandi JS, Mishra NC, Singh SP, et al. T cells express alpha7-nicotinic acetylcholine receptor subunits that require a functional TCR and leukocyte-specific protein tyrosine kinase for nicotine-induced Ca2+ response. J Immunol. (2007) 179:2889–98. doi: 10.4049/jimmunol.179.5.2889
47. Stein PH, Singer A. Similar co-stimulation requirements of CD4+ and CD8+ primary T helper cells: role of IL-1 and IL-6 in inducing IL-2 secretion and subsequent proliferation. Int Immunol. (1992) 4:327–35.
48. Eto D, Lao C, DiToro D, Barnett B, Escobar TC, Kageyama R, et al. IL-21 and IL-6 are critical for different aspects of B cell immunity and redundantly induce optimal follicular helper CD4 T cell (Tfh) differentiation. PLoS ONE. (2011) 6:e17739. doi: 10.1371/journal.pone.0017739
49. Austin JW, Lu P, Majumder P, Ahmed R, Boss JM. STAT3, STAT4, NFATc1, and CTCF regulate PD-1 through multiple novel regulatory regions in murine T cells. J Immunol. (2014) 192:4876–86. doi: 10.4049/jimmunol.1302750
50. O'Shea JJ, Murray PJ. Cytokine signaling modules in inflammatory responses. Immunity. (2008) 28:477–87. doi: 10.1016/j.immuni.2008.03.002
51. Chaudhry A, Rudra D, Treuting P, Samstein RM, Liang Y, Kas A, et al. CD4+ regulatory T cells control TH17 responses in a Stat3-dependent manner. Science. (2009) 326:986–91. doi: 10.1126/science.1172702
52. Eddahri F, Denanglaire S, Bureau F, Spolski R, Leonard WJ, Leo O, et al. Interleukin-6/STAT3 signaling regulates the ability of naive T cells to acquire B-cell help capacities. Blood. (2009) 113:2426–33. doi: 10.1182/blood-2008-04-154682
53. Ray JP, Marshall HD, Laidlaw BJ, Staron MM, Kaech SM, Craft J. Transcription factor STAT3 and type I interferons are corepressive insulators for differentiation of follicular helper and T helper 1 cells. Immunity. (2014) 40:367–77. doi: 10.1016/j.immuni.2014.02.005
54. Stritesky GL, Muthukrishnan R, Sehra S, Goswami R, Pham D, Travers J, et al. The transcription factor STAT3 is required for T helper 2 cell development. Immunity. (2011) 34:39–49. doi: 10.1016/j.immuni.2010.12.013
55. Vinuesa CG, Linterman MA, Yu D, MacLennan IC. Follicular Helper T Cells. Annu Rev Immunol. (2016) 34:335–68. doi: 10.1146/annurev-immunol-041015-055605
56. Yang XO, Panopoulos AD, Nurieva R, Chang SH, Wang D, Watowich SS, et al. STAT3 regulates cytokine-mediated generation of inflammatory helper T cells. J Biol Chem. (2007) 282:9358–63. doi: 10.1074/jbc.C600321200
57. de Jonge WJ, van der Zanden EP, The FO, Bijlsma MF, van Westerloo DJ, Bennink RJ, et al. Stimulation of the vagus nerve attenuates macrophage activation by activating the Jak2-STAT3 signaling pathway. Nat Immunol. (2005) 6:844–51. doi: 10.1038/ni1229
58. Arredondo J, Chernyavsky AI, Jolkovsky DL, Webber RJ, Grando SA. SLURP-2: a novel cholinergic signaling peptide in human mucocutaneous epithelium. J Cell Physiol. (2006) 208:238–45. doi: 10.1002/jcp.20661
59. Chernyavsky AI, Arredondo J, Galitovskiy V, Qian J, Grando SA. Upregulation of nuclear factor-kappaB expression by SLURP-1 is mediated by alpha7-nicotinic acetylcholine receptor and involves both ionic events and activation of protein kinases. Am J Physiol Cell Physiol. (2010) 299:C903–11. doi: 10.1152/ajpcell.00216.2010
Keywords: α7 nAChR, DO11. 10 mouse, GTS-21, regulatory T cells, Th1, Th2, Th17
Citation: Mashimo M, Komori M, Matsui YY, Murase MX, Fujii T, Takeshima S, Okuyama H, Ono S, Moriwaki Y, Misawa H and Kawashima K (2019) Distinct Roles of α7 nAChRs in Antigen-Presenting Cells and CD4+ T Cells in the Regulation of T Cell Differentiation. Front. Immunol. 10:1102. doi: 10.3389/fimmu.2019.01102
Received: 01 November 2018; Accepted: 30 April 2019;
Published: 31 May 2019.
Edited by:
Anne Cooke, University of Cambridge, United KingdomReviewed by:
David Bending, University of Birmingham, United KingdomNick Holmes, University of Cambridge, United Kingdom
Copyright © 2019 Mashimo, Komori, Matsui, Murase, Fujii, Takeshima, Okuyama, Ono, Moriwaki, Misawa and Kawashima. This is an open-access article distributed under the terms of the Creative Commons Attribution License (CC BY). The use, distribution or reproduction in other forums is permitted, provided the original author(s) and the copyright owner(s) are credited and that the original publication in this journal is cited, in accordance with accepted academic practice. No use, distribution or reproduction is permitted which does not comply with these terms.
*Correspondence: Koichiro Kawashima, koichiro-jk@piano.ocn.ne.jp; kawashimak@pharm.kitasato-u.ac.jp