- 1The Research Institute at Nationwide Children's Hospital, Columbus, OH, United States
- 2Division of Hematology/Oncology, Nationwide Children's Hospital, Columbus, OH, United States
- 3Department of Pediatrics, College of Medicine, The Ohio State University, Columbus, OH, United States
- 4Division of Rheumatology, Allergy and Immunology, The University of North Carolina at Chapel Hill, Chapel Hill, NC, United States
- 5Division of Rheumatology, Nationwide Children's Hospital, Columbus, OH, United States
- 6Division of Biostatistics, College of Public Health, The Ohio State University, Columbus, OH, United States
- 7Department of Microbiology and Immunology, Loyola University Chicago, Maywood, IL, United States
APS is the association of antiphospholipid antibodies (aPL) with thromboses and/or recurrent pregnancy loss (RPL). Among patients with SLE, one-third have aPL and 10–15% have a manifestation of secondary APS. Animal studies suggested that complement activation plays an important role in the pathogenesis of thrombosis and pregnancy loss in APS. We performed a cross-sectional study on complement proteins and genes in 525 patients with aPL. Among them, 237 experienced thromboses and 293 had SLE; 111 had both SLE and thromboses, and 106 had neither SLE nor thrombosis. Complement protein levels were determined by radial immunodiffusion for C4, C3 and factor H; and by functional ELISA for mannan binding lectin (MBL). Total C4, C4A and C4B gene copy numbers (GCN) were measured by TaqMan-based realtime PCR. Two to six copies of C4 genes are frequently present in a diploid genome, and each copy may code for an acidic C4A or a basic C4B protein. We observed significantly (a) higher protein levels of total C4, C4A, C4B, C3, and anticardiolipin (ACLA) IgG, (b) increased frequencies of lupus anticoagulant and males, and (c) decreased levels of complement factor H, MBL and ACLA-IgM among patients with thrombosis than those without thrombosis (N = 288). We also observed significantly lower GCNs of total C4 and C4A among aPL-positive patients with both SLE and thrombosis than others. By contrast, aPL-positive subjects with SLE had significantly reduced protein levels of C3, total C4, C4A, C4B and ACLA-IgG, and higher frequency of females than those without SLE. Patients with thrombosis but without SLE (N = 126), and patients with SLE but without thrombosis (N = 182) had the greatest differences in mean protein levels of C3 (p = 2.6 × 10−6), C4 (p = 2.2 × 10−9) and ACLA-IgG (p = 1.2 × 10−5). RPL occurred in 23.7% of female patients and thrombotic SLE patients had the highest frequency of RPL (41.0%; p = 3.8 × 10−10). Compared with non-RPL females, RPL had significantly higher frequency of thrombosis and elevated C4 protein levels. Female patients with homozygous C4A deficiency all experienced RPL (p = 0.0001) but the opposite was true for patients with homozygous C4B deficiency (p = 0.017). These results provide new insights and biomarkers for diagnosis and management of APS and SLE.
Introduction
Antiphospholipid syndrome (APS) is characterized by vascular thrombosis and/or pregnancy morbidity such as recurrent fetal loss in the persistent presence of antiphospholipid antibodies (aPL) (1–7). aPL are a heterogeneous group of autoantibodies that include antibodies against phospholipid binding protein β2-glycoprotein I (β2GPI), anticardiolipin antibodies (ACLA), and lupus anticoagulant (LAC) (8). Human subjects with triple positivity for all three groups of aPL appeared to be at high risk to experience recurrent thromboembolic events (9). A majority of clinical tests for aPL detects antibodies against β2GPI. β2GPI is a plasma protein consisting of five structural domains known as short consensus repeats that are characteristic features of controlling proteins for the complement system (10–12).
SLE is a common autoimmune disease associated with APS. SLE features the generation of autoantibodies against nuclear antigens including double-stranded DNA (13, 14). In a study of European APS patients, over 40% were found to have SLE or a lupus-like disease (15). Among the general SLE population, between 30 and 40% have aPL; and 10–15% of patients with SLE also have clinical manifestations of APS (15–20). In addition to the presence of autoantibodies, hypocomplementemia is another hallmark of human SLE (21–25). Low serum complement levels for C4 and C3 in patients with SLE can be triggered by a combination of heritable and acquired factors: genetic deficiencies, low copy number of complement C4 genes, robust consumption caused by immune complex-mediated complement activation, or the presence of inhibitors that inactivate or prevent accessibility. A complete genetic deficiency in any one of the early components specific for the classical complement activation pathway almost always lead to pathogenesis of human SLE, inferring that an intact classical pathway of the complement system is essential for the protection against systemic autoimmunity (26–28).
Activations of complement C3 and C5 in the presence of antigen-antibody complexes occur via the formation of the C1 complex (C1q-C1r2-C1s2), followed by the activations of C4 and C2 to form C4b and C2a, respectively (29). C4b and C2a are subunits of the C3 and C5 convertases, essential for the classical and lectin activation pathways (26). There are two isotypes of native C4 proteins. C4A is the acidic isotype believed to play an essential role in immune clearance and immunotolerance. C4B is the basic isotype that is capable of rapid propagation of complement activation (30–34). In a diploid genome, complement C4 gene copy number varies among different individuals. Two to eight copies of C4 genes are generally present in a diploid genome among most human subjects (35, 36). Each C4 gene either codes for a C4A or a C4B protein. Such gene copy number variation contributes to quantitative and qualitative diversities in C4 protein levels and function, and therefore different intrinsic strengths for effector functions of innate and adaptive immune responses (25, 34, 36–40). Among European and East-Asian subjects, low copy number of total C4 or C4A is a risk factor for SLE, while high copy number of total C4 or C4A is protective against susceptibility to SLE (22, 38, 41, 42).
An injection of human aPL into animal models including wild-type mice induced an increase in thrombus size (43, 44). An injection of human aPL into pregnant mice resulted in fetal resorption. (45, 46). Mice deficient in complement C3 or C5, as well as mice injected with a monoclonal antibody against C5, did not exhibit an increase in thrombus size in the presence of aPL. Blockade of complement activation by genetic deletion of C3 or C4, or with transgenic insertion of complement regulatory protein Crry-Ig, a soluble inhibitor of mouse C3 convertase, protected mice, rats or hamsters from pregnancy complications induced by injections of human aPL (45, 47–56). These phenomena suggest that complement proteins or their activated products are engaged in the pathogenesis of APS, as they probably provide immune effectors for aPL-mediated thromboses, tissue injury and/or fetal loss in mouse models. The generation of immune complexes between aPL and ligands (such as β2GPI binding to phospholipids) leads to activation of the complement classical pathway, release of C5a and C3a anaphylatoxins (50, 57, 58), which may attract neutrophils and other granulocytes to the site of complement activation, increase vascular permeability, and elicit inflammatory response that contributes to tissue injuries including pregnancy morbidity. Culmination of complement activation pathways leads to the assembly of the membrane attack complex (C5b-9) and provides the “second-hit” to trigger vascular thrombosis (53). Consistent with this notion, it was shown that C3, C5, or C6-deficient rodents were protected from aPL induced thrombosis (56, 59). Such protective effects of complement deficiency in APS-associated disorders observed in animal models are opposite to the causal effects of deficiencies in early components for the classical complement pathway in human lupus (26, 60). Among human patients with APS, elevated levels of complement activation products (C4a, C3a, C5a, C5b-9) have been demonstrated (55, 61, 62). However, systematic and meticulous studies on how complement proteins and genes contribute to the pathology of human APS (recurrent vascular thrombosis or pregnancy morbidity) and the concurrence of SLE and APS were scarce or limited by small sample size.
Here we performed a cross-sectional study on 525 human subjects with aPL from the Antiphospholipid Syndrome Collaborative Registry. Based on clinical presentations of thrombosis and SLE, these subjects were categorized to four groups: patients with thrombosis only (To), with thrombosis and SLE (TS), with SLE only (So), and without thrombosis and without SLE (NTS). Plasma protein concentrations for complement total C4, C4A, C4B, C3, factor H, and functional mannan binding lectin (MBL) were measured. Total C4, C4A and C4B gene copy numbers were elucidated. The results reveal substantial phenotypic differences for complement protein concentrations among patients with thromboses or recurrent pregnancy loss, and SLE. There was also a significant difference in C4 gene copy number variations between patients with both thrombosis and SLE, and patients without SLE and thrombosis.
Patients and Methods
Study Population
This study was approved by the Institutional Review Board at Nationwide Children's Hospital. Peripheral blood plasma and matched genomic DNA samples without personal identifiers from 525 patients with aPL and clinical status were provided by the APS Core at University of North Carolina (8, 63). These aPL-positive patients were recruited with written informed consent. Of these patients, 444 (84.57%) were female and 81 (15.43%) were male. The mean age (±SD) was 45.01 ± 12.97 years old. Among these aPL-positive patients, 184 (35.05%) met the Sapporo criteria for definite APS (1); an additional 175 subjects (33.33%) met the extended definition of APS, and 166 asymptomatic subjects (31.6%) who had aPL but no manifestations of thrombosis or pregnancy morbidity. Patients with definite APS as defined by the preliminary or modified Sapporo criteria (1, 6) must have one or more clinical episodes of vascular thrombosis and/or pregnancy morbidity as well as ACLA, anti-β2GPI IgG and/or IgM or LAC present on two or more occasions at least 6 weeks apart. The expanded APS group was defined by institutions participating in APSCORE and include those patients with one or more clinical manifestations characteristic of APS but not fulfilling the strict definition and either the Sapporo laboratory criteria or one of a group of APS-associated autoantibodies. Asymptomatic patients fulfill the Sapporo laboratory criteria but have no clinical manifestations related to APS.
To perform refined analyses based on clinical presentations, we segregated the aPL subjects based on the presence and absence of thrombosis, SLE and recurrent pregnancy loss. Among the study cohort, 237 subjects had a history of thrombosis and 288 subjects did not have thrombosis. A total of 293 subjects were diagnosed with SLE according to the American College of Rheumatology criteria (64) and 232 subjects did not have a diagnosis of SLE at the time of recruitment. Of the 444 female subjects with aPL, 106 (23.87%) experienced recurrent pregnancy loss.
Quantifications of Total C4, C4A, and C4B Genes by Real-Time PCR
A series of real-time PCR assays was applied to determine the copy number variations of total C4, C4A, and C4B genes (35). All real time PCR assays used the TaqMan MGB probes (ABI). The target probes (C4, C4A, and C4B) were VIC-labeled. The endogenous control probe, which targeted an invariant exon 4 of the RP1 gene, was FAM-labeled. Each reaction consisted of 0.5 to 1 μM of both forward and reverse primers for the target and control amplicons, 100 nM of the target and endogenous control probes, 25 ng of sample DNA and TaqMan Universal PCR master mix (ABI, PN 4323018). All assays were performed in triplicates using the ABI 7500 RT-PCR system per manufacturer's recommendations. The relative standard curve method was utilized to calculate the copy number of each target gene. The accuracy of C4A and C4B gene copy number assignments for each sample was cross-confirmed as the gene copy number of total C4 equals the sum of C4A and C4B.
Complement C3, C4, Factor H (CFH) and Mannan Binding Lectin (MBL) Protein Concentrations in Citrate-Plasma
Platelet poor plasma samples were processed with a consistent protocol. Briefly, blood samples in citrate tubes were centrifuged at 1,500 g for 10 min, at 4–8°C. Plasma samples were transferred to microcentrifuge tubes and spun again at 2,000 g for 5 min. Aliquots were kept frozen at −80°C. Plasma protein concentrations of complement C3 and C4 were determined by single radial immunodiffusion assays using commercial kits from The Binding Site (Birmingham, United Kingdom). A comparison of C4 protein concentrations of (a) SLE patients without thrombosis from this study and (b) an independent cross-sectional study of Ohio SLE (38) revealed that protein concentrations of complement C4 assayed from platelet-poor citrate plasma, which were subjected to two rounds of centrifugation, were ~14.5% lower than that of EDTA-plasma C4 harvested after a single round of centrifugation.
Complement factor H plasma protein concentrations were measured using homemade RID plates according to a standard protocol (65). Plasma concentrations for MBL were determined using a functional assay kit from the Antibody Shop (Denmark).
Complement C4 Protein Allotyping
Plasma C4A and C4B protein allotypes were determined by immunofixation and immunoblot techniques, as described previously (66–68). The relative band intensities of C4A and C4B allotypes from each sample were quantified by ImageQuant Software. The corresponding plasma C4A and C4B protein concentrations were calculated from the total C4 protein concentrations.
Statistical Analyses
Descriptive statistics, including means, standard deviations (SD), and 95% confidence intervals (95% CI) were computed for numeric data, and frequency distributions were determined for categorical variables, using statistical software JMP13 (SAS Institute) and GraphPad Prism6 software. Two group comparisons were based on t-tests that accounted for unequal variances if appropriate. Specifically, Tukey HSD test with an alpha set at 0.05 was applied, and was followed by pairwise Student's t-tests that yielded p-values. Dunnett's test with an alpha of 0.05 was applied for comparing study groups to controls. Categorical data were compared by χ2 analyses and odds ratios were calculated whenever appropriate.
To allow a standardized comparison of all continuous parameters contributing to thrombosis without SLE, SLE without thrombosis, SLE with thrombosis, and no thrombosis and no SLE, we determined the root mean square error (RMSE) of each parameter in these four groups by analysis of variance (ANOVA). The difference in the mean protein levels for each protein between any two groups divided by its RMSE to give the effect size index (69). The mean values of parameters in the NTS group were used as references and the effect size indices for To, So, and TS groups were graphically plotted. This enabled a uniform depiction on effects of all continuous parameters under different clinical conditions.
Stepwise multiple logistic regression analyses were used to identify independent parameters significant for clinical outcomes: thrombosis, arterial thrombosis, venous thrombosis, pregnancy loss, and SLE. Such analyses allowed controlled studies for continuous and categorical parameters. For analyses of a clinical presentation as a response, we first put all parameters studied [i.e., C3 or C4, factor H, MBL, ACLA-IgM, ACLA-IgG, BMI, age, gender (F/M), LAC (presence or absence), SLE (presence or absence)] into the regression model. Those that did not give a p-value smaller than 0.1 were removed from the subsequent analyses. The last best model with parameters represented by p-values smaller than 0.05 was maintained and presented. Unit Odds Ratio (OR) and range OR were computed. Parameters that could not coexist in the regression model because of strong correlation (e.g., C3 and C4) were put into the regression models separately and the stronger parameter was kept. When C4 was identified as a significant parameter in a model, we further asked whether C4A or C4B or both C4 isotypes were playing a major role.
Results
The study population consisted of 525 human subjects with antiphospholipid antibodies (aPL), recruited through the APSCORE. The mean age of subjects at the time of recruitment was 45.0 ± 13.0 (mean ± SD) years old. These study subjects were initially segregated into three groups: definite APS, extended APS, and non-APS based on clinical manifestations associated with APS, which included vascular thromboses and pregnancy morbidity. Results for an initial characterization for these three groups of patients are shown in Table 1. One remarkable feature emerged was the steady and highly significant increase in the mean protein concentration of complement C4 from non-APS (16.6 ± 8.8 mg/dL), to extended APS (20.0 ± 9.2 mg/dL), and definitive APS (22.9 ± 10.0 mg/dL) (p = 2.6 × 10−8). These three groups of patients had different frequencies of SLE, thromboses and pregnancy morbidities. Thus, we set to examine quantitative variations of plasma complement proteins among aPL subjects with different clinical manifestations for thrombosis and/or SLE in both female and male patients, and recurrent pregnancy loss among female patients. The demographic and clinical features for these aPL-positive subjects are shown in Table 2.
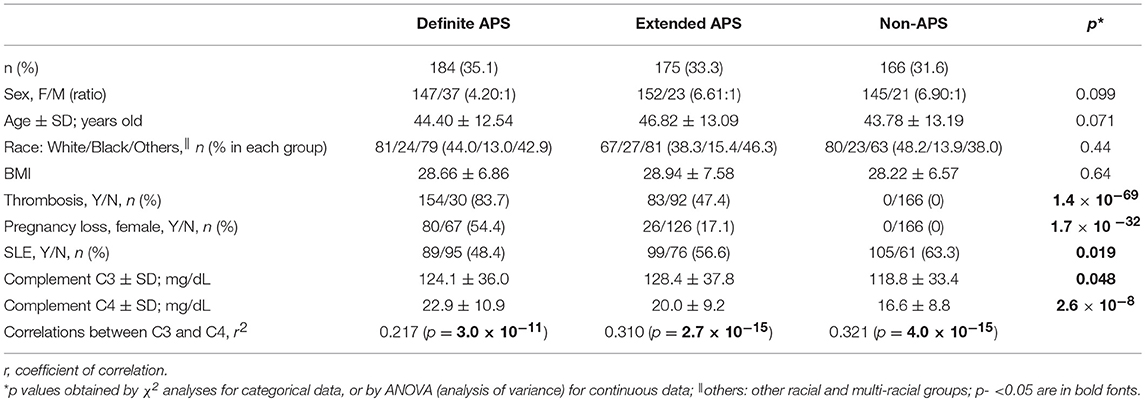
Table 1. Demographics of study populations: aPL patients with definite APS, extended APS and non-APS.
Variations of Plasma Complement Protein and ACLA Levels in aPL-Positive Subjects With and Without Thromboses
Among the aPL-positive subjects, 45.1% had a past history of thrombosis, and 55.8% were diagnosed with SLE at the time of recruitment. When the mean plasma complement protein concentrations and aPL between thrombotic and non-thrombotic groups were compared, highly significant phenotype differences for total C4, C4A, C4B, MBL, ACLA-IgM, and ACLA-IgG were observed (Table 3). The mean protein level (and 95% confidence interval) for total C4 was 22.7 (21.3–24.0) mg/dL in the thrombotic group, and 17.7 (16.6–18.7) mg/dL in the non-thrombotic group, which represented a difference of 28.2% (p = 1.3 × 10−8).
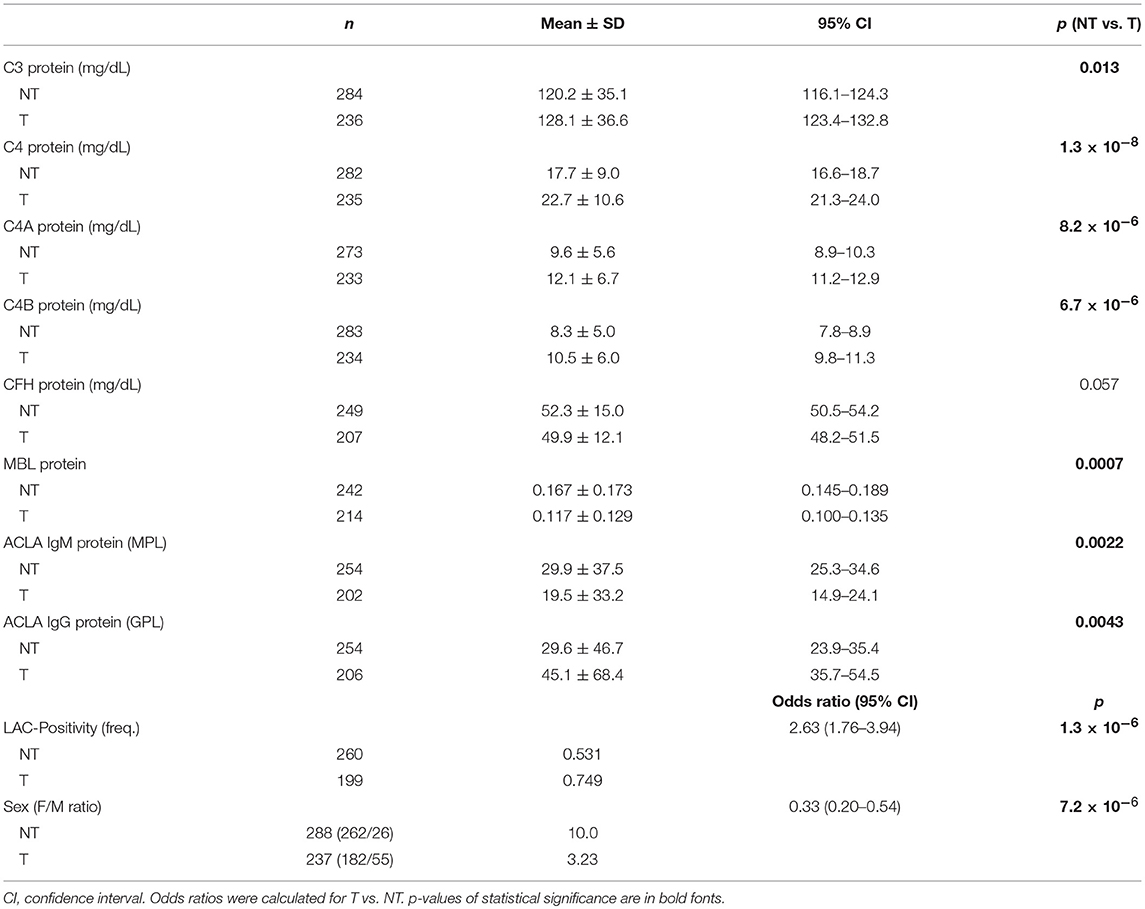
Table 3. Mean plasma complement and ACLA protein levels in aPL-positive subjects with (T) and without thrombosis (NT).
Parallel increases in complement C4A and C4B were observed in the thrombotic group. Mean concentration of plasma C4A in the thrombotic group was 12.1 (11.2–12.9) mg/dL, and 9.6 (8.9–10.3) mg/dL in the non-thrombotic group (p = 8.2 × 10−6). Plasma C4B mean protein concentration in the thrombotic group was 10.5 (9.8–11.3) mg/dL, and 8.3 (7.8–8.9) mg/dL in the non-thrombotic group (p = 6.7 × 10−6). For complement C3, moderately higher mean protein level was observed in the thrombotic group than the non-thrombotic group (T: 128.1 mg/dL; NT: 120.2 mg/dL; p = 0.013).
By contrast, mean plasma protein level for functional MBL among thrombotic subjects was significantly lower than that of non-thrombotic subjects, which were 0.117 (0.100–0.135) mg/dL and 0.167 (0.145–0.189) mg/dL, respectively (p = 0.0007). Slightly lower levels of factor H protein were also observed in the thrombotic group (T: 49.9 mg/dL; NT: 52.3 mg/dL; p = 0.057).
The mean values of anticardiolipn antibodies among thrombotic subjects were 19.5 (14.9–24.1) units for ACLA-IgM and 45.1 (35.7–54.5) units for ACLA-IgG. In non-thrombotic subjects, the corresponding values were 29.9 (25.3–34.6) and 29.6 (23.9–35.4) units (p = 0.0022 for ACLA-IgM; p = 0.0043 for ACLA-IgG). Thrombotic subjects had lower levels of ACLA-IgM but higher levels of ACLA-IgG. Three-quarters (74.9%) of thrombotic subjects tested positive for the presence of lupus anticoagulant (LAC), compared to slightly over one-half (53.3%) among non-thrombotic subjects (p = 1.7 × 10−6).
Thrombotic subjects had significantly lower female to male ratio (3.23 to 1) when compared with non-thrombotic subjects (10.0 to 1; p = 7.2 × 10−6).
Plasma Complement Protein and ACLA Levels in aPL-Positive Subjects With and Without SLE
Quantitative variations of complement and ACLA plasma protein were compared between the aPL-positive patients with and without SLE (Table 4). The mean C3 concentrations were 118.3 (114.2–122.4) mg/dL in SLE and 130.8 (126.2–135.4) mg/dL in non-SLE, which represented a reduction of 9.6% of mean C3 level in SLE (p = 8 × 10−5). The mean total C4 concentrations were 18.6 (17.4–19.8) mg/dL in SLE and 21.6 (20.4–22.9) mg/dL in non-SLE, which corresponded to a reduction of 13.9% of total C4 in SLE (p = 0.0006). The mean C4A and C4B concentrations were 10.0 (9.2–10.8) mg/dL and 8.7 (8.1–9.4) mg/dL, respectively, in the SLE group; and were 11.6 (10.9–12.4) mg/dL and 10.1 (9.4–10.8) mg/dL, respectively, in the non-SLE group (p = 0.0038 for C4A; p = 0.005 for C4B). Thus, lower plasma levels of complement C3 and C4 were conspicuous in the SLE group.
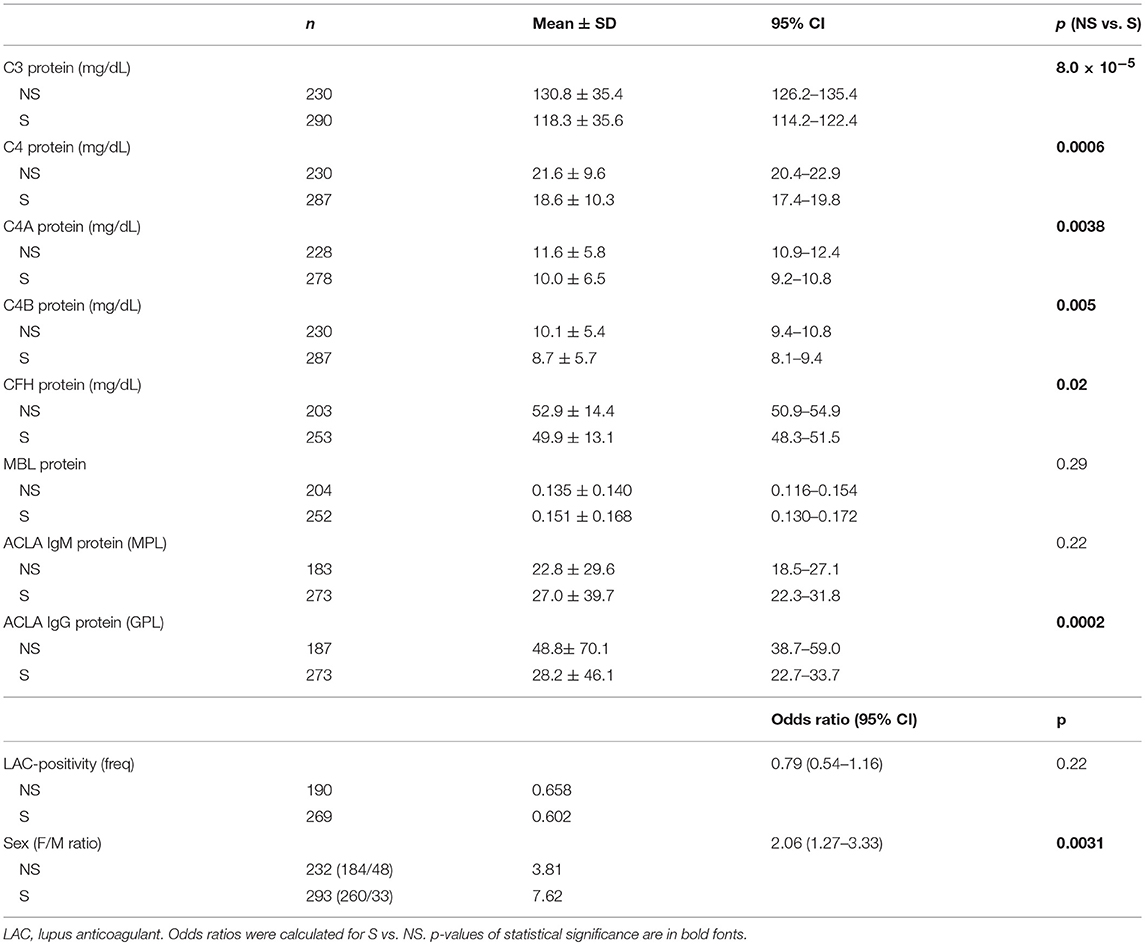
Table 4. Mean plasma protein concentrations of complement and ACLA in aPL-positive subjects with (S) and without (NS) SLE.
The mean plasma protein levels of MBL between the SLE and non-SLE groups were not significantly different [S: 0.151 (0.130–0.172) mg/dL, NS: 0.135 (0.116–0.154) mg/dL; p = 0.29]. The mean plasma protein level of factor H was slightly lower in the SLE group [49.9 (48.3–51.5) mg/dL] than that in the non-SLE group [52.9 (50.9–54.9) mg/dL; p = 0.02].
The mean ACLA-IgG level was significantly lower in the SLE than non-SLE [S: 28.2 (22.7–33.7) GPL, NS: 48.8 (38.7–59.0); p = 0.0002]. By contrast, the mean ACLA-IgM levels and the frequency for the presence of LAC were similar between the SLE and the non-SLE groups.
Among the aPL subjects, SLE patients had a higher female to male ratio (7.62 to 1) than non-SLE patients (3.81 to 1; p = 0.0031).
Differential Plasma Protein Levels of Complement and ACLA in aPL-Positive Subjects With Thrombosis, SLE, Both Thrombosis and SLE, and Neither Thrombosis Nor SLE
Our study results revealed higher levels of mean plasma complement C4, C3, and ACLA-IgG in aPL-positive subjects with a history of thrombosis, but lower levels of complement C4, C3, and ACLA-IgG among aPL-positive subjects with SLE. A proportion (37.9%) of aPL-positive subjects diagnosed with SLE also experienced thromboses. To distinguish the roles of complement proteins and ACLA in thromboses and SLE, we segregated the study subjects according to their thrombosis and SLE status: thrombosis only (To), thrombotic SLE (TS), SLE only (So), and no thrombosis and no SLE (NTS) (Figure 1, Table 5). Among these four groups, significantly higher mean protein levels were observed for patients with thrombosis only than with SLE only for plasma protein levels of total C4 (p = 2.2 × 10−9), C4A (p = 1.9 × 10−6), C4B (p = 1.3 × 10−6), C3, (p = 2.6 × 10−5), ACLA-IgG (p = 1.2 × 10−5), and in female to male ratio sex ratio (p = 1.6 × 10−6).
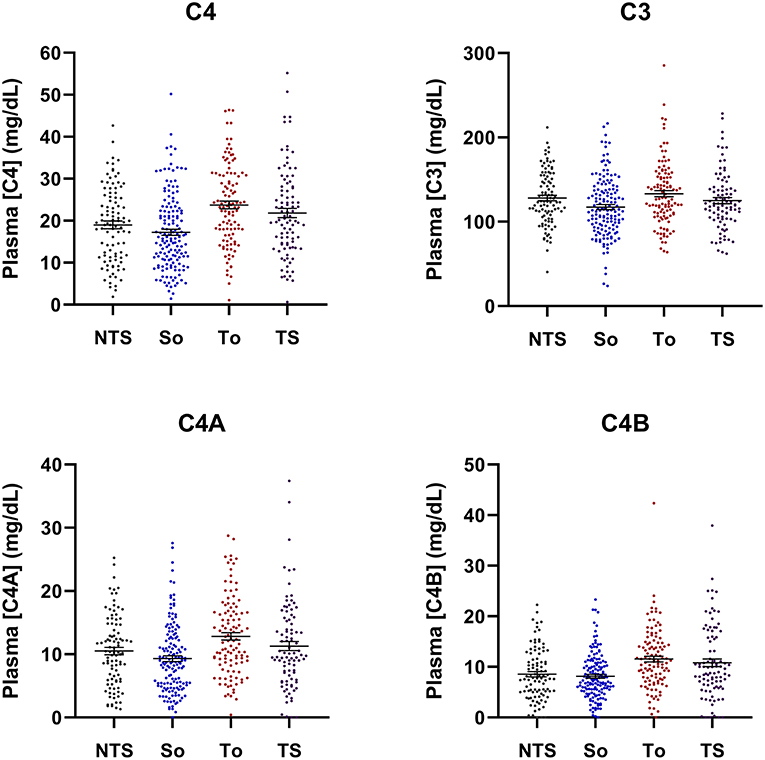
Figure 1. Scattered-plots of complement C4 and C3 plasma protein concentrations in aPL-positive subjects segregated by thrombosis and SLE status and compared. NTS, no thrombosis and no SLE; So, SLE only, To, thrombosis only; TS, with both thrombosis and SLE. Horizontal bars represent means and standard errors. Overall p-values are: 2.8 × 10−7 for C4; 0.0024 for C3; 5.9 × 10−5 for C4A; and 2.7 × 10−6 for C4B.
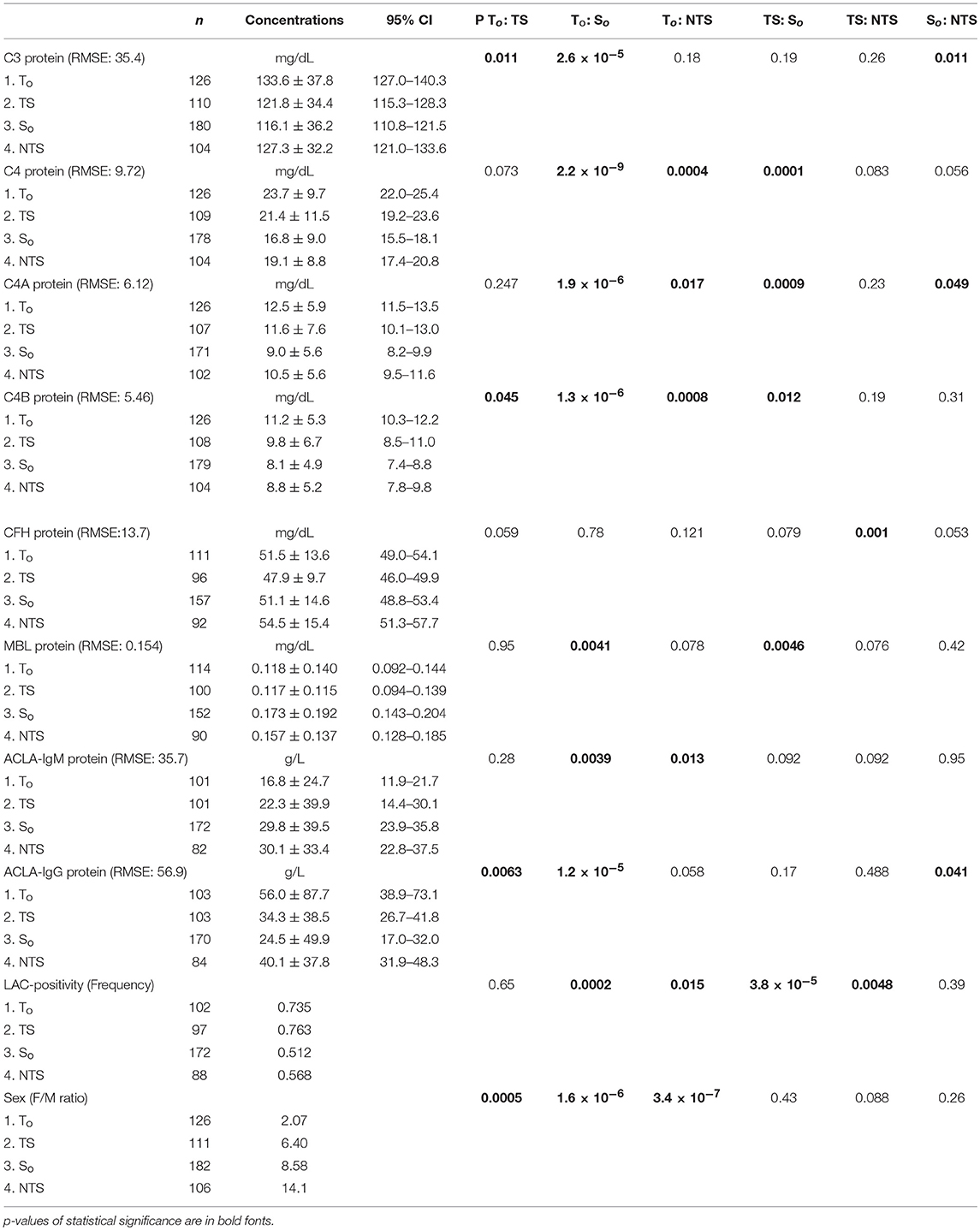
Table 5. Mean plasma protein levels of complement and ACLA in aPL-positive subjects segregated by thrombosis and SLE status.
For functional MBL and the presence of LAC, main differences were observed between the thrombotic groups and the non-thrombotic groups. Mean MBL protein levels were significantly lower in To and TS than in So (To vs. So, p = 0.0041; TS vs. So, p = 0.0046). The mean level of MBL in aPL-positive subjects without thrombosis and SLE (NTS) was slightly higher than those of To and TS (p = 0.078 and 0.076), and similar to that of So (p = 0.416). For lupus anticoagulant, 73.5% of patients in To and 76.3% of patients in TS were tested positive, compared to 51.2% in So (To vs. So, p = 0.0002; TS vs. So, p = 0.000038) and 56.8% in NTS (To vs. NTS, p = 0.015; TS vs. NTS, p = 0.0048).
Gene Copy Number Variations of Total C4, C4A, and C4B in Patients With aPL
Gene copy numbers (GCN) for total C4, C4A, and C4B from 472 aPL-positive subjects were elucidated by TaqMan based real-time PCR using genomic DNA (35). The copy number of total C4 genes in this study cohort varied from 2 to 6; C4A from 0 to 5; and C4B from 0 to 4. The distribution of total C4, C4A, and C4B gene copy number variations among To, TS, So, and NTS are shown in Table 6.
The distribution of GCN groups was analyzed first as categorical data. The distribution of C4A genes was statistically different among To, TS, So, and NTS (p = 0.034, χ2 analysis). Variations of GCNs for total C4 (p = 0.088) and C4B (p = 0.13) had not reached statistical significance. The median GCN groups for total C4 is 4, and for C4A and C4B are both 2. Low and high copy number groups are defined as those below and above median GCN groups, respectively. Variations in frequencies were observed for the low and high GCN groups of C4 genes. For example, 41.0% of the TS group had 2 or 3 copies of total C4 genes (low GCN), compared to 27.5% in To and 31.6% in NTS. By contrast, only 2.0% of the TS group had 5 or 6 copies of total C4 (high GCN), compared to 10.2% in the NTS group. A similar pattern was observed for C4A genes. There was an increase in the frequency of low C4A GCN (27.0% in TS, 17.3% in NTS), and a decrease in the frequency of high C4A GCN in the TS group (12.0% in TS, 24.5% in NTS).
The GCN values were analyzed as continuous data to compare means by Student's t-test. The means for total C4, C4A, and C4B were 3.67, 1.87, and 1.80, respectively, for the thrombotic subjects; and were 3.74, 1.99, and 1.75, respectively, for the non-thrombotic subjects. Lower mean C4A gene copy number was observed in the thrombotic group (p = 0.049) (Table 6).
The mean GCN for total C4, C4A, and C4B were 3.65, 1.87, and 1.78, respectively, for the SLE patients; and were 3.78, 2.01, and 1.77, respectively, for non-SLE subjects. Significantly lower mean GCNs for total C4 and C4A were present in the SLE group (p = 0.035 for total C4; p = 0.022 for C4A) (Table 6B). The mean C4B gene copy numbers were almost identical between patients with and without SLE.
When the aPL subjects were segregated and compared based on both thrombosis and SLE status, it revealed that the NTS group without thrombosis and SLE had the highest mean GCNs for total C4 and C4A (3.78 and 2.12, respectively), but the lowest C4B mean GCN (1.66). The thrombotic SLE group (TS) had the lowest values of total C4 at 3.55 and C4A at 1.81.
The mean GCN of total C4 for NTS was significantly higher than that of TS (p = 0.012); of C4A for NTS was significantly higher than those of To (p = 0.027), So (p = 0.012) and TS (p = 0.001); and of C4B was significantly lower than that of To (p = 0.015).
For TS, the mean GCN of total C4 was significantly lower than those of To (p = 0.011), So (p = 0.042), and NTS (p = 0.012); and of C4A was significantly lower than that of NTS (p = 0.001).
Plasma C4 Protein Concentrations Per C4 Gene Copy in Thrombosis and SLE
Both C4 gene copy number variation and clinical conditions of aPL subjects are important determining factors for C4 plasma protein concentrations. To examine the respective roles of genetic variants and clinical status on plasma protein levels of complement C4, we calculated the C4 protein per gene copy in each study subject by dividing the C4 plasma protein concentration with the C4 gene copy number. The mean C4 protein concentrations per gene dose among To, TS, So and NTS were 6.42, 6.21, 4.72, and 5.11 mg/dL, respectively. Thus, the C4 plasma protein yield per gene copy was the highest in aPL subjects with thrombosis only, and the lowest in aPL subjects with SLE only. Highly significant differences were present between the two thrombotic groups (To and TS) and the two non-thrombotic groups (So and NTS) (Figure 2).
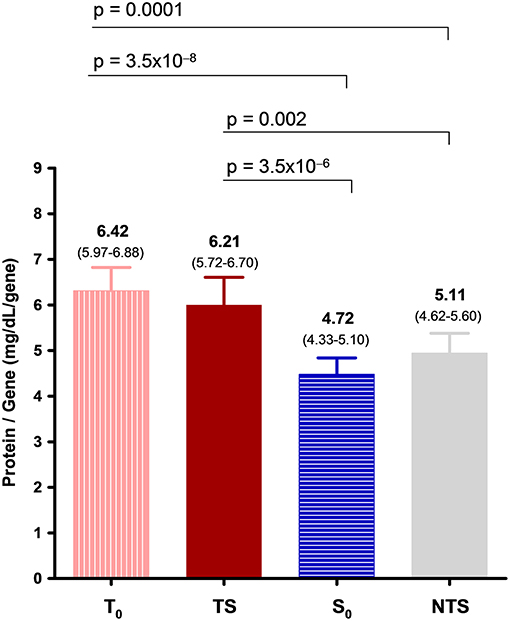
Figure 2. A comparison of mean total plasma C4 protein concentrations per gene-copy (with 95% confidence levels) among aPL-positive subjects categorized by thrombosis and SLE status. Protein concentration per gene-copy allows a comparison of C4 protein levels under various clinical conditions by eliminating the effects of C4 gene copy number variations among patients.
Differential Complement and ACLA Plasma Protein Levels and C4 Genetic Deficiencies in aPL-Positive Subjects With and Without Recurrent Pregnancy Loss (RPL)
Of the 444 female aPL-positive subjects, 106 experienced recurrent pregnancy loss (RPL). Thromboses occurred in 63.8% of the RPL patients, compared to 33.7% in non-RPL patients (p = 5.1 × 10−8). SLE were diagnosed in 54.3% of the RPL patients and 59.8% in non-RPL patients (p = 0.32). Strikingly, RPL had the highest frequency in patients with both thrombosis and SLE (TS, 41.0%) but lowest in patients with SLE only (So, 13.3%); patients of the NTS and To groups each had a frequency of 22.9%. The frequencies of RPL are significantly different among aPL-positive patients when segregated into To, So, TS, and NTS (Table 6; χ2 = 46.2, degree of freedom = 3, p = 3.8 × 10−10). TS patients had an odds ratio (95% confidence interval) of 8.63 (4.37–17.0) over So patients to experience recurrent pregnancy loss (p = 1.6 × 10−11).
Lupus anticoagulant was present in 67.1% of patients with RPL and 58.4% of non-RPL female patients (p = 0.057). Mean total C4 and ACLA-IgG protein levels were significantly increased, while mean CFH level was reduced (Table 7) among RPL patients. The mean total C4 level was 21.8 (19.9 ± 23.7) mg/dL in RPL and 19.1 (18.1 ± 20.2) mg/dL in non-RPL (p = 0.015). The ACLA-IgG was 44.2 (35.1 ± 53.3) g/l in RPL and 30.0 (24.2 ± 35.8) g/l in non-RPL (p = 0.01). The CFH mean concentrations in RPL were 48.8 (46.6 ± 51.0) mg/dL and 52.1 (50.5 ± 53.7) mg/dL in non-RPL (p = 0.019).
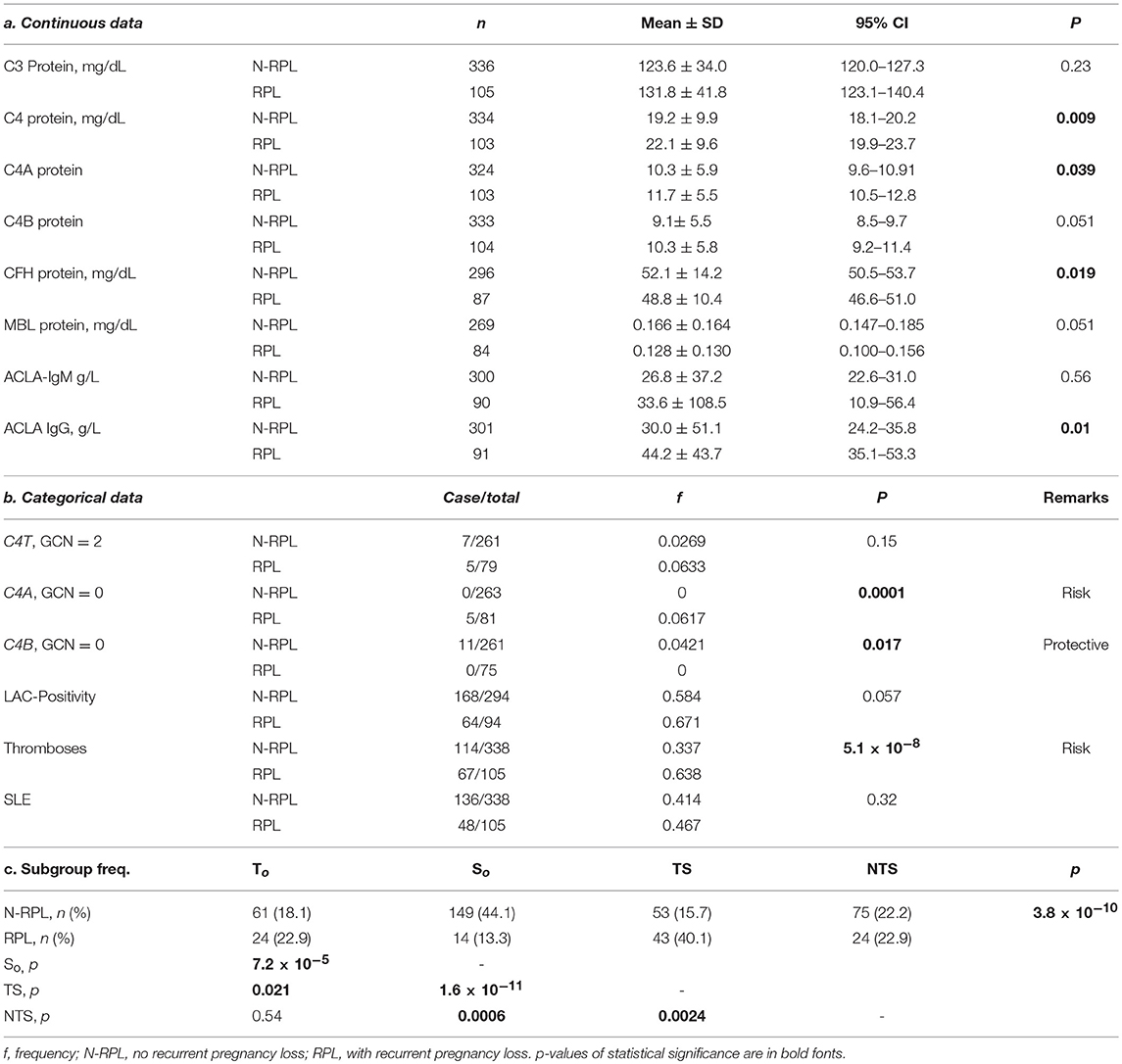
Table 7. Plasma complement and ACLA levels and risk factors for recurrent pregnancy loss (RPL) in female aPL-positive subjects.
Homozygous C4A deficiency (GCN of C4A = 0) was present in five female aPL patients and all of these five subjects experienced RPL (p = 0.0001). On the contrary, homozygous C4B deficiency (GCN of C4B = 0) was present in 11 female aPL patients and none of them experienced RPL (p = 0.017). Thus, homozygous C4A deficiency was a strong risk factor for, and homozygous C4B deficiency was a strong protective factor against, recurrent pregnancy loss.
Standardized Comparison of Numeric Parameters Associated With Thrombosis and SLE
To allow a standardized comparison of complement and ACLA protein variations in thrombosis and SLE, we determined the effect size index (69) of each parameter in To, So, and TS, using the mean protein concentrations or antibody levels of the group without thrombosis and SLE (NTS) as a reference. The difference of mean protein levels for each protein at To, So, or TS from NTS was divided by its root mean square error (RMSE), which was computed by ANOVA, to yield the effect size index. The effect size indices for complement and ACLA proteins with quantitative variations are depicted in Figure 3. In descending order, the greatest intergroup effect size indices are: C4, To vs. So: 0.709; C4B, To vs. So: 0.570; C4A, To vs. So: 0.566; ACLA-IgG, To vs. So: 0.554; complement factor H, TS vs. NTS: 0.483; ACLA-IgM, To vs. NTS: 0.373; and MBL, TS vs. So: 0.367.
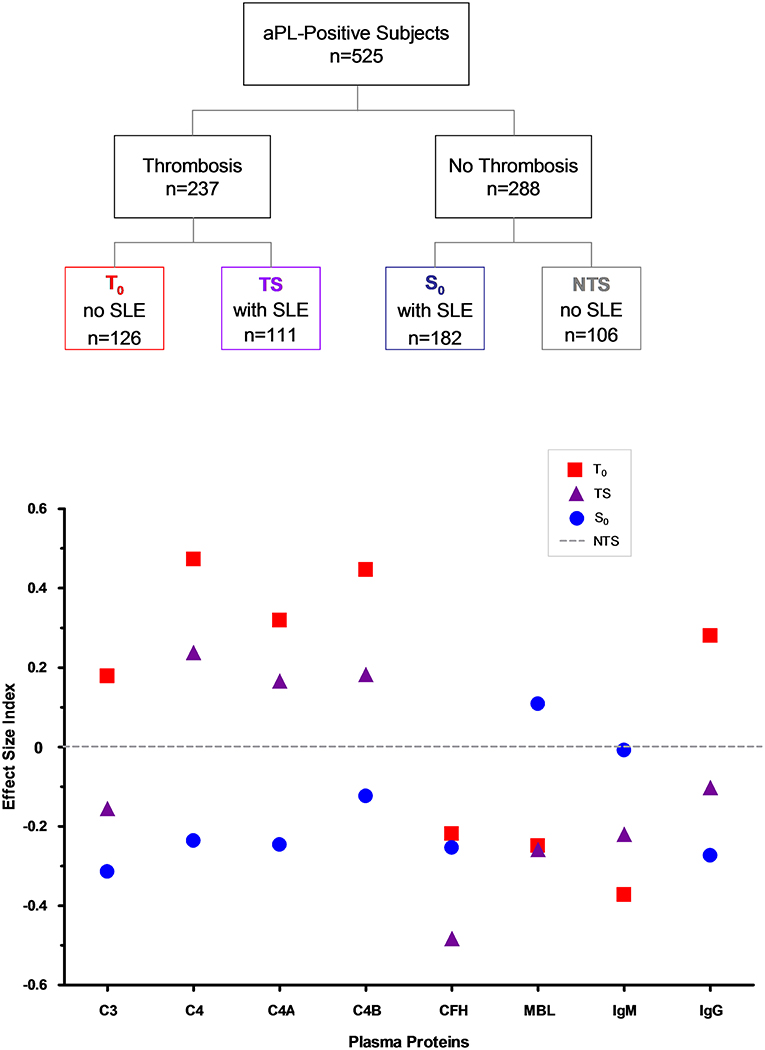
Figure 3. (Upper) Categorization of aPL-positive subjects according to their thrombosis and SLE status. (Lower) Standardized differences (effect size index) of mean complement protein levels and ACLA levels among aPL-positive subjects with thrombosis without SLE (To), thrombosis and SLE (TS), SLE without thrombosis (So) when compared to the non-thrombotic and non-SLE (NTS) group. Root mean square error (RMSE) value for each protein was derived from Oneway ANOVA. Differences of mean protein levels for To, TS and So from NTS were each divided by their associated RMSE and charted to derive the effect size index. The effect size indices allow a standardized comparison of different parameters under different clinical conditions.
Discussion
This is a cross-sectional study of complement protein profiles and copy number variations of C4 genes in a relatively large cohort of human subjects with aPL antibodies. These aPL-positive subjects had a variety of clinical presentations: thrombosis, SLE, thrombosis and SLE, no thrombosis and no SLE. Many female aPL-positive subjects also experienced pregnancy morbidity such as recurrent pregnancy losses. Extensive analyses of gene copy number variations for total C4, C4A, and C4B, plasma protein levels of total C4, C4A, C4B, C3, factor H and MBL, and antiphospholipid antibodies revealed distinct patterns of diversity that can be relevant and effective quantitative biomarkers for thrombosis, SLE and recurrent pregnancy loss.
An intriguing aspect of complement C4 genetics is the frequent gene copy number variations (30, 38, 40). Genetic deficiency (60, 70) or low gene copy numbers of total C4 or C4A has been shown to be a prevalent risk factor for SLE in European and East-Asian Americans (22, 38, 41, 71–73). C4 gene copy number variations in the aPL-positive subjects were determined and validated by quantitative real-time PCR (35). When SLE and non-SLE subjects were compared, lower total C4 and C4A mean gene copy numbers were found among the SLE subjects, suggesting that aPL-positive subjects with low total C4 or C4A gene copy numbers carried a greater risk of developing SLE, as we reported in earlier studies (22, 38). Among the NTS subjects who were not afflicted with thrombosis and SLE, there were higher mean GCN of C4A, which would be protective against SLE; and low GCN of C4B that would lead to lower C4B protein levels and thereby reducing the risk of thrombosis.
Using thrombosis as a response, multiple logistic regression analyses suggested that higher plasma C4 protein levels and the presence of lupus anticoagulant (LAC) were among the strongest independent biomarkers associated with thrombosis (C4, p = 6.2 × 10−9; LAC, p = 6.9 × 10−5) (Supplementary Table). Other relevant parameters for increased risk of thrombosis included male sex and a reduction of complement factor H level, which was also observed by Nakamura and colleagues (74). Higher plasma C4 protein levels and the presence of LAC were the two most prominent risk factors for arterial thrombosis. The higher level of C4 protein in arterial thrombosis was mainly attributable to higher C4B (p = 2.1 × 10−5). The risk factors identified for venous thrombosis also included increased protein level of total C4 (p = 0.01) and the presence of LAC (p = 0.012). Reduced protein level of functional MBL (p = 0.0012) and male gender also had prominent effects.
While the presence of LAC and elevated ACLA-IgG levels have long been recognized for their connections with thrombosis and recurrent pregnancy loss (75), this report provides a firm documentation on the significance of higher C4 plasma protein levels among aPL-positive subjects with APS-related clinical manifestations. The presence of LAC and elevated protein level of complement C4 together are predictors for increased risk of thrombosis with values of sensitivity at 0.707 and specificity at 0.664. This study also reveals lower complement factor H protein levels among subjects with SLE and thrombosis. Deficiency, mutation or autoantibody of complement factor H have been linked to atypical hemolytic uremic syndrome that is characterized by thrombotic microangiopathy (76, 77). Along with observations that plasma protein levels of MBL were decreased, evidence for an involvement of complement proteins in human thrombosis or pregnancy loss are compelling and deserve clinical attention (78). High levels of plasma C4 among patients with thrombosis could result in a procoagulation or thromboinflammatory state, which provide large quantities of reagents to fuel the complement cascades, leading to greater extent of complement-mediated tissue injuries. The abundance of the fast-reacting C4B could aggravate the pathogenic process in arterial thrombosis.
Using SLE as a response, multiple logistic regression analysis of plasma protein data suggested that reduced levels of C3, C4, and ACLA-IgG, and female gender were strong risk factors for SLE C3 is downstream of C4 in the classical and the MBL activation pathways, the activation and consumption of C3 are amplified by a positive feedback mechanism (79–81). In other words, moderate activation of C4 can lead to large consumption of C3. Thus, fluctuations of serum C3 levels tend to be a more sensitive biomarker for SLE disease activity than C4 does.
Among the female aPL-positive subjects, patients with thrombosis and particularly, thrombotic SLE, had high frequencies of recurrent pregnancy loss. RPL patients had elevated levels of complement C4 and ACLA-IgG, and decreased concentration of factor H. Remarkably, female aPL-positive subjects with homozygous C4B deficiency were all protected from RPL, which is consistent with observations in mouse models that complement C4 deficiency or C3 deficiency were protective from RPL induced by injection of human aPL (16). It is also of interest to note that aPL-positive female (human) patients with homozygous C4A deficiency all experienced RPL, which underlies the importance of C4A protein in achieving tolerance or defense against autoimmunity and fetal rejections.
It is important to recognize that the direction of changes for plasma protein levels of complement C3, C4, and ACLA-IgG among aPL-positive patients with SLE and with thrombosis or pregnancy morbidity are mostly opposite to each other. The highest mean protein levels for these proteins were present in the To group (thrombosis without SLE), and the lowest in the So group (SLE without thrombosis). Thus, the inter-group differences of these proteins were highly significant (To vs. So: p = 2.6 × 10−5 for C3; p = 2.2 × 10−9 for C4; p = 1.2 × 10−5 for ACLA-IgG). The resultant effects for these two opposing forces, SLE and thrombosis, are shown in the thrombotic SLE group TS, by which the mean plasma protein levels of C3, C4, C4A, C4B, and ACLA-IgG all fell between those of To and So groups, and their values were closer to those present in the NTS group. When standardized by the gene copy numbers, highly significant differences for mean C4 protein concentrations per gene-copy were observed between the thrombotic subjects and non-thrombotic subjects, and the greatest difference remained between To and So (6.42 mg/dL/gene for To, 4.72 mg/dL/gene for So; p = 3.5 × 10−8). The mean C4 protein per gene-copy in TS (6.21 mg/dL/gene) was only slightly lower than that of To. This implies the presence of trans-acting factor(s) among patients with thrombosis that upregulates C4 protein biosynthesis, and/or reduces its turnover that would have decreased the protein levels. While complement activation is a noted feature for clinical manifestations of APS, such activation likely occurs locally that may not result in systemic and parallel decline of plasma protein levels for C4 and C3, a phenomenon analogous to what we observed in many patients with juvenile dermatomyositis (82).
The target sites for most aPL appear to be located at the domain D1 or complement controlling protein repeat on β2GPI. Recombinant antibody recognizing this domain D1 induced fetal loss and coagulation in animal models (83). Interestingly, an engineered β2GPI antibody without the IgG heavy chain CH2-domain, which was devoid of the C1q binding site and unable to fix or activate complement, was shown to compete and control the coagulation and abortive effects in animals burgeoned by injection of human aPL (83). Biochemical studies revealed that β2GPI in its linear conformation can serve as a regulator for the classical and alternative pathway C3 convertases, as it diminished the activation of C3 (to form C3a) and the assembly of C5b-9 in a dose-dependent manner. Active β2GPI also enhanced the degradation of C3b in the presence of factor I and factor H (84, 85). The effects of aPL on the functional activities of β2GPI and plasma complement protein concentrations and activities remain to be elucidated.
The relationships among MBL deficiency, SLE and thrombosis were complex and it was not clear whether MBL deficiency was a risk factor for SLE (86, 87). In a study of 91 SLE patients, Garred et al. demonstrated a near doubling of thrombosis in individuals homozygous for MBL protein structural variants (B,D,C) that led to functional deficiencies of MBL (88). Subsequently, an association was made between MBL deficiency and arterial thrombosis (89). In a study of structural variants and promoter alleles for high and low expression of MBL2 gene in 114 SLE patients, Font et al. observed that low MBL expression genotypes were associated with venous thrombosis (90). Data from our study further clarifies the role of MBL in SLE and thrombosis: reduced plasma protein concentrations of functional MBL were present among aPL-positive patients with thrombosis, regardless of SLE status. Therefore, the link between MBL-deficiency (or low expression of MBL) and SLE could be secondary to low functional MBL in SLE patients with APS. As a lectin binding protein that binds to simple carbohydrate (mannose) components on cell membranes, it is possible that MBL could compete with aPL for binding to phospholipids or phospholipid-binding proteins to reduce the risk of aPL on initiating thrombotic events.
Our study population includes multiple racial and ethnic backgrounds but the majority were of Northern European ancestry. When we analyzed the complement and ACLA data on this specific ethnic group, similar conclusions on the contrasting patterns of complement C4 and ACLA in thromboses and SLE can be reached. Results on three clinical studies on stroke or recurrent pregnancy loss were in accord with our observations that high C4 and/or high C3 plasma protein levels are associated with thrombosis or recurrent pregnancy loss (91–93).
Our observations are consistent with a parallel and independent study that revealed that pediatric SLE patients undergoing a clinical trial (94) with a history of hypertension had persistently higher serum levels of complement C4 and C3 and higher gene copy number of C4B (Mulvihill et al, submitted). Here, we further show that patients with both SLE and thrombosis had the lowest mean GCNs for total C4 (3.55) and C4A (1.81), which underscores the importance of C4A deficiency as a genetic risk factor for systemic autoimmune disease. Paradoxically, hypocomplementemia is both a cause and an effect of human SLE. SLE-associated disorders such as lupus nephritis, hemolytic anemia, high titers of anti-dsDNA, and lupus disease flares are notably marked by low serum complement levels due to massive consumption of C3 and C4 (21–23, 25). Systemic and concurrent consumptions of C4 and C3 can be reflected by higher coefficients of correlation (r or r2) between these two proteins, which are conspicuous among aPL-positive patients in the So and NTS groups (Table 2) (25). Detailed diagnostic disorders of SLE (64) and triple positivity of aPL autoantibodies (9) were not available for this study to examine the extent of hypocomplementemia in various organ involvement and tissue damage, but these would be relevant topics for future investigations.
This cross-sectional study represents a snapshot of complement and aPL in a population of human subjects with antiphospholipid antibodies. The relatively large study population provided an informative dataset to examine specific patterns of complement and aPL among patients with thrombosis, SLE and recurrent pregnancy loss. Along time courses of patients with chronic, systemic autoimmune disease, plasma or serum complement C3 and C4 levels and their cell-bound products would fluctuate with disease activities. The status of SLE/APS disease activities including flare and remission for each patient at the time of sample collection was not available and therefore not accounted for in our data analyses. The lack of data from longitudinal studies, and blood samples from healthy subjects with and without aPL being processed in parallel with similar methodologies are other limitations of this study. Further studies with large sample size of patients for measurements of complement component protein levels under defined genetic backgrounds, plus determination of activation products C3a, C4a, and C5a, cell-bound and fluid phase levels of C4d and C3d, and membrane attack complexes may provide important insights into mechanism(s) on how complement modulate aPL associated clinical manifestations and disease activities of SLE and APS (55, 58, 61, 62). In addition, effects of complement-mediated tissue damage and thromboses would be more readily demonstrated by immunohistochemical methods.
In conclusion, our results can serve as a foundation for further studies of SLE and APS disease mechanisms, more sensitive disease diagnosis, and possibly better prognosis of disease course and profile. It would be desirable to elucidate the C4 gene copy numbers among aPL-positive subjects for a prevention purpose, as those with low total C4 or C4A gene copy number would have a higher risk to develop SLE, and high C4B GCN would have greater risk for complement-mediated complications such as thrombosis, recurrent pregnancy loss in females, and tissue injuries.
Author Contributions
C-YY, SS, YW, and RR designed the research. RR contributed patient samples and clinical data. SS, KK, DZ, YW, and C-YY performed experiments. HN, YW, SS, and C-YY performed statistical analyses. SS, YW, DZ, EM, FB-S, SA, RR, and C-YY analyzed and interpreted data. SS, YW, KK, DZ, EM, FB-S, SA, RR, HN, and C-YY wrote the paper.
Conflict of Interest Statement
The authors declare that the research was conducted in the absence of any commercial or financial relationships that could be construed as a potential conflict of interest.
Acknowledgments
We thank Gail Buxton and Bi Zhou for their assistance. This work was supported by grants 1R01 AR050078, 1R21 AR070905, 1R01 AR073311 from the National Institute of Arthritis, Musculoskeletal and Skin Diseases of the NIH (C-YY), a grant from the General Clinical Research Centers Program of the Division of Research Resources, National Institutes of Health, M01 RR00034 (OSU) and RR00046 (UNC).
Supplementary Material
The Supplementary Material for this article can be found online at: https://www.frontiersin.org/articles/10.3389/fimmu.2019.00885/full#supplementary-material
Abbreviations
ANOVA, analysis of variance; aPL, antiphospholipid antibodies; APS, antiphospholipid syndrome; CNV, copy number variation; GCN, gene copy number; LAC, lupus anticoagulant; MBL, mannan binding lectin; NS, no SLE; NT, no thrombosis; NTS, no thrombosis and no SLE; RMSE, root mean square error; RPL, recurrent pregnancy loss; S, SLE; So, SLE without thrombosis; SLE, systemic lupus erythematosus; T, thrombosis, To, thrombosis without SLE; TS, thrombosis and SLE.
References
1. Wilson WA, Gharavi AE, Koike T, Lockshin MD, Branch DW, Piette JC, et al. International consensus statement on preliminary classification criteria for definite antiphospholipid syndrome: report of an international workshop. Arthritis Rheum. (1999) 42:1309–11. doi: 10.1002/1529-0131(199907)42:7<1309::AID-ANR1>3.0.CO;2-F
2. Levine JS, Branch DW, Rauch J. The antiphospholipid syndrome. N Engl J Med. (2002) 346:752–63. doi: 10.1056/NEJMra002974
3. Cervera R. Antiphospholipid syndrome. Thromb Res. (2017) 151(Suppl 1):S43–7. doi: 10.1016/S0049-3848(17)30066-X
4. Schur PH. Pathogenesis of antiphospholipid syndrome. In: Pisetsky DS, ed. Up-To-Date. (2019). Available online at: https://www.uptodate.com/contents/pathogenesis-of-antiphospholipid-syndrome
5. Schreiber K, Sciascia S, de Groot PG, Devreese K, Jacobsen S, Ruiz-Irastorza G, et al. Antiphospholipid syndrome. Nat Rev Dis Primers. (2018) 4:17103. doi: 10.1038/nrdp.2018.5
6. Miyakis S, Lockshin MD, Atsumi T, Branch DW, Brey RL, Cervera R, et al. International consensus statement on an update of the classification criteria for definite antiphospholipid syndrome (APS). J Thromb Haemost. (2006) 4:295–306. doi: 10.1111/j.1538-7836.2006.01753.x
7. Viall CA, Chamley LW. Histopathology in the placentae of women with antiphospholipid antibodies: a systematic review of the literature. Autoimmun Rev. (2015) 14:446–71. doi: 10.1016/j.autrev.2015.01.008
8. Roubey RA. Antiphospholipid antibodies: immunological aspects. Clin Immunol. (2004) 112:127–8. doi: 10.1016/j.clim.2004.02.010
9. Pengo V, Ruffatti A, Legnani C, Gresele P, Barcellona D, Erba N, et al. Clinical course of high-risk patients diagnosed with antiphospholipid syndrome. J Thromb Haemost. (2010) 8:237–42. doi: 10.1111/j.1538-7836.2009.03674.x
10. Steinkasserer A, Estaller C, Weiss EH, Sim RB, Day AJ. Complete nucleotide and deduced amino acid sequence of human beta 2-glycoprotein I. Biochem J. (1991) 277(Pt 2):387–91. doi: 10.1042/bj2770387
11. Koike T. Antiphospholipid syndrome: 30 years and our contribution. Int J Rheum Dis. (2015) 18:233–41. doi: 10.1111/1756-185X.12438
12. Lozier J, Takahashi N, Putnam FW. Complete amino acid sequence of human plasma beta 2-glycoprotein I. Proc Natl Acad Sci USA. (1984) 81:3640–4. doi: 10.1073/pnas.81.12.3640
14. Davidson A, Diamond B. Autoimmune diseases. N Engl J Med. (2001) 345:340–50. doi: 10.1056/NEJM200108023450506
15. Cervera R, Piette JC, Font J, Khamashta MA, Shoenfeld Y, Camps MT, et al. Antiphospholipid syndrome: clinical and immunologic manifestations and patterns of disease expression in a cohort of 1,000 patients. Arthritis Rheum. (2002) 46:1019–27. doi: 10.1002/art.10187
16. Harris EN, Gharavi AE, Boey ML, Patel BM, Mackworth-Young CG, Loizou S, et al. Anticardiolipin antibodies: detection by radioimmunoassay and association with thrombosis in systemic lupus erythematosus. Lancet. (1983) 2:1211–4. doi: 10.1016/S0140-6736(83)91267-9
17. Love PE, Santoro SA. Antiphospholipid antibodies: anticardiolipin and the lupus anticoagulant in systemic lupus erythematosus (SLE) and in non-SLE disorders. prevalence and clinical significance. Ann Intern Med. (1990) 112:682–98. doi: 10.7326/0003-4819-112-9-682
18. Sestak A, O'Neil KM. Familial lupus and antiphospholipid syndrome. Lupus. (2007) 16:556–63. doi: 10.1177/0961203307078071
19. Pons-Estel GJ, Andreoli L, Scanzi F, Cervera R, Tincani A. The antiphospholipid syndrome in patients with systemic lupus erythematosus. J Autoimmun. (2017) 76:10–20. doi: 10.1016/j.jaut.2016.10.004
20. de Groot PG, de Laat B. Mechanisms of thrombosis in systemic lupus erythematosus and antiphospholipid syndrome. Best Pract Res Clin Rheumatol. (2017) 31:334–41. doi: 10.1016/j.berh.2017.09.008
21. Birmingham DJ, Irshaid F, Nagaraja HN, Zou X, Tsao BP, Wu H, et al. The complex nature of serum C3 and C4 as biomarkers of lupus renal flare. Lupus. (2010) 19:1272–80. doi: 10.1177/0961203310371154
22. Chen JY, Wu YL, Mok MY, Wu YJ, Lintner KE, Wang CM, et al. Effects of complement C4 gene copy number variations, size dichotomy, and C4A deficiency on genetic risk and clinical presentation of systemic lupus erythematosus in east asian populations. Arthritis Rheumatol. (2016) 68:1442–53. doi: 10.1002/art.39589
23. Schur PH. Complement and lupus erythematosus. Arthritis Rheum. (1982) 25:793–8. doi: 10.1002/art.1780250715
24. Ramos-Casals M, Campoamor MT, Chamorro A, Salvador G, Segura S, Botero JC, et al. Hypocomplementemia in systemic lupus erythematosus and primary antiphospholipid syndrome: prevalence and clinical significance in 667 patients. Lupus. (2004) 13:777–83. doi: 10.1191/0961203304lu1080oa
25. Wu YL, Higgins GC, Rennebohm RM, Chung EK, Yang Y, Zhou B, et al. Three distinct profiles of serum complement C4 proteins in pediatric systemic lupus erythematosus (SLE) patients: tight associations of complement C4 and C3 protein levels in SLE but not in healthy subjects. Adv Exp Med Biol. (2006) 586:227–47. doi: 10.1007/0-387-34134-X_16
26. Lintner KE, Wu YL, Yang Y, Spencer CH, Hauptmann G, Hebert LA, et al. Early components of the complement classical activation pathway in human systemic autoimmune diseases. Front Immunol. (2016) 7:36. doi: 10.3389/fimmu.2016.00036
27. Wu YL, Brookshire BP, Verani RR, Arnett FC, Yu CY. Clinical presentations and molecular basis of complement C1r deficiency in a male African-American patient with systemic lupus erythematosus. Lupus. (2011) 20:1126–34. doi: 10.1177/0961203311404914
28. Wu YL, Hauptmann G, Viguier M, Yu CY. Molecular basis of complete complement C4 deficiency in two North-African families with systemic lupus erythematosus. Genes Immun. (2009) 10:433–45. doi: 10.1038/gene.2009.10
29. Reid KB, Porter RR. The proteolytic activation systems of complement. Annu Rev Biochem. (1981) 50:433–64. doi: 10.1146/annurev.bi.50.070181.002245
30. Chung EK, Yang Y, Rennebohm RM, Lokki ML, Higgins GC, Jones KN, et al. Genetic sophistication of human complement components C4A and C4B and RP-C4-CYP21-TNX (RCCX) modules in the major histocompatibility complex. Am J Hum Genet. (2002) 71:823–37. doi: 10.1086/342777
31. Yu CY, Chung EK, Yang Y, Blanchong CA, Jacobsen N, Saxena K, et al. Dancing with complement C4 and the RP-C4-CYP21-TNX (RCCX) modules of the major histocompatibility complex. Prog Nucleic Acid Res Mol Biol. (2003) 75:217–92. doi: 10.1016/S0079-6603(03)75007-7
32. Isenman DE, Young JR. The molecular basis for the difference in immune hemolysis activity of the chido and rodgers isotypes of human complement component C4. J Immunol. (1984) 132:3019–27.
33. Law SK, Dodds AW, Porter RR. A comparison of the properties of two classes, C4A and C4B, of the human complement component C4. EMBO J. (1984) 3:1819–23. doi: 10.1002/j.1460-2075.1984.tb02052.x
34. Yu CY, Belt KT, Giles CM, Campbell RD, Porter RR. Structural basis of the polymorphism of human complement components C4A and C4B: gene size, reactivity and antigenicity. EMBO J. (1986) 5:2873–81. doi: 10.1002/j.1460-2075.1986.tb04582.x
35. Wu YL, Savelli SL, Yang Y, Zhou B, Rovin BH, Birmingham DJ, et al. Sensitive and specific real-time polymerase chain reaction assays to accurately determine copy number variations (CNVs) of human complement C4A, C4B, C4-long, C4-short, and RCCX modules: elucidation of C4 CNVs in 50 consanguineous subjects with defined HLA genotypes. J Immunol. (2007) 179:3012–25. doi: 10.4049/jimmunol.179.5.3012
36. Blanchong CA, Zhou B, Rupert KL, Chung EK, Jones KN, Sotos JF, et al. Deficiencies of human complement component C4A and C4B and heterozygosity in length variants of RP-C4-CYP21-TNX (RCCX) modules in caucasians. the load of RCCX genetic diversity on major histocompatibility complex-associated disease. J Exp Med. (2000) 191:2183–96. doi: 10.1084/jem.191.12.2183
37. Yang Z, Mendoza AR, Welch TR, Zipf WB, Yu CY. Modular variations of the human major histocompatibility complex class III genes for serine/threonine kinase RP, complement component C4, steroid 21-hydroxylase CYP21, and tenascin TNX (the RCCX module). A mechanism for gene deletions and disease associations. J Biol Chem. (1999) 274:12147–56. doi: 10.1074/jbc.274.17.12147
38. Yang Y, Chung EK, Wu YL, Savelli SL, Nagaraja HN, Zhou B, et al. Gene copy-number variation and associated polymorphisms of complement component C4 in human systemic lupus erythematosus (SLE): low copy number is a risk factor for and high copy number is a protective factor against SLE susceptibility in European Americans. Am J Hum Genet. (2007) 80:1037–54. doi: 10.1086/518257
39. Dangel AW, Mendoza AR, Baker BJ, Daniel CM, Carroll MC, Wu LC, et al. The dichotomous size variation of human complement C4 genes is mediated by a novel family of endogenous retroviruses, which also establishes species-specific genomic patterns among old world primates. Immunogenetics. (1994) 40:425–36. doi: 10.1007/BF00177825
40. Yang Y, Chung EK, Zhou B, Blanchong CA, Yu CY, Füst G, et al. Diversity in intrinsic strengths of the human complement system: serum C4 protein concentrations correlate with C4 gene size and polygenic variations, hemolytic activities, and body mass index. J Immunol. (2003) 171:2734–45. doi: 10.4049/jimmunol.171.5.2734
41. Jüptner M, Flachsbart F, Caliebe A, Lieb W, Schreiber S, Zeuner R, et al. Low copy numbers of complement C4 and homozygous deficiency of C4A may predispose to severe disease and earlier disease onset in patients with systemic lupus erythematosus. Lupus. (2018) 27:600–9. doi: 10.1177/0961203317735187
42. Pereira KM, Faria AG, Liphaus BL, Jesus AA, Silva CA, Carneiro-Sampaio M, et al. Low C4, C4A and C4B gene copy numbers are stronger risk factors for juvenile-onset than for adult-onset systemic lupus erythematosus. Rheumatology (Oxford). (2016) 55:869–73. doi: 10.1093/rheumatology/kev436
43. Pierangeli SS, Harris EN. Antiphospholipid antibodies in an in vivo thrombosis model in mice. Lupus. (1994) 3:247–51. doi: 10.1177/096120339400300408
44. Pierangeli SS, Liu XW, Barker JH, Anderson G, Harris EN. Induction of thrombosis in a mouse model by IgG, IgM and IgA immunoglobulins from patients with the antiphospholipid syndrome. Thromb Haemost. (1995) 74:1361–7. doi: 10.1055/s-0038-1649940
45. Branch DW, Dudley DJ, Mitchell MD, Creighton KA, Abbott TM, Hammond EH, et al. Immunoglobulin G fractions from patients with antiphospholipid antibodies cause fetal death in BALB/c mice: a model for autoimmune fetal loss. Am J Obstet Gynecol. (1990) 163:210–6. doi: 10.1016/S0002-9378(11)90700-5
46. Blank M, Cohen J, Toder V, Shoenfeld Y. Induction of anti-phospholipid syndrome in naive mice with mouse lupus monoclonal and human polyclonal anti-cardiolipin antibodies. Proc Natl Acad Sci USA. (1991) 88:3069–73. doi: 10.1073/pnas.88.8.3069
47. Salmon JE, Girardi G. The role of complement in the antiphospholipid syndrome. Curr Dir Autoimmun. (2004) 7:133–48. doi: 10.1159/000075690
48. Holers VM, Girardi G, Mo L, Guthridge JM, Molina H, Pierangeli SS, et al. Complement C3 activation is required for antiphospholipid antibody-induced fetal loss. J Exp Med. (2002) 195:211–20. doi: 10.1084/jem.200116116
49. Atkinson JP. Complement system on the attack in autoimmunity. J Clin Invest. (2003) 112:1639–41. doi: 10.1172/JCI20309
50. Girardi G, Berman J, Redecha P, Spruce L, Thurman JM, Kraus D, et al. Complement C5a receptors and neutrophils mediate fetal injury in the antiphospholipid syndrome. J Clin Invest. (2003) 112:1644–54. doi: 10.1172/JCI200318817
51. Fleming SD, Egan RP, Chai C, Girardi G, Holers VM, Salmon J, et al. Anti-phospholipid antibodies restore mesenteric ischemia/reperfusion-induced injury in complement receptor 2/complement receptor 1-deficient mice. J Immunol. (2004) 173:7055–61. doi: 10.4049/jimmunol.173.11.7055
52. Fleming SD, Mastellos D, Karpel-Massler G, Shea-Donohue T, Lambris JD, Tsokos GC. C5a causes limited, polymorphonuclear cell-independent, mesenteric ischemia/reperfusion-induced injury. Clin Immunol. (2003) 108:263–73. doi: 10.1016/S1521-6616(03)00160-8
53. Pierangeli SS, Girardi G, Vega-Ostertag M, Liu X, Espinola RG, Salmon J. Requirement of activation of complement C3 and C5 for antiphospholipid antibody-mediated thrombophilia. Arthritis Rheum. (2005) 52:2120–4. doi: 10.1002/art.21157
54. Cohen D, Buurma A, Goemaere NN, Girardi G, le Cessie S, Scherjon S, et al. Classical complement activation as a footprint for murine and human antiphospholipid antibody-induced fetal loss. J Pathol. (2011) 225:502–11. doi: 10.1002/path.2893
55. Kim MY, Guerra MM, Kaplowitz E, Laskin CA, Petri M, Branch DW, et al. Complement activation predicts adverse pregnancy outcome in patients with systemic lupus erythematosus and/or antiphospholipid antibodies. Ann Rheum Dis. (2018) 77:549–55. doi: 10.1136/annrheumdis-2017-212224
56. Tedesco F, Borghi MO, Gerosa M, Chighizola CB, Macor P, Lonati PA, et al. Pathogenic role of complement in antiphospholipid syndrome and therapeutic implications. Front Immunol. (2018) 9:1388. doi: 10.3389/fimmu.2018.01388
57. Samarkos M, Mylona E, Kapsimali V. The role of complement in the antiphospholipid syndrome: a novel mechanism for pregnancy morbidity. Semin Arthritis Rheum. (2012) 42:66–9. doi: 10.1016/j.semarthrit.2012.01.001
58. Devreese KM, Hoylaerts MF. Is there an association between complement activation and antiphospholipid antibody-related thrombosis? Thromb Haemost. (2010) 104:1279–81. doi: 10.1160/TH10-06-0410
59. Fischetti F, Durigutto P, Pellis V, Debeus A, Macor P, Bulla R, et al. Thrombus formation induced by antibodies to beta2-glycoprotein I is complement dependent and requires a priming factor. Blood. (2005) 106:2340–6. doi: 10.1182/blood-2005-03-1319
60. Yang Y, Chung EK, Zhou B, Lhotta K, Hebert LA, Birmingham DJ, et al. The intricate role of complement component C4 in human systemic lupus erythematosus. Curr Dir Autoimmun. (2004) 7:98–132. doi: 10.1159/000075689
61. Oku K, Atsumi T, Bohgaki M, Amengual O, Kataoka H, Horita T, et al. Complement activation in patients with primary antiphospholipid syndrome. Ann Rheum Dis. (2009) 68:1030–5. doi: 10.1136/ard.2008.090670
62. Oku K, Amengual O, Hisada R, Ohmura K, Nakagawa I, Watanabe T, et al. Autoantibodies against a complement component 1 q subcomponent contribute to complement activation and recurrent thrombosis/pregnancy morbidity in anti-phospholipid syndrome. Rheumatology (Oxford). (2016) 55:1403–11. doi: 10.1093/rheumatology/kew196
63. Roubey RA. Antiphospholipid syndrome: antibodies and antigens. Curr Opin Hematol. (2000) 7:316–20. doi: 10.1097/00062752-200009000-00010
64. Tan EM, Cohen AS, Fries JF, Masi AT, McShane DJ, Rothfield NF, et al. The 1982 revised criteria for the classification of systemic lupus erythematosus. Arthritis Rheum. (1982) 25:1271–7. doi: 10.1002/art.1780251101
65. Giclas PC. Measurement of complement component levels by radial immunodiffusion. Curr Prot Immunol. (2003) 13:1.9–1.26. doi: 10.1002/0471142735.im1301s09
66. Sim E, Cross SJ. Phenotyping of human complement component C4, a class-III HLA antigen. Biochem J. (1986) 239:763–7. doi: 10.1042/bj2390763
67. Awdeh ZL, Alper CA. Inherited structural polymorphism of the fourth component of human complement. Proc Natl Acad Sci USA. (1980) 77:3576–80. doi: 10.1073/pnas.77.6.3576
68. Chung EK, Wu YL, Yang Y, Zhou B, Yu CY. Human complement components C4A and C4B genetic diversities: complex genotypes and phenotypes. Curr Protoc Immunol. (2005) Chapter 13:Unit 13 18. doi: 10.1002/0471142735.im1308s68
69. Cohen J. Statistical Power Analysis for Behavioral Sciences, 2nd ed. Hillsdale, NJ: Lawrence Earlbaum Associates (1988). p. 20–21.
70. Yang Y, Lhotta K, Chung EK, Eder P, Neumair F, Yu CY. Complete complement components C4A and C4B deficiencies in human kidney diseases and systemic lupus erythematosus. J Immunol. (2004) 173:2803–14. doi: 10.4049/jimmunol.173.4.2803
71. Lv Y, He S, Zhang Z, Li Y, Hu D, Zhu K, et al. Confirmation of C4 gene copy number variation and the association with systemic lupus erythematosus in Chinese Han population. Rheumatol Int. (2012) 32:3047–53. doi: 10.1007/s00296-011-2023-7
72. Kemp ME, Atkinson JP, Skanes VM, Levine RP, Chaplin DD. Deletion of C4A genes in patients with systemic lupus erythematosus. Arthritis Rheum. (1987) 30:1015–22. doi: 10.1002/art.1780300908
73. Fielder AH, Walport MJ, Batchelor JR, Rynes RI, Black CM, Dodi IA, et al. Family study of the major histocompatibility complex in patients with systemic lupus erythematosus: importance of null alleles of C4A and C4B in determining disease susceptibility. Br Med J (Clin Res Ed). (1983) 286:425–8. doi: 10.1136/bmj.286.6363.425
74. Nakamura H, Oku K, Ogata Y, Ohmura K, Yoshida Y, Kitano E, et al. Alternative pathway activation due to low level of complement factor H in primary antiphospholipid syndrome. Thromb Res. (2018) 164:63–8. doi: 10.1016/j.thromres.2018.02.142
75. Galli M, Luciani D, Bertolini G, Barbui T. Lupus anticoagulants are stronger risk factors for thrombosis than anticardiolipin antibodies in the antiphospholipid syndrome: a systematic review of the literature. Blood. (2003) 101:1827–32. doi: 10.1182/blood-2002-02-0441
76. Ying L, Katz Y, Schlesinger M, Carmi R, Shalev H, Haider N, et al. Complement factor H gene mutation associated with autosomal recessive atypical hemolytic uremic syndrome. Am J Hum Genet. (1999) 65:1538–46. doi: 10.1086/302673
77. Kavanagh D, Richards A, Fremeaux-Bacchi V, Noris M, Goodship T, Remuzzi G, et al. Screening for complement system abnormalities in patients with atypical hemolytic uremic syndrome. Clin J Am Soc Nephrol. (2007) 2:591–6. doi: 10.2215/CJN.03270906
78. Ekdahl KN, Teramura Y, Hamad OA, Asif S, Duehrkop C, Fromell K, et al. Dangerous liaisons: complement, coagulation, and kallikrein/kinin cross-talk act as a linchpin in the events leading to thromboinflammation. Immunol Rev. (2016) 274:245–69. doi: 10.1111/imr.12471
79. Fearon DT. Activation of the alternative complement pathway. CRC Crit Rev Immunol. (1979) 1:1–32.
80. Lachmann PJ, Hughes-Jones NC. Initiation of complement activation. Springer Semin Immunopathol. (1984) 7:143–62. doi: 10.1007/BF01893018
81. Fearon DT, Daha MR, Weiler JM, Austen KF. The natural modulation of the amplification phase of complement activation. Transplant Rev. (1976) 32:12–25. doi: 10.1111/j.1600-065X.1976.tb00226.x
82. Lintner KE, Patwardhan A, Rider LG, Abdul-Aziz R, Wu YL, Lundström E, et al. Gene copy-number variations (CNVs) of complement C4 and C4A deficiency in genetic risk and pathogenesis of juvenile dermatomyositis. Ann Rheum Dis. (2016) 75:1599–606. doi: 10.1136/annrheumdis-2015-207762
83. Agostinis C, Durigutto P, Sblattero D, Borghi MO, Grossi C, Guida F, et al. A non-complement-fixing antibody to beta2 glycoprotein I as a novel therapy for antiphospholipid syndrome. Blood. (2014) 123:3478–87. doi: 10.1182/blood-2013-11-537704
84. Gropp K, Weber N, Reuter M, Micklisch S, Kopka I, Hallström T, et al. beta(2)-glycoprotein I, the major target in antiphospholipid syndrome, is a special human complement regulator. Blood. (2011) 118:2774–83. doi: 10.1182/blood-2011-02-339564
85. Zhu X, Zhang J, Wang Q, Fu H, Chang Y, Kong Y, et al. Diminished expression of beta2-GPI is associated with a reduced ability to mitigate complement activation in anti-GPIIb/IIIa-mediated immune thrombocytopenia. Ann Hematol. (2018) 97:641–54. doi: 10.1007/s00277-017-3215-3
86. Garred P, Voss A, Madsen HO, Junker P. Association of mannose-binding lectin gene variation with disease severity and infections in a population-based cohort of systemic lupus erythematosus patients. Genes Immun. (2001) 2:442–50. doi: 10.1038/sj.gene.6363804
87. Piao W, Liu CC, Kao AH, Manzi S, Vogt MT, Ruffing MJ, et al. Mannose-binding lectin is a disease-modifying factor in North American patients with systemic lupus erythematosus. J Rheumatol. (2007) 34:1506–13.
88. Garred P, Madsen HO, Halberg P, Petersen J, Kronborg G, Svejgaard A, et al. Mannose-binding lectin polymorphisms and susceptibility to infection in systemic lupus erythematosus. Arthritis Rheum. (1999) 42:2145–52. doi: 10.1002/1529-0131(199910)42:10<2145::AID-ANR15>3.0.CO;2-#
89. Øhlenschlaeger T, Garred P, Madsen HO, Jacobsen S. Mannose-binding lectin variant alleles and the risk of arterial thrombosis in systemic lupus erythematosus. N Engl J Med. (2004) 351:260–7. doi: 10.1056/NEJMoa033122
90. Font J, Ramos-Casals M, Brito-Zerón P, Nardi N, Ibañez A, Suarez B, et al. Association of mannose-binding lectin gene polymorphisms with antiphospholipid syndrome, cardiovascular disease and chronic damage in patients with systemic lupus erythematosus. Rheumatology (Oxford). (2007) 46:76–80. doi: 10.1093/rheumatology/kel199
91. Cavusoglu E, Eng C, Chopra V, Ruwende C, Yanamadala S, Clark LT, et al. Usefulness of the serum complement component C4 as a predictor of stroke in patients with known or suspected coronary artery disease referred for coronary angiography. Am J Cardiol. (2007) 100:164–8. doi: 10.1016/j.amjcard.2007.02.075
92. Sugiura-Ogasawara M, Nozawa K, Nakanishi T, Hattori Y, Ozaki Y. Complement as a predictor of further miscarriage in couples with recurrent miscarriages. Hum Reprod. (2006) 21:2711–4. doi: 10.1093/humrep/del229
93. Shamonki JM, Salmon JE, Hyjek E, Baergen RN. Excessive complement activation is associated with placental injury in patients with antiphospholipid antibodies. Am J Obstet Gynecol. (2007) 196:167 e161–5. doi: 10.1016/j.ajog.2006.10.879
Keywords: C3 and C4, C4A and C4B, Copy number variation, Factor H, Lupus anticoagulant, Mannan binding lectin, Recurrent pregnancy loss, Thrombosis
Citation: Savelli SL, Roubey RAS, Kitzmiller KJ, Zhou D, Nagaraja HN, Mulvihill E, Barbar-Smiley F, Ardoin SP, Wu YL and Yu C-Y (2019) Opposite Profiles of Complement in Antiphospholipid Syndrome (APS) and Systemic Lupus Erythematosus (SLE) Among Patients With Antiphospholipid Antibodies (aPL). Front. Immunol. 10:885. doi: 10.3389/fimmu.2019.00885
Received: 11 December 2018; Accepted: 05 April 2019;
Published: 07 May 2019.
Edited by:
José Carlos Crispín, Instituto Nacional de Ciencias Médicas y Nutrición Salvador Zubirán (INCMNSZ), MexicoReviewed by:
Kenji Oku, Hokkaido University, JapanGabriela Hernandez-Molina, Instituto Nacional de Ciencias Médicas y Nutrición Salvador Zubirán (INCMNSZ), Mexico
Copyright © 2019 Savelli, Roubey, Kitzmiller, Zhou, Nagaraja, Mulvihill, Barbar-Smiley, Ardoin, Wu and Yu. This is an open-access article distributed under the terms of the Creative Commons Attribution License (CC BY). The use, distribution or reproduction in other forums is permitted, provided the original author(s) and the copyright owner(s) are credited and that the original publication in this journal is cited, in accordance with accepted academic practice. No use, distribution or reproduction is permitted which does not comply with these terms.
*Correspondence: Stephanie L. Savelli, ssavelli@akronchildrens.org
Robert A. S. Roubey, robert_roubey@med.unc.edu
Yee Ling Wu, ywu24@luc.edu
Chack-Yung Yu, chack-yung.yu@nationwidechildrens.org