- 1Laboratory of Experimental Vaccines, Department of Microbiology, Immunology and Parasitology, Federal University of São Paulo, São Paulo, Brazil
- 2Institute for Investigation in Immunology (iii)—INCT, São Paulo, Brazil
- 3Department of Parasitology, Institute of Biomedical Sciences, University of São Paulo, São Paulo, Brazil
- 4Laboratory of Clinical Immunology and Allergy (LIM60), School of Medicine–University of São Paulo, São Paulo, Brazil
Cellular immune responses are implicated in resistance to HIV and have been considered for the development of an effective vaccine. Despite their safety profile, subunit vaccines need to be delivered combined with an adjuvant. In the last years, in vivo antigen targeting to dendritic cells (DCs) using chimeric monoclonal antibodies (mAb) against the DC endocytic receptor DEC205/CD205 was shown to support long-term T cell immunity. Here, we evaluated the ability of different adjuvants to modulate specific cellular immune response when eight CD4+ HIV-derived epitopes (HIVBr8) were targeted to DEC205+ DCs in vivo. Immunization with two doses of αDECHIVBr8 mAb along with poly(I:C) induced Th1 cytokine production and higher frequency of HIV-specific polyfunctional and long-lived T cells than MPL or CpG ODN-assisted immunization. Although each adjuvant elicited responses against the 8 epitopes present in the vaccine, the magnitude of the T cell response was higher in the presence of poly(I:C). Moreover, poly(I:C) up regulated the expression of costimulatory molecules in both cDC1 and cDC2 DCs subsets. In summary, the use of poly(I:C) in a vaccine formulation that targets multiple epitopes to the DEC205 receptor improved the potency and the quality of HIV-specific responses when compared to other vaccine-adjuvant formulations. This study highlights the importance of the rational selection of antigen/adjuvant combination to potentiate the desired immune responses.
Introduction
Vaccine induced T cell immunity is required for effective protection against intracellular pathogens responsible for diseases classified as global threats like AIDS, tuberculosis, malaria, and also against cancer. The ability of dendritic cells (DCs) to uptake, process and present antigens is crucial to induce and regulate T cell immunity (1). Thus, activation of DCs has been considered key in vaccines designed to induce cellular immunity (2). DCs express a wide range of receptors including pattern recognition receptors (PRRs), like toll-like receptors (TLRs), cytosolic receptors, and C-type lectin receptors, that are able to recognize pathogen- or damage- associated molecular patterns (PAMPs or DAMPs, respectively) (3). The nature of the signal delivered to the DC does not only affect the magnitude of T cell responses, but also influences the generation of memory precursors and the overall quality of immune response (4, 5).
Human and mouse DCs can be divided in two major subsets: plasmacytoid DCs and conventional/myeloid DCs with specific functions in the steady state (6–8). Recently, DCs were classified based on their ontogeny in conventional type 1 DCs (cDC1) and conventional type 2 DCs (cDC2) (9, 10). Conventional type 1 DCs encompass lymphoid CD8α+ and non-lymphoid CD103+, both of which express DEC205. DEC205 also known as CD205 is a C-type lectin endocytic receptor and was the first identified DC-specific receptor (11). DEC205 is highly expressed on cDC1, but can also be found on thymic epithelial cells, Langerhans cells and, at relatively low levels, on B cells (12, 13). Recently, synthetic CpG oligonucleotides (ODNs), a potent immunostimulator, were identified as ligands that bind to the surface DEC205 (14, 15).
A promising strategy to improve vaccine efficacy is to selectively target the desired antigen to a DC subset by linking it to a monoclonal antibody (mAb) against the specific DC receptor. During the last decade, several reports revealed the feasibility of in vivo antigen targeting to cDC1 using a mAb against DEC205 (αDEC205) to improve both humoral and cellular responses (2, 16–20). Vaccination with DEC205 targeted antigens also induced protection in different infection and tumor models (21–23). However, for this particular receptor, inflammatory signals such as adjuvants must be co-administered with the targeted antigen to induce DC maturation, cellular immunity and avoid tolerance (24–26).
Different microbial products such as TLR ligands have been characterized and used as adjuvants to trigger intracellular signaling cascades that result in cytokine production, up regulation of costimulatory molecules and DCs maturation (27–30). Mouse conventional DC subsets differentially express a broad repertoire of TLRs that result in different activating phenotypes and adaptive immunity (31). The co-delivery of TLR ligands and DEC205 targeted antigens has been shown to significantly improve vaccine immunogenicity in mice and in non-human primates (16).
Polyinosinic:polycytidylic acid [poly(I:C)] is a synthetic analog of viral double-stranded RNAs (dsRNAs) that activates TLR3 and RIG-I-like receptors (retinoic acid-inducible gene -I- like receptors, or RLRs) (32). Poly(I:C) is the most commonly administered adjuvant in mice in the context of DC-targeted vaccines using αDEC205 mAbs fused with proteins of interest (18). This strategy has already been tested with chimeric mAbs containing proteins derived from dengue virus (33), Trypanosoma cruzi (34), Plasmodium sp (26, 35, 36), Mycobacterium tuberculosis (37), Yersinia pestis (22), Toxoplasma gondii (23), HIV (21, 38, 39) and also from tumors (40). The excellent results obtained with this adjuvant, justified its use in clinical trials. To improve poly I:C stability (32) in humans, a modified version (poly-ICLC) was developed and used in different trials (41, 42).
Monophosphoryl lipid A (MPL), a chemically derivative of bacterial lipopolysaccharide (LPS), is a TLR4 agonist that preferentially activates the TIR-domain-containing adapter-inducing interferon-β (TRIF) signaling pathway to drive the production of Th1 cytokines and activate CD4+ T cells (43) (44, 45). MPL is the first and only TLR ligand licensed in a human vaccine (Melacine™, approved as a melanoma vaccine). More recently, other MPL-containing vaccines became available (Fendrix™ and Cervarix™, both from GSK) (46). CpG oligodeoxynucleotides (ODN) are unmethylated CpG motifs that interact with endosomal TLR9 and lead to proinflammatory cytokine production by DCs (47). B type ODN has a protective phosphorothioate backbone that protects it from nuclease digestion and enhances its half-life in vivo (48). Several clinical trials were conducted and CpG ODN emerged as a potent adjuvant to induce high antibody titers more quickly and after fewer doses (49, 50). Moreover, CpG ODN has been used along with αDEC205 mAb to target HIV and Plasmodium proteins (51, 52).
Here, we used eight promiscuous HIV-derived CD4+ T cell epitopes (HIVBr8) fused with αDEC205 to target CD11c+ CD8α+ DCs in the presence of different TLR ligands. The hierarchy of adjuvant potency shows that poly(I:C) is a superior adjuvant for the multiepitope DC-targeted vaccine in magnitude, breadth, and longevity.
Materials and Methods
Generation of the Fusion Monoclonal Antibody (mAb)
Plasmids encoding the light and heavy chain of the mouse αDEC205 antibody were kindly provided by Dr. Michel C. Nussenzweig (The Rockefeller University, New York, USA). The plasmid encoding the heavy chain of the mouse DEC205 fused to eight HIV-1 epitopes was previously described and contains the following epitopes: p6 (32-46), p17 (73-89), pol (785-799), gp160 (188-201), rev (11-27), vpr (65-82), vif (144-158), and nef (180-194) (39).
Expression and Purification of αDECHIVBr8 mAb
The production of αDECHIVBr8 mAb [original clone NLDC145 (24)] was performed after transient transfection of human embryonic kidney (HEK) 293T cells (ATCC, CRL-11268) exactly as described elsewhere (33). Briefly, 293T cells were cultured in 150 mm plates (Sarstedt) under standard conditions in Dulbecco's modified Eagle's medium (Invitrogen) supplemented with 1% (v/v) antibiotic-antimycotic (Invitrogen), 1% (v/v) l-glutamine (Invitrogen), and 5% (v/v) Ultra low IgG Fetal Bovine Serum (Invitrogen). When cell confluence reached 70%, 293T cells were transfected using 10 μg of the plasmids encoding the light and the heavy chains in the presence of 150 mM NaCl and 0.45 mg/mL polyethyleneimine (PEI) (Sigma Aldrich). After 7 days in culture at 37°C with 5% CO2, the culture supernatants containing secreted antibodies were collected by centrifugation at 1,000 x g for 30 min at 4°C and filtered through 0.22 μM membrane. The chimeric αDECHIVBr8 mAb was precipitated by addition of ammonium sulfate (Sigma Aldrich) to 60% of the total culture volume, and resuspended/dialyzed overnight against PBS at 4°C. After purification by affinity chromatography with protein G beads column (GE Healthcare), fusion mAb was dialyzed against PBS, resolved on a SDS-12% polyacrylamide gel, quantified, and stored at −20°C until use.
Mice
Female BALB/c (H-2d) mice with 6-to 8-week old were purchased from Centro de Desenvolvimento de Modelos Experimentais para Medicina e Biologia (CEDEME)- Brazil. Mice were housed and manipulated under specific-pathogen-free (SPF) conditions at the animal care facility of the Division of Immunology, Federal University of São Paulo (UNIFESP).
Immunization
Groups of six mice were immunized twice, 2 weeks apart, with 4 μg of αDECHIVBr8 mAb by intraperitoneal (I.P) route in the presence of the following adjuvants: 50 μg of poly(I:C) (Invivogen), 20 μg of Monophosphoryl Lipid A (MPL) (Invivogen), or 10 μg of CpG ODN 1826 (Invivogen). The amount of adjuvants used was previously determined (53). Control groups were immunized with 4 μg of αDECHIVBr8 in the absence of adjuvant or with PBS only.
Spleen and Mesenteric Lymph Node Cell Isolation
Fifteen and sixty days after the administration of the second dose (boost), mice were deeply anesthetized by ketamine/xylazine solution (300 and 30 mg/kg, respectively) and mesenteric lymph nodes and the spleen were aseptically removed. After obtaining single cell suspensions, cells were washed in 10 mL of RPMI 1640 (Gibco). Splenic red blood cells were lysed with 1 mL of ACK solution (150 mM NH3Cl, 10 mM KHCO3, 0.1 mM EDTA) for 2 min at room temperature. After two additional washes with RPMI 1640, splenocytes and lymph node cells were then resuspended in R10 (RPMI supplemented with 10% of fetal bovine serum, 2 mM L-glutamine, 1% v/v vitamin solution, 1mM sodium pyruvate, 1% v/v non-essential amino acids solution, 40 μg/mL of Gentamicin, 5 x 10−5 M 2-mercaptoetanol (all from Gibco) and 20 μg/mL of Cyprofloxacin (Ciprobacter, Isofarma). The viability of cells was evaluated using 0.2% Trypan Blue exclusion dye to discriminate between live and dead cells. Cell concentration was estimated with the aid of a cell counter (Countess, Invitrogen) and adjusted in cell culture medium.
Cytokine Determination
One million splenocytes were incubated for 48 h in the presence of pooled HIV-1 peptides (5 μM) or medium alone as negative control. Culture supernatants were harvested and stored at −20°C until analysis. IL-2, IL-4, IL-6, IL-10, IL-17, IFNγ, and TNFα were detected simultaneously using mouse Th1/Th2/Th17 cytokine bead array (CBA) kit (BD Pharmingen), according to the manufacturer's instructions. The range of detection was 20–5,000 pg/mL for each cytokine.
T Cell ELISpot Assay
The ELISpot assay was performed using mouse IFNγ ELISpot Ready-SET-Go! (eBiosciences) according to manufacturer's instructions. Splenocytes from immunized mice were obtained as described and assayed for their ability to secrete IFNγ after in vitro stimulation with individual or pooled HIV-1 peptides (5 μM) or medium alone as negative control. Spots were counted using an AID ELISPOT Reader System (Autoimmun Diagnostika GmbH, Germany). The number of IFN-γ producing cells/106 splenocytes was calculated after subtracting the negative control values and the cutoff was 15 SFU per million splenocytes.
Analysis of HIV-Specific Proliferation and Intracellular Cytokine Production by Flow Cytometry
To analyze HIV-specific T cell expansion, proliferation, and cytokine production, splenocytes from immunized mice were labeled with carboxyfluorescein succinimidyl ester (CFSE) (54). In summary, freshly isolated splenocytes were resuspended (50 × 106/mL) in PBS and labeled with 1.25 μM of CFSE (Molecular Probes) at 37°C for 10 min. The reaction was quenched with RPMI 1640 supplemented with 10% FBS (R10) and cells were washed/resuspended with R10. Cells were cultured in 96-well round-bottomed plates (5 × 105/well in triplicate) for 5 days at 37°C and 5% CO2 with medium alone or with pooled HIV-1 peptides (5 μM). After 4 days, cells were restimulated with pooled HIV-1 peptides (5 μM) in the presence of 2 μg/mL anti-CD28 (BD Pharmingen) and Brefeldin A- GolgiPlugTM (BD Pharmingen) for further 12 h. After the incubation period, cells were washed with FACS buffer (PBS with 0.5% BSA and 2 mM EDTA) and surface stained with anti-mouse CD3 APCCy7 (clone 145-2C11), CD4 PerCP (clone RM4-5), and CD8 Pacific Blue (clone 53-6.7) monoclonal antibodies for 30 min at 4°C. Cells were fixed and permeabilized using Cytofix/Cytoperm™ kit (BD Pharmingen), according to manufacturer's instructions. After permeabilization, cells were washed with Perm/Wash buffer (BD Biosciences) and stained with anti-mouse IL2 PE (clone JES6-5H4), TNFα PECy7 (clone MP6-XT22), and IFNγ APC (clone XMG1.2) monoclonal antibodies for 30 min at 4°C. Following staining, cells were washed twice and resuspended in FACS buffer. All antibodies were from BD Pharmingen. Samples were acquired on a FACSCanto II flow cytometer (BD Biosciences) and then analyzed using FlowJo software (version 9.9, Tree Star, San Carlo, CA). To analyze cellular polyfunctionality, we used the Boolean gate platform (FlowJo software) to create combinations of the three cytokines (IL-2, TNFα, and IFNγ) within the CFSElow population (cells that have undergone at least one cycle of division) resulting in seven distinct patterns. Polyfunctionality was defined as the ability of cells to exert at least two functions. The gating strategy, illustrated using data from one representative experiment, is shown in Figure S1. The frequencies of cytokine producing cells were calculated by subtracting the frequency of cells that were stimulated in vitro with HIV peptides by the frequency of the cells that were cultured in the presence of medium alone (background). For each experiment performed, unstained and all single-color controls were processed to allow proper compensation.
Expression of Costimulatory Molecules on DC Surface
Mice were immunized once with 4 μg of αDECHIVBr8 mAb combined with the different adjuvants (poly(I:C), MPL or CpG ODN 1826). After 12 h, splenocytes were stained with biotinylated anti-mouse CD3 (clone 145-2C11), CD19 (clone 1D3), and CD49b (clone DX5). After 30 min, cells were washed with FACS buffer and stained with streptavidin APCCy7, anti-mouse CD11c APC (clone HL3), IAIE PE (clone 2G9), CD8 Pacific Blue (clone 53-6.7), CD40 FITC (clone 3.23), CD80 PerCP (clone 16-10A1), and CD86 PECy7 (clone GL1). Samples were acquired on a FACSCanto II flow cytometer (BD Biosciences) and then analyzed using FlowJo software (version 9.9, Tree Star, San Carlo, CA). For each experiment performed, unstained and all single-color controls were processed to allow proper compensation. Three million events were acquired in a live lymphocyte gate.
Data Analysis
Statistical significance (p-value) was calculated by Two-way ANOVA followed by Bonferroni post hoc test or unpaired t-test (different time points comparison). Statistical analysis and graphical representation of data was performed using GraphPad Prism version 7.0 software.
Results
Multiepitope Targeting to DEC205+ DCs With Different Adjuvants Induces Type 1 Cytokine Production
To examine the effect of different adjuvants on HIV-specific cellular immune response, mice were immunized with two doses of αDECHIVBr8 mAb in the presence of the TLR agonists poly(I:C), MPL or CpG ODN 1826. Fifteen or Sixty days after the boost, splenocytes from immunized mice were incubated with pooled HIV-1 peptides to analyze specific cytokine production. First, we evaluated IFNγ production by ELISpot assay (Figure 1A). We observed that 15 days after the boost splenocytes from mice immunized with αDECHIVBr8 mAb combined with poly(I:C) presented higher number of specific IFNγ producing cells (716 SFU/106 cells) when compared to the groups immunized in the presence of MPL or CpG ODN 1826 (404 and 286 SFU/106 cells, respectively). Moreover, a significant difference was observed between MPL and CpG ODN 1826 groups (Figure 1A, left). Sixty days after the boost, we detected the same profile albeit with lower magnitude. Mice immunized with αDECHIVBr8 combined with poly(I:C) displayed 514 SFU/106 cells while MPL and CpG ODN 1826 presented 284 and 142 SFU/106 cells, respectively (Figure 1A, right). A comparison between 15 and 60 days revealed a significant decrease in the magnitude for poly(I:C) (p < 0.001), CpG ODN 1826 (p < 0.001), and MPL (p < 0.01) immunized groups. Splenocytes from mice immunized with αDECHIVBr8 in the absence of adjuvant or PBS (control groups) presented negligible numbers of IFNγ producing cells.
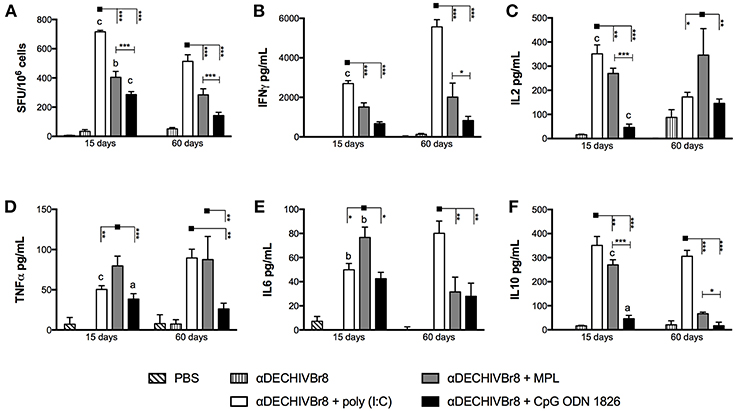
Figure 1. Properties of HIV-specific cellular imune response after immunization with multiepitope αDECHIVBr8 mAb in the presence of adjuvants. BALB/c mice (n = 6) were immunized with two doses of 4 μg of αDECHIVBr8 along with poly(I:C), MPL or CpG ODN 1826. Control groups received αDECHIVBr8 only or PBS. Fifteen and sixty days after the boost the splenocytes were (A) cultured in the presence of pooled HIV-1 peptides (5 μM) for 18 h to evaluate the number of IFN-γ producing cells by ELISpot assay. SFU, spot forming units. Cutoff = 15 SFU/106 cells and is represented by the dotted line. (B–F) cultured in the presence of pooled HIV-1 peptides for 48 h to measure IFNγ (B), IL2 (C), TNFα (D), IL6 (E), and IL10 (F) in culture supernatants by flow cytometry. Data were analyzed by two-way ANOVA followed by Bonferroni post hoc test or unpaired t-test (different time points comparison) *p < 0.05, **p < 0.01; ***p < 0.001; a p < 0.05; b p < 0.01; c p < 0.001 when 15 days was compared to 60 days time point. Data represent mean ± SD and are representative of 3 independent experiments.
We also analyzed the cytokine profile by CBA assay using supernatant culture of splenocytes stimulated with pooled HIV peptides. Splenocytes from mice that received αDECHIVBr8 combined with poly(I:C) produced higher levels of IFNγ when compared to MPL or CpG ODN 1826, corroborating the ELISpot findings (Figure 1B). Interestingly, in the poly(I:C) adjuvanted group IFNγ production was even higher 60 days after the boost when compared to the 15 days time point (p < 0.001). Poly(I:C) also induced superior IL-2 production 15 days after the boost (Figure 1C, left). However, 60 days after the boost, IL-2 production significantly decreased in the group immunized with αDECHIVBr8 plus poly(I:C) and increased in the group that received the mAb in the presence of MPL (p < 0.001) (Figure 1C, right). IL-2 production by the group that received the mAb with CpG ODN 1826 slightly increased 60 days after the boost when compared to 15 days time point (p < 0.001). Regarding TNFα production 15 days after the boost, we observed that αDECHIVBr8 mixed with MPL produced the highest levels (Figure 1D). TNFα levels increased 60 days after the boost for the poly(I:C) group (p < 0.001) and decreased for the CpG ODN 1826 group (p < 0.05). No difference was observed for the MPL immunized group. Inflammatory IL-6 (Figure 1E) was higher in the group immunized with αDECHIVBr8 plus MPL 15 days after boost, but at the later time point the levels of this cytokine significantly decreased (p < 0.01). In contrast, 60 days after the boost with mAb and poly(I:C), IL-6 (p < 0.01) production increased considerably. IL-10 (Figure 1F) was superior in the poly(I:C) immunized group in both time points followed by MPL immunized group. However, after 60 days, IL-10 production decreased in the MPL (p < 0.001) and in the CpG ODN 1826 (p < 0.05) groups. Of note, IL-4 and IL-17 production was below the assay detection limit (data not shown). Taken together, these results indicate that different adjuvants induce a type 1 immune response when multiple HIV-antigens are delivered to CD8α+ DCs by the endocytic receptor DEC205.
Poly(I:C) Promotes Robust and Long-Lived Polyfunctional T Cell Responses
In an attempt to evaluate HIV-specific CD4+ and CD8+ T cell responses, splenocytes from immunized mice were labeled with CFSE and pulsed in vitro with HIV-1 peptides. After culture, the frequency of CD3+CD4+CFSElow (Figure 2A) and CD3+CD8+CFSElow (Figure 2B) were evaluated by flow cytometry. Fifteen days after boost, splenocytes from mice that received αDECHIVBr8 along with poly(I:C) presented higher frequency of proliferating CD4+ (9.96%) and CD8+ (5.90%) T cells when compared to MPL immunized groups (6.83 and 4.86%, respectively). In contrast, CpG ODN 1826 displayed the lowest frequency of proliferating T cells. The same profile was observed 60 days after the boost, with the group that received αDECHIVBr8 plus poly(I:C) displaying higher CD4+ (11.30%) and CD8+ (6.17%) specific proliferation when compared to MPL (CD4+CFSElow 4.86% and CD8+CFSElow 2.47%) or CpG ODN 1826 (CD4+CFSElow 3.60% and CD8+CFSElow 1.31%) (Figures 2A,B right, respectively). Comparative analyses showed significant difference on the frequency of CD4+CFSElow cells between 15 and 60 days only for the group that received αDECHIVBr8 plus MPL (p < 0.05). Regarding the CD8+ T cell compartment (CD8+CFSElow cells), a significant difference was observed for MPL (p < 0.05) or CpG ODN 1826 (p < 0.01) groups. In contrast, mice immunized with αDECHIVBr8 in the presence of poly(I:C) displayed similar frequency of proliferating CD4+ and CD8+ T cells in all time points. To further characterize the functional profile of antigen-specific T cells, we assessed the ability of single cells to proliferate and produce the cytokines IFNγ, TNFα, and IL2 individually or simultaneously. The flow cytometry profile demonstrated that immunization with αDECHIVBr8 mAb along with poly(I:C) induced higher frequency of CD4+ T cells that proliferated and produced IFNγ+IL2+TNFα+ or IFNγ+TNFα+ simultaneously or only one cytokine (IFNγ or TNFα) 15 or 60 days after the boost (Figures 3A,B, respectively). Interestingly, for the poly(I:C) and MPL groups 60 days after the boost, the frequency of polyfunctional CD4+ T cells that proliferated and produced IFNγ, TNFα, and IL-2 simultaneously decreased, leading to an increase in the double or single cytokine producers (Figure 3–pie charts). Moreover, αDECHIVBr8 mixed with poly(I:C) also displayed higher frequency of proliferating CD8+ T cells that produce IFNγ or TNFα 15 or 60 days after the boost when compared with other groups (Figures 3C,D, respectively). Similarly to what was observed with the CD4 compartment at the later time point (60 days), there was also a shift in the CD8+ T cell polyfunctional profile in all groups when compared to 15 days after the boost; the frequency of three cytokine producing cells diminished while the single cytokine producers augmented (Figure 3 pie charts). Altogether, these results demonstrated that immunization with two doses of αDECHIVBr8 along with poly(I:C) induced higher and long-lasting specific polyfunctional CD4+ and CD8+ T cells responses.
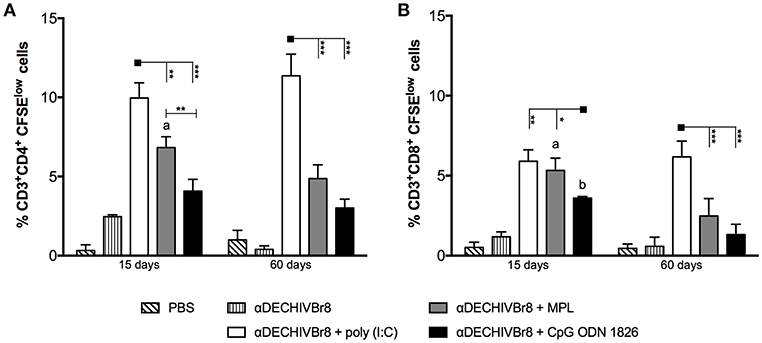
Figure 2. Immunization with αDECHIVBr8 mAb in the presence of poly(I:C) induces robust HIV-specific T cell proliferation. BALB/c mice (n = 6) were immunized as in Figure 1. Fifteen and 60 days after the boost the splenocytes were labeled with CFSE and cultured in the presence of pooled HIV-1 peptides (5 μM) for 5 days to evaluate specific proliferation. CFSE dilution on gated (A) CD3+CD4+ or (B) CD3+CD8+ cells was used as readout for antigen-specific proliferation. One million events were acquired in a live lymphocyte gate. Data were analyzed by two-way ANOVA followed by Bonferroni post hoc test or unpaired t-test (time points comparison). *p < 0.05, **p < 0.01; ***p < 0.001. a p < 0.05; b p < 0.01; c p < 0.001 when 15 days was compared to 60 days time points. Data represent mean ± SD and are representative of 3 independent experiments.
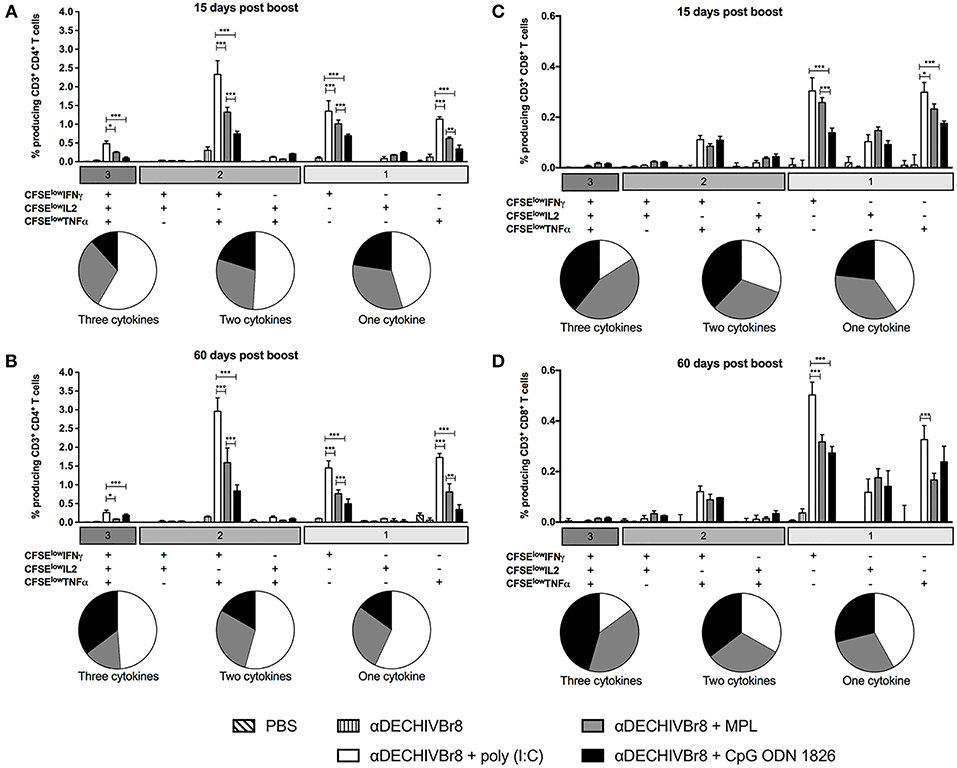
Figure 3. Poly(I:C) induces superior polyfunctional and long-lived HIV- specific T cell responses. BALB/c mice (n = 6) were immunized as in Figure 1. The splenocytes were labeled with CFSE and cultured in the presence of pooled HIV-1 peptides (5 μM) to evaluate specific proliferation and cytokine production by multiparameter flow cytometry. After gating on proliferating (CFSElow) and cytokine-producing cells, boolean combinations were created using FlowJo software to determine the frequency of each response based on all possible combinations of cytokine-producing CD4+ T cells (A,B) and CD8+ T cells (C,D) 15 and 60 days after the boost. Pie charts represent the proportion of T cells producing 1, 2, or all 3 cytokines. One million events were acquired in a live lymphocyte gate. Data were analyzed by two-way ANOVA followed by Bonferroni post hoc test.*p < 0.05, **p < 0.01; ***p < 0.001. Data represent mean ± SD and are representative of 3 independent experiments.
Poly(I:C) Increases Epitope Coverage
To assess the breadth of T cell responses, splenocytes from immunized mice were incubated with single HIV-1 peptides present in the fusion vaccine and the number of IFNγ producing cells was determined by ELISpot. Fifteen days after last dose (Figure 4A), all adjuvants tested were able to induce positive responses against all peptides, albeit at different magnitudes (poly(I:C) > MPL > CpG ODN). At a later time point (Figure 4B), poly(I:C), and CpG ODN adjuvanted groups sustained IFNγ production against all peptides (head-to-head comparison in Figures S2A,C). On the contrary, in the MPL group, the magnitude of the response was more significantly reduced when we compared the 15 and 60 days time points (Figure S2B). Thus, multiepitope in vivo targeting to DEC205+ DCs when combined with poly(I:C) induced broad, potent and long-lasting T cell responses.
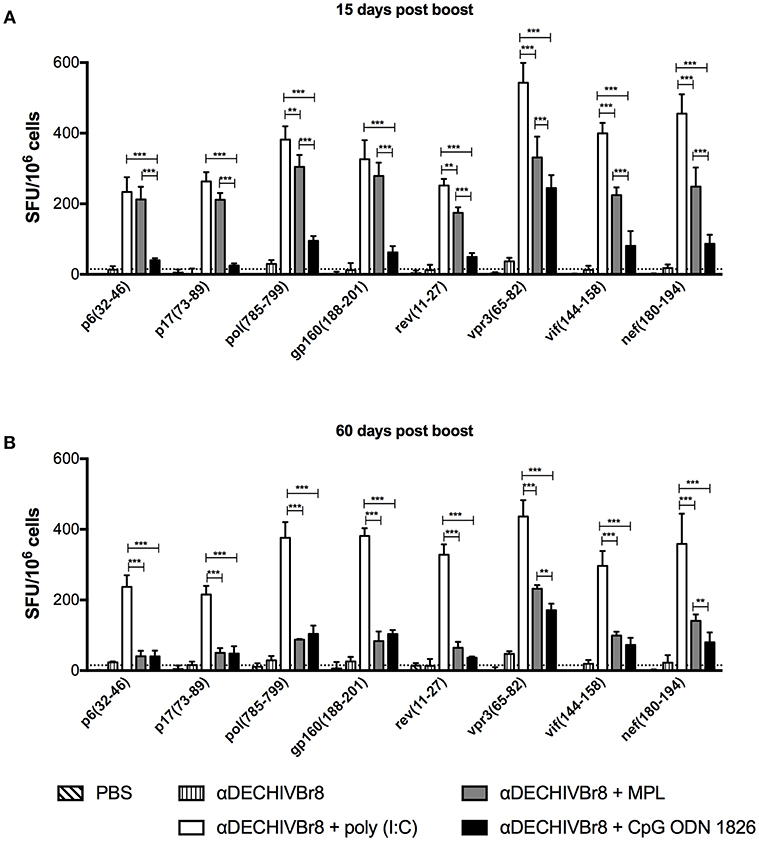
Figure 4. Multiepitope DC targeting in the presence poly(I:C) induces broad T cell responses. BALB/c mice (n = 6) were immunized as described in Figure 1. (A) Fifteen and (B) 60 days after the boost the splenocytes were cultured with single HIV-1 peptides (5 μM) for 18 h to evaluate the number of IFN-γ producing cells by ELISpot assay. SFU, spot forming units. Cutoff = 15 SFU/106 cells and is represented by the dotted line. Data were analyzed by two-way ANOVA followed by Bonferroni post hoc test. **p < 0.01; ***p < 0.001. Data represent mean ± SD and are representative of 4 independent experiments.
Differential Expression of Costimulatory Molecules in Splenic DCs Subsets
To further characterize phenotypic differences among the adjuvants, we compared the maturation status of splenic DCs after in vivo administration of the mAb combined with poly(I:C), MPL or CpG ODN 1826. The gating strategy, illustrated using data from one representative experiment, is shown in Figure S3. Twelve hours after injection, CD11c+CD8α+ DCs from poly(I:C) group considerably up-regulated the expression of CD80 compared to other groups (Figures 5A,B). CpG ODN 1826 slightly increased CD80 expression only when compared to MPL. However, none of the adjuvants up regulated CD80 expression on CD11c+CD8α− DCs. Furthermore, poly(I:C) was the only adjuvant to significantly up regulate CD86 expression in both DCs subsets (Figures 5C,D). Similarly, we observed a significant increase in the MFI of CD40 molecule by poly(I:C) in both DCs subsets when compared to other adjuvants (Figures 5E,F). In addition, to assess whether DC activation could occur earlier than 12 h, we analyzed the expression of costimulatory molecules 6 h after injection, and observed the same pattern of CD80, CD86, and CD40 expression in both DCs subsets (Figures S4A–C, respectively). We also analyzed the activation profile on mesenteric lymph nodes and the same pattern of expression was observed (data not shown). Taken together, these results strength the idea that poly(I:C) is a superior adjuvant than MPL or CpG ODN 1826 since it up regulates costimulatory molecules in both splenic DCs subsets (CD8α+ and CD8α−).
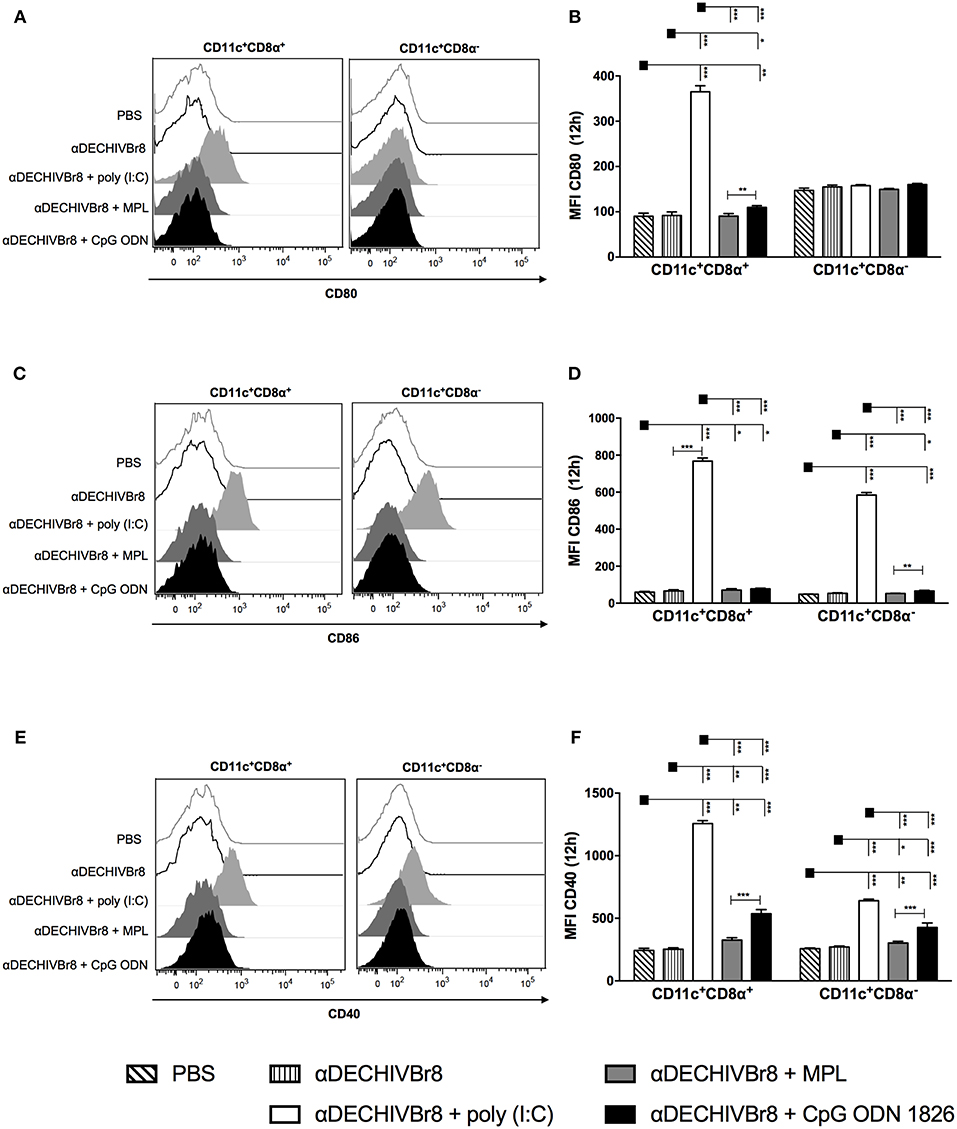
Figure 5. DC targeting in the presence of different adjuvants modulates the expression of costimulatory molecules in splenic DC subsets. BALB/c mice (n = 5) were injected with 4 μg of αDECHIVBr8 plus poly(I:C), MPL or CpG ODN 1826. Control groups received αDECHIVBr8 only or PBS. After 12 h the splenocytes were labeled with fluorescent antibodies and 3 million events were acquired. Initial gating included a single cell gate followed by selection of CD3−CD19−CD49b− population. DCs were identified as CD11c+ IAIE +, subsequently gated on CD8α+ and CD8α− and the median fluorescence intensity (MFI) of CD80 (A,B), CD86 (C,D), and CD40 (E,F) was determined in each DC subset. Data were analyzed by two-way ANOVA followed by Bonferroni post hoc test. *p < 0.05, **p < 0.01; ***p < 0.001. Data represent mean ± SD and are representative of 3 independent experiments.
Discussion
Antigen targeting to DCs through DEC205 endocytic receptor is an effective way to enhance antigen uptake. However, the induction of cell immunity is only accomplished when αDEC205 chimeric mAbs are delivered together with an adjuvant (55–57). Adjuvants enhance immunity to vaccine antigens by influencing the magnitude, breadth/immunodominance, and persistence of immune responses (27). Hence, the choice of the adjuvant formulation is of utmost importance to induce the desired immune response (58). Although a limited number of vaccine adjuvants are currently licensed for human use (aluminum salts, MF59, AS03, and AS04), several compounds have entered clinical trials with demonstrated efficacy (27).
Antigen targeting to DCs through DEC205 receptor is used as a vaccination strategy to induce strong antigen-specific immune responses against several pathogens (26, 34, 55) and tumors In the HIV vaccine scenario, antigen targeting to cDC1 through DEC205 was performed using the full-length gag (p24) protein (21, 51, 59–62). The success in different pre-clinical studies using mice and non-human primates (16) quickly pushed forward the translation of this strategy to humans. Recently, two phase I clinical trials (NCT01889719 and NCT01127464) delivered HIV p24 using a human αDEC205 mAb plus poly-ICLC as adjuvant. Promising results were obtained when a human αDEC205 mAb fused to the full-length tumor antigen NY-ESO-1 was administered together with poly-ICLC (41, 42). In fact, three phase I/IIb clinical trials are currently under way (NCT02166905, NCT03206047, NCT03358719) and two others are already completed (NCT01522820, NCT00948961, NCT01834248).
Previously, we generated an αDEC205 multiepitope fusion mAb (αDECHIVBr8) to target eight promiscuous CD4+ T cell epitopes from several HIV proteins to cDC1s. The αDECHIVBr8 mAb was administered to mice in the presence of poly(I:C) as adjuvant and compared to DNA plasmid immunization in homologous and heterologous prime-boost regimens. We found that αDECHIVBr8 homologous prime-boost regimen induced stronger T cell immune responses against all epitopes when compared to homologous DNA vaccination (39). Here, we compared the adjuvant properties of poly(I:C), MPL, and CpG ODN 1826 to induce HIV-specific cellular immune response when formulated with the fusion αDECHIVBr8 mAb. To our knowledge, this is the first time that multiple epitopes derived from different proteins of the same pathogen are targeted in vivo to DCs and tested in the context of different adjuvants. This is an important issue since adjuvants can influence immunodominance by altering the immune repertoire of CD4 T cell responses (63). Overall, our data reveal the potential of poly(I:C) as a superior adjuvant for the development of a multiepitope-based vaccine that targets CD8α+ DCs through the DEC205 endocytic receptor.
Initially, we found that poly(I:C) induced higher magnitude of specific IFNγ producing cells and also Th1 cytokine production when compared to MPL or CpG ODN 1826. Likewise, Longhi et al. showed that poly(I:C) is a more potent adjuvant to induce specific immune responses against a DC-targeted HIV gag protein (51). Indeed, poly(I:C) has been the most commonly administered adjuvant with DC-targeted vaccines using αDEC205 mAbs fused with full-length proteins from different pathogens in both mice and non-human primates (21–23, 33–39, 60).
Poly(I:C) is sensed by TLR3 and RLR receptors, and triggers up regulation of costimulatory molecules, strong type I IFN production by DCs and Th1 responses (32). Type I IFNs mediate the adjuvant effect of poly(I:C) acting as a third signal by promoting and sustaining clonal expansion of T cells (64–68). Indeed, our results demonstrate that immunization with αDECHIVBr8 along with poly(I:C) also induced higher frequency of proliferating CD4+ and CD8+ T cells. Moreover, we found that administration of the αDECHIVBr8 mAb concomitant with poly(I:C) induced higher frequency of specific polyfunctional T cells, i.e., cells that proliferated and simultaneously produced Th1 cytokines (IFNγ, IL2, and TNFα). Ours results corroborate with previous reports showing the development of polyfunctional T cells after HIV gag protein targeting to DCs along with poly(I:C) (21, 38, 62). Additionally, the presence of polyfunctional T cells is also a hallmark after vaccinia and yellow fever virus vaccinations (69, 70), and correlates with non-progressive HIV infection (71, 72). Recent HIV vaccine trials suggest that a broad (multiple specificities) and potent (high magnitude) response against conserved epitopes would be a desirable attribute of a T-cell based vaccine (73, 74). Indeed, vaccine induced broad T cell responses conferred protection after simian immunodeficiency virus challenge (75). We showed that poly(I:C) and MPL induced T cell responses against all epitopes (broad responses) present in the αDECHIVBr8 fusion mAb, although poly(I:C) was more potent. Likewise, Teixeira et al. demonstrated the ability of a bacterial adjuvant (Propionibacterium acnes) to expand the breath of a multiepitope DNA-based HIV vaccine (76).
A central feature of successful vaccines is their ability to induce immunological memory. Cross-sectional studies of smallpox and yellow fever vaccines showed that specific humoral and T cell responses can be detected for many years (77, 78). When we analyzed the longevity of the immune response, only poly(I:C) vaccine group had sustained T cell proliferation and IFNγ responses against all peptides ~2 months after the second immunization. It is important to note that MPL was the second most potent adjuvant tested and better to induce pro-inflammatory cytokines such as TNFα and IL-6. Previous reports provided evidence that MPL, a TLR2, and TLR4 agonist, is effective to induce TNFα, IL-10, and IL-12 production (44, 79). MPL induced a broad T cell response after the boost but narrowed after 2 months. Previous reports using αDEC205 mAb fused with HIV gag protein showed that MPL or LPS were as effective as poly(I:C) to induce specific humoral responses but less potent to induce Th1 CD4+ T cell immunity (38, 51).
Interestingly, immunization with αDECHIVBr8 in the presence of CpG ODN induced weak T cell responses and narrowed epitope positivity. B class CpG ODN is a fully phosphorothioate TLR9 agonist that binds to surface DEC205 receptor (14, 15) and could therefore compete with the fusion αDEC205 mAb for cellular uptake. Our data are in line with a previous study demonstrating that immunization with αDEC-Gag plus CpG ODN 1826 induces lower frequency of responding CD4+ T cells compared with poly(I:C) (51).
Anti-DECHIVBr8 combined with poly(I:C) was the most effective strategy to modulate DC activation by up regulating costimulatory molecules in a more pronounced way in the CD11c+ CD8α+ subset but also in CD11c+ CD8α− DCs. This may be due to the fact that CD8α+ DEC205+ DCs express higher levels of TLR3 when compared to CD8α− DCs (2, 18, 80). As a consequence of DC maturation, poly(I:C) enhanced T cell immunity. As stated before, it was shown that poly(I:C) was most effective to induce Th1 CD4+ T cell immunity compared to LPS or CpG ODN 1826 using the HIV gag targeted protein (51).
The use of mouse model to select an adjuvant may be a caveat since the pattern of expression of TLR in the target DEC205+ DC subset can differ between human and mouse (18). However, the adjuvant effect after antigen targeting does not necessarily rely on the direct activation of its respective TLR. For example, the effect of poly(I:C) on cDC1 is mediated by type I IFN receptor (51) suggesting that it is possible to have immune activation even if the targeted DC does not express a certain TLR.
Collectively, the observations demonstrate that combination of poly(I:C) with multiepitope targeting to DEC205+ DCs modulates DC activation and elicits strong, broad, polyfunctional, and long-lived Th1 responses superior to other adjuvants both in quantity and quality. Therefore, the pursuit of a safe and effective T cell-based vaccine may benefit from the proper association of multiple epitope targeting to DC populations using a potent adjuvant formulation.
Ethics Statement
This study was carried out in accordance with the recommendations of the Federal Law 11.794 (2008) and the Guide for the Care and Use of Laboratory Animals of the Brazilian National Council of Animal Experimentation (CONCEA). The protocol was approved by the UNIFESP Animal Care and Use Committee (IACUC).
Author Contributions
JA, SB, and DR conceived and designed the experiments. JA, VL, MY, and DR performed the experiments. JA, VL, and DR analyzed the data and prepared the figures. DR, SB, and EC-N contributed with reagents and materials. JA, VL, SB, and DR wrote the manuscript. SB, EC-N, and DR performed the final review of the article. All authors read and approved the final article.
Funding
This research was supported by the São Paulo Research Foundation (FAPESP, grant numbers 2014/50631-0 and 2017/17471-7), the Brazilian National Research Council (CNPq)/Institute for Investigation in Immunology and the Coordenação de Aperfeiçoamento de Pessoal de Nível Superior-Brasil (CAPES, Finance Code 001). JA, VL, EC-N, SB, and DR received fellowships from CNPq/ FAPESP.
Conflict of Interest Statement
The authors declare that the research was conducted in the absence of any commercial or financial relationships that could be construed as a potential conflict of interest.
Acknowledgments
We thank Mr. Geová Santos for assistance in the animal facility.
Supplementary Material
The Supplementary Material for this article can be found online at: https://www.frontiersin.org/articles/10.3389/fimmu.2019.00843/full#supplementary-material
References
1. Lopez-Bravo M, Ardavin C. In vivo induction of immune responses to pathogens by conventional dendritic cells. Immunity. (2008) 29:343–51. doi: 10.1016/j.immuni.2008.08.008
2. Cohn L, Delamarre L. Dendritic cell-targeted vaccines. Front Immunol. (2014) 5:255. doi: 10.3389/fimmu.2014.00255
3. Iwasaki A, Medzhitov R. Control of adaptive immunity by the innate immune system. Nat Immunol. (2015) 16:343–53. doi: 10.1038/ni.3123
4. Joshi NS, Cui W, Chandele A, Lee HK, Urso DR, Hagman J, et al. Inflammation directs memory precursor and short-lived effector CD8(+) T cell fates via the graded expression of T-bet transcription factor. Immunity. (2007) 27:281–95. doi: 10.1016/j.immuni.2007.07.010
5. Sarkar S, Teichgraber V, Kalia V, Polley A, Masopust D, Harrington LE, et al. Strength of stimulus and clonal competition impact the rate of memory CD8 T cell differentiation. J Immunol. (2007) 179:6704–14. doi: 10.4049/jimmunol.179.10.6704
6. Shortman K, Naik SH. Steady-state and inflammatory dendritic-cell development. Nat Rev Immunol. (2007) 7:19–30. doi: 10.1038/nri1996
7. Villadangos JA, Schnorrer P. Intrinsic and cooperative antigen-presenting functions of dendritic-cell subsets in vivo. Nat Rev Immunol. (2007) 7:543–55. doi: 10.1038/nri2103
8. Steinman RM, Idoyaga J. Features of the dendritic cell lineage. Immunol Rev. (2010) 234:5–17. doi: 10.1111/j.0105-2896.2009.00888.x
9. Guilliams M, Ginhoux F, Jakubzick C, Naik SH, Onai N, Schraml BU, et al. Dendritic cells, monocytes and macrophages: a unified nomenclature based on ontogeny. Nat Rev Immunol. (2014) 14:571–8. doi: 10.1038/nri3712
10. Guilliams M, Dutertre CA, Scott CL, McGovern N, Sichien D, Chakarov S, et al. Unsupervised high-dimensional analysis aligns dendritic cells across tissues and species. Immunity. (2016) 45:669–84. doi: 10.1016/j.immuni.2016.08.015
11. Kraal G, Breel M, Janse M, Bruin G. Langerhans' cells, veiled cells, and interdigitating cells in the mouse recognized by a monoclonal antibody. J Exp Med. (1986) 163:981–97.
12. Inaba K, Swiggard WJ, Inaba M, Meltzer J, Mirza A, Sasagawa T, et al. Tissue distribution of the DEC-205 protein that is detected by the monoclonal antibody NLDC-145. I Expression on dendritic cells and other subsets of mouse leukocytes. Cell Immunol. (1995) 163:148–56.
13. Merad M, Sathe P, Helft J, Miller J, Mortha A. The dendritic cell lineage: ontogeny and function of dendritic cells and their subsets in the steady state and the inflamed setting. Annu Rev Immunol. (2013) 31:563–604. doi: 10.1146/annurev-immunol-020711-074950
14. Lahoud MH, Ahmet F, Zhang JG, Meuter S, Policheni AN, Kitsoulis S, et al. DEC-205 is a cell surface receptor for CpG oligonucleotides. Proc Natl Acad Sci USA. (2012) 109:16270–5. doi: 10.1073/pnas.1208796109
15. Caminschi I, Meuter S, Heath WR. DEC-205 is a cell surface receptor for CpG oligonucleotides. Oncoimmunology. (2013) 2:e23128. doi: 10.4161/onci.23128
16. Trumpfheller C, Longhi MP, Caskey M, Idoyaga J, Bozzacco L, Keler T, et al. Dendritic cell-targeted protein vaccines: a novel approach to induce T-cell immunity. J Intern Med. (2012) 271:183–92. doi: 10.1111/j.1365-2796.2011.02496.x
17. Apostolopoulos V, Thalhammer T, Tzakos AG, Stojanovska L. Targeting antigens to dendritic cell receptors for vaccine development. J Drug Deliv. (2013) 2013:869718. doi: 10.1155/2013/869718
18. Kastenmuller W, Kastenmuller K, Kurts C, Seder RA. Dendritic cell-targeted vaccines–hope or hype? Nat Rev Immunol. (2014) 14:705–11. doi: 10.1038/nri3727
19. Park CG. Vaccine strategies utilizing C-type lectin receptors on dendritic cells in vivo. Clin Exp Vaccine Res. (2014) 3:149–54. doi: 10.7774/cevr.2014.3.2.149
20. Macri C, Dumont C, Johnston AP, Mintern JD. Targeting dendritic cells: a promising strategy to improve vaccine effectiveness. Clin Transl Immunol. (2016) 5:e66. doi: 10.1038/cti.2016.6
21. Trumpfheller C, Finke JS, Lopez CB, Moran TM, Moltedo B, Soares H, et al. Intensified and protective CD4+ T cell immunity in mice with anti-dendritic cell HIV gag fusion antibody vaccine. J Exp Med. (2006) 203:607–17. doi: 10.1084/jem.20052005
22. Do Y, Koh H, Park CG, Dudziak D, Seo P, Mehandru S, et al. Targeting of LcrV virulence protein from Yersinia pestis to dendritic cells protects mice against pneumonic plague. Eur J Immunol. (2010) 40:2791–6. doi: 10.1002/eji.201040511
23. Lakhrif Z, Moreau A, Herault B, Di-Tommaso A, Juste M, Moire N, et al. Targeted delivery of Toxoplasma gondii antigens to dendritic cells promote immunogenicity and protective efficiency against toxoplasmosis. Front Immunol. (2018) 9:317. doi: 10.3389/fimmu.2018.00317
24. Hawiger D, Inaba K, Dorsett Y, Guo M, Mahnke K, Rivera M, et al. Dendritic cells induce peripheral T cell unresponsiveness under steady state conditions in vivo. J Exp Med. (2001) 194:769–79. doi: 10.1084/jem.194.6.769
25. Bonifaz L, Bonnyay D, Mahnke K, Rivera M, Nussenzweig MC, Steinman RM. Efficient targeting of protein antigen to the dendritic cell receptor DEC-205 in the steady state leads to antigen presentation on major histocompatibility complex class I products and peripheral CD8+ T cell tolerance. J Exp Med. (2002) 196:1627–38. doi: 10.1084/jem.20021598
26. Boscardin SB, Hafalla JC, Masilamani RF, Kamphorst AO, Zebroski HA, Rai U, et al. Antigen targeting to dendritic cells elicits long-lived T cell help for antibody responses. J Exp Med. (2006) 203:599–606. doi: 10.1084/jem.20051639
27. Coffman RL, Sher A, Seder RA. Vaccine adjuvants: putting innate immunity to work. Immunity. (2010) 33:492–503. doi: 10.1016/j.immuni.2010.10.002
28. Kwissa M, Nakaya HI, Oluoch H, Pulendran B. Distinct TLR adjuvants differentially stimulate systemic and local innate immune responses in nonhuman primates. Blood. (2012) 119:2044–55. doi: 10.1182/blood-2011-10-388579
29. Gutjahr A, Tiraby G, Perouzel E, Verrier B, Paul S. Triggering intracellular receptors for vaccine adjuvantation. Trends Immunol. (2016) 37:P716. doi: 10.1016/j.it.2016.08.005
30. McElrath MJ. Adjuvants: tailoring humoral immune responses. Curr Opin HIV AIDS. (2017) 12:278–84. doi: 10.1097/COH.0000000000000365
31. Hemmi H, Akira S. TLR signalling and the function of dendritic cells. Chem Immunol Allergy. (2005) 86:120–35. doi: 10.1159/000086657
32. Martins KA, Bavari S, Salazar AM. Vaccine adjuvant uses of poly-IC and derivatives. Exp Rev Vaccines. (2015) 14:447–59. doi: 10.1586/14760584.2015.966085
33. Henriques HR, Rampazo EV, Goncalves AJ, Vicentin EC, Amorim JH, Panatieri RH, et al. Targeting the non-structural protein 1 from dengue virus to a dendritic cell population confers protective immunity to lethal virus challenge. PLoS Negl Trop Dis. (2013) 7:e2330. doi: 10.1371/journal.pntd.0002330
34. Rampazo EV, Amorim KN, Yamamoto MM, Panatieri RH, Rodrigues MM, Boscardin SB. Antigen targeting to dendritic cells allows the identification of a CD4 T-cell epitope within an immunodominant Trypanosoma cruzi antigen. PLoS ONE. (2015) 10:e0117778. doi: 10.1371/journal.pone.0117778
35. Tewari K, Flynn BJ, Boscardin SB, Kastenmueller K, Salazar AM, Anderson CA, et al. Poly(I:C) is an effective adjuvant for antibody and multi-functional CD4+ T cell responses to Plasmodium falciparum circumsporozoite protein (CSP) and alphaDEC-CSP in non human primates. Vaccine. (2010) 28:7256–66. doi: 10.1016/j.vaccine.2010.08.098
36. Amorim KN, Rampazo EV, Antonialli R, Yamamoto MM, Rodrigues MM, Soares IS, et al. The presence of T cell epitopes is important for induction of antibody responses against antigens directed to DEC205(+) dendritic cells. Sci Rep. (2016) 6:39250. doi: 10.1038/srep39250
37. Silva-Sanchez A, Meza-Perez S, Flores-Langarica A, Donis-Maturano L, Estrada-Garcia I, Calderon-Amador J, et al. ESAT-6 targeting to DEC205+ Antigen presenting cells induces specific-T cell responses against ESAT-6 and reduces pulmonary infection with virulent Mycobacterium tuberculosis. PLoS ONE. (2015) 10:e0124828. doi: 10.1371/journal.pone.0124828
38. Trumpfheller C, Caskey M, Nchinda G, Longhi MP, Mizenina O, Huang Y, et al. The microbial mimic poly IC induces durable and protective CD4+ T cell immunity together with a dendritic cell targeted vaccine. Proc Natl Acad Sci USA. (2008) 105:2574–9. doi: 10.1073/pnas.0711976105
39. Apostolico JS, Lunardelli VA, Yamamoto MM, Souza HF, Cunha-Neto E, Boscardin SB, et al. Dendritic cell targeting effectively boosts T cell responses elicited by an HIV multiepitope DNA vaccine. Front Immunol. (2017) 8:101. doi: 10.3389/fimmu.2017.00101
40. Gurer C, Strowig T, Brilot F, Pack M, Trumpfheller C, Arrey F, et al. Targeting the nuclear antigen 1 of Epstein-Barr virus to the human endocytic receptor DEC-205 stimulates protective T-cell responses. Blood. (2008) 112:1231–9. doi: 10.1182/blood-2008-03-148072
41. Dhodapkar MV, Sznol M, Zhao B, Wang D, Carvajal RD, Keohan ML, et al. Induction of antigen-specific immunity with a vaccine targeting NY-ESO-1 to the dendritic cell receptor DEC-205. Sci Transl Med. (2014) 6:232ra251. doi: 10.1126/scitranslmed.3008068
42. Griffiths EA, Srivastava P, Matsuzaki J, Brumberger Z, Wang ES, Kocent J, et al. NY-ESO-1 vaccination in combination with decitabine induces antigen-specific T-lymphocyte responses in patients with myelodysplastic syndrome. Clin Cancer Res. (2018) 24:1019–29. doi: 10.1158/1078-0432.CCR-17-1792
43. Thompson BS, Chilton PM, Ward JR, Evans JT, Mitchell TC. The low-toxicity versions of LPS, MPL adjuvant and RC529, are efficient adjuvants for CD4+ T cells. J Leukoc Biol. (2005) 78:1273–80. doi: 10.1189/jlb.0305172
44. Mata-Haro V, Cekic C, Martin M, Chilton PM, Casella CR, Mitchell TC. The vaccine adjuvant monophosphoryl lipid A as a TRIF-biased agonist of TLR4. Science. (2007) 316:1628–32. doi: 10.1126/science.1138963
45. Gandhapudi SK, Chilton PM, Mitchell TC. TRIF is required for TLR4 mediated adjuvant effects on T cell clonal expansion. PLoS ONE. (2013) 8:e56855. doi: 10.1371/journal.pone.0056855
46. Garcon N, Di Pasquale A. From discovery to licensure, the adjuvant system story. Hum Vaccin Immunother. (2017) 13:19–33. doi: 10.1080/21645515.2016.1225635
47. Hemmi H, Kaisho T, Takeda K, Akira S. The roles of Toll-like receptor 9, MyD88, and DNA-dependent protein kinase catalytic subunit in the effects of two distinct CpG DNAs on dendritic cell subsets. J Immunol. (2003) 170:3059–64. doi: 10.4049/jimmunol.170.6.3059
48. Mutwiri GK, Nichani AK, Babiuk S, Babiuk LA. Strategies for enhancing the immunostimulatory effects of CpG oligodeoxynucleotides. J Control Release. (2004) 97:1–17. doi: 10.1016/j.jconrel.2004.02.022
49. Barry M, Cooper C. Review of hepatitis B surface antigen-1018 ISS adjuvant-containing vaccine safety and efficacy. Expert Opin Biol Ther. (2007) 7:1731–7. doi: 10.1517/14712598.7.11.1731
50. Shirota H., and Klinman D. M. (2017). “CpG Oligodeoxynucleotides as adjuvants for clinical use,” in Immunopotentiators in Modern Vaccines, eds V.Schijns, and D. O'Hagan (Cambridge, MA: Academic Press), 167–198.
51. Longhi MP, Trumpfheller C, Idoyaga J, Caskey M, Matos I, Kluger C, et al. Dendritic cells require a systemic type I interferon response to mature and induce CD4+ Th1 immunity with poly IC as adjuvant. J Exp Med. (2009) 206:1589–602. doi: 10.1084/jem.20090247
52. Antonialli R, Sulczewski FB, Amorim K, Almeida BDS, Ferreira NS, Yamamoto MM, et al. CpG oligodeoxinucleotides and flagellin modulate the immune response to antigens targeted to CD8alpha(+) and CD8alpha(-) conventional dendritic cell subsets. Front Immunol. (2017) 8:1727. doi: 10.3389/fimmu.2017.01727
53. Apostolico Jde S, Boscardin SB, Yamamoto MM, de Oliveira-Filho JN, Kalil J, Cunha-Neto E, et al. HIV envelope trimer specific immune response is influenced by different adjuvant formulations and heterologous prime-boost. PLoS ONE. (2016) 11:e0145637. doi: 10.1371/journal.pone.0145637
54. Rosa DS, Ribeiro SP, Almeida RR, Mairena EC, Postol E, Kalil J, et al. A DNA vaccine encoding multiple HIV CD4 epitopes elicits vigorous polyfunctional, long-lived CD4+ and CD8+ T cell responses. PLoS ONE. (2011) 6:e16921. doi: 10.1371/journal.pone.0016921
55. Bonifaz LC, Bonnyay DP, Charalambous A, Darguste DI, Fujii S, Soares H, et al. In vivo targeting of antigens to maturing dendritic cells via the DEC-205 receptor improves T cell vaccination. J Exp Med. (2004) 199:815–24. doi: 10.1084/jem.20032220
56. Dudziak D, Kamphorst AO, Heidkamp GF, Buchholz VR, Trumpfheller C, Yamazaki S, et al. Differential antigen processing by dendritic cell subsets in vivo. Science. (2007) 315:107–11. doi: 10.1126/science.1136080
57. Kwissa M, Kasturi SP, Pulendran B. The science of adjuvants. Expert Rev Vaccines. (2007) 6:673–84. doi: 10.1586/14760584.6.5.673
58. Apostolico Jde S, Lunardelli VA, Coirada FC, Boscardin SB, Rosa DS. Adjuvants: classification, modus operandi, and licensing. J Immunol Res. (2016) 2016:1459394. doi: 10.1155/2016/1459394
59. Cheong C, Choi JH, Vitale L, He LZ, Trumpfheller C, Bozzacco L, et al. Improved cellular and humoral immune responses in vivo following targeting of HIV Gag to dendritic cells within human anti-human DEC205 monoclonal antibody. Blood. (2010) 116:3828–38. doi: 10.1182/blood-2010-06-288068
60. Flynn BJ, Kastenmuller K, Wille-Reece U, Tomaras GD, Alam M, Lindsay RW, et al. Immunization with HIV Gag targeted to dendritic cells followed by recombinant New York vaccinia virus induces robust T-cell immunity in nonhuman primates. Proc Natl Acad Sci USA. (2011) 108:7131–6. doi: 10.1073/pnas.1103869108
61. Idoyaga J, Lubkin A, Fiorese C, Lahoud MH, Caminschi I, Huang Y, et al. Comparable T helper 1 (Th1) and CD8 T-cell immunity by targeting HIV gag p24 to CD8 dendritic cells within antibodies to Langerin, DEC205, and Clec9A. Proc Natl Acad Sci USA. (2011) 108:2384–9. doi: 10.1073/pnas.1019547108
62. Ruane D, Do Y, Brane L, Garg A, Bozzacco L, Kraus T, et al. A dendritic cell targeted vaccine induces long-term HIV-specific immunity within the gastrointestinal tract. Mucosal Immunol. (2016) 9:1340–52. doi: 10.1038/mi.2015.133
63. Baumgartner CK, Malherbe LP. Regulation of CD4 T-cell receptor diversity by vaccine adjuvants. Immunology. (2010) 130:16–22. doi: 10.1111/j.1365-2567.2010.03265.x
64. Marrack P, Kappler J, Mitchell T. Type I interferons keep activated T cells alive. J Exp Med. (1999) 189:521–30.
65. Curtsinger JM, Valenzuela JO, Agarwal P, Lins D, Mescher MF. Type I IFNs provide a third signal to CD8 T cells to stimulate clonal expansion and differentiation. J Immunol. (2005) 174:4465–9.
66. Havenar-Daughton C, Kolumam GA, Murali-Krishna K. Cutting edge: the direct action of type I IFN on CD4 T cells is critical for sustaining clonal expansion in response to a viral but not a bacterial infection. J Immunol. (2006) 176:3315–9. doi: 10.4049/jimmunol.176.6.3315
67. Wiesel M, Kratky W, Oxenius A. Type I IFN substitutes for T cell help during viral infections. J Immunol. (2011) 186:754–63. doi: 10.4049/jimmunol.1003166
68. Wiesel M, Crouse J, Bedenikovic G, Sutherland A, Joller N, Oxenius A. Type-I IFN drives the differentiation of short-lived effector CD8+ T cells in vivo. Eur J Immunol. (2012) 42:320–9. doi: 10.1002/eji.201142091
69. Precopio ML, Betts MR, Parrino J, Price DA, Gostick E, Ambrozak DR, et al. Immunization with vaccinia virus induces polyfunctional and phenotypically distinctive CD8(+) T cell responses. J Exp Med. (2007) 204:1405–16. doi: 10.1084/jem.20062363
70. Gaucher D, Therrien R, Kettaf N, Angermann BR, Boucher G, Filali-Mouhim A, et al. Yellow fever vaccine induces integrated multilineage and polyfunctional immune responses. J Exp Med. (2008) 205:3119–31. doi: 10.1084/jem.20082292
71. Harari A, Petitpierre S, Vallelian F, Pantaleo G. Skewed representation of functionally distinct populations of virus-specific CD4 T cells in HIV-1-infected subjects with progressive disease: changes after antiretroviral therapy. Blood. (2004) 103:966–72. doi: 10.1182/blood-2003-04-1203
72. Kannanganat S, Kapogiannis BG, Ibegbu C, Chennareddi L, Goepfert P, Robinson HL, et al. Human immunodeficiencyvirus type 1 controllers but not noncontrollers maintain CD4 T cells coexpressing three cytokines. J Virol. (2007) 81:12071–6. doi: 10.1128/JVI.01261-07
73. McElrath MJ, Haynes BF. Induction of immunity to human immunodeficiency virus type-1 by vaccination. Immunity. (2010) 33:542–54. doi: 10.1016/j.immuni.2010.09.011
74. Sahay B, Nguyen CQ, Yamamoto JK. Conserved HIV epitopes for an effective HIV vaccine. J Clin Cell Immunol. (2017) 8:518. doi: 10.4172/2155-9899.1000518
75. Wilson NA, Keele BF, Reed JS, Piaskowski SM, MacNair CE, Bett AJ, et al. Vaccine-induced cellular responses control simian immunodeficiency virus replication after heterologous challenge. J Virol. (2009) 83:6508–21. doi: 10.1128/JVI.00272-09
76. Teixeira D, Ishimura ME, Apostolico JS, Viel JM, Passarelli VC, Cunha-Neto E, et al. Propionibacterium acnes enhances the immunogenicity of HIVBr18 human immunodeficiency virus-1 vaccine. Front Immunol. (2018) 9:177. doi: 10.3389/fimmu.2018.00177
77. Akondy RS, Monson ND, Miller JD, Edupuganti S, Teuwen D, Wu H, et al. The yellow fever virus vaccine induces a broad and polyfunctional human memory CD8+ T cell response. J Immunol. (2009) 183:7919–30. doi: 10.4049/jimmunol.0803903
78. Wang M, Tang ST, Lund O, Dziegiel MH, Buus S, Claesson MH. High-affinity human leucocyte antigen class I binding variola-derived peptides induce CD4+ T cell responses more than 30 years post-vaccinia virus vaccination. Clin Exp Immunol. (2009) 155:441–6. doi: 10.1111/j.1365-2249.2008.03856.x
79. Martin M, Michalek SM, Katz J. Role of innate immune factors in the adjuvant activity of monophosphoryl lipid A. Infect Immun. (2003) 71:2498–507. doi: 10.1128/IAI.71.5.2498-2507.2003
Keywords: HIV, multiepitope vaccine, dendritic cell targeting, DEC205, adjuvants
Citation: Apostólico JS, Lunardelli VAS, Yamamoto MM, Cunha-Neto E, Boscardin SB and Rosa DS (2019) Poly(I:C) Potentiates T Cell Immunity to a Dendritic Cell Targeted HIV-Multiepitope Vaccine. Front. Immunol. 10:843. doi: 10.3389/fimmu.2019.00843
Received: 28 September 2018; Accepted: 01 April 2019;
Published: 24 April 2019.
Edited by:
Irina Caminschi, Monash University, AustraliaReviewed by:
Pablo Alejandro Silveira, Anzac Research Institute, AustraliaKirsteen Tullett, Monash University, Australia
Jessica Li, Peter MacCallum Cancer Centre, Australia
Copyright © 2019 Apostólico, Lunardelli, Yamamoto, Cunha-Neto, Boscardin and Rosa. This is an open-access article distributed under the terms of the Creative Commons Attribution License (CC BY). The use, distribution or reproduction in other forums is permitted, provided the original author(s) and the copyright owner(s) are credited and that the original publication in this journal is cited, in accordance with accepted academic practice. No use, distribution or reproduction is permitted which does not comply with these terms.
*Correspondence: Daniela Santoro Rosa, ZHNhbnRvcm9yb3NhQGdtYWlsLmNvbQ==