- 1Immunology Operative Unit, IRCCS Ospedale Policlinico San Martino, Genoa, Italy
- 2Department of Virology, Parasitology and Immunology, Faculty of Veterinary Medicine, Ghent University, Merelbeke, Belgium
- 3Laboratory of Molecular Nephrology, IRCCS Istituto Giannina Gaslini, Genoa, Italy
- 4Department of Experimental Medicine, University of Genoa, Genoa, Italy
- 5Center of Excellence for Biomedical Research, University of Genoa, Genoa, Italy
- 6Department of Immunology, IRCCS Ospedale Pediatrico Bambino Gesù, Rome, Italy
- 7Laboratory of Clinical and Experimental Immunology, IRCCS Istituto Giannina Gaslini, Genoa, Italy
Natural Killer (NK) cells are potent cytotoxic cells belonging to the family of Innate Lymphoid Cells (ILCs). Their most characterized effector functions are directed to the control of aberrant cells in the body, including both transformed and virus-infected cells. NK cell-mediated recognition of abnormal cells primarily occurs through receptor-ligand interactions, involving an array of inhibitory and activating NK receptors and different types of ligands expressed on target cells. While most of the receptors have become known over many years, their respective ligands were only defined later and their impressive complexity has only recently become evident. NKp44, a member of Natural Cytotoxicity Receptors (NCRs), is an activating receptor playing a crucial role in most functions exerted by activated NK cells and also by other NKp44+ immune cells. The large and heterogeneous panel of NKp44 ligands (NKp44L) now includes surface expressed glycoproteins and proteoglycans, nuclear proteins that can be exposed outside the cell, and molecules that can be either released in the extracellular space or carried in extracellular vesicles. Recent findings have extended our knowledge on the nature of NKp44L to soluble plasma glycoproteins, such as secreted growth factors or extracellular matrix (ECM)-derived glycoproteins. NKp44L are induced upon tumor transformation or viral infection but may also be expressed in normal cells and tissues. In addition, NKp44-NKp44L interactions are involved in the crosstalk between NK cells and different innate and adaptive immune cell types. NKp44 expression in different ILCs located in tissues further extends the potential role of NKp44-NKp44L interactions.
Introduction
NK cells are cytotoxic Innate Lymphoid Cells (ILCs) that patrol the body and play a crucial role in the defense against viruses and tumors by circulating in peripheral blood (PB) and extravasating into tissues, in particular at the sites of injury (1–8). In addition to recirculating NK cells, specific NK cell subsets reside in different tissues and organs fulfilling unique regulatory functions. Depending on the organ and the local microenvironment, tissue-resident NK (trNK) cells release proangiogenic factors, regulatory cytokines or specific chemokines, and interact with different cell types. Recirculating NK cells, besides killing target cells, can promote inflammation by cytokine and chemokine release and interaction with dendritic cells (DC), monocytes/macrophages, granulocytes, and T cells (9–14).
NKp44, together with the other Natural Cytotoxicity Receptors (NCRs), NKp46 and NKp30 (14–16) can play an important role in most functions exerted by NK cells and also by other immune cell types (17). Indeed, NKp44 expression is wider than initially thought, and includes activated PB-NK cells (15), interferon-producing intraepithelial ILC1 in tonsils (18, 19), a subset of ILC3 in the decidua (20), and in mucosal-associated lymphoid tissue (MALT) (21), a small subset of decidual trNK cells (22), and plasmacytoid dendritic cells (pDC) (23, 24). Typically, NKp44 is implicated in the killing of virus-infected or tumor cells; however, the increasing panel of NKp44+ cells and the identification of new NKp44-ligands (NKp44L), possibly expressed in different tissues or released in the circulation, supports an important role for this receptor in tissue homeostasis and immune regulation (25–29).
NKp44 is a transmembrane glycoprotein characterized by a single extracellular V-type Ig-like domain and a cytoplasmic tail containing an Immunoreceptor Tyrosine-based Inhibitory Motif (ITIM) and no known activating signaling motifs (16). The crystal structure of the NKp44 Ig-V domain reveals the presence of a prominent positively charged groove, likely involved in recognition of anionic patterns shared by different ligands (30). The transmembrane domain contains a charged amino acid (Lys) that allows the association with the Immunoreceptor Tyrosine-based Activating Motif (ITAM)-containing KARAP/DAP12 adaptor protein, a strong transducer of activating signals (16, 31–33). Even if the NKp44 ITIM sequence was originally shown to be non-functional (34), in certain contexts NKp44 can also transduce inhibitory signals. Indeed, NKp44 expressed on pDC inhibits IFN-α release induced upon TLR stimulation (23). More recently, three NKp44 mRNA splice variants (NKp44-1,−2,−3) have been demonstrated to display different signaling capabilities based on the presence (NKp44-1) or absence (NKp44-2 and−3) of the ITIM in their cytoplasmic tail. Local physiologic or pathologic cytokine milieus could influence NKp44 splicing, determining the relative expression levels of these variants and, accordingly, affecting the functional features of NK cells in different locations (see below) (35–37).
The local microenvironment can also determine the function and role of NKp44 by orchestrating development, recruitment, and modulation of specific regulatory, tissue-remodeling, or cytotoxic NKp44+ cell subsets (either NK cells or ILCs). Thus, for example, lungs contain a large majority of CD56dim NK cells recirculating from blood; spleen and liver also include CD56bright trNK cells; lymphoid tissues associated to epithelia include NKp44+ ILCs; uterus comprises NKp44+ NK cells and NKp44+ ILC3 with peculiar regulatory and tissue-remodeling functions (2, 4, 5, 18, 19, 38, 39).
Pathologic conditions, and in particular tumors, can result in altered patterns of NKp44+ cells. Tumor tissues can produce specific cytokines and chemokines that can drive recruitment of cytokine-producing or cytotoxic NK cell subsets (i.e., CD56bright or CD56dim cells) (40, 41) and induce NKp44 expression. In addition, NKp44+ ILC3 have also been described in lung tumors and have been found to be associated with a better clinical outcome (39). On the other hand, in the tumor microenvironment, hypoxic conditions (42), or soluble mediators such as PGE2 (43–45) and TGF-β (46) can induce NK cells to down-regulate expression and/or function of major activating NK receptors including NKp44. Similar effects can also be induced by tumor cells and tumor-associated fibroblasts (45, 47).
Thus, depending on the type of available NKp44L, NKp44-expressing cells, tissues, or environmental conditions, the role of NKp44 greatly varies and extends to novel functional contexts, beyond the classical NK-mediated target cell killing.
NKp44-NKp44L Interactions Involved in Recognition of Tumor and Virus-Infected Cells
NKp44-Mediated Recognition of Tumor Cells
Although NKp44L have been elusive for a long time since the discovery of NKp44, experimental evidence suggested that NKp44-NKp44L interactions occurring in the context of tumor cell recognition could play an important role in potentiating NK-mediated cytotoxicity against tumor cells (15, 16, 48, 49). Figure 1A summarizes information on the presently known NKp44-L and their role in the NK-tumor cell interaction.
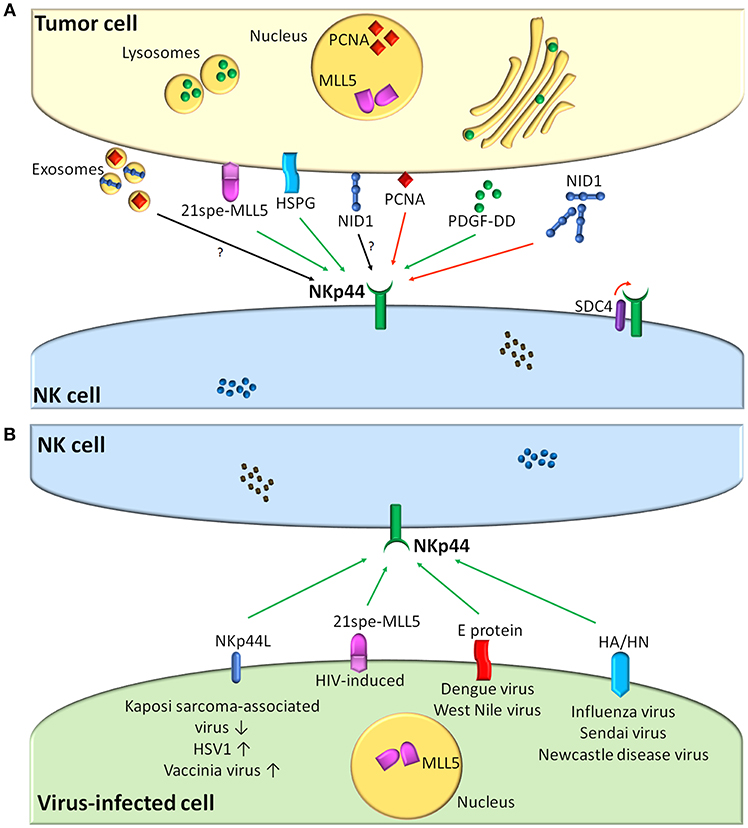
Figure 1. NKp44-NKp44L interactions and their role in the recognition of tumor (A) or virus-infected cells (B) by human NK cells. (A) Shows cellular and cell-released NKp44 ligands expressed by tumor cells. (B) Shows viral and viral-regulated NKp44 ligands. Viruses up-regulating/down-regulating NKp44L expression on infected cells are indicated with up and down arrows. Green arrows indicate interactions resulting in NK cell activation, while red arrows indicate inhibition. Black arrows refer to still undefined interactions. MLL5, mixed-lineage leukemia protein-5; PCNA, Proliferating Cell Nuclear Antigen; HSPG, heparan sulfate proteoglycans; NID1, Nidogen-1; PDGF, Platelet-Derived Growth Factor; SDC4, syndecan-4.
The first evidence of an activating cellular NKp44L was provided in 2005, when Vielliard et al. reported that an NKp44L is induced on CD4+ T lymphocytes from HIV-1-infected individuals (see below) (50). Later on, such NKp44L was identified as a peculiar isoform of mixed-lineage leukemia protein-5 (MLL5), termed 21spe-MLL5, encoded by a splice variant lacking the last five exons of MLL5 and containing a unique C-terminal exon, required for both localization at the cell surface and interaction with NKp44 (51). MLL5 (or lysine methyltransferase 2E), is primarily a nuclear protein with a wide expression pattern in healthy tissues and plays a role in regulating cell cycle progression and hematopoietic differentiation; on the other hand, 21spe-MLL5 is mainly found in the cytoplasm and at the cell surface, is barely expressed in healthy tissues but is frequently detected at high levels in hematopoietic and non-hematopoietic tumor cells. The mechanism allowing the expression of this ligand on the surface of cancer cells is still to be defined, but 21spe-MLL5 surface expression on tumor cells triggers NKp44-mediated cytotoxicity (51).
Another cellular NKp44L is represented by Proliferating Cell Nuclear Antigen (PCNA) (52), a ubiquitously expressed nuclear protein involved in the regulation of DNA replication, DNA repair, and cell cycle progression. Interestingly, it may be overexpressed in cancer cells where it contributes to tumor survival and enhanced malignancy (53). PCNA can be recruited to the plasma membrane of tumor cells, probably through the formation of a PCNA/HLA-I complex (54) or shuttling via exosomes (52). NKp44 engagement by PCNA results in the inhibition of NK-mediated cytotoxicity and IFN-γ secretion by NK cells; this inhibitory effect is mediated by the ITIM in NKp44 cytoplasmic tail and is observed only following an interaction with the ITIM-containing isoform NKp44-1, whose expression has been associated with poor survival in AML patients (36). In view of these findings, PCNA has been proposed as an immune evasion mechanism, enhancing tumor cell survival by promoting a paradoxical NKp44-mediated inhibition of tumor cell killing by NK cells. Recently, the NKp44 binding site for PCNA was identified and an NKp44-derived peptide has been shown to target intracellular PCNA, resulting in the apoptosis of cancer cell lines in vitro and tumor growth arrest in vivo (55).
Cell surface-associated heparan sulfate (HS) proteoglycans (HSPGs) represent a peculiar category of NCR ligands (56). The three NCRs display a distinct pattern of HS/heparin recognition, based on the heterogeneity and structural complexity of these macromolecules (57). NKp44 recognizes highly sulfated HS/heparin-type structures by binding to negatively charged stretches of HS. Mutations of basic residues in the positively charged NKp44 groove resulted in a decreased binding to HS/heparin. In the context of tumor cell recognition, NKp44 may bind to HS expressed on different cancer cell lines. Moreover, HS was able to enhance NKp44-induced IFN-γ secretion, while the role of HS in the induction of NK-mediated cytotoxicity is less clear (58). Although membrane-associated HSPGs are present on all cells, their expression is heterogeneous in different tissues and can be altered in tumor cells (59). Modified levels of HS in cancer cells may result in altered recognition, in their association with other ligands, or in their structural alterations by tumor-induced modifying enzymes. In this context, HS moieties of HSPGs may be considered as self “modified” ligands for NCRs and may serve as co-ligands, cooperating with other ligands to influence NK cell functions.
NKp44 has also been shown to interact in cis with syndecan-4 (SDC4), one of the HSPGs expressed on the surface of NK cells, thereby constitutively dampening NKp44-mediated activation by preventing the receptor binding to other ligands expressed on target cells (60).
More recently, the search for glycolipid ligands by microarray screening led to the identification of Globo-A (GalNAcα1,3(Fucα1,2) Galβ1,3GalNAcβ1,3Galα1,4Galβ1,4Galβ1-Cer) as NKp44L (61). This glycolipid, which was originally isolated from human kidney, displays a globo-series structure and includes a terminal part similar to that of blood group A antigen (62). At present, its functional relevance in the regulation of NK cell function has not been demonstrated yet.
NKp44-Mediated Recognition of Virus-Infected Cells
Concerning the role of NKp44 in the context of virus recognition, roughly three types of viral interactions have been described: viral NKp44L, virus-induced up-regulation of cellular NKp44L, and virus-mediated inhibition of NKp44 recognition (Figure 1B).
In 2001, Mandelboim et al. reported that the hemagglutinin (HA) of the orthomyxovirus H1N1 influenza virus and the hemagglutinin-neuraminidase (HN) of the paramyxovirus Sendai virus, both expressed on the surface of infected cells, are recognized by NKp46, and thereby trigger the lysis of infected cells by NK cells (63). Shortly thereafter, these viral proteins were also found to serve as NKp44L, but not NKp30L, and the interaction with NKp44 could contribute to the killing activity of certain NK cell clones (64). NKp44 not only recognizes the influenza virus HA of H1 strains but also of H5 strains (65). In addition, HN of other paramyxoviruses, avian Newcastle disease virus and human parainfluenza virus 3 (HPIV3), also appear to serve as NKp44L and trigger NK cell activity (66, 67).
The recognition of both HA and HN depends on sialylation of NKp44, similar to that reported for NKp46 (63–65). Remarkably, the E envelope glycoproteins of two flaviviruses, West Nile and Dengue viruses, also bind to NKp44 thereby increasing NK cell activity, but in a sialylation-independent manner (68).
As mentioned above, in 2005, Vieillard et al. reported that a fraction of CD4+ T cells from HIV-infected patients, but not from healthy subjects, showed an increased expression of NKp44L (50). The percentage of NKp44L+ CD4+ T cells was inversely correlated with the CD4+ T cell count and correlated with the viral load, suggesting that NKp44L expression may be implicated in T cell depletion and disease course. In addition, the HIV gp41 envelope protein (and its precursor gp160) was found to trigger NKp44L expression and NK-mediated lysis. In particular, a highly conserved peptide in gp41 (NH2-SWSNKS-COOH or 3S) triggers NKp44L expression (50). Another study suggested that a role for virus-induced NKp44L expression in CD4+ T cell depletion during HIV infection may be virus strain-dependent (69).
Members of two large DNA virus families, the poxviruses and the herpesviruses, also up-regulate the expression of NKp44L early after infection of host cells. The immediate early ICP0 protein of herpes simplex virus 1 and an unidentified early protein of the poxvirus vaccinia virus up-regulate expression of NKp30L, NKp44L, and NKp46L, thereby triggering NCR-mediated lysis of infected human fibroblast cells (70, 71).
Viral interference with the NKp44-mediated killing of infected cells typically consists in the down-regulation of cellular or viral NKp44L from the cell surface. Remarkably, the same HIV gp41 S3 peptide that triggers NKp44L expression in CD4+ T cells, suppresses NKp44L expression in astrocytes. This led the authors to hypothesize that HIV may use this strategy to protect astrocytes from NK cell-mediated killing during HIV infection of the CNS (72). How the same viral peptide exerts such opposing effects on NKp44L expression and NK cell-mediated cytotoxicity in two different cell types is currently unclear.
The viral ORF54-encoded protein of Kaposi's sarcoma-associated herpesvirus, but not that of other human herpesviruses, suppresses the expression of NKp44L during lytic infection. Although this viral protein is a dUTPase, its enzymatic activity is neither necessary nor sufficient to down-regulate NKp44L (73).
Finally, although the influenza virus HA binds NKp44 and NKp46, thereby contributing to NK-mediated lysis of infected cells, the virus can suppress this effect via expression of its NA protein (74, 75). NA displays sialidase activity which may remove the sialic acids from NKp44 and NKp46, thereby interfering with their binding to HA (74, 75). Although the HA-NA-NKp44 interplay may appear paradoxical at first sight, perhaps this should be looked upon from an evolutionary point of view. The highly conserved function of influenza HA is to interact with sialic acids on the cell surface as an essential initial step to enter host cells. It has been hypothesized that NK cells utilize this general HA sialic acid-binding property to recognize the infected cell via NKp46 and NKp44 (75). The NA protein may then serve as a viral countermeasure against these and other unwanted side-effects of the essential sialic acid-binding activity of HA.
Regulatory and Homeostatic Functions of NKp44-NKp44L Interactions
NKp44 is also expressed on helper ILCs, which typically reside at the epithelial/mucosal surfaces, becoming involved in tissue remodeling, inflammation, and maintenance of barrier integrity (76). Notably, NKp44 expression allows to discriminate two ILC3 subsets characterized by unique cytokine patterns: NKp44+ ILC3 are able to produce IL-22 (21) and are dependent on the Aryl Hydrocarbon Receptor, whereas human LTi and NKp44neg ILC3 produce IL-17A (77). Remarkably, in NKp44+ ILC3, IL-22 expression is preferentially induced by cytokine stimulation, whereas NKp44 triggering selectively activates a coordinated pro-inflammatory program via TNF-α (78). In this context, NKp44 has been suggested to play a potential role in the pathogenesis of immune-mediated diseases, including psoriasis. Indeed, more than 50% of circulating NKp44+ ILC3 in the blood of psoriasis patients express cutaneous lymphocyte-associated antigen, suggesting their role in skin homing. Moreover, NKp44+ ILC3 frequency in non-lesional psoriatic skin is significantly increased when compared to that in normal skin (79).
NKp44+ ILC3 are also present in secondary lymphoid organs (SLO). Differently from ILC3 in inflamed tonsils, ILC3 in non-inflamed lymph nodes and spleen, irrespective of NKp44 expression, lack the transcription of cytokines typically mediating ILC3 function. However, both NKp44neg and NKp44+ resting ILC3 can produce IL-22 in response to inflammatory stimuli. According to these data, SLO-residing ILC3 may represent a pool of resting cells that can be rapidly activated by inflammatory signals present in the local microenvironment (80).
NKp44+ ILC3 can also infiltrate tumor tissues. In particular, in non-small cell lung cancer, NKp44 can interact with tumor cells and synergize with IL-1β and IL-23 for IL-22 production (39). In addition, NKp44-mediated recognition of tumor associated fibroblasts can lead to the release of high amounts of TNF-α, thus influencing the vascular permeability and inducing pro-inflammatory responses in the tumor microenvironment (39).
In the uterus, the NKp44+ pool is composed of ILC3, LTi-like cells, IFN-γ-producing ILC1-like cells, and NK cells (20, 22). These cell populations are variably involved in tissue remodeling related to decidua development and vascularization during pregnancy, the induction of maternal-fetal tolerance, and the control of viral infections (i.e., CMV) (81). For some of these functions the role of NKp44 has been suggested by its ability to induce IP10, IL-8, and VEGF release (82). It has also been recently shown that the decidual cytokine milieu can favor the expression of the ITIM-bearing NKp44-1 inhibitory isoform (37), which indeed can induce cytokine release but inhibits cytotoxicity. In this context, the overexpression of the NKp44-L PCNA in decidual throphoblasts in the first trimester of gestation suggests that inhibitory signaling of NKp44 might contribute to the maintenance of immune tolerance of maternal NK cells during pregnancy (83).
As mentioned in the Introduction, the functional role of NKp44 also depends on the nature and body distribution of its ligands. Very recently, the discovery of Platelet-Derived Growth Factor (PDGF)-DD as a novel NKp44L represented a major breakthrough, since for the first time NKp44 was demonstrated to recognize a soluble molecule (28, 84). PDGF-DD belongs to the PDGF family and represents the active processed form of PDGF-D (85, 86). Noteworthy, PDGF-DD-NKp44 interaction does not trigger NK cell-mediated cytotoxicity, rather, it induces potent release of TNF-α and IFN-γ by IL-2-activated NK cells. Transcriptomic analysis clearly indicated that stimulation of activated NK cells by PDGF-DD induced genes encoding pro-inflammatory cytokines and chemokines, cell surface activation markers, and transcription factors involved in ITAM signaling, cellular activation, and proliferation. Notably, the induction of an NKp44-dependent pro-inflammatory program upon PDGF-DD-NKp44 interaction has been demonstrated not only in activated NK cells but also in other NKp44+ ILCs, namely ILC3 and intraepithelial ILC1. In particular, PDGF-DD induced IFN-γ and TNF-α production in ILC1, while it stimulated TNF-α but not IL-22 secretion in ILC3 (28).
PDGF-DD is involved in embryonic development, placenta formation, angiogenesis, and wound healing, and has been implicated in various pathological conditions, including vascular diseases, mesangioproliferative glomerulonephritis, and fibrosis (85, 87). Thus, PDGF-DD interaction with NKp44+ cells present in various tissues (including decidua) may play diverse biological roles. Nevertheless, PDGF-DD can be secreted by different tumors and promotes cellular proliferation, stromal cell recruitment, angiogenesis, epithelial-mesenchymal transition, and metastasis through autocrine and paracrine PDGFRβ signaling (88, 89). In this context, PDGF-DD, as NKp44L, can also induce NK-, ILC1- and ILC3-mediated release of cytokines with anti-tumor activity. Indeed, supernatants of PDGF-DD-activated NK cells inhibit tumor cell growth in vitro, while in vivo experiments indicated that the introduction of the NCR2 transgene limited the spread of PDGF-DD-expressing tumor cells in mice (28).
The example of PDGF-DD as an extracellular ligand is not unique among NCRs, since NKp46 can also bind to a soluble molecule, namely the plasma glycoprotein Complement Factor P (CFP or properdin) (90). Both CFP and PDGF-DD activate NK cell functions. However, soluble ligands of activating receptors generally represent mechanisms to modulate NK cell functions (91–97). In this context, another peculiar NKp44L was recently described (29). Nidogen-1 (NID1) glycoprotein is an essential component of ECM and basement membrane (BM) (98, 99) able to interact with NKp44. When released as soluble molecule, NID1 modulates NK cell function by reducing NKp44-induced IFN-γ release or cytotoxicity. Notably, it also regulates IFN-γ production induced by PDGF-DD following NKp44 engagement. Thus, the release of NID1 in extracellular fluids may act as regulatory mechanism for NKp44+ NK cells in the blood stream or in tissues. Interestingly, NID1 release has been observed in different cancer types (100–102), and may represent a suppressive mechanism exploited by tumors to avoid NK-mediated attack. Actually, the functional outcome of the NKp44-NID1 interaction may be rather complex, as NID1 can associate with other ECM components, including laminin, collagen type IV, and perlecan (98, 99), or be modified by extracellular proteases secreted in the tumor microenvironment (103, 104). In addition, NID1 can also be exposed at the cell surface of different NID1-releasing tumor cell lines (29).
As shown by the examples of PDGF-DD and NID1, NKp44L can be expressed not only on neoplastic or virus-infected cells, but also on normal cells. Thus, NKp44L expression was observed on the surface of articular chondrocytes (105), although the nature of this ligand is not well-defined. Since NK cells can kill human chondrocytes, it has been suggested that the NKp44-NKp44L interaction may play a role in cartilage destruction occurring in chronic inflammatory joint disorders. Interestingly, human NK cells were previously shown to kill porcine chondrocytes mainly through NKp44-NKp44L interaction (106).
More recently, it has been demonstrated that human astrocytes express an NKp44L whose interaction with NKp44 contributes to NK-mediated cytotoxicity against astrocytes and to IFN-γ production (72).
Concluding Remarks
The finding that NKp44 is expressed by different immune cell types and tissues (Table 1) and can recognize multiple ligands (soluble or associated to the cell surface or ECM) strongly suggests that this receptor is used to fulfill various functions, adapted to different body compartments or even to temporary micro-environmental changes.
The expanding knowledge on the multifaceted role of NK cell subsets and ILCs, residing in the different tissues, suggests that NKp44-NKp44L interactions may be involved in the activation/regulation of several biological/immunological processes. A deeper understanding of these issues will be beneficial for the design of NK-based immunotherapeutic approaches in different pathologic conditions, including but not limited to tumors and infections.
Author Contributions
MP, HF, SS, MV, and CC wrote the manuscript. GC, SG, MCM, and LM reviewed the manuscript and provided critical input.
Funding
This work was supported by the following grants: AIRC 5X1000, 2018 Project Code 21147 (LM and SS); AIRC IG 2017, Project Code 19920 (LM); V806A 5X1000 Ministero della Salute 2015 (MCM); AIRC IG 2017 Id. 20312 (SS); AIRC IG 2014 project no. 15428 (MV); Funds from Renal Child Foundation (GC); MP was a recipient of the AIRC fellowship no. 18274, year 2016.
Conflict of Interest Statement
The authors declare that the research was conducted in the absence of any commercial or financial relationships that could be construed as a potential conflict of interest.
References
1. Vivier E, Raulet DH, Moretta A, Caligiuri MA, Zitvogel L, Lanier LL, et al. Innate or adaptive immunity? The example of natural killer cells. Science. (2011) 331:44–9. doi: 10.1126/science.1198687
2. Vivier E, Artis D, Colonna M, Diefenbach A, Di Santo JP, Eberl G, et al. Innate lymphoid cells: 10 years on. Cell. (2018) 174:1054–66. doi: 10.1016/j.cell.2018.07.017
3. Vacca P, Munari E, Tumino N, Moretta F, Pietra G, Vitale M, et al. Human natural killer cells and other innate lymphoid cells in cancer: Friends or foes? Immunol Lett. (2018) 201:14–9. doi: 10.1016/j.imlet.2018.11.004
4. Freud AG, Mundy-Bosse BL, Yu J, Caligiuri MA. The broad spectrum of human natural killer cell diversity. Immunity. (2017) 47:820–33. doi: 10.1016/j.immuni.2017.10.008
5. Bjorkstrom NK, Ljunggren HG, Michaelsson J. Emerging insights into natural killer cells in human peripheral tissues. Nature reviews. Immunology. (2016) 16:310–20. doi: 10.1038/nri.2016.34
6. Castriconi R, Carrega P, Dondero A, Bellora F, Casu B, Regis S, et al. Molecular mechanisms directing migration and retention of natural killer cells in human tissues. Front Immunol. (2018) 9:2324. doi: 10.3389/fimmu.2018.02324
7. Locatelli F, Pende D, Falco M, Della Chiesa M, Moretta A, Moretta L. NK cells mediate a crucial graft-versus-leukemia effect in haploidentical-HSCT to cure high-risk acute leukemia. Trends Immunol. (2018) 39:577–90. doi: 10.1016/j.it.2018.04.009
8. Long EO, Kim HS, Liu D, Peterson ME, Rajagopalan S. Controlling natural killer cell responses: integration of signals for activation and inhibition. Annu Rev Immunol. (2013) 31:227–58. doi: 10.1146/annurev-immunol-020711-075005
9. Moretta A. Natural killer cells and dendritic cells: rendezvous in abused tissues. Nature reviews. Immunology. (2002) 2:957–64. doi: 10.1038/nri956
10. Thoren FB, Riise RE, Ousback J, Della Chiesa M, Alsterholm M, Marcenaro E, et al. Human NK cells induce neutrophil apoptosis via an NKp46- and Fas-dependent mechanism. J Immunol. (2012) 188:1668–74. doi: 10.4049/jimmunol.1102002
11. Bellora F, Castriconi R, Dondero A, Reggiardo G, Moretta L, Mantovani A, et al. The interaction of human natural killer cells with either unpolarized or polarized macrophages results in different functional outcomes. Proc Natl Acad Sci USA. (2010) 107:21659–64. doi: 10.1073/pnas.1007654108
12. Martin-Fontecha A, Thomsen LL, Brett S, Gerard C, Lipp M, Lanzavecchia A, et al. Induced recruitment of NK cells to lymph nodes provides IFN-gamma for T(H)1 priming. Nat Immunol. (2004) 5:1260–5. doi: 10.1038/ni1138
13. Ardolino M, Zingoni A, Cerboni C, Cecere F, Soriani A, Iannitto ML, et al. DNAM-1 ligand expression on Ag-stimulated T lymphocytes is mediated by ROS-dependent activation of DNA-damage response: relevance for NK-T cell interaction. Blood. (2011) 117:4778–86. doi: 10.1182/blood-2010-08-300954
14. Moretta A, Bottino C, Vitale M, Pende D, Cantoni C, Mingari MC, et al. Activating receptors and coreceptors involved in human natural killer cell-mediated cytolysis. Annu Rev Immunol. (2001) 19:197–223. doi: 10.1146/annurev.immunol.19.1.197
15. Vitale M, Bottino C, Sivori S, Sanseverino L, Castriconi R, Marcenaro E, et al. NKp44, a novel triggering surface molecule specifically expressed by activated natural killer cells, is involved in non-major histocompatibility complex-restricted tumor cell lysis. J Exp Med. (1998) 187:2065–72. doi: 10.1084/jem.187.12.2065
16. Cantoni C, Bottino C, Vitale M, Pessino A, Augugliaro R, Malaspina A, et al. NKp44, a triggering receptor involved in tumor cell lysis by activated human natural killer cells, is a novel member of the immunoglobulin superfamily. J Exp Med. (1999) 189:787–96. doi: 10.1084/jem.189.5.787
17. Hudspeth K, Silva-Santos B, Mavilio D. Natural cytotoxicity receptors: broader expression patterns and functions in innate and adaptive immune cells. Front Immunol. (2013) 4:69. doi: 10.3389/fimmu.2013.00069
18. Fuchs A, Vermi W, Lee JS, Lonardi S, Gilfillan S, Newberry RD, et al. Intraepithelial type 1 innate lymphoid cells are a unique subset of IL-12- and IL-15-responsive IFN-gamma-producing cells. Immunity. (2013) 38:769–81. doi: 10.1016/j.immuni.2013.02.010
19. Simoni Y, Fehlings M, Kloverpris HN, McGovern N, Koo SL, Loh CY, et al. Human innate lymphoid cell subsets possess tissue-type based heterogeneity in phenotype and frequency. Immunity. (2017) 46:148–61. doi: 10.1016/j.immuni.2016.11.005
20. Vacca P, Montaldo E, Croxatto D, Loiacono F, Canegallo F, Venturini PL, et al. Identification of diverse innate lymphoid cells in human decidua. Mucosal Immunol. (2015) 8:254–64. doi: 10.1038/mi.2014.63
21. Cella M, Fuchs A, Vermi W, Facchetti F, Otero K, Lennerz JK, et al. A human natural killer cell subset provides an innate source of IL-22 for mucosal immunity. Nature. (2009) 457:722–5. doi: 10.1038/nature07537
22. Vacca P, Montaldo E, Croxatto D, Moretta F, Bertaina A, Vitale C, et al. NK cells and other innate lymphoid cells in hematopoietic stem cell transplantation. Front Immunol. (2016) 7:188. doi: 10.3389/fimmu.2016.00188
23. Fuchs A, Cella M, Kondo T, Colonna M. Paradoxic inhibition of human natural interferon-producing cells by the activating receptor NKp44. Blood. (2005) 106:2076–82. doi: 10.1182/blood-2004-12-4802
24. Bonaccorsi I, Cantoni C, Carrega P, Oliveri D, Lui G, Conte R, et al. The immune inhibitory receptor LAIR-1 is highly expressed by plasmacytoid dendritic cells and acts complementary with NKp44 to control IFNalpha production. PLoS ONE. (2010) 5:e15080. doi: 10.1371/journal.pone.0015080
25. Koch J, Steinle A, Watzl C, Mandelboim O. Activating natural cytotoxicity receptors of natural killer cells in cancer and infection. Trends Immunol. (2013) 34:182–91. doi: 10.1016/j.it.2013.01.003
26. Kruse P. H., Matta J, Ugolini S, Vivier E. Natural cytotoxicity receptors and their ligands. Immunol Cell Biol. (2014) 92:221–9. doi: 10.1038/icb.2013.98
27. Horton NC, Mathew PA. NKp44 and natural cytotoxicity receptors as damage-associated molecular pattern recognition receptors. Front Immunol. (2015) 6:31. doi: 10.3389/fimmu.2015.00031
28. Barrow AD, Edeling MA, Trifonov V, Luo J, Goyal P, Bohl B, et al. Natural killer cells control tumor growth by sensing a growth factor. Cell. (2018) 172:534–48 e519. doi: 10.1016/j.cell.2017.11.037
29. Gaggero S, Bruschi M, Petretto A, Parodi M, Zotto GD, Lavarello C, et al. Nidogen-1 is a novel extracellular ligand for the NKp44 activating receptor. Oncoimmunology. (2018) 7:e1470730. doi: 10.1080/2162402X.2018.1470730
30. Cantoni C, Ponassi M, Biassoni R, Conte R, Spallarossa A, Moretta A, et al. The three-dimensional structure of the human NK cell receptor NKp44, a triggering partner in natural cytotoxicity. Structure. (2003) 11:725–34. doi: 10.1016/S0969-2126(03)00095-9
31. Tomasello E, Vivier E. KARAP/DAP12/TYROBP: three names and a multiplicity of biological functions. Eur J Immunol. (2005) 35:1670–7. doi: 10.1002/eji.200425932
32. Olcese L, Cambiaggi A, Semenzato G, Bottino C, Moretta A, Vivier E. Human killer cell activatory receptors for MHC class I molecules are included in a multimeric complex expressed by natural killer cells. J Immunol. (1997) 158:5083–6.
33. Lanier LL, Corliss BC, Wu J, Leong C, Phillips JH. Immunoreceptor DAP12 bearing a tyrosine-based activation motif is involved in activating NK cells. Nature. (1998) 391:703–7. doi: 10.1038/35642
34. Campbell KS, Yusa S, Kikuchi-Maki A, Catina TL. NKp44 triggers NK cell activation through DAP12 association that is not influenced by a putative cytoplasmic inhibitory sequence. J Immunol. (2004) 172:899–906. doi: 10.4049/jimmunol.172.2.899
35. Pazina T, Shemesh A, Brusilovsky M, Porgador A, Campbell KS. Regulation of the functions of natural cytotoxicity receptors by interactions with diverse ligands and alterations in splice variant expression. Front Immunol. (2017) 8:369. doi: 10.3389/fimmu.2017.00369
36. Shemesh A, Brusilovsky M, Hadad U, Teltsh O, Edri A, Rubin E, et al. Survival in acute myeloid leukemia is associated with NKp44 splice variants. Oncotarget. (2016) 7:32933–45. doi: 10.18632/oncotarget.8782
37. Siewiera J, Gouilly J, Hocine HR, Cartron G, Levy C, Al-Daccak R, et al. Natural cytotoxicity receptor splice variants orchestrate the distinct functions of human natural killer cell subtypes. Nat Commun. (2015) 6:10183. doi: 10.1038/ncomms10183
38. Vacca P, Vitale C, Munari E, Cassatella MA, Mingari MC, Moretta L. Human innate lymphoid cells: their functional and cellular interactions in decidua. Front Immunol. (2018) 9:1897. doi: 10.3389/fimmu.2018.01897
39. Carrega P, Loiacono F, Di Carlo E, Scaramuccia A, Mora M, Conte R, et al. NCR(+)ILC3 concentrate in human lung cancer and associate with intratumoral lymphoid structures. Nat Commun. (2015) 6:8280. doi: 10.1038/ncomms9280
40. Carrega P, Bonaccorsi I, Di Carlo E, Morandi B, Paul P, Rizzello V, et al. CD56(bright)perforin(low) noncytotoxic human NK cells are abundant in both healthy and neoplastic solid tissues and recirculate to secondary lymphoid organs via afferent lymph. J Immunol. (2014) 192:3805–15. doi: 10.4049/jimmunol.1301889
41. Parodi M, Raggi F, Cangelosi D, Manzini C, Balsamo M, Blengio F, et al. Hypoxia modifies the transcriptome of human NK cells, modulates their immunoregulatory profile, and influences NK cell subset migration. Front Immunol. (2018) 9:2358. doi: 10.3389/fimmu.2018.02358
42. Balsamo M, Manzini C, Pietra G, Raggi F, Blengio F, Mingari MC, et al. Hypoxia downregulates the expression of activating receptors involved in NK-cell-mediated target cell killing without affecting ADCC. Eur J Immunol. (2013) 43:2756–64. doi: 10.1002/eji.201343448
43. Park A, Lee Y, Kim MS, Kang YJ, Park YJ, Jung H, et al. Prostaglandin E2 secreted by thyroid cancer cells contributes to immune escape through the suppression of natural killer (NK) cell cytotoxicity and NK cell differentiation. Front Immunol. (2018) 9:1859. doi: 10.3389/fimmu.2018.01859
44. Spaggiari GM, Capobianco A, Abdelrazik H, Becchetti F, Mingari MC, Moretta L. Mesenchymal stem cells inhibit natural killer-cell proliferation, cytotoxicity, and cytokine production: role of indoleamine 2,3-dioxygenase and prostaglandin E2. Blood. (2008) 111:1327–33. doi: 10.1182/blood-2007-02-074997
45. Balsamo M, Scordamaglia F, Pietra G, Manzini C, Cantoni C, Boitano M, et al. Melanoma-associated fibroblasts modulate NK cell phenotype and antitumor cytotoxicity. Proc Natl Acad Sci USA. (2009) 106:20847–52. doi: 10.1073/pnas.0906481106
46. Castriconi R, Cantoni C, Della Chiesa M, Vitale M, Marcenaro E, Conte R, et al. Transforming growth factor beta 1 inhibits expression of NKp30 and NKG2D receptors: consequences for the NK-mediated killing of dendritic cells. Proc Natl Acad Sci USA. (2003) 100:4120–5. doi: 10.1073/pnas.0730640100
47. Huergo-Zapico L, Parodi M, Cantoni C, Lavarello C, Fernandez-Martinez JL, Petretto A, et al. NK-cell editing mediates epithelial-to-mesenchymal transition via phenotypic and proteomic changes in melanoma cell lines. Cancer Res. (2018) 78:3913–25. doi: 10.1158/0008-5472.CAN-17-1891
48. Sivori S, Parolini S, Marcenaro E, Millo R, Bottino C, Moretta A. Triggering receptors involved in natural killer cell-mediated cytotoxicity against choriocarcinoma cell lines. Hum Immunol. (2000) 61:1055–8. doi: 10.1016/S0198-8859(00)00201-9
49. Byrd A, Hoffmann SC, Jarahian M, Momburg F, Watzl C. Expression analysis of the ligands for the Natural Killer cell receptors NKp30 and NKp44. PLoS ONE. (2007) 2:e1339. doi: 10.1371/journal.pone.0001339
50. Vieillard V, Strominger JL, Debre P. NK cytotoxicity against CD4+ T cells during HIV-1 infection: a gp41 peptide induces the expression of an NKp44 ligand. Proc Natl Acad Sci USA. (2005) 102:10981–6. doi: 10.1073/pnas.0504315102
51. Baychelier F, Sennepin A, Ermonval M, Dorgham K, Debre P, Vieillard V. Identification of a cellular ligand for the natural cytotoxicity receptor NKp44. Blood. (2013) 122:2935–42. doi: 10.1182/blood-2013-03-489054
52. Rosental B, Hadad U, Brusilovsky M, Campbell KS, Porgador A. A novel mechanism for cancer cells to evade immune attack by NK cells: The interaction between NKp44 and proliferating cell nuclear antigen. Oncoimmunology. (2012) 1:572–4. doi: 10.4161/onci.19366
53. Stoimenov I, Helleday T. PCNA on the crossroad of cancer. Biochem Soc Trans. (2009) 37:605–13. doi: 10.1042/BST0370605
54. Horton NC, Mathew SO, Mathew PA. Novel interaction between proliferating cell nuclear antigen and HLA I on the surface of tumor cells inhibits NK cell function through NKp44. PLoS ONE. (2013) 8:e59552. doi: 10.1371/journal.pone.0059552
55. Shemesh A, Kundu K, Peleg R, Yossef R, Kaplanov I, Ghosh S, et al. NKp44-derived peptide binds proliferating cell nuclear antigen and mediates tumor cell death. Front Immunol. (2018) 9:1114. doi: 10.3389/fimmu.2018.01114
56. Hecht ML, Rosental B, Horlacher T, Hershkovitz O, De Paz JL, Noti C, et al. Natural cytotoxicity receptors NKp30, NKp44 and NKp46 bind to different heparan sulfate/heparin sequences. J Proteome Res. (2009) 8:712–20. doi: 10.1021/pr800747c
57. Turnbull J, Powell A, Guimond S. Heparan sulfate: decoding a dynamic multifunctional cell regulator. Trends Cell Biol. (2001) 11:75–82. doi: 10.1016/S0962-8924(00)01897-3
58. Hershkovitz O, Jivov S, Bloushtain N, Zilka A, Landau G, Bar-Ilan A, et al. Characterization of the recognition of tumor cells by the natural cytotoxicity receptor, NKp44. Biochemistry. (2007) 46:7426–36. doi: 10.1021/bi7000455
59. Blackhall FH, Merry CL, Davies EJ, Jayson GC. Heparan sulfate proteoglycans and cancer. Br J Cancer. (2001) 85:1094–8. doi: 10.1054/bjoc.2001.2054
60. Brusilovsky M, Radinsky O, Cohen L, Yossef R, Shemesh A, Braiman A, et al. Regulation of natural cytotoxicity receptors by heparan sulfate proteoglycans in -cis: A lesson from NKp44. Eur J Immunol. (2015) 45:1180–91. doi: 10.1002/eji.201445177
61. Ito K, Shiraishi R, Higai K. Globo-A binds to the recombinant natural cytotoxicity receptor NKp44. Biol Pharm Bull. (2018) 41:1480–4. doi: 10.1248/bpb.b18-00312
62. Breimer ME, Jovall PA. Structural characterization of a blood group A heptaglycosylceramide with globo-series structure. The major glycolipid based blood group A antigen of human kidney. FEBS Lett. (1985) 179:165–72. doi: 10.1016/0014-5793(85)80213-1
63. Mandelboim O, Lieberman N, Lev M, Paul L, Arnon TI, Bushkin Y, et al. Recognition of haemagglutinins on virus-infected cells by NKp46 activates lysis by human NK cells. Nature. (2001) 409:1055–60. doi: 10.1038/35059110
64. Arnon TI, Lev M, Katz G, Chernobrov Y, Porgador A, Mandelboim O. Recognition of viral hemagglutinins by NKp44 but not by NKp30. Eur J Immunol. (2001) 31:2680–9. doi: 10.1002/1521-4141(200109)31:9<2680::AID-IMMU2680>3.0.CO;2-A
65. Ho JW, Hershkovitz O, Peiris M, Zilka A, Bar-Ilan A, Nal B, et al. H5-type influenza virus hemagglutinin is functionally recognized by the natural killer-activating receptor NKp44. J Virol. (2008) 82:2028–32. doi: 10.1128/JVI.02065-07
66. Jarahian M, Watzl C, Fournier P, Arnold A, Djandji D, Zahedi S, et al. Activation of natural killer cells by newcastle disease virus hemagglutinin-neuraminidase. J Virol. (2009) 83:8108–21. doi: 10.1128/JVI.00211-09
67. McQuaid S, Loughran S, Power P, Maguire P, Walls D, Cusi MG, et al. Haemagglutinin-neuraminidase from HPIV3 mediates human NK regulation of T cell proliferation via NKp44 and NKp46. J Gen Virol. (2018) 99:763–7. doi: 10.1099/jgv.0.001070
68. Hershkovitz O, Rosental B, Rosenberg LA, Navarro-Sanchez ME, Jivov S, Zilka A, et al. NKp44 receptor mediates interaction of the envelope glycoproteins from the West Nile and dengue viruses with NK cells. J Immunol. (2009) 183:2610–21. doi: 10.4049/jimmunol.0802806
69. Vieillard V, Habib RE, Brochard P, Delache B, Bovendo HF, Calvo J, et al. CCR5 or CXCR4 use influences the relationship between CD4 cell depletion, NKp44L expression and NK cytotoxicity in SHIV-infected macaques. AIDS. (2008) 22:185–92. doi: 10.1097/QAD.0b013e3282f35551
70. Chisholm SE, Reyburn HT. Recognition of vaccinia virus-infected cells by human natural killer cells depends on natural cytotoxicity receptors. J Virol. (2006) 80:2225–33. doi: 10.1128/JVI.80.5.2225-2233.2006
71. Chisholm SE, Howard K, Gomez MV, Reyburn HT. Expression of ICP0 is sufficient to trigger natural killer cell recognition of herpes simplex virus-infected cells by natural cytotoxicity receptors. J Infect Dis. (2007) 195:1160–8. doi: 10.1086/512862
72. Bowen KE, Mathew SO, Borgmann K, Ghorpade A, Mathew PA. A novel ligand on astrocytes interacts with natural cytotoxicity receptor NKp44 regulating immune response mediated by NK cells. PLoS ONE. (2018) 13:e0193008. doi: 10.1371/journal.pone.0193008
73. Madrid AS, Ganem D. Kaposi's sarcoma-associated herpesvirus ORF54/dUTPase downregulates a ligand for the NK activating receptor NKp44. J Virol. (2012) 86:8693–704. doi: 10.1128/JVI.00252-12
74. Bar-On Y, Glasner A, Meningher T, Achdout H, Gur C, Lankry D, et al. Neuraminidase-mediated, NKp46-dependent immune-evasion mechanism of influenza viruses. Cell Rep. (2013) 3:1044–50. doi: 10.1016/j.celrep.2013.03.034
75. Bar-On Y, Seidel E, Tsukerman P, Mandelboim M, Mandelboim O. Influenza virus uses its neuraminidase protein to evade the recognition of two activating NK cell receptors. J Infect Dis. (2014) 210:410–8. doi: 10.1093/infdis/jiu094
76. Montaldo E, Juelke K, Romagnani C. Group 3 innate lymphoid cells (ILC3s): Origin, differentiation, and plasticity in humans and mice. Eur J Immunol. (2015) 45:2171–82. doi: 10.1002/eji.201545598
77. Hoorweg K, Peters CP, Cornelissen F, Aparicio-Domingo P, Papazian N, Kazemier G, et al. Functional differences between human NKp44(-) and NKp44(+) RORC(+) innate lymphoid cells. Front Immunol. (2012) 3:72. doi: 10.3389/fimmu.2012.00072
78. Glatzer T, Killig M, Meisig J, Ommert I, Luetke-Eversloh M, Babic M, et al. RORgammat(+) innate lymphoid cells acquire a proinflammatory program upon engagement of the activating receptor NKp44. Immunity. (2013) 38:1223–35. doi: 10.1016/j.immuni.2013.05.013
79. Villanova F, Flutter B, Tosi I, Grys K, Sreeneebus H, Perera GK, et al. Characterization of innate lymphoid cells in human skin and blood demonstrates increase of NKp44+ ILC3 in psoriasis. J Invest Dermatol. (2014) 134:984–91. doi: 10.1038/jid.2013.477
80. Bar-Ephraim YE, Cornelissen F, Papazian N, Konijn T, Hoogenboezem RM, Sanders MA, et al. Cross-tissue transcriptomic analysis of human secondary lymphoid organ-residing ILC3s reveals a quiescent state in the absence of inflammation. Cell Rep. (2017) 21:823–33. doi: 10.1016/j.celrep.2017.09.070
81. Siewiera J, El Costa H, Tabiasco J, Berrebi A, Cartron G, Le Bouteiller P, et al. Human cytomegalovirus infection elicits new decidual natural killer cell effector functions. PLoS Pathog. (2013) 9:e1003257. doi: 10.1371/journal.ppat.1003257
82. Hanna J, Goldman-Wohl D, Hamani Y, Avraham I, Greenfield C, Natanson-Yaron S, et al. Decidual NK cells regulate key developmental processes at the human fetal-maternal interface. Nat Med. (2006) 12:1065–74. doi: 10.1038/nm1452
83. Korgun ET, Celik-Ozenci C, Acar N, Cayli S, Desoye G, Demir R. Location of cell cycle regulators cyclin B1, cyclin A, PCNA, Ki67 and cell cycle inhibitors p21, p27 and p57 in human first trimester placenta and deciduas. Histochem Cell Biol. (2006) 125:615–24. doi: 10.1007/s00418-006-0160-y
84. Trinchieri G. Natural killer cells detect a tumor-produced growth factor: a vestige of antiviral resistance? Trends Immunol. (2018) 39:357–8. doi: 10.1016/j.it.2018.02.002
85. Li X, Eriksson U. Novel PDGF family members: PDGF-C and PDGF-D. Cytokine Growth Factor Rev. (2003) 14:91–8. doi: 10.1016/S1359-6101(02)00090-4
86. Bergsten E, Uutela M, Li X, Pietras K, Ostman A, Heldin CH, et al. PDGF-D is a specific, protease-activated ligand for the PDGF beta-receptor. Nat Cell Biol. (2001) 3:512–6. doi: 10.1038/35074588
87. Reigstad LJ, Varhaug JE, Lillehaug JR. Structural and functional specificities of PDGF-C and PDGF-D, the novel members of the platelet-derived growth factors family. FEBS J. (2005) 272:5723–41. doi: 10.1111/j.1742-4658.2005.04989.x
88. Lokker NA, Sullivan CM, Hollenbach SJ, Israel MA, Giese NA. Platelet-derived growth factor (PDGF) autocrine signaling regulates survival and mitogenic pathways in glioblastoma cells: evidence that the novel PDGF-C and PDGF-D ligands may play a role in the development of brain tumors. Cancer Res. (2002) 62:3729–35.
89. Wang Z, Ahmad A, Li Y, Kong D, Azmi AS, Banerjee S, et al. Emerging roles of PDGF-D signaling pathway in tumor development and progression. Biochim Biophys Acta. (2010) 1806:122–30. doi: 10.1016/j.bbcan.2010.04.003
90. Narni-Mancinelli E, Gauthier L, Baratin M, Guia S, Fenis A, Deghmane AE, et al. Complement factor P is a ligand for the natural killer cell-activating receptor NKp46. Sci Immunol. (2017) 2:eaam9628. doi: 10.1126/sciimmunol.aam9628
91. Raulet DH, Gasser S, Gowen BG, Deng W, Jung H. Regulation of ligands for the NKG2D activating receptor. Annu Rev Immunol. (2013) 31:413–41. doi: 10.1146/annurev-immunol-032712-095951
92. Lanier LL. NKG2D receptor and its ligands in host defense. Cancer Immunol Res. (2015) 3:575–82. doi: 10.1158/2326-6066.CIR-15-0098
93. Baury B, Masson D, McDermott BM Jr Jarry A, Blottiere H. M., Blanchardie P, et al. Identification of secreted CD155 isoforms. Biochem Biophys Res Commun. (2003) 309:175–82. doi: 10.1016/S0006-291X(03)01560-2
94. Iguchi-Manaka A, Okumura G, Kojima H, Cho Y, Hirochika R, Bando H, et al. Increased soluble CD155 in the serum of cancer patients. PLoS ONE. (2016) 11:e0152982. doi: 10.1371/journal.pone.0152982
95. Schlecker E, Fiegler N, Arnold A, Altevogt P, Rose-John S, Moldenhauer G, et al. Metalloprotease-mediated tumor cell shedding of B7-H6, the ligand of the natural killer cell-activating receptor NKp30. Cancer Res. (2014) 74:3429–40. doi: 10.1158/0008-5472.CAN-13-3017
96. Pesce S, Tabellini G, Cantoni C, Patrizi O, Coltrini D, Rampinelli F, et al. B7-H6-mediated downregulation of NKp30 in NK cells contributes to ovarian carcinoma immune escape. Oncoimmunology. (2015) 4:e1001224. doi: 10.1080/2162402X.2014.1001224
97. Reiners KS, Topolar D, Henke A, Simhadri VR, Kessler J, Sauer M, et al. Soluble ligands for NK cell receptors promote evasion of chronic lymphocytic leukemia cells from NK cell anti-tumor activity. Blood. (2013) 121:3658–65. doi: 10.1182/blood-2013-01-476606
98. Kruegel J, Miosge N. Basement membrane components are key players in specialized extracellular matrices. Cell Mol Life Sci. (2010) 67:2879–95. doi: 10.1007/s00018-010-0367-x
99. Pozzi A, Yurchenco PD, Iozzo RV. The nature and biology of basement membranes. Matrix Biol. (2017) 58:1–11. doi: 10.1016/j.matbio.2016.12.009
100. Li L, Zhang Y, Li N, Feng L, Yao H, Zhang R, et al. Nidogen-1: a candidate biomarker for ovarian serous cancer. Jpn J Clin Oncol. (2015) 45:176–82. doi: 10.1093/jjco/hyu187
101. Willumsen N, Bager CL, Leeming DJ, Bay-Jensen AC, Karsdal MA. Nidogen-1 degraded by cathepsin S can be quantified in serum and is associated with non-small cell lung cancer. Neoplasia. (2017) 19:271–8. doi: 10.1016/j.neo.2017.01.008
102. Aleckovic M, Wei Y, LeRoy G, Sidoli S, Liu DD, Garcia BA, et al. Identification of Nidogen 1 as a lung metastasis protein through secretome analysis. Genes Dev. (2017) 31:1439–55. doi: 10.1101/gad.301937.117
103. Sage J, Leblanc-Noblesse E, Nizard C, Sasaki T, Schnebert S, Perrier E, et al. Cleavage of nidogen-1 by cathepsin S impairs its binding to basement membrane partners. PLoS ONE. (2012) 7:e43494. doi: 10.1371/journal.pone.0043494
104. Martino-Echarri E, Fernandez-Rodriguez R, Rodriguez-Baena FJ, Barrientos-Duran A, Torres-Collado AX, Plaza-Calonge Mdel C, et al. Contribution of ADAMTS1 as a tumor suppressor gene in human breast carcinoma. Linking its tumor inhibitory properties to its proteolytic activity on nidogen-1 and nidogen-2. Int Jo Cancer. (2013) 133:2315–24. doi: 10.1002/ijc.28271
105. Bialoszewska A, Baychelier F, Niderla-Bielinska J, Czop A, Debre P, Vieillard V, et al. Constitutive expression of ligand for natural killer cell NKp44 receptor (NKp44L) by normal human articular chondrocytes. Cell Immunol. (2013) 285:6–9. doi: 10.1016/j.cellimm.2013.08.005
106. Sommaggio R, Cohnen A, Watzl C, Costa C. Multiple receptors trigger human NK cell-mediated cytotoxicity against porcine chondrocytes. J Immunol. (2012) 188:2075–83. doi: 10.4049/jimmunol.1100433
Keywords: natural kiiler cells, innate lymphoid cells (ILC), natural cytotoxicity receptors (NCR), NKp44, ligands, tumor immunology, viral infections
Citation: Parodi M, Favoreel H, Candiano G, Gaggero S, Sivori S, Mingari MC, Moretta L, Vitale M and Cantoni C (2019) NKp44-NKp44 Ligand Interactions in the Regulation of Natural Killer Cells and Other Innate Lymphoid Cells in Humans. Front. Immunol. 10:719. doi: 10.3389/fimmu.2019.00719
Received: 07 February 2019; Accepted: 18 March 2019;
Published: 09 April 2019.
Edited by:
Marina Cella, Washington University School of Medicine in St. Louis, United StatesReviewed by:
Kerry S. Campbell, Fox Chase Cancer Center, United StatesCatharina C. Gross, University of Münster, Germany
Copyright © 2019 Parodi, Favoreel, Candiano, Gaggero, Sivori, Mingari, Moretta, Vitale and Cantoni. This is an open-access article distributed under the terms of the Creative Commons Attribution License (CC BY). The use, distribution or reproduction in other forums is permitted, provided the original author(s) and the copyright owner(s) are credited and that the original publication in this journal is cited, in accordance with accepted academic practice. No use, distribution or reproduction is permitted which does not comply with these terms.
*Correspondence: Claudia Cantoni, claudia.cantoni@unige.it