- 1Laboratório de Genômica Funcional e Bioinformática, Instituto Oswaldo Cruz, Fundação Oswaldo Cruz, Rio de Janeiro, Brazil
- 2Laboratório Interdisciplinar de Pesquisas Médicas, Instituto Oswaldo Cruz, Fundação Oswaldo Cruz—FIOCRUZ, Rio de Janeiro, Brazil
- 3Unidade de Espectrometria de Massas e Proteômica, Instituto de Bioquímica Médica Leopoldo de Meis, Universidade Federal do Rio de Janeiro, Rio de Janeiro, Brazil
- 4Laboratório de Microbiologia Celular, Instituto Oswaldo Cruz, Fundação Oswaldo Cruz, Rio de Janeiro, Brazil
Surface-associated proteins from Mycobacterium bovis BCG Moreau RDJ are important components of the live Brazilian vaccine against tuberculosis. They are important targets during initial BCG vaccine stimulation and modulation of the host's immune response, especially in the bacterial-host interaction. These proteins might also be involved in cellular communication, chemical response to the environment, pathogenesis processes through mobility, colonization, and adherence to the host cell, therefore performing multiple functions. In this study, the proteomic profile of the surface-associated proteins from M. bovis BCG Moreau was compared to the BCG Pasteur reference strain. The methodology used was 2DE gel electrophoresis combined with mass spectrometry techniques (MALDI-TOF/TOF), leading to the identification of 115 proteins. Of these, 24 proteins showed differential expression between the two BCG strains. Furthermore, 27 proteins previously described as displaying moonlighting function were identified, 8 of these proteins showed variation in abundance comparing BCG Moreau to Pasteur and 2 of them presented two different domain hits. Moonlighting proteins are multifunctional proteins in which two or more biological functions are fulfilled by a single polypeptide chain. Therefore, the identification of such proteins with moonlighting predicted functions can contribute to a better understanding of the molecular mechanisms unleashed by live BCG Moreau RDJ vaccine components.
Introduction
Tuberculosis (TB) is one of the 10 major causes of death worldwide. According to the World Health Organization (WHO), TB killed 1.7 million people in 2016 with 10.4 million new cases estimated worldwide ratifying the need for more effective treatment and prevention (1). To date, Bacillus Calmette-Guérin (BCG) is the only widely used prophylactic measure against TB (1). BCG is an attenuated Mycobacterium bovis strain obtained at the beginning of the Twentieth century at the Pasteur Institute, in Lille. It was distributed to more than 34 countries and maintained in culture without adequate standardization for a long time (2). Because of in vitro evolution, slightly different BCG substrains emerged from the parental BCG, such as those with deletions and duplications of genomic regions and/or single nucleotide polymorphism (SNPs) well documented through analysis and genome sequencing (3–9). These genetic differences among the various BCG strains in use worldwide partially explain the variable efficacy in protection against pulmonary TB in adults (2). In Brazil, the strain used for vaccine production since 1927 is M. bovis BCG Moreau. The genomic comparison between BCG Pasteur, reference strain, and BCG Moreau showed regions of difference (RD), for example the loss of RD2 and RD14 in BCG Pasteur and RD16 in BCG Moreau, leading to unique genomic/proteomic characteristics (5, 10). These particularities justify more detailed proteomic studies in order to elucidate which proteins are effectively expressed by these bacteria.
BCG is a live attenuated vaccine, and the expression of secreted and surface-associated proteins is extremely relevant since these proteins may play a role in the bacteria-host cell interaction at the beginning of infection (11, 12). These proteins can also be released from the surface since they are non-covalently linked to the mycomembrane (13). Many intracellular proteins with a known function in cell metabolism have also been found on the cell surface, such as glutamine synthetase, gamma-glutamyl phosphate reductase, and cysteine desulfurase (14). Different factors may contribute to the switch between functions such as release to the extracellular space, changes in temperature, redox state of the cell, oligomeric state of the protein, direct interactions with a variety of binding partner proteins, or even to changes in the cellular concentration of a ligand/substrate, cofactor or product, bringing to light the importance of surface-associated proteins playing different roles in cell system (15, 16). This switch between functions is a characteristic called moonlighting (15, 17).
Considering this variability on protein expression, localization and function(s) in different strains of BCG, we compared the surface-associated proteome from the Brazilian strain used in TB vaccine production, M. bovis BCG Moreau, to that of BCG Pasteur through 2DE gel electrophoresis combined with mass spectrometry. Complementary to the information already available in the literature, our approach allows a more confident evaluation of expression, abundance, localization and function(s) of proteins between these two BCG strains. The results presented here may lead to the identification of key components of the M. bovis BCG Moreau vaccine strain which can be related to the variability in immunological response observed in vaccinated individuals.
Materials and Methods
Mycobacterial Cultivation
Mycobacterium bovis BCG Pasteur strain 1173P2 was obtained from the Pasteur Institute (Paris, France) and seed-stocks maintained at −80°C. M. bovis BCG Moreau was supplied in Sauton/potato medium by the Ataulpho de Paiva Foundation (FAP), producer of the BCG vaccine in Brazil. Both strains were cultured as surface pellicles for 2 weeks at 37°C in Sauton medium (18).
Surface Fraction Preparation
After the removal of the culture filtrate, the surface associated material was obtained through vigorous manual shaking of the bacterial pellicle with 2 mm glass beads, essentially as described (19). The surface components were recovered in Milli-Q sterile water and centrifuged twice at 2,500 g for 10 min at 4°C. Aliquots of 1 mL were further centrifuged twice at 16,000 g in order to remove any remaining bacteria. The surface-associated protein fraction was obtained using an adaptation of the method described by Wessel and Flugge (20). Briefly, proteins were precipitated with 15% TCA/acetone, the resulting pellets were washed sequentially with 400 μL of 100% cold acetone, 200 μL of diethyl ether, and 200 μL of chloroform. The final pellets were resuspended in 100 μL Isoelectric focusing (IEF) buffer (8M ureum/2% CHAPS).
Bi-Dimensional Electrophoresis
The immobilized pH gradient (IPG) strips and all 2DE reagents were purchased from Bio-Rad, (Hercules, CA, USA). For the first dimension, 500 μg of proteins were diluted to a final volume of 300 μL of rehydration solution (8M urea, 2% CHAPS), 4 mM Tributyl phosphine (TBP), 0.4% ampholytes pH 3-10, trace of bromophenol blue). The samples were applied to IPG strips (17 cm, pH interval of 4–7) by in-gel rehydration and incubated for 1 h at room temperature. All isoelectric focusing was performed on a Protean® IEF cell (Bio-Rad) with a temperature of 20°C and a maximum current of 50 μA/strip. Running conditions: active rehydration (50V) for 11 h; step 1- linear gradient from 1 to 250V over 20 min; step 2 - linear gradient from 250 to 10,000V over 2 h; step 3- constant 10,000V until complete 80,000 V/h. After isoelectric focusing, proteins were reduced in 130 mM dithiothreitol (DTT) and alkylated in 270 mM iodoacetamide, both in equilibration buffer (6M urea, 2% SDS, 375 mM Tris-HCl pH 8.8, 20% glycerol). Second dimension separation was done in 17 cm, 12% SDS-PAGE gels, 1.0 mm thick, using a vertical system (Bio-Rad) in standard Tris/glycine/SDS buffer at 40 mA/gel, 10°C, until the tracking dye left the gel. Proteins were visualized with Comassie Brilliant Blue (CBB) following procedures described elsewhere (21).
Image Analysis
Gel images were documented using a GS-800TM calibrated imaging densitometer (Bio-Rad) and images were analyzed using PDQuestTM software (Bio-Rad). During the alignment of the images, to compensate for subtle differences in sample loading, gel staining, and destaining, the volume of each spot was normalized in relation to the total density of valid spots present in the gel image. Comparison of 2DE maps derived from three independent protein preparations, each one obtained from three independent BCG cultures, was performed. To determine experimental isoelectric point (pI) and molecular mass (Mr) coordinates for each single spot, 2DE gels were calibrated using a select set of reliable identification landmarks distributed throughout the entire gel. The theoretical pI and Mr of proteins identified by mass spectrometry were obtained using the BCG Moreau RDJ genome reference sequence (9; accession number: AM412059.2).
Protein Digestion, Peptide Extraction and MALDI-TOF/TOF Analysis
In-gel digestion of the 2DE SDS-PAGE separated proteins was carried out using the procedure according to Shevchenko (22). Briefly, protein spots were excised, and the gel pieces were washed three times with 50% (v/v) acetonitrile in 25 mM ammonium bicarbonate for 15 min each, dehydrated in acetonitrile, and dried in a vacuum centrifuge. Gel pieces were rehydrated in 15 μL of 50 mM ammonium bicarbonate containing 20 ng of sequencing grade modified trypsin (Promega). After 15 min, 20 μL of 50 mM ammonium bicarbonate was added to keep the gel pieces wet during tryptic digestion (37°C, 16 h). To extract peptides, 20 μL of 0.5% (v/v) trifluoroacetic acid (TFA) in 50% (v/v) acetonitrile were added and samples were sonicated for 30 min. The separated liquid was concentrated under vacuum to an approximate volume of 10 μL. The resulting peptides were extracted, partially dried, and salts were removed using ZipTipC18 columns (Millipore, Bedford, MA) following the manufacturer's instructions. The tryptic peptides were analyzed on a 4700-Proteomics Analyzer MALDI-TOF/TOF (Applied Biosystems, Foster City, CA). All mass spectra were acquired on positive ion reflector mode with 2,000 shots per spot and externally mass calibrated with a peptide mixture. The 10 most intense ion peaks from the peptide mass fingerprinting (or MS run) were further submitted to fragmentation using post source decay (PSD) mode with collision induced dissociation (CID) gas off and 1 keV collision energy.
Data Analyses and Protein Identification
Following MS/MS acquisition, the processed data files (ppw files) from the MALDI-TOF/TOF were analyzed on a Mascot Server license v. 2.2 (23, 24). The mass spectra were searched against the M. bovis BCG str. Moreau protein database (9; accession number: AM412059.2). The parameters used for the search were as follows: peptide and fragment ions mass tolerance was set at 0.5 Da; maximum of one miss cleavage site by trypsin; carbamidomethylation of cysteine residues as fixed modification, whereas oxidation of methionine/tryptophan, acetylation of the N-terminal, pyroglutamic acid, pyroglutamine, and deamidation of asparagine/glutamine were considered as variable modifications. Positive protein hit identification was accepted with at least 1 matched unique peptide. False discovery rate was estimated at <1%. The peptide ion score was considered >15 with a significance threshold of p < 0.05, whereas the protein score was above 20. Finally, a good correlation between the experimental and theoretical molecular mass and pI was also considered for positive identifications. The mass spectrometry proteomics data have been deposited to the ProteomeXchange Consortium via the PRIDE partner repository (25) with the dataset identifier PXD006141.
Statistical Analysis
Differences between spot intensity observed in 2DE gel images of M. bovis BCG strains Moreau and Pasteur were considered statistically significant when *p < 0.05; **p < 0.01; or ***p < 0.001, as determined by Graph Pad Prism 4.0 software (Graph- Pad Software Inc., San Diego, CA, USA). The unpaired Student‘s t-test was used to analyze the significant differences among both strains, using data obtained from at least 3 different sets of independent biological samples.
Bioinformatic Data
Regions of difference RD2 and RD14 both present on BCG Moreau were evaluated in terms of protein identification and predicted function according to BCG Moreau genome while the region of difference RD16 absent from BCG Moreau was evaluated according to M. tuberculosis H37Rv reference genome (accession numbers: AM412059.2 and NC_000962, respectively). For this purpose, TubercuList (http://genolist.pasteur.fr/TubercuList/) was used as the reference site to find the information about predicted function and amino acid sequence from all the proteins in M. tuberculosis H37Rv encompassing RD2, RD14, and RD16 allowing the construction of Table 1.
Putative signal peptide for protein export were predicted using SignalP 4.1 (26–28), LipoP 1.0 (29, 30), TatP 1.0 (31, 32) and SecretomeP 2.0 (33, 34) in order to predict protein localization. Potential transmembrane domains were predicted with TMHMM 2.0 (35, 36). Beta-barrel membrane proteins structural subclass from integral membrane proteins were discriminated using a Hidden Markov Model method with PRED-TMBB (37–39).
The protein sequences described with moonlighting function were retrieved from the MoonProt (40, 41) or Multitasking Protein databases (42) as well as references on Table 3 and Table S3. These sequences were used to construct the sequence database used in comparison to the sequences of the proteins identified in this work. The similarity search was performed using BLASTP (BLOSUM62 matrix), assuming the results with bitscore > 50 and E-value < e-10. Domain analyses were accomplished with proteins that obtained hit in the MoonProt and Multitasking databases using CDD (43, 44) and PFAM (45, 46).
Results
Proteins Encoded by the Genes From Regions of Difference RD2, RD14, and RD16
According to the literature, the regions of difference RD2 and RD14 are present in BCG Moreau, whereas RD16 is absent, when compared to BCG Pasteur (5). Table 1 lists the proteins encoded by these RDs. Our analysis on the surface associated proteome map of BCG Moreau (Figure 2 and Table 2) detected Mpb64, encoded in RD2, and revealed the Rv3406 protein, whose regulation is affected by the truncation of gene rv3405c, due to deletion of RD16 (10).
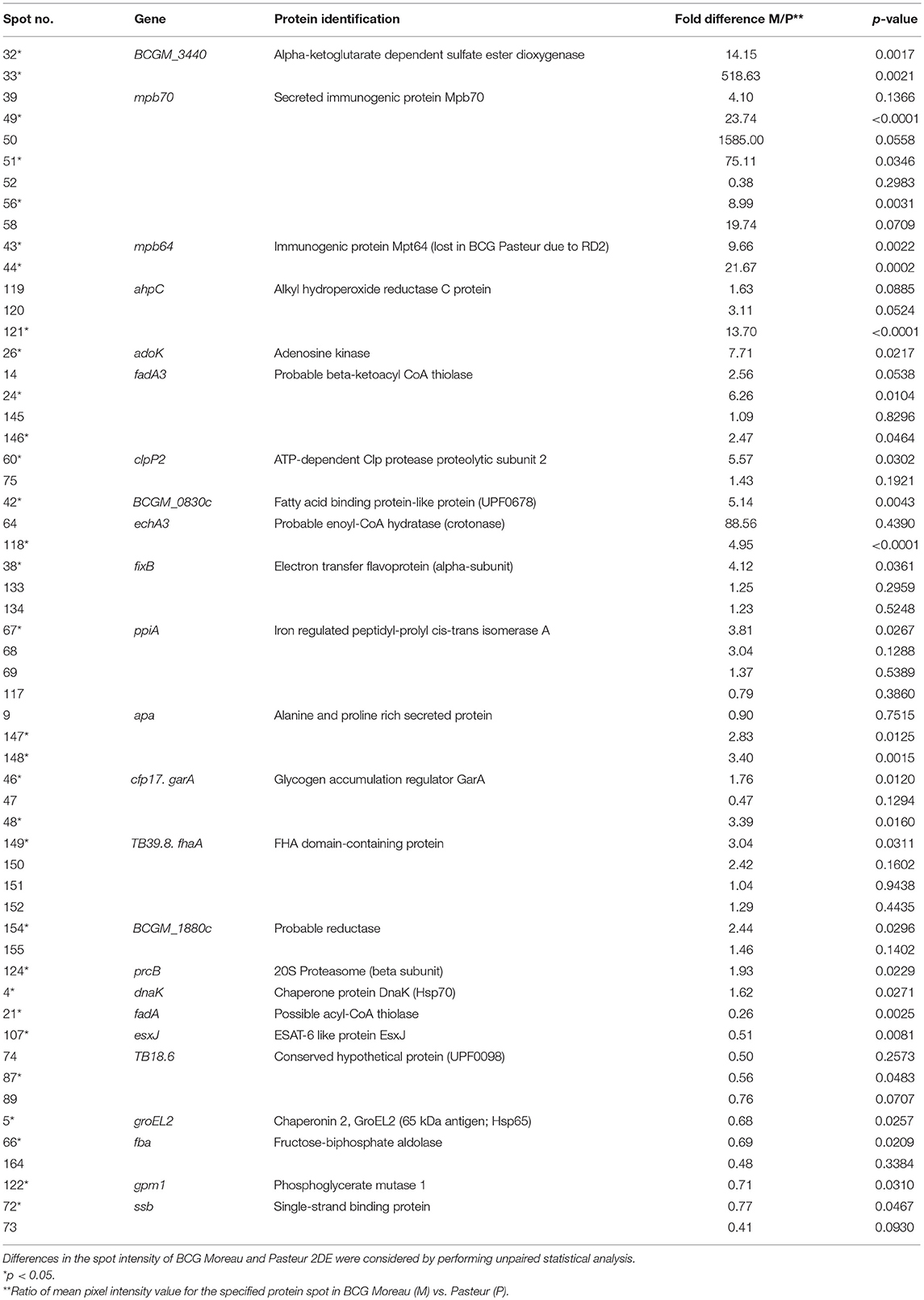
Table 2. Differential surface-associated proteins between M. bovis BCG Moreau and M. bovis BCG Pasteur.
Identification of M. bovis BCG Moreau Surface-Associated Proteins From 2DE Gels Using MALDI-TOF-TOF
The first goal of this study was to perform a proteomic analysis of surface-associated proteins of M. bovis BCG Moreau through 2DE gel electrophoresis and MALDI-TOF-TOF and compare it to M. bovis BCG Pasteur. The confirmation of the proteomic profile and the differences in protein expression between the two strains analyzed were done by comparing the surface proteomic maps in the pH 4–7 range through five biological replicates. Figure 1A shows a representative map of the surface-associated proteins of BCG Moreau. According to the statistical analysis and overlapping of the processed gel image (or virtual image), all replicates presented a reproducible profile related to the total number of spots as well as the localization (migration) and intensity (data not shown). A total of 173 protein spots are reported and they ranged in Mr between 19 and 97 kDa, mostly concentrated above the 31 kDa range. Analysis of the 2DE profiles showed that, in some cases, different spots were identified as representing the same peptide sequence (Table S1), possibly due to the occurrence of proteolysis, different protein isoforms, post-translational modifications (PTMs) or the formation of complexes or heterodimers between proteins. These events may cause differences between theoretical and experimental Mr and pI. The occurrence of different proteins identified in the same spot was also observed and can be explained by the limit of resolution in the 2DE technique (47). Among the 173 spots detected, 115 different proteins were identified by mass spectrometry (Table S1), which were classified according to the M. bovis BCG Moreau gene annotation and orthologs in M. tuberculosis H37Rv and M. bovis BCG Pasteur (accession numbers: AM412059.2, NC_000962 and AM408590.1, respectively). Functional classification of all identified proteins per spot, according to the TubercuList databank (http://genolist.pasteur.fr/TubercuList/) revealed that the major group composed by 70 proteins are related to intermediary metabolism/respiration whereas 37 proteins, the second major group, are conserved hypothetical proteins, without associated function (Figure 1B).
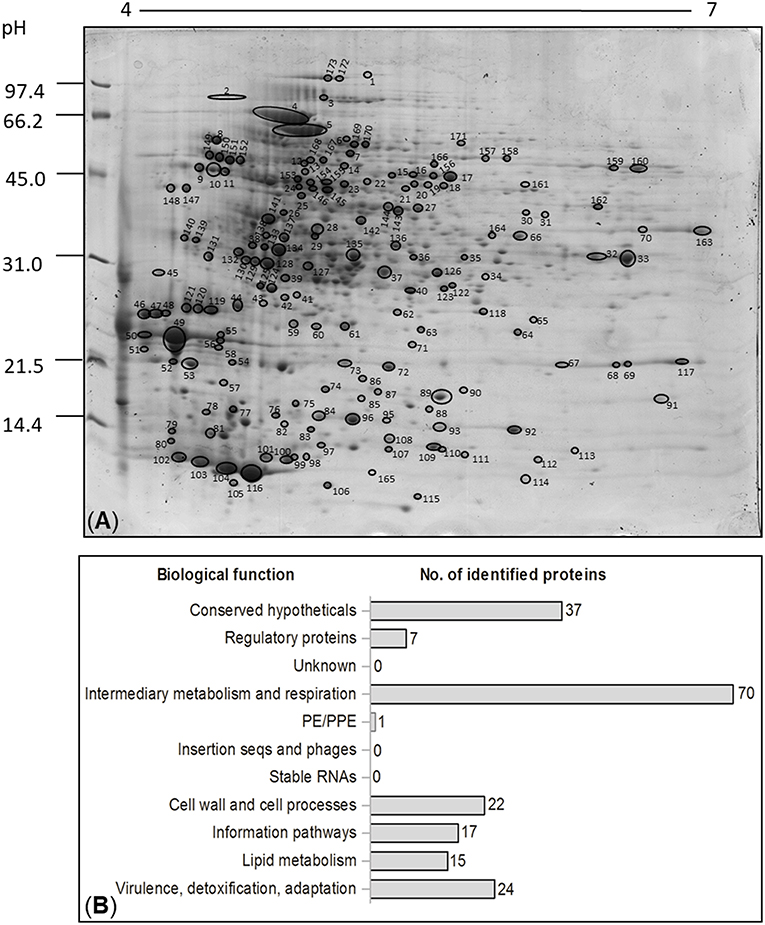
Figure 1. (A) Representative 2DE gel showing the proteomic profile of surface-associated proteins from the Brazilian vaccine strain, BCG Moreau. Molecular weight marker is indicated to the left in kDa. All identified proteins described in Table S1 are indicated and numbered. (B) Functional classification of all the identified proteins of the surface fraction from M. bovis BCG Moreau, cultured in Sauton medium. Categories of identified proteins grouped according to the biological function described on the left. The number of identified proteins is shown on the right. The classifications were generated using Tuberculist (http://genolist.pasteur.fr/TubercuList/).
Bioinformatics Analyses of the Identified Surface-Associated Proteins
In order to predict the type of secretion process, transmembrane portion unit on the surface-associated proteins and their comparison with the membrane and secreted proteins already described in the literature, a combination of bioinformatic online programs and mycobacterium genome evaluation was used (Table S2). 2DE comparative quantification with non-paired t-test statistical analysis allowed the identification of 31 spots differentially expressed between BCG Moreau and Pasteur that represented 24 distinct proteins (Figure 2 and Table 2)−17 proteins were more abundant in BCG Moreau and 7 proteins in BCG Pasteur (Table 2). All 115 different proteins identified by mass spectrometry were searched against the Moonprot and Multitasking databases and we could predict moonlighting function for 27 of them (Table 3). Moreover, among the 27 moonlighting proteins, 5 of them were more abundant in BCG Moreau whereas 3 protein spots were up-regulated in BCG Pasteur (Table S3). Domain analysis on the moonlighting predicted proteins using CDD and pFam databases allowed the identification of two domain hits for fructose-biphosphate aldolase (Fba) and aldehyde dehydrogenase (AldC), thus, suggesting distinct functions (data not shown). However, the spot corresponding to the Fba protein was found 2-fold more expressed in BCG Pasteur than in BCG Moreau.
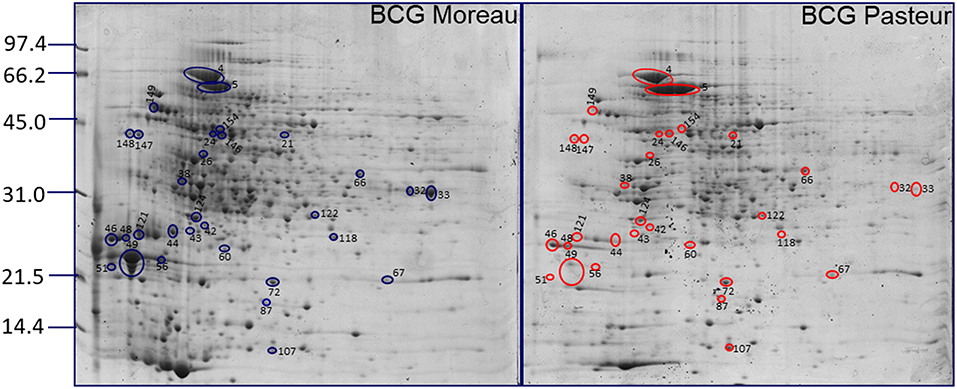
Figure 2. Differential surface-associated proteins between BCG Moreau and BCG Pasteur. Representative 2DE proteomic profiles between BCG Moreau (left) and BCG Pasteur (right) with differential spots (intensity) are marked and identified in blue (Moreau) and red (Pasteur). Molecular weight marker indicated to the left in kDa.
Discussion
In this study, the surface-associated proteins from M. bovis BCG Moreau were investigated by 2DE combined with MALDI-TOF-TOF and bioinformatic analysis. Two-dimensional gel electrophoresis maps in the pH range of 4–7 led to the identification of 173 spots (Figure 1A) that could be assigned by mass spectrometry to a total of 115 different proteins (Table S1). This choice of pH range was based on previous analysis carried out in the broader pH range of 3–10, which showed that the majority of protein spots occurred in the pH range of 4–7 (data not shown). These results complement our previous report on the secretome of BCG strains Moreau and Pasteur (12) (Table 1).
The genome of BCG Moreau differs from BCG Pasteur, among others, by the presence of two regions (RD2 and RD14; 5). Several proteins encoded in these two regions have been described as potentially immunogenic (130–133), reinforcing the importance of performing studies to address the protein expression in these two strains. In this context, differentially expressed proteins present on the bacterial surface can partially account for some differences observed in individual response to vaccination. Among the proteins identified by mass spectrometry, the Mpb64 protein (encoded in RD2) was observed to be expressed in M. bovis BCG Moreau. The quantification performed by the PDQuest software indicated a very low expression of the Mpb64 protein in BCG Pasteur proteome map, probably due to the background observed in the area where the protein should be located (Figure 2, spots 43 and 44).
Mpb64 is an immunodominant antigen capable of inducing protective immunity by T cell response; however the role of this protein in the pathogenesis of tuberculosis is not known (134, 135). We found Mpb64 expression on the cell surface proteome of BCG Moreau (spots 43 and 44, Figure 1A) with the expected predicted signal peptide and transmembrane domains (Table S2), suggesting that vaccination with BCG Moreau could trigger a better cellular immune response when compared to BCG Pasteur. With respect to RD16, this characteristic deletion found in BCG Moreau (between genes rv3400, and rv3405c) leads to the truncation and functional loss of a TetR transcriptional regulator encoded by rv3405c, resulting in the constitutive expression of the adjacent rv3406 gene (10); the resulting protein (Rv3406) was identified in the surface proteome of BCG Moreau, and is absent in Pasteur (Figure 2).
Considering the surface-associated proteomic profiles from M. bovis BCG Moreau and Pasteur, differences between the experimental and theoretical protein molecular mass and pI were observed for 49% of the identified spots, which may be due to post-translational modifications (PTMs), protein degradation, the presence of isoforms and conformers of the proteins (136, 137). For example, Apa (spots 9, 147, and 148) is already described as glycosylated on diverse threonine residues, which could result in the observation of multiple protein species by 2DE gel (138). In fact, the experimental molecular mass and pI of spots 9, 147, and 148 are in disagreement to the theoretical ones (Table S1). We observed that the ESAT-6 like protein EsxJ and glutamine synthetase (GS) found in spots 107 and 169/170, respectively, were identified as N-formylation-containing proteins (Table S1). Such PTM could contribute to electrophoretic mobility shift in 2DE gels, and therefore, would account for the differences between experimental and theoretical pI. It has been reported that N-formylated peptides may serve as good candidates for a universal vaccine against M. tuberculosis when administered in combination with drugs (139–141).
Overall, these PTMs could be important factors for eliciting the immune response. It has been reported that the glycosylated motif found in Apa protein was related to the high capacity of BCG to stimulate an immune response in BCG-immunized guinea pigs (142, 143). Our results indicate that the Apa protein shows higher expression on the cell surface of BCG Moreau compared to Pasteur (Figure 2 and Table 2). Other important proteins identified in the BCG Moreau surface-associated proteome were secreted antigens 85A and B (spots 37, 126, and 127 of Figure 1A). These proteins belong to the antigen Ag-85 complex and are found in association with the mycobacteria cell surface, constituting the major secreted proteins observed on mycobacteria culture filtrate (12, 144, 145). These proteins are strongly immunogenic and can trigger both humoral and cellular immune responses in vivo (144, 146–148). Ag85 proteins have mycolyltransferase activity, crucial to maintain the cell wall integrity of mycobacteria (149–151). In addition, they can bind to human fibronectin (152) and elastin (153) present in the extracellular matrix having, therefore, moonlighting functions. Recent studies demonstrated that Ag-85 complex was identified as a target for mannose-binding lectin and ficolins (154). These proteins have been extensively studied as potential candidates for new vaccines against tuberculosis (155, 156).
Protein fate in terms of cellular localization is an important aspect that might be explored on surface-associated proteins (Table S2). Non-covalently surface-associated proteins can be secreted through different systems such as via signal peptide (as predicted by Signal P and Lipo P software), non-classical secretory pathway like proteins without an N-terminal signal peptide (according to Secretome P analysis), and twin-arginine translocation pathway (as found by Tat prediction program) in which a twin-arginine consensus motif is located within the signal peptide itself (29, 31, 157–159). The non-classical secretory system was predicted for aconitase (spots 172 and 173) and glutamine synthetase (spots 169 and 170). In M. tuberculosis, aconitase is found in the cytosol, cell wall, and cell membrane fractions and it has also been described as a bifunctional protein acting as an enzyme in the presence of iron and RNA-binding in the absence of iron (115, 160). Glutamine synthetase (GS) presented moonlighting features of acyltransferase in M. tuberculosis (161). Previous studies have shown that this enzyme is secreted into the culture medium and plays a crucial role in pathogenicity as well as in bacterial growth (162, 163). In Lactobacillus crispatus, GS is a novel adhesive moonlighting enzyme that associates to the cell surface at an acidic pH (119). Further experiments must be carried out in order to show that aconitase, glutamine synthetase and other surface-associated proteins (Table 3) have moonlighting behavior also in M. bovis BCG Moreau.
Bioinformatic tools have been used to predict protein moonlighting function by primary sequence analysis and hence domain investigations of predicted moonlighting proteins can be pursued to propose novel functions that corroborate with in vitro and in vivo studies (40, 42, 164, 165). Nevertheless, most moonlighting proteins described to date have been identified by chance (166). In general, highly conserved proteins, often metabolic proteins/enzymes (167, 168) or molecular chaperones (169), receptors (170), ribosomal proteins, and transmembrane channels (171), were shown to be moonlighting proteins (172). These findings suggest that the presence of intracellular proteins at “unexpected” locations is not always due to experimental artifacts such as cellular lysis. The methodology used here for obtaining the fraction of surface-associated proteins is well characterized (19), strengthening the fact that the intracellular proteins identified in this study may be performing moonlighting functions. In addition, the appearance of a new function for the same protein can be considered a great advantage for the microorganism since it optimizes the functional repertoire encoded by a compact genome. In this context, bioinformatic investigation using the Moonprot/Multitask programs led to the prediction of moonlighting functions for some of the identified proteins, already described for other organisms/species (Table 3). Besides, Conserved Domains Database (CDD) and pFam were also used to infer the number of domains these proteins could present (data not shown). Most of the identified proteins grouped in the “intermediary metabolism” functional category (Figure 1B), raising the question of why proteins normally encountered intracellularly had been found surface-associated to the mycomembrane. This could be explained by different functions these proteins may have depending on localization. Finally, other features such as the presence of distinct protein surfaces or domains influencing the oligomeric state of the protein, concentration of cellular ligands, substrates and cofactors must also be considered to presume moonlighting function for these kind of proteins (16, 173–175).
Interestingly, we found that fructose-biphosphate aldolase (Fba) and the aldehyde dehydrogenase (AldC) contained two domains hit: one representative of a canonical function in mycobacteria and another representing a probable moonlighting function already described in other organisms (Table 3). In mycobacteria, the canonical domain hit for fructose-biphosphate aldolase is FTBP_aldolase_II representative of an enzyme that controls the condensation of dihydroxyacetone phosphate with glyceraldehyde-3-phosphate to yield fructose-1,6-bisphosphate (176). Nevertheless, the moonlighting domain hit is ICL_KPHMT that represents an enzyme superfamily that catalyzes the formation and cleavage of either P-C (proline-cysteine) or C-C (cysteine-cysteine) bonds (data not shown). In other organisms, as described in Table 3, the moonlighting function of fructose- biphosphate aldolase is related to protein binding and cell adherence. (177) confirmed experimentally by in vitro assays that Fba from M. tuberculosis binds to human plasminogen. This generates the proteolytic enzyme plasmin leading to the breakdown of extracellular matrix and basal membrane proteins, contributing to tissue injury in tuberculosis. More recently, de la Paz Santangelo et al. (178) reported that Fba of M. tuberculosis binds to human plasminogen in a dose dependent manner and is important for M. tuberculosis growth. According to our results Fba is less expressed on the cell surface of BCG Moreau than in BCG Pasteur (Table S3). On the other hand, the canonical domain hit for aldehyde dehydrogenase is ALDH_F1AB_F2_RALDH1 that corresponds to NAD+-dependent retinal dehydrogenase 1 also known as aldehyde dehydrogenase family 1 member A1 (ALDH1A1) in humans. It is a cytosolic enzyme that catalyzes the oxidation of retinaldehyde to retinoic acid (RA). RA is the active metabolite of vitamin A and it is required for spermatogenesis and many other biological processes (179). The moonlighting domain hit is PutA, a trifunctional protein in bacteria: transcriptional regulator, proline dehydrogenase and pyrroline-5- carboxylate dehydrogenase (data not shown). Christgen et al. (180) discovered a membrane binding region on the PutA domain from Escherichia coli AldC that explains the PutA functional switch from self-regulating transcriptional repressor to membrane binding domain. Our results indicate that AldC is a surface-associated protein from M. bovis BCG Moreau (spot 171 of Figure 1A) not found in the culture filtrate of BCG Moreau (Table S2). Fba and AldC may play a role in the immunopathology of tuberculosis, but this still needs further investigation.
Altogether, the differences in abundance of surface-associated proteins identified between BCG strains Moreau and Pasteur could have an impact on vaccine efficacy. The finding that some of these proteins have moonlighting functions opens new possibilities for investigating the role of extracellular proteins on the bacterial-host interface.
Author Contributions
TP, MB-P, and LM-L conceived, designed, performed experiments and analyzed results. AG performed bioinformatic analysis. MW and PC performed statistical analysis of results. DK organized, analyzed, processed, and deposited the mass spectrometry data on PRIDE repository. WD conceived and designed experiments. TP, DK, PC, MB-P, and LM-L wrote the paper.
Funding
This work received financial support from the WHO/TDR Special Programme for Research and Training in Tropical Diseases and the following Brazilian agencies: CAPES, CNPq, and PDTIS/FIOCRUZ.
Conflict of Interest Statement
The authors declare that the research was conducted in the absence of any commercial or financial relationships that could be construed as a potential conflict of interest.
Acknowledgments
We thank Dr. Jonas Perales and Dr. André Teixeira from Oswaldo Cruz Foundation-FIOCRUZ/PDTIS 2DE and Mass Spectrometry platform facilities for the MALDI-TOF-TOF data acquisition and in particular to Dr. André Teixeira for kindly recruit the raw mass spectrometry data. We are also thankful for the Proteomics and Mass Spectrometry Unit platform (UEMP) at the Rio de Janeiro Federal University (UFRJ) run by Dr. Russolina Benedeta Zingali for support in the use of the license Mascot Server for the protein identification searches. We would like to thank the post-doctoral fellow Dr. Marjolly Caruso Brígido and the technician Augusto Vieira Magalhães both members of UEMP for helping us with the PRIDE mass spectrometry repository. We are also in debt to Carolina Zavareze (FAP) that kindly provided the Sauton culture medium and the BCG Moreau vaccine strain.
Supplementary Material
The Supplementary Material for this article can be found online at: https://www.frontiersin.org/articles/10.3389/fimmu.2019.00716/full#supplementary-material
Abbreviations
TB, Tuberculosis; WHO, World Health Organization; BCG, Bacillus Calmette-Guerin; SNPs, single nucleotide polymorphisms; RD, Region of Difference; 2DE gel, Two-dimensional gel electrophoresis; PTMs, Post-translational modifications; FH, Fumarate hydratase; TCA, trichloroacetic acid; GS, glutamine synthetase; TDM, trehalose dimycolate; CDD, Conserved Domains Database; Fba, fructose-biphosphate aldolase; AldC, aldehyde dehydrogenase.
References
1. WHO. Tuberculosis. (2018). Available online at: http://www.who.int/mediacentre/factsheets/fs104/en/ (accessed February 26, 2019).
2. Benevolo-de-Andrade TC, Monteiro-Maia R, Cosgrove C, Castello-Branco LR. BCG Moreau Rio de Janeiro: an oral vaccine against tuberculosis–review. Mem Inst Oswaldo Cruz. (2005) 100:459–65. doi: 10.1590/S0074-02762005000500002
3. Mahairas GG, Sabo PJ, Hickey MJ, Singh DC, Stover CK. Molecular analysis of genetic differences between Mycobacterium bovis BCG and virulent M. bovis J Bacteriol. (1996) 178:1274–82. doi: 10.1128/jb.178.5.1274-1282.1996
4. Behr MA, Small PM. A historical and molecular phylogeny of BCG strains. Vaccine. (1999) 17:915–22. doi: 10.1016/S0264-410X(98)00277-1
5. Behr MA, Wilson MA, Gill WP, Salamon H, Schoolnik GK, Rane S, et al. Comparative genomics of BCG vaccines by whole-genome DNA microarray. Science. (1999) 284:1520–3. doi: 10.1126/science.284.5419.1520
6. Brosch R, Gordon SV, Garnier T, Eiglmeier K, Frigui W, Valenti P, et al. Genome plasticity of BCG and impact on vaccine efficacy. Proc Natl Acad Sci USA. (2007) 104:5596–601. doi: 10.1073/pnas.0700869104
7. Leung AS, Tran V, Wu Z, Yu X, Alexander DC, Gao GF, et al. Novel genome polymorphisms in BCG vaccine strains and impact on efficacy. BMC Genomics. (2008) 9:413. doi: 10.1186/1471-2164-9-413
8. Garcia Pelayo MC, Uplekar S, Keniry A, Mendoza Lopez P, Garnier T, Nunez Garcia J, et al. A comprehensive survey of single nucleotide polymorphisms (SNPs) across Mycobacterium bovis strains and M. bovis BCG vaccine strains refines the genealogy and defines a minimal set of SNPs that separate virulent M bovis strains and M bovis BCG strains. Infect Immun. (2009) 77:2230–8. doi: 10.1128/IAI.01099-08
9. Gomes LH, Otto TD, Vasconcellos EA, Ferrao PM, Maia RM, Moreira AS, et al. Genome sequence of Mycobacterium bovis BCG Moreau, the Brazilian vaccine strain against tuberculosis. J Bacteriol. (2011) 193:5600–1. doi: 10.1128/JB.05827-11
10. Galvao TC, Lima CR, Gomes LH, Pagani TD, Ferreira MA, Goncalves AS, et al. The BCG Moreau RD16 deletion inactivates a repressor reshaping transcription of an adjacent gene. Tuberculosis (Edinb). (2014) 94:26–33. doi: 10.1016/j.tube.2013.11.004
11. El-Etr SH, Cirillo JD. Entry mechanisms of mycobacteria. Front Biosci. (2001) 6:D737–747. doi: 10.2741/sahar
12. Berredo-Pinho M, Kalume DE, Correa PR, Gomes LH, Pereira MP, da Silva RF, et al. Proteomic profile of culture filtrate from the Brazilian vaccine strain Mycobacterium bovis BCG Moreau compared to M. bovis BCG Pasteur BMC Microbiol. (2011) 11:80. doi: 10.1186/1471-2180-11-80
13. Daffe M, Etienne G. The capsule of Mycobacterium tuberculosis and its implications for pathogenicity. Tuber Lung Dis. (1999) 79:153–69. doi: 10.1054/tuld.1998.0200
14. Wang W, Jeffery CJ. An analysis of surface proteomics results reveals novel candidates for intracellular/surface moonlighting proteins in bacteria. Mol Biosyst. (2016) 12:1420–31. doi: 10.1039/C5MB00550G
15. Jeffery CJ. Moonlighting proteins–an update. Mol Biosyst. (2009) 5:345–50. doi: 10.1039/b900658n
16. Huberts DH, van der Klei IJ. Moonlighting proteins: an intriguing mode of multitasking. Biochim Biophys Acta. (2010) 1803:520–5. doi: 10.1016/j.bbamcr.2010.01.022
17. Jeffery CJ. Moonlighting proteins. Trends Biochem Sci. (1999) 24:8–11. doi: 10.1016/S0968-0004(98)01335-8
18. Eickhoff TC. The current status of BCG immunization against tuberculosis. Annu Rev Med. (1977) 28:411–23. doi: 10.1146/annurev.me.28.020177.002211
19. Ortalo-Magne A, Dupont MA, Lemassu A, Andersen AB, Gounon P, Daffe M. Molecular composition of the outermost capsular material of the tubercle bacillus. Microbiology. (1995) 141:1609–20. doi: 10.1099/13500872-141-7-1609
20. Wessel D, Flugge UI. A method for the quantitative recovery of protein in dilute solution in the presence of detergents and lipids. Anal Biochem. (1984) 138:141–3. doi: 10.1016/0003-2697(84)90782-6
21. Neuhoff V, Arold N, Taube D, Ehrhardt W. Improved staining of proteins in polyacrylamide gels including isoelectric focusing gels with clear background at nanogram sensitivity using Coomassie Brilliant Blue G-250 and R-250. Electrophoresis. (1988) 9:255–62. doi: 10.1002/elps.1150090603
22. Shevchenko A, Shevchenko A. Evaluation of the efficiency of in-gel digestion of proteins by peptide isotopic labeling and MALDI mass spectrometry. Anal Biochem. (2001) 296:279–83. doi: 10.1006/abio.2001.5321
23. Perkins DN, Pappin DJ, Creasy DM, Cottrell JS. Probability-based protein identification by searching sequence databases using mass spectrometry data. Electrophoresis. (1999) 20:3551–67. doi: 10.1002/(SICI)1522-2683(19991201)20:18 < 3551::AID-ELPS3551>3.0.CO;2-2
24. MatrixScience. Matrix Science. London, UK. (2016). Available online at: http://www.matrixscience.com (accessed June 1, 2018).
25. Vizcaino JA, Csordas A, del-Toro N, Dianes JA, Griss J, Lavidas I, et al. 2016 update of the PRIDE database and its related tools. Nucleic Acids Res. (2016) 44:D447–56. doi: 10.1093/nar/gkw880
26. Nielsen H, Engelbrecht J, Brunak S, von Heijne G. Identification of prokaryotic and eukaryotic signal peptides and prediction of their cleavage sites. Protein Eng. (1997) 10:1–6. doi: 10.1093/protein/10.1.1
27. Petersen TN, Brunak S, von Heijne G, Nielsen H. SignalP 4.0: discriminating signal peptides from transmembrane regions. Nat Methods. (2011) 8:785–6. doi: 10.1038/nmeth.1701
28. SignalP4.1. SignalP 4.1 Server. DK. (2016). Available online at: http://www.cbs.dtu.dk/services/SignalP/ (accessed March 14, 2018).
29. Juncker AS, Willenbrock H, Von Heijne G, Brunak S, Nielsen H, Krogh A. Prediction of lipoprotein signal peptides in Gram-negative bacteria. Protein Sci. (2003) 12:1652–62. doi: 10.1110/ps.0303703
30. LipoP1.0. LipoP 1.0 Server. DK. (2015). Available online at: http://www.cbs.dtu.dk/services/LipoP/ (accessed November 25, 2018).
31. Bendtsen JD, Nielsen H, Widdick D, Palmer T, Brunak S. Prediction of twin-arginine signal peptides. BMC Bioinformatics. (2005b) 6:167. doi: 10.1186/1471-2105-6-167
32. TatP1.0. TatP 1.0 Server. DK. (2016). Available online at: http://www.cbs.dtu.dk/services/TatP/ (accessed September 16, 2018).
33. Bendtsen JD, Kiemer L, Fausboll A, Brunak S. Non-classical protein secretion in bacteria. BMC Microbiol. (2005a) 5:58. doi: 10.1186/1471-2180-5-58
34. SecretomeP 2,.0. SecretomeP 2.0 Server. DK. (2015). Available online at: http://www.cbs.dtu.dk/services/SecretomeP/ (accessed December 14, 2018).
35. Emanuelsson O, Brunak S, von Heijne G, Nielsen H. Locating proteins in the cell using TargetP, SignalP and related tools. Nat Protoc. (2007) 2:953–71. doi: 10.1038/nprot.2007.131
36. TMHMM 2,.0. TMHMM 2.0 Server. DK. (2015). Available online at: http://www.cbs.dtu.dk/services/TMHMM/ (accessed August 4, 2018).
37. Bagos PG, Liakopoulos TD, Spyropoulos IC, Hamodrakas SJ. PRED-TMBB: a web server for predicting the topology of beta-barrel outer membrane proteins. Nucleic Acids Res. (2004b) 32:W400–04. doi: 10.1093/nar/gkh417
38. Bagos PG, Liakopoulos TD, Spyropoulos IC, Hamodrakas SJ. A hidden markov model method, capable of predicting and discriminating beta-barrel outer membrane proteins. BMC Bioinformatics. (2004a) 5:29. doi: 10.1186/1471-2105-5-29
39. PRED-TMBB. PRED-TMBB Server. (2014). Available online at: http://bioinformatics.biol.uoa.gr/PRED-TMBB/input.jsp (accessed November 5, 2018).
40. Mani M, Chen C, Amblee V, Liu H, Mathur T, Zwicke G, et al. MoonProt: a database for proteins that are known to moonlight. Nucleic Acids Res. (2015) 43:D277–82. doi: 10.1093/nar/gku954
41. MoonProt. MoonProt. (2014). Available online at: http://www.moonlightingproteins.org/ (accessed December 3, 2018).
42. Hernandez S, Ferragut G, Amela I, Perez-Pons J, Pinol J, Mozo-Villarias A, et al. MultitaskProtDB: a database of multitasking proteins. Nucleic Acids Res. (2014) 42:D517–20. doi: 10.1093/nar/gkt1153
43. Marchler-Bauer A, Bo Y, Han L, He J, Lanczycki CJ, Lu S, et al. CDD/SPARCLE: functional classification of proteins via subfamily domain architectures. Nucleic Acids Res. (2017) 45:D200–3. doi: 10.1093/nar/gkw1129
44. ConservedDomains. Conserved Domains within a Protein or Coding Nucleotide Sequence. (2015). Available online at: https://www.ncbi.nlm.nih.gov/Structure/cdd/wrpsb.cgi (accessed December 6, 2018).
45. Pfam. Pfam. (2015). Available online at: http://pfam.xfam.org (accessed December 4, 2018).
46. Finn RD, Coggill P, Eberhardt RY, Eddy SR, Mistry J, Mitchell AL, et al. The Pfam protein families database: towards a more sustainable future. Nucleic Acids Res. (2016) 44:D279–85. doi: 10.1093/nar/gkv1344
47. Bunai K, Yamane K. Effectiveness and limitation of two-dimensional gel electrophoresis in bacterial membrane protein proteomics and perspectives. J Chromatogr B Analyt Technol Biomed Life Sci. (2005) 815:227–36. doi: 10.1016/j.jchromb.2004.08.030
48. Jin ZG, Lungu AO, Xie L, Wang M, Wong C, Berk BC. Cyclophilin A is a proinflammatory cytokine that activates endothelial cells. Arterioscler Thromb Vasc Biol. (2004) 24:1186–91. doi: 10.1161/01.ATV.0000130664.51010.28
49. Basak C, Pathak SK, Bhattacharyya A, Pathak S, Basu J, Kundu M. The secreted peptidyl prolylcis, trans-isomerase HP0175 of Helicobacter pylori induces apoptosis of gastric epithelial cells in a TLR4- and apoptosis signal- regulating kinase 1-dependent manner. J Immunol. (2005) 174:5672–80. doi: 10.4049/jimmunol.174.9.5672
50. Pandey S, Tripathi D, Khubaib M, Kumar A, Sheikh JA, Sumanlatha G, et al. Mycobacterium tuberculosis peptidyl-prolyl isomerases are immunogenic, alter cytokine profile and aid in intracellular survival. Front Cell Infect Microbiol. (2017) 7:38. doi: 10.3389/fcimb.2017.00038
51. Krojer T, Garrido-Franco M, Huber R, Ehrmann M, Clausen T. Crystal structure of DegP (HtrA) reveals a new protease-chaperone machine. Nature. (2002) 416:455–9. doi: 10.1038/416455a
52. Sawa J, Malet H, Krojer T, Canellas F, Ehrmann M, Clausen T. Molecular adaptation of the DegQ protease to exert protein quality control in the bacterial cell envelope. J Biol Chem. (2011) 286:30680–90. doi: 10.1074/jbc.M111.243832
53. Malet H, Canellas F, Sawa J, Yan J, Thalassinos K, Ehrmann M, et al. Newly folded substrates inside the molecular cage of the HtrA chaperone DegQ. Nat Struct Mol Biol. (2012) 19:152–7. doi: 10.1038/nsmb.2210
54. Markova NG, Pinkas-Sarafova A, Simon M. A metabolic enzyme of the short-chain dehydrogenase/reductase superfamily may moonlight in the nucleus as a repressor of promoter activity. J Invest Dermatol. (2006) 126:2019–31. doi: 10.1038/sj.jid.5700347
55. Wistow GJ, Lietman T, Williams LA, Stapel SO, de Jong WW, Horwitz J, et al. Tau-crystallin/alpha-enolase: one gene encodes both an enzyme and a lens structural protein. J Cell Biol. (1988) 107(6 Pt 2):2729–36. doi: 10.1083/jcb.107.6.2729
56. Wood JM. Genetics of L-proline utilization in Escherichia coli. J Bacteriol. (1981) 146:895–901.
57. Menzel R, Roth J. Enzymatic properties of the purified putA protein from Salmonella typhimurium. J Biol Chem. (1981) 256:9762–6.
58. Ostrovsky de Spicer P, O'Brien K, Maloy S. Regulation of proline utilization in Salmonella typhimurium: a membrane-associated dehydrogenase binds DNA in vitro. J Bacteriol. (1991) 173:211–9. doi: 10.1128/jb.173.1.211-219.1991
59. Ostrovsky de Spicer P, Maloy S. PutA protein, a membrane-associated flavin dehydrogenase, acts as a redox- dependent transcriptional regulator. Proc Natl Acad Sci USA. (1993) 90:4295–8. doi: 10.1073/pnas.90.9.4295
60. Candela M, Bergmann S, Vici M, Vitali B, Turroni S, Eikmanns BJ, et al. Binding of human plasminogen to Bifidobacterium. J Bacteriol. (2007) 189:5929–36. doi: 10.1128/JB.00159-07
61. Knaust A, Weber MV, Hammerschmidt S, Bergmann S, Frosch M, Kurzai O. Cytosolic proteins contribute to surface plasminogen recruitment of Neisseria meningitidis. J Bacteriol. (2007) 189:3246–55. doi: 10.1128/JB.01966-06
62. Crowe JD, Sievwright IK, Auld GC, Moore NR, Gow NA, Booth NA. Candida albicans binds human plasminogen: identification of eight plasminogen-binding proteins. Mol Microbiol. (2003) 47:1637–51. doi: 10.1046/j.1365-2958.2003.03390.x
63. Lu M, Ammar D, Ives H, Albrecht F, Gluck SL. Physical interaction between aldolase and vacuolar H+- ATPase is essential for the assembly and activity of the proton pump. J Biol Chem. (2007) 282:24495–503. doi: 10.1074/jbc.M702598200
64. Lu M, Holliday LS, Zhang L, Dunn WAJr, Gluck SL. Interaction between aldolase and vacuolar H+-ATPase: evidence for direct coupling of glycolysis to the ATP-hydrolyzing proton pump. J Biol Chem. (2001) 276:30407–13. doi: 10.1074/jbc.M008768200
65. Lu M, Sautin YY, Holliday LS, Gluck SL. The glycolytic enzyme aldolase mediates assembly, expression, and activity of vacuolar H+-ATPase. J Biol Chem. (2004) 279:8732–9. doi: 10.1074/jbc.M303871200
66. Blau K, Portnoi M, Shagan M, Kaganovich A, Rom S, Kafka D, et al. Flamingo cadherin: a putative host receptor for Streptococcus pneumoniae. J Infect Dis. (2007) 195:1828–37. doi: 10.1086/518038
67. Tunio SA, Oldfield NJ, Berry A, Ala'Aldeen DA, Wooldridge KG, Turner DP. The moonlighting protein fructose-1, 6-bisphosphate aldolase of Neisseria meningitidis: surface localization and role in host cell adhesion. Mol Microbiol. (2010) 76:605–15. doi: 10.1111/j.1365-2958.2010.07098.x
68. Yoshida N, Oeda K, Watanabe E, Mikami T, Fukita Y, Nishimura K, et al. Protein function. chaperonin turned insect toxin. Nature. (2001) 411:44. doi: 10.1038/35075148
69. Garduno RA, Garduno E, Hoffman PS. Surface-associated hsp60 chaperonin of Legionella pneumophila mediates invasion in a HeLa cell model. Infect Immun. (1998) 66:4602–10.
70. Basu D, Khare G, Singh S, Tyagi A, Khosla S, Mande SC. A novel nucleoid-associated protein of Mycobacterium tuberculosis is a sequence homolog of GroEL. Nucleic Acids Res. (2009) 37:4944–54. doi: 10.1093/nar/gkp502
71. Rha YH, Taube C, Haczku A, Joetham A, Takeda K, Duez C, et al. Effect of microbial heat shock proteins on airway inflammation and hyperresponsiveness. J Immunol. (2002) 169:5300–7. doi: 10.4049/jimmunol.169.9.5300
72. Kol A, Sukhova GK, Lichtman AH, Libby P. Chlamydial heat shock protein 60 localizes in human atheroma and regulates macrophage tumor necrosis factor-alpha and matrix metalloproteinase expression. Circulation. (1998) 98:300–7. doi: 10.1161/01.CIR.98.4.300
73. Yamaguchi H, Osaki T, Taguchi H, Hanawa T, Yamamoto T, Fukuda M, et al. Growth inhibition of Helicobacter pylori by monoclonal antibody to heat-shock protein 60. Microbiol Immunol. (1997) 41:909–16. doi: 10.1111/j.1348-0421.1997.tb01949.x
74. Ojha A, Anand M, Bhatt A, Kremer L, Jacobs WRJr, Hatfull GF. GroEL1: a dedicated chaperone involved in mycolic acid biosynthesis during biofilm formation in mycobacteria. Cell. (2005) 123:861–73. doi: 10.1016/j.cell.2005.09.012
75. Reddi K, Meghji S, Nair SP, Arnett TR, Miller AD, Preuss M, et al. The Escherichia coli chaperonin 60 (groEL) is a potent stimulator of osteoclast formation. J Bone Miner Res. (1998) 13:1260–6. doi: 10.1359/jbmr.1998.13.8.1260
76. Babady NE, Pang YP, Elpeleg O, Isaya G. Cryptic proteolytic activity of dihydrolipoamide dehydrogenase. Proc Natl Acad Sci USA. (2007) 104:6158–63. doi: 10.1073/pnas.0610618104
77. Kinnby B, Booth NA, Svensater G. Plasminogen binding by oral streptococci from dental plaque and inflammatory lesions. Microbiology. (2008) 154(Pt 3):924–31. doi: 10.1099/mic.0.2007/013235-0
78. Chuong SD, Mullen RT, Muench DG. Identification of a rice RNA- and microtubule-binding protein as the multifunctional protein, a peroxisomal enzyme involved in the beta -oxidation of fatty acids. J Biol Chem. (2002) 277:2419–29. doi: 10.1074/jbc.M109510200
79. Granato D, Bergonzelli GE, Pridmore RD, Marvin L, Rouvet M, Corthesy-Theulaz IE. Cell surface-associated elongation factor Tu mediates the attachment of Lactobacillus johnsonii NCC533 (La1) to human intestinal cells and mucins. Infect Immun. (2004) 72:2160–9. doi: 10.1128/IAI.72.4.2160-2169.2004
80. Dallo SF, Kannan TR, Blaylock MW, Baseman JB. Elongation factor Tu and E1 beta subunit of pyruvate dehydrogenase complex act as fibronectin binding proteins in Mycoplasma pneumoniae. Mol Microbiol. (2002) 46:1041–51. doi: 10.1046/j.1365-2958.2002.03207.x
81. Kesimer M, Kilic N, Mehrotra R, Thornton DJ, Sheehan JK. Identification of salivary mucin MUC7 binding proteins from Streptococcus gordonii. BMC Microbiol. (2009) 9:163. doi: 10.1186/1471-2180-9-163
82. Kunert A, Losse J, Gruszin C, Huhn M, Kaendler K, Mikkat S, et al. Immune evasion of the human pathogen Pseudomonas aeruginosa: elongation factor Tuf is a factor H and plasminogen binding protein. J Immunol. (2007) 179:2979–88. doi: 10.4049/jimmunol.179.5.2979
83. Sun Y, Carneiro N, Clore AM, Moro GL, Habben JE, Larkins BA. Characterization of maize elongationfactor 1A and its relationship to protein quality in the endosperm. Plant Physiol. (1997) 115:1101–7. doi: 10.1104/pp.115.3.1101
84. Antikainen J, Kuparinen V, Lahteenmaki K, Korhonen TK. Enolases from Gram-positive bacterial pathogens and commensal lactobacilli share functional similarity in virulence-associated traits. FEMS Immunol Med Microbiol. (2007) 51:526–34. doi: 10.1111/j.1574-695X.2007.00330.x
85. Castaldo C, Vastano V, Siciliano RA, Candela M, Vici M, Muscariello L, et al. Surface displaced alfa-enolase of Lactobacillus plantarum is a fibronectin binding protein. Microb Cell Fact. (2009) 8:14. doi: 10.1186/1475-2859-8-14
86. Esgleas M, Li Y, Hancock MA, Harel J, Dubreuil JD, Gottschalk M. Isolation and characterization of alpha-enolase, a novel fibronectin-binding protein from Streptococcus suis. Microbiology. (2008) 154(Pt. 9):2668–79. doi: 10.1099/mic.0.2008/017145-0
87. Floden AM, Watt JA, Brissette CA. Borrelia burgdorferi enolase is a surface-exposed plasminogen binding protein. PLoS ONE. (2011) 6:e27502. doi: 10.1371/journal.pone.0027502
88. Jong AY, Chen SH, Stins MF, Kim KS, Tuan TL, Huang SH. Binding of Candida albicans enolase to plasmin(ogen) results in enhanced invasion of human brain microvascular endothelial cells. J Med Microbiol. (2003) 52:615–22. doi: 10.1099/jmm.0.05060-0
89. Carneiro CR, Postol E, Nomizo R, Reis LF, Brentani RR. Identification of enolase as a laminin-binding protein on the surface of Staphylococcus aureus. Microbes Infect. (2004) 6:604–8. doi: 10.1016/j.micinf.2004.02.003
90. Sha J, Erova TE, Alyea RA, Wang S, Olano JP, Pancholi V, et al. Surface-expressed enolase contributes to the pathogenesis of clinical isolate SSU of Aeromonas hydrophila. J Bacteriol. (2009) 191:3095–107. doi: 10.1128/JB.00005-09
91. Lorenzatto KR, Monteiro KM, Paredes R, Paludo GP, da Fonseca MM, Galanti N, et al. Fructose- bisphosphate aldolase and enolase from Echinococcus granulosus: genes, expression patterns and protein interactions of two potential moonlighting proteins. Gene. (2012) 506:76–84. doi: 10.1016/j.gene.2012.06.046
92. Mundodi V, Kucknoor AS, Alderete JF. Immunogenic and plasminogen-binding surface-associated alpha-enolase of Trichomonas vaginalis. Infect Immun. (2008) 76:523–31. doi: 10.1128/IAI.01352-07
93. Hattori T, Takei N, Mizuno Y, Kato K, Kohsaka S. Neurotrophic and neuroprotective effects of euron-specific enolase on cultured neurons from embryonic rat brain. Neurosci Res. (1995) 21:191–8. doi: 10.1016/0168-0102(94)00849-B
94. Decker BL, Wickner WT. Enolase activates homotypic vacuole fusion and protein transport to the vacuole in yeast. J Biol Chem. (2006) 281:14523–8. doi: 10.1074/jbc.M600911200
95. Bhowmick IP, Kumar N, Sharma S, Coppens I, Jarori GK. Plasmodium falciparum enolase: stage-specific expression and sub-cellular localization. Malar J. (2009) 8:179. doi: 10.1186/1475-2875-8-179
96. Entelis N, Brandina I, Kamenski P, Krasheninnikov IA, Martin RP, Tarassov I. A glycolytic enzyme, enolase, is recruited as a cofactor of tRNA targeting toward mitochondria in Saccharomyces cerevisiae. Genes Dev. (2006) 20:1609–20. doi: 10.1101/gad.385706
97. Liu X, Reig B, Nasrallah IM, Stover PJ. Human cytoplasmic serine hydroxymethyltransferase is an mRNA binding protein. Biochemistry. (2000) 39:11523–31. doi: 10.1021/bi000665d
98. Wistow G, Piatigorsky J. Recruitment of enzymes as lens structural proteins. Science. (1987) 236:1554–6. doi: 10.1126/science.3589669
99. Tomlinson IP, Alam NA, Rowan AJ, Barclay E, Jaeger EE, Kelsell D, et al. Germline mutations in FH predispose to dominantly inherited uterine fibroids, skin leiomyomata and papillary renal cell cancer. Nat Genet. (2002) 30:406–10. doi: 10.1038/ng849
100. Alvarez RA, Blaylock MW, Baseman JB. Surface localized glyceraldehyde-3-phosphate dehydrogenase of Mycoplasma genitalium binds mucin. Mol Microbiol. (2003) 48:1417–25. doi: 10.1046/j.1365-2958.2003.03518.x
101. Gozalbo D, Gil-Navarro I, Azorin I, Renau-Piqueras J, Martinez JP, Gil ML. The cell wall-associated glyceraldehyde-3-phosphate dehydrogenase of Candida albicans is also a fibronectin and laminin binding protein. Infect Immun. (1998) 66:2052–9.
102. Bergmann S, Rohde M, Hammerschmidt S. Glyceraldehyde-3-phosphate dehydrogenase of Streptococcus pneumoniae is a surface-displayed plasminogen-binding protein. Infect Immun. (2004) 72:2416–9. doi: 10.1128/IAI.72.4.2416-2419.2004
103. Egea L, Aguilera L, Gimenez R, Sorolla MA, Aguilar J, Badia J, et al. Role of secreted glyceraldehyde-3- phosphate dehydrogenase in the infection mechanism of enterohemorrhagic and enteropathogenic Escherichia coli: interaction of the extracellular enzyme with human plasminogen and fibrinogen. Int J Biochem Cell Biol. (2007) 39:1190–203. doi: 10.1016/j.biocel.2007.03.008
104. Sriram G, Martinez JA, McCabe ER, Liao JC, Dipple KM. Single-gene disorders: what role could moonlighting enzymes play? Am J Hum Genet. (2005) 76:911–24. doi: 10.1086/430799
105. Pancholi V, Fischetti VA. A major surface protein on group A streptococci is a glyceraldehyde-3-phosphate- dehydrogenase with multiple binding activity. J Exp Med. (1992) 176:415–26. doi: 10.1084/jem.176.2.415
106. Jin H, Song YP, Boel G, Kochar J, Pancholi V. Group A streptococcal surface GAPDH, SDH, recognizes uPAR/CD87 as its receptor on the human pharyngeal cell and mediates bacterial adherence to host cells. J Mol Biol. (2005) 350:27–41. doi: 10.1016/j.jmb.2005.04.063
107. Sampath P, Mazumder B, Seshadri V, Gerber CA, Chavatte L, Kinter M, et al. Noncanonical function of glutamyl-prolyl-tRNA synthetase: gene-specific silencing of translation. Cell. (2004) 119:195–208. doi: 10.1016/j.cell.2004.09.030
108. Nagata H, Iwasaki M, Maeda K, Kuboniwa M, Hashino E, Toe M, et al. Identification of the binding domain of Streptococcus oralis glyceraldehyde-3-phosphate dehydrogenase for Porphyromonas gingivalis major fimbriae. Infect Immun. (2009) 77:5130–8. doi: 10.1128/IAI.00439-09
109. Modun B, Morrissey J, Williams P. The staphylococcal transferrin receptor: a glycolytic enzyme with novel functions. Trends Microbiol. (2000) 8:231–7. doi: 10.1016/S0966-842X(00)01728-5
110. Lu GT, Xie JR, Chen L, Hu JR, An SQ, Su HZ, et al. Glyceraldehyde-3-phosphate dehydrogenase of Xanthomonas campestris pv. campestris is required for extracellular polysaccharide production and full virulence. Microbiology. (2009) 155:1602–12. doi: 10.1099/mic.0.023762-0
111. Fulde M, Bernardo-Garcia N, Rohde M, Nachtigall N, Frank R, Preissner KT, et al. Pneumococcal phosphoglycerate kinase interacts with plasminogen and its tissue activator. Thromb Haemost. (2014) 111:401–16. doi: 10.1160/TH13-05-0421
112. Lay AJ, Jiang XM, Kisker O, Flynn E, Underwood A, Condron R, et al. Phosphoglycerate kinase acts in tumour angiogenesis as a disulphide reductase. Nature. (2000) 408:869–73. doi: 10.1038/35048596
113. Kennedy MC, Mende-Mueller L, Blondin GA, Beinert H. Purification and characterization of cytosolic aconitase from beef liver and its relationship to the iron-responsive element binding protein. Proc Natl Acad Sci USA. (1992) 89:11730–4. doi: 10.1073/pnas.89.24.11730
114. Philpott CC, Klausner RD, Rouault TA. The bifunctional iron-responsive element binding protein/cytosolic aconitase: the role of active-site residues in ligand binding and regulation. Proc Natl Acad Sci USA. (1994) 91:7321–5. doi: 10.1073/pnas
115. Banerjee S, Nandyala AK, Raviprasad P, Ahmed N, Hasnain SE. Iron-dependent RNA-binding activity of Mycobacterium tuberculosis aconitase. J Bacteriol. (2007) 189:4046–52. doi: 10.1128/JB.00026-07
116. Chen XJ, Wang X, Butow RA. Yeast aconitase binds and provides metabolically coupled protection to mitochondrial DNA. Proc Natl Acad Sci USA. (2007) 104:13738–43. doi: 10.1073/pnas.0703078104
117. Chen XJ, Wang X, Kaufman BA, Butow RA. Aconitase couples metabolic regulation to mitochondrial DNA maintenance. Science. (2005) 307:714–7. doi: 10.1126/science.1106391
118. Commichau FM, Stulke J. Trigger enzymes: bifunctional proteins active in metabolism and in controlling gene expression. Mol Microbiol. (2008) 67:692–702. doi: 10.1111/j.1365-2958.2007.06071.x
119. Kainulainen V, Loimaranta V, Pekkala A, Edelman S, Antikainen J, Kylvaja R, et al. Glutamine synthetase and glucose-6-phosphate isomerase are adhesive moonlighting proteins of Lactobacillus crispatus released by epithelial cathelicidin LL-37. J Bacteriol. (2012) 194:2509–19. doi: 10.1128/JB.06704-11
120. Jang HH, Lee KO, Chi YH, Jung BG, Park SK, Park JH, et al. Two enzymes in one; two yeast peroxiredoxins display oxidative stress-dependent switching from a peroxidase to a molecular chaperone function. Cell. (2004) 117:625–35. doi: 10.1016/j.cell.2004.05.002
121. Urban C, Xiong X, Sohn K, Schroppel K, Brunner H, Rupp S. The moonlighting protein Tsa1p is implicated in oxidative stress response and in cell wall biogenesis in Candida albicans. Mol Microbiol. (2005) 57:1318–41. doi: 10.1111/j.1365-2958.2005.04771.x
122. Chen JW, Dodia C, Feinstein SI, Jain MK, Fisher AB. 1-Cys peroxiredoxin, a bifunctional enzyme with glutathione peroxidase and phospholipase A2 activities. J Biol Chem. (2000) 275:28421–7. doi: 10.1074/jbc.M005073200
123. Moon H, Lee B, Choi G, Shin D, Prasad DT, Lee O, et al. NDP kinase 2 interacts with two oxidative stress- activated MAPKs to regulate cellular redox state and enhances multiple stress tolerance in transgenic plants. Proc Natl Acad Sci USA. (2003) 100:358–63. doi: 10.1073/pnas.252641899
124. Bateman OA, Purkiss AG, van Montfort R, Slingsby C, Graham C, Wistow G. Crystal structure of beta-crystallin: adaptation of a class 1 aldehyde dehydrogenase for a new role in the eye lens. Biochemistry. (2003) 42:4349–56. doi: 10.1021/bi027367w
125. Fujii Y, Kimoto H, Ishikawa K, Watanabe K, Yokota Y, Nakai N, et al. Taxon-specific zeta -crystallin in Japanese tree frog (Hyla japonica) lens. J Biol Chem. (2001) 276:28134–9. doi: 10.1074/jbc.M102880200
126. Rao PV, Zigler JSJr. Zeta-crystallin from guinea pig lens is capable of functioning catalytically as an oxidoreductase. Arch Biochem Biophys. (1991) 284:181–5. doi: 10.1016/0003-9861(91)90281-M
127. Rao PV, Krishna CM, Zigler JSJr. Identification and characterization of the enzymatic activity of zeta- crystallin from guinea pig lens. a novel NADPH:quinone oxidoreductase. J Biol Chem. (1992) 267:96–102.
128. Huang QL, Russell P, Stone SH, Zigler JSJr. Zeta-crystallin, a novel lens protein from the guinea pig. CurrEye Res. (1987) 6:725–32. doi: 10.3109/02713688709034836
129. Noah CE, Malik M, Bublitz DC, Camenares D, Sellati TJ, Benach JL, et al. GroEL and lipopolysaccharide from Francisella tularensis live vaccine strain synergistically activate human macrophages. Infect Immun. (2010) 78:1797–806. doi: 10.1128/IAI.01135-09
130. Cockle PJ, Gordon SV, Lalvani A, Buddle BM, Hewinson RG, Vordermeier HM. Identification of novel Mycobacterium tuberculosis antigens with potential as diagnostic reagents or subunit vaccine candidates by comparative genomics. Infect Immun. (2002) 70:6996–7003. doi: 10.1128/IAI.70.12.6996-7003.2002
131. Ernst JD, Lewinsohn DM, Behar S, Blythe M, Schlesinger LS, Kornfeld H, et al. Meeting report: NIH workshop on the tuberculosis immune epitope database. Tuberculosis (Edinb). (2008) 88:366–70. doi: 10.1016/j.tube.2007.11.002
132. Lu M, Xia ZY, Bao L. Enhancement of antimycobacterial Th1-cell responses by a Mycobacterium bovis BCG prime-protein boost vaccination strategy. Cell Immunol. (2013) 285:111–7. doi: 10.1016/j.cellimm.2013.10.001
133. Jiang Y, Liu H, Wang X, Xiao S, Li M, Li G, et al. Genetic diversity of immune-related antigens in region of difference 2 of Mycobacterium tuberculosis strains. Tuberculosis (Edinb). (2017) 104:1–7. doi: 10.1016/j.tube.2016.05.002
134. Andersen P. Host responses and antigens involved in protective immunity to Mycobacterium tuberculosis. Scand J Immunol. (1997) 45:115–31. doi: 10.1046/j.1365-3083.1997.d01-380.x
135. Mustafa T, Wiker HG, Morkve O, Sviland L. Reduced apoptosis and increased inflammatory cytokines in granulomas caused by tuberculous compared to non-tuberculous mycobacteria: role of MPT64 antigen in apoptosis and immune response. Clin Exp Immunol. (2007) 150:105–13. doi: 10.1111/j.1365-2249.2007.03476.x
136. Backus KM, Dolan MA, Barry CS, Joe M, McPhie P, Boshoff HI, et al. The three Mycobacterium tuberculosis antigen 85 isoforms have unique substrates and activities determined by non-active site regions. J Biol Chem. (2014) 289:25041–53. doi: 10.1074/jbc.M114.581579
137. van Els CA, Corbiere V, Smits K, van Gaans-van den Brink JA, Poelen MC, Mascart F, et al. Toward Understanding the Essence of Post-Translational Modifications for the Mycobacterium tuberculosis immunoproteome. Front Immunol. (2014) 5:361. doi: 10.3389/fimmu.2014.00361
138. Smith GT, Sweredoski MJ, Hess S. O-linked glycosylation sites profiling in Mycobacterium tuberculosis culture filtrate proteins. J Proteomics. (2014) 97:296–306. doi: 10.1016/j.jprot.2013.05.011
139. Lauvau G, Pamer EG. CD8 T cell detection of bacterial infection: sniffing for formyl peptides derived from Mycobacterium tuberculosis. J Exp Med. (2001) 193:F35–39. doi: 10.1084/jem.193.10.F35
140. Doi T, Yamada H, Yajima T, Wajjwalku W, Hara T, Yoshikai Y. H2-M3-restricted CD8+ T cells induced by peptide-pulsed dendritic cells confer protection against Mycobacterium tuberculosis. J Immunol. (2007) 178:3806–13. doi: 10.4049/jimmunol.178.6.3806
141. Mir SA, Sharma S. Immunotherapeutic potential of N-formylated peptides of ESAT-6 and glutamine synthetase in experimental tuberculosis. Int Immunopharmacol. (2014) 18:298–303. doi: 10.1016/j.intimp.2013.09.010
142. Dobos KM, Khoo KH, Swiderek KM, Brennan PJ, Belisle JT. Definition of the full extent of glycosylation of the 45-kilodalton glycoprotein of Mycobacterium tuberculosis. J Bacteriol. (1996) 178:2498–506. doi: 10.1128/jb.178.9.2498-2506.1996
143. Horn C, Namane A, Pescher P, Riviere M, Romain F, Puzo G, et al. Decreased capacity of recombinant 45/47- kDa molecules (Apa) of Mycobacterium tuberculosis to stimulate T lymphocyte responses related to changes in their mannosylation pattern. J Biol Chem. (1999) 274:32023–30.
144. Wiker HG, Harboe M. The antigen 85 complex: a major secretion product of Mycobacterium tuberculosis. Microbiol Rev. (1992) 56:648–61.
145. Malen H, Softeland T, Wiker HG. Antigen analysis of Mycobacterium tuberculosis H37Rv culture filtrate proteins. Scand J Immunol. (2008) 67:245–52. doi: 10.1111/j.1365-3083.2007.02064.x
146. Wiker HG, Harboe M, Nagai S, Patarroyo ME, Ramirez C, Cruz N. MPB59, a widely cross-reacting protein of Mycobacterium bovis BCG. Int Arch Allergy Appl Immunol. (1986) 81:307–14. doi: 10.1159/000234154
147. Harboe M, Wiker HG, Duncan JR, Garcia MM, Dukes TW, Brooks BW, et al. Protein G-based enzyme-linked immunosorbent assay for anti-MPB70 antibodies in bovine tuberculosis. J Clin Microbiol. (1990) 28:913–21.
148. Baldwin SL, D'Souza CD, Orme IM, Liu MA, Huygen K, Denis O, et al. Immunogenicity and protective efficacy of DNA vaccines encoding secreted and non-secreted forms of Mycobacterium tuberculosis Ag85A. Tuber Lung Dis. (1999) 79:251–9. doi: 10.1054/tuld.1998.0196
149. Belisle JT, Vissa VD, Sievert T, Takayama K, Brennan PJ, Besra GS. Role of the major antigen of Mycobacterium tuberculosis in cell wall biogenesis. Science. (1997) 276:1420–2. doi: 10.1126/science.276.5317.1420
150. Kremer L, Maughan WN, Wilson RA, Dover LG, Besra GS. The M. tuberculosis antigen 85 complex and mycolyltransferase activity. Lett Appl Microbiol. (2002) 34:233–7. doi: 10.1046/j.1472-765x.2002.01091.x
151. Goins CM, Dajnowicz S, Smith MD, Parks JM, Ronning DR. Mycolyltransferase from Mycobacterium tuberculosis in covalent complex with tetrahydrolipstatin provides insights into antigen 85 catalysis. J Biol Chem. (2018) 293:3651–62. doi: 10.1074/jbc.RA117.001681
152. Abou-Zeid C, Ratliff TL, Wiker HG, Harboe M, Bennedsen J, Rook G, et al. Characterization of fibronectin-binding antigens released by Mycobacterium tuberculosis and Mycobacterium bovis BCG. Infect Immun. (1988) 56:3046–51.
153. Kuo CJ, Ptak CP, Hsieh CL, Akey BL, Chang YF. Elastin, a novel extracellular matrix protein adhering to mycobacterial antigen 85 complex. J Biol Chem. (2013) 288:3886–96. doi: 10.1074/jbc.M112.415679
154. Swierzko AS, Bartlomiejczyk MA, Brzostek A, Lukasiewicz J, Michalski M, Dziadek J, et al. Mycobacterial antigen 85 complex (Ag85) as a target for ficolins and mannose-binding lectin. Int J Med Microbiol. (2016) 306:212–21. doi: 10.1016/j.ijmm.2016.04.004
155. Pathan AA, Sander CR, Fletcher HA, Poulton I, Alder NC, Beveridge NE, et al. Boosting BCG with recombinant modified vaccinia ankara expressing antigen 85A: different boosting intervals and implications for efficacy trials. PLoS ONE. (2007) 2:e1052. doi: 10.1371/journal.pone.0001052
156. Karbalaei Zadeh Babaki M, Soleimanpour S, Rezaee SA. Antigen 85 complex as a powerful Mycobacterium tuberculosis immunogene: biology, immune-pathogenicity, applications in diagnosis, and vaccine design. Microb Pathog. (2017) 112:20–9. doi: 10.1016/j.micpath.2017.08.040
157. Berks BC. A common export pathway for proteins binding complex redox cofactors? Mol Microbiol. (1996) 22:393–404. doi: 10.1046/j.1365-2958.1996.00114.x
158. Bendtsen JD, Jensen LJ, Blom N, Von Heijne G, Brunak S. Feature-based prediction of non-classical and leaderless protein secretion. Protein Eng Des Sel. (2004) 17:349–56. doi: 10.1093/protein/gzh037
159. Palmer T, Sargent F, Berks BC. The tat protein export pathway. EcoSal Plus. (2010) 4:1–35. doi: 10.1128/ecosalplus.4.3.2
160. Mawuenyega KG, Forst CV, Dobos KM, Belisle JT, Chen J, Bradbury EM, et al. Mycobacterium tuberculosis functional network analysis by global subcellular protein profiling. Mol Biol Cell. (2005) 16:396–404. doi: 10.1091/mbc.e04-04-0329
161. Baghel AS, Tandon R, Gupta G, Kumar A, Sharma RK, Aggarwal N, et al. Characterization of protein acyltransferase function of recombinant purified GlnA1 from Mycobacterium tuberculosis: a moon lighting property. Microbiol Res. (2011) 166:662–72. doi: 10.1016/j.micres.2011.02.001
162. Harth G, Clemens DL, Horwitz MA. Glutamine synthetase of Mycobacterium tuberculosis: extracellular release and characterization of its enzymatic activity. Proc Natl Acad Sci USA. (1994) 91:9342–6. doi: 10.1073/pnas.91.20.9342
163. Harth G, Maslesa-Galic S, Tullius MV, Horwitz MA. All four Mycobacterium tuberculosis glnA genes encode glutamine synthetase activities but only GlnA1 is abundantly expressed and essential for bacterial homeostasis. Mol Microbiol. (2005) 58:1157–72. doi: 10.1111/j.1365-2958.2005.04899.x
164. Hernandez S, Franco L, Calvo A, Ferragut G, Hermoso A, Amela I, et al. Bioinformatics and moonlighting proteins. Front Bioeng Biotechnol. (2015) 3:90. doi: 10.3389/fbioe.2015.00090
165. Wong A, Gehring C, Irving HR. Conserved functional motifs and homology modeling to predict hidden moonlighting functional sites. Front Bioeng Biotechnol. (2015) 3:82. doi: 10.3389/fbioe.2015.00082
166. Khan IK, Kihara D. Computational characterization of moonlighting proteins. Biochem Soc Trans. (2014) 42:1780–5. doi: 10.1042/BST20140214
167. Chaput M, Claes V, Portetelle D, Cludts I, Cravador A, Burny A, et al. The neurotrophic factor neuroleukin is 90% homologous with phosphohexose isomerase. Nature. (1988) 332:454–5. doi: 10.1038/332454a0
168. Xu W, Seiter K, Feldman E, Ahmed T, Chiao JW. The differentiation and maturation mediator for human myeloid leukemia cells shares homology with neuroleukin or phosphoglucose isomerase. Blood. (1996) 87:4502–6.
169. Suzuki CK, Rep M, van Dijl JM, Suda K, Grivell LA, Schatz G. ATP-dependent proteases that alsochaperone protein biogenesis. Trends Biochem Sci. (1997) 22:118–23. doi: 10.1016/S0968-0004(97)01020-7
170. Soker S, Takashima S, Miao HQ, Neufeld G, Klagsbrun M. Neuropilin-1 is expressed by endothelial andtumor cells as an isoform-specific receptor for vascular endothelial growth factor. Cell. (1998) 92:735–45. doi: 10.1016/S0092-8674(00)81402-6
171. Stutts MJ, Canessa CM, Olsen JC, Hamrick M, Cohn JA, Rossier BC, et al. CFTR as a cAMP-dependent regulator of sodium channels. Science. (1995) 269:847–50. doi: 10.1126/science.7543698
172. Henderson B, Martin A. Bacterial virulence in the moonlight: multitasking bacterial moonlighting proteins are virulence determinants in infectious disease. Infect Immun. (2011) 79:3476–91. doi: 10.1128/IAI.00179-11
173. Jeffery CJ. Moonlighting proteins: old proteins learning new tricks. Trends Genet. (2003) 19:415–7. doi: 10.1016/S0168-9525(03)00167-7
174. Jeffery CJ. Molecular mechanisms for multitasking: recent crystal structures of moonlighting proteins. Curr OpinStruct Biol. (2004) 14:663–8. doi: 10.1016/j.sbi.2004.10.001
175. Nobeli I, Favia AD, Thornton JM. Protein promiscuity and its implications for biotechnology. Nat Biotechnol. (2009) 27:157–67. doi: 10.1038/nbt1519
176. Shams F, Oldfield NJ, Wooldridge KG, Turner DP. Fructose-1, 6-bisphosphate aldolase (FBA)-a conserved glycolytic enzyme with virulence functions in bacteria: ‘ill met by moonlight'. Biochem Soc Trans. (2014) 42:1792–5. doi: 10.1042/BST20140203
177. Xolalpa W, Vallecillo AJ, Lara M, Mendoza-Hernandez G, Comini M, Spallek R, et al. Identification of novel bacterial plasminogen-binding proteins in the human pathogen Mycobacterium tuberculosis. Proteomics. (2007) 7:3332–41. doi: 10.1002/pmic.200600876
178. de la Paz Santangelo M, Gest PM, Guerin ME, Coincon M, Pham H, Ryan G, et al. (2011). Glycolytic and non-glycolytic functions of Mycobacterium tuberculosis fructose-1, 6-bisphosphate aldolase, an essential enzyme produced by replicating and non-replicating bacilli. J Biol Chem. 286:40219–31. doi: 10.1074/jbc.M111.259440
179. Arnold SL, Kent T, Hogarth CA, Schlatt S, Prasad B, Haenisch M, et al. Importance of ALDH1A enzymes in determining human testicular retinoic acid concentrations. J Lipid Res. (2015) 56:342–57. doi: 10.1194/jlr.M054718
Keywords: Mycobacterium bovis BCG Moreau, bi-dimensional electrophoresis, MALDI-TOF-TOF, surface-associated proteins, moonlighting proteins
Citation: Pagani TD, Guimarães ACR, Waghabi MC, Corrêa PR, Kalume DE, Berrêdo-Pinho M, Degrave WM and Mendonça-Lima L (2019) Exploring the Potential Role of Moonlighting Function of the Surface-Associated Proteins From Mycobacterium bovis BCG Moreau and Pasteur by Comparative Proteomic. Front. Immunol. 10:716. doi: 10.3389/fimmu.2019.00716
Received: 11 December 2018; Accepted: 18 March 2019;
Published: 26 April 2019.
Edited by:
Juraj Ivanyi, King's College London, United KingdomReviewed by:
Patrick Brennan, Colorado State University, United StatesMahavir Singh, Lionex GmbH, Germany
Copyright © 2019 Pagani, Guimarães, Waghabi, Corrêa, Kalume, Berrêdo-Pinho, Degrave and Mendonça-Lima. This is an open-access article distributed under the terms of the Creative Commons Attribution License (CC BY). The use, distribution or reproduction in other forums is permitted, provided the original author(s) and the copyright owner(s) are credited and that the original publication in this journal is cited, in accordance with accepted academic practice. No use, distribution or reproduction is permitted which does not comply with these terms.
*Correspondence: Talita Duarte Pagani, tdpagani@yahoo.com
Leila Mendonça-Lima, lmlima@ioc.fiocruz.br
†Present Address: Talita Duarte Pagani, Laboratório de Genômica Estrutural, Instituto de Biofísica Carlos Chagas Filho, Universidade Federal do Rio de Janeiro, Rio de Janeiro, Brazil