- 1Department of General Practice and Primary Health Care, University of Auckland, Auckland, New Zealand
- 2Department of Molecular Medicine and Pathology, University of Auckland, Auckland, New Zealand
Have potential clues to an effective gonorrhea vaccine been lurking in international disease surveillance data for decades? While no clinically effective vaccines against gonorrhea have been developed we present direct and indirect evidence that a vaccine is not only possible, but may already exist. Experience from Cuba, New Zealand, and Canada suggest that vaccines containing Group B Neisseria meningitides outer membrane vesicles (OMV) developed to control type-specific meningococcal disease may also prevent a significant proportion of gonorrhea. The mechanisms for this phenomenon have not yet been elucidated but we present some strategies for unraveling potential cross protective antigens and effector immune responses by exploiting stored sera from clinical trials and individuals primed with a meningococcal group B OMV vaccine (MeNZB). Elucidating these will contribute to the ongoing development of high efficacy vaccine options for gonorrhea. While the vaccine used in New Zealand, where the strongest empirical evidence has been gathered, is no longer available, the OMV has been included in the multi component recombinant meningococcal vaccine 4CMenB (Bexsero) which is now licensed and used in numerous countries. Several lines of evidence suggest it has the potential to affect gonorrhea prevalence. A vaccine to control gonorrhea does not need to be perfect and modeling supports that even a moderately efficacious vaccine could make a significant impact in disease prevalence. How might we use an off the shelf vaccine to reduce the burden of gonorrhea? What are some of the potential societal barriers in a world where vaccine hesitancy is growing? We summarize the evidence and consider some of the remaining questions.
Lessons From N. meningitidis
Unlike Neisseria meningitidis serogroups A, C, W, and Y, for which effective polysaccharide-protein conjugate vaccines have been developed, serogroup B has required alternative strategies. This is because of the poor immunogenicity of the group B capsular polysaccharide and its likely homology to fetal neural tissue (1). The original solution to this problem was the development of group B strain specific vaccines based on the outer membrane vesicle (OMV). These vaccines are based on the immunodominant protein Porin A (PorA), which is highly variable across strains, therefore their use has traditionally been considered restricted to situations where disease is dominated by a single PorA strain (2, 3). Reports on the duration of protection afforded by OMV vaccines against meningococcal disease vary according to age at vaccination and the target population, but serum bactericidal activity (SBA), which is the correlate of protection, has typically diminished among a significant proportion of vaccinees by 2 years, due to the waning of serum antibody (4).
While devastating, meningococcal disease is rare and meningococcal vaccines rely on immunogenicity data as a proxy for likely efficacy (5). This is because a randomized efficacy trial powered to detect meningococcal disease cases as a primary outcome would need in the order of 100,000 participants (6–8), rendering this approach unaffordable and impractical. Fortunately the presence of SBA provides a correlate of protection that can be used to estimate efficacy (5, 9, 10).
Estimating both the efficacy and effectiveness of meningococcal vaccines directly is hindered by the low case numbers. Both efficacy and effectiveness are generally estimated by calculating the risk of disease among vaccinated and unvaccinated persons and determining the percentage reduction in risk among each group relative to each other. Because of the low case numbers, and in the case of polysaccharide-protein-based, the significant impact on carriage (11–13) associated with meningococcal vaccines the estimates have wide confidence intervals (14).
Ultimately the public health value of these vaccines is revealed by real world experience and ecological observations on overall incidence and prevalence of disease which indirectly supports the vaccine impact. Where meningococcal vaccines are concerned estimates of 70 or 80 percent efficacy may translate to much higher effectiveness and near elimination of disease (12, 15–17).
Because the immunogenicity, efficacy, and effectiveness of OMV vaccines has generally been considered limited in terms of strain and type coverage compared with conjugate vaccines, and they have not been as widely used with less data published compared with their purer conjugated relations. While immunogenicity of OMV vaccines generally predicts efficacy (particularly for younger age groups) it may be less predictive of effectiveness (5). Less explored too are the minor components present in the OMV and their potential role in inducing not only protection against homologous PorA types but heterologous protection against a range of Neisseria. The traditional IgG activated complement mechanism that has perhaps driven and dominated the meningococcal vaccine field may miss a cocktail of novel antigens with powerful adjuvant effects (18).
Potential Impact of a Gonococcal Vaccine
An effective vaccine does not have to be a highly efficacious vaccine. When the basic reproduction number of an infection is low, and a vaccine affects transmission, then disease control can be achieved with a vaccine that has moderate efficacy. While vaccines are given to individuals to protect them against disease, many vaccines also reduce transmission, thereby protecting the broader community (19). Consideration of a vaccine's effect on carriage and transmission is a vital component of immunization programme planning.
The basic reproduction number (R0) describes the maximal potential for spread of an infection within a population. It depends on the contact rate, the duration of the infectious period and the probability that the contact between an infectious person and a susceptible person leads to an infection (19). For the most infectious diseases (measles and pertussis) a single infectious individual entering a community of non-immune individuals can infect a further (R0) 12–18 and 5–17 people, respectively (20). Effective vaccines against these diseases need to be highly efficacious and affect transmission in order to successfully control or eliminate disease. They also need to provide sustained immunity, or be given as regular boosters, and be administered to 92–94% of the population (19).
In contrast, mumps has an R0 of around 4–7 and influenza 1.4–4. The proportion of the community that needs to be immune to prevent transmission of these diseases is 75–85 and 30–75%, respectively (20). Estimates of R0 for gonorrhea are in the order of 1.18–3.6 depending on the method used. The lower estimates rely on the assumption of acquired immunity after infection, the higher estimates do not assume complete acquired immunity and are therefore likely to be more accurate (21).
Using an individual-based, epidemiological simulation model the potential impact of a hypothetical gonorrhea vaccine was modeled and the prevalence of gonorrhea in a heterosexual population estimated using various assumptions of efficacy and duration of protection. The individual-based approach applies the dynamics of the probability that an individual is in a certain state (susceptible, infected, recovered) as opposed to a static network where an individual is in one of the states (22). In this study it was assumed that there was no immunity following resolution of natural infection. The modeling demonstrated that a vaccine of moderate efficacy could have a significant impact on the prevalence of gonorrhea if strategically implemented (23). While encouraging it does, of course, depend on the availability of a vaccine.
From Ecological Data to Evidence
The epidemiological evidence from Cuba, Brazil, and New Zealand demonstrates that N. meningitidis OMV vaccines are possibly able to provide some broader protection against meningococcal disease (17, 24), particularly in older children and adults (25). These observations led to the hypothesis that they may affect a more distantly related bacterium. Examination of surveillance data clearly show a marked decline in the incidence of gonorrhea in Cuba following implementation of the VA-MENGOCBC (Figure 1). The pattern of decline in incidence for gonorrhea contrasts with syphilis and genital warts for which incidences have remained the same (8). This phenomenon was also observed in NZ, where a decline in reported gonorrhea cases during and shortly after use of the tailor made meningococcal Group B OMV vaccine MeNZB is evident (Figure 2). Like Cuba, no other sexually transmitted infections (STIs) described in the national surveillance reports declined during that period (26). While purely ecological, these observations suggested that N. meningitidis OMV vaccines might offer cross protection against gonorrhea.
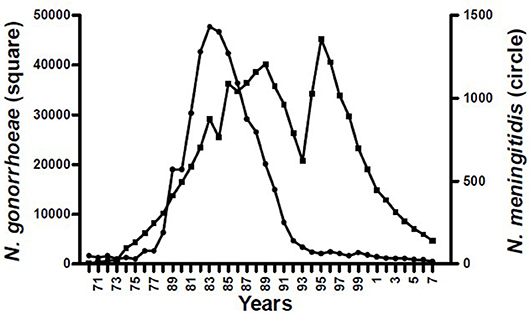
Figure 1. Morbidity of Neisseria pathogenic species in Cuba 1970–2007 (8).
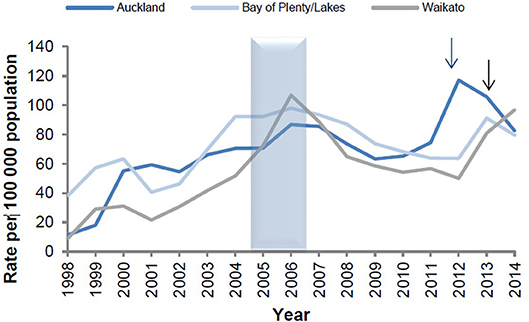
Figure 2. Reported gonorrhea rates in New Zealand 1998–2014, selected sentinel regions (26). Arrows denote shifts from culture testing to NAAT testing.
This hypothesis was first tested in New Zealand where both the MeNZB exposure and gonorrhea outcome data could be linked in a retrospective case-control study. The demographic details and vaccine status of 14,730 deidentified sexual health clinic patients aged 15–30 years, who had been eligible to receive the MeNZB vaccine, were determined via the linkage to the country's National Immunization Register and database of demographic information. The outcomes of interest were laboratory confirmed gonorrhea and, as a control, chlamydia. The odds of disease outcomes in vaccinated and unvaccinated patients were compared. Individuals who had received the MeNZB vaccine were significantly less likely to be gonorrhea cases than chlamydia controls, with an adjusted OR 0.69 (95% CI 0.61–0.79%); p < 0·0001. This translated to a vaccine effectiveness estimate of 31% (95% CI 21–39) (27).
Following on from the case-control study a national cohort study of gonorrhea hospitalizations was conducted. The eligible cohort consisted of 1,143,897 individuals born 1984–1999 residing in New Zealand during 2004–2008 and therefore eligible to receive the MeNZB vaccine during that time. In this study administrative datasets of demographics, customs, hospitalization, education, income tax, and immunization, were linked using a national data collection called the National Integrated Data Infrastructure. The primary outcome was hospitalization with a primary diagnosis of gonorrhea. Using Cox's proportional hazards models with a Firth correction for rare outcomes, estimates of hazard ratios were generated. Vaccine effectiveness estimates were calculated as 1-Hazard Ratio expressed as percent. After adjustment for gender, ethnicity and deprivation, MeNZB vaccine effectiveness against gonorrhea associated hospitalization was estimated to be 24% (95% CI 1–42%) for the whole eligible cohort and 47% (95% CI 18–66) for those vaccinated in adolescence and therefore most likely to be at risk for gonorrhea during the follow-up period (28). While limited by small numbers the findings supported the earlier case-control study.
The MeNZB vaccine was developed to control a meningococcal group B epidemic and it is no longer available. However, the same OMV used in the MeNZB vaccine is included in the new generation meningococcal group B 4CMenB (Bexsero) vaccine. Bexsero also includes three recombinant proteins that are conserved across N. meningitides [Neisserial Heparin Binding Antigen (NHBA), factor H binding protein (fHbp), and Neisseria Adhesion A (NadA)], two of which are shared variably with Neisseria gonorrhoeae (N. gonorrhoeae) (29). NHBA and fHbp, along with accessory fusion proteins GNA1030 and GNA2091 in the formulation, are capable of inducing immune responses against the gonococcus (30, 31). If additional immunogenicity and cross protection is afforded by this new generation vaccine, on account of the additional antigens and synergistic combination, then the potential for effectiveness is likely to be higher than that demonstrated by the MeNZB vaccine.
A mass vaccination campaign in Quebec, Canada, using Bexsero, provided an opportunity to observe a potential vaccine effect on gonorrhea. In 2014 Bexsero was administered to individuals aged 6-months to 20-years of age. Gonorrhea and chlamydia notifications to public health authorities during the pre-vaccination period and post-vaccination period (2006–June 2017) were analyzed and the impact of the campaign estimated by a Poisson regression model. Vaccine coverage was 82% in the target group. There were 231 gonorrhea cases reported among persons 14 years and older (IR: 8.4/100,000 person-years) in the region of the mass campaign during the study period. A decrease in the number of cases and risk among individuals 14–20 years was observed during the post-vaccination period. In contrast, it increased in those 21 years and older. As observed in both New Zealand and Cuba, chlamydia infections increased among both the vaccinated and unvaccinated age groups. The estimate of vaccination impact was a risk reduction in gonorrhea of 59% (95% CI: −22 to 84%; P = 0.1) (32). While the confidence intervals are wide and include “0” this is likely due to the low study power.
Together the NZ, Cuban, and Canadian data suggest that we likely already have a vaccine to hand, that if used strategically, could impact on the prevalence of gonorrhea. But what are the mechanisms?
Strategies to Define the Mechanisms of Cross-Protection
The intriguing observation that vaccination with meningococcal B OMVs confers a degree of protection to gonorrhea raises some interesting questions about possible mechanisms of action. Historically there have been some major confounders for development of a gonococcal vaccine—earlier trials proved unsuccessful, there are no defined correlates of protective immunity and the optimum route of delivery is unknown. The MeNZB data suggest it may be possible to elicit protective immunity to gonococci with a parenteral vaccine and there is now an opportunity to examine resources generated both from the clinical trials of this vaccine and New Zealand's large MeNZB primed cohort for clues as to how this vaccine confers this effect.
The lack of known correlates of protective immunity is a major stumbling block to identifying vaccine candidate antigens or novel approaches for developing a gonococcal vaccine. Population data show that prior exposure does not protect individuals from re-infection (33) and human challenge studies have demonstrated that recently infected individuals remain susceptible to re-infection with the challenge strain (34). Longitudinal studies of individuals regularly infected with gonorrhea have proved invaluable in demonstrating how host antibody mediated responses to selected antigens have been used to subvert the immune response. Gonococci can be typed into serovars on the basis of expression of variants of a major outer membrane protein, porin (PorB). Both porB typing alone or in combination with additional molecular analyses have shown that por mutates in response to immunological pressure, suggesting that the effective immunity to gonococci may develop, but is confounded via a shifting of phenotype by the bacteria (35, 36). Development of an elevated antibody response to another major outer membrane protein, reduction modifiable protein (Rmp)/protein III, is associated with enhanced susceptibility to infection (37). In vitro studies have shown that IgG antibodies to Rmp prevent other potentially protective antibodies from initiating bactericidal activity to gonococci (38). However, individuals with high occupational exposure to gonococci do eventually develop serovar-specific immunity (35), suggesting a vaccine may ultimately be feasible (39). Experimental, self-limiting infection of human subjects with gonococci results in development of modest local and systemic specific antibody responses, with a suggestion that serum antibody responses to lipo-oligosaccharides (LOS) may confer a degree of protection from re-infection (34).
Several prospective gonococcal vaccines have been tested in humans without success. To date these attempts have included parenteral administration of non-adjuvanted material including partially lysed whole bacteria (40), pilin (41), and protein I/PorB, a major porin/outer membrane protein [reviewed in (42)]. One of the most promising pre-clinical vaccine candidates identified to date is a highly conserved oligosaccharide epitope (2C7) common to most gonococcal isolates—targeting this epitope elicits sialylation-independent bactericidal activity in vitro and leads to enhanced clearance of infection in mice (43, 44). Pre-clinical evaluations suggest that gonococcal OMVs are also a promising vaccine candidate (45–47) but they have not been tested in humans. In contrast serogroup B N. meningitidis was the first organism to be screened for meningococcal vaccine candidate antigens using a sophisticated genomic-led approach termed “reverse vaccinology,” which led to the selection of the highly conserved vaccine candidate antigens incorporated into Bexsero (48). Importantly though for full efficacy and enhancement of coverage to a broader range of subserotypes and better immunogenicity in younger age groups, this vaccine still requires the addition of OMV from MeNZB, with porin A considered to be a particularly important constituent (49, 50).
The OMVs are comprised of a complex mix of periplasmic, cytoplasmic, and outer membrane proteins (51). The dominant components include outer membrane proteins such as porin A, porin B, Rmp, NspA, and the OpcA invasin which are incorporated into vesicles in conjunction with lipopolysaccharide [summarized in (52)]. An immuno-proteomics analysis of the related Cuban meningococcal OMV vaccine suggests that the antibody response is primarily targeted to these major antigens (53). Population genomics has demonstrated the close genetic relationship between N. meningitidis and N. gonorrhoeae (50) suggesting OMVs are likely to be the source of numerous conserved vaccine candidates, both protein and glycolipid. Screening of gonococcal OMVs from multiple strains with human anti-meningococcal sera would be an effective approach to identify cross-reactive, conserved “human relevant” antigens. Although this approach is likely to reveal multiple cross-reactive antigens, some of which are unlikely to be suitable vaccine candidates, selection of novel candidate antigens can be informed and complemented by the rapid expansion of knowledge of gonococcal biology. For example, conserved novel antigens of importance for survival of the gonococcus have recently been identified by quantitative proteomics, which has successfully been applied to cell envelope and OMVs from multiple strains of N. gonorrhoeae (54).
As N. gonorrhoeae is an exclusive human pathogen the relevance of animal models, particularly non-primate models, is contentious. Female mice can be transiently infected with N. gonorrhoeae if they are pre-treated with estradiol and inoculated during the pro-estrous phase (55), but lack important features such as carcinoembryogenic antigen-related cell adhesion molecules (CEACAM; CD66) on neutrophils and epithelial cells, which are typically targeted by the gonococcal opacity associated proteins (Opas) (56, 57). Gonorrhea infection in mice does recapitulate several known features of human infection, eliciting a polarized neutrophilic Th17 driven response and production of pro-inflammatory cytokines (58–60). Conversely, accelerated clearance of the infection is linked to a Th-1 driven immune response in mice, particularly the production of interferon-γ, in conjunction with the development of both systemic and local IgG and A (45, 61–63). Of note, vaginal vaccination with gonococcal OMVs delivered with encapsulated IL-12 can elicit enduring protection to multiple distinct gonococcal isolates (45) and intranasal administration of gonococcal OMVs can expedite resolution of infection (46), however it is not yet known whether parenteral vaccination with either gonococcal or meningococcal OMVs can elicit a comparable effect.
Although gonococci can infect other mucosal sites, the most important site of entry is the genital mucosa. Unlike other mucosal locations, the genitals lack inductive sites for local antibody production and IgG (produced locally and systemically), not IgA, is considered to be the most important class of antibody (64). It has been traditionally accepted that the optimal way of developing vaccine-elicited immunity to mucosal pathogens is to immunize by the mucosal route, preferably one which mimics the natural route of infection (65). Accordingly there are several examples of highly effective vaccines—most notably the live oral vaccine for poliomyelitis—which are administered orally, although further refinements in this arena have been stymied by the lack of safe, effective mucosal adjuvants. More recently it has been proposed that parenteral vaccines may be an equally feasible means of stimulating strong and appropriate protective mucosal responses (66). Of particular relevance to gonorrhea, the Human Papillomavirus (HPV) vaccine is comprised of an adjuvanted preparation of virus-like particles given intramuscularly, which results in the development of enduring antibody responses in both systemic (serum) and local (cervicovaginal) sites, with a strong correlation between IgG levels in the two locations, supporting the possibility of serum transudation or exudation into the genital mucosa (67). Evidence from gonococcal vaccine studies in mice also indicate that antibody responses—including systemic IgG responses—are associated with protective immunity to gonococci, suggesting serum antibody responses can reasonably be used as a correlate of protection (45).
But how does MeNZB, which is reported to confer a relatively short-lived period of protective immunity in infants and toddlers (4, 68), confer cross-protective immunity to gonococci for some years after vaccination? An elevated SBA is the benchmark for verifying sustained protective immunity to group B meningococci, but there may well be other aspects of the vaccine initiated immune responses that are equally or more important for development of immunity to gonococci. Specific mucosal immune responses are likely to be necessary for limiting the development—and ideally transmission—of symptomatic gonorrhea infections. While the systemic antibody responses elicited by MeNZB have been thoroughly examined, there have been no long term studies on the development of the mucosal antibodies in response to vaccination. An assessment of adults shortly after vaccination showed either no change or very modest increases in salivary antibody responses in response to parenteral vaccination with the Norwegian MenBVac (69) or MeNZB (70). Conversely, anti-meningococcal salivary IgA responses were reported to increase with age and/or meningococcal carriage (71), suggesting that an examination of the long-term effects of vaccination in childhood may yield useful information on whether nasopharyngeal acquisition and/or carriage of commensal Neisseria can boost mucosal antibody responses. Given the issue of rising rates of oral gonorrhea and evidence of oral-genital transmission of infection (41), induction of strong mucosal immune responses in both sites is likely to be important.
Analysis of specific cellular responses in the tonsils suggest that parenteral vaccination of adults with MeNZB results in re-programming of the mucosal immune response to meningococci in the nasopharynx (70). OMVs contain a complex package of virulence factors, TLR agonists, and other secreted or membrane associated components that interact and modulate host immunity (72). Detergent extracted MeNZB OMVs are reported to consistently contain ~100 distinct proteins predominantly from the outer membrane compartment (73) and although delivered with an adjuvant, they are also intrinsically immunogenic. Perhaps OMVs from mucosal pathogens such as Neisseria have the capacity to stimulate homing and development of immune effector cells at mucosal sites after parenteral immunization? This may be important for eliciting strong mucosal immune responses (66) as has recently been reported for the detoxified form of heat-labile toxin (dmLT) (74) and linked to properties of the adjuvant itself, rather than the route of administration. This would be an interesting concept to explore further in mice vaccinated parenterally or mucosally with OMVs; or translated to humans through application of emerging technologies in the immunogenomics and systems biology arena (75) with the potential to provide vital information to support the utility of this class of vaccines.
Human mucosa, particularly the oropharynx, is frequently host to several different species of Neisseria. Genetic analyses of both pathogenic and non-pathogenic Neisseria show they are closely related, with evolutionary studies suggesting frequent exchange of genetic material including virulence genes (76). Notably, early exposure to commensals such as N. lactamica may not only confer some degree of protection from N. meningitidis, but could be an approach for identifying novel vaccine candidate antigens (77). Carriage of non-pathogenic Neisseria may also enhance the development of immunity to Neisseria OMV vaccines (78). N. meningitidis and N. gonorrhoeae are most closely related and primarily separated from the remaining members of the genus by the presence of additional virulence genes (76). The impact of vaccination with meningococcal OMVs, which contain multiple highly conserved antigens common to many Neisseria species, on nasopharyngeal colonization has not yet been determined. A genetic analysis of the additional antigens in Bexsero suggest that this vaccine could impact non-target Neisseria species as both NHBA and the additional fusion proteins GNA1030 and GNA2091 contained in Bexsero are highly conserved across both pathogenic and commensal Neisseria, whereas N. gonorrhoeae does not express NadA and this bacterium contains only one of three possible variants of fHbp (79, 80). It has been suggested that NHBA could form the basis of a putative gonococcal vaccine, but would likely require augmentation with additional conserved proteins to enhance the effectiveness of such a vaccine (80). MeNZB anti-sera is highly likely to be a useful source for identifying additional highly conserved gonococcal immunogens. The utility of this approach has been demonstrated in rabbits, which develop cross-reactive antibodies to several strains of gonococci by ELISA and western blot in response to vaccination with MeNZB/Bexsero OMVs (31). Human MeNZB anti-sera will almost certainly show a similar level of cross-reactivity, with the potential complication of reactivity to antigens common to commensal Neisseria species.
New Zealand currently has a large cohort of individuals at or approaching the age of prime interest for studying the long-term impact of the MeNZB vaccine. Notably there is an opportunity to examine recall responses ~10 years post-priming in a population of adolescents and/or young adults, which is a good match for the likely timing of administration of a gonococcal vaccine and corresponds with the age groups at greatest risk of contracting gonorrhea. The impact of boosting these individuals with Bexsero (as the source of MeNZB OMVs) on both local and systemic antibody responses as well as the development of cellular immunity has the potential to provide valuable data on the possible targets or mechanisms of cross-reactive immunity. A large collection of sera also remains from the original MeNZB clinical trials, which were commenced in adults (81), followed by pre-teens (82), toddlers then infants (83) with serum samples obtained prior to and at regular intervals during vaccination. Untouched duplicate samples were retained after the completion of these trials and can be accessed for investigation of anti-gonococcal responses. Meningococcal SBA titres were quantified as part of the MeNZB vaccine development programme, which offers a unique opportunity to not only determine whether MeNZB vaccination elicited a bactericidal antibody response to gonococci in humans, but also to ascertain whether this correlates with elevated anti-meningococcal SBA titres.
A key question remains as to which approaches would be the most appropriate for examining possible cross-reactive immune responses to gonococci in these vaccinated individuals. Induction of functional antibody responses such as complement-mediated bacterial killing, inhibition of binding to reproductive tract epithelial cells and stimulation of opsonophagocytosis are generally considered to be suitable starting points. The development of an increased serum bactericidal antibody (SBA) response is the standard for determining the protective efficacy of vaccines to meningococci (84–86) whilst the presence of bactericidal antibodies to gonococci are frequently used as a likely surrogate of protective immunity in pre-clinical studies (47, 87, 88). Cross-reactive bactericidal antibodies are, unsurprisingly, likely to be directed to gonococcal lipopolysaccharides and surface proteins (89). Therefore, analyzing SBA responses is a valid starting point, although it will be important to incorporate multiple gonococcal strains to confirm broad, cross-protective bactericidal activity to gonococci. A limitation of the SBA is that it requires use of bacteria that are resistant to complement-mediated killing by normal serum, whereas serum sensitivity is reported to be common in gonococci after in vitro culture (90). Serum resistance can be induced by the use of additives to sialylate LOS (91, 92) and screening of resistant phenotypes is more likely to represent the low susceptibility to serum-mediated killing seen in vivo. Enhanced opsonophagocytosis is considered to be another likely correlate of protective immunity to gonococci and this can readily be inferred by detection of C3b deposition on the surface of the gonococci using flow cytometry (93), as a pre-cursor to MAC-mediated lysis, and corroborated by determining whether opsonophagocytic uptake of gonococci by a neutrophil-like cell line, such as retinoic acid differentiated HL-60 cells (94), is enhanced in the presence of immune sera.
A critical step in the pathogenesis of N. gonorrhoeae is adherence to target epithelial cells where initial adherence is predominantly mediated by pili, followed by tight attachment to CEACAM via expression of OpA. The interactions of gonococci with human reproductive tract cell lines (95, 96) and primary cultures (97) have been described and these model systems can be used to establish whether introduction of sera from MeNZB vaccinated individuals inhibits adherence or invasion of gonococci. These sera can also be used to determine whether there is a quantifiable increase in antibodies to gonococcal OMVs or cell surface exposed antigens by ELISA or development of cross-reactive antibodies to antigens conserved across multiple isolates of N. gonorrheae using similar immunoproteomics approaches to those applied to N. meningitidis (53, 98, 99). Both approaches can be used to identify or validate potential gonococcal vaccine candidates, in conjunction with pre-clinical testing in in vivo models.
There have been few studies on the development of cellular immune responses to MeNZB (70) or other meningococcal OMV vaccines in adults (100, 101). None have examined early immune kinetics in naïve individuals, the development of recall responses, or induction of responses to gonococcal OMVs. The type of cellular response required to prevent gonococcal infection in humans is unknown, but murine studies link development of protective immunity with Th-1 immune responses (45). An assessment of Th profiles in response to stimulation with gonococcal antigens could ascertain whether MeNZB vaccination causes a similar skewing in humans.
Mining resources from New Zealand's MeNZB vaccination programme may be fruitful for identifying potential correlates of immunity in human subjects. Ultimately a prospective clinical trial or high quality observational study with a large cohort of high risk individuals will be necessary to acquire a complete and accurate picture of how well a vaccine containing OMVs (or other potential antigens) will perform in reducing rates of gonorrhea.
Societal and Policy Issues in Gonococcal Vaccine Deployment
Modeling has suggested that a vaccine with moderate protection might have a significant effect on the burden of gonorrhea (23). Considering Bexsero as a candidate intervention, how would we best use it?
While we do not yet know the mechanism of protection induced by these OMV vaccines that has resulted in some resistance to gonorrhea we might assume that it is not long lasting based on experience with protection against meningococcal disease as well as the waning observed in the New Zealand case-control study (27). There are still questions to be answered about the effectiveness of Bexsero. It does appear broadly protective against meningococcal disease, including against the hypervirulent Group W strain, which is increasing in prevalence in some countries (102, 103). However, to what extent this affects carriage in adolescents, and duration of protection at the population level remain to be demonstrated (103, 104). Therefore, in order to optimize protection against gonorrhea such a vaccine would need to be delivered prior to sexual debut and use of boosters possibly maintained throughout the risk period. Early adolescence also happens to coincide with a high risk period for meningococcal disease. Administering a dose of Bexsero during early adolescence in a population previously primed in infancy, or two doses in a previously naive population, might be a pragmatic strategy to reduce gonorrhea whilst at the same time improving the community immunity to meningococcal disease. If protection was demonstrated to wane during the risk period a further dose could be considered to maintain protection.
Even the most efficacious vaccine cannot prevent disease if it is not used. Funding and policy aside perhaps the greatest challenge facing immunization programmes today is the growing presence of vaccine hesitancy (105, 106). The dream of global measles and rubella elimination is unraveling as a tide of trolls, bots, and organized opposition, facilitated by social media, plays havoc with trust and confidence (107–109). Vaccine coverage rates have dived in many countries and measles resurgence is occurring among populations that had previously achieved elimination status, such as the America's and some European countries (110). How might a gonorrhea vaccine fair in this environment of growing public resistance to vaccines?
If we consider the societal factors that might be relevant to a vaccine against a disease that is largely sexually transmitted then we need look no further than the experience with the human papillomavirus (HPV) vaccines. The focus of the original marketing of HPV vaccines was prevention of cervical cancer as opposed to prevention of a sexually transmitted infection (111, 112) however, skepticism about the vaccine effectiveness and safety arose quickly from a variety of quarters (113, 114). Even with the focus on cancer there has been ongoing public outrage fed by organized lobby groups since 2007, when the first vaccine was licensed (115). After the introduction of HPV vaccine there was a shift toward a more conservative backing for the anti-vaccine movement. Presumably this was because despite the vaccine being marketed as an anti-cancer vaccine the fact that the virus is primarily sexually transmitted invoked discomfort among those with conservative and religious views about sex (116). Consequently whilst some countries have delivered to over 70–80% of the target population (117) others have fared less well with some countries either failing to implement a programme, or experiencing interruption to programs due to widespread movements aimed at discrediting the vaccine (118). Some countries have had their previously high coverage eroded to below 30% (119).
Given the multiple challenges in marketing and delivering a vaccine against a sexually transmitted infection across diverse cultures there may be an argument for desexualizing it and packaging it as a vaccine against Neisseria. However, efforts to desexualize vaccines can backfire. Withholding information that is seen to be less agreeable to the public can result in accusations of paternalism (116). This will likely result in the erosion of trust. How public health officials communicate the facts about a gonorrhea vaccine across multi-cultural societies will likely have an impact on acceptance.
Some societies have chosen to place HPV vaccine at the 9-year age mark, other societies have elected to place it at the 12–13 year mark. While the 9-year mark might desexualize the vaccine, the luxury of this choice is unlikely to be an option for a gonorrhea vaccine. The HPV vaccine, like the Hepatitis B vaccine, has a long duration of protection (120). It is unlikely that a gonorrhea vaccine based on current options will provide long-term protection, therefore placement in a national immunization programme will need to be at the age that provides highest immunity just before sexual debut.
While research continues into gonorrhea vaccine antigen discovery there is also a need for further data on the two OMV-containing N. meningitidis vaccines currently available (Bexsero and VA-MENGOCBC) that might have some utility if deployed into a sexually active population. For example, further knowledge about the effect of age and immunological experience on the vaccine response, along with boosting responses in older children, and adults who have been primed in infancy or early childhood. Other outstanding questions include the number of doses required to optimize responses to the gonococcus both qualitatively and quantitatively, and the potential to affect carriage.
Several lines of evidence suggest a vaccine that could impact on the growing burden of gonorrhea already exists. While the mechanisms are not yet understood, elucidating these will contribute to the ongoing development of high efficacy vaccine options for this disease. In order to successfully deploy a vaccine that could impact on the prevalence of gonorrhea the development of formulations that target all pathogenic Neisseria species might be the most socially acceptable, while also the most pragmatic when considering implementation into already crowded immunization schedules.
Author Contributions
All authors listed have made a substantial, direct and intellectual contribution to the work, and approved it for publication.
Conflict of Interest Statement
The authors declare that the research was conducted in the absence of any commercial or financial relationships that could be construed as a potential conflict of interest.
References
1. Finne J, Leinonen M, Makela PH. Antigenic similarities between brain components and bacteria causing meningitis - implications for vaccine development and pathogenesis. Lancet. (1983) 2:355–7. doi: 10.1016/S0140-6736(83)90340-9
2. Tauseef I, Ali YM, Bayliss CD. Phase variation of PorA, a major outer membrane protein, mediates escape of bactericidal antibodies by Neisseria meningitidis. Infect Immun. (2013) 81:1374–80. doi: 10.1128/IAI.01358-12
3. Urwin R, Russell JE, Thompson EAL, Holmes EC, Feavers IM, Maiden MCJ. Distribution of surface protein variants among hyperinvasive meningococci: implications for vaccine design. Infect Immun. (2004) 72:5955–62. doi: 10.1128/IAI.72.10.5955-5962.2004
4. Jackson C, Lennon D, Wong S, Yan J, Stewart J, Reid S, et al. Antibody persistence following MeNZB vaccination of adults and children and response to a fourth dose in toddlers. Arch Dis Childhood. (2011) 96:744–51. doi: 10.1136/adc.2009.180596
5. Harder T, Koch J, Wichmann O, Hellenbrand W. Predicted vs observed effectiveness of outer membrane vesicle (OMV) vaccines against meningococcal serogroup B disease: systematic review. J Infect. (2017) 75:81–94. doi: 10.1016/j.jinf.2017.05.001
6. Bjune G, Hoiby EA, Gronnesby JK, Arnesen O, Fredriksen JH, Halstensen A, et al. Effect of outer membrane vesicle vaccine against group B meningococcal disease in Norway. Lancet. (1991) 338:1093–6. doi: 10.1016/0140-6736(91)91961-S
7. Sierra G, Campa H, Varcacel N, Garcia I, Izquierdo P, Sotolongo P, et al. Vaccine against group B Neisseria meningitidis: protection trial and mass vaccination results in Cuba. NIPH Ann. (1991) 14:195–207. discussion:8–10.
8. Pérez O, Campo Jd, Cuello M, Gonalez E, Nunez N, Cabrera O, et al. Mucosal approaches in neisseria vaccinology. Vacc Monitor. (2009) 18:53–5. Available online at: https://www.redalyc.org/comocitar.oa?id=203414610001
9. Qin L, Gilbert PB, Corey L, McElrath MJ, Self SG. A Framework for assessing immunological correlates of protection in vaccine trials. J Infect Dis. (2007) 196:1304–12. doi: 10.1086/522428
10. Plotkin SA, Gilbert PB. Nomenclature for immune correlates of protection after vaccination. Clin Infect Dis. (2012) 54:1615–7. doi: 10.1093/cid/cis238
11. Maiden MC, Stuart JM, Group UMC. Carriage of serogroup C meningococci 1 year after meningococcal C conjugate polysaccharide vaccination. Lancet. (2002) 359:1829–30. doi: 10.1016/S0140-6736(02)08679-8
12. Daugla D, Gami J, Gamougam K, Naibei N, Mbainadji L, Narbé M, et al. Effect of a serogroup A meningococcal conjugate vaccine (PsA–TT) on serogroup A meningococcal meningitis and carriage in Chad: a community study. Lancet. (2014) 383:40–7. doi: 10.1016/S0140-6736(13)61612-8
13. Read RC, Baxter D, Chadwick DR, Faust SN, Finn A, Gordon SB, et al. Effect of a quadrivalent meningococcal ACWY glycoconjugate or a serogroup B meningococcal vaccine on meningococcal carriage: an observer-blind, phase 3 randomised clinical trial. Lancet. (2014) 384:2123–31. doi: 10.1016/S0140-6736(14)60842-4
14. Lennon DR, Jackson C, Thornley C. Why stop MeNZB vaccination? Supporting evidence for withdrawal of epidemic strain outer membrane vesicle (OMV) vaccine in New Zealand. In: van Alphen GvdD L, van der Ley P, editors. 16th International Pathogenic Neisseria Conference. Rotterdam (2008).
15. Campbell H, Andrews N, Borrow R, Trotter C, Miller E. Updated postlicensure surveillance of the meningococcal C conjugate vaccine in England and Wales: effectiveness, validation of serological correlates of protection, and modeling predictions of the duration of herd immunity. Clin Vacc Immunol. (2010) 17:840–7. doi: 10.1128/CVI.00529-09
16. Perez AE, Dickinson F, Llanes R. Invasive meningococcal disease. Cuba, 1983–2006. VaccinMonitor. (2010) 19:8–14.
18. Pérez O, Lastre M, Lapinet J, Bracho G, Diaz M, Zayas C, et al. Immune response induction and new effector mechanisms possibly involved in protection conferred by the cuban anti-meningococcal BC vaccine. Infect Immun. (2001) 69:4502–8. doi: 10.1128/IAI.69.7.4502-4508.2001
19. Fine P, Eames K, Heymann DL. “Herd Immunity”: a rough guide. Clin Infect Dis. (2011) 52:911–6. doi: 10.1093/cid/cir007
20. Fine PE. Herd immunity: history, theory, practice. Epidemiol Rev. (1993) 15:265–302. doi: 10.1093/oxfordjournals.epirev.a036121
21. Brunham RC, Nagelkerke NJ, Plummer FA, Moses S. Estimating the basic reproductive rates of Neisseria gonorrhoeae and chlamydia trachomatis: the implications of acquired immunity. Sex Transm Dis. (1994) 21:353–6. doi: 10.1097/00007435-199411000-00010
22. Gray RT, Beagley KW, Timms P, Wilson DP. Modeling the impact of potential vaccines on epidemics of sexually transmitted chlamydia trachomatis infection. J Infect Dis. (2009) 199:1680–8. doi: 10.1086/598983
23. Craig AP, Gray RT, Edwards JL, Apicella MA, Jennings MP, Wilson DP, et al. The potential impact of vaccination on the prevalence of gonorrhea. Vaccine. (2015) 33:4520–5. doi: 10.1016/j.vaccine.2015.07.015
24. Arnold R, Galloway Y, McNicholas A, O'Hallahan J. Effectiveness of a vaccination programme for an epidemic of meningococcal B in New Zealand. Vaccine. (2011) 29:7100–6. doi: 10.1016/j.vaccine.2011.06.120
25. Tappero JW, Lagos R, Ballesteros AM, Plikaytis B, Williams D, Dykes J, et al. Immunogenicity of 2 serogroup b outer-membrane protein meningococcal vaccines: a randomized controlled trial in chile. JAMA. (1999) 281:1520–7. doi: 10.1001/jama.281.16.1520
26. The Institute of Environmental Science and Research Ltd. Sexually Transmitted Infections in New Zealand: Annual Surveillance Report 2014. Porirua, New Zealand. (2015).
27. Petousis-Harris H, Paynter J, Morgan J, Saxton P, McArdle B, Goodyear-Smith F, et al. Effectiveness of a group B outer membrane vesicle meningococcal vaccine against gonorrhoea in New Zealand: a retrospective case-control study. Lancet. (2017) 390:1603–10. doi: 10.1016/S0140-6736(17)31449-6
28. Paynter J, Goodyear-Smith F, Morgan J, Saxton P, Black S, Petousis-Harris H. Effectiveness of a group B outer membrane vesicle meningococcal vaccine in preventing hospitalization from gonorrhea in New Zealand: a retrospective cohort study. Vaccines. (2019) 7:5. doi: 10.3390/vaccines7010005
29. Comanducci M, Bambini S, Brunelli B, Adu-Bobie J, Aricò B, Capecchi B, et al. NadA, a Novel vaccine candidate of Neisseria meningitidis. J Exp Med. (2002) 195:1445–54. doi: 10.1084/jem.20020407
30. Pizza M inventor; Glaxosmithkline Biologicals SA assignee. Vaccines for Neisseria Gonorrhoeae. USA (2017).
31. Semchenko EA, Tan A, Borrow R, Seib KL. The serogroup B meningococcal vaccine Bexsero elicits antibodies to Neisseria gonorrhoeae. Clin Infect Dis. (2018). doi: 10.1093/cid/ciy1061. [Epub ahead of print].
32. Longtin J, Dion R, Simard M, Betala Belinga JF, Longtin Y, Lefebvre B, et al. Possible impact of wide-scale vaccination against serogroup B Neisseria meningitidis on gonorrhea incidence rates in one region of quebec, Canada. Open Forum Infect Dis. (2017) 4(Suppl. 1):S734–S5. doi: 10.1093/2Fofid/2Fofx180.002
33. Mehta SD, Erbelding EJ, Zenilman JM, Rompalo AM. Gonorrhoea reinfection in heterosexual STD clinic attendees: longitudinal analysis of risks for first reinfection. Sex Transm Infect. (2003) 79:124–8. doi: 10.1136/sti.79.2.124
34. Schmidt KA, Schneider H, Lindstrom JA, Boslego JW, Warren RA, Van de Verg L, et al. Experimental gonococcal urethritis and reinfection with homologous gonococci in male volunteers. Sex Transm Dis. (2001) 28:555–64. doi: 10.1097/00007435-200110000-00001
35. Plummer FA, Simonsen JN, Chubb H, Slaney L, Kimata J, Bosire M, et al. Epidemiologic evidence for the development of serovar-specific immunity after gonococcal infection. J Clin Invest. (1989) 83:1472–6. doi: 10.1172/JCI114040
36. Hobbs MM, Alcorn TM, Davis RH, Fischer W, Thomas JC, Martin I, et al. Molecular typing of Neisseria gonorrhoeae causing repeated infections: evolution of porin during passage within a community. J Infect Dis. (1999) 179:371–81. doi: 10.1086/314608
37. Plummer FA, Chubb H, Simonsen JN, Bosire M, Slaney L, Maclean I, et al. Antibody to Rmp (outer membrane protein 3) increases susceptibility to gonococcal infection. J Clin Invest. (1993) 91:339–43. doi: 10.1172/JCI116190
38. Rice PA, Vayo HE, Tam MR, Blake MS. Immunoglobulin G antibodies directed against protein III block killing of serum-resistant Neisseria gonorrhoeae by immune serum. J Exp Med. (1986) 164:1735–48. doi: 10.1084/jem.164.5.1735
39. Gotschlich EC. Development of a gonorrhoea vaccine: prospects, strategies and tactics. Bull World Health Organ. (1984) 62:671–80.
40. Greenberg L, Diena BB, Ashton FA, Wallace R, Kenny CP, Znamirowski R, et al. Gonococcal vaccine studies in Inuvik. Can J Public Health. (1974) 65:29–33.
41. Boslego JW, Tramont EC, Chung RC, McChesney DG, Ciak J, Sadoff JC, et al. Efficacy trial of a parenteral gonococcal pilus vaccine in men. Vaccine. (1991) 9:154–62. doi: 10.1016/0264-410X(91)90147-X
43. Gulati S, McQuillen DP, Mandrell RE, Jani DB, Rice PA. Immunogenicity of Neisseria gonorrhoeae lipooligosaccharide epitope 2C7, widely expressed in vivo with no immunochemical similarity to human glycosphingolipids. J Infect Dis. (1996) 174:1223–37. doi: 10.1093/infdis/174.6.1223
44. Gulati S, Zheng B, Reed GW, Su X, Cox AD, St Michael F, et al. Immunization against a saccharide epitope accelerates clearance of experimental gonococcal infection. PLoS Pathog. (2013) 9:e1003559. doi: 10.1371/journal.ppat.1003559
45. Liu Y, Hammer LA, Liu W, Hobbs MM, Zielke RA, Sikora AE, et al. Experimental vaccine induces Th1-driven immune responses and resistance to Neisseria gonorrhoeae infection in a murine model. Mucosal Immunol. (2017) 10:1594–608. doi: 10.1038/mi.2017.11
46. Plante M, Jerse A, Hamel J, Couture F, Rioux CR, Brodeur BR, et al. Intranasal immunization with gonococcal outer membrane preparations reduces the duration of vaginal colonization of mice by Neisseria gonorrhoeae. J Infect Dis. (2000) 182:848–55. doi: 10.1086/315801
47. Zhu W, Thomas CE, Chen CJ, Van Dam CN, Johnston RE, Davis NL, et al. Comparison of immune responses to gonococcal PorB delivered as outer membrane vesicles, recombinant protein, or Venezuelan equine encephalitis virus replicon particles. Infect Immun. (2005) 73:7558–68. doi: 10.1128/IAI.73.11.7558-7568.2005
48. Giuliani MM, Adu-Bobie J, Comanducci M, Aricò B, Savino S, Santini L, et al. A universal vaccine for serogroup B meningococcus. Proc Natl Acad Sci USA. (2006) 103:10834–9. doi: 10.1073/pnas.0603940103
49. Snape MD, Dawson T, Oster P, Evans A, John TM, Ohene-Kena B, et al. Immunogenicity of two investigational serogroup B meningococcal vaccines in the first year of life: a randomized comparative trial. Pediatr Infect Dis J. (2010) 29:e71–9. doi: 10.1097/INF.0b013e3181f59f6d
50. Findlow J, Borrow R, Snape MD, Dawson T, Holland A, John TM, et al. Multicenter, open-label, randomized phase II controlled trial of an investigational recombinant Meningococcal serogroup B vaccine with and without outer membrane vesicles, administered in infancy. Clin Infect Dis. (2010) 51:1127–37. doi: 10.1086/656741
51. Vipond C, Suker J, Jones C, Tang C, Feavers IM, Wheeler JX. Proteomic analysis of a meningococcal outer membrane vesicle vaccine prepared from the group B strain NZ98/254. Proteomics. (2006) 6:3400–13. doi: 10.1002/pmic.200500821
52. Holst J, Martin D, Arnold R, Huergo CC, Oster P, O'Hallahan J, et al. Properties and clinical performance of vaccines containing outer membrane vesicles from Neisseria meningitidis. Vaccine. (2009) 27(Suppl. 2):B3–12. doi: 10.1016/j.vaccine.2009.04.071
53. Williams JN, Weynants V, Poolman JT, Heckels JE, Christodoulides M. Immuno-proteomic analysis of human immune responses to experimental Neisseria meningitidis outer membrane vesicle vaccines identifies potential cross-reactive antigens. Vaccine. (2014) 32:1280–6. doi: 10.1016/j.vaccine.2013.12.070
54. Zielke RA, Wierzbicki IH, Weber JV, Gafken PR, Sikora AE. Quantitative proteomics of the Neisseria gonorrhoeae cell envelope and membrane vesicles for the discovery of potential therapeutic targets. Mol Cell Proteom. (2014) 13:1299–317. doi: 10.1074/mcp.M113.029538
55. Jerse AE. Experimental gonococcal genital tract infection and opacity protein expression in estradiol-treated mice. Infect Immun. (1999) 67:5699–708.
56. Sarantis H, Gray-Owen SD. Defining the roles of human carcinoembryonic antigen-related cellular adhesion molecules during neutrophil responses to Neisseria gonorrhoeae. Infect Immun. (2012) 80:345–58. doi: 10.1128/IAI.05702-11
57. Sadarangani M, Pollard AJ, Gray-Owen SD. Opa proteins and CEACAMs: pathways of immune engagement for pathogenic Neisseria. FEMS Microbiol Rev. (2011) 35:498–514. doi: 10.1111/j.1574-6976.2010.00260.x
58. Hedges SR, Sibley DA, Mayo MS, Hook EW III, Russell MW. Cytokine and antibody responses in women infected with Neisseria gonorrhoeae: effects of concomitant infections. J Infect Dis. (1998) 178:742–51. doi: 10.1086/515372
59. Ramsey KH, Schneider H, Cross AS, Boslego JW, Hoover DL, Staley TL, et al. Inflammatory cytokines produced in response to experimental human gonorrhea. J Infect Dis. (1995) 172:186–91. doi: 10.1093/infdis/172.1.186
60. Feinen B, Jerse AE, Gaffen SL, Russell MW. Critical role of Th17 responses in a murine model of Neisseria gonorrhoeae genital infection. Mucosal Immunol. (2010) 3:312–21. doi: 10.1038/mi.2009.139
61. Liu Y, Islam EA, Jarvis GA, Gray-Owen SD, Russell MW. Neisseria gonorrhoeae selectively suppresses the development of Th1 and Th2 cells, and enhances Th17 cell responses, through TGF-beta-dependent mechanisms. Mucosal Immunol. (2012) 5:320–31. doi: 10.1038/mi.2012.12
62. Liu Y, Liu W, Russell MW. Suppression of host adaptive immune responses by Neisseria gonorrhoeae: role of interleukin 10 and type 1 regulatory T cells. Mucosal Immunol. (2014) 7:165–76. doi: 10.1038/mi.2013.36
63. Liu Y, Russell MW. Diversion of the immune response to Neisseria gonorrhoeae from Th17 to Th1/Th2 by treatment with anti-transforming growth factor beta antibody generates immunological memory and protective immunity. mBio. (2011) 2:e00095–11. doi: 10.1128/mBio.00095-11
64. Russell MW, Mestecky J. Humoral immune responses to microbial infections in the genital tract. Microbes Infect Institut Pasteur. (2002) 4:667–77. doi: 10.1016/S1286-4579(02)01585
65. Su F, Patel GB, Hu S, Chen W. Induction of mucosal immunity through systemic immunization: Phantom or reality? Hum Vaccin Immunother. (2016) 12:1070–9. doi: 10.1080/21645515.2015.1114195
66. Clements JD, Freytag LC. Parenteral vaccination can be an effective means of inducing protective mucosal responses. Clin Vaccine Immunol. (2016) 23:438–41. doi: 10.1128/CVI.00214-16
67. Petaja T, Pedersen C, Poder A, Strauss G, Catteau G, Thomas F, et al. Long-term persistence of systemic and mucosal immune response to HPV-16/18 AS04-adjuvanted vaccine in preteen/adolescent girls and young women. Int J Cancer. (2011) 129:2147–57. doi: 10.1002/ijc.25887
68. Loring BJ, Turner N, Petousis-Harris H. MeNZB vaccine and epidemic control: when do you stop vaccinating? Vaccine. (2008) 26:5899–904. doi: 10.1016/j.vaccine.2008.08.062
69. Findlow J, Taylor S, Aase A, Horton R, Heyderman R, Southern J, et al. Comparison and correlation of Neisseria meningitidis serogroup B immunologic assay results and human antibody responses following three doses of the Norwegian meningococcal outer membrane vesicle vaccine MenBvac. Infect Immun. (2006) 74:4557–65. doi: 10.1128/IAI.00466-06
70. Davenport V, Groves E, Horton RE, Hobbs CG, Guthrie T, Findlow J, et al. Mucosal immunity in healthy adults after parenteral vaccination with outer-membrane vesicles from Neisseria meningitidis serogroup B. J Infect Dis. (2008) 198:731–40. doi: 10.1086/590669
71. Horton RE, Stuart J, Christensen H, Borrow R, Guthrie T, Davenport V, et al. Influence of age and carriage status on salivary IgA to Neisseria meningitidis. Epidemiol Infect. (2005) 133:883–9. doi: 10.1017/S0950268805004097
72. Kaparakis-Liaskos M, Ferrero RL. Immune modulation by bacterial outer membrane vesicles. Nat Rev Immunol. (2015) 15:375–87. doi: 10.1038/nri3837
73. Tani C, Stella M, Donnarumma D, Biagini M, Parente P, Vadi A, et al. Quantification by LC-MS(E) of outer membrane vesicle proteins of the Bexsero(R) vaccine. Vaccine. (2014) 32:1273–9. doi: 10.1016/j.vaccine.2014.01.011
74. Frederick DR, Goggins JA, Sabbagh LM, Freytag LC, Clements JD, McLachlan JB. Adjuvant selection regulates gut migration and phenotypic diversity of antigen-specific CD4(+) T cells following parenteral immunization. Mucosal Immunol. (2018) 11:549–61. doi: 10.1038/mi.2017.70
75. Buonaguro L, Pulendran B. Immunogenomics and systems biology of vaccines. Immunol Rev. (2011) 239:197–208. doi: 10.1111/j.1600-065X.2010.00971.x
76. Marri PR, Paniscus M, Weyand NJ, Rendon MA, Calton CM, Hernandez DR, et al. Genome sequencing reveals widespread virulence gene exchange among human Neisseria species. PLoS ONE. (2010) 5:e11835. doi: 10.1371/journal.pone.0011835
77. Troncoso G, Sanchez S, Moreda M, Criado MT, Ferreiros CM. Antigenic cross-reactivity between outer membrane proteins of Neisseria meningitidis and commensal Neisseria species. FEMS Immunol Med Microbiol. (2000) 27:103–9. doi: 10.1111/j.1574-695X.2000.tb01419.x
78. Petousis-Harris H. Impact of meningococcal group B OMV vaccines, beyond their brief. Hum Vaccin Immunother. (2018) 14:1058–63. doi: 10.1080/21645515.2017.1381810
79. Muzzi A, Mora M, Pizza M, Rappuoli R, Donati C. Conservation of meningococcal antigens in the genus Neisseria. mBio. (2013) 4:e00163–13. doi: 10.1128/mBio.00163-13
80. Hadad R, Jacobsson S, Pizza M, Rappuoli R, Fredlund H, Olcen P, et al. Novel meningococcal 4CMenB vaccine antigens - prevalence and polymorphisms of the encoding genes in Neisseria gonorrhoeae. APMIS. (2012) 120:750–60. doi: 10.1111/j.1600-0463.2012.02903.x
81. Thornton V, Lennon D, Rasanathan K, O'Hallahan J, Oster P, Stewart J, et al. Safety and immunogenicity of New Zealand strain meningococcal serogroup B OMV vaccine in healthy adults: beginning of epidemic control. Vaccine. (2006) 24:1395–400. doi: 10.1016/j.vaccine.2005.09.043
82. Hosking J, Rasanathan K, Mow FC, Jackson C, Martin D, O'Hallahan J, et al. Immunogenicity, reactogenicity, and safety of a P1.7b,4 strain-specific serogroup B meningococcal vaccine given to preteens. Clin Vaccine Immunol. (2007) 14:1393–9. doi: 10.1128/CVI.00167-07
83. Oster P, O'Hallahan J, Aaberge I, Tilman S, Ypma E, Martin D. Immunogenicity and safety of a strain-specific MenB OMV vaccine delivered to under 5-year olds in New Zealand. Vaccine. (2007) 25:3075–9. doi: 10.1016/j.vaccine.2007.01.023
84. Holst J, Feiring B, Fuglesang JE, Hoiby EA, Nokleby H, Aaberge IS, et al. Serum bactericidal activity correlates with the vaccine efficacy of outer membrane vesicle vaccines against Neisseria meningitidis serogroup B disease. Vaccine. (2003) 21:734–7. doi: 10.1016/S0264-410X(02)00591-1
85. Borrow R, Balmer P, Miller E. Meningococcal surrogates of protection–serum bactericidal antibody activity. Vaccine. (2005) 23:2222–7. doi: 10.1016/j.vaccine.2005.01.051
86. Frasch CE, Borrow R, Donnelly J. Bactericidal antibody is the immunologic surrogate of protection against meningococcal disease. Vaccine. (2009) 27(Suppl. 2):B112–6. doi: 10.1016/j.vaccine.2009.04.065
87. Li G, Jiao H, Jiang G, Wang J, Zhu L, Xie R, et al. Neisseria gonorrhoeae NspA induces specific bactericidal and opsonic antibodies in mice. Clin Vaccine Immunol. (2011) 18:1817–22. doi: 10.1128/CVI.05245-11
88. Semchenko EA, Day CJ, Seib KL. MetQ of Neisseria gonorrhoeae is a surface-expressed antigen that elicits bactericidal and functional blocking antibodies. Infect Immun. (2017) 85:1–17. doi: 10.1128/IAI.00898-16
89. Tramont EC, Sadoff JC, Artenstein MS. Cross-reactivity of Neisseria gonorrhoeae and Neisseria meningitidis and the nature of antigens involved in the bactericidal reaction. J Infect Dis. (1974) 130:240–7. doi: 10.1093/infdis/130.3.240
90. Rice PA. Molecular basis for serum resistance in Neisseria gonorrhoeae. Clin Microbiol Rev. (1989) 2(Suppl):S112–7.
91. Wetzler LM, Barry K, Blake MS, Gotschlich EC. Gonococcal lipooligosaccharide sialylation prevents complement-dependent killing by immune sera. Infect Immun. (1992) 60:39–43.
92. Ram S, Sharma AK, Simpson SD, Gulati S, McQuillen DP, Pangburn MK, et al. A novel sialic acid binding site on factor H mediates serum resistance of sialylated Neisseria gonorrhoeae. J Exp Med. (1998) 187:743–52. doi: 10.1084/jem.187.5.743
93. Lewis LA, Carter M, Ram S. The relative roles of factor H binding protein, neisserial surface protein A, and lipooligosaccharide sialylation in regulation of the alternative pathway of complement on meningococci. J Immunol. (2012) 188:5063–72. doi: 10.4049/jimmunol.1103748
94. Pantelic M, Chen I, Parker J, Zhang P, Grunert F, Chen T. Retinoic acid treated HL60 cells express CEACAM1 (CD66a) and phagocytose Neisseria gonorrhoeae. FEMS Immunol Med Microbiol. (2004) 42:261–6. doi: 10.1016/j.femsim.2004.05.010
95. Shaw JH, Falkow S. Model for invasion of human tissue culture cells by Neisseria gonorrhoeae. Infect Immun. (1988) 56:1625–32.
96. Jarvis GA, Li J, Swanson KV. Invasion of human mucosal epithelial cells by Neisseria gonorrhoeae upregulates expression of intercellular adhesion molecule 1 (ICAM-1). Infect Immun. (1999) 67:1149–56.
97. Christodoulides M, Everson JS, Liu BL, Lambden PR, Watt PJ, Thomas EJ, et al. Interaction of primary human endometrial cells with Neisseria gonorrhoeae expressing green fluorescent protein. Mol Microbiol. (2000) 35:32–43. doi: 10.1046/j.1365-2958.2000.01694.x
98. Christodoulides M. Neisseria proteomics for antigen discovery and vaccine development. Exp Rev Proteom. (2014) 11:573–91. doi: 10.1586/14789450.2014.938640
99. Williams JN, Christodoulides M, Heckels JE. Analysis of the immune response to Neisseria meningitidis using a proteomics approach. Methods Mol Biol. (2012) 799:343–60. doi: 10.1007/978-1-61779-346-2_20
100. Oftung F, Korsvold GE, Aase A, Naess LM. Cellular immune responses in humans induced by two serogroup B meningococcal outer membrane vesicle vaccines given separately and in combination. Clin Vaccine Immunol. (2016) 23:353–62. doi: 10.1128/CVI.00666-15
101. van der Voort ER, van Dijken H, Kuipers B, van der Biezen J, van der Ley P, Meylis J, et al. Human B- and T-cell responses after immunization with a hexavalent PorA meningococcal outer membrane vesicle vaccine. Infect Immun. (1997) 65:5184–90.
102. Ladhani SN, Giuliani MM, Biolchi A, Pizza M, Beebeejaun K, Lucidarme J, et al. Effectiveness of Meningococcal B Vaccine against Endemic Hypervirulent Neisseria meningitidis W Strain, England. Emerg Infect Dis. (2016) 22:309–11. doi: 10.3201/eid2202.150369
103. Wilkins A, Snape M. Emerging clinical experience with vaccines against group B meningococcal disease. Vaccine. (2017) 36:5470–6. doi: 10.1016/j.vaccine.2017.07.056
104. Marshall HS, McMillan M, Koehler A, Lawrence A, MacLennan JM, Maiden MC, et al. B Part of it protocol: a cluster randomised controlled trial to assess the impact of 4CMenB vaccine on pharyngeal carriage of Neisseria meningitidis in adolescents. BMJ Open. (2018) 8:e020988. doi: 10.1136/bmjopen-2017-020988
105. Larson HJ, Jarrett C, Eckersberger E, Smith DM, Paterson P. Understanding vaccine hesitancy around vaccines and vaccination from a global perspective: a systematic review of published literature, 2007–2012. Vaccine. (2014) 32:2150–9. doi: 10.1016/j.vaccine.2014.01.081
106. Kata A. A postmodern Pandora's box: anti-vaccination misinformation on the internet. Vaccine. (2010) 28:1709–16. doi: 10.1016/j.vaccine.2009.12.022
107. Broniatowski DA, Jamison AM, Qi S, AlKulaib L, Chen T, Benton A, et al. Weaponized health communication: twitter bots and Russian trolls amplify the vaccine debate. Am J Public Health. (2018) 108:1378–84. doi: 10.2105/2FAJPH.2018.304567T
108. Larson HJ. Politics and public trust shape vaccine risk perceptions. Nat Hum Behav. (2018) 2:316. doi: 10.1038/s41562-018-0331-6
109. Dube E, Vivion M, MacDonald NE. Vaccine hesitancy, vaccine refusal and the anti-vaccine movement: influence, impact and implications. Expert Rev Vacc. (2015) 14:99–117. doi: 10.1586/14760584.2015.964212
110. Wise J. European countries are urged to carry out catch-up campaigns as measles outbreaks continue. BMJ. (2018) 361:k1771. doi: 10.1136/bmj.k1771
111. Saslow D, Castle PE, Cox JT, Davey DD, Einstein MH, Ferris DG, et al. American Cancer Society Guideline for human papillomavirus (HPV) vaccine use to prevent cervical cancer and its precursors. CA Cancer J Clin. (2007) 57:7–28. doi: 10.3322/canjclin.57.1.7
112. Rothman SM, Rothman DJ. Marketing HPV vaccine: implications for adolescent health and medical professionalism. JAMA. (2009) 302:781–6. doi: 10.1001/jama.2009.1179
113. Lippman A, Melnychuk R, Shimmin C, Boscoe M. Human papillomavirus, vaccines and women's health: questions and cautions. Can Med Assoc J. (2007) 177:484–7. doi: 10.1503/cmaj.070944
114. Coombes R. Life saving treatment or giant experiment? BMJ. (2007) 334:721–3. doi: 10.1136/bmj.39164.510127.AD
115. Markowitz LE, Tsu V, Deeks SL, Cubie H, Wang SA, Vicari AS, et al. Human papillomavirus vaccine introduction–the first five years. Vaccine. (2012) 30:F139–48. doi: 10.1016/j.vaccine.2012.05.039
116. Velan B, Yadgar Y. On the implications of desexualizing vaccines against sexually transmitted diseases: health policy challenges in a multicultural society. Israel J Health Policy Res. (2017) 6:30. doi: 10.1186/s13584-017-0153-4
117. Brotherton JM, Winch KL, Bicknell L, Chappell G, Saville M. HPV vaccine coverage is increasing in Australia. Med J Austr. (2017) 206:262. doi: 10.5694/mja16.00958
118. Dunn AG, Surian D, Leask J, Dey A, Mandl KD, Coiera E. Mapping information exposure on social media to explain differences in HPV vaccine coverage in the United States. Vaccine. (2017) 35:3033–40. doi: 10.1016/j.vaccine.2017.04.060
119. Nogueira-Rodrigues A, Bukowski A, Paulino E St, Louis J, Barrichello A, Sternberg C, et al. An alert to Latin America: current human papillomavirus vaccination trends highlight key barriers to successful implementation. Cancer. (2017) 123:2193–9. doi: 10.1002/cncr.30647
Keywords: gonorrhea, OMV vaccine, MeNZB, Neisseria meningitides, Neisseria gonorrhoeae
Citation: Petousis-Harris H and Radcliff FJ (2019) Exploitation of Neisseria meningitidis Group B OMV Vaccines Against N. gonorrhoeae to Inform the Development and Deployment of Effective Gonorrhea Vaccines. Front. Immunol. 10:683. doi: 10.3389/fimmu.2019.00683
Received: 31 August 2018; Accepted: 13 March 2019;
Published: 09 April 2019.
Edited by:
Michael W. Russell, University at Buffalo, United StatesReviewed by:
Marcia Metzgar Hobbs, University of North Carolina at Chapel Hill, United StatesMargaret Bash, United States Food and Drug Administration, United States
Copyright © 2019 Petousis-Harris and Radcliff. This is an open-access article distributed under the terms of the Creative Commons Attribution License (CC BY). The use, distribution or reproduction in other forums is permitted, provided the original author(s) and the copyright owner(s) are credited and that the original publication in this journal is cited, in accordance with accepted academic practice. No use, distribution or reproduction is permitted which does not comply with these terms.
*Correspondence: Helen Petousis-Harris, h.petousis-harris@auckland.ac.nz
†These authors have contributed equally to this work