- 1Department of Neurology, University of Ulm, Ulm, Germany
- 2Institute of Epidemiology and Medical Biometry, Ulm University, Ulm, Germany
- 3Specialty Hospital of Neurology Dietenbronn, Schwendi, Germany
Objectives: The importance of immunoglobulin G (IgG) oligoclonal bands (OCB) in the diagnosis of multiple sclerosis (MS) was reaffirmed again in the recently revised MS diagnostic criteria. Since OCB testing is based on non-quantitative techniques and demands considerable methodological experience, measurement of CSF immunoglobulin free light chains (FLC) has been suggested as quantitative alternative to OCB. We aimed to establish reference values for FLC measures and evaluate their diagnostic accuracy with regard to the diagnosis of MS.
Methods: Immunoglobulin kappa (KFLC) and lambda (LFLC) free light chains were prospectively measured by nephelometry in CSF and serum sample pairs in 1,224 patients. The analyzed cohort included patients with MS, other autoimmune or infectious inflammatory diseases of the nervous system as well as 989 patients without signs for nervous system inflammation.
Results: Regarding diagnosis of MS, the diagnostic sensitivity and specificity of intrathecal KFLC ratio were 93.3 and 93.7% using the CSF-serum albumin ratio-dependent reference values, 92.0 and 95.9% for intrathecal KFLC ratio applying the ROC-curve determined cut-off levels, 62.7 and 98.3% for IgG index, 64.0 and 98.8% for intrathecal IgG synthesis according to Reiber diagrams, and 94.7 and 93.3% for OCB. Diagnostic sensitivity and specificity of intrathecal LFLC were clearly lower than KFLC.
Conclusions: Intrathecal KFLC and OCB showed the highest diagnostic sensitivities for MS. However, specificity was slightly lower compared to other quantitative IgG parameters. Consequently, CSF FLC may not replace OCB, but it may support diagnosis in MS as a quantitative parameter.
Introduction
Multiple sclerosis (MS) is a chronic inflammatory demyelinating disease of the central nervous system (CNS) affecting predominantly young adults and leading to neurological disability (1–3). CSF investigation is indispensable in the diagnostic process of MS and the detection of immunoglobulin G (IgG) oligoclonal bands (OCB) again gained more importance in the recently revised MS diagnostic criteria (4). So far, OCB are the most widely used CSF test to support or rule out the diagnosis of MS (5–7). Furthermore, OCB offer prognostic information concerning the development of MS after a first clinical suggestive event, known as clinically isolated syndrome (CIS) (8, 9). In these cases, detection of OCB has prognostic relevance and can help to identify patients with a high risk of future relapses. However, determination of OCB using isoelectric focusing (IEF) on gels followed by immunoblotting demands considerable methodological expertise and is both labor-intensive and difficult to standardize (10). Human IgG molecules contain two identical heavy chains and two identical light chains, which exist either as kappa or lambda isotypes and are linked to the heavy chains by covalent and non-covalent bonds (11, 12). During the production of intact immunoglobulins, B cells produce an excess of kappa and lambda light chains, which are secreted as free light chains (FLC) (i.e., not bound to heavy chains within an Ig) (13). These FLC can exist as monomers (22–27 kDa, usually kappa) or dimers (44–55 kDa, usually lambda) (14), and can be detected in many biological fluids including serum, urine, synovial fluid as well as, in the CSF (15, 16).
Several studies have indicated that elevated immunoglobulin kappa (KFLC) and lambda (LFLC) free light chains in the CSF may represent a quantitative tool to demonstrate intrathecal IgG synthesis and thereby support the diagnosis of MS (17–28), some even proposing FLC quantification as an alternative to OCB analysis (29, 30). However, diverse methods, both qualitative, e.g., IEF with immunoblotting (31), and quantitative, e.g., radioimmunoassay (24), ELISA (18), and nephelometry (20–22), have been applied for FLC determination. In addition, divergent approaches to calculate intrathecal FLC synthesis were employed, e.g., FLC CSF-serum ratios, CSF KFLC to LFLC ratio, and FLC index. In summary, comparability between the published studies is limited due to different methodologies, lack of appropriate disease controls (usually non-inflammatory neurological cases were used as controls with a lack of other autoimmune CNS diseases than MS), and finally rarity of prospective data.
The aim of the present study was (i) to prospectively measure FLC (both KFLC and LFLC) in CSF and serum by nephelometry in a large cohort, (ii) to establish reference values for FLC as a function of the blood-CSF barrier function based on patients without any clinical and laboratory signs for nervous system inflammation, and (iii) to compare the diagnostic value of different previously proposed methods to calculate intrathecal FLC synthesis, e.g., CSF-serum ratio of FLC (Q FLC), FLC index (Q FLC/Q Albumin), CSF KFLC-LFLC ratio, with well-proven indicators of intrathecal IgG synthesis (OCB and IgG Index) within the same cohort.
Methods
Patients
Cross-sectional data of CSF and serum sample pairs of 1,224 patients from the Department of Neurology, University of Ulm (Germany) were investigated prospectively over a period of 18 months.
The analyzed cohort included 75 patients with multiple sclerosis (MS), diagnosed according to the modified McDonald criteria (32), five with clinically isolated syndrome (CIS), 36 patients with other autoimmune CNS diseases (AI-CNS-D), 13 with chronic inflammatory demyelinating polyneuropathy (CIDP), 13 with Guillain-Barré syndrome (GBS), 29 with viral and bacterial CNS infection (CNS-I), seven with CNS tumor, 38 with post-infectious CSF syndrome (P-CNS-I), five with metabolic encephalopathy (ME), 14 with paraproteinemic neuropathy and/or neuropathy with monoclonal gammopathy of unknown significance (PP-PNP), and 989 patients without any signs of nervous system inflammation (no signs of inflammation in CSF, no clinical signs of inflammation, no signs of blood contamination in CSF, and no evidence of haemorrhagic or inflammatory lesion in cerebral MRI, NIND) (Table 1). Lumbar puncture was performed as part of the routine diagnostic work-up. All samples were handled and stored in accordance with BioMS guidelines (33).
Determination of OCB
OCB were detected by isoelectric focusing (IEF) on polyacrylamide gels followed by immunoblotting using an IgG-specific antibody staining. Paired CSF and serum samples were adjusted for IgG concentrations and analyzed on the same gel run as we previously described in detail (34). OCB were evaluated by at least two long-standing experienced technicians and at least two board-certified neurologists with extensive experience in the field of CSF analysis. Two or more IgG bands restricted to the CSF were rated as positive OCBs.
Determination of KFLC and LFLC
Immunoglobulin kappa and lambda free light chains (KFLC and LFLC) were measured by nephelometry (Siemens N Latex FLC kappa and lambda assays on Siemens BN ProSpec®) according to the instructions supplied by the manufacturer. The detection antibodies in these assays are monoclonal. All samples were analyzed within the same working day together with other CSF measurements according to the sampling protocol of BioMS (33).
We calculated the CSF-serum ratio of albumin (Q Alb), KFLC (Q KFLC), and LFLC (Q LFLC) and determined the KFLC index (Q KFLC/Q Alb) and LFLC index (Q KFLC/Q Alb) by correcting for Q Alb. In addition, the CSF KFLC to LFLC ratio (CSF KFLC/CSF LFLC) was calculated.
CSF leukocyte count (cells/μl), CSF total protein (g/L), CSF lactate (mmol/L), the albumin CSF-serum concentration ratio (Q Alb), CSF and serum immunoglobulin G, A, and M levels were obtained as previously described (35, 36).
MRI Analysis
MRI scans of the brain and spinal cord were performed on a 1.5 tesla whole-body MRI (Symphony Siemens, Erlangen, Germany) according to a previously fixed protocol including T1-weighted spin-echo (SE) axial slices with and without application of gadolinium-DTPA as well as T2-weighted SE axial slices.
Statistical Analysis
Statistical analysis and the graphical representation of the data was performed using SPSS (version 24.0; SPSS Inc., Chicago, IL, USA), GraphPad Prism (version 6.0, GraphPad Software, San Diego, CA, USA), and R software (version 3.4.0). Differences of FLC levels between two disease groups were analyzed using the non-parametric Mann-Whitney U-test due to skewed data. The correlation between two parameters was analyzed using Spearman rank order correlation. P-values below 0.05 were considered to be significant.
FLC reference values dependent on Q Alb were estimated by linear quantile regression (37, 38) using the R package quantreg and plotted on the log scale. Receiver Operating Characteristic (ROC) curve analysis, calculating the area under the ROC curve (AUROCC), was used to determine the diagnostic accuracy of FLC values as diagnosis markers for MS. The Youden index (sensitivity+specificity-1) was calculated for a range of cut-off values to find for each FLC value the optimal value with the highest discriminatory accuracy (39).
Sensitivity was calculated as [true-positive/[true-positive + false-negative]], specificity was calculated as [true-negative/[true-negative + false-positive]]. The positive predictive value (PPV) was calculated as [true-positive/[true-positive + false-positive]], and the negative predictive value (NPV) as [true-negative/[true-negative + false-negative]]. For all diagnostic values the exact 95% confidence intervals were given (40).
Results
Clinical Findings and Main CSF Examination Results
A total of 1,224 CSF-serum pairs from 1,224 patients were included in this study. Demographic, clinical and main CSF characteristics are summarized in Table 1.
FLC in Non-inflammatory Neurological Diseases—Establishment of Reference Values
In NIND (n = 989), CSF FLC levels correlated significantly with serum FLC levels (KFLC: p < 0.001, r = 0.744; LFLC: p < 0.001, r = 0.737) as well as with the respective Q Alb (KFLC: p < 0.001, r = 0.638; LFLC: p < 0.001, r = 0.653) (Figure 1).
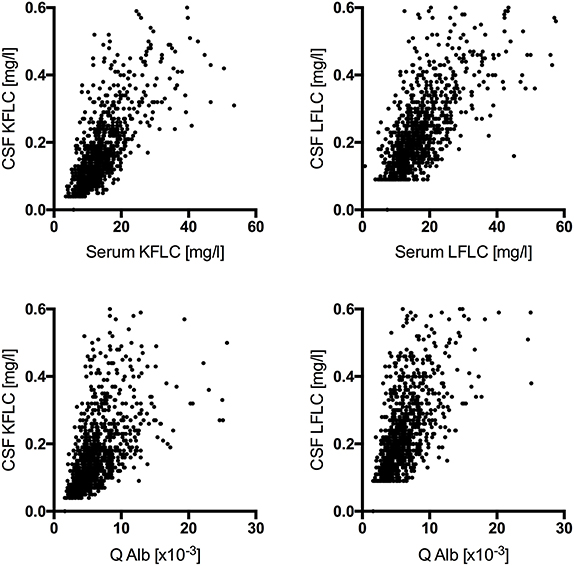
Figure 1. Correlation among cerebrospinal fluid free light chain levels in non-inflammatory neurologic diseases (n = 989) with the respective serum levels (KFLC: p < 0.001, r = 0.744; LFLC: p < 0.001, r = 0.737) and the respective CSF-serum ratio of albumin (Q Alb) (KFLC: p < 0.001, r = 0.638; LFLC: p < 0.001, r = 0.653) are shown.
To establish blood-CSF barrier related reference values for Q FLC, the Q FLC of all NIND was plotted against the respective Q Alb on the log scale. The 99% quantile estimated by linear quantile regression was indicated as the upper reference value of the respective Q FLC (Figure 2). This resulted in the following equations for the Q Alb-dependent upper reference values:
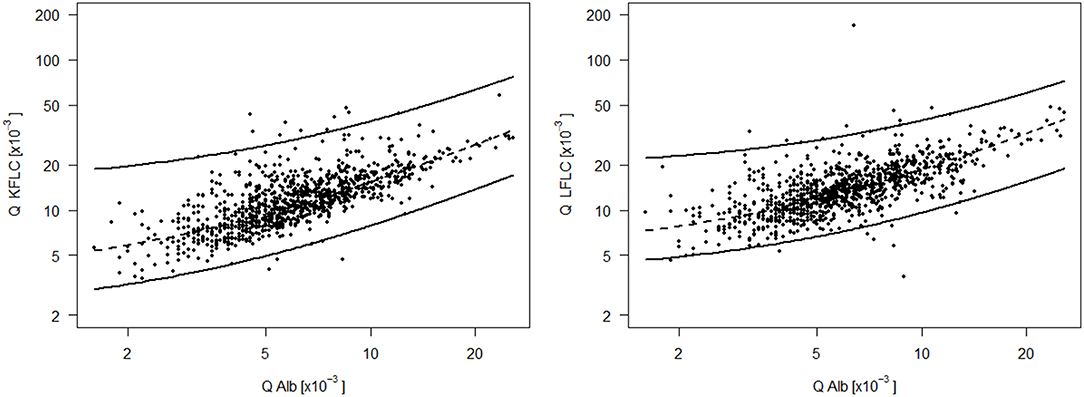
Figure 2. CSF-serum ratio of kappa (Q KFLC) and lambda (Q LFLC) free light chain is plotted against the respective CSF-serum ratio of albumin (Q Alb) on the log scale. The upper line is the 99% quantile estimated by linear quantile regression and indicates the upper reference value of Q KFLC and Q LFLC dependent on Q Alb and based on a control group of 989 non-inflammatory neurologic diseases and a range of Q Alb from 1.6*10−3 to 25.7*10−3. Formula for the Q Alb dependent upper reference value (upper line): Q KFLC = 9.50 + 2.08*Q Alb; Q LFLC = 16.37 + 1.36*Q Alb. The lower line is the 1% quantile line and the dashed line in the middle is the 50% quantile (median) line.
FLC in CSF and Serum—Group Differences
In the 75 MS patients, Q KFLC was positive (i.e., above Q Alb-dependent reference value described in Figure 2) in 70 (93.3%) and Q LFLC in 53 (70.7%) (Figure 3). OCB were detected in 71 of 75 patients (94.7%) (Table 1) and IgG-index was elevated (>0.7) in 47 (62.7%) and intrathecal IgG synthesis according to Reiber was found in 48 (64%). One of the four OCB negative MS patients showed positive Q KFLC, and two of the five patients with normal Q KFLC showed positive OCB. Thus, either increased Q KFLC or CSF OCB or both were found in 72/75 (96%) of the patients with MS. Q KFLC was significantly more often elevated than the IgG index (p < 0.0001) and Q IgG according to Reiber (p < 0.0001).
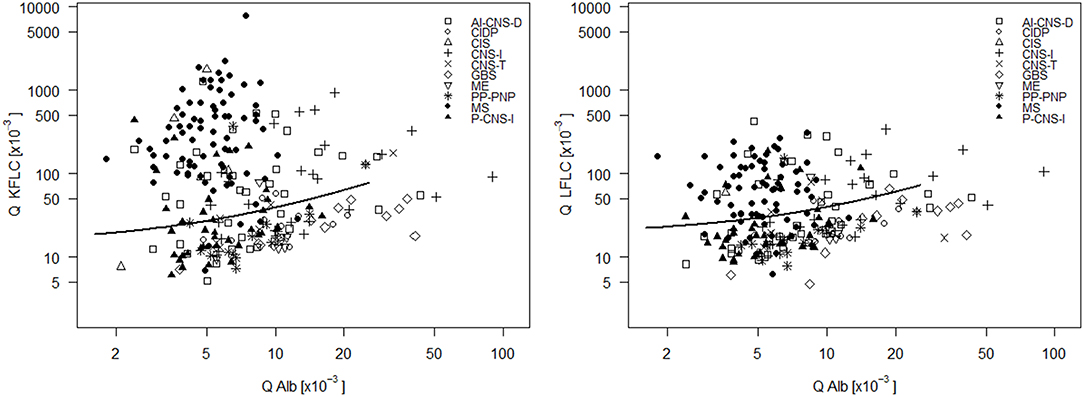
Figure 3. CSF- serum ratio of kappa free light chain (Q KFLC) (left) and lambda free light chain (Q LFLC) (right) is plotted against CSF-serum ratio of albumin (Q Alb). Solid line indicates the upper reference value introduced in Figure 2. AI-CNS-D, other autoimmune disease of the central nervous system; CIDP, chronic inflammatory demyelinating polyneuropathy; CIS, clinically isolated syndrome; CNS-I, viral and bacterial CNS infection; GBS, Guillain-Barré syndrome; ME, metabolic encephalopathy; MS, multiple sclerosis; PP-PNP, paraproteinemic neuropathy and/or neuropathy with monoclonal gammopathy of unknown significance; P-CNS-I, post-infectious CSF syndrome.
Q KFLC was higher than the Q Alb-dependent upper reference value in 55.6% of patients with AI-CNS-D, in 80.0% of patients with CIS, in 23.1% of patients with CIDP, in 0% of patients with GBS, in 51.7% of patients with CNS-I, in 42.9% of patients with CNS tumor, in 36.8% patients with P-CNS-I, in 20.0% of patients with ME, in 21.4% of patients with PP-PNP, and in 0.9% of patients with NIND.
In comparison, Q LFLC was higher than the Q Alb-dependent upper reference value in 36.1% of patients with AI-CNS-D, in 40.0% of patients with CIS, in 7.7% of patients with CIDP, in 7.7% of patients with GBS, in 37.9% of patients with CNS-I, in 42.9% of patients with CNS tumor, in 21.1% patients with P-CNS-I, in 20.0% of patients with ME, in 7.1% of patients with PP-PNP, and in 0.9% of patients with NIND.
Patients with MS showed significantly elevated CSF KFLC levels as compared with all other groups except for CIS and CSF LFLC levels were also elevated significantly as compared with NIND, PP-PNP, P-CNS-I, and CIS (Figure 4). CSF KFLC and CSF LFLC were also elevated significantly in other disease groups as compared with NIND. In contrast, serum KFLC and serum LFLC were significantly lower in cases of MS (p < 0.001) but showed otherwise no significant difference as compared with NIND.
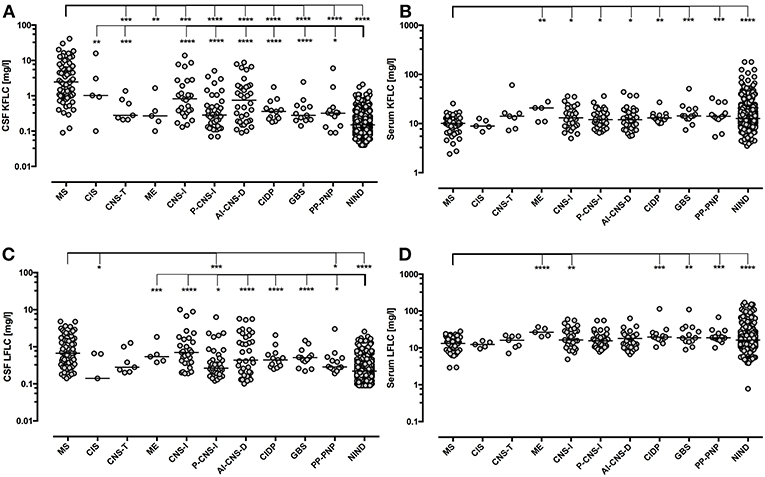
Figure 4. Immunoglobulin Free light chain levels in neurological diseases. Horizontal solid line indicates median. All groups have been compared to NIND and MS. Significant P-values for pairwise comparisons (Mann-Whitney U-test) are displayed. ****p < 0.0001, ***p < 0.001, **p < 0.01, *p < 0.05. AI-CNS-D, other autoimmune disease of the central nervous system; CIDP, chronic inflammatory demyelinating polyneuropathy; CIS, clinically isolated syndrome; CNS-I, viral and bacterial CNS infection; CSF, cerebrospinal fluid; GBS, Guillain-Barré syndrome; ME, metabolic encephalopathy; MS, multiple sclerosis; PP-PNP, paraproteinemic neuropathy and/or neuropathy with monoclonal gammopathy of unknown significance; NIND, non-inflammatory neurological diseases; P-CNS-I, post-infectious CSF syndrome. CSF (A) and serum (B) levels of KFLC as well as CSF (C) and serum (D) levels of LFLC are displayed.
Diagnostic Accuracy of Different FLC Values, OCB, and IgG-Index for the Diagnosis of MS
ROC analysis was performed to determine optimal cut-off levels of Q KFLC, Q LFLC, KFLC index, LFLC index, and CSF KFLC to LFLC ratio (Figure 5 and Table 2). To distinguish MS patients from all other patients, KFLC index (Q KFLC/Q Alb) showed the highest AUC with 97.0% (95% CI: 94.6–99.4) as compared to other quantitative FLC values. AUCs of KFLC measures were generally larger than those of LFLC measures.
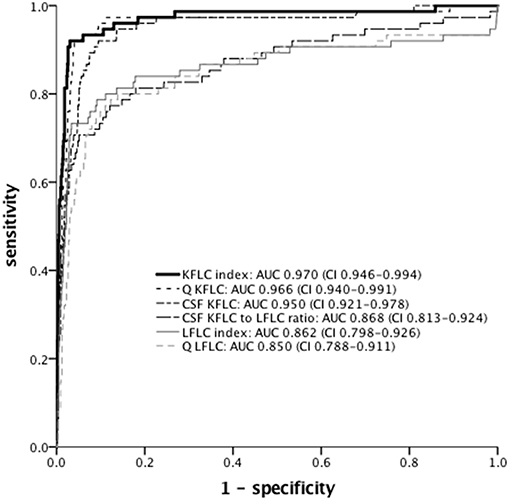
Figure 5. ROC curves of different FLC measures for discrimination between MS (n = 75) and non-MS (n = 1,149) patients. AUC and 95% confidence interval are given. KFLC, immunoglobulin kappa free light chain; LFLC, immunoglobulin lambda free light chain; Q Alb, CSF-serum ratio of albumin; Q KFLC, CSF-serum ratio of KFLC; Q LFLC, CSF-serum ratio of LFLC; KFLC index (Q KFLC/Q Alb); LFLC index (Q LFLC/Q Alb); ROC, receiver operating characteristics; AUC, area under the curve.
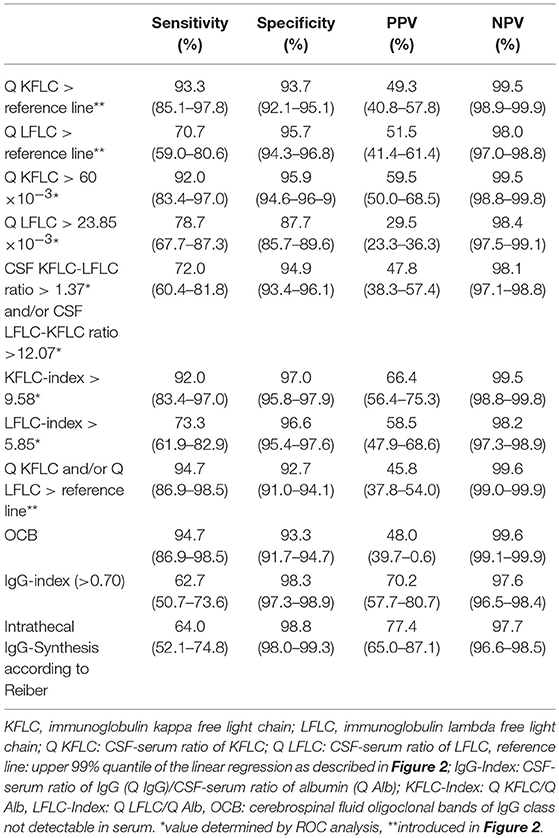
Table 2. Sensitivity, specificity, positive, and negative predictive value with corresponding 95% confidence interval for different FLC values, IgG-index, and OCB regarding diagnosis of multiple sclerosis.
Sensitivity, specificity, positive, and negative predictive values of all parameters investigated are given in Table 2. Of all markers investigated, OCB and Q KFLC (applying the Q Alb-dependent reference values described in Figures 2, 3) showed the highest sensitivities with 94.7 and 93.3%, respectively. Combination of Q KFLC and Q LFLC improved the sensitivity to 94.7%. IgG-index (above >0.7) and intrathecal IgG synthesis calculated according to Reiber showed the highest specificities of 98.3 and 98.8%, respectively. The KFLC index showed the highest combined sensitivity (92%) and specificity (97%).
Discussion
Several findings support the role of B cells and intrathecal immunoglobulins in the pathogenesis of MS including (i) presence of B-cell infiltrates in the CNS parenchyma and meningeal tissues (41, 42), (ii) elevated CSF B-cell activation markers (e.g., the polyspecific intrathecal B cell response against neurotropic viruses MRZR as well as the B-cell attracting chemokine CXCL13) in MS (43, 44), (iii) upregulation of Ig-related genes in cortical sections of MS patients (45), and (iv) efficacy of B-cell targeting therapies (e.g., rituximab, ocrelizumab) (46, 47) as well as positive effect of apharesis treatments (plamapheresis, immunoadsorption) (48).
The presence of OCB in the CSF is known since the 1970s (49, 50) as the most prominent immunological hallmark of MS. In concordance with this, the CSF B-cell immunoglobulin transcriptome shows remarkable overlap with the corresponding immunoglobulin proteome (51–53) indicating immunoglobulin production by intrathecal B-cells. Besides intact immunoglobulins B-cells produce FLC as a by-product, which have been reported to be increased in the CSF of MS patients and proposed to be a quantitative diagnostic and prognostic biomarker for MS (17–26).
In line with previous studies from others and us (17, 18, 26) the present study confirms elevated intrathecal KFLC and LFLC production in patients with MS leading to high diagnostic accuracy. To allow an assessment of the utility and strengths of different FLC measures, we determined not only CSF and serum levels of FLC but also compared various FLC measures including CSF-serum ratio (Q KFLC, Q LFLC), FLC index (KFLC index, LFLC index), and CSF KFLC to LFLC ratio within one large cohort for their diagnostic accuracy in MS.
A significant correlation of CSF FLC levels (both KFLC and LFLC) with serum FLC levels and CSF-serum ratio of albumin (Q Alb) in NIND patients could be shown. Based on the large cohort of patients without any inflammatory CNS reaction we were able to introduce reference values of Q FLC (for both KFLC and LFLC) in relation to a wide range of Q Alb, which is a widely accepted quantitative measure of blood-CSF barrier function (54). Furthermore, ROC curve determined reference values were established for Q KFLC, Q LFLC, KFLC index, LFLC index, and CSF KFLC to LFLC ratio.
This study allowed to assess the diagnostic utility of the different FLC measures within one large cohort. Q KFLC (applying Q Alb- depending reference values) showed the highest sensitivity and the KFLC index showed the highest combined sensitivity and specificity. With regard to the diagnostic accuracy of Q KFLC in MS, however, applying the Q Alb-dependent reference values (introduced in Figure 2) showed no relevant difference as compared to the ROC curve determined cut-off value. This observation is possibly due to generally intact blood-CSF barrier function (normal Q Alb) in MS.
In general, KFLC showed higher diagnostic relevance in MS as compared with LFLC. This could be possibly explained by the dominance of KFLC in the human body, since the kappa chain is rearranged first during IgG production and is quantitatively more common. Furthermore, LFLC are usually dimeric in form while KFLC are generally monomeric but can exist as non-covalently linked dimer (55).
Here we compared not only the diagnostic accuracy of FLC values with OCB but also with other quantitative values of intrathecal IgG synthesis (IgG index and synthesis according to Reiber). Intrathecal KFLC and OCB showed nearly the same value (93.3 and 94.67%) with regard to diagnostic sensitivity in patients with MS, which is in line with earlier reports concerning diagnostic sensitivity of OCB in MS (56–58). As it is known for OCB (58), specificity of KFLC in MS diagnosis was significantly reduced when other inflammatory etiologies were considered.
Does the determination of FLC have any advantage over the established markers of intrathecal IgG synthesis? In comparison to OCB, measurement of KFLC is reliable, rapid, methodologically simple, can be performed using either ELISA or nephelometry, and can be applied in the clinical setting together with testing of basic CSF variables. In comparison to other quantitative parameters of intrathecal IgG synthesis (according to Reiber or IgG index), Q KFLC is more sensitive.
On the other hand, quantitative FLC values do not provide any insight into the clonality of intrathecal IgG, while the qualitative detection of IgG by immunoblotting can discriminate between monoclonal, oligoclonal, and polyclonal patterns.
In conclusion, CSF FLC may not replace OCB but may be supportive quantitative parameters in particular cases, for example in OCB negative MS cases or equivocal OCB findings.
Furthermore, this study again underlines the utility and accuracy of CSF examination in the diagnostic procedure of MS since very high diagnostic accuracy could be confirmed once again for well-established (OCB, IgG index) and demonstrated for promising (intrathecal KFLC) markers of intrathecal IgG production.
Data Availability
The datasets generated for this study are available on request to the corresponding author.
Ethics Statement
Written informed consent was obtained from all patients in accordance with the Declaration of Helsinki, and the study was approved by the ethics committee of the University of Ulm (No. 10/20).
Author Contributions
MS, FM-Y, and HT were involved in the conception and design of the study. Data were acquired by FM-Y, MS, FB, JL, AL, MO, and HT. MS, FM-Y, UB, and HT were involved in the statistical methods and analysis. The first draft of the manuscript was designed by MS and HT, followed by critical revision of all authors. The final version for submission was approved by all authors.
Conflict of Interest Statement
MS has received honoraria for speaking and/or travel from Bayer, Biogen, Sanofi Genzyme, and TEVA and research funding from the Hertha-Nathorff-Program and University of Ulm, none related to this study. JL has received honoraria for speaking and travel grants from Bayer, TEVA, CHDI, and the Movement Disorders Society. HT received funding for research projects, lectures, and travel from Bayer, Biogen, Genzyme, Fresenius, Merck, Mylan, Novartis, Roche, Siemens Health Diagnostics, Teva, and received research support from Hertie-Stiftung, DMSG, BMBF, University of Ulm and Landesstiftung BW.
The remaining authors declare that the research was conducted in the absence of any commercial or financial relationships that could be construed as a potential conflict of interest.
Acknowledgments
We thank Vera Lehmensiek and Refika Aksamija for technical support and André Huss for reviewing. This work was in part supported by the BMBF grant (competence net multiple sclerosis KKNMS) and the University of Ulm.
References
1. Noseworthy JH, Lucchinetti C, Rodriguez M, Weinshenker BG. Multiple sclerosis. N Engl J Med. (2000) 343:938–52. doi: 10.1056/NEJM200009283431307
2. Hohlfeld R. Multiple sclerosis: human model for EAE? Eur J Immunol. (2009) 39:2036–9. doi: 10.1002/eji.200939545
3. Compston A, Coles A. Multiple sclerosis. Lancet. (2008) 372:1502–17. doi: 10.1016/S0140-6736(08)61620-7
4. Thompson AJ, Banwell BL, Barkhof F, Carroll WM, Coetzee T, Comi G, et al. Diagnosis of multiple sclerosis: 2017 revisions of the McDonald criteria. Lancet Neurol. (2018) 17:162–73. doi: 10.1016/S1474-4422(17)30470-2
5. Stangel M, Fredrikson S, Meinl E, Petzold A, Stuve O, Tumani H. The utility of cerebrospinal fluid analysis in patients with multiple sclerosis. Nat Rev Neurol. (2013) 9:267–76. doi: 10.1038/nrneurol.2013.41
6. Tintore M, Rovira A, Rio J, Tur C, Pelayo R, Nos C, et al. Do oligoclonal bands add information to MRI in first attacks of multiple sclerosis? Neurology. (2008) 70:1079–83. doi: 10.1212/01.wnl.0000280576.73609.c6
7. Tumani H, Hartung HP, Hemmer B, Teunissen C, Deisenhammer F, Giovannoni G, et al. Cerebrospinal fluid biomarkers in multiple sclerosis. Neurobiol Dis. (2009) 35:117–27. doi: 10.1016/j.nbd.2009.04.010
8. Huss AM, Halbgebauer S, Ockl P, Trebst C, Spreer A, Borisow N, et al. Importance of cerebrospinal fluid analysis in the era of McDonald 2010 criteria: a German-Austrian retrospective multicenter study in patients with a clinically isolated syndrome. J Neurol. (2016) 263:2499–504. doi: 10.1007/s00415-016-8302-1
9. Heussinger N, Kontopantelis E, Gburek-Augustat J, Jenke A, Vollrath G, Korinthenberg R, et al. Oligoclonal bands predict multiple sclerosis in children with optic neuritis. Ann Neurol. (2015) 77:1076–82. doi: 10.1002/ana.24409
10. Franciotta D, Lolli F. Interlaboratory reproducibility of isoelectric focusing in oligoclonal band detection. Clin Chem. (2007) 53:1557–8. doi: 10.1373/clinchem.2007.089052
11. Kaplan B, Livneh A, Sela BA. Immunoglobulin free light chain dimers in human diseases. Sci World J. (2011) 11:726–35. doi: 10.1100/tsw.2011.65
12. van der Heijden M, Kraneveld A, Redegeld F. Free immunoglobulin light chains as target in the treatment of chronic inflammatory diseases. Eur J Pharmacol. (2006) 533:319–26. doi: 10.1016/j.ejphar.2005.12.065
13. Hopper JE, Papagiannes E. Evidence by radioimmunoassay that mitogen-activated human blood mononuclear cells secrete significant amounts of light chain Ig unassociated with heavy chain. Cell Immunol. (1986) 101:122–31. doi: 10.1016/0008-8749(86)90191-7
14. Abraham RS, Charlesworth MC, Owen BA, Benson LM, Katzmann JA, Reeder CB, et al. Trimolecular complexes of lambda light chain dimers in serum of a patient with multiple myeloma. Clin Chem. (2002) 48:1805–11. http://clinchem.aaccjnls.org/content/48/10/1805/tab-article-info
15. Abe M, Goto T, Kosaka M, Wolfenbarger D, Weiss DT, Solomon A. Differences in kappa to lambda (kappa:lambda) ratios of serum and urinary free light chains. Clin Exp Immunol. (1998) 111:457–62. doi: 10.1046/j.1365-2249.1998.00487.x
16. Bradwell AR, Carr-Smith HD, Mead GP, Tang LX, Showell PJ, Drayson MT, et al. Highly sensitive, automated immunoassay for immunoglobulin free light chains in serum and urine. Clin Chem. (2001) 47:673–80.
17. Voortman MM, Stojakovic T, Pirpamer L, Jehna M, Langkammer C, Scharnagl H, et al. Prognostic value of free light chains lambda and kappa in early multiple sclerosis. Mult Scler. (2017) 23:1496–505. doi: 10.1177/1352458516681503
18. Senel M, Tumani H, Lauda F, Presslauer S, Mojib-Yezdani R, Otto M, et al. Cerebrospinal fluid immunoglobulin kappa light chain in clinically isolated syndrome and multiple sclerosis. PLoS ONE. (2014) 9:e88680. doi: 10.1371/journal.pone.0088680
19. Kaplan B, Aizenbud BM, Golderman S, Yaskariev R, Sela BA. Free light chain monomers in the diagnosis of multiple sclerosis. J Neuroimmunol. (2010) 229:263–71. doi: 10.1016/j.jneuroim.2010.09.002
20. Presslauer S, Milosavljevic D, Brucke T, Bayer P, Hubl W. Elevated levels of kappa free light chains in CSF support the diagnosis of multiple sclerosis. J Neurol. (2008) 255:1508–14. doi: 10.1007/s00415-008-0954-z
21. Desplat-Jego S, Feuillet L, Pelletier J, Bernard D, Cherif AA, Boucraut J. Quantification of immunoglobulin free light chains in cerebrospinal fluid by nephelometry. J Clin Immunol. (2005) 25:338–45. doi: 10.1007/s10875-005-5371-9
22. Fischer C, Arneth B, Koehler J, Lotz J, Lackner KJ. Kappa free light chains in cerebrospinal fluid as markers of intrathecal immunoglobulin synthesis. Clin Chem. (2004) 50:1809–13. doi: 10.1373/clinchem.2004.033977
23. Krakauer M, Schaldemose Nielsen H, Jensen J, Sellebjerg F. Intrathecal synthesis of free immunoglobulin light chains in multiple sclerosis. Acta Neurol Scand. (1998) 98:161–5. doi: 10.1111/j.1600-0404.1998.tb07287.x
24. Rudick RA, French CA, Breton D, Williams GW. Relative diagnostic value of cerebrospinal fluid kappa chains in MS: comparison with other immunoglobulin tests. Neurology. (1989) 39:964–8. doi: 10.1212/WNL.39.7.964
25. Fagnart OC, Sindic CJ, Laterre C. Free kappa and lambda light chain levels in the cerebrospinal fluid of patients with multiple sclerosis and other neurological diseases. J Neuroimmunol. (1988) 19:119–32. doi: 10.1016/0165-5728(88)90041-0
26. Presslauer S, Milosavljevic D, Huebl W, Aboulenein-Djamshidian F, Krugluger W, Deisenhammer F, et al. Validation of kappa free light chains as a diagnostic biomarker in multiple sclerosis and clinically isolated syndrome: a multicenter study. Mult Scler. (2016) 22:502–10. doi: 10.1177/1352458515594044
27. Villar LM, Espino M, Costa-Frossard L, Muriel A, Jimenez J, Alvarez-Cermeno JC. High levels of cerebrospinal fluid free kappa chains predict conversion to multiple sclerosis. Clin Chim Acta. (2012) 413:1813–16. doi: 10.1016/j.cca.2012.07.007
28. Hassan-Smith G, Durant L, Tsentemeidou A, Assi LK, Faint JM, Kalra S, et al. High sensitivity and specificity of elevated cerebrospinal fluid kappa free light chains in suspected multiple sclerosis. J Neuroimmunol. (2014) 276:175–9. doi: 10.1016/j.jneuroim.2014.08.003
29. Goffette S, Schluep M, Henry H, Duprez T, Sindic CJ. Detection of oligoclonal free kappa chains in the absence of oligoclonal IgG in the CSF of patients with suspected multiple sclerosis. J Neurol Neurosurg Psychiatry. (2004) 75:308–10. doi: 10.1136/jnnp.2003.010710
30. Duranti F, Pieri M, Centonze D, Buttari F, Bernardini S, Dessi M. Determination of kappaFLC and kappa index in cerebrospinal fluid: a valid alternative to assess intrathecal immunoglobulin synthesis. J Neuroimmunol. (2013) 263:116–20. doi: 10.1016/j.jneuroim.2013.07.006
31. Lamers KJ, de Jong JG, Jongen PJ, Kock-Jansen MJ, Teunesen MA, Prudon-Rosmulder EM. Cerebrospinal fluid free kappa light chains versus IgG findings in neurological disorders: qualitative and quantitative measurements. J Neuroimmunol. (1995) 62:19–25. doi: 10.1016/0165-5728(95)00086-H
32. Polman CH, Reingold SC, Banwell B, Clanet M, Cohen JA, Filippi M, et al. Diagnostic criteria for multiple sclerosis: 2010 revisions to the McDonald criteria. Ann Neurol. (2011) 69:292–302. doi: 10.1002/ana.22366
33. Teunissen CE, Petzold A, Bennett JL, Berven FS, Brundin L, Comabella M, et al. A consensus protocol for the standardization of cerebrospinal fluid collection and biobanking. Neurology. (2009) 73:1914–22. doi: 10.1212/WNL.0b013e3181c47cc2
34. Halbgebauer S, Haussmann U, Klafki H, Tumani H, Wiltfang J, Otto M. Capillary isoelectric focusing immunoassay as a new nanoscale approach for the detection of oligoclonal bands. Electrophoresis. (2015) 36:355–62. doi: 10.1002/elps.201400339
35. Reiber H, Peter JB. Cerebrospinal fluid analysis: disease-related data patterns and evaluation programs. J Neurol Sci. (2001) 184:101–22. doi: 10.1016/S0022-510X(00)00501-3
36. Reiber H, Otto M, Trendelenburg C, Wormek A. Reporting cerebrospinal fluid data: knowledge base and interpretation software. Clin Chem Lab Med. (2001) 39:324–32. doi: 10.1515/CCLM.2001.051
38. Davino C, Furno M, Vistocco D. Quantile Regression: Theory and Applications. Chichester: Wiley (2014).
40. Newcombe RG. Confidence intervals: enlightening or mystifying? BMJ. (1992) 304:381–2. doi: 10.1136/bmj.304.6823.381-c
41. Serafini B, Rosicarelli B, Magliozzi R, Stigliano E, Aloisi F. Detection of ectopic B-cell follicles with germinal centers in the meninges of patients with secondary progressive multiple sclerosis. Brain Pathol. (2004) 14:164–74. doi: 10.1111/j.1750-3639.2004.tb00049.x
42. Lucchinetti CF, Popescu BF, Bunyan RF, Moll NM, Roemer SF, Lassmann H, et al. Inflammatory cortical demyelination in early multiple sclerosis. N Engl J Med. (2011) 365:2188–97. doi: 10.1056/NEJMoa1100648
43. Brettschneider J, Czerwoniak A, Senel M, Fang L, Kassubek J, Pinkhardt E, et al. The chemokine CXCL13 is a prognostic marker in clinically isolated syndrome (CIS). PLoS ONE. (2010) 5:e11986. doi: 10.1371/journal.pone.0011986
44. Brettschneider J, Tumani H, Kiechle U, Muche R, Richards G, Lehmensiek V, et al. IgG antibodies against measles, rubella, and varicella zoster virus predict conversion to multiple sclerosis in clinically isolated syndrome. PLoS ONE. (2009) 4:e7638. doi: 10.1371/journal.pone.0007638
45. Torkildsen O, Stansberg C, Angelskar SM, Kooi EJ, Geurts JJ, van der Valk P, et al. Upregulation of immunoglobulin-related genes in cortical sections from multiple sclerosis patients. Brain Pathol. (2010) 20:720–9. doi: 10.1111/j.1750-3639.2009.00343.x
46. Hauser SL, Waubant E, Arnold DL, Vollmer T, Antel J, Fox RJ, et al. B-cell depletion with rituximab in relapsing-remitting multiple sclerosis. N Engl J Med. (2008) 358:676–88. doi: 10.1056/NEJMoa0706383
47. Hauser SL, Bar-Or A, Comi G, Giovannoni G, Hartung HP, Hemmer B, et al. Ocrelizumab versus Interferon Beta-1a in Relapsing Multiple Sclerosis. N Engl J Med. (2017) 376:221–34. doi: 10.1056/NEJMoa1601277
48. Keegan M, Konig F, McClelland R, Bruck W, Morales Y, Bitsch A, et al. Relation between humoral pathological changes in multiple sclerosis and response to therapeutic plasma exchange. Lancet. (2005) 366:579–82. doi: 10.1016/S0140-6736(05)67102-4
49. Laterre EC, Callewaert A, Heremans JF, Sfaello Z. Electrophoretic morphology of gamma globulins in cerebrospinal fluid of multiple sclerosis and other diseases of the nervous system. Neurology. (1970) 20:982–90. doi: 10.1212/WNL.20.10.982
50. Link H, Tibbling G. Principles of albumin and IgG analyses in neurological disorders. III. Evaluation of IgG synthesis within the central nervous system in multiple sclerosis. Scand J Clin Lab Invest. (1977) 37:397–401. doi: 10.3109/00365517709091498
51. Brandle SM, Obermeier B, Senel M, Bruder J, Mentele R, Khademi M, et al. Distinct oligoclonal band antibodies in multiple sclerosis recognize ubiquitous self-proteins. Proc Natl Acad Sci USA. (2016) 113:7864–9. doi: 10.1073/pnas.1522730113
52. Beltran E, Obermeier B, Moser M, Coret F, Simo-Castello M, Bosca I, et al. Intrathecal somatic hypermutation of IgM in multiple sclerosis and neuroinflammation. Brain. (2014) 137:2703–14. doi: 10.1093/brain/awu205
53. Obermeier B, Lovato L, Mentele R, Bruck W, Forne I, Imhof A, et al. Related B cell clones that populate the CSF and CNS of patients with multiple sclerosis produce CSF immunoglobulin. J Neuroimmunol. (2011) 233:245–8. doi: 10.1016/j.jneuroim.2011.01.010
54. Felgenhauer K, Schliep G, Rapic N. Evaluation of the blood-CSF barrier by protein gradients and the humoral immune response within the central nervous system. J Neurol Sci. (1976) 30:113–28. doi: 10.1016/0022-510X(76)90259-8
55. Solomon A. Bence-Jones proteins and light chains of immunoglobulins (first of two parts). N Engl J Med. (1976) 294:17–23. doi: 10.1056/NEJM197601012940105
56. Freedman MS, Thompson EJ, Deisenhammer F, Giovannoni G, Grimsley G, Keir G, et al. Recommended standard of cerebrospinal fluid analysis in the diagnosis of multiple sclerosis: a consensus statement. Arch Neurol. (2005) 62:865–70. doi: 10.1001/archneur.62.6.865
57. Link H, Huang YM. Oligoclonal bands in multiple sclerosis cerebrospinal fluid: an update on methodology and clinical usefulness. J Neuroimmunol. (2006) 180:17–28. doi: 10.1016/j.jneuroim.2006.07.006
Keywords: immunoglobulin free light chains, oligoclonal bands, OCB, intrathecal IgG synthesis, IgG index, multiple sclerosis, cerebrospinal fluid, serum
Citation: Senel M, Mojib-Yezdani F, Braisch U, Bachhuber F, Lewerenz J, Ludolph AC, Otto M and Tumani H (2019) CSF Free Light Chains as a Marker of Intrathecal Immunoglobulin Synthesis in Multiple Sclerosis: A Blood-CSF Barrier Related Evaluation in a Large Cohort. Front. Immunol. 10:641. doi: 10.3389/fimmu.2019.00641
Received: 20 November 2018; Accepted: 08 March 2019;
Published: 29 March 2019.
Edited by:
Maja Jagodic, Karolinska Institutet (KI), SwedenReviewed by:
Bonaventura Casanova, Hospital Universitari i Politècnic La Fe, SpainSeema Kalra, University Hospitals of North Midlands NHS Trust, United Kingdom
Copyright © 2019 Senel, Mojib-Yezdani, Braisch, Bachhuber, Lewerenz, Ludolph, Otto and Tumani. This is an open-access article distributed under the terms of the Creative Commons Attribution License (CC BY). The use, distribution or reproduction in other forums is permitted, provided the original author(s) and the copyright owner(s) are credited and that the original publication in this journal is cited, in accordance with accepted academic practice. No use, distribution or reproduction is permitted which does not comply with these terms.
*Correspondence: Makbule Senel, makbule.senel@uni-ulm.de
Hayrettin Tumani, hayrettin.tumani@uni-ulm.de