- 1State Key Laboratory of Research on Bioactivities and Clinical Applications of Medicinal Plants, Institute of Chinese Medicine, The Chinese University of Hong Kong, Shatin, Hong Kong
- 2State Key Laboratory of Quality Research in Chinese Medicines, Macau Institute for Applied Research in Medicine and Health, Macau University of Science and Technology, Taipa, Macau
- 3Department of Medicine and Therapeutics, The Chinese University of Hong Kong, Shatin, Hong Kong
- 4Department of Chemical Pathology, The Chinese University of Hong Kong, Shatin, Hong Kong
Interleukin (IL)-33 belongs to IL-1 cytokine family which is constitutively produced from the structural and lining cells including fibroblasts, endothelial cells, and epithelial cells of skin, gastrointestinal tract, and lungs that are exposed to the environment. Different from most cytokines that are actively secreted from cells, nuclear cytokine IL-33 is passively released during cell necrosis or when tissues are damaged, suggesting that it may function as an alarmin that alerts the immune system after endothelial or epithelial cell damage during infection, physical stress, or trauma. IL-33 plays important roles in type-2 innate immunity via activation of allergic inflammation-related eosinophils, basophils, mast cells, macrophages, and group 2 innate lymphoid cells (ILC2s) through its receptor ST2. In this review, we focus on the recent advances of the underlying intercellular and intracellular mechanisms by which IL-33 can regulate the allergic inflammation in various allergic diseases including allergic asthma and atopic dermatitis. The future pharmacological strategy and application of traditional Chinese medicines targeting the IL-33/ST2 axis for anti-inflammatory therapy of allergic diseases were also discussed.
Introduction
Interleukin33 (IL-33) is a member of the IL-1 cytokine family that includes IL-1α, IL-1β, and IL-18 (1) and constitutively expressed in structural and lining cells including fibroblasts, endothelial, and epithelial cells of skin, gastrointestinal tract, and lungs that are exposed to the environment (2). IL-33 lacks a secretory signal peptide encoded by the Il1rl1 gene (1), an IL-1 family trait for releasing via the classical endoplasmic reticulum and Golgi pathway (1). Under the inactive state, IL-33 is harbored in the cell nuclei and associated with chromatin by a chromatin-binding motif, belonging to the cellular homeostasis and acting as a transcriptional repressor (2, 3). The N-terminus of IL-33 contains a nuclear localization sequence, a homeodomain-like helix-turn-helix DNA-binding domain and a chromatin-binding domain (3). Different from most cytokines that are actively secreted from cells, IL-33 is released passively in its full length form (amino acids 1–270, IL-33FL) during cell necrosis, cellular activation through ATP signaling without cell death or when tissues are damaged, suggesting that it may function as an alarmin that alerts the immune system after endothelial or epithelial cell damage during infection, physical stress or trauma (4, 5). IL-33 activates signaling pathways depending on the myeloid differentiation primary response gene 88 (Myd88) of immune cells expressing the cytokine receptor interleukin 1 receptor-like 1 (ST2) and signals through a heterodimeric receptor complex comprising an IL-33-specific ST2 coupled with the co-receptor IL-1 receptor accessory protein (IL-1 RAcP) (6, 7). ST2 is selectively and stably expressed on the cell surface of Th2 cells (8), CD4+ T cells, group 2 innate lymphoid cells (ILC2s) and also other immune cells such as mast cells, basophils, eosinophils, macrophages, dendritic cells and natural killer cells (9–18). Signaling of IL-33 can be activated through nuclear factor kappa-B (NF-κB), c-Jun N-terminal kinase (JNK), and p38 mitogen activated protein kinase (MAPK) cascades (19).
In humans, both IL-33 mRNA and protein are substantially elevated in the inflamed skin lesions of atopic dermatitis (AD) patients when compared with non-inflamed skin (20). IL-33 is a Th2-oriented cytokine which enhances the production of Th2 cytokines, particularly IL-5 and IL-13 (21). In addition, IL-33 is also a chemoattractant for Th2 cells in vitro and in vivo, indicating the importance of IL-33 in Th2 cells mobilization (22). In large-scale genome-wide association studies, genes encoding IL-33 and its receptors have been identified as susceptibility loci in asthma (23–26).The alarmin activities of IL-33 are regulated at multiple levels (6, 7, 27). Several hours after its extracellular release, IL-33FL is transiently inactivated by oxidation of critical cysteine residues (28). Inflammatory proteases from immune cells such as neutrophils (cathepsin G and elastase) and mast cells (chymase and tryptase) degrade IL-33FL into shorter mature forms containing the C-terminal IL-1-like cytokine domain with much higher activity than IL-33FL (29). A recent study has further shown that IL-33FL functions as a protease sensor that detects proteolytic activities associated with various environmental allergens including house dust mite, pollens, bacteria and fungi (30). When exposed to allergen proteases, IL-33FL is rapidly cleaved in its central “sensor” domain, which leads to the activation of the generation of ILC2s, and allergic inflammation can be reduced by preventing the IL-33FL cleavage (30, 31). In this review, we focus on the recent advances of the underlying intercellular and intracellular mechanisms by which IL-33 can regulate various key immune cells in the allergic inflammatory diseases including allergic asthma and AD, and the future pharmacological strategy and the potential application of traditional Chinese medicines targeting the IL-33/ST2 axis for the treatment of allergic inflammatory diseases.
Effects of IL-33 on Immune Cells Activation in Allergic Inflammations
Eosinophils
IL-33 potently induces eosinophilia in in vivo murine models (32, 33) and activates eosinophils, the principal effector cells in allergic inflammation, to produce superoxide (34), upregulates the expression of adhesion molecules and enhances eosinophil survival (35), suggesting that it can play an important role in the exacerbation of inflammation in allergic diseases mediated by the activation of eosinophils. Polymorphism of human IL-33 and ST2 genes has been shown to associate with increased numbers of eosinophils (36). In our previous studies, we have shown the activation of eosinophils, by different stimuli and its interactions with structural cells in atopic dermatitis (AD) and allergic asthma (37–44). Such findings showed that intercellular interaction of eosinophils and dermal fibroblasts could provoke the release of pro-inflammatory cytokines and chemokines, implying the pathogenic effects of eosinophils infiltration in the inner dermal fibroblast layer in AD skin lesions.
In our study of allergic inflammation, IL-33 significantly promote eosinophil survival and cell surface expression of the adhesion molecule intercellular adhesion molecule (ICAM)-1, but ICAM-3, and L-selectin expressions were suppressed. In addition, IL-33 stimulates significant release of pro-inflammatory cytokine IL-6 and the chemokines CXCL8 and CCL2 from eosinophils (41).The release of cytokines and chemokines were differentially regulated by the activation of nuclear factor (NF)-kB, p38 mitogen-activated protein kinase (MAPK) and extracellular signal-regulated kinase (ERK) pathways in eosinophils (41, 45). In our study of IL-33 in AD using eosinophils and fibroblasts co-culture, we found that there was significant increase in the production of pro-inflammatory cytokines such as IL-6 and AD-associated chemokines CXCL1, CXCL10, CCL2, and CCL5 (45). Such increase was further upregulated by IL-33 stimulation, and significant production of CXCL8 from eosinophils and fibroblasts co-culture was observed (42). The main source in co-culture for the release of CCL5, and IL-6, CXCL1, CXCL8, CXCL10, and CCL2 was eosinophils and fibroblasts, respectively, and direct contact between eosinophils and fibroblasts was essential for the release of AD-related chemokine CXCL1, CXCL10, CXCL8, and CCL5. IL-33 stimulation also upregulated the cell surface expression of intercellular adhesion molecule-1 (ICAM-1) on both eosinophils and fibroblasts in co-culture, with differential activation of ERK, JNK, p38 MAPK, NF-kB, and phosphatidylinositol 3-kinase–Akt (PI3K/Akt) pathways (42).
T Cells and ILC2
Besides eosinophils, IL-33 has also been shown to be an active and soluble co-stimulator of T cells, by promoting the expansion and functional differentiation of both effector T cells and GATA-3+ regulatory T cells (9, 46). Study of IL-33 signaling-deficient mice has also demonstrated the crucial role of IL-33 in protective anti-viral T cell immunity (9). Activated Th1 and CD8+ T cells have been shown to transiently express lower amounts of IL-33 receptor ST2, when compared with Th2 cells. However, IL-33 signaling can induce the expression of the lineage-specific transcription factors FOXP3, GATA-3, and T-bet for the positive activation of ST2 expression on Th1 cells (47). For regulatory T cells in the intestine, high level of ST2 are constitutively expressed and associated to the pathogenesis of eosinophilic pneumonia (48, 49).
In the mucosal barrier sites, IL-33 has been shown to coordinate the type 2 immune response through the activation of ST2-positive immune cells, such as ILC2s and CD4+ T cells (50). ILC2s are primarily localized at mucosal surfaces of lung, skin, gut and adipose tissues (51, 52) and play an important role in IL-33 associated allergic inflammatory diseases. Although ILC2s lack antigen receptors, they can be rapidly activated by the epithelial derived cytokines IL-33, IL-25, and thymic stromal lymphopoietin (TSLP), prostaglandin D2 from mast cells or cysteinyl leukotrienes secreted by activated hematopoietic cells (53–55). Activated ILC2 cells proliferate rapidly and act as an early innate source by producing large amount of the Th2 cytokines IL-5 and IL-13 in a synergistic manner (56). Bone marrow ILC2s has been shown to be a local source of IL-5 in IL-33-driven eosinophilia (57). Impaired Th2 cell differentiation was observed in ILC2 knockout mice (48), and the differentiation is in a cell-contact manner through major histocompatibility complex class II (49).
Mast Cells and Basophils
Mast cells and its blood counterpart basophils, both play an important roles in allergic inflammation by generation and release of a panel of inflammatory mediators, such as histamine (58). Allergens can cross link with IgE sensitized mast cells to activate and release of large amounts of preformed and newly formed mediators: histamine, heparin, and proteases such as carboxypeptidase A3, chymase and tryptase (59–61). Most of these active proteases in the granule can cleave targets in nearby tissue compartments upon secreted from the activated mast cells (61). In human, mucosal mast cells express only tryptase and connective tissue mast cells express tryptase, chymase, and carboxypeptidase A3 (62). In mouse, mucosal mast cells express 2 chymase subtypes, mast cell protease (MCPT) 1 and MCPT2, whereas connective tissue type mast cells express the chymase MCPT4 and the elastase MCPT5, the tryptases MCPT6 and MCPT7, and carboxypeptidase A3 (62). IL-33 associated mast cell functions are involved in the pathogenesis of different allergic inflammations such as food anaphylaxis (63) and respiratory allergy induced by house dust mite or aspirin (54, 64, 65). Since the IL-33 receptor ST2 is constitutively expressed on mast cells, basophils and their progenitors cells, it is a critical amplifiers of IL-33–mediated allergic inflammation with the capacity in secreting a wide array of inflammatory cytokines and mediators (66). Antigen or IL-33 activated human mast cells can also release soluble ST2, which may further modulate the biologic effects of IL-33 (67). IL-33 has been demonstrated to enhance the adhesion of mast cells onto laminin, fibronectin, and vitronectin, increase the expression of adhesion molecules, such as ICAM-1 and vascular cell adhesion molecule-1 (VCAM-1) on endothelial cells, thus promoting mast cell adhesion to blood vessel walls (59). Mast cell survival, growth, development and maturation can be enhanced by IL-33 via the ST2/Myd88 pathway (68). Mast cell-derived tryptase and chymase have been demonstrated to cleave extracellular IL-33 into mature active forms (29) and IL-33 isoforms may have additional abilities to activate mast cells, thereby further provoking inflammation (69). Human mast cell chymase (HC) seems to be substrate specific. In a study using 51 active recombinant cytokines and chemokines (70), only 3 of them were substantially cleaved (IL-15 and two IL-1–related alarmins: IL-18 and IL-33) by HC.
The roles of mast cells proteases are not only associated with pro-inflammatory activities. In an in vivo study using ovalbumin (OVA)-sensitized mice lacking mouse MC protease 4 (mMCP4) (71), a chymase that is functionally equivalent to human chymase, the airway hyperresponsiveness when challenged with OVA was significantly higher in mMCP-4(−/−) mice when compared with wild type mice. The thickness of the smooth muscle cell (SMC) layer was more pronounced in mMCP-4(−/−) mice than in wild type control mice, thus indicating that chymase may have a modulating effect on airway SMCs. Taken together, the regulating role of chymase present in the upper airways could protect the animals against allergic airway responses. Therefore, the pro-inflammatory and anti-inflammatory effects of mast cells proteases may occur during different time frames, for example, initial activities that promote the airway response being followed by the mounting of protective activities that down-regulate the initial pro-inflammatory activities. Similar to mast cells, IL-33 mediates activation of human basophils and enhances their effector functions (58–60). Compared to mast cells, human basophils seem to have less amounts of proteases (61, 72). IL-33 also promotes asthma-related IL-4 and IL-13 production from basophils via MyD88-signaling pathway (73).
Macrophages
With abundant localization in lung tissue, macrophages are the important innate immune cells participating in allergic asthmatic inflammation (74). Th2 cytokines (IL-4 and IL-13) can polarize macrophage into alternative activated macrophage (AAM) phenotypes (75). Depletion of alveolar macrophages in murine acute allergic lung inflammation model demonstrated that Th2-immunity of allergic lung inflammation and airway remodeling were attenuated (76). IL-33 has been shown to promote the polarization of AAM that expressed mannose receptor and secreted CCL17 and CCL24 in an IL-13-dependent manner, thereby contributing to the airway inflammation in mice (77). IL-33 can enhance the lipopolysaccharide-mediated in vitro activation of macrophages, with the upregulation of the expression of toll-like receptor (TLR)4, myeloid differentiation protein 2, soluble CD14, and MyD88 (78). The updated effects of IL-33 on the activation of eosinophils, basophils, mast cells, macrophages, ILC2 cells and T cells in allergic inflammation is summarized in Figure 1.
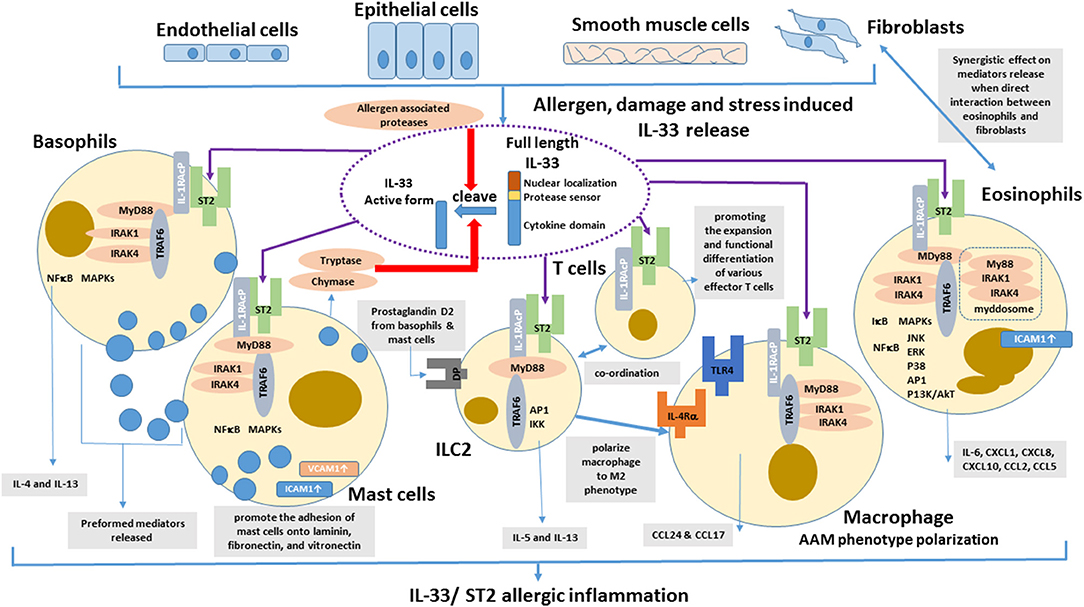
Figure 1. Effects of IL-33 on the activation of eosinophils, basophils, mast cells, DCs, ILC2 cells, and T cells in allergic inflammation. IL-33 is normally sequestered in the nucleus of various cells via nuclear-localization and chromatin-binding motifs in its amino terminus. After the cells are damaged, under stress, or stimulated by allergens, full-length IL-33 is released extracellularly, but it has low activity as a cytokine. Mast cells proteases and some allergens possess protease activity and can directly process IL-33 by cleaving within the protease-sensor domain to generate a more potent cytokine domain, which will directly activate local and infiltrating basophils, mast cells, group 2 innate lymphoid cells (ILC2), T cells, and eosinophils to induce allergic inflammation.
IL-33 in the Development of Allergy During Early Life
Recent murine studies have reported that there is a spontaneous accumulation of ILC2s, eosinophils, basophils and mast cells in the developing lung soon after birth, which is IL-33 dependent (79). Moreover, IL-33 is produced from type 2 airway epithelial cells (AEC2) during postnatal lung inflation (80). Large amount of IL-13 secreted from IL-33-activated ILC2 has been shown to polarize alveolar macrophages (AM) to anti-inflammatory M2 phenotype in newborn mice and contributed to lung quiescence in homeostasis with a delay in antibacterial effector responses for lifetime (80). On the other hand, exposure of allergen house dust mite during postnatal lung alveolarization further enhanced subsequent IL-33-induced Th2 cytokine production in activated ILC2s and CD11b+ dendritic cells (79, 81). Moreover, IL-33 inhibited IL-12 production and stimulated OX40L in neonatal dendritic cells, thereby promoting Th2 cell predominant for lung remodeling (79). House dust mites (HDM)-induced long-lasting Th2 immune response could be significantly neutralized by the intraperitoneal injection with recombinant soluble IL-33 decoy receptor in sensitization phase (79). This suppressive effect was even more significant in mice of young age than that of adult. Therefore, IL-33-ST2 axis is crucial for asthma development at childhood and intervention of such allergic axis is beneficial for the prevention of the later development of allergic asthma (79). Similarly, IL-33 concentration was found to be increased in the airways after exposure to Staphylococcus aureus–derived serine protease–like protein D (82).
Recent Study of IL-33 and AD
Severe pruritus and skin inflammation are the main manifestations of poison ivy-induced allergic contact dermatitis (ACD). In a murine study, the central role of IL-33/ST2 signaling in pruritus and skin inflammation of this ACD has been illustrated (83), and the pruritic mechanism is associated with the interaction of IL-33/ST2 signaling with primary sensory neurons. Therefore, blockage of IL-33/ST2 signaling may represent a therapeutic target to relieve pruritus and skin inflammation of IL-33/ST2 signaling-related dermatitis (83). Apart from pruritic conditions, it has been shown that IL-33 are involved in boosting pain in a formalin-induced inflammatory pain mice model (84).
IL-33 and Inflammatory Bowel Diseases (IBD)
Inflammatory bowel disease (IBD) is highly complex immune mediated sickness and mainly involved two disorders: Crohn's disease and ulcerative colitis with unclear pathophysiology. However, there are some clinical and pathophysiological similarities between IBD with asthma and non-pulmonary allergic diseases such as mast cell activity and the involvement of IgE (85). Moreover, upregulation of IL-33 and ST2 has been repeatedly demonstrated in the inflamed intestinal mucosa of IBD (86–90). Elevated IL-33 serum level of IBD patient has been shown to be reversed after anti-TNF-α treatment (87, 90). Different from asthma in which the AAM polarization is pro-inflammatory, IL-33 could prime macrophages into AAM in murine TNBS-induced colitis for inhibiting disease activity and the release of inflammatory mediators (91).
IL-33 as The New Therapeutic Target for Allergic Diseases
Production of pro-inflammatory cytokines induced by IL-33 from ST2-expressing structural cells and hematopoietic cells including ILC2s, mast cells, Th2 cells, eosinophils, basophils, dendritic cells, and alternatively activated macrophages (AAM) is crucial to provoke atopic diseases such as allergic asthma and AD (83, 92, 93). In vivo murine studies with IL-33- and ST2-deficient transgenic mice, together with the analysis of patient samples further support the crucial role of the IL-33/ST2 axis in those allergic conditions (7, 33, 94, 95). Therefore, IL-33-blocking agents may be a novel therapeutic modality to treat allergic diseases and some promising compounds have recently been developed. Conventionally, glucocorticoids suppress the mRNA expression of pro-inflammatory mediators and exert broadly suppressive activities on inflammatory reactions via binding to the glucocorticoid receptors. IL-33-mediated pulmonary inflammation can be glucocorticoid resistant because other cytokines such as TSLP and IL-17 synergistically expressed at local inflammatory sites (50, 96). For example, allergic airway IL-33 production in house dust mite-induced murine asthmatic model was found to be corticosteroid-resistant (96). Compared with healthy controls, serum levels of IL-33 were significantly increased in psoriasis, psoriatic arthritis, and pustular psoriasis patients, and related to TNF-α. Anti-TNF-α therapy may also be effective against IL-33-related diseases (97). As the production of IL-33 is regulated by the upstream activation of ERK1/2, ERK1/2 inhibitors have also been shown to suppress the IL-33 production (98). The activation of β2-receptors and protein kinase A (PKA) could promote the IL-33 mRNA expression in dendritic cells, thereby suggesting that β-receptor blockers and PKA inhibitors may also be the candidates for IL-33–blocking agents (99, 100). Using mice model, butyrate has recently been found to inhibit proliferation and function of ILC2s by inhibiting intracellular GATA3 activity to suppress IL-33-mediated airway hyperresponsiveness and airway inflammation (101). Similar observations were found in human ILC2s, both in vivo and in vitro (101).
Proteases play important role in IL-33-mediated allergic diseases. Mast cell proteases are capable to cleave full length IL-33 to a more active IL-33 domain. It becomes a potential therapeutic target for IL-33 mediated allergic diseases. Endogenous protease inhibitors (cystatin A and SPINK5) have been shown to protect the airway epithelium from exogenous protease of patients with eosinophilic chronic rhinosinusitis (102). The development of protease inhibitor may exert therapeutic benefit in eosinophilic airway diseases.
The prolyl cis-trans isomerase proteinase inhibitor I (PIN1) is known to abnormally induce cytokines for eosinophil survival and activation by stabilizing cytokine mRNAs (103). Interleukin receptor associated kinase M (IRAK-M) is a PIN1 target critical for IL-33 signaling in allergic asthma (104). Nuclear magnetic resonance analysis with docking simulations suggests that PIN1 might regulate IRAK-M conformation and function in IL-33 signaling. The IL-33/ST2 signaling pathway recruits adapter protein MyD88 to transduce intracellular signaling (105, 106). MyD88 forms a complex with IL-R–associated kinases (IRAKs), IRAK4, and IRAK2, called the myddosome (MyD88–IRAK4–IRAK2). The myddosome subsequently activates downstream NF-kB, p38 MAPK, and JNK. A small synthetic molecule mimetics of α-helical domain of IRAK2 called compound 7004, which can inhibit the IL-33–induced NF-kB activity, disrupt myddosome formation, and attenuate the pro-inflammatory effects in an asthma-like animal model (105).
Traditional Chinese Medicines (TCM) Targeting IL-33/ST2 Axis Against Allergic Inflammatory Diseases
Apart from the small molecules with specific target in the IL-33/ST2 axis as mentioned above, blocking IL-33 and its receptor by monoclonal antibodies is the major therapeutic approach in targeting IL-33/ST2 axis of allergic inflammatory diseases, and serval clinical trials are in progress (105, 107–111). The main side effect of monoclonal antibody administration is the risk of immune reactions such as serum sickness and acute anaphylaxis which may be fatal (112, 113). TCM and natural products may provide a great source of blockings agents against IL-33 activities. Some TCM formulae have been shown to be effective in attenuating IL-33 activities in both in vitro and in vivo studies (Table 1). Most of the component herbs in those formulae have been traditionally used to treat allergic and inflammatory diseases (39, 43, 127, 128).
Besides TCM formulae, some natural compounds include flavonoids and alkaloids have been shown to be active against targeting IL-33/ST2 axis. Calycosin, a flavonoid, is a major component in Radix Astragli (117) that has been used in the treatment of allergy-related symptoms. When AD mice were treated with calycosin (0.4–10 mg/kg), the protein levels of TSLP and IL-33 were significantly suppressed (118). The inhibitory mechanism was associated to TLR4-mediated NF-κB signaling, with the significant inhibition of the expression of MyD88, toll/interleukin-1 receptor domain-containing adapter protein (TIRAP), and transforming growth factor beta-activated kinase 1 (TAK1) (118).
Cimifugin is a bioactive and major component of Radix Saposhnikoviae, a TCM has been used for treating allergy. Using FITC sensitized and challenged AD mice, cimifugin can significantly inhibit TSLP and IL-33 production in the initial stage of AD model. Moreover, cimifugin could reduce the separated gap among the epithelial cells and increase the expression of tight junctions (TJs). Similar effects on TSLP/IL-33 and TJs were obtained using keratinocyte HaCaT cells. Using siRNA blockage, cimifugin was found to inhibit initiative cytokines through restoring TJs. In addition, cimifugin administered alone in the initial stage obviously attenuated the ultimate allergic inflammation, thereby indicating the sufficient impact of cimifugin in the initial stage on TSLP/IL-33 and TJs for suppressing allergic inflammation. This study therefore implies the possibility of key cytokines such as IL-33 and TJs can be the therapeutic targets for AD (119).
Eupatilin (5,7-dihydroxy-30,40,6-trimethoxyflavone) is the major lipophilic flavonoid isolated from the Artemisia species (120). Eupatilin has been shown to promote the transcriptional activity and expression of peroxisome proliferator-activated receptor α (PPARα) in keratinocyte HaCaT cells (121) and acts as an agonist of PPARα to ameliorate atopic dermatitis (AD) and restore the skin barrier function. Eupatilin (20 ml of 1.5% or 3.0%) improved AD-like symptoms in an oxazolone-induced AD-like mouse model by suppressing the serum levels of IgE, IL-4, and AD-related cytokines including TNFα, IFN-γ, IL-1β, TSLP, IL-25, and IL-33 (122).
Protostemonine (PSN), an alkaloid isolated from Radix Stemonae was found to suppress inflammatory conditions, IL-33 production and polarization of macrophage into AAM phenotype in the lung tissues of a dust mites, ragweed and aspergillus-induced murine asthma model (123).
Tetramethoxyluteolin (methlut), a natural flavonoid, has been shown to inhibit mast cells stimulated by IL-33, substance P, or their combination. This has been further validated in a clinical trial in which a skin lotion containing tetramethoxyluteolin that can reduce skin inflammation in AD patients. In experimental study, methlut has also been shown to be effective in psoriasis conditions (124, 125).
Apart from Chinese herbs and natural products, acupuncture seems to be effective in attenuating the IL-33 associated airway inflammation in an OVA-induced mouse model by reducing the serum concentrations of IL-33, sST2, and other inflammatory cytokines (129). In summary, these TCM formulae and natural compounds could lower the IL-33 production and other inflammatory cytokines from the tested targets, thereby ameliorating the allergic symptoms. Some of them could target on the specific IL-33 associated immune cells type such as IL-33-mediated mast cell activation and macrophage polarization into AAM phenotypes (Table 1). In view of limited clinical evidence and laboratory studies on the action mechanism, further investigations on these two aspects are essential for the future development of TCM in IL-33-related diseases (126).
Potential Future Developments and Concluding Remarks
With ample experimental evidences, the multiple roles of IL-33 in allergic and inflammatory diseases are not only restricted as an alarmin, but also as a cytokine for additional stimulatory signals: (i) to increase IL-33 expression in the nucleus or cytoplasm, and (ii) to induce IL-33 production into the extracellular space without cell death (29). Those stimulatory signals provide an amplification system for IL-33–mediated inflammatory responses. IL-33–blocking agents which target precisely at different molecular levels (both signaling and amplification pathways) could be potential therapeutic drugs for treatment of allergic and inflammatory diseases. For instance, an important mechanism for the direct activation of IL-33 by proteases from environmental allergens has been recently discovered (30). Targeting the “sensor” domain to prevent the cleavage and activation of IL-33FL, as well as the mast cell protease inhibitors might represent a new approach for reducing allergic responses in asthma and other allergic diseases. Apart from the pathological role in allergic diseases, IL-33 participates in diverse immune regulatory events. Therefore, to optimize the therapeutic outcomes, further evaluations, are essential to manipulate the IL-33/ST2 axis in diseases state and regulatory/physiological roles (130). As with other immunomodulating therapies, investigations on the effect of attenuating IL-33/ST2 axis on immune defense against infection and other immune responses are essential before further therapeutic development (131).
Since most of previous studies on IL-33 blocking agents are at the stage of in vitro and animal testing, pharmacological evaluations to develop IL-33–blocking agents are still on-going (132) and some are in phase I–II clinical trials for asthma and chronic obstructive pulmonary disease (133). The combination of IL-33 blocking agents may also be the synergistic intervention in IL-33-associated allergic and inflammatory diseases. For the future translational elucidation of IL-33, human studies are essential such as large scale clinical trials. Furthermore, the IL-33/ST2 axis is participating in both Th2/IL-31 and Th17 immune response during the progression of allergic airway diseases (92). Natural products and herbal medicines with the pluripotent activities to inhibit the production and actions of IL-33 are also promising candidates for further pharmacological evaluation for the treatment of allergic diseases. TCM and natural products, especially flavonoids with proven in vitro and in vivo activities to target the IL-33/ST2 axis, are potential candidates and warrant further development for the lead compounds as adjuvant anti-allergic and anti-inflammatory agents.
Author Contributions
CW and BC wrote the manuscript and prepared the figure. L-ST, CL, and CW drafted sections, structured, and edited the manuscript.
Funding
This study was supported by funding of the State Key Laboratory of Research on Bioactivities and Clinical Applications of Medicinal Plants (The Chinese University of Hong Kong/CUHK) from Innovation and Technology Commission and CUHK.
Conflict of Interest Statement
The authors declare that the research was conducted in the absence of any commercial or financial relationships that could be construed as a potential conflict of interest.
References
1. Schmitz J, Owyang A, Oldham E, Song Y, Murphy E, McClanahan TK, et al. IL-33, an interleukin-1-like cytokine that signals via the IL-1 receptor-related protein ST2 and induces T helper type 2-associated cytokines. Immunity. (2005) 23:479–90. doi: 10.1016/j.immuni.2005.09.015
2. Moussion C, Ortega N, Girard JP. The IL-1-like cytokine IL-33 is constitutively expressed in the nucleus of endothelial cells and epithelial cells in vivo: a novel 'alarmin'? PLoS ONE. (2008) 3:e3331. doi: 10.1371/journal.pone.0003331
3. Carriere V, Roussel L, Ortega N, Lacorre DA, Americh L, Aguilar L, et al. IL-33, the IL-1-like cytokine ligand for ST2 receptor, is a chromatin-associated nuclear factor in vivo. Proc Natl Acad Sci USA. (2007) 104:282–7. doi: 10.1073/pnas.0606854104
4. Haraldsen G, Balogh J, Pollheimer J, Sponheim J, Kuchler AM. Interleukin-33 - cytokine of dual function or novel alarmin? Trends Immunol. (2009) 30:227–33. doi: 10.1016/j.it.2009.03.003
5. Cayrol C, Girard JP. The IL-1-like cytokine IL-33 is inactivated after maturation by caspase-1. Proc Natl Acad Sci USA. (2009) 106:9021–6. doi: 10.1073/pnas.0812690106
6. Cayrol C, Girard JP. IL-33: an alarmin cytokine with crucial roles in innate immunity, inflammation and allergy. Curr Opin Immunol. (2014) 31:31–7. doi: 10.1016/j.coi.2014.09.004
7. Cayrol C, Girard JP. Interleukin-33 (IL-33): a nuclear cytokine from the IL-1 family. Immunol Rev. (2018) 281:154–68. doi: 10.1111/imr.12619
8. Xu D, Chan WL, Leung BP, Huang F, Wheeler R, Piedrafita D, et al. Selective expression of a stable cell surface molecule on type 2 but not type 1 helper T cells. J Exp Med. (1998) 187:787–94.
9. Bonilla WV, Frohlich A, Senn K, Kallert S, Fernandez M, Johnson S, et al. The alarmin interleukin-33 drives protective antiviral CD8(+) T cell responses. Science. (2012) 335:984–9. doi: 10.1126/science.1215418
10. Moritz DR, Rodewald HR, Gheyselinck J, Klemenz R. The IL-1 receptor-related T1 antigen is expressed on immature and mature mast cells and on fetal blood mast cell progenitors. J Immunol. (1998) 161:4866–74.
11. Moro K, Yamada T, Tanabe M, Takeuchi T, Ikawa T, Kawamoto H, et al. Innate production of T(H)2 cytokines by adipose tissue-associated c-Kit(+)Sca-1(+) lymphoid cells. Nature. (2010) 463:540–4. doi: 10.1038/nature08636
12. Neill DR, Wong SH, Bellosi A, Flynn RJ, Daly M, Langford TK, et al. Nuocytes represent a new innate effector leukocyte that mediates type-2 immunity. Nature. (2010) 464:1367–70. doi: 10.1038/nature08900
13. Price AE, Liang HE, Sullivan BM, Reinhardt RL, Eisley CJ, Erle DJ, et al. Systemically dispersed innate IL-13-expressing cells in type 2 immunity. Proc Natl Acad Sci USA. (2010) 107:11489–94. doi: 10.1073/pnas.1003988107
14. Rank MA, Kobayashi T, Kozaki H, Bartemes KR, Squillace DL, Kita H. IL-33-activated dendritic cells induce an atypical TH2-type response. J Allergy Clin Immunol. (2009) 123:1047–54. doi: 10.1016/j.jaci.2009.02.026
15. Pecaric-Petkovic T, Didichenko SA, Kaempfer S, Spiegl N, Dahinden CA. Human basophils and eosinophils are the direct target leukocytes of the novel IL-1 family member IL-33. Blood. (2009) 113:1526–34. doi: 10.1182/blood-2008-05-157818
16. Bourgeois E, Van LP, Samson M, Diem S, Barra A, Roga S, et al. The pro-Th2 cytokine IL-33 directly interacts with invariant NKT and NK cells to induce IFN-gamma production. Eur J Immunol. (2009) 39:1046–55. doi: 10.1002/eji.200838575
17. Nabekura T, Girard JP, Lanier LL. IL-33 receptor ST2 amplifies the expansion of NK cells and enhances host defense during mouse cytomegalovirus infection. J Immunol. (2015) 194:5948–52. doi: 10.4049/jimmunol.1500424
18. Smith DE. The biological paths of IL-1 family members IL-18 and IL-33. J Leukoc Biol. (2011) 89:383–92. doi: 10.1189/jlb.0810470
19. Arend WP, Palmer G, Gabay C. IL-1, IL-18, and IL-33 families of cytokines. Immunol Rev. (2008) 223:20–38. doi: 10.1111/j.1600-065X.2008.00624.x
20. Pushparaj PN, Tay HK, H'Ng S C, Pitman N, Xu D, McKenzie A, et al. The cytokine interleukin-33 mediates anaphylactic shock. Proc Natl Acad Sci USA. (2009) 106:9773–8. doi: 10.1073/pnas.0901206106
21. Smith DE. IL-33: a tissue derived cytokine pathway involved in allergic inflammation and asthma. Clin Exp Allergy. (2010) 40:200–8. doi: 10.1111/j.1365-2222.2009.03384.x
22. Komai-Koma M, Xu D, Li Y, McKenzie AN, McInnes IB, Liew FY. IL-33 is a chemoattractant for human Th2 cells. Eur J Immunol. (2007) 37:2779–86. doi: 10.1002/eji.200737547
23. Savenije OE, Mahachie John JM, Granell R, Kerkhof M, Dijk FN, de Jongste JC, et al. Association of IL33-IL-1 receptor-like 1 (IL1RL1) pathway polymorphisms with wheezing phenotypes and asthma in childhood. J Allergy Clin Immunol. (2014) 134:170–7. doi: 10.1016/j.jaci.2013.12.1080
24. Makrinioti H, Toussaint M, Jackson DJ, Walton RP, Johnston SL. Role of interleukin 33 in respiratory allergy and asthma. Lancet Respir Med. (2014) 2:226–37. doi: 10.1016/S2213-2600(13)70261-3
25. Moffatt MF, Gut IG, Demenais F, Strachan DP, Bouzigon E, Heath S, et al. A large-scale, consortium-based genomewide association study of asthma. N Engl J Med. (2010) 363:1211–21. doi: 10.1056/NEJMoa0906312
26. Torgerson DG, Ampleford EJ, Chiu GY, Gauderman WJ, Gignoux CR, Graves PE, et al. Meta-analysis of genome-wide association studies of asthma in ethnically diverse North American populations. Nat Genet. (2011) 43:887–92. doi: 10.1038/ng.888
27. Molofsky AB, Savage AK, Locksley RM. Interleukin-33 in tissue homeostasis, injury, and inflammation. Immunity. (2015) 42:1005–19. doi: 10.1016/j.immuni.2015.06.006
28. Cohen ES, Scott IC, Majithiya JB, Rapley L, Kemp BP, England E, et al. Oxidation of the alarmin IL-33 regulates ST2-dependent inflammation. Nat Commun. (2015) 6:8327. doi: 10.1038/ncomms9327
29. Morita H, Nakae S, Saito H, Matsumoto K. IL-33 in clinical practice: size matters? J Allergy Clin Immunol. (2017) 140:381–3. doi: 10.1016/j.jaci.2017.03.042
30. Cayrol C, Duval A, Schmitt P, Roga S, Camus M, Stella A, et al. Environmental allergens induce allergic inflammation through proteolytic maturation of IL-33. Nat Immunol. (2018) 19:375–85. doi: 10.1038/s41590-018-0067-5
31. Luthi AU, Cullen SP, McNeela EA, Duriez PJ, Afonina IS, Sheridan C, et al. Suppression of interleukin-33 bioactivity through proteolysis by apoptotic caspases. Immunity. (2009) 31:84–98. doi: 10.1016/j.immuni.2009.05.007
32. Kondo Y, Yoshimoto T, Yasuda K, Futatsugi-Yumikura S, Morimoto M, Hayashi N, et al. Administration of IL-33 induces airway hyperresponsiveness and goblet cell hyperplasia in the lungs in the absence of adaptive immune system. Int Immunol. (2008) 20:791–800. doi: 10.1093/intimm/dxn037
33. Mitchell PD, Salter BM, Oliveria JP, El-Gammal A, Tworek D, Smith SG, et al. IL-33 and its receptor ST2 after inhaled allergen challenge in allergic asthmatics. Int Arch Allergy Immunol. (2018) 176:133–42. doi: 10.1159/000488015
34. Cherry WB, Yoon J, Bartemes KR, Iijima K, Kita H. A novel IL-1 family cytokine, IL-33, potently activates human eosinophils. J Allergy Clin Immunol. (2008) 121:1484–90. doi: 10.1016/j.jaci.2008.04.005
35. Suzukawa M, Koketsu R, Iikura M, Nakae S, Matsumoto K, Nagase H, et al. Interleukin-33 enhances adhesion, CD11b expression and survival in human eosinophils. Lab Invest. (2008) 88:1245–53. doi: 10.1038/labinvest.2008.82
36. Gudbjartsson DF, Bjornsdottir US, Halapi E, Helgadottir A, Sulem P, Jonsdottir GM, et al. Sequence variants affecting eosinophil numbers associate with asthma and myocardial infarction. Nat Genet. (2009) 41:342–7. doi: 10.1038/ng.323
37. Wong CK, Cheung PF, Lam CW. Leptin-mediated cytokine release and migration of eosinophils: implications for immunopathophysiology of allergic inflammation. Eur J Immunol. (2007) 37:2337–48. doi: 10.1002/eji.200636866
38. Ip WK, Wong CK, Li ML, Li PW, Cheung PF, Lam CW. Interleukin-31 induces cytokine and chemokine production from human bronchial epithelial cells through activation of mitogen-activated protein kinase signalling pathways: implications for the allergic response. Immunology. (2007) 122:532–41. doi: 10.1111/j.1365-2567.2007.02668.x
39. Leung TF, Wong KY, Wong CK, Fung KP, Lam CW, Fok TF, et al. In vitro and clinical immunomodulatory effects of a novel Pentaherbs concoction for atopic dermatitis. Br J Dermatol. (2008) 158:1216–23. doi: 10.1111/j.1365-2133.2008.08502.x
40. Wong CK, Ng SS, Lun SW, Cao J, Lam CW. Signalling mechanisms regulating the activation of human eosinophils by mast-cell-derived chymase: implications for mast cell-eosinophil interaction in allergic inflammation. Immunology. (2009) 126:579–87. doi: 10.1111/j.1365-2567.2008.02916.x
41. Chow JY, Wong CK, Cheung PF, Lam CW. Intracellular signaling mechanisms regulating the activation of human eosinophils by the novel Th2 cytokine IL-33: implications for allergic inflammation. Cell Mol Immunol. (2010) 7:26–34. doi: 10.1038/cmi.2009.106
42. Wong CK, Leung KM, Qiu HN, Chow JY, Choi AO, Lam CW. Activation of eosinophils interacting with dermal fibroblasts by pruritogenic cytokine IL-31 and alarmin IL-33: implications in atopic dermatitis. PLoS ONE. (2012) 7:e29815. doi: 10.1371/journal.pone.0029815
43. Liu KY, Hu S, Chan BC, Wat EC, Lau CB, Hon KL, et al. Anti-inflammatory and anti-allergic activities of Pentaherb formula, Moutan Cortex (Danpi) and gallic acid. Molecules. (2013) 18:2483–500. doi: 10.3390/molecules18032483
44. Jiao D, Wong CK, Qiu HN, Dong J, Cai Z, Chu M, et al. NOD2 and TLR2 ligands trigger the activation of basophils and eosinophils by interacting with dermal fibroblasts in atopic dermatitis-like skin inflammation. Cell Mol Immunol. (2016) 13:535–50. doi: 10.1038/cmi.2015.77
45. Cheung PF, Wong CK, Ho AW, Hu S, Chen DP, Lam CW. Activation of human eosinophils and epidermal keratinocytes by Th2 cytokine IL-31: implication for the immunopathogenesis of atopic dermatitis. Int Immunol. (2010) 22:453–67. doi: 10.1093/intimm/dxq027
46. Chen CC, Kobayashi T, Iijima K, Hsu FC, Kita H. IL-33 dysregulates regulatory T cells and impairs established immunologic tolerance in the lungs. J Allergy Clin Immunol. (2017) 140:1351–63.e7. doi: 10.1016/j.jaci.2017.01.015
47. Baumann C, Bonilla WV, Frohlich A, Helmstetter C, Peine M, Hegazy AN, et al. T-bet- and STAT4-dependent IL-33 receptor expression directly promotes antiviral Th1 cell responses. Proc Natl Acad Sci USA. (2015) 112:4056–61. doi: 10.1073/pnas.1418549112
48. Halim TY, Steer CA, Matha L, Gold MJ, Martinez-Gonzalez I, McNagny KM, et al. Group 2 innate lymphoid cells are critical for the initiation of adaptive T helper 2 cell-mediated allergic lung inflammation. Immunity. (2014) 40:425–35. doi: 10.1016/j.immuni.2014.01.011
49. Oliphant CJ, Hwang YY, Walker JA, Salimi M, Wong SH, Brewer JM, et al. MHCII-mediated dialog between group 2 innate lymphoid cells and CD4(+) T cells potentiates type 2 immunity and promotes parasitic helminth expulsion. Immunity. (2014) 41:283–95. doi: 10.1016/j.immuni.2014.06.016
50. Hirahara K, Mato N, Hagiwara K, Nakayama T. The pathogenicity of IL-33 on steroid-resistant eosinophilic inflammation via the activation of memory-type ST2(+) CD4(+) T cells. J Leukoc Biol. (2018) 104:895–901. doi: 10.1002/JLB.MR1117-456R
51. Drake LY, Kita H. Group 2 innate lymphoid cells in the lung. Adv Immunol. (2014) 124:1–16. doi: 10.1016/B978-0-12-800147-9.00001-7
52. Brestoff JR, Kim BS, Saenz SA, Stine RR, Monticelli LA, Sonnenberg GF, et al. Group 2 innate lymphoid cells promote beiging of white adipose tissue and limit obesity. Nature. (2015) 519:242–6. doi: 10.1038/nature14115
53. Doherty TA, Khorram N, Lund S, Mehta AK, Croft M, Broide DH. Lung type 2 innate lymphoid cells express cysteinyl leukotriene receptor 1, which regulates TH2 cytokine production. J Allergy Clin Immunol. (2013) 132:205–13. doi: 10.1016/j.jaci.2013.03.048
54. Liu T, Barrett NA, Kanaoka Y, Yoshimoto E, Garofalo D, Cirka H, et al. Type 2 Cysteinyl leukotriene receptors drive IL-33-dependent type 2 immunopathology and aspirin sensitivity. J Immunol. (2018) 200:915–27. doi: 10.4049/jimmunol.1700603
55. Yazdani R, Sharifi M, Shirvan AS, Azizi G, Ganjalikhani-Hakemi M. Characteristics of innate lymphoid cells (ILCs) and their role in immunological disorders (an update). Cell Immunol. (2015) 298:66–76. doi: 10.1016/j.cellimm.2015.09.006
56. Lund S, Walford HH, Doherty TA. Type 2 innate lymphoid cells in allergic disease. Curr Immunol Rev. (2013) 9:214–21. doi: 10.2174/1573395510666140304235916
57. Johansson K, Malmhall C, Ramos-Ramirez P, Radinger M. Bone marrow type 2 innate lymphoid cells: a local source of interleukin-5 in interleukin-33-driven eosinophilia. Immunology. (2018) 153:268–78. doi: 10.1111/imm.12842
58. Yu Y, Blokhuis BR, Garssen J, Redegeld FA. Non-IgE mediated mast cell activation. Eur J Pharmacol. (2016) 778:33–43. doi: 10.1016/j.ejphar.2015.07.017
59. Jang TY, Kim YH. Interleukin-33 and mast cells bridge innate and adaptive immunity: from the allergologist's perspective. Int Neurourol J. (2015) 19:142–50. doi: 10.5213/inj.2015.19.3.142
60. Mukai K, Tsai M, Saito H, Galli SJ. Mast cells as sources of cytokines, chemokines, and growth factors. Immunol Rev. (2018) 282:121–50. doi: 10.1111/imr.12634
61. Caughey GH. Mast cell proteases as pharmacological targets. Eur J Pharmacol. (2016) 778:44–55. doi: 10.1016/j.ejphar.2015.04.045
62. Roy A, Ganesh G, Sippola H, Bolin S, Sawesi O, Dagalv A, et al. Mast cell chymase degrades the alarmins heat shock protein 70, biglycan, HMGB1, and interleukin-33 (IL-33) and limits danger-induced inflammation. J Biol Chem. (2014) 289:237–50. doi: 10.1074/jbc.M112.435156
63. Galand C, Leyva-Castillo JM, Yoon J, Han A, Lee MS, McKenzie ANJ, et al. IL-33 promotes food anaphylaxis in epicutaneously sensitized mice by targeting mast cells. J Allergy Clin Immunol. (2016) 138:1356–66. doi: 10.1016/j.jaci.2016.03.056
64. Zoltowska AM, Lei Y, Fuchs B, Rask C, Adner M, Nilsson GP. The interleukin-33 receptor ST2 is important for the development of peripheral airway hyperresponsiveness and inflammation in a house dust mite mouse model of asthma. Clin Exp Allergy. (2016) 46:479–90. doi: 10.1111/cea.12683
65. Liu T, Kanaoka Y, Barrett NA, Feng C, Garofalo D, Lai J, et al. Aspirin-exacerbated respiratory disease involves a Cysteinyl leukotriene-driven IL-33-mediated mast cell activation pathway. J Immunol. (2015) 195:3537–45. doi: 10.4049/jimmunol.1500905
66. Olivera A, Beaven MA, Metcalfe DD. Mast cells signal their importance in health and disease. J Allergy Clin Immunol. (2018) 142:381–93 doi: 10.1016/j.jaci.2018.01.034
67. Bandara G, Beaven MA, Olivera A, Gilfillan AM, Metcalfe DD. Activated mast cells synthesize and release soluble ST2-a decoy receptor for IL-33. Eur J Immunol. (2015) 45:3034–44. doi: 10.1002/eji.201545501
68. Wang JX, Kaieda S, Ameri S, Fishgal N, Dwyer D, Dellinger A, et al. IL-33/ST2 axis promotes mast cell survival via BCLXL. Proc Natl Acad Sci USA. (2014) 111:10281–6. doi: 10.1073/pnas.1404182111
69. Gordon ED, Simpson LJ, Rios CL, Ringel L, Lachowicz-Scroggins ME, Peters MC, et al. Alternative splicing of interleukin-33 and type 2 inflammation in asthma. Proc Natl Acad Sci USA. (2016) 113:8765–70. doi: 10.1073/pnas.1601914113
70. Fu Z, Thorpe M, Alemayehu R, Roy A, Kervinen J, de Garavilla L, et al. Highly Selective cleavage of cytokines and chemokines by the human mast cell chymase and neutrophil cathepsin G. J Immunol. (2017) 198:1474–83. doi: 10.4049/jimmunol.1601223
71. Waern I, Jonasson S, Hjoberg J, Bucht A, Abrink M, Pejler G, et al. Mouse mast cell protease 4 is the major chymase in murine airways and has a protective role in allergic airway inflammation. J Immunol. (2009) 183:6369–76. doi: 10.4049/jimmunol.0900180
72. Jogie-Brahim S, Min HK, Fukuoka Y, Xia HZ, Schwartz LB. Expression of alpha-tryptase and beta-tryptase by human basophils. J Allergy Clin Immunol. (2004) 113:1086–92. doi: 10.1016/j.jaci.2004.02.032
73. Kroeger KM, Sullivan BM, Locksley RM. IL-18 and IL-33 elicit Th2 cytokines from basophils via a MyD88- and p38alpha-dependent pathway. J Leukoc Biol. (2009) 86:769–78. doi: 10.1189/jlb.0708452
74. Locksley RM. Asthma and allergic inflammation. Cell. (2010) 140:777–83. doi: 10.1016/j.cell.2010.03.004
75. Melgert BN, ten Hacken NH, Rutgers B, Timens W, Postma DS, Hylkema MN. More alternative activation of macrophages in lungs of asthmatic patients. J Allergy Clin Immunol. (2011) 127:831–3. doi: 10.1016/j.jaci.2010.10.045
76. Lee YG, Jeong JJ, Nyenhuis S, Berdyshev E, Chung S, Ranjan R, et al. Recruited alveolar macrophages, in response to airway epithelial-derived monocyte chemoattractant protein 1/CCl2, regulate airway inflammation and remodeling in allergic asthma. Am J Respir Cell Mol Biol. (2015) 52:772–84. doi: 10.1165/rcmb.2014-0255OC
77. Kurowska-Stolarska M, Stolarski B, Kewin P, Murphy G, Corrigan CJ, Ying S, et al. IL-33 amplifies the polarization of alternatively activated macrophages that contribute to airway inflammation. J Immunol. (2009) 183:6469–77. doi: 10.4049/jimmunol.0901575
78. Espinassous Q, Garcia-de-Paco E, Garcia-Verdugo I, Synguelakis M, von Aulock S, Sallenave JM, et al. IL-33 enhances lipopolysaccharide-induced inflammatory cytokine production from mouse macrophages by regulating lipopolysaccharide receptor complex. J Immunol. (2009) 183:1446–55. doi: 10.4049/jimmunol.0803067
79. de Kleer IM, Kool M, de Bruijn MJ, Willart M, van Moorleghem J, Schuijs MJ, et al. Perinatal activation of the interleukin-33 pathway promotes type 2 immunity in the developing lung. Immunity. (2016) 45:1285–98. doi: 10.1016/j.immuni.2016.10.031
80. Saluzzo S, Gorki AD, Rana BMJ, Martins R, Scanlon S, Starkl P, et al. First-breath-induced type 2 pathways shape the lung immune environment. Cell Rep. (2017) 18:1893–905. doi: 10.1016/j.celrep.2017.01.071
81. Mindt BC, Fritz JH, Duerr CU. Group 2 innate lymphoid cells in pulmonary immunity and tissue homeostasis. Front Immunol. (2018) 9:840. doi: 10.3389/fimmu.2018.00840
82. Teufelberger AR, Nordengrun M, Braun H, Maes T, De Grove K, Holtappels G, et al. The IL-33/ST2 axis is crucial in type 2 airway responses induced by Staphylococcus aureus-derived serine protease-like protein D. J Allergy Clin Immunol. (2018) 141:549–59.e7. doi: 10.1016/j.jaci.2017.05.004
83. Liu B, Tai Y, Achanta S, Kaelberer MM, Caceres AI, Shao X, et al. IL-33/ST2 signaling excites sensory neurons and mediates itch response in a mouse model of poison ivy contact allergy. Proc Natl Acad Sci USA. (2016) 113:E7572–9. doi: 10.1073/pnas.1606608113
84. Han P, Zhao J, Liu SB, Yang CJ, Wang YQ, Wu GC, et al. Interleukin-33 mediates formalin-induced inflammatory pain in mice. Neuroscience. (2013) 241:59–66. doi: 10.1016/j.neuroscience.2013.03.019
85. Kotlyar DS, Shum M, Hsieh J, Blonski W, Greenwald DA. Non-pulmonary allergic diseases and inflammatory bowel disease: a qualitative review. World J Gastroenterol. (2014) 20:11023–32. doi: 10.3748/wjg.v20.i32.11023
86. Seidelin JB, Bjerrum JT, Coskun M, Widjaya B, Vainer B, Nielsen OH. IL-33 is upregulated in colonocytes of ulcerative colitis. Immunol Lett. (2010) 128:80–5. doi: 10.1016/j.imlet.2009.11.001
87. Beltran CJ, Nunez LE, Diaz-Jimenez D, Farfan N, Candia E, Heine C, et al. Characterization of the novel ST2/IL-33 system in patients with inflammatory bowel disease. Inflamm Bowel Dis. (2010) 16:1097–107. doi: 10.1002/ibd.21175
88. Pastorelli L, Garg RR, Hoang SB, Spina L, Mattioli B, Scarpa M, et al. Epithelial-derived IL-33 and its receptor ST2 are dysregulated in ulcerative colitis and in experimental Th1/Th2 driven enteritis. Proc Natl Acad Sci USA. (2010) 107:8017–22. doi: 10.1073/pnas.0912678107
89. Sponheim J, Pollheimer J, Olsen T, Balogh J, Hammarstrom C, Loos T, et al. Inflammatory bowel disease-associated interleukin-33 is preferentially expressed in ulceration-associated myofibroblasts. Am J Pathol. (2010) 177:2804–15. doi: 10.2353/ajpath.2010.100378
90. Nunes T, Bernardazzi C, de Souza HS. Interleukin-33 and inflammatory bowel diseases: lessons from human studies. Mediators Inflamm. (2014) 2014:423957. doi: 10.1155/2014/423957
91. Tu L, Chen J, Xu D, Xie Z, Yu B, Tao Y, et al. IL-33-induced alternatively activated macrophage attenuates the development of TNBS-induced colitis. Oncotarget. (2017) 8:27704–14. doi: 10.18632/oncotarget.15984
92. Vocca L, Di Sano C, Uasuf CG, Sala A, Riccobono L, Gangemi S, et al. IL-33/ST2 axis controls Th2/IL-31 and Th17 immune response in allergic airway diseases. Immunobiology. (2015) 220:954–63. doi: 10.1016/j.imbio.2015.02.005
93. Fattori V, Borghi SM, Verri WA Jr. IL-33/ST2 signaling boosts inflammation and pain. Proc Natl Acad Sci USA. (2017) 114:E10034–5. doi: 10.1073/pnas.1716120114
94. Khaitov MR, Gaisina AR, Shilovskiy IP, Smirnov VV, Ramenskaia GV, Nikonova AA, et al. The role of interleukin-33 in pathogenesis of bronchial asthma. New experimental data. Biochemistry. (2018) 83:13–25. doi: 10.1134/S0006297918010029
95. Johnston LK, Hsu CL, Krier-Burris RA, Chhiba KD, Chien KB, McKenzie A, et al. IL-33 precedes IL-5 in regulating eosinophil commitment and is required for eosinophil homeostasis. J Immunol. (2016) 197:3445–53. doi: 10.4049/jimmunol.1600611
96. Saglani S, Lui S, Ullmann N, Campbell GA, Sherburn RT, Mathie SA, et al. IL-33 promotes airway remodeling in pediatric patients with severe steroid-resistant asthma. J Allergy Clin Immunol. (2013) 132:676–85.e13. doi: 10.1016/j.jaci.2013.04.012
97. Balato A, Di Caprio R, Canta L, Mattii M, Lembo S, Raimondo A, et al. IL-33 is regulated by TNF-alpha in normal and psoriatic skin. Arch Dermatol Res. (2014) 306:299–304. doi: 10.1007/s00403-014-1447-9
98. Kobori A, Yagi Y, Imaeda H, Ban H, Bamba S, Tsujikawa T, et al. Interleukin-33 expression is specifically enhanced in inflamed mucosa of ulcerative colitis. J Gastroenterol. (2010) 45:999–1007. doi: 10.1007/s00535-010-0245-1
99. Yanagawa Y, Matsumoto M, Togashi H. Adrenoceptor-mediated enhancement of interleukin-33 production by dendritic cells. Brain Behav Immun. (2011) 25:1427–33. doi: 10.1016/j.bbi.2011.04.012
100. Nabe T. Interleukin (IL)-33: new therapeutic target for atopic diseases. J Pharmacol Sci. (2014) 126:85–91. doi: 10.1254/jphs.14R12CP
101. Thio CL, Chi PY, Lai AC, Chang YJ. Regulation of type 2 innate lymphoid cell-dependent airway hyperreactivity by butyrate. J Allergy Clin Immunol. (2018) 142:1867–83.e12. doi: 10.1016/j.jaci.2018.02.032
102. Kouzaki H, Matsumoto K, Kikuoka H, Kato T, Tojima I, Shimizu S, et al. Endogenous protease inhibitors in airway epithelial cells contribute to eosinophilic chronic rhinosinusitis. Am J Respir Crit Care Med. (2017) 195:737–47. doi: 10.1164/rccm.201603-0529OC
103. Shen ZJ, Esnault S, Malter JS. The peptidyl-prolyl isomerase Pin1 regulates the stability of granulocyte-macrophage colony-stimulating factor mRNA in activated eosinophils. Nat Immunol. (2005) 6:1280–7. doi: 10.1038/ni1266
104. Nechama M, Kwon J, Wei S, Kyi AT, Welner RS, Ben-Dov IZ, et al. The IL-33-PIN1-IRAK-M axis is critical for type 2 immunity in IL-33-induced allergic airway inflammation. Nat Commun. (2018) 9:1603. doi: 10.1038/s41467-018-03886-6
105. Li J, Saruta K, Dumouchel JP, Magat JM, Thomas JL, Ajami D, et al. Small molecule mimetics of alpha-helical domain of IRAK2 attenuate the proinflammatory effects of IL-33 in asthma-like mouse models. J Immunol. (2018) 200:4036–43. doi: 10.4049/jimmunol.1700693
106. Lin SC, Lo YC, Wu H. Helical assembly in the MyD88-IRAK4-IRAK2 complex in TLR/IL-1R signalling. Nature. (2010) 465:885–90. doi: 10.1038/nature09121
107. Park CS, Jang TY, Heo MJ, Jung AY, Kim YH. Antiallergic effects of anti-interleukin-33 are associated with suppression of immunoglobulin light chain and inducible nitric oxide synthase. Am J Rhinol Allergy. (2016) 30:17–22. doi: 10.2500/ajra.2015.29.4251
108. Fursov N, Lu J, Healy C, Wu SJ, Lacy E, Filer A, et al. Monoclonal antibodies targeting ST2L Domain 1 or Domain 3 differentially modulate IL-33-induced cytokine release by human mast cell and basophilic cell lines. Mol Immunol. (2016) 75:178–87. doi: 10.1016/j.molimm.2016.05.019
109. Ohta K, Nagase H, Suzukawa M, Ohta S. Antibody therapy for the management of severe asthma with eosinophilic inflammation. Int Immunol. (2017) 29:337–43. doi: 10.1093/intimm/dxx045
110. Katial RK, Bensch GW, Busse WW, Chipps BE, Denson JL, Gerber AN, et al. Changing paradigms in the treatment of severe asthma: the role of biologic therapies. J Allergy Clin Immunol Pract. (2017) 5:S1–14. doi: 10.1016/j.jaip.2016.11.029
111. Hodzic Z, Schill EM, Bolock AM, Good M. IL-33 and the intestine: the good, the bad, and the inflammatory. Cytokine. (2017) 100:1–10. doi: 10.1016/j.cyto.2017.06.017
112. Hansel TT, Kropshofer H, Singer T, Mitchell JA, George AJ. The safety and side effects of monoclonal antibodies. Nat Rev Drug Discov. (2010) 9:325–38. doi: 10.1038/nrd3003
113. Liu L, Li Y. The unexpected side effects and safety of therapeutic monoclonal antibodies. Drugs Today. (2014) 50:33–50. doi: 10.1358/dot.2014.50.1.2076506
114. Jeon WY, Shin HK, Shin IS, Kim SK, Lee MY. Soshiho-tang water extract inhibits ovalbumin-induced airway inflammation via the regulation of heme oxygenase-1. BMC Complement Altern Med. (2015) 15:329. doi: 10.1186/s12906-015-0857-3
115. Ohtake N, Nakai Y, Yamamoto M, Ishige A, Sasaki H, Fukuda K, et al. The herbal medicine Shosaiko-to exerts different modulating effects on lung local immune responses among mouse strains. Int Immunopharmacol. (2002) 2:357–66. doi: 10.1016/S1567-5769(01)00161-8
116. Chen M, Ding P, Yang L, He X, Gao C, Yang G, et al. Evaluation of anti-inflammatory activities of Qingre-Qushi Recipe (QRQS) against atopic dermatitis: potential mechanism of inhibition of IL-33/ST2 signal transduction. Evid Based Complement Alternat Med. (2017) 2017:2489842. doi: 10.1155/2017/2489842
117. Qiu R, Ma G, Zheng C, Qiu X, Li X, Li X, et al. Antineoplastic effect of calycosin on osteosarcoma through inducing apoptosis showing in vitro and in vivo investigations. Exp Mol Pathol. (2014) 97:17–22. doi: 10.1016/j.yexmp.2014.04.014
118. Tao Y, Wang Y, Wang X, Wang C, Bao K, Ji L, et al. Calycosin suppresses epithelial derived initiative key factors and maintains epithelial barrier in allergic inflammation via TLR4 mediated NF-kappaB pathway. Cell Physiol Biochem. (2017) 44:1106–19. doi: 10.1159/000485416
119. Wang X, Jiang X, Yu X, Liu H, Tao Y, Jiang G, et al. Cimifugin suppresses allergic inflammation by reducing epithelial derived initiative key factors via regulating tight junctions. J Cell Mol Med. (2017) 21:2926–36. doi: 10.1111/jcmm.13204
120. Giangaspero A, Ponti C, Pollastro F, Del Favero G, Della Loggia R, Tubaro A, et al. Topical anti-inflammatory activity of Eupatilin, a lipophilic flavonoid from mountain wormwood (Artemisia umbelliformis Lam.). J Agric Food Chem. (2009) 57:7726–30. doi: 10.1021/jf901725p
121. Jung Y, Kim JC, Choi Y, Lee S, Kang KS, Kim YK, et al. Eupatilin with PPARalpha agonistic effects inhibits TNFalpha-induced MMP signaling in HaCaT cells. Biochem Biophys Res Commun. (2017) 493:220–6. doi: 10.1016/j.bbrc.2017.09.043
122. Jung Y, Kim JC, Park NJ, Bong SK, Lee S, Jegal H, et al. Eupatilin, an activator of PPARalpha, inhibits the development of oxazolone-induced atopic dermatitis symptoms in Balb/c mice. Biochem Biophys Res Commun. (2018) 496:508–14. doi: 10.1016/j.bbrc.2018.01.098
123. Song Y, Wu Y, Li X, Shen Y, Ding Y, Zhu H, et al. Protostemonine attenuates alternatively activated macrophage and DRA-induced asthmatic inflammation. Biochem Pharmacol. (2018) 155:198–206. doi: 10.1016/j.bcp.2018.07.003
124. Weng Z, Patel AB, Panagiotidou S, Theoharides TC. The novel flavone tetramethoxyluteolin is a potent inhibitor of human mast cells. J Allergy Clin Immunol. (2015) 135:1044–52.e5. doi: 10.1016/j.jaci.2014.10.032
125. Theoharides TC, Stewart JM, Tsilioni I. Tolerability and benefit of a tetramethoxyluteolin-containing skin lotion. Int J Immunopathol Pharmacol. (2017) 30:146–51. doi: 10.1177/0394632017707610
126. Patel AB, Tsilioni I, Weng Z, Theoharides TC. TNF stimulates IL-6, CXCL8 and VEGF secretion from human keratinocytes via activation of mTOR, inhibited by tetramethoxyluteolin. Exp Dermatol. (2018) 27:135–43. doi: 10.1111/exd.13461
127. Tsang MS, Jiao D, Chan BC, Hon KL, Leung PC, Lau CB, et al. Anti-inflammatory activities of pentaherbs formula, berberine, gallic acid and chlorogenic acid in atopic dermatitis-like skin inflammation. Molecules. (2016) 21:519. doi: 10.3390/molecules21040519
128. Chan BC, Li LF, Hu SQ, Wat E, Wong EC, Zhang VX, et al. Gallic acid is the major active component of cortex moutan in inhibiting immune maturation of human monocyte-derived dendritic cells. Molecules. (2015) 20:16388–403. doi: 10.3390/molecules200916388
129. Dong M, Ma C, Wang WQ, Chen J, Wei Y. Regulation of the IL-33/ST2 pathway contributes to the anti-inflammatory effect of acupuncture in the ovalbumin-induced murine asthma model. Acupunct Med. (2018) 36:319–26. doi: 10.1136/acupmed-2017-011377
130. Chen WY, Tsai TH, Yang JL, Li LC. Therapeutic strategies for targeting IL-33/ST2 signalling for the treatment of inflammatory diseases. Cell Physiol Biochem. (2018) 49:349–58. doi: 10.1159/000492885
131. Salas A. The IL-33/ST2 axis: yet another therapeutic target in inflammatory bowel disease? Gut. (2013) 62:1392–3. doi: 10.1136/gutjnl-2012-303920
132. Alves MF, da Fonseca DV, de Melo SAL, Scotti MT, Scotti L, Dos Santos SG, et al. New therapeutic targets and drugs for the treatment of asthma. Mini Rev Med Chem. (2018) 18:684–96. doi: 10.2174/1389557517666170927145011
Keywords: IL-33, allergic inflammation, signal transduction, eosinophils, mast cells, innate lymphoid cells (ILC), Chinese herbal medicine, therapeutics
Citation: Chan BCL, Lam CWK, Tam L-S and Wong CK (2019) IL33: Roles in Allergic Inflammation and Therapeutic Perspectives. Front. Immunol. 10:364. doi: 10.3389/fimmu.2019.00364
Received: 18 May 2018; Accepted: 12 February 2019;
Published: 04 March 2019.
Edited by:
Rong Mu, Peking University People's Hospital, ChinaReviewed by:
Remo Castro Russo, Federal University of Minas Gerais, BrazilDong Li, Jilin University, China
Copyright © 2019 Chan, Lam, Tam and Wong. This is an open-access article distributed under the terms of the Creative Commons Attribution License (CC BY). The use, distribution or reproduction in other forums is permitted, provided the original author(s) and the copyright owner(s) are credited and that the original publication in this journal is cited, in accordance with accepted academic practice. No use, distribution or reproduction is permitted which does not comply with these terms.
*Correspondence: Chun K. Wong, ck-wong@cuhk.edu.hk