- 1Institute of Molecular and Clinical Immunology, Medical Faculty, Otto-von-Guericke University, Magdeburg, Germany
- 2TWINCORE, Centre for Experimental and Clinical Infection Research, a joint venture between the Helmholtz Centre for Infection Research and the Medical School Hannover, Institute for Experimental Infection Research, Hannover, Germany
- 3Institute of Immunology, Charité-Universitätsmedizin Berlin, Berlin, Germany
- 4Max-Delbrück-Center for Molecular Medicine, Berlin, Germany
- 5Berlin Institute of Health, Berlin, Germany
- 6Institute of Inflammation and Neurodegeneration, Medical Faculty, Otto-von-Guericke University, Magdeburg, Germany
In lymphopenic mice, T cells become activated and undergo lymphopenia-induced proliferation (LIP). However, not all T cells are equally sensitive to lymphopenia. Several lymphopenia-insensitive T cell clones were described and their non-responsiveness was mainly attributed to clone-specific properties. Here, we provide evidence for an additional, host-dependent mechanism restraining LIP of lymphopenia-insensitive CD4+ T cells. We show that such cells undergo LIP in lymphopenic mice lacking IFN-γ receptor (IFN-γR) expression, a process, which is promoted by the autocrine action of T cell-derived IFN-γ. Additionally, LIP of lymphopenia-insensitive CD4+ T cells requires an intact microflora and is accompanied by the massive accumulation of IL-6 and dendritic cells (DCs). Consistent with these results, IL-6 neutralization and the DC-specific restoration of IFN-γR expression are both sufficient to restrict LIP. Hence, the insensitivity of CD4+ T cells to lymphopenia relies on cell-intrinsic properties and a complex interplay between the commensal microflora, IL-6, IFN-γR+ DCs, and T cell-derived IFN-γ.
Introduction
In lymphocyte-competent hosts, T cells continuously utilize homeostatic factors such as Interleukin-7 (IL-7) and self-peptide-MHC complexes and thereby limit their availability (1). Due to the lack of IL-7-consuming T cells, IL-7 accumulates in lymphopenic mice (2) and humans (3). IL-7 is a potent activation and survival signal for T cells and its overabundance promotes T cell responses (4). Consequently, the adoptive transfer of polyclonal naive CD4+ T cells into lymphopenic mice leads to their activation and subsequent lymphopenia-induced proliferation (LIP) (5, 6). However, LIP represents a mixed reaction in response to different stimuli. While IL-7 overabundance induces a comparably slow homeostatic proliferation (HP) of T cells, the commensal microflora triggers a rapid response referred to as spontaneous proliferation (SP) (7–11). Nevertheless, naive T cells undergoing LIP differentiate into interferon-γ (IFN-γ)-producing effector/memory T cells, which is frequently associated with autoimmunity (12, 13).
The degree of LIP varies strongly between T cell clones (14–16). For example, ovalbumin (OVA)-specific CD4+ TCR-transgenic (tg) OT-II T cells, contrary to polyclonal CD4+ T cells, do not undergo LIP in irradiated hosts (14) and expand only moderately in fully lymphopenic Rag-deficient (Rag−/−) mice (10). TCR signal strength is a major factor that regulates the sensitivity of a T cell to lymphopenia (15, 16). It is affected by a complex interplay between TCR avidity and molecules modulating TCR signal transduction (15, 17, 18). Hence, cell-intrinsic mechanisms appear to determine whether a T cell is sensitive to lymphopenia or not. However, it remained unclear whether extrinsic mechanisms prevent LIP of lymphopenia-insensitive CD4+ T cells.
In the present study, we show that lymphopenia-insensitive OT-II cells expand massively in IFN-γ receptor (IFN-γR)-deficient Rag−/− (RagγRko) mice, a phenomenon that is not observed in IFN-γ-deficient Rag−/− (Ragγko) mice. LIP of OT-II cells is associated with a strong increase in systemic IL-6 and subsequent T cell accumulation. The lack of IFN-γ and IFN-γR expression by OT-II cells impaired LIP to some degree arguing for a growth promoting, autocrine effect of OT-II-derived IFN-γ. Furthermore, we show that the commensal microflora is crucial for OT-II LIP in RagγRko mice, which is accompanied by the massive expansion of dendritic cells (DCs). Finally, we show that IFN-γR expression exclusively in DCs is sufficient to restrict OT-II expansion, DC accumulation and IL-6 production in RagγRko mice. In summary, we provide evidence that the suppression of CD4+ T cell activation in response to lymphopenia is determined by a combination of both, clone-specific properties and environmental factors such as the commensal microflora, IL-6 and IFN-γR expression by DCs.
Materials and Methods
Mice and Adoptive T Cell Transfer
Thy1.1+ B6.PL-Thy1a/Cy and Thy1.2+ B6.129S7-Rag1tm1Mom/J (Rag−/−), C57BL/6J (B6), B6.SJL-PtprcaPepcb/BoyJ (CD45.1+), B6.129S7-Ifnγtm1Ts (IFN-γ−/−), B6.129S7-Ifngrtm1Agt (IFN-γR−/−), B6.Cg-Tg(TcraTcrb)425Cbn/J (OT-II) (expressing a transgenic TCR specific for the chicken ovalbumin (OVA)-derived, I-Ab-restricted peptide OVA323−339), B6.Cg-Tg(Itgax-EGFP-CRE-DTR-LUC)2Gjh/Crl (CD11c-GCDL) (19) and pCAGloxPSTOPloxP-IFNγR-IRES-GFP (IFN-γRSO) transgenic mice (20) were housed under specific pathogen-free conditions. Mice were crossed to generate Thy1.1/.2/CD45.1/.2-disparate Rag−/−OT-II (OT-IIWT), Rag−/−IFN-γR−/−OT-II (OT-IIγRko), and Rag−/−IFN-γ−/−OT-II (OT-IIγko) T cell donors. Lymphopenic Rag−/− (RagWT), Rag−/−IFN-γ−/− (Ragγko), Rag−/−IFN-γR−/− (RagγRko), and Rag−/−IFN-γR−/− × CD11c-GCDL × IFN-γRSO (RagγRko × IFN-γRCD11c−ON) mice served as T cell recipients. For the adoptive transfers shown in Figures 2A,B, B6 or CD45.1+ mice served as non-lymphopenic controls. For T cell transfers, single cell suspensions were prepared from spleens and lymph nodes of donor mice by forcing the organs through metal sieves. To lyse erythrocytes, cell suspensions were incubated with Ammonium-Chloride-Potassium lysis buffer for 90 s and subsequent addition of RPMI with 10% FCS. After washing with PBS/2mM EDTA, cell suspensions were resuspended in PBS and filtered through 40 μm cell strainers (BD and Corning, Durham, NC). Single cell suspensions were counted, stained with fluorochrome-labeled antibodies for 30 min at 4°C and analyzed by flow cytometry to determine the frequency and activation state of OT-II cells (Supplementary Figure 1). Cell suspensions containing 1.6–10 × 105 naive CD4+ OT-II T cells were injected i.v. into the tail vein of recipient mice. For CFSE labeling, donor single cell suspensions (2.2–3.2 × 107 cells/ml) were incubated with 7.5 μM CFSE (Biolegend) in PBS for 20 min at 37°C. Subsequently, cells were washed twice with ice cold PBS or RPMI/10% FCS and were resuspended in PBS prior to injection. Cell suspensions containing 7.5–8 × 105 CFSE+ OT-II T cells were injected i.v. into the tail vein of recipient mice. Ten to thirteen days after transfer, spleens and lymph nodes were isolated and single cell suspensions were prepared as described. Erythrocyte lysis was performed with spleen cell samples. Cells were counted and directly stained with fluorochrome-labeled antibodies for 30 min at 4°C after blocking FcR with purified anti-CD32/CD16 monoclonal antibodies (2.4G2 ATCC® HB-197™). To neutralize IL-6 in vivo, mice were i.p. injected with 500 μg of anti-IL-6 (MP5-20F3; BioXCell) 2 days prior to OT-II transfer. Treatment was repeated every third day. Control mice received 500 μg control IgG1 (HRPN; BioXCell). To deplete the commensal microflora, mice were treated with 0.5 g/l vancomycin, 1.0 g/l metronidazole, 1.0 g/l ampicillin, and 1.0 g/l neomycinsulfate via the drinking water 4 weeks prior to and during the experiment (21). Mice treated with antibiotics did not show any obvious clinical symptoms. At the day of analysis, however, their cecum was enlarged indicating successful depletion of the commensal microflora.
Flow Cytometry
The following antibodies and reagents were used: anti-CD4 (RM4-5; Biolegend/eBioscience), -CD11c (N418; BD/Biolegend), -CD44 (IM7; Biolegend), -CD45.1 (A20; Biolegend), -CD62L (MEL-14; Biolegend), CD127 (A7R34; BD/Biolegend), -KLRG-1 (2F1; Biolegend/eBioscience), -Ki67 (SolA15; eBioscience), -I-Ab (AF6-120.1; Biolegend), -Thy1.1 (OX-7; Biolegend), -TCR Vα2 (B20.1; Biolegend), streptavidin-BV510 (Biolegend) and streptavidin-PE (Biolegend). For intranuclear staining of Ki67, cells were first stained with the indicated antibodies directed against cell surface molecules. Afterwards cells were fixed with the Foxp3/Transcription Factor Staining Buffer Set (eBioscience) according to the manufacturer's instructions and subsequently incubated with anti-Ki67 for 30 min at 4°C. Samples were measured on LSRFortessa flow cytometer (Becton Dickinson) and analyzed by FlowJo 9 and 10 software (FlowJo, LLC). To calculate the fold expansion of OT-II cells or DCs, the respective cell populations were quantified. For each experiment a mean value was calculated for the RagWT group. Finally, cell numbers of individual mice, including RagWT mice, were calculated in relation to the mean value of the RagWT group. Relative mean fluorescence intensities (MFIs) and relative frequencies of OT-II cells or DCs were calculated in analogy.
IFN-γ and IL-6 Detection
Blood (supplemented with EDTA) was centrifuged 10 min at 500 × g and 4°C. The supernatant was centrifuged again 10 min at 900 × g and 4°C to obtain the plasma that was analyzed by an IFN-γ or IL-6 specific ELISA (eBioscience) according to manufacturer's instructions.
Statistical Analysis
Statistical analysis and graphical representations were done using Prism 5 software (GraphPad Software). Statistical significance was determined using a non-parametric two-tailed Mann-Whitney U-test. *p ≤ 0.05; **p ≤ 0.01; ***p ≤ 0.001; ****p ≤ 0.0001.
Results
Host IFN-γR Expression Restrains Commensal-Driven OT-II LIP
We have shown that host IFN-γR signaling restricts LIP of CD8+ T cells (22). Whether this mechanism prevents LIP of CD4+ OT-II T cells was unclear. To address this issue, naive CD4+ T cells from Rag−/− OT-II TCRtg mice (OT-IIWT cells) were adoptively transferred into IFN-γR-deficient Rag−/− (RagγRko) and IFN-γR-competent Rag−/− (RagWT) mice. To elucidate a potential contribution of host-derived IFN-γ, IFN-γ-deficient Rag−/− mice (Ragγko) were reconstituted with OT-IIWT cells in parallel. Within 10–12 days, OT-IIWT cells expanded massively in RagγRko but not in RagWT or Ragγko spleens (Figure 1A). LIP was associated with the up-regulation of CD44, CD127, KLRG-1, and Ki67 indicating full activation and proliferation of OT-IIWT cells in RagγRko mice (Figures 1B,C). LIP is induced in T cell areas of secondary lymphoid organs (SLOs) (23) and IFN-γ regulates T cell migration to and positioning in SLOs (24–26), which is guided by chemokine-producing stromal cells (27). However, stromal cell composition differs significantly between lymph nodes (LNs) and spleen (28). We therefore asked next whether OT-II expansion is equally well induced in either SLO. To address this question, CFSE-labeled OT-IIWT cells were transferred into RagWT and RagγRko mice. C57BL/6 (B6) served as non-lymphopenic controls. After 12 days, recipient LNs and spleens were analyzed. As shown in Figures 2A,B, the frequencies of CFSElo OT-IIWT cells were lower in LNs than in spleen of both recipients. However, CFSElo OT-IIWT cells were clearly more abundant in RagγRko spleens and LNs (Figures 2A,B) indicating higher frequencies of rapidly dividing OT-IIWT cells in either organ. Of note, in addition to the rapidly dividing CFSElo OT-II cells, a population of CFSEint cells was detectable in the spleen, but not LNs, of RagγRko mice (Figures 2A,B). This suggests different, organ-specific velocities of OT-II LIP. Nonetheless, OT-IIWT LIP was most pronounced in the spleens of RagγRko mice. We therefore focused on this organ in the following experiments.
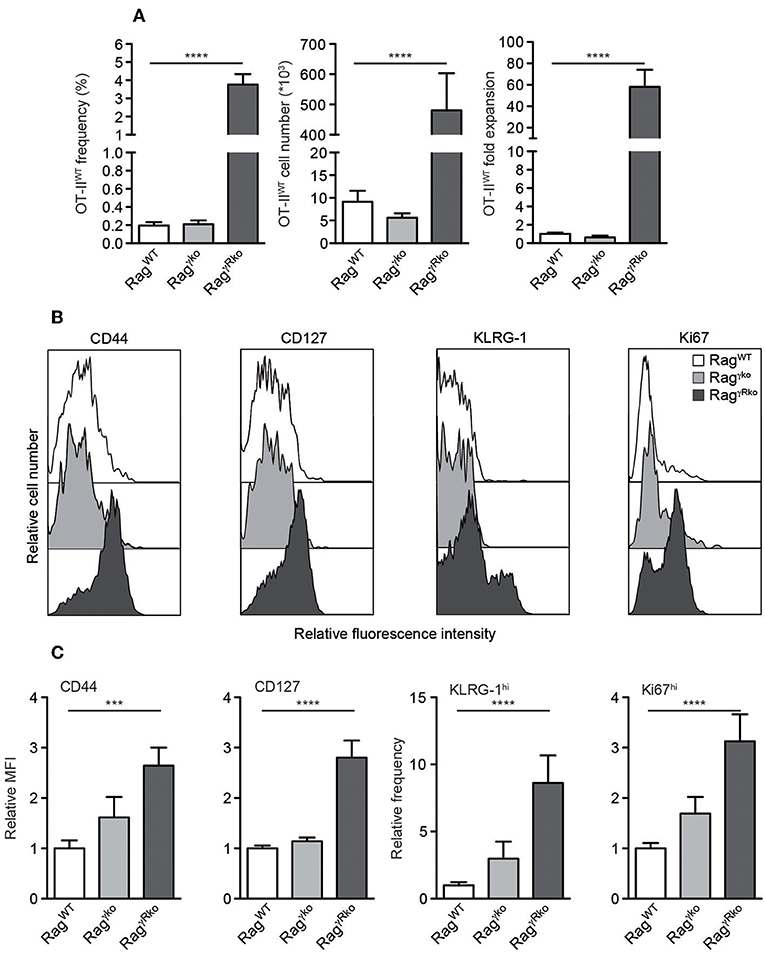
Figure 1. CD4+ T cell LIP is amplified in IFN-γR-deficient mice. (A–C) CD4+Thy1.1+ OT-IIWT T cells were adoptively transferred into RagWT, Ragγko and RagγRko mice (all Thy1.1−). After 10–12 days, recipient splenocytes were analyzed by flow cytometry. (A) Shown are frequencies, cell numbers and fold expansion of OT-IIWT cells. (B) Relative fluorescence intensities, (C) relative MFIs for CD44 and CD127 and relative frequencies of KLRG-1hi and Ki67hi cells were determined after gating on CD4+Thy1.1+ OT-IIWT cells. (A,C) Shown are pooled results from 3 to 4 independent experiments with a total of 11–17 mice per group and (B) representative histograms from corresponding samples. (A,C) Graphs show mean values + SEM and statistical significances (***p ≤ 0.001; ****p ≤ 0.0001) were calculated to values in RagWT mice.
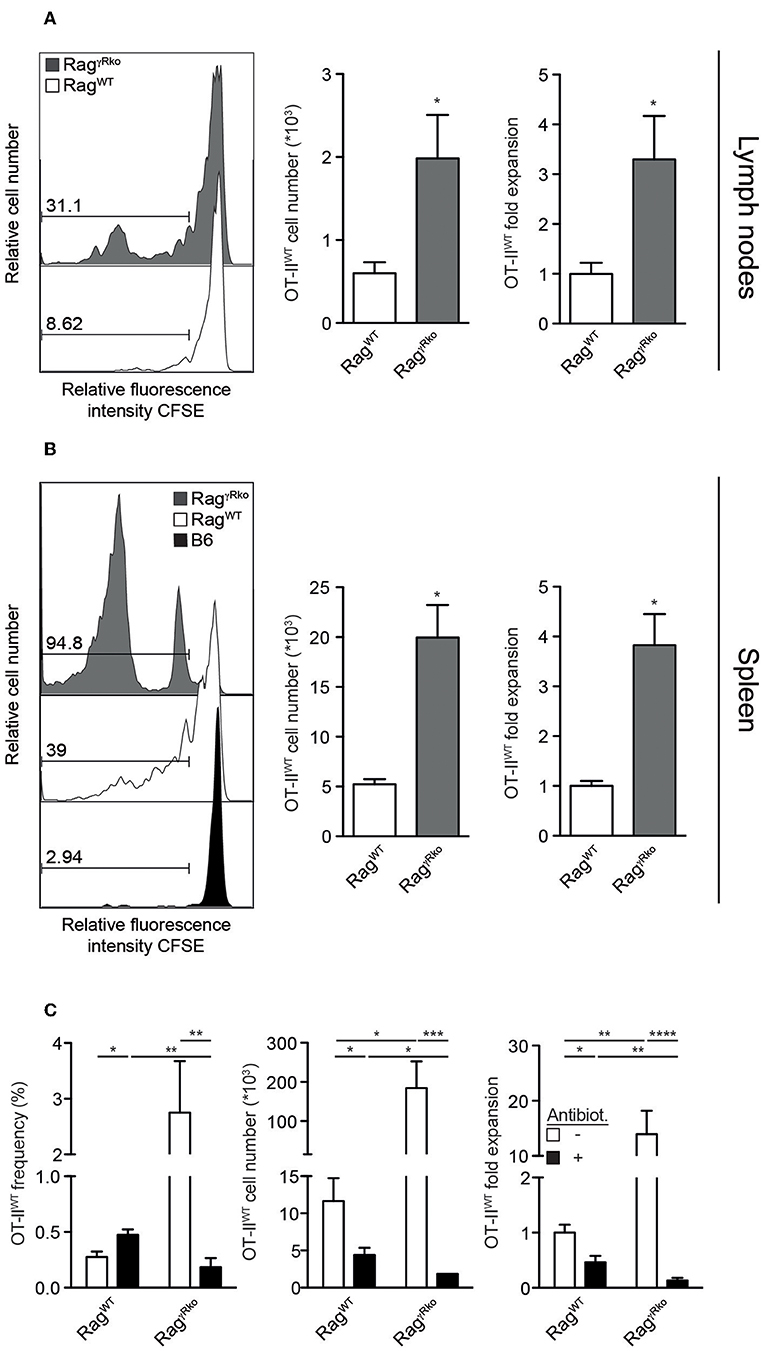
Figure 2. OT-II LIP is more pronounced in spleen than in lymph nodes. (A,B) CFSE-labeled OT-IIWT cells were adoptively transferred into RagWT, RagγRko mice and (B) B6 mice. After 12 days, recipient (A) lymph nodes and (B) spleen were analyzed by flow cytometry. (A,B) Histograms show relative fluorescence intensities for CFSE after gating on CD4+CD45.1+ OT-IIWT cells and numbers indicate percentages. Bar diagrams show cell numbers and fold expansion of OT-IIWT cells (mean values + SEM; *p ≤ 0.05). Results in bar diagrams were pooled from 6 mice per group analyzed in one experiment. (A) Histograms are representative of one experiment with 6 RagWT and 6 RagγRko. (B) Histograms are representative of 2 independent experiments with a total of 10 RagWT, 10 RagγRko, and 4 B6 mice. (C) OT-IIWT cells were adoptively transferred into RagWT and RagγRko mice. After 11–13 days, recipient splenocytes were analyzed by flow cytometry. Four weeks prior to and during T cell transfer, mice were treated with antibiotics (Antibiot.) or were left untreated. Shown are pooled results (mean values + SEM; *p ≤ 0.05; **p ≤ 0.01; ***p ≤ 0.001; ****p ≤ 0.0001) from 2 independent experiments with a total of 8–9 mice per group.
Under lymphopenic conditions, the rapid-type of T cell proliferation relies on the presence of an intact commensal microflora (7, 10). Whether this is also the case for OT-II expansion in RagγRko mice was studied next. For this purpose, RagWT and RagγRko mice were treated with a mixture of antibiotics prior to and during reconstitution with OT-IIWT cells. This treatment regimen efficiently depletes commensals (21, 29). As expected, OT-IIWT expansion was impaired in untreated RagWT mice but was very efficient in untreated RagγRko mice (Figure 2C, white bars). On the contrary, antibiotic treatment blocked OT-IIWT LIP in RagγRko mice (Figure 2C). Together, the data presented so far indicate that recipient IFN-γR expression restrains commensal-driven spontaneous proliferation (SP) (7–11) of OT-II cells under lymphopenic conditions.
IL-6 Accumulates in RagγRko Mice and Promotes OT-II SP
IL-6 promotes commensal-dependent SP of CD4+ and CD8+ T cells in lymphopenic mice (9, 10). To elucidate whether IL-6 levels are altered in our experimental system, plasma samples from OT-IIWT-reconstituted RagWT and RagγRko were analyzed 10–12 days after T cell transfer. As shown in Figure 3A, plasma levels of IL-6 were strongly elevated in OT-IIWT-reconstituted RagγRko mice (Figure 3A; + OT-IIWT) but not in untreated controls (Figure 3A; –OT-IIWT). In order to test whether IL-6 promotes OT-IIWT SP in RagγRko mice, RagWT, and RagγRko mice were treated with neutralizing monoclonal anti-IL-6 antibodies (αIL-6 mAb) prior to and after reconstitution with OT-IIWT cells. Control mice received isotype-matched control mAbs. As shown in Figure 3B, αIL-6 treatment did not affect frequencies, cell numbers or relative expansion rates of OT-IIWT cells in RagWT mice. As expected, OT-IIWT cells were by far most abundant in isotype-treated RagγRko mice, an effect that was fully reverted by IL-6 neutralization. Accordingly, expression levels of CD44 and Ki67 were strongly reduced in OT-IIWT cells recovered from αIL-6-treated RagγRko mice as compared to isotype-treated controls (Figures 3C,D). Hence, IL-6 is up-regulated upon T cell transfer and is crucial for OT-IIWT activation, proliferation and subsequent accumulation in RagγRko mice.
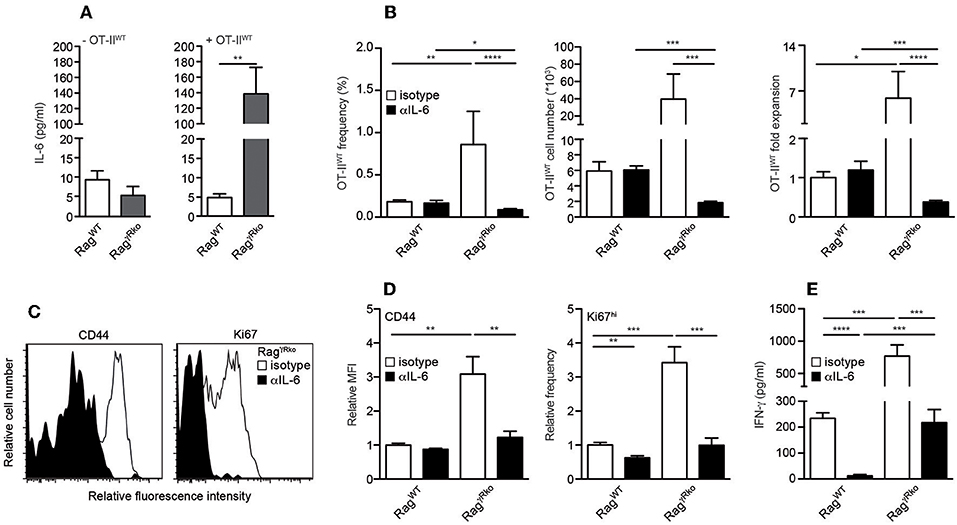
Figure 3. IL-6 accumulates in RagγRko mice and promotes OT-II SP. (A–E) RagWT and RagγRko mice were reconstituted with OT-IIWT cells as described in Figure 1. (A) Untreated mice served as controls (-OT-IIWT). (B–D) Prior to and after T cell reconstitution, mice were treated with neutralizing anti-IL-6 (αIL-6) or isotype-machted control antibodies (isotype). Ten to twelve days after T cell transfer, (A) IL-6 and (E) IFN-γ plasma levels were determined by ELISA and (B–D) recipient splenocytes were analyzed by flow cytometry. (B) Shown are frequencies, cell numbers and fold expansion of OT-IIWT cells in isotype- and αIL-6-treated RagWT and RagγRko mice. (C) Relative fluorescence intensities, (D) relative MFIs for CD44 and relative frequencies of Ki67hi cells were determined after gating on CD4+Thy1.1+ OT-IIWT cells in isotype- and αIL-6-treated RagγRko mice. (A,B,D,E) Shown are pooled results from 2 to 3 independent experiments with a total of 5–11 mice per group and (C) representative histograms from corresponding samples. (A,B,D,E) Graphs show mean values + SEM; *p ≤ 0.05; **p ≤ 0.01; ***p ≤ 0.001; ****p ≤ 0.0001.
OT-II-Derived IFN-γ Promotes SP in an Autocrine Fashion
T cell-intrinsic IL-6R signaling promotes the expansion of IFN-γ-producing effector/memory CD4+ T cells under lymphopenic and non-lymphopenic conditions (30, 31). Consequently, the blockade of OT-IIWT activation and subsequent SP in αIL-6-treated RagγRko mice (Figures 3B–D) correlated with a strong reduction of plasma IFN-γ levels (Figure 3E).
Since IFN-γ directly promotes CD4+ T cell responses (32–34), we hypothesized that OT-II-derived IFN-γ supports SP in RagγRko mice in an autocrine fashion. To test this hypothesis, IFN-γ-deficient OT-II (OT-IIγko) cells were transferred into RagγRko and RagWT mice. After 11–12 days, OT-IIγko frequencies, cell numbers and relative expansion rates were determined. As shown in Figure 4A, some expansion of OT-IIγko cells was detectable in RagγRko. This was associated with the up-regulation of CD44, KLRG-1 and Ki67 (Figures 4B,C). Importantly, however, OT-IIγko cells expanded less well in RagγRko mice (~10-fold; Figure 4A) than OT-IIWT cells (~50-fold; Figure 1A) suggesting a growth-promoting effect of autocrine IFN-γ.
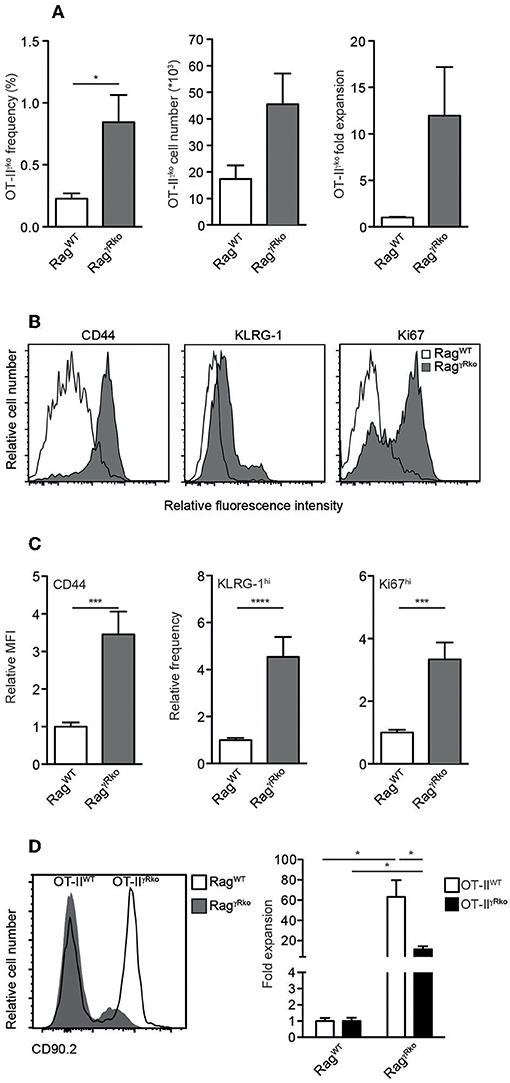
Figure 4. CD4+ T cell-derived IFN-γ promotes SP in an autocrine manner. (A–C) OT-IIγko (3–4 independent experiments with 12–17 mice per group) or (D) equal numbers of Thy1.1+ OT-IIWT and Thy1.1/1.2+ OT-IIγRko T cells (4 mice per group) were transferred simultaneously into Thy1.2+ RagWT and RagγRko mice. After 11–12 days, recipient splenocytes were analyzed by flow cytometry as described in Figure 1. Overlay shows the relative abundance of Thy1.1+ OT-IIWT and Thy1.1/1.2+ OT-IIγRko T cells in RagWT and RagγRko mice. (A,C,D) Graphs show mean values + SEM; *p ≤ 0.05; ***p ≤ 0.001; ****p ≤ 0.0001.
To further test this possibility, equal numbers of OT-IIWT and OT-IIγRko cells were co-transferred into RagγRko and RagWT mice. OT-IIWT cells expanded ~60-fold while OT-IIγRko cells expanded only ~20-fold (Figure 4D). Thus, SP of OT-IIγko and OT-IIγRko cells occurs in RagγRko mice. Compared to OT-IIWT cells, OT-IIγko and OT-IIγRko expansion was less pronounced suggesting that OT-II-derived IFN-γ promotes SP in an autocrine fashion. However, we cannot exclude a contribution of host-derived IFN-γ, which accumulates in IFN-γR-deficient mice due to lack of its consumption (22).
IFN-γR+ DCs Restrain CD4+ T Cell SP in RagγRko Mice
Dendritic cells (DCs) producing elevated levels of IL-6 promote aberrant T cell activation and subsequent IFN-γ synthesis (35). Furthermore, the induction of EAE relies on the accumulation of IL-6-producing DCs (36). Under lymphopenic conditions, MyD88-dependent recognition of the commensal microflora is sufficient to induce IL-6 production by DCs thereby promoting SP of CD4+ T cells (10) similar to what we have observed in OT-IIWT-reconstituted RagγRko mice. Furthermore, DCs express high levels of MHCII, which is crucial for CD4+ T cell LIP (14, 37). Based on these data we speculated that DC responses were altered in RagγRko mice. When splenic CD11c+MHCIIhi DCs were quantified in OT-IIWT-reconstituted RagWT and RagγRko mice, their numbers were strongly increased in the latter (Figure 5A; + OT-IIWT). This was not the case in untreated RagγRko mice (Figure 5A; –OT-IIWT) suggesting that OT-IIWT activation is a prerequisite for DC accumulation in RagγRko recipients.
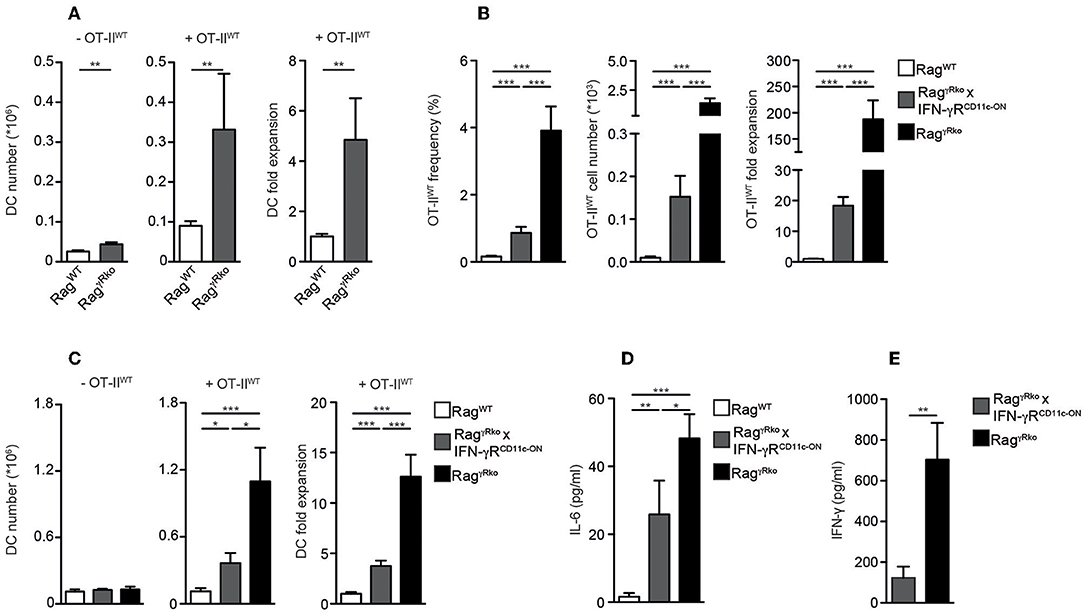
Figure 5. IFN-γR+ DCs restrain CD4+ T cell SP in RagγRko mice. (A–D) OT-IIWT cells were adoptively transferred into RagWT and RagγRko mice. After 11–13 days, recipient splenocytes were analyzed by flow cytometry. (A) Results of 2–6 independent experiments with a total of 10–25 mice were pooled to calculate the numbers and fold expansion of CD11c+MHCIIhi DCs after reconstitution with OT-IIWT cells (+OT-IIWT). DC numbers from untreated RagWT and RagγRko mice were determined as well (-OT-IIWT). (B–D) Frequencies, cell numbers and fold expansion of OT-IIWT cells/DCs as well as plasma IL-6 levels were analyzed in RagWT, RagγRko × CD11c-GCDL × IFN-γRSO (RagγRko × IFN-γRCD11c−ON) and RagγRko mice. Pooled results of 2 independent experiments with a total of 8 mice per group are shown. (E) Steady-state levels of IFN-γ were determined in plasma samples of 8–9 untreated RagγRko × IFN-γRCD11c−ON and RagγRko mice. (A–E) Graphs show mean values + SEM; *p ≤ 0.05; **p ≤ 0.01; ***p ≤ 0.001.
Whether the DC-specific restoration of IFN-γR expression is sufficient to block OT-IIWT SP and subsequent DC accumulation in RagγRko mice was tested next. For this purpose, we made use of a novel transgenic mouse line, allowing IFN-γR expression after the Cre-mediated deletion of a loxP-flanked DNA-Stop cassette (20). To activate this “switch-on” (IFN-γRSO) construct and express the transgenic IFN-γR specifically in DCs, IFN-γRSO mice were crossed to CD11c-GCDL mice expressing Cre under the control of the CD11c promoter (19). Subsequently, CD11c-GCDL × IFN-γRSO mice were crossed to RagγRko mice in order to generate T and B cell-deficient, fully lymphopenic RagγRko × CD11c-GCDL × IFN-γRSO mice lacking IFN-γR expression on all cells except DCs. These mice are termed RagγRko × IFN-γRCD11c−ON hereafter. Finally, OT-IIWT cells were transferred into RagWT mice, RagγRko × IFN-γRCD11c−ON, and RagγRko controls. After 11–13 days, the numbers of splenic OT-IIWT cells were determined. As opposed to RagWT mice, OT-IIWT cells expanded strongly in RagγRko mice (Figure 5B). The values obtained with RagγRko × IFN-γRCD11c−ON mice reached intermediate levels showing that IFN-γR expression by DCs is sufficient to restrain OT-IIWT SP. Similarly, DC expansion was most pronounced in OT-IIWT-reconstituted RagγRko mice, reached intermediate levels in RagγRko × IFN-γRCD11c−ON mice and was least efficient in RagWT mice (Figure 5C; +OT-IIWT). On the contrary, DC numbers did not differ between untreated RagWT, RagγRko × IFN-γRCD11c−ON and RagγRko mice (Figure 5C; –OT-IIWT) suggesting a causal link between OT-IIWT SP and DC expansion in RagγRko mice (Figures 5A,C). Importantly, specific IFN-γR expression by DCs was sufficient to limit expansion of OT-IIWT cells and DCs as well as IL-6 up-regulation (Figure 5D) in RagγRko × IFN-γRCD11c−ON mice.
The efficacy of CD4+ T cell responses correlates positively with the amount of IFN-γ available in the early phase of the response (32, 34). We have shown previously that IFN-γ accumulates in IFN-γR-deficient mice, most probably due to the lack of its receptor-mediated clearance (22). Hence, elevated levels of steady-state IFN-γ may explain the rapid and strong induction of OT-IIWT responses in RagγRko mice. To test whether decreased OT-IIWT responses in RagγRko × IFN-γRCD11c−ON mice (Figure 5B) correlate with reduced steady-state IFN-γ levels, we compared plasma samples of untreated RagγRko and RagγRko × IFN-γRCD11c−ON mice. As shown in Figure 5E, IFN-γ levels were significantly lower in RagγRko × IFN-γRCD11c−ON mice. This suggests that IFN-γR+ DCs consume IFN-γ thereby reducing its availability for OT-IIWT cells. This competition for IFN-γ would provide an explanation for the reduced levels of SP in RagγRko × IFN-γRCD11c−ON mice (Figure 5B).
Discussion
T cell clones are not equally sensitive to lymphopenia-related activation signals (14–16). For example, ovalbumin-specific CD4+ T cells from OT-II TCRtg mice represent one of several T cells clones, which are resistant to lymphopenia-induced activation (14). It is well accepted that T cell clone-specific features such as CD5 levels correlate closely with the sensitivity to lymphopenia (15, 16, 38). Here, we provide evidence for an additional, recipient-dependent mechanism that restrains expansion of adoptively transferred CD4+ T cells. This mechanism relies on a complex interplay between the commensal microflora, IFN-γR+ DCs and CD4+ T cells.
The commensal microflora triggers IFN-γ production by various immune cells in the steady-state (39, 40). In IFN-γR-deficient mice, IFN-γ accumulates due to the lack of its consumption (22). Thus, elevated IFN-γ levels in RagγRko mice may provide early activation signals to OT-II cells initiating the rapid expansion we have observed. This interpretation is in accordance with our finding that both, OT-IIWT expansion and steady-state levels of IFN-γ, were decreased in RagγRko × IFN-γRCD11c−ON mice. This suggests that IFN-γR+ DCs efficiently reduce amounts of circulating IFN-γ thereby restricting its availability for OT-II cells.
However, increased rates of OT-II expansion in RagγRko mice do not only rely on host-derived IFN-γ. As we have shown here, OT-II-derived IFN-γ acts in an autocrine manner. Hence, host- and OT-II-derived IFN-γ may synergize in promoting full-blown OT-II expansion in RagγRko mice. OT-II expansion is accompanied by the up-regulation of CD127, which would facilitate their IL-7-dependent survival (41–43) and provides one explanation for the accumulation of OT-II cells in RagγRko mice. Importantly, the accumulation of DCs and IL-6 correlates positively with the degree of OT-II expansion in RagγRko mice and might be interrelated. DCs produce IL-6 in response to the commensal microflora (10) and express MHCII, which are both required for CD4+ T cell expansion under lymphopenic conditions (10, 14, 37). Since (i) T cell-intrinsic IL-6R signaling is critical for CD4+ T cell responses (30, 31), (ii) IL-6 prevents apoptosis of naive and effector CD4+ T cells (44, 45), and (iii) counter-regulates DC function (35, 46–50) we suggest a direct, growth-promoting and/or anti-apoptotic effect of IL-6 on OT-II cells expanding in RagγRko mice. Although the T cell-stimulatory potential of DC-derived IL-6 is well established (10, 35, 36) recent findings identified multiple hematopoietic and non-hematopoietic cell types as potential IL-6 producers (36). Importantly, different IL-6 producers appear to regulate different aspects of the same CD4+ T cell response (36). Hence, it remains to be shown for our experimental system whether (i) DCs and/or other cell types up-regulate IL-6 expression in OT-II-reconstituted RagγRko mice, whether (ii) the elevation of IL-6 levels in these mice results from the accumulation of DCs producing constant amounts of IL-6, and whether (iii) there is a causal relationship between the cellular origin of IL-6 and its growth-promoting effect. As reported only recently, definite answers to such questions would require the combined use of cell type-specific IL-6 reporter as well as conditional IL-6 knockout mice (36) and their integration into our experimental systems. However, this would be beyond the scope of this study and therefore remains an important task for the future.
From previous experiments we know that only effector, but not naive, OT-IIWT cells activate immature DCs (51). This suggests that IFN-γ-associated OT-II activation is an integral part of a self-amplifying loop in RagγRko mice, which involves the T cell-dependent accumulation of DCs, which in turn promote OT-II expansion. The lack of IFN-γR signaling in DCs increases their lifespan (52) and T cell-stimulatory potential (53) providing an additional explanation for the accumulation of DCs in RagγRko mice. In accordance with this interpretation, IFN-γR re-expression in DCs is sufficient to disrupt this self-amplifying loop and to down-modulate DC accumulation, IL-6 levels and OT-II cell expansion.
In summary, we demonstrate that the sensitivity of CD4+ T cells to lymphopenia is not only determined by cell-intrinsic properties but also by a complex interplay between CD4+ T cells, the commensal microflora and IFN-γR+ DCs. We postulate that T cell- and host cell-specific mechanisms have to co-operate to restrain spontaneous proliferation, the commensal-driven form of LIP. The molecular nature and the relative importance of either mechanism may vary for different T cell clones.
Ethics Statement
Animal experiments were performed according to institutional guidelines and were approved by the Landesverwaltungsamt Sachsen-Anhalt (Permit Number: 2-1155/2-1288 Uni MD).
Author Contributions
LK, CF, DS, and KD performed and analyzed the experiments. LK substantially contributed to manuscript preparation. UK and ID analyzed and discussed the data. TB and TK provided essential material, analyzed and discussed the data. TS designed and supervised the study, analyzed and discussed the data and wrote the manuscript with the help of the other co-authors.
Funding
This work was supported by the Deutsche Forschungsgemeinschaft [Sonderforschungsbereich TR36 (B2, B7), SFB854 (B15), and DU 1112/5-1].
Conflict of Interest Statement
The authors declare that the research was conducted in the absence of any commercial or financial relationships that could be construed as a potential conflict of interest.
Acknowledgments
We thank E. Denks and J. Giese for excellent technical assistance and Natalio Garbi for CD11c-GCDL mice.
Supplementary Material
The Supplementary Material for this article can be found online at: https://www.frontiersin.org/articles/10.3389/fimmu.2019.00140/full#supplementary-material
References
1. Takada K, Jameson SC. Naive T cell homeostasis: from awareness of space to a sense of place. Nat Rev Immunol. (2009) 9:823–32. doi: 10.1038/nri2657
2. Guimond M, Veenstra RG, Grindler DJ, Zhang H, Cui Y, Murphy RD, et al. Interleukin 7 signaling in dendritic cells regulates the homeostatic proliferation and niche size of CD4(+) T cells. Nat Immunol. (2009) 10:149–57. doi: 10.1038/ni.1695
3. Napolitano LA, Grant RM, Deeks SG, Schmidt D, De Rosa SC, Herzenberg LA, et al. Increased production of IL-7 accompanies HIV-1-mediated T-cell depletion: implications for T-cell homeostasis. Nat Med. (2001) 7:73–9. doi: 10.1038/83381
4. Mackall CL, Fry TJ, Gress RE. Harnessing the biology of IL-7 for therapeutic application. Nat Rev Immunol. (2011) 11:330–42. doi: 10.1038/nri2970
5. Tan JT, Dudl E, LeRoy E, Murray R, Sprent J, Weinberg KI, et al. IL-7 is critical for homeostatic proliferation and survival of naive T cells. Proc Natl Acad Sci USA. (2001) 98:8732–7. doi: 10.1073/pnas.161126098
6. Tan JT, Ernst B, Kieper WC, LeRoy E, Sprent J, Surh CD. Interleukin (IL)-15 and IL-7 jointly regulate homeostatic proliferation of memory phenotype CD8+ cells but are not required for memory phenotype CD4+ cells. J Exp Med. (2002) 195:1523–32. doi: 10.1084/jem.20020066
7. Kieper WC, Troy A, Burghardt JT, Ramsey C, Lee JY, Jiang H-Q, et al. Recent immune status determines the source of antigens that drive homeostatic T cell expansion. J Immunol. (2005) 174:3158–63. doi: 10.4049/jimmunol.174.6.3158
8. Min B, Foucras G, Meier-Schellersheim M, Paul WE. Spontaneous proliferation, a response of naive CD4 T cells determined by the diversity of the memory cell repertoire. Proc Natl Acad Sci USA. (2004) 101:3874–9. doi: 10.1073/pnas.0400606101
9. Tajima M, Wakita D, Noguchi D, Chamoto K, Yue Z, Fugo K, et al. IL-6-dependent spontaneous proliferation is required for the induction of colitogenic IL-17-producing CD8+ T cells. J Exp Med. (2008) 205:1019–27. doi: 10.1084/jem.20071133
10. Feng T, Wang L, Schoeb TR, Elson CO, Cong Y. Microbiota innate stimulation is a prerequisite for T cell spontaneous proliferation and induction of experimental colitis. J Exp Med. (2010) 207:1321–32. doi: 10.1084/jem.20092253
11. Do J-S, Foucras G, Kamada N, Schenk AF, Shaw M, Nuñez G, et al. Both exogenous commensal and endogenous self antigens stimulate T cell proliferation under lymphopenic conditions. Cell Immunol. (2012) 272:117–23. doi: 10.1016/j.cellimm.2011.11.002
12. Totsuka T, Kanai T, Nemoto Y, Makita S, Okamoto R, Tsuchiya K, et al. IL-7 Is essential for the development and the persistence of chronic colitis. J Immunol. (2007) 178:4737–48. doi: 10.4049/jimmunol.178.8.4737
13. Calzascia T, Pellegrini M, Lin A, Garza KM, Elford AR, Shahinian A, et al. CD4 T cells, lymphopenia, and IL-7 in a multistep pathway to autoimmunity. Proc Natl Acad Sci USA. (2008) 105:2999–3004. doi: 10.1073/pnas.0712135105
14. Ernst B, Lee DS, Chang JM, Sprent J, Surh CD. The peptide ligands mediating positive selection in the thymus control T cell survival and homeostatic proliferation in the periphery. Immunity (1999) 11:173–81. doi: 10.1016/S1074-7613(00)80092-8
15. Kassiotis G, Zamoyska R, Stockinger B. Involvement of avidity for major histocompatibility complex in homeostasis of naive and memory T cells. J Exp Med. (2003) 197:1007–16. doi: 10.1084/jem.20021812
16. Kieper WC, Burghardt JT, Surh CD. A role for TCR affinity in regulating naive T cell homeostasis. J Immunol. (2004) 172:40–4. doi: 10.4049/jimmunol.172.1.40
17. Smith K, Seddon B, Purbhoo MA, Zamoyska R, Fisher AG, Merkenschlager M. Sensory adaptation in naive peripheral CD4 T cells. J Exp Med. (2001) 194:1253–61. doi: 10.1084/jem.194.9.1253
18. Salmond RJ, Brownlie RJ, Morrison VL, Zamoyska R. The tyrosine phosphatase PTPN22 discriminates weak self peptides from strong agonist TCR signals. Nat Immunol. (2014) 15:875–83. doi: 10.1038/ni.2958
19. Tittel AP, Heuser C, Ohliger C, Llanto C, Yona S, Hämmerling GJ, et al. Functionally relevant neutrophilia in CD11c diphtheria toxin receptor transgenic mice. Nat Methods (2012) 9:385–90. doi: 10.1038/nmeth.1905
20. Kammertoens T, Friese C, Arina A, Idel C, Briesemeister D, Rothe M, et al. Tumour ischaemia by interferon-γ resembles physiological blood vessel regression. Nature (2017) 545:98–102. doi: 10.1038/nature22311
21. Rakoff-Nahoum S, Paglino J, Eslami-Varzaneh F, Edberg S, Medzhitov R. Recognition of commensal microflora by toll-like receptors is required for intestinal homeostasis. Cell (2004) 118:229–41. doi: 10.1016/j.cell.2004.07.002
22. Sercan O, Stoycheva D, Hämmerling GJ, Arnold B, Schüler T. IFN-gamma receptor signaling regulates memory CD8+ T cell differentiation. J Immunol. (2010) 184:2855–62. doi: 10.4049/jimmunol.0902708
23. Dummer W, Ernst B, LeRoy E, Lee D, Surh C. Autologous regulation of naive T cell homeostasis within the T cell compartment. J Immunol. (2001) 166:2460–8. doi: 10.4049/jimmunol.166.4.2460
24. Mueller SN, Hosiawa-Meagher KA, Konieczny BT, Sullivan BM, Bachmann MF, Locksley RM, et al. Regulation of homeostatic chemokine expression and cell trafficking during immune responses. Science (2007) 317:670–4. doi: 10.1126/science.1144830
25. Sung JH, Zhang H, Moseman EA, Alvarez D, Iannacone M, Henrickson SE, et al. Chemokine guidance of central memory T cells is critical for antiviral recall responses in lymph nodes. Cell (2012) 150:1249–63. doi: 10.1016/j.cell.2012.08.015
26. Kastenmüller W, Torabi-Parizi P, Subramanian N, Lämmermann T, Germain RN. A spatially-organized multicellular innate immune response in lymph nodes limits systemic pathogen spread. Cell (2012) 150:1235–48. doi: 10.1016/j.cell.2012.07.021
27. Bajénoff M, Egen JG, Koo LY, Laugier JP, Brau F, Glaichenhaus N, et al. Stromal cell networks regulate lymphocyte entry, migration, and territoriality in lymph nodes. Immunity (2006) 25:989–1001. doi: 10.1016/j.immuni.2006.10.011
28. Onder L. A novel bacterial artificial chromosome-transgenic Podoplanin–Cre mouse targets lymphoid organ stromal cells in vivo. Front Immunol. (2011) 2:50. doi: 10.3389/fimmu.2011.00050
29. Shalapour S, Deiser K, Sercan O, Tuckermann J, Minnich K, Willimsky G, et al. Commensal microflora and interferon-gamma promote steady-state interleukin-7 production in vivo. Eur J Immunol. (2010) 40:2391–400. doi: 10.1002/eji.201040441
30. Nish SA, Schenten D, Wunderlich FT, Pope SD, Gao Y, Hoshi N, et al. T cell-intrinsic role of IL-6 signaling in primary and memory responses. Elife (2014) 3:e01949. doi: 10.7554/eLife.01949
31. Li B, Jones LL, Geiger TL. IL-6 promotes T cell proliferation and expansion under inflammatory conditions in association with low-level RORγt expression. J Immunol. (2018) 201:2934–46. doi: 10.4049/jimmunol.1800016
32. Whitmire JK, Benning N, Whitton JL. Cutting edge: early IFN-gamma signaling directly enhances primary antiviral CD4+ T cell responses. J Immunol. (2005) 175:5624–8. doi: 10.4049/jimmunol.175.9.5624
33. Whitmire JK, Eam B, Benning N, Whitton JL. Direct interferon-gamma signaling dramatically enhances CD4+ and CD8+ T cell memory. J Immunol. (2007) 179:1190–7. doi: 10.4049/jimmunol.179.2.1190
34. Whitmire JK, Benning N, Eam B, Whitton JL. Increasing the CD4+ T cell precursor frequency leads to competition for IFN-gamma thereby degrading memory cell quantity and quality. J Immunol. (2008) 180:6777–85. doi: 10.4049/jimmunol.180.10.6777
35. Liu J, Han C, Xie B, Wu Y, Liu S, Chen K, et al. Rhbdd3 controls autoimmunity by suppressing the production of IL-6 by dendritic cells via K27-linked ubiquitination of the regulator NEMO. Nat Immunol. (2014) 15:612–22. doi: 10.1038/ni.2898
36. Heink S, Yogev N, Garbers C, Herwerth M, Aly L, Gasperi C, et al. Trans-presentation of IL-6 by dendritic cells is required for the priming of pathogenic TH17 cells. Nat Immunol. (2017) 18:74–85. doi: 10.1038/ni.3632
37. Do J-S, Min B. Differential requirements of MHC and of DCs for endogenous proliferation of different T-cell subsets in vivo. Proc Natl Acad Sci USA. (2009) 106:20394–8. doi: 10.1073/pnas.0909954106
38. Cho J-H, Kim H-O, Surh CD, Sprent J. T cell receptor-dependent regulation of lipid rafts controls naive CD8+ T cell homeostasis. Immunity (2010) 32:214–26. doi: 10.1016/j.immuni.2009.11.014
39. Keilbaugh SA, Shin ME, Banchereau RF, McVay LD, Boyko N, Artis D, et al. Activation of RegIIIbeta/gamma and interferon gamma expression in the intestinal tract of SCID mice: an innate response to bacterial colonisation of the gut. Gut (2005) 54:623–9. doi: 10.1136/gut.2004.056028
40. Niess JH, Leithäuser F, Adler G, Reimann J. Commensal gut flora drives the expansion of proinflammatory CD4 T cells in the colonic lamina propria under normal and inflammatory conditions. J Immunol. (2008) 180:559–68. doi: 10.4049/jimmunol.180.1.559
41. Schluns KS, Kieper WC, Jameson SC, Lefrançois L. Interleukin-7 mediates the homeostasis of naïve and memory CD8 T cells in vivo. Nat Immunol. (2000) 1:426–32. doi: 10.1038/80868
42. Rathmell JC, Farkash EA, Gao W, Thompson CB. IL-7 enhances the survival and maintains the size of naive T cells. J Immunol. (2001) 167:6869–76. doi: 10.4049/jimmunol.167.12.6869
43. Shklovskaya E, Fazekas de St Groth B. Severely impaired clonal deletion of CD4+ T cells in low-dose irradiated mice: role of T cell antigen receptor and IL-7 receptor signals. J Immunol. (2006) 177:8320–30. doi: 10.4049/jimmunol.177.12.8320
44. Teague TK, Marrack P, Kappler JW, Vella AT. IL-6 rescues resting mouse T cells from apoptosis. J Immunol. (1997) 158:5791–6.
45. Rochman I, Paul WE, Ben-Sasson SZ. IL-6 increases primed cell expansion and survival. J Immunol. (2005) 174:4761–7. doi: 10.4049/jimmunol.174.8.4761
46. Chomarat P, Banchereau J, Davoust J, Palucka AK. IL-6 switches the differentiation of monocytes from dendritic cells to macrophages. Nat Immunol. (2000) 1:510–4. doi: 10.1038/82763
47. Ratta M, Fagnoni F, Curti A, Vescovini R, Sansoni P, Oliviero B, et al. Dendritic cells are functionally defective in multiple myeloma: the role of interleukin-6. Blood (2002) 100:230–7. doi: 10.1182/blood.V100.1.230
48. Park S-J, Nakagawa T, Kitamura H, Atsumi T, Kamon H, Sawa S, et al. IL-6 regulates in vivo dendritic cell differentiation through STAT3 activation. J Immunol. (2004) 173:3844–54. doi: 10.4049/jimmunol.173.6.3844
49. Bleier JI, Pillarisetty VG, Shah AB, DeMatteo RP. Increased and long-term generation of dendritic cells with reduced function from IL-6-deficient bone marrow. J Immunol. (2004) 172:7408–16. doi: 10.4049/jimmunol.172.12.7408
50. Kitamura H, Kamon H, Sawa S, Park SJ, Katunuma N, Ishihara K, et al. IL-6-STAT3 controls intracellular MHC class II alphabeta dimer level through cathepsin S activity in dendritic cells. Immunity (2005) 23:491–502. doi: 10.1016/j.immuni.2005.09.010
51. Schüler T, Blankenstein T. Naive CD8(+) but not CD4(+) T cells induce maturation of dendritic cells. J Mol Med. (2002) 80:533–41. doi: 10.1007/s00109-002-0360-4
52. Do J-S, Asosingh K, Baldwin WM, Min B. Cutting edge: IFN-γR signaling in non-T cell targets regulates T cell-mediated intestinal inflammation through multiple mechanisms. J Immunol. (2014) 192:2537–41. doi: 10.4049/jimmunol.1303101
Keywords: CD4+ T cells, interferon-γ, lymphopenia, lymphopenia-induced proliferation (LIP), dendritic cells
Citation: Knop L, Frommer C, Stoycheva D, Deiser K, Kalinke U, Blankenstein T, Kammertoens T, Dunay IR and Schüler T (2019) Interferon-γ Receptor Signaling in Dendritic Cells Restrains Spontaneous Proliferation of CD4+ T Cells in Chronic Lymphopenic Mice. Front. Immunol. 10:140. doi: 10.3389/fimmu.2019.00140
Received: 31 August 2018; Accepted: 17 January 2019;
Published: 07 February 2019.
Edited by:
Loretta Tuosto, Sapienza University of Rome, ItalyReviewed by:
Niklas Beyersdorf, Universität Würzburg, GermanyHyun Park, National Cancer Institute (NCI), United States
Copyright © 2019 Knop, Frommer, Stoycheva, Deiser, Kalinke, Blankenstein, Kammertoens, Dunay and Schüler. This is an open-access article distributed under the terms of the Creative Commons Attribution License (CC BY). The use, distribution or reproduction in other forums is permitted, provided the original author(s) and the copyright owner(s) are credited and that the original publication in this journal is cited, in accordance with accepted academic practice. No use, distribution or reproduction is permitted which does not comply with these terms.
*Correspondence: Thomas Schüler, thomas.schueler@med.ovgu.de
†These authors have contributed equally to this work