- 1Department of Biochemistry, WHO-Immunology Research and Training Center, University of Lausanne, Epalinges, Switzerland
- 2Centro Internacional de Entrenamiento e Investigaciones Médicas, Cali, Colombia
- 3CIDEIM, Universidad ICESI, Cali, Colombia
Leishmania (Viannia) panamensis (L. (V.) p.) is the main causative agent of cutaneous leishmaniasis in Colombia and is usually treated with either meglumine antimoniate (MA) or miltefosine (MIL). In recent years, there has been increasing evidence of the emergence of drug-resistance against these compounds. Neutrophils are known to play an important role in immunity against Leishmania. These cells are rapidly recruited upon infection and are also present in chronic lesions. However, their involvement in the outcome of infection with drug-resistant Leishmania has not been examined. In this study, human and murine neutrophils were infected in vitro with MA or MIL drug-resistant L. (V.) p. lines derived from a parental L. (V.) p. drug-susceptible strain. Neutrophil effector functions were assessed analyzing the production of reactive oxygen species (ROS), the formation of neutrophil extracellular trap (NET) and the expression of cell surface activation markers. Parasite killing by neutrophils was assessed using L. (V.) p. transfected with a luciferase reporter. We show here that MA and MIL-resistant L. (V.) p. lines elicited significantly increased NET formation and MA-resistant L. (V.) p. induced significantly increased ROS production in both murine and human neutrophils, compared to infections with the parental MIL and MA susceptible strain. Furthermore, neutrophils exposed to drug-resistant lines showed increased activation, as revealed by decreased expression of CD62L and increased expression of CD66b in human neutrophils yet presented higher survival within neutrophils than the drug-susceptible strain. These results provide evidence that parasite drug-susceptibility may influences neutrophil activation and function as well as parasite survival within neutrophils. Further investigaton of the inter-relationship of drug susceptibility and neutrophil effector function should contribute to better understanding of the factors involved in susceptibility to anti-Leishmania drugs.
Introduction
Cutaneous leishmaniasis is an important public health problem affecting 98 countries and territories worldwide. Approximately 350 million people live in endemic areas and over 2 million new cases are reported annually (1). In Colombia, L. (V.) p. is responsible for the majority of reported cases of cutaneous leishmaniasis (2) though L. (V) braziliensis may be more frequent among occupationally exposed military personnel (3). The first line of treatment in Latin America (4) and most of the world, consists of parenteral administration of meglumine antimoniate (MA) (5). Oral miltefosine (MIL) is generally administered as the second line treatment. In recent years, there has been an increasing number of reports of treatment failure and loss of susceptibility of clinical Leishmania strains to antimonial drugs, and association of resistance in some cases of therapeutic failure in leishmaniasis patients. The reported frequency of MA and MIL tolerance ranges from 10 to 70% and varies among Leishmania species and by geographic origin. The underlying mechanisms contributing to anti-leishmanial treatment failure in Colombia and the rest of the world are poorly understood (6–8).
It is well established that the outcome of anti-leishmanial treatment is not solely dependent on parasite drug-susceptibility, but also on intrinsic host factors. The interplay between parasites, the host and the immune response is also a factor determining the clinical outcome of Leishmania infection and treatment (9, 10). There is currently a lack of information on the role of neutrophils in Leishmania infection and its impact on drug-susceptibility. Recently, high levels of transcriptional signatures associated with chemokines promoting neutrophil recruitment were observed in L. (V.) p. infected primary macrophages and in chronic cutaneous lesions of CL patients, suggesting a potential role for neutrophils in the chronicity of infection (11).
Neutrophils are essential phagocytes of the innate immune system and the most abundant leukocytes in human circulation (12). They usually are the first cells recruited to the site of infection upon pathogen entry into a host (13). Neutrophils possess three major effector killing strategies: phagocytosis and subsequent production of reactive oxygen species (ROS), the release of granules that contain microbicidal proteins (14) and the formation of neutrophil extracellular traps (NETs). NETs are fibrous structures composed of dsDNA coated with a variety of different microbicidial proteins that can trap and kill a variety of pathogens (15). Upon phagocytosis of pathogens, neutrophils produce different reactive oxygen species that are synthesized by the enzymatic activity of NADPH-synthase (16). Indeed, a lack of NADPH-function manifests itself in higher susceptibility to a variety of infections and is known as chronic granulomatous disease (17). Also a lack of Myeloperoxidase (MPO), an enzyme that catalyzes the synthesis of highly microbicidal hypochlorous acid, is thought to lead to increased susceptibility to infection (18). Neutrophil granules can either fuse with pathogen containing phagosomes or be released into the extracellular space (19).
Increasing evidence points toward a crucial role of neutrophils in leishmaniasis (20, 21). It has been shown that neutrophils are massively recruited to the site of infection upon infection with L. major (22–25), L. amazonensis (26), L. brazilensis (27), L. mexicana (28), L. infantum (29), and L. donovani (30, 31) and that a subset of L. mexicana can even use these cells to replicate (32). While neutrophils are well known to have a protective role in many infections they can have either beneficial or detrimental roles in leishmaniasis depending on the Leishmania spp. involved (21). In most studies performed in murine models, the lack of neutrophils was beneficial to disease outcome in Leishmania infection. Furthermore, parasites are known to use these cells as “Trojan horses” to enter silently into the host macrophages (33). In contrast, neutrophil presence has been reported to positively affect disease outcome in the case of L. braziliensis (25, 34–36), L. amazonensis (37) and L. donovani infection (38).
Here, using both human and mouse neutrophils, we have investigated in vitro the role of these cells in the context of anti-Leishmania drug resistance following L. (V.) p infection, focusing on whether drug resistance could be linked to elicitation of distinct neutrophil activation profiles.
We found that L. (V.) p. lines with divergent drug-susceptibility to miltefosine and meglumine antimoniate have different effects on neutrophil phenotype and functionality. Our data show that neutrophils are differentially activated by drug-resistant and drug-susceptible parasites and that drug-resistant parasites are less affected by neutrophil microbial function than drug-susceptible parasites. These differences in the elicitation of neutrophil function and parasite killing may influence anti-leishmanial drug therapy.
Materials and Methods
Mice
C57BL/6 mice (5–10 weeks old) were purchased from Envigo (Cambridgeshire, United Kingdom) and bred under specified pathogen-free conditions at the animal facility of the University of Lausanne in Epalinges.
Ethics Statement
All procedures involving human blood samples were approved by the Ethical Committee of the Canton of Vaud (CER-VD 2017-00182). Written informed consent was obtained from the healthy blood donors participating in this study. The study was conducted in compliance with the legislation of the Canton of Vaud and the Swiss Confederation as well as the declaration of Helsinki. Animal experimentation protocols were approved by the veterinary office of the Canton of Vaud (Authorization 1266.6-7 to F.T-C.) and were done in accordance to cantonal and federal law as well as the principles of the declaration of Basel. This study and the use of patient derived Leishmania strains and lines derived from these strains were approved by the Ethics Committees of CIDEIM- Colombia, in accordance with the national and international guidelines for Good Clinical Practice.
Leishmania (Viannia) Panamensis Parasites
The L. (V.) p. strain (MHOM/COL/86/1166LUC) WT-control was used to derive the strains resistant to potassium antimony (III) tartrate hydrate (39) and miltefosine as described previously (40). Briefly, L. (V.) p. promastigotes were cultured in media containing increasing concentrations of drugs, either up to 2885 μM Sb or up to 60 μM of miltefosine. Upon establishment of drug-resistance, the strains were cultured in RPMI (Gibco) with 10% FCS and 1% PNS at 26°C. Antimony resistant parasites (MHOM/COL/86/ LUC 1166-1000.1) were cultured in medium containing 1 mM/L SbV. The miltefosine resistant parasites (MHOM/COL/86/1166-LUC056) were cultured in medium containing 60 μM/L miltefosine. Luciferase expressing strains were grown in medium containing 60 μM G418 (Sigma).
Snarf-1 Staining of Leishmania (Viannia) Panamensis Parasites
Parasites were stained with the intracellular dye SNARF-1 (Thermofisher) to render them fluorescent. Parasites were incubated in 6 μM solution of SNARF-1 in PBS for 30 min and subsequently washed twice. SNARF-1 stained fluorescent parasites were detected at an excitation wavelength of 488 nm and an emission wavelength of 610 nm.
Drug-Susceptibility Screening
Drug-susceptibility of parasites was measured as a reduction of intracellular L. (V.) p. amastigote burden in macrophages derived from a U-937 cell line as described previously (40). Briefly, 1.2 × 105 U-937 cells were treated with phorbol 12-myristate 13-acetate (PMA; 100 ng/ml; Sigma) to differentiate them into macrophages. Cells were co-cultured with L. (V.) p. promastigotes opsonized with 10% AB positive human serum at a MOI of 5:1 and incubated for 24 h at 34°C. Subsequently, the culture medium was replaced with RPMI containing either 16 μM miltefosine or 32 μg/ml Sb(V) and the cells were incubated for another 48 h. The Sb-containing medium was replaced after 24 h whereas the miltefosine-containing medium was not replaced during the 48 h of incubation. Parasite burden was assessed by light microscopy in four replicates by 2 microscopists in a blinded manner. The cut-offs defining sensitive respectively resistant strains were based on a previously published analysis (40). A dose response survival curve was done to establish the half maximal response of the drugs on intracellular amastigote macrophage killing (EC50), as previoulsy described (8). Briefly, the EC50 in presence of miltefosine for the WT L. (V.) p. strain was 2.1 μM/liter and the macrophage infection was 67% for the L. (V.) p MIL-R stain; the EC50 was >32 μM/liter with 70% of macrophage infection. In presence of Sb(V), the EC50 for the WT L. (V.) p. was 10 μM/liter with 73% macrophage infection, and for the L. (V.) p. MA-R strain the EC50 was >128 μM/liter with macrophage infection of 69% (mean of three independent experiments).
Isolation Metacyclic Leishmania (Viannia) Panamensis Parasites
Metacyclic L. (V.) p. parasites were isolated using a Percoll (GE Healthcare) density gradient. Parasites were resuspended in 45% Percoll-medium suspension and layered onto 60% and 90% Percoll-medium suspension phases. After centrifugation without brakes at 4300 g for 45 min, metacyclic parasites were isolated from the 45%/60% Percoll-medium suspension interphase (41). The parasites were washed twice and counted using a Neubauer chamber.
Human Neutrophil Isolation
Peripheral blood neutrophils were isolated from venous blood of healthy volunteers. Density gradient centrifugation using polymorphprep (Progen) was performed and the enriched neutrophils were isolated according to the manufacturer's instructions. The remaining red blood cells were lysed using ACK-Buffer. Neutrophil purity was assessed using Cyto-Spin and Quick-Fix staining and was established to be ≥95%. Twenty-four hours post culture, 70% of neutrophils were viable (DAPI-). Infection with parasites, independently of the susceptibility of the parasites diminished neutrophil viability to 50–60%.
Murine Neutrophil Isolation
Bone marrow of the femora and tibia of C57BL/6 mice were flushed with RPMI. Erythrocytes were lysed with ACK buffer, the remaining leukocytes were washed and the neutrophils were then isolated by negative MACS using the neutrophil isolation kit (Miltenyi Biotec) according to the manufacturer's indications. At 24 h of culture, 90% of neutrophils were viable (DAPI−).
Assessment of Net Formation
3 × 105 Neutrophils were seeded on poly-L-Lysine coated coverslips, primed with 25 ng/mL GM-CSF and exposed for 4 h with either L. (V.) p., PMA (Phorbol-12-myristate-13-acetate, Sigma), PMA + DNase (Sigma) or without a stimulus. Subsequently, cells were fixed with 4% PFA (Paraformaldehyde, Sigma) and stained with rabbit anti-human MPO (Dako) primary antibody and Alexa Fluor-488 goat anti-rabbit secondary antibody (Life Technologies). Coverslips were mounted on glass slides using a DAPI containing mounting medium (Molecular Probes) and analyzed by confocal microscopy (ZEISS LSM 510). To quantify NET formation, NETs were counted using fluorescence microscopy in a blinded manner based on at least in three replicates. NET formation was also assessed through the measurement of dsDNA in the supernatant using the Quant-iT PicoGreen kit (Thermo Fisher) by adapting a technique previously described (42). 2 × 106 neutrophils were incubated for 4 h in X-vivo medium (Lonza) with either L. (V.) p., PMA or PMA + DNase or without stimulus. After incubation, the cell suspensions were centrifuged, and the supernatants were collected and transferred into black 96-well plates (Perkin Elmer). The picogreen dye was added and fluorescence was measured in using a plate reader (Molecular Devices, SpectraMax MiniMax 300) at an excitation wavelength of 480 nm and an emission wavelength of 520 nm.
Measurement of Reactive Oxygen Species Production
ROS production was measured using a luminol-based chemiluminescence assay. 5 × 105 neutrophils were incubated with either L. (V.) p., PMA or without stimulus in white, opaque 96-well plates (Perkin Elmer). Luminol (Carbosynth) was added at a final concentration of 20 μg/mL. ROS induced chemiluminescence was measured at all wavelengths with a plate reader at 5 min intervals during 1 h.
Parasite Viability Measurement With Luciferase Assay
5 × 105 neutrophils were infected with luciferase-expressing L. (V.) p. strains with different drug-susceptibility phenotypes during 2 h at 34°C at a MOI of 5:1, washed and subsequently incubated for 24 h. Parasite viability after incubation was measured using a luciferase assay system (Promega) according to the manufacturer's instructions. To measure parasite viability in presence or absence of NETs, neutrophils were incubated with or without 10 μg/ml DNase and parasite survival measured by chemi-luminescence as previously described (28). Chemiluminescence was measured in white, opaque 96-well plates in a plate reader (Molecular Devices, SpectraMax MiniMax 300) at all wavelengths.
Identification of Neutrophil Cell Surface Markers and Measurement of Cell Viability by Flow Cytometry
Surface activation markers and cell viability markers in blood derived human neutrophils were analyzed using Flow Cytometry analyzer of either the BD LSR II or the BD LSR Fortessa series (Becton Dickinson) and analyzed with FlowJo software (Tree Star). The following antibodies were used: Anti-human: CD15-APC, CD62L-PerCP-efluor710, CD66b-PE-Cy7. Anti-mouse: Ly6G(1A8)-APC-Cy7, CD62L-PE, CD11b-PE-Cy7 (all from e-Bioscience). Cell viability: Live/Dead fixable Aqua Dead Cell Stain Kit (Invitrogen).
Results
Increased Reactive Oxygen Species Production by Murine and Human Neutrophils Challenged With Miltefosine-Resistant Leishmania (Viannia) Panamensis Strains
To investigate the contribution of neutrophils in the intracellular survival of Leishmania during drug exposure, we first investigated whether L. (V.) p. strains susceptible or resistant to MA or MIL would induce different phenotype and function in neutrophils. The production of ROS by neutrophils is known to be an important defense mechanism against pathogens (43) and also contributes to NET formation (44). Therefore, we analyzed ROS production during the first hour of incubation of neutrophils with the different L. (V.) p. populations. Parasites that are resistant to miltefosine induced 1.5 times more ROS production compared to drug-susceptible parasites in BM-derived murine neutrophils (Figures 1A,B) and more than two times as much ROS production than drug-susceptible parasites in blood-derived human neutrophils from healthy donors (Figures 1C,D). In contrast, the induction of ROS by antimony resistant parasites strains in neutrophils did not differ from drug-susceptible L. (V.) p. parasites. Even though all L. (V.) p. strains induced ROS in neutrophils, these results show that the drug-susceptibility phenotype, specifically MIL-resistance, influences neutrophil ROS production.
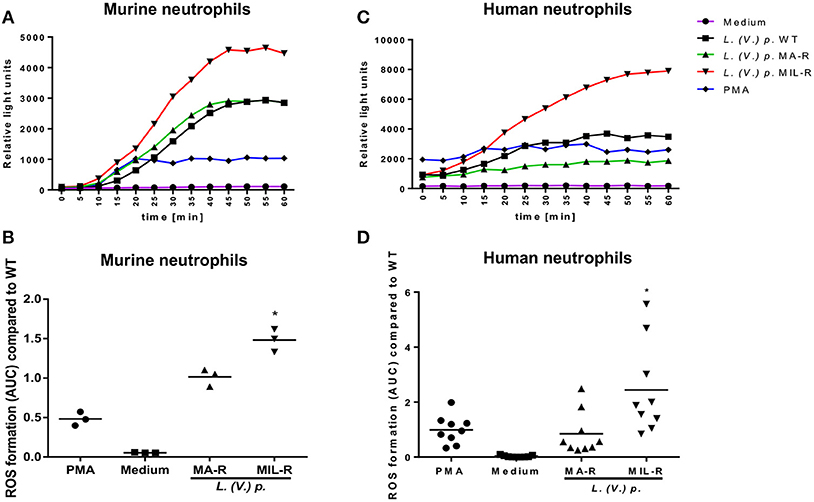
Figure 1. Miltefosine (MIL)-resistant L. (V.) p. parasites induce more ROS production in murine and human neutrophils than the meglumine antimony (MA)-resistant or drug susceptible strains. ROS production was measured using a luminol based chemiluminescence assay. Bone marrow derived murine neutrophils and blood-derived human neutrophils from healthy donors were incubated with either MIL-, MA-resistant or susceptible L. (V.) p., PMA or without stimulus. Luminol was added and ROS-induced chemiluminescence was measured with a plate reader every 5 min during 1 h. (A,C) One representative curve of ROS-induced luminol chemiluminescence values in murine (A) and human (C) neutrophils. (B,D) The corresponding area under the curve (AUC) at 60 min of measurement for murine (B) and human (D) neutrophils. *p < 0.05, *a MIL-R compared to WT, *b MIL-R compared to MA-R, WT, wild type; MA-R, Resistant to meglumine antimoniate; MIL-R, Resistant to miltefosine; the data are pooled from three (human) or two (mouse) independent experiments.
Drug-Resistant Leishmania (Viannia) Panamensis Induce More Neutrophil Extracellular Trap Formation Than Drug-Susceptible Strains in Murine and Human Neutrophils
Next, to visualize NETs, neutrophils were infected with L. (V.) p. of different drug susceptibility and NET formation analyzed by confocal microscopy. NETs were stained with DAPI to detect DNA filaments and an MPO mAb to detect the MPO associated with it. All parasite populations tested induced NET formation in both murine and human neutrophils as observed by DNA-MPO co-localization (Figure 2A). Drug resistant parasites induced substantially more NETs (two to three times) in murine and human neutrophils compared to drug-susceptible parasites (Figures 2B,C). We observed parasites in association with the NETs, a process more easily detectable in human NETs. To confirm these results, we used the picogreen assay that measures the amount of dsDNA released by neutrophils into the supernatant (42). We again observed that drug-resistant L. (V.) p. parasites induced a significantly higher amount of dsDNA release compared to drug-susceptible parasites in human and murine neutrophils (Figure 3). Collectively, these results demonstrate that MIL and MA-resistant L. (V.) p lines induce more NET formation than the parental drug-susceptible strain.
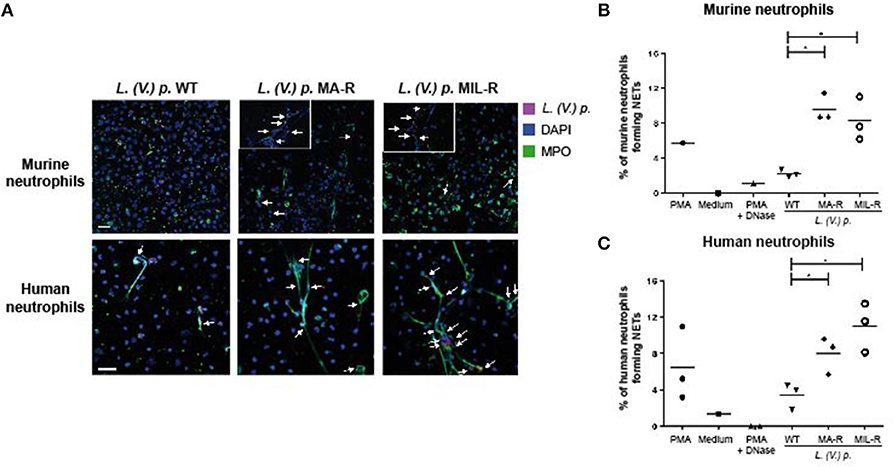
Figure 2. Murine and human neutrophils form more NETs when exposed to L. (V) p. strains that are resistant to either meglumine antimonate or miltefosine. Neutrophils were seeded on poly-L-Lysine coated coverslips and exposed for 4 h with either L. (V.) p.of the indicated drug suceptibility, PMA, PMA + DNase or without stimulus. Subsequently, cells were fixed, stained with anti-human MPO and DAPI (A) Confocal microscopy images of neutrophils upon exposure to L. (V.) p. murine or human neutrophils exposed to strains of SNARF-1 labeled L. (V.) p. promastigotes lines that were either resistant or susceptible to meglumine antimonate (MA) or miltefosine (MIL) at a 5:1 parasite-cell MOI. (B,C) The frequency of murine (B) or human (C) neutrophils forming NETs was counted with a fluorescence microscope. *p < 0.05, each point corresponds to one healthy blood donor or one mouse. One representative experiment out of four is shown. The arrows point to colocalization of parasites with NETs. Zoom in of NETs are shown for murine neutrophils -The scale bar corresponds to 50 μM.
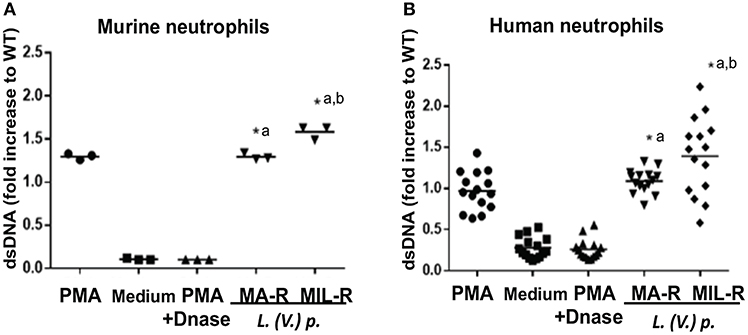
Figure 3. Murine and human neutrophils release more dsDNA when exposed to L. (V) p. strains that are resistant to either MA or MIL. Bone marrow derived murine neutrophils (A) and blood-derived human neutrophils from healthy donors (B) were exposed to L. (V.) p. of the indicated susceptibility at a 5:1 MOI. As controls, incubations with PMA, PMA + DNase or without stimulus were also performed. Four hours later, NET formation was quantified by measuring the levels of double stranded DNA (dsDNA) released in the supernatant using the PicoGreen fluorescent dye assay. *p < 0.05, *a MA-R or MIL-R compared to WT, *b MIL-R compared to MA-R, Each point corresponds to one healthy blood donor or one mouse. One representative experiment out of four (human) and three (mice)independent experiments is shown.
Modulation of Neutrophil Activation Markers by Leishmania (Viannia) Panamensis of Different Drug-Susceptibility Phenotypes
We next investigated if exposure of neutrophils to L. (V.) p. of different drug-susceptibility would result in distinct modulations of cell surface markers. Using flow cytometry, we observed that BM-derived murine neutrophils exposed to MIL and MA drug-resistant L. (V.) p lines expressed more CD11b (Mac-1) and CD62L (L-selectin) on their surface than neutrophils exposed to drug-susceptible L. (V.) p. (Figure 4). CD62L and CD66b (exocytosis of secondary granules) expression by human neutrophils derived from the blood of healthy individuals exposed to drug-susceptible and resistant L. (V.) p was analyzed by flow cytometry (Figures 5A–C). MA and MIL resistant parasites elicited a decrease in expression of CD62L and an increase in expression of CD66b (Figures 5D–E). These data show that both human and murine neutrophils exposed to drug-resistant L. (V.) p. lines are activated to a greater extent and have a phenotype that is more associated with extravasation, compared to neutrophils challenged with drug-susceptible L. (V.) p.
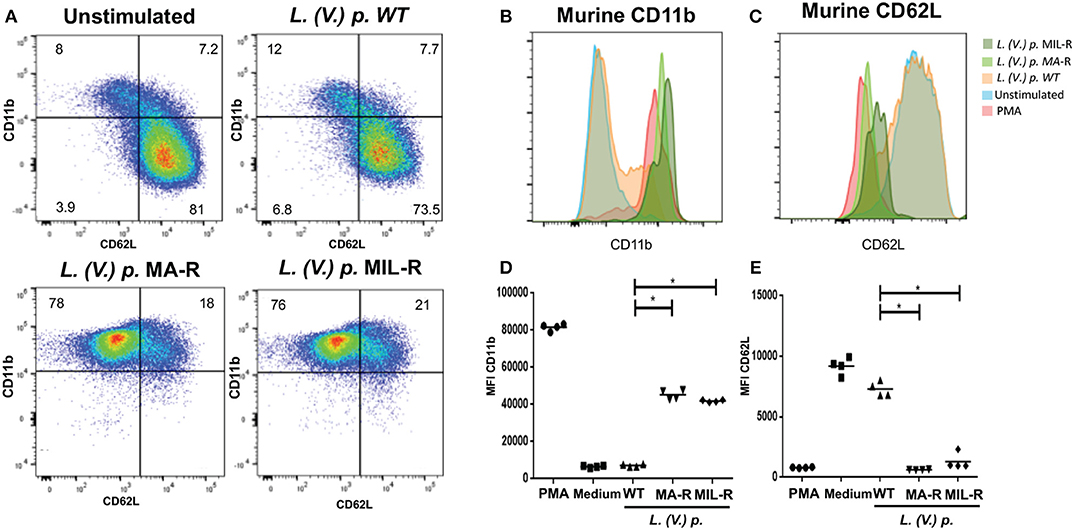
Figure 4. Expression of cell surface activation markers of murine neutrophils in response to exposure to L. (V.) p. promastigotes with different drug-susceptibility phenotypes. Bone marrow derived murine neutrophils were exposed to the indicated L. (V.) p. lines at a MOI of 1:10 or without stimulus for 90 min. Incubation with PMA was used as a positive control. Neutrophils were then stained with the CD11b and CD62L mAbs and surface expression was analyzed by flow cytometry. (A) Representative flow cytometry plot indicating CD11b and CD62L positive and negative populations. (B,C) A representative histogram plot of normalized fluorescence values of murine neutrophils stained with antibodies against CD11b and CD62L is shown. Normalized fluorescence values of murine neutrophils stained with antibodies against CD11b and CD62L is shown. (C,D) MFI values of murine neutrophils stained with antibodies against CD62L and CD11b. WT, Wild type; MA-R, Resistant to meglumine antimoniate; MIL-R, Resistant to miltefosine. The data is representative of three experiments. *p < 0.05.
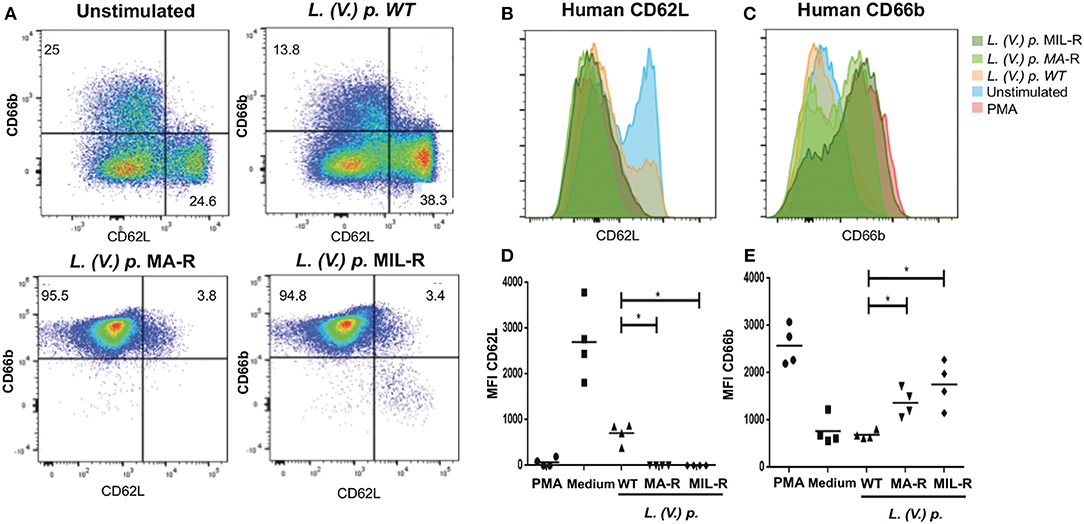
Figure 5. Expression of surface activation markers of human neutrophils in response to exposure to L. (V.) p. metacyclic promastigotes of different drug-susceptibility phenotypes. Blood-derived neutrophils from healthy donors were exposed to the indicated L. (V.) p. lines at a MOI of 1:10 or without stimulus for 90 min. Incubation with PMA was used as a positive control. Neutrophils were then stained with the CD62L and CD66b mAbs and surface expression was analyzed by flow cytometry. (A) Representative flow cytometry plot indicating CD62L and CD66b positive and negative populations. (B,C) One representative flow cytometry plot of normalized fluorescence values of human neutrophils stained with antibodies against CD62L and CD66b that were exposed to different L. (V.) p. strains at a MOI of 10. Is shown (D,E) MFI values of human neutrophils stained with antibodies against CD62L and CD11b. WT, Wild type; MA-R, Resistant to meglumine antimoniate; MIL-R, Resistant to miltefosine. The data is representative of a total of five experiments. *p < 0.05.
Drug-Resistant Leishmania (Viannia) Panamensis Strains Are More Resistant to Neutrophil Parasite Killing 24 h After Infection
As we observed differences in ROS production, NET formation and neutrophil activation in neutrophils exposed to L. (V.) p. of different drug-susceptibility phenotypes, we assessed whether this would have an impact on parasite killing. Luciferase expressing L. (V.) p. lines of the indicated drug-susceptibility phenotypes were exposed to murine or human neutrophils for 24 h and parasite viability was analyzed by measuring luciferase activity. Drug-resistant parasites were significantly more resistant to neutrophil killing by murine and human neutrophils than the drug-susceptible strain (Figures 6A,B). The frequency of neutrophil infection observed 4 h after infection with MA-R and MIL-R L. (V.) was significantly higher for drug-resistant parasites than WT parasites (Figure 6C) and this difference persisted 24 h post infection, suggesting diminished susceptibility to neutrophil killing. To evaluate the possible impact of NETs on parasite killing, luciferase-expressing WT, MIL-R and MA-R L. (V.) p parasites were exposed to netting neutrophils in presence or absence of DNAse. Four hours later, the frequency of viable luciferase expressing parasites was determined as previously described (28). The presence of DNAse did not change the relative number of WT parasites, suggesting no impact of NETs on parasite viability. In contrast, treatment of NETs with DNAse significantly increased the number of MA-R and even more so that of MIL-R L.(V.) p parasites, suggesting partial killing of the parasites by NETs (Figure 6D). Taken together, our data demonstrate that in both murine and human neutrophils, drug-resistant L. (V.) p. lines induce more mechanisms known to kill pathogens than the drug-susceptible parasites, yet they are better able to resist neutrophil killing even though partial killing by NETs was observed.
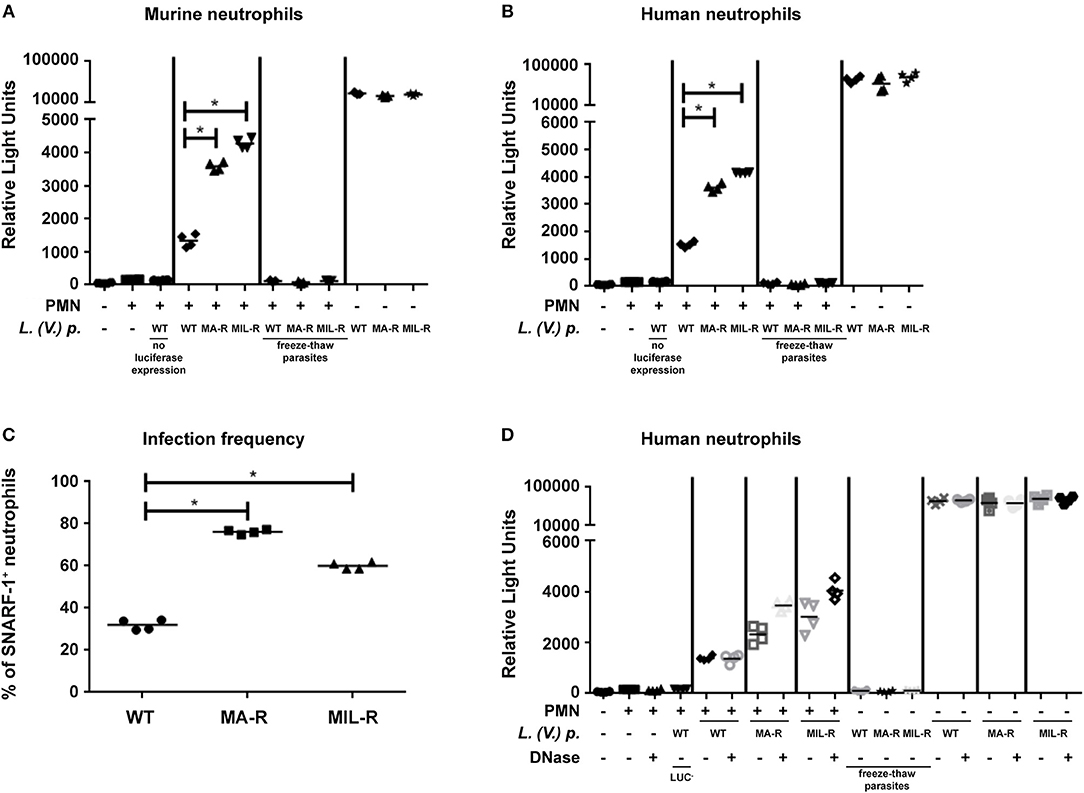
Figure 6. Killing of L. (V.) p. of different drug susceptibilities by murine and human neutrophils. Neutrophils were infected with luciferase expressing L. (V.) p. lines with different drug-susceptibility phenotypes for 2 h at 34°C, washed and further incubated for 24 h prior to analysis. Parasite viability after incubation was measured using a luciferase assay system. Murine (A) and human (B) neutrophils were exposed to luciferase expressing L. (V.) p. of the indicated drug susceptibility at a 5:1 MOI. 24 h later chemiluminescence was measured represented as relative light units and parasite killing was assessed MIL-R: Resistant to miltefosine, MA-R: Resistant to meglumine antimoniate, freeze-thaw parasites were killed by 10 times of subsequent freezing and thawing, PMN: Neutrophils. One representative experiment is shown out of four. (C) Infection frequency of neutrophils 4 h after exposure to the parasites of the indicated drug susceptibility. (D) Assessment of parasite killing by NETs. Human neutrophils were exposed to parasites of the indicated susceptibility as in (B), but in addition, DNAse was added to the neutrophils as indicated. This are representative experiments of two.
Discussion
Anti-leishmanial drug-resistance is an increasing concern in Colombia, but also worldwide (45–47). In India miltefosine has replaced antimony as the first line of treatment against visceral leishmaniasis due to widespread evidence of antimony-resistance (48). However, the efficacy of miltefosine has also dropped considerably during the first 10 years following its introduction (49–51). It is crucial to consider that parasite drug-resistance is not equal to clinical treatment failure. Therapeutic response is also determined by host factors, such as previous infection, co-morbidities, nutritional status, pharmacokinetics etc. (52). Nevertheless, drug-resistance is a very important determinant of treatment failure, thus understanding how drug-resistance phenotype of L. (V.) p. impacts the anti-leishmanial immune response is relevant to the understanding of the potential role of neutrophils in therapeutic outcome.
Here, we have demonstrated for the first time that L. (V.) p. parasites having different drug-susceptibility phenotypes elicit distinct neutrophil effector functions, shown by a higher induction of ROS production, NET formation and the expression of surface markers characteristic of neutrophil activation. ROS production is a crucial microbicidal mechanism of neutrophils, but the impact of ROS on Leishmania survival is species and host dependent (53). Here, we showed that L. (V.) p. induces ROS production in murine and human neutrophils and that ROS production is increased when murine and human neutrophils are infected with the miltefosine-resistant L (V.) p. compared to the drug-susceptible parasites. In contrast, exposure of neutrophils to MA-R L. (V.) p. parasites had no significant impact on ROS production, suggesting that increased ROS production is not a general feature of parasites resistant to different drugs.
It has been reported that ROS production is critical for the induction of ROS-dependent NET formation (54). Activation and nuclear translocation of neutrophil elastase are crucial in ROS-dependent NET formation, reviewed in Papayannopoulos (55) and Brinkmann (56). However, there is also an NOX-independent pathway of NET formation that does not depend on ROS (57). L. amazonensis has been reported to induce ROS-independent and ROS-dependent NET formation. In our study, we show that MA-resistant and MIL-resistant L. (V.) p. induce increased in NET formation compared to that of susceptible strains, however only MIL-resistant L. (V.) p. induced an increased ROS production compared to that obtained from the drug-susceptible strain. These data suggests that the NET formation induced by MA-resistant L. (V.) p. may be partly ROS-independent.
The distinct activation profiles elicited by parasites having a different drug resistance phenotype are consistent with the absence of cross resistance i.e., Sb resistant parasites are not also resistant to miltefosine and vice-versa nor is there implication of a generic multi drug resistance mechanism. Resistance to different drugs is likely the outcome of the convergence of host cell and parasite responses to infection, their response to the drug, and drug effects on the host response. Our data implicate an association between the characteristics of the resistant and susceptible parasite population and neutrophil response. These characteristics could segregate with the susceptibility phenotype without being directly and causally involved in drug susceptibility. The neutrophil response elicited could enable the microbicidal effect of the drug, or drug resistance could provide a protective effect to neutrophil defense mechanisms as described for Sb resistance and lower nitric oxide susceptibility reported for some Leishmania spp. (58, 59).
Neutrophil effector functions contribute to microbial destruction in most infections, however, their role in leishmaniasis varies, depending on host factors and the Leishmania spp. involved (21, 53). We observed L. (V.) p. parasites associated with the filamentous structures of NETs, which substantiates parasite trapping by these structures. Most Leishmania spp. are trapped by NETs. Some, such as L. mexicana (28), L. donovani (60) or L. infantum (61) survive NET exposure, while others such as L. amazonensis (61–63) are killed by NETs, at least in their promastigote stage. The higher survival of drug-resistant parasites despite induction of greater ROS and NET response suggests that these L. (V.) p. lines resist better to neutrophil killing than L. (V.) p. susceptible strains. This is in line with studies performed on macrophages, reporting that MA as well as MIL-resistant Leishmania donovani strains were more resistant to ROS mediated killing due to increased intracellular thiol-levels (64–68). Neutrophil recruitment and increased neutrophil activation are known to play a key role in many inflammatory diseases (69, 70). Our results demonstrate that there is an increase in neutrophil activation upon stimulation by drug-resistant L. (V.) p. compared to drug-susceptible parasites. It is therefore conceivable that treatment failure may result at least in part due to the increased resistance of L. (V.) p. strains to neutrophil effector functions. An increase in parasite survival would then lead to an increase of inflammation and in turn would lead to more neutrophil recruitment and activation, and potentially constitute a self-sustaining circle.
In this study, we used laboratory-derived L. (V.) p. drug-resistant lines. Since there are differences in the drug-resistance mechanisms of parasites that develop drug-resistance in vivo compared to those experimentally selected for drug-resistance in vitro (7, 71) the assessment of the interaction of neutrophils and L. (V.) p. with different drug-susceptibility phenotypes that have been isolated from patients in a clinical setting should further elucidate of the role of the interaction of L. (V.) p. and neutrophils in anti-leishmanial responses. Also, the study of the in vivo immune response of neutrophils against those parasites would contribute to better understanding of the role the immune system plays in anti-leishmanial treatment outcome.
The concurrence of the present findings in mouse and human neutrophils strengthens the observations and provides a surrogate model to identify the mechanisms involved, in follow-up investigations based on these findings. Further understanding of the effect of drug-resistant parasites on neutrophil function, including the analysis ROS production and NET formation ex vivo (e.g., neutrophils from lesion biopsies or patient blood samples), should be further investigated to assess ehether this neutrophil activation could constitute a surrogate marker for treatment outcome.
Author Contributions
IR and FT-C conceived the experiments. NS, OF, and MG contributed to the design and interpretation of the experiments and provided and authenticated the L. (V.) p. strains and lines of defined drug susceptibility phenotype. IR, OF, and BM-S performed the experiments and analyzed the data. IR wrote the manuscript, FT-C, OF, BM-S, MG, and NS contributed to and critically reviewed the manuscript.
Funding
This work was supported by a bilateral research collaboration between Switzerland and Colombia (Seed money grant and Merger grant) from the Leading House for the Latin American Region, and in part by NIH/NIAID Tropical Medicine Research Centers (TMRC) grant U19AI129910. It was also in part supported by the Swiss National Science Foundation (300030_166651 to FT-C).
Conflict of Interest Statement
The authors declare that the research was conducted in the absence of any commercial or financial relationships that could be construed as a potential conflict of interest.
Acknowledgments
We thank Yazmin Hauyon-La Torre, Lady Giovanna Ramirez Hincapie and Maryori Vidarte for technical assistance, Dr. Benjamin Hurrell for discussion, CIDEIM personnel in Calí, Colombia, the Cellular Imaging Facility and the Flow Cytometry Facility of the University of Lausanne, Switzerland.
References
1. Alvar J, Velez ID, Bern C, Herrero M, Desjeux P, Cano J, et al. Leishmaniasis worldwide and global estimates of its incidence. PLoS ONE (2012) 7:e35671. doi: 10.1371/journal.pone.0035671
2. Ramirez JD, Hernandez C, Leon CM, Ayala MS, Florez C, Gonzalez C. Taxonomy, diversity, temporal and geographical distribution of Cutaneous Leishmaniasis in Colombia: a retrospective study. Sci Rep. (2016) 6:28266. doi: 10.1038/srep28266
3. Patino LH, Mendez C, Rodriguez O, Romero Y, Velandia D, Alvarado M, et al. Spatial distribution, Leishmania species and clinical traits of Cutaneous Leishmaniasis cases in the Colombian army. PLoS Negl Trop Dis. (2017) 11:e0005876. doi: 10.1371/journal.pntd.0005876
4. Organización Panamericana de la Salud. Leishmaniasis en las Américas: Recomendaciones Para el Tratamiento. Washington, DC: Organización Panamericana de la Salud (2013).
5. Mitropoulos P, Konidas P, Durkin-Konidas M. New World cutaneous leishmaniasis: updated review of current and future diagnosis and treatment. J Am Acad Dermatol. (2010) 63:309–22. doi: 10.1016/j.jaad.2009.06.088
6. Rojas R, Valderrama L, Valderrama M, Varona MX, Ouellette M, Saravia NG. Resistance to antimony and treatment failure in human Leishmania (Viannia) infection. J Infect Dis. (2006) 193:1375–83. doi: 10.1086/503371
7. Goyeneche-Patino DA, Valderrama L, Walker J, Saravia NG. Antimony resistance and trypanothione in experimentally selected and clinical strains of Leishmania panamensis. Antimicrob Agents Chemother. (2008) 52:4503–6. doi: 10.1128/AAC.01075-08
8. Obonaga R, Fernandez OL, Valderrama L, Rubiano LC, Castro Mdel M, Barrera MC, et al. Treatment failure and miltefosine susceptibility in dermal leishmaniasis caused by Leishmania subgenus Viannia species. Antimicrob Agents Chemother (2014) 58:144–52. doi: 10.1128/AAC.01023-13
9. Croft SL, Sundar S, Fairlamb AH. Drug resistance in leishmaniasis. Clin Microbiol Rev. (2006) 19:111–26. doi: 10.1128/CMR.19.1.111-126.2006
10. Uliana SRB, Trinconi CT, Coelho AC. Chemotherapy of leishmaniasis: present challenges. Parasitology (2018) 145:464–80. doi: 10.1017/S0031182016002523
11. Navas A, Vargas DA, Freudzon M, McMahon-Pratt D, Saravia NG, Gomez MA. Chronicity of dermal leishmaniasis caused by Leishmania panamensis is associated with parasite-mediated induction of chemokine gene expression. Infect Immun. (2014) 82:2872–80. doi: 10.1128/IAI.01133-13
12. Nauseef WM, Borregaard N. Neutrophils at work. Nat Immunol. (2014) 15:602–11. doi: 10.1038/ni.2921
13. Branzk N, Papayannopoulos V. Molecular mechanisms regulating NETosis in infection and disease. Semin Immunopathol. (2013) 35:513–30. doi: 10.1007/s00281-013-0384-6
14. Segal AW. How neutrophils kill microbes. Annu Rev Immunol. (2005) 23:197–223. doi: 10.1146/annurev.immunol.23.021704.115653
15. Brinkmann V, Reichard U, Goosmann C, Fauler B, Uhlemann Y, Weiss DS, et al. Neutrophil extracellular traps kill bacteria. Science (2004) 303:1532–5. doi: 10.1126/science.1092385
16. Roos D, van Bruggen R, Meischl C. Oxidative killing of microbes by neutrophils. Microbes Infect. (2003) 5:1307–15. doi: 10.1016/j.micinf.2003.09.009
17. Thomas DC. How the phagocyte NADPH oxidase regulates innate immunity. Free Radic Biol. Med. (2018) 125:44–52. doi: 10.1016/j.freeradbiomed.2018.06.011
18. Winterbourn CC, Kettle AJ, Hampton MB. Reactive oxygen species and neutrophil function. Annu Rev Biochem. (2016) 85:765–92. doi: 10.1146/annurev-biochem-060815-014442
19. Mayadas TN, Cullere X, Lowell CA. The multifaceted functions of neutrophils. Annu Rev Pathol. (2014) 9:181–218. doi: 10.1146/annurev-pathol-020712-164023
20. Ribeiro-Gomes FL, Sacks D. The influence of early neutrophil-Leishmania interactions on the host immune response to infection. Front Cell Infect Microbiol. (2012) 2:59. doi: 10.3389/fcimb.2012.00059
21. Hurrell BP, Regli IB, Tacchini-Cottier F. Different leishmania species drive distinct neutrophil functions. Trends Parasitol. (2016) 32:392–401. doi: 10.1016/j.pt.2016.02.003
22. Tacchini-Cottier F, Zweifel C, Belkaid Y, Mukankundiye C, Vasei M, Launois P, et al. An immunomodulatory function for neutrophils during the induction of a CD4+ Th2 response in BALB/c mice infected with Leishmania major. J Immunol. (2000) 165:2628–36. doi: 10.4049/jimmunol.165.5.2628
23. Peters NC, Egen JG, Secundino N, Debrabant A, Kimblin N, Kamhawi S, et al. In vivo imaging reveals an essential role for neutrophils in leishmaniasis transmitted by sand flies. Science (2008) 321:970–4. doi: 10.1126/science.1159194
24. Ribeiro-Gomes FL, Roma EH, Carneiro MB, Doria NA, Sacks DL, Peters NC. Site-dependent recruitment of inflammatory cells determines the effective dose of Leishmania major. Infect Immun. (2014) 82:2713–27. doi: 10.1128/IAI.01600-13
25. Lee SH, Charmoy M, Romano A, Paun A, Chaves MM, Cope FO, et al. Mannose receptor high, M2 dermal macrophages mediate nonhealing Leishmania major infection in a Th1 immune environment. J Exp Med. (2018) 215:357–75. doi: 10.1084/jem.20171389
26. Sousa LM, Carneiro MB, Resende ME, Martins LS, Dos Santos LM, Vaz LG, et al. Neutrophils have a protective role during early stages of Leishmania amazonensis infection in BALB/c mice. (2014) Parasite Immunol. 36:13–31. doi: 10.1111/pim.12078
27. Falcao SA, Weinkopff T, Hurrell BP, Celes FS, Curvelo RP, Prates DB, et al. Exposure to Leishmania braziliensis triggers neutrophil activation and apoptosis. PLoS Negl Trop Dis. (2015) 9:e0003601. doi: 10.1371/journal.pntd.0003601
28. Hurrell BP, Schuster S, Grun E, Coutaz M, Williams RA, Held W, et al. Rapid sequestration of Leishmania mexicana by neutrophils contributes to the development of chronic lesion. PLoS Pathog. (2015) 11:e1004929. doi: 10.1371/journal.ppat.1004929
29. Thalhofer CJ, Chen Y, Sudan B, Love-Homan L, Wilson ME. Leukocytes infiltrate the skin and draining lymph nodes in response to the protozoan Leishmania infantum chagasi. Infect Immun. (2011) 79:108–17. doi: 10.1128/IAI.00338-10
30. McFarlane E, Carter KC, McKenzie AN, Kaye PM, Brombacher F, Alexander J. Endogenous IL-13 plays a crucial role in liver granuloma maturation during Leishmania donovani infection, independent of IL-4Ralpha-responsive macrophages and neutrophils. J Infect Dis. (2011) 204:36–43. doi: 10.1093/infdis/jir080
31. Dey R, Joshi AB, Oliveira F, Pereira L, Guimaraes-Costa AB, Serafim TD, et al. Gut microbes egested during bites of infected sand flies augment severity of leishmaniasis via inflammasome-derived IL-1beta. Cell Host Microbe (2018) 23:134–143 e136. doi: 10.1016/j.chom.2017.12.002
32. Hurrell BP, Beaumann M, Heyde S, Regli IB, Muller AJ, Tacchini-Cottier F. Frontline Science: Leishmania mexicana amastigotes can replicate within neutrophils. J Leukoc Biol. (2017) 102:1187–98. doi: 10.1189/jlb.4HI0417-158R
33. Laskay T, van Zandbergen G, Solbach W. Neutrophil granulocytes–Trojan horses for Leishmania major and other intracellular microbes? Trends Microbiol. (2003) 11:210–4. doi: 10.1016/S0966-842X(03)00075-1
34. Souza-Lemos C, de-Campos SN, Teva A, Corte-Real S, Fonseca EC, Porrozzi R, et al. Dynamics of immune granuloma formation in a Leishmania braziliensis-induced self-limiting cutaneous infection in the primate Macaca mulatta. J Pathol. (2008) 216:375–86. doi: 10.1002/path.2403
35. Novais FO, Santiago RC, Bafica A, Khouri R, Afonso L, Borges VM, et al. Neutrophils and macrophages cooperate in host resistance against Leishmania braziliensis infection. J Immunol. (2009) 183:8088–98. doi: 10.4049/jimmunol.0803720
36. Carlsen ED, Jie Z, Liang Y, Henard CA, Hay C, Sun J, et al. Interactions between neutrophils and Leishmania braziliensis amastigotes facilitate cell activation and parasite clearance. J Innate Immun. (2015) 7:354–63. doi: 10.1159/000373923
37. Tavares NM, Araujo-Santos T, Afonso L, Nogueira PM, Lopes UG, Soares RP, et al. Understanding the mechanisms controlling Leishmania amazonensis infection in vitro: the role of LTB4 derived from human neutrophils. J Infect Dis. (2014) 210:656–66. doi: 10.1093/infdis/jiu158
38. McFarlane E, Perez C, Charmoy M, Allenbach C, Carter KC, Alexander J, et al. Neutrophils contribute to development of a protective immune response during onset of infection with Leishmania donovani. Infect Immun. (2008) 76:532–41. doi: 10.1128/IAI.01388-07
39. Brochu C, Wang J, Roy G, Messier N, Wang XY, Saravia NG, et al. Antimony uptake systems in the protozoan parasite Leishmania and accumulation differences in antimony-resistant parasites. Antimicrob Agents Chemother. (2003) 47:3073–9. doi: 10.1128/AAC.47.10.3073-3079.2003
40. Fernandez O, Diaz-Toro Y, Valderrama L, Ovalle C, Valderrama M, Castillo H, et al. Novel approach to in vitro drug susceptibility assessment of clinical strains of Leishmania spp. J Clin Microbiol. (2012) 50:2207–11. doi: 10.1128/JCM.00216-12
41. Castilho TM, Goldsmith-Pestana K, Lozano C, Valderrama L, Saravia NG, McMahon-Pratt D. Murine model of chronic L. (Viannia) panamensis infection: role of IL-13 in disease. Eur J Immunol. (2010) 40:2816–29. doi: 10.1002/eji.201040384
42. Amini P, Stojkov D, Wang X, Wicki S, Kaufmann T, Wong WW, et al. NET formation can occur independently of RIPK3 and MLKL signaling. Eur J Immunol. (2016) 46:178–84. doi: 10.1002/eji.201545615
43. Nguyen GT, Green ER, Mecsas J. Neutrophils to the ROScue: mechanisms of NADPH Oxidase Activation and Bacterial Resistance. Front Cell Infect Microbiol. (2017) 7:373. doi: 10.3389/fcimb.2017.00373
44. Papayannopoulos V. (2015). Sweet NETs, bitter wounds. Immunity 43:223–5. doi: 10.1016/j.immuni.2015.08.002
45. Azeredo-Coutinho RB, Mendonca SC, Callahan H, Portal AC, Max G. Sensitivity of Leishmania braziliensis promastigotes to meglumine antimoniate (glucantime) is higher than that of other Leishmania species and correlates with response to therapy in American tegumentary leishmaniasis. J Parasitol. (2007) 93:688–93. doi: 10.1645/GE-1031R.1
46. Fernandez OL, Diaz-Toro Y, Ovalle C, Valderrama L, Muvdi S, Rodriguez I, et al. Miltefosine and antimonial drug susceptibility of Leishmania Viannia species and populations in regions of high transmission in Colombia. PLoS Negl Trop Dis. (2014) 8:e2871. doi: 10.1371/journal.pntd.0002871
47. Bilbao-Ramos P, Dea-Ayuela MA, Cardenas-Alegria O, Salamanca E, Santalla-Vargas JA, Benito C, et al. Leishmaniasis in the major endemic region of Plurinational State of Bolivia: species identification, phylogeography and drug susceptibility implications. Acta Trop. (2017) 176:150–61. doi: 10.1016/j.actatropica.2017.07.026
48. Vanaerschot M, De Doncker S, Rijal S, Maes L, Dujardin JC, Decuypere S. Antimonial resistance in Leishmania donovani is associated with increased in vivo parasite burden. PLoS ONE (2011) 6:e23120. doi: 10.1371/journal.pone.0023120
49. Sundar S, Chakravarty J. Recent advances in the diagnosis and treatment of kala-azar. Natl Med J India (2012) 25:85–89.
50. Rijal S, Ostyn B, Uranw S, Rai K, Bhattarai NR, Dorlo TP, et al. Increasing failure of miltefosine in the treatment of Kala-azar in Nepal and the potential role of parasite drug resistance, reinfection, or noncompliance. Clin Infect Dis. (2013) 56:1530–8. doi: 10.1093/cid/cit102
51. Sundar S, Sinha P, Jha TK, Chakravarty J, Rai M, Kumar N, et al. Oral miltefosine for Indian post-kala-azar dermal leishmaniasis: a randomised trial. Trop Med Int Health (2013) 18:96–100. doi: 10.1111/tmi.12015
52. Castro MDM, Cossio A, Velasco C, Osorio L. Risk factors for therapeutic failure to meglumine antimoniate and miltefosine in adults and children with cutaneous leishmaniasis in Colombia: a cohort study. PLoS Negl Trop Dis. (2017) 11:e0005515. doi: 10.1371/journal.pntd.0005515
53. Regli IB, Passelli K, Hurrell BP, Tacchini-Cottier F. Survival mechanisms used by some leishmania species to escape neutrophil killing. Front Immunol. (2017) 8:1558. doi: 10.3389/fimmu.2017.01558
54. Branzk N, Lubojemska A, Hardison SE, Wang Q, Gutierrez MG, Brown GD, et al. Neutrophils sense microbe size and selectively release neutrophil extracellular traps in response to large pathogens. Nat Immunol. (2014) 15:1017–25. doi: 10.1038/ni.2987
55. Papayannopoulos V. Neutrophil extracellular traps in immunity and disease. Nat Rev Immunol. (2018) 18:134–47. doi: 10.1038/nri.2017.105
56. Brinkmann V. Neutrophil extracellular traps in the second decade. J Innate Immun. (2018) 10:414–21. doi: 10.1159/000489829
57. Manfredi AA, Ramirez GA, Rovere-Querini P, Maugeri N. The neutrophil's choice: phagocytose vs make neutrophil extracellular traps. Front Immunol. (2018) 9:288. doi: 10.3389/fimmu.2018.00288
58. Holzmuller P, Sereno D, Lemesre JL. Lower nitric oxide susceptibility of trivalent antimony-resistant amastigotes of Leishmania infantum. Antimicrob Agents Chemother. (2005) 49:4406–9. doi: 10.1128/AAC.49.10.4406-4409.2005
59. de Albuquerque AC, Moraes MF, Silva AC, Lapera IM, Tebaldi JH, Lux Hoppe EG. Helminth fauna of chiropterans in Amazonia: biological interactions between parasite and host. Parasitol Res. (2016) 115:3229–37. doi: 10.1007/s00436-016-5085-3
60. Gabriel C, McMaster WR, Girard D, Descoteaux A. Leishmania donovani promastigotes evade the antimicrobial activity of neutrophil extracellular traps. J Immunol. (2010) 185:4319–27. doi: 10.4049/jimmunol.1000893
61. Guimaraes-Costa AB, DeSouza-Vieira TS, Paletta-Silva R, Freitas-Mesquita AL, Meyer-Fernandes JR, Saraiva EM. 3'-nucleotidase/nuclease activity allows Leishmania parasites to escape killing by neutrophil extracellular traps. Infect Immun. (2014) 82:1732–40. doi: 10.1128/IAI.01232-13
62. Guimaraes-Costa AB, Nascimento MT, Froment GS, Soares RP, Morgado FN, Conceicao-Silva F, et al. Leishmania amazonensis promastigotes induce and are killed by neutrophil extracellular traps. Proc Natl Acad Sci USA (2009) 106:6748–53. doi: 10.1073/pnas.0900226106
63. Rochael NC, Guimaraes-Costa AB, Nascimento MT, DeSouza-Vieira TS, Oliveira MP, Garcia e Souza LF, et al. Classical ROS-dependent and early/rapid ROS-independent release of Neutrophil Extracellular Traps triggered by Leishmania parasites. Sci Rep. (2015) 5:18302. doi: 10.1038/srep18302
64. Mandal G, Wyllie S, Singh N, Sundar S, Fairlamb AH, Chatterjee M. Increased levels of thiols protect antimony unresponsive Leishmania donovani field isolates against reactive oxygen species generated by trivalent antimony. Parasitology (2007) 134:1679–87. doi: 10.1017/S0031182007003150
65. Mittal MK, Rai S, Ashutosh Ravinder Gupta S, Sundar S, et al. Characterization of natural antimony resistance in Leishmania donovani isolates. Am J Trop Med Hyg. (2007) 76:681–8.
66. Das M, Saudagar P, Sundar S, Dubey VK. Miltefosine-unresponsive Leishmania donovani has a greater ability than miltefosine-responsive L. donovani to resist reactive oxygen species. FEBS J. (2013) 280:4807–15. doi: 10.1111/febs.12449
67. Mishra J, Singh S. Miltefosine resistance in Leishmania donovani involves suppression of oxidative stress-induced programmed cell death. Exp Parasitol. (2013) 135:397–406. doi: 10.1016/j.exppara.2013.08.004
68. Deep DK, Singh R, Bhandari V, Verma A, Sharma V, Wajid S, et al. Increased miltefosine tolerance in clinical isolates of Leishmania donovani is associated with reduced drug accumulation, increased infectivity and resistance to oxidative stress. PLoS Negl Trop Dis. (2017) 11:e0005641. doi: 10.1371/journal.pntd.0005641
69. Yang H, Biermann MH, Brauner JM, Liu Y, Zhao Y, Herrmann M. New insights into neutrophil extracellular traps: mechanisms of formation and role in inflammation. Front Immunol. (2016) 7:302. doi: 10.3389/fimmu.2016.00302
70. Delgado-Rizo V, Martinez-Guzman MA, Iniguez-Gutierrez L, Garcia-Orozco A, Alvarado-Navarro A, Fafutis-Morris M. Neutrophil extracellular traps and its implications in inflammation: an overview. Front Immunol. (2017) 8:81. doi: 10.3389/fimmu.2017.00081
Keywords: Leishmania, neutrophils, drug resistance, miltefosine, antimony, NETs
Citation: Regli IB, Fernández OL, Martínez-Salazar B, Gómez MA, Saravia NG and Tacchini-Cottier F (2018) Resistance of Leishmania (Viannia) Panamensis to Meglumine Antimoniate or Miltefosine Modulates Neutrophil Effector Functions. Front. Immunol. 9:3040. doi: 10.3389/fimmu.2018.03040
Received: 14 September 2018; Accepted: 10 December 2018;
Published: 21 December 2018.
Edited by:
Wanderley De Souza, Universidade Federal do Rio de Janeiro, BrazilReviewed by:
Arup Sarkar, Trident Academy of Creative Technology, IndiaHerbert Leonel de Matos Guedes, Universidade Federal do Rio de Janeiro, Brazil
Copyright © 2018 Regli, Fernández, Martínez-Salazar, Gómez, Saravia and Tacchini-Cottier. This is an open-access article distributed under the terms of the Creative Commons Attribution License (CC BY). The use, distribution or reproduction in other forums is permitted, provided the original author(s) and the copyright owner(s) are credited and that the original publication in this journal is cited, in accordance with accepted academic practice. No use, distribution or reproduction is permitted which does not comply with these terms.
*Correspondence: Fabienne Tacchini-Cottier, fabienne.tacchini-cottier@unil.ch