- 1Instituto de Investigação e Inovação em Saúde, Universidade do Porto, Porto, Portugal
- 2Instituto de Biologia Molecular e Celular, Porto, Portugal
- 3Instituto de Ciências Biomédicas Abel Salazar and Faculdade de Ciências, Universidade do Porto, Porto, Portugal
Following T cell receptor triggering, T cell activation is initiated and amplified by the assembly at the TCR/CD3 macrocomplex of a multitude of stimulatory enzymes that activate several signaling cascades. The potency of signaling is, however, modulated by various inhibitory components already at the onset of activation, long before co-inhibitory immune checkpoints are expressed to help terminating the response. CD5 and CD6 are surface glycoproteins of T cells that have determinant roles in thymocyte development, T cell activation and immune responses. They belong to the superfamily of scavenger receptor cysteine-rich (SRCR) glycoproteins but whereas the inhibitory role of CD5 has been established for long, there is still controversy on whether CD6 may have similar or antagonistic functions on T cell signaling. Analysis of the structure and molecular associations of CD5 and CD6 indicates that these molecules assemble at the cytoplasmic tail a considerable number of signaling effectors that can putatively transduce diverse types of intracellular signals. Biochemical studies have concluded that both receptors can antagonize the flow of TCR-mediated signaling; however, the impact that CD5 and CD6 have on T cell development and T cell-mediated immune responses may be different. Here we analyze the signaling function of CD6, the common and also the different properties it exhibits comparing with CD5, and interpret the functional effects displayed by CD6 in recent animal models.
Introduction
Antigen-specific T cell activation is triggered by the T cell receptor (TCR) recognition of a cognate peptide presented by antigen presenting cells (APC), but it is overall controlled by a plethora of other cell surface receptors that either increase or repress the strength of the signals, the combination of which determines the outcome of T cell-mediated responses. Most of the receptors do not contain intrinsic enzymatic activities so their function relies on the establishment of interactions with signaling effectors, and also on an appropriate localization where they can exert their role, determined extracellularly by the binding to specific ligands expressed on the APC and intracellularly through connecting with the cytoskeleton.
Inhibitory co-receptors, such as the immune checkpoints Programmed cell death protein 1 (PD-1), Cytotoxic T-lymphocyte-associated protein 4 (CTLA4), T cell immunoreceptor with Ig and ITIM domains (TIGIT), Lymphocyte-activation gene 3 (LAG-3), and B- and T-lymphocyte attenuator (BTLA) are crucial to halt the progression or to terminate cell activation once they become expressed, given that they are strongly induced upon activation (1–5). They exert their inhibitory effect intracellularly through very limited and defined interactions utilizing ITIM, ITSF, or other sequences of their cytoplasmic tails that are bound by inhibitory enzymes, typically serine/threonine or tyrosine phosphatases (6–10). Moreover, some of these inhibitory co-receptors also interfere with co-stimulatory receptors through extracellular competition for the same ligands (11–14).
A different class of inhibitory receptors that can be active immediately following the triggering of the TCR and thus modulate T cell signaling at the onset of activation is exemplified by CD5: constitutively expressed on nearly all T cell subsets, including naïve and non-activated cells (15, 16), and loosely interacting with the TCR/CD3 complex (17), CD5 is able to modulate responses concomitantly with TCR triggering (18, 19). The expression of CD5 can increase significantly upon thymocyte and mature T cell activation such that the potency of the inhibition is proportionally adjusted in accordance to the affinity of TCR recognition of peptide/MHC and TCR-dependent signaling intensity (20, 21). This indicates that the variable levels of CD5 expression are important to counteract the strength of TCR signaling (22).
CD6 shares with CD5 many genetic, structural, and functional characteristics, among them the capacity to interact with the TCR/CD3 complex, to be tyrosine-phosphorylated and activated upon TCR triggering, and slightly increases its surface expression upon T cell activation (23–26). However, some differences between the kinetics of expression of CD5 and CD6 during thymocyte ontogeny and selection and T cell subset polarization as well as apparent different requirements for ligand binding suggest that CD5 and CD6 may have non-coincident roles during thymocyte development, T cell activation and immune responses. Nevertheless, in cellular systems it was also shown that the strength of activation correlates inversely with the expression of CD6 (27). Therefore, similarly to CD5 and distinctive from the immune checkpoints that can shut down activation at later stages, CD6 may be a rheostat-type regulator of activation, fine-tuning the response depending on the strength of the antigenic challenge.
CD6 Is a Hub for the Assembly of Stimulatory and Inhibitory Effectors
At the time of the cloning of their genes and initial functional characterization of the proteins, CD5 and CD6 were regarded as co-stimulatory receptors that amplified TCR-dependent activation (28–31). In the case of CD5, the function of the molecule was soon after revised following the development of mice with a disrupted Cd5 gene (18). The lack of similar definitive models addressing the role of CD6 in vivo until very recently delayed significantly the progress on CD6 research, and caused that the knowledge on the function of CD6 is still lagging considerably behind.
There are many common aspects in the biochemical behavior of CD5 and CD6 and in fact they can interact with each other in non-activated T cells (32, 33). Upon antigen recognition and T cell-APC conjugation, both receptors localize at the center of the immunological synapse (33). In contact with the TCR/CD3 signaling machinery, CD5 and CD6 are very rapidly phosphorylated on tyrosine residues (19, 24), presumably by the SRC-family kinase LCK, with the concomitant docking of intracellular mediators that contain SH2 domains, semi-autonomous conserved structural domains that bind to phosphorylated tyrosine residues. The net contribution of either CD5 and CD6 appears to be inhibitory, given that cells that lack any of the receptors are significantly more responsive to antigenic or mitogenic stimulation (22, 34). However, the number and diversity of effectors that associate with CD5 and/or CD6, depending or not on tyrosine phosphorylation, would not give an obvious idea of the repressive potential of the receptors, given that many interacting partners are effectively protein tyrosine kinases that are normally associated with signaling progression. These include LCK, FYN, ZAP70, and additionally in the case of CD6, the TEC-family kinase ITK (32, 35–37).
Perhaps this aggregation of kinases at the cytoplasmic tail of CD5 and CD6 explains the behavior observed in their initial characterization when either receptor, when triggered together with the TCR/CD3 complex with monoclonal antibodies, amplified the activation signals originated at the TCR complex. Notwithstanding this possibly artifactual contribution to activation determined by the in vitro experimental design, it is also possible that the kinases may actually contribute to positive signaling via CD5 and CD6 in very defined contexts, thus explaining the dual function that has been many times attributed to CD6 and occasionally to CD5.
CD5 contains four tyrosine residues on its cytoplasmic domain, that when phosphorylated constitute putative sites for the docking of SH2 domain-containing cytoplasmic molecules. Tyrosine 402 is close or even buried within the plasma membrane and therefore it is disputable whether it can actually be phosphorylated. Nonetheless, the remaining tyrosine residues of CD5, when phosphorylated, have been for a long time shown to bind to the tyrosine kinase LCK (35), the tyrosine phosphatase SHP1 (38, 39), the ubiquitin ligases CBL and CBLB (40, 41), the GTPase activating protein for RAS (RASGAP) (40) and the lipid kinase PI3K (42), while the associations of CD5 with the protein kinases FYN and ZAP70 have not been shown to be direct (Figure 1A).
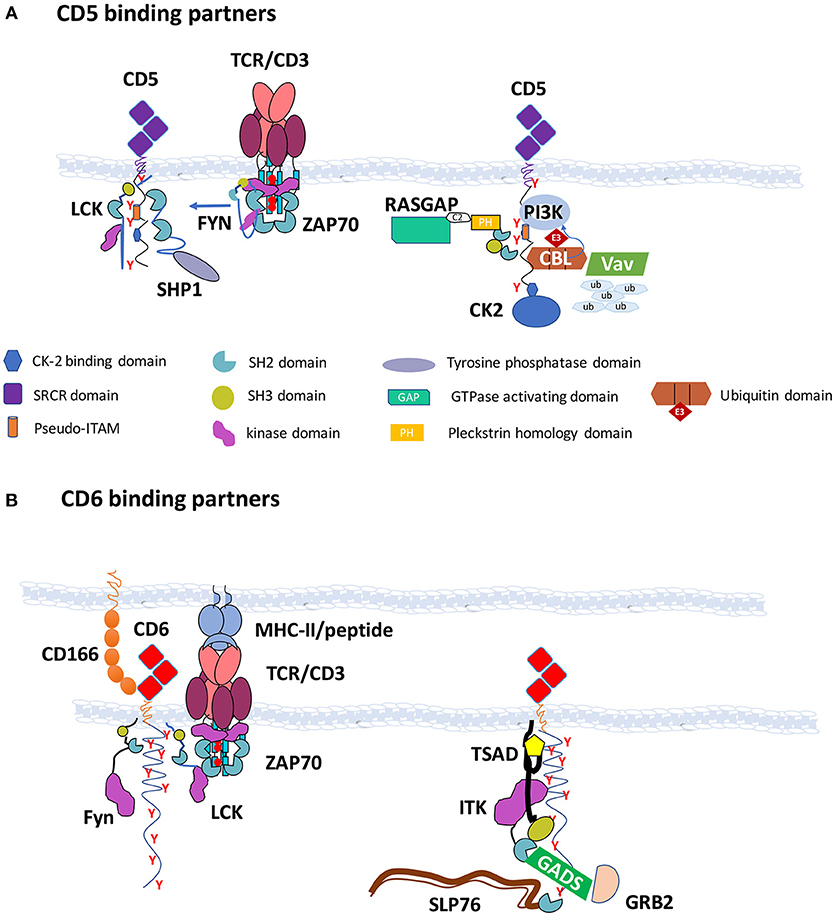
Figure 1. CD5 and CD6 are hubs for the assembly of effector enzymes and adaptors—(A) CD5 binding partners: CD5 contains in its cytoplasmic tail four tyrosine residues, of which three (Y453, Y465, and Y487) are believed to be phosphorylated upon TCR triggering and can bind the SH2 domains of LCK, RASGAP, CBL, CBLB, SHP1, and PI3K. Recruitment of CBL to the C-terminal region of CD5 is important for the ubiquitylation and degradation of several substrates following TCR engagement, including VAV. CK2 is also able to bind to the cytoplasmic tail of CD5 through other mechanisms. The interaction with FYN is also not dependent on tyrosine phosphorylation. CSK associates with the CD5 signalosome possibly through the cooperation with PAG, CBL, or CBLB. CD5 is represented in duplicate to accommodate all binding partners; (B) CD6 binding partners: CD6 contains in its cytoplasmic tail nine tyrosine residues that when phosphorylated can dock the SH2 domains of SLP76, TSAD, GADS, GRB2, and SHP1. The interactions with LCK, FYN, ZAP70, and ITK were not shown to be dependent on SH2 domain binding to phosphotyrosine residues, but ITK may be recruited through its association with TSAD. CD6 binds through the C-terminal sequence to the PDZ domains of syntenin. The CD6 signalosome is depicted in the right. Structures are not drawn to scale.
CD6 possesses possibly the longest cytoplasmic tail of the known receptors of leukocytes, containing amongst other signaling motifs nine tyrosine residues. However, fewer than expected interactions of CD6 with SH2 domain-containing effectors have been reported to date, possibly because research on CD6 function has been performed less systematically than that on CD5 (Figure 1B). Moreover, most of the disclosed interactors of CD6 seem to be more related to activation pathways rather than to repression mechanisms, including the tyrosine kinases LCK, FYN, ZAP70, and ITK that were shown to be associated with CD6 but not confirmed to be dependent on phosphotyrosine-SH2 domain binding. Additionally, the adaptors SLP76 (27), TSAD (43), GADS, and GRB2 (44), that have established roles in T cell activation have been shown to bind to the two most carboxyl-terminal phosphotyrosines of CD6. CD6 also binds to the scaffolding protein syntenin-1, but the interaction is likely mediated by the tandemly arranged PDZ domains of syntenin-1 (45).
Interestingly, some of the papers that reported the phosphoprotein-dependent molecular interactions of CD6 described the coincident finding that the same molecules could also dock onto the phosphotyrosine sites of Linker for activation of T cells (LAT), a membrane-bound adaptor of the main axis of the TCR-mediated pathway. In an unbiased in vivo proteomics screening, Roncagalli et al. described the LAT-independent association of SLP76 to CD6 (46), while Hem et al. showed that TSAD bound to both LAT and CD6 (43). Given that additionally GRB2 and GADS are well-established binders of LAT (47), it emerges that CD6 displays some characteristics of membrane-bound adaptors, such as LAT and Phosphoprotein associated with glycosphingolipid-enriched microdomains 1 (PAG) in that it contains multiple tyrosine residues that once phosphorylated can couple to a diverse set of signaling effectors, possibly feeding onto various, convergent or divergent, signaling pathways (48).
Therefore, and much like LAT and PAG, CD6 seems to constitute a signalosome that assembles many different enzymes and adaptors that can impact on signal propagation in different pathways and potentially with divergent outcomes. Lat knockout mice, however, show a very different behavior than those deficient of CD6 (described later in this paper), displaying a block in the development of thymocytes at the double negative stage and a complete absence of mature T cells (49). This established LAT as a crucial adaptor for T cell signaling leading to lymphocyte differentiation and also of T cell activation. On the other hand, mice with a disrupted Pag gene, much similarly to Cd6-deficient but also to Cd5-deficient animals, have no overall differences in total numbers of T cells than wild-type mice; and effector T cells, although not naïve, are more prone to activation upon TCR targeting (50). PAG is essential for the phosphotyrosine-dependent docking of the protein tyrosine kinase CSK, a major inhibitory enzyme of T cell activation that phosphorylates the inhibitory carboxyl-terminal tyrosine residues of LCK and FYN, inactivating these kinases (51, 52). Interestingly, CD5 seems to be a relevant alternative docking receptor for CSK, and in fact PAG and CD5 may cooperate in the inhibition of FYN (36, 41). Overall, it appears that while LAT is a hub for the assembling of positive effectors, CD5 as well as PAG can be viewed as inhibitory signalosomes. Whether CD6 is an activating, inhibitory, or multi-purpose scaffolding transmembrane receptor is still under debate.
Given that CD6, besides being structurally very similar to CD5, shares with it many features, such as profile of expression and functional roles in the biochemical repression of T cell activation, it would be expected or at least plausible that, similarly to CD5, it could couple to analogous signaling inhibitory partners. A recent report has in fact described the interaction of CD6 with the protein phosphatase SHP1 (26), constituting this the first solid biochemical evidence that CD6 can couple to inhibitory signaling.
Can Ligand Binding and Signaling Inhibition be Uncoupled Events?
Unlike PAG or LAT, CD6 contains structured ectodomains that are suitable to establish interactions with extracellular ligands. CD6 binds to CD166, widely expressed in many cell types and tissues (53, 54). and recently CD318 was identified as an alternative ligand in cells derived from human thymus, skin, synovium, and cartilage (55, 56). However, there is no absolute requirement for CD6 to bind to ligands to be able to exert its inhibitory function (34). This is a characteristic common to CD5 (57). Therefore, these two receptors can be general attenuators of TCR-mediated signaling independent of any mechanical effects of ligand binding or of any particular membrane localization. So, if there can be a functional uncoupling between these two features of the molecules, what may be the role of the ectodomains and the consequences of binding to ligands?
During thymocyte development, the expression of CD6 increases steadily from double negative (DN) to double positive (DP) and to single CD4+ or CD8+ thymocytes, decreasing then slightly in the negatively selected single CD4+ or CD8+ thymocytes just before thymic emigration, and to mature T cells (58). The increasing expression of CD6 favors the interaction with CD166, highly expressed on thymic epithelium, possibly providing anti-apoptotic signals and also increasing the adhesion of thymocytes to thymic epithelial cells. However, this signal tuning-related variation of expression is a general characteristic and not a differentiation feature, i.e., thymocytes are exposed to APCs that all express the same putative CD6 ligands and thus there should be no distinctive outcome in selection or subset polarization between different thymocytes based solely on whether or not CD6 binds to its ligand.
The scenario can be remarkably different regarding the activation of mature T cells, though. The interaction between CD6 and CD166 is one of the strongest between cell surface adhesion molecules, with a dissociation constant (KD) of 0.4–1.0 μM measured by surface plasmon resonance (59), and contributing to binding between T cells and APC with forces equivalent to those of integrins, as quantified by atomic force microscopy (60). Integrating this information with the inhibitory contribution toward signaling, it emerges that CD6 can possibly have a dual role, the first of which is to promote cellular adhesion, facilitating the TCR scanning of specific peptides. Upon antigen recognition and the formation of immunological synapses, CD6 can then adjust the strength of T cell activation through the attenuation of the signaling cascades. These features are also generic considering T cell activation as a whole, given that most APCs do express the ligand CD166. But the fact is that in different contexts with distinct APCs, possibly expressing varied levels of CD166 but also armed with different sets of costimulatory or co-inhibitory ligands for the many cell surface receptors of T cells, CD6 may impact differently on the signaling pathways and can eventually influence on the polarization of T cell subsets and responses, namely in the development of Th1 and Th17 sub-populations (61).
As for CD5, no APC-expressed ligand has been demonstrated so far; however, it was recently shown that it can serve as an alternative receptor for IL-6, leading to the activation of the transcription factor STAT3 (62). Although this observation was made in B1a cells, it nevertheless opens the perspective of IL-6 being able to promote Th17 responses when binding to T cell-expressed CD5.
The Modulatory Role of CD6 During Thymocyte Development
Two recent reports on independent Cd6 knockout models have finally confirmed that at the cellular level, the net contribution of CD6 to signaling is generally inhibitory (24, 25). Isolated CD4+ and CD8+ T cells from the mutant mice displayed significantly augmented activation upon anti-CD3 triggering than cells isolated from wild-type mice (24, 25), highlighting the inhibitory role of CD6 in T cell activation. It appears, however, that the strength of inhibition is milder than that of CD5.
The generation of Cd6 knockout mouse models has been helpful in understanding the role of the protein during thymocyte selection. Although the frequency and total numbers of most cell populations in the periphery are identical comparing wild-type and CD6−/− mice, in the thymus there seems to be a partial impairment in the transition from double-positive to single positive thymocytes (63). Namely, there is an increase in the percentage of DP thymocytes undergoing selection, which is accompanied by a decrease of CD4+ or CD8+ SP thymocytes that complete selection. Besides, the percentage of CD4+ SP thymocytes is reduced in these animals. The percentage of CD8+ SP cells is unaltered but a deeper look into that subpopulation revealed that, in Cd6 knockout mice, the percentage of CD8+ immature cells was increased while that of CD8+ mature cells was decreased (63). In summary, CD6 is able to modulate signaling during T cell development as the lack of the molecule changes the threshold for negative selection in the thymus resulting in a reduced number of T cells that fully mature (Figure 2).
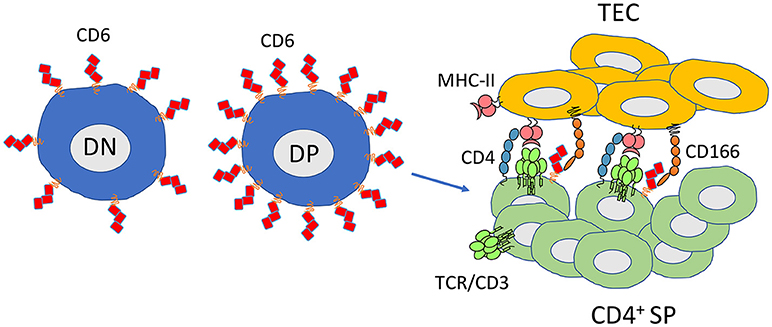
Figure 2. CD6 favors thymocyte differentiation and maturation and is required for selection of thymocytes with high-avidity TCRs. CD6 is expressed at all stages of thymocyte development. Its expression is increased at the double positive stage in cells that are assigned to maturation (CD69high). CD6 has an important role limiting the threshold for negative selection. The CD6-CD166 interaction promotes higher affinity TCR-MHC-II/peptide interactions contributing to CD4+ selection. CD6−/− mice have an increased frequency of DP cells undergoing selection. Conversely, CD4+ SP and CD8+ SP have lower numbers or are less mature, respectively. DN, double negative thymocytes; DP, double positive thymocytes; CD4+ SP, single positive CD4 thymocytes; TEC, thymic epithelial cells.
Similar studies had been conducted to assess the role of CD5 expression in development and how it affects the generation of either CD8+ or CD4+ T cell populations (18, 64). Despite the fact that the overall effect of CD5 expression in development was initially disguised by the large repertoire of expressed TCRs (65), the use of TCR-transgenic mice allowed to understand that CD5 acts as a negative regulator during T cell maturation (18, 64). In fact, a subsequent study encompassing different TCR-transgenic animal models clarified that the effect of CD5 is related to its levels of expression (22). In T cells with high-affinity TCRs (and consequent high expression of CD5), lack of CD5 markedly decreases positive selection, while increasing negative selection. In low-affinity TCR-expressing T cells, however, the loss of CD5 expression did not result in such significant changes (22).
CD6 is also able to impact on the efficiency of Tregs, a particular subset of T cells responsible for suppressing immune responses by inducing antigen tolerance. The ability of Tregs to downregulate both effector and helper T cells in response to self-antigens renders them important players in preventing autoimmune diseases. Tregs isolated from the spleen of Cd6 knockout mice were shown to be less efficient in suppressing the proliferation of conventional T cells than those extracted from wild-type mice (63). Taking into consideration the many features common to both CD6 and CD5, this observation was somewhat surprising, as the opposite phenotype had been seen for Cd5 knockout mice (66). Specifically, it was observed that Tregs from Cd5 knockout mice were more efficient in their suppressive activity than cells from wild-type mice, consistent with CD5 being a negative regulator (66). The explanation for this discrepancy may be found in the global view of the system: CD4+ T cells from the spleen of Cd5 knockout mice were described to express slightly more CD6; on the other hand, splenic CD4+ T cells from Cd6 knockout mice tend to express less CD5 (63). Therefore, Cd5 knockout mice ultimately display Tregs that are more able to repress immune responses whereas Cd6 knockout mice, expressing less CD5 but also less CD6, are characterized by less efficient Tregs. This translates into less suppression of the immune system. In summary, when CD6 expression is increased directly or indirectly, the overall result seems to be a system more equipped to tone down immune responses.
Ligand Binding in the Promotion of T Cell Migration vs. Subset Polarization
Mice with disrupted Cd6 genes display differing responses in the two main inflammatory disease settings investigated so far. In the collagen-induced arthritis (CIA) model, Cd6 knockout mice were characterized by earlier disease onset and increased clinical score as well as worsened hallmarks for the disease, namely IL-6 and TNF expression in the joints (63), whereas in a model of multiple sclerosis, experimental autoimmune encephalomyelitis (EAE), the absence of CD6 confers resistance to the demyelinating disorder (61). Although the mice strains were different and not the conventional usually used in the two different disease models, the disparate responses could again point to dual alternative roles of CD6 in T cell activation leading to divergent immune responses. However, when isolated and responding in vitro to antigenic or antibody-induced activation, CD6-negative cells were more responsive in both cases (61, 63). This observation again suggests that CD6 may have other roles than just its participation in signal transduction mechanisms.
Whereas, in the CIA model the augmented reactivity of CD6−/− T cells is consistent with a cellular signaling inhibitory function for CD6, or an inhibitory CD6-mediated T cell response, in EAE the absence of CD6 was suggested, rather, to impair T cell migration through brain microvascular endothelial cells resulting in a lower infiltrate in the spinal cord of, among others, the autoreactive T cells. Although the experiments performed did not address leukocyte transmigration in vivo but actually used brain microvascular endothelial cells isolated from wild-type and knockout mice (61), these observations are concordant with a recent report where the re-engineered additional expression in CD6 molecules of the domain (d3) that interacts with CD166 significantly promotes T cell migration into the brain in a brain cancer model, where cancer endothelium upregulates CD166 expression (67). On the whole, a plausible explanation for the divergent impact of the lack of CD6 in the two models is that in CIA, CD6neg cells are more autoreactive and inflict tissue damage whereas in EAE the lower T cell infiltration into the brain is the dominant effect.
The hypothesis that the lower EAE scores in the absence of CD6 are due to less transmigration is, however, in apparent contradiction with the interpretation that is given for the CD6-dependent corresponding human pathology: CD6 is a susceptibility gene for multiple sclerosis (68), and individuals carrying a disease-related polymorphism at the locus rs17828933 within the first intron have increased levels of CD6Δd3 (69), a naturally occurring CD6 isoform that lacks the CD166-binding domain (70). Thus, in both mouse and human studies, the defective T cells are in disadvantage to cross the blood-brain barrier; but whereas in the mouse model the lack of cell infiltration is protective, in the human setting, paradoxically, the conditions are set to induce or aggravate the illness.
One main difference is that in the mouse the whole molecule is missing whereas in humans the full signaling potential is present. Furthermore, cells carrying the CD6Δd3 isoform are more reactive upon mAb challenge than cells expressing wild-type CD6. How can all these features be reconciled to explain the progression and severity of the disease? Unless we consider that the mouse and human studies are too different to be comparable, there is at present no simple answer to that question if only the signaling aspects of CD6 are taken into account. Therefore, a possible function of CD6 that should be considered is in fact its impact on the polarization of different T cell subsets in different disease conditions.
Therapeutic CD6 mAbs
Bughani et al. have developed an anti-mouse CD6 mAb, mCD6D1, that recognizes the membrane distal domain of CD6 (d1), to ameliorate the incidence of EAE in C57BL/6 mice (26), while Li et al. have used a mouse anti-human CD6-d1 mAb, UMCD6, to reverse EAE progression in DBA/1 humanized mice (61). Itolizumab is a mouse mAb that also binds to CD6-d1 (71) and that has been found to be very efficient in treating autoimmune pathologies (72). Clinical trials for Itolizumab have been conducted to treat rheumatoid arthritis and psoriasis, yielding very encouraging results (73, 74). This antibody has already received approval from the Drugs Controller General of India as treatment for chronic plaque psoriasis in 2013, with treated patients presenting less proliferative T cells and decreased levels of pro-inflammatory cytokines in the serum (75). Despite the positive outcomes that are emerging from the use of this antibody, very little is known about its mechanism of action and how exactly it impacts on T cell signaling (72).
UMCD6 and Itolizumab recognize overlapping epitopes and can partly inhibit the binding of soluble CD166 to T cell-expressed CD6, although substantially less than anti-CD6-d3 (76). The structure of the CD6 extracellular region has only recently been solved (77), and it was speculated that antibody binding to CD6-d1 could perhaps hinder the ability of CD6 to interact with CD166 (26). However, in different experimental settings the effect of blocking of the direct interaction between T cell expressed-CD6 and APC-expressed CD166 by anti-CD6d1 mAbs, or even the reduction of T cell-APC conjugates, has produced contradictory results (70, 71, 78). Although it is possible that the presence of massive amounts of antibodies decorating the surface of T cells may actually reduce the number of T cell-APC conjugates, another plausible explanation is that the action of the reagents may have a direct effect on CD6-mediated signaling, or alternatively on CD6-mediated T cell polarization. The first case has been extensively documented in vitro and delivers very disparate results depending on the mAb clone used and conditions of cell culture and activation, ranging from significant activation to marked inhibition (79). As for the second, there is still insufficient documentation of in vivo studies to allow for any conclusions to be withdrawn for the time being; nevertheless, Itolizumab has proven efficacy to treat human diseases that are characterized by having Th17 polarization and concordantly, in human PBMC cultured in Th17 polarizing conditions, addition of Itolizumab seems to decrease the differentiation of CD4+ T cells into the Th17 sub-set and decrease the production of IL-17 (26).
Concluding Remarks
Although perhaps counterintuitively at a first glance, signal inhibition relies heavily on intracellular tyrosine phosphorylation. Moreover, the phosphorylation of activation and inhibitory motifs can be catalyzed by exactly the same kinases and also be coincidental in time. Therefore, it cannot be unexpected that T cell-expressed inhibitory receptors functionally and physically associate with kinases that are known to be crucial to T cell activation. CD6 associates with LCK, FYN, ZAP70, and ITK, protein tyrosine kinases that are regarded as components of signaling progression, and yet, this complex molecule has been shown to bestow T cells with a strong inhibitory potential both in vitro as well as in ex vivo systems (34, 61, 63).
However, a straightforward categorization of CD6 as an inhibitor, or alternatively, as a co-stimulatory receptor, does not suffice to explain the apparent diversity of functions that the molecule displays in many different situations. CD6 seems to exhibit many features analogous to membrane-bound intracellular adaptors, such as LAT and PAG, as it has multiple tyrosine residues on its cytoplasmic domain that once phosphorylated can couple to a range of different enzymes and adaptors, possibly feeding into multiple parallel signaling pathways. Perhaps this can explain the antagonistic roles attributed to the molecule in different experimental setups. On the other hand, the fact that CD6 establishes robust extracellular interactions with APC-expressed ligands strongly favors the adhesion between T cells and APCs and unequivocally promotes T cell activation. Whether binding to CD166 can, alternatively in different situations, promote T cell activation or repression, or impact on T cell subset polarization requires further investigation. CD6 is being recognized as an important target for therapy against several autoimmune diseases and the use of therapeutic CD6 mAbs is steadily increasing. A major challenge facing ahead is to understand how these reagents can regulate CD6 function to be able to devise the most appropriate treatment for human disease.
Author Contributions
CMG, SNH, RFS, and AMC wrote the manuscript. CMG and AMC designed the figures.
Conflict of Interest Statement
The authors declare that the research was conducted in the absence of any commercial or financial relationships that could be construed as a potential conflict of interest.
Acknowledgments
This work was financed by FEDER-Fundo Europeu de Desenvolvimento Regional funds through the COMPETE 2020-Operacional Programme for Competitiveness and Internationalization (POCI), Portugal 2020, and by Portuguese funds through FCT-Fundação para a Ciência e a Tecnologia/Ministério da Ciência, Tecnologia e Ensino Superior in the framework of the project POCI-01-0145-FEDER-032296 (PTDC/MED-IMU/32296/2017). Additional funds, including funding for CMG, were from the project Norte-01-0145-FEDER-000012-Structured program on bioengineered therapies for infectious diseases and tissue regeneration, supported by Norte Portugal Regional Operational Programme (NORTE 2020), under the PORTUGAL 2020 Partnership Agreement, through the European Regional Development Fund (FEDER). SNH and RFS are recipients of Ph.D. studentships from FCT, references SFRH/BD/133312/2017 and SFRH/BD/110691/2015, respectively.
References
1. Agata Y, Kawasaki A, Nishimura H, Ishida Y, Tsubata T, Yagita H, et al. Expression of the PD-1 antigen on the surface of stimulated mouse T and B lymphocytes. Int Immunol. (1996) 8:765–72. doi: 10.1093/intimm/8.5.765
2. Metzler B, Burkhart C, Wraith DC. Phenotypic analysis of CTLA-4 and CD28 expression during transient peptide-induced T cell activation in vivo. Int Immunol. (1999) 11:667–75. doi: 10.1093/intimm/11.5.667
3. Yu X, Harden K, Gonzalez LC, Francesco M, Chiang E, Irving B, et al. The surface protein TIGIT suppresses T cell activation by promoting the generation of mature immunoregulatory dendritic cells. Nat Immunol. (2009) 10:48–57. doi: 10.1038/ni.1674
4. Triebel F, Jitsukawa S, Baixeras E, Roman-Roman S, Genevee C, Viegas-Pequignot E, et al. LAG-3, a novel lymphocyte activation gene closely related to CD4. J Exp Med. (1990) 171:1393–405. doi: 10.1084/jem.171.5.1393
5. Han P, Goularte OD, Rufner K, Wilkinson B, Kaye J. An inhibitory Ig superfamily protein expressed by lymphocytes and APCs is also an early marker of thymocyte positive selection. J Immunol. (2004) 172:5931–9. doi: 10.4049/jimmunol.172.10.5931
6. Yokosuka T, Takamatsu M, Kobayashi-Imanishi W, Hashimoto-Tane A, Azuma M, Saito T. Programmed cell death 1 forms negative costimulatory microclusters that directly inhibit T cell receptor signaling by recruiting phosphatase SHP2. J Exp Med. (2012) 209:1201–17. doi: 10.1084/jem.20112741
7. Chuang E, Fisher TS, Morgan RW, Robbins MD, Duerr JM, Vander Heiden MG, et al. The CD28 and CTLA-4 receptors associate with the serine/threonine phosphatase PP2A. Immunity (2000) 13:313–22. doi: 10.1016/S1074-7613(00)00031-5
8. Liu S, Zhang H, Li M, Hu D, Li C, Ge B, et al. Recruitment of Grb2 and SHIP1 by the ITT-like motif of TIGIT suppresses granule polarization and cytotoxicity of NK cells. Cell Death Differ. (2013) 20:456–64. doi: 10.1038/cdd.2012.141
9. Workman CJ, Dugger KJ, Vignali DA. Cutting edge: molecular analysis of the negative regulatory function of lymphocyte activation gene-3. J Immunol. (2002) 169:5392–5. doi: 10.4049/jimmunol.169.10.5392
10. Watanabe N, Gavrieli M, Sedy JR, Yang J, Fallarino F, Loftin SK, et al. BTLA is a lymphocyte inhibitory receptor with similarities to CTLA-4 and PD-1. Nat Immunol. (2003) 4:670–9. doi: 10.1038/ni944
11. Linsley PS, Brady W, Urnes M, Grosmaire LS, Damle NK, Ledbetter JA. CTLA-4 is a second receptor for the B cell activation antigen B7. J Exp Med. (1991) 174:561–9. doi: 10.1084/jem.174.3.561
12. Azuma M, Ito D, Yagita H, Okumura K, Phillips JH, Lanier LL, et al. B70 antigen is a second ligand for CTLA-4 and CD28. Nature (1993) 366:76–9. doi: 10.1038/366076a0
13. Lozano E, Dominguez-Villar M, Kuchroo V, Hafler DA. The TIGIT/CD226 axis regulates human T cell function. J Immunol. (2012) 188:3869–75. doi: 10.4049/jimmunol.1103627
14. Liang B, Workman C, Lee J, Chew C, Dale BM, Colonna L, et al. Regulatory T cells inhibit dendritic cells by lymphocyte activation gene-3 engagement of MHC class II. J Immunol. (2008) 180:5916–26. doi: 10.4049/jimmunol.180.9.5916
15. Huang HJ, Jones NH, Strominger JL, Herzenberg LA. Molecular cloning of Ly-1, a membrane glycoprotein of mouse T lymphocytes and a subset of B cells: molecular homology to its human counterpart Leu-1/T1 (CD5). Proc Natl Acad Sci USA. (1987) 84:204–8. doi: 10.1073/pnas.84.1.204
16. Hayakawa K, Hardy RR, Parks DR, Herzenberg LA. The “Ly-1 B” cell subpopulation in normal immunodefective, and autoimmune mice. J Exp Med. (1983) 157:202–18. doi: 10.1084/jem.157.1.202
17. Beyers AD, Spruyt LL, Williams AF. Molecular associations between the T-lymphocyte antigen receptor complex and the surface antigens CD2, CD4, or CD8 and CD5. Proc Natl Acad Sci USA. (1992) 89:2945–9. doi: 10.1073/pnas.89.7.2945
18. Tarakhovsky A, Kanner SB, Hombach J, Ledbetter JA, Müller W, Killeen N, et al. A role for CD5 in TCR-mediated signal transduction and thymocyte selection. Science (1995) 269:535–7.
19. Burgess KE, Yamamoto M, Prasad KV, Rudd CE. CD5 acts as a tyrosine kinase substrate within a receptor complex comprising T-cell receptor zeta chain/CD3 and protein-tyrosine kinases p56lck and p59fyn. Proc Natl Acad Sci USA. (1992) 89:9311–5. doi: 10.1073/pnas.89.19.9311
20. Groves T, Parsons M, Miyamoto NG, Guidos CJ. TCR engagement of CD4+CD8+ thymocytes in vitro induces early aspects of positive selection, but not apoptosis. J Immunol. (1997) 158:65–75.
21. Azzam HS, Grinberg A, Lui K, Shen H, Shores EW, Love PE. CD5 expression is developmentally regulated by T cell receptor (TCR) signals and TCR avidity. J Exp Med. (1998) 188:2301–11. doi: 10.1084/jem.188.12.2301
22. Azzam HS, DeJarnette JB, Huang K, Emmons R, Park CS, Sommers CL, et al. Fine tuning of TCR signaling by CD5. J Immunol. (2001) 166:5464–72. doi: 10.4049/jimmunol.166.9.5464
23. Gimferrer I, Calvo M, Mittelbrunn M, Farnós M, Sarrias MR, Enrich C, et al. Relevance of CD6-mediated interactions in T cell activation and proliferation. J Immunol. (2004) 173:2262–70. doi: 10.4049/jimmunol.173.4.2262
24. Wee S, Schieven GL, Kirihara JM, Tsu TT, Ledbetter JA, Aruffo A. Tyrosine phosphorylation of CD6 by stimulation of CD3: augmentation by the CD4 and CD2 coreceptors. J Exp Med. (1993) 177:219–23. doi: 10.1084/jem.177.1.219
25. da Gloria VG, de Araujo MM, Santos AM, Leal R, de Almeida SF, Carmo AM, et al. T Cell activation regulates CD6 alternative splicing by transcription dynamics and SRSF1. J Immunol. (2014) 193:391–9. doi: 10.4049/jimmunol.1400038
26. Bughani U, Saha A, Kuriakose A, Nair R, Sadashivarao RB, Venkataraman R, et al. T cell activation and differentiation is modulated by a CD6 domain 1 antibody Itolizumab. PLoS ONE (2017) 12:e0180088. doi: 10.1371/journal.pone.0180088
27. Hassan NJ, Simmonds SJ, Clarkson NG, Hanrahan S, Puklavec MJ, Bomb M, et al. CD6 regulates T-cell responses through activation-dependent recruitment of the positive regulator SLP-76. Mol Cell Biol. (2006) 26:6727–38. doi: 10.1128/MCB.00688-06
28. Ceuppens JL, Baroja ML. Monoclonal antibodies to the CD5 antigen can provide the necessary second signal for activation of isolated resting T cells by solid-phase-bound OKT3. J Immunol. (1986) 137:1816–21.
29. June CH, Rabinovitch PS, Ledbetter JA. CD5 antibodies increase intracellular ionized calcium concentration in T cells. J Immunol. (1987) 138:2782–92.
30. Gangemi RM, Swack JA, Gaviria DM, Romain PL. Anti-T12, an anti-CD6 monoclonal antibody, can activate human T lymphocytes. J Immunol. (1989) 143:2439–47.
31. Osorio LM, Garcia CA, Jondal M, Chow SC. The anti-CD6 mAb, IOR-T1, defined a new epitope on the human CD6 molecule that induces greater responsiveness in T cell receptor/CD3-mediated T cell proliferation. Cell Immunol. (1994) 154:123–33. doi: 10.1006/cimm.1994.1062
32. Castro M, Nunes R, Oliveira M, Tavares P, Simoes C, Parnes J, et al. OX52 is the rat homologue of CD6: evidence for an effector function in the regulation of CD5 phosphorylation. J Leuk Biol. (2003) 73:183–90. doi: 10.1189/jlb.0902437
33. Gimferrer I, Farnós M, Calvo M, Mittelbrunn M, Enrich C, Sánchez-Madrid F, et al. The accessory molecules CD5 and CD6 associate on the membrane of lymphoid T cells. J Biol Chem. (2003) 278:8564–71. doi: 10.1074/jbc.M209591200
34. Oliveira MI, Gonçalves CM, Pinto M, Fabre S, Santos AM, Lee SF, et al. CD6 attenuates early and late signaling events, setting thresholds for T-cell activation. Eur J Immunol. (2012) 42:195–205. doi: 10.1002/eji.201040528
35. Raab M, Yamamoto M, Rudd CE. The T-cell antigen CD5 acts as a receptor and substrate for the protein-tyrosine kinase p56lck. Mol Cell Biol. (1994) 14:2862–70. doi: 10.1128/MCB.14.5.2862
36. Bamberger M, Santos AM, Gonçalves CM, Oliveira MI, James JR, Moreira A, et al. A new pathway of CD5 glycoprotein-mediated T cell inhibition dependent on inhibitory phosphorylation of fyn kinase. J Biol Chem. (2011) 286:30324–36. doi: 10.1074/jbc.M111.230102
37. Gary-Gouy H, Lang V, Sarun S, Boumsell L, Bismuth G. In vivo association of CD5 with tyrosine-phosphorylated ZAP-70 and p21 phospho-zeta molecules in human CD3+ thymocytes. J Immunol. (1997) 159:3739–47.
38. Perez-Villar JJ, Whitney GS, Bowen MA, Hewgill DH, Aruffo AA, Kanner SB. CD5 negatively regulates the T-cell antigen receptor signal transduction pathway: involvement of SH2-containing phosphotyrosine phosphatase SHP-1. Mol Cell Biol. (1999) 19:2903–12. doi: 10.1128/MCB.19.4.2903
39. Carmo A, Castro M, Arosa F. CD2 and CD3 associate independently with CD5 and differentially regulate signaling through CD5 in Jurkat T cells. J Immunol. (1999) 163:4238–45.
40. Dennehy KM, Broszeit R, Ferris WF, Beyers AD. Thymocyte activation induces the association of the proto-oncoprotein c-cbl and ras GTPase-activating protein with CD5. Eur J Immunol. (1998) 28:1617–25. doi: 10.1002/(SICI)1521-4141(199805)28:05<1617::AID-IMMU1617>3.0.CO;2-7
41. Voisinne G, García-Blesa A, Chaoui K, Fiore F, Bergot E, Girard L, et al. Co-recruitment analysis of the CBL and CBLB signalosomes in primary T cells identifies CD5 as a key regulator of TCR-induced ubiquitylation. Mol Syst Biol. (2016) 12:876. doi: 10.15252/msb.20166837
42. Dennehy KM, Broszeit R, Garnett D, Durrheim GA, Spruyt LL, Beyers AD. Thymocyte activation induces the association of phosphatidylinositol 3-kinase and pp120 with CD5. Eur J Immunol. (1997) 27:679–86. doi: 10.1002/eji.1830270316
43. Hem CD, Ekornhol M, Granum S, Sundvold-Gjerstad V, Spurkland A. CD6 and linker of activated T cells are potential interaction partners for T cell-specific adaptor protein. Scand J Immunol. (2017) 85:104–12. doi: 10.1111/sji.12513
44. Breuning J, Brown MH. T cell costimulation by CD6 is dependent on bivalent binding of a GADS/SLP-76 complex. Mol Cell Biol. (2017) 37:e00071–00017. doi: 10.1128/MCB.00071-17
45. Gimferrer I, Ibáñez A, Farnós M, Sarrias MR, Fenutría R, Roselló S, et al. The lymphocyte receptor CD6 interacts with syntenin-1, a scaffolding protein containing PDZ domains. J Immunol. (2005) 175:1406–14. doi: 10.4049/jimmunol.175.3.1406
46. Roncagalli R, Hauri S, Fiore F, Liang Y, Chen Z, Sansoni A, et al. Quantitative proteomics analysis of signalosome dynamics in primary T cells identifies the surface receptor CD6 as a Lat adaptor-independent TCR signaling hub. Nat Immunol. (2014) 15:384–92. doi: 10.1038/ni.2843
47. Zhang W, Trible RP, Zhu M, Liu SK, McGlade CJ, Samelson LE. Association of Grb2, Gads, and phospholipase C-gamma 1 with phosphorylated LAT tyrosine residues. Effect of LAT tyrosine mutations on T cell angigen receptor-mediated signaling. J Biol Chem. (2000) 275:23355–61. doi: 10.1074/jbc.M000404200
48. Santos RF, Oliveira L, Carmo AM. Tuning T cell activation: the function of CD6 at the immunological synapse and in T cell responses. Curr Drug Targets (2016) 17:630–9. doi: 10.2174/1389450116666150531152439
49. Zhang W, Sommers CL, Burshtyn DN, Stebbins CC, DeJarnette JB, Trible RP, et al. Essential role of LAT in T cell development. Immunity (1999) 10:323–32. doi: 10.1016/S1074-7613(00)80032-1
50. Dobenecker MW, Schmedt C, Okada M, Tarakhovsky A. The ubiquitously expressed Csk adaptor protein Cbp is dispensable for embryogenesis and T-cell development and function. Mol Cell Biol. (2005) 25:10533–42. doi: 10.1128/MCB.25.23.10533-10542.2005
51. Brdicka T, Pavlistová D, Leo A, Bruyns E, Korínek V, Angelisová P, et al. Phosphoprotein associated with glycosphingolipid-enriched microdomains (PAG), a novel ubiquitously expressed transmembrane adaptor protein, binds the protein tyrosine kinase csk and is involved in regulation of T cell activation. J Exp Med. (2000) 191:1591–604. doi: 10.1084/jem.191.9.1591
52. Kawabuchi M, Satomi Y, Takao T, Shimonishi Y, Nada S, Nagai K, et al. Transmembrane phosphoprotein Cbp regulates the activities of Src-family tyrosine kinases. Nature (2000) 404:999–1003. doi: 10.1038/35010121
53. Bowen MA, Patel DD, Li X, Modrell B, Malacko AR, Wang WC, et al. Cloning, mapping, and characterization of activated leukocyte-cell adhesion molecule (ALCAM), a CD6 ligand. J Exp Med. (1995) 181:2213–20. doi: 10.1084/jem.181.6.2213
54. Patel DD, Wee SF, Whichard LP, Bowen MA, Pesando JM, Aruffo A, et al. Identification and characterization of a 100-kD ligand for CD6 on human thymic epithelial cells. J Exp Med. (1995) 181:1563–8. doi: 10.1084/jem.181.4.1563
55. Saifullah MK, Fox DA, Sarkar S, Abidi SM, Endres J, Piktel J, et al. Expression and characterization of a novel CD6 ligand in cells derived from joint and epithelial tissues. J Immunol. (2004) 173:6125–33. doi: 10.4049/jimmunol.173.10.6125
56. Enyindah-Asonye G, Li Y, Ruth JH, Spassov DS, Hebron KE, Zijlstra A, et al. CD318 is a ligand for CD6. Proc Natl Acad Sci USA. (2017) 114:E6912–21. doi: 10.1073/pnas.1704008114
57. Bhandoola A, Bosselut R, Yu Q, Cowan ML, Feigenbaum L, Love PE, et al. CD5-mediated inhibition of TCR signaling during intrathymic selection and development does not require the CD5 extracellular domain. Eur J Immunol. (2002) 32:1811–7. doi: 10.1002/1521-4141(200206)32:6<1811::AID-IMMU1811>3.0.CO;2-G
58. Singer NG, Fox DA, Haqqi TM, Beretta L, Endres JS, Prohaska S, et al. CD6: expression during development, apoptosis and selection of human and mouse thymocytes. Int Immunol. (2002) 14:585–97. doi: 10.1093/intimm/dxf025
59. Hassan NJ, Barclay AN, Brown MH. Optimal T cell activation requires the engagement of CD6 and CD166. Eur J Immunol. (2004) 34:930–40. doi: 10.1002/eji.200424856
60. Te Riet J, Zimmerman AW, Cambi A, Joosten B, Speller S, Torensma R, et al. Distinct kinetic and mechanical properties govern ALCAM-mediated interactions as shown by single-molecule force spectroscopy. J Cell Sci. (2007) 120:3965–76. doi: 10.1242/jcs.004010
61. Li Y, Singer NG, Whitbred J, Bowen MA, Fox DA, Lin F. CD6 as a potential target for treating multiple sclerosis. Proc Natl Acad Sci USA. (2017) 114:2687–92. doi: 10.1073/pnas.1615253114
62. Zhang C, Xin H, Zhang W, Yazaki PJ, Zhang Z, Le K, et al. CD5 Binds to interleukin-6 and induces a feed-forward loop with the transcription factor STAT3 in B cells to promote cancer. Immunity (2016) 44:913–23. doi: 10.1016/j.immuni.2016.04.003
63. Orta-Mascaró M, Consuegra-Fernández M, Carreras E, Roncagalli R, Carreras-Sureda A, Alvarez P, et al. CD6 modulates thymocyte selection and peripheral T cell homeostasis. J Exp Med. (2016) 213:1387–97. doi: 10.1084/jem.20151785
64. Peña-Rossi C, Zuckerman LA, Strong J, Kwan J, Ferris W, Chan S, et al. Negative regulation of CD4 lineage development and responses by CD5. J Immunol. (1999) 163:6494–501.
65. Tarakhovsky A, Müller W, Rajewsky K. Lymphocyte populations and immune responses in CD5-deficient mice. Eur J Immunol. (1994) 24:1678–84.
66. Dasu T, Qualls JE, Tuna H, Raman C, Cohen DA, Bondada S. CD5 plays an inhibitory role in the suppressive function of murine CD4(+) CD25(+) T(reg) cells. Immunol Lett. (2008) 119:103–13. doi: 10.1016/j.imlet.2008.05.008
67. Samaha H, Pignata A, Fousek K, Ren J, Lam FW, Stossi F, et al. A homing system targets therapeutic T cells to brain cancer. Nature (2018) 561:331–7. doi: 10.1038/s41586-018-0499-y
68. De Jager PL, Jia X, Wang J, de Bakker PI, Ottoboni L, Aggarwal NT, et al. Meta-analysis of genome scans and replication identify CD6, IRF8 and TNFRSF1A as new multiple sclerosis susceptibility loci. Nat Genet. (2009) 41:776–82. doi: 10.1038/ng.401
69. Kofler DM, Severson CA, Mousissian N, De Jager PL, Hafler DA. The CD6 multiple sclerosis susceptibility allele is associated with alterations in CD4+ T cell proliferation. J Immunol. (2011) 187:3286–91. doi: 10.4049/jimmunol.1100626
70. Castro M, Oliveira M, Nunes R, Fabre S, Barbosa R, Peixoto A, et al. Extracellular Isoforms of CD6 generated by alternative splicing regulate targeting of CD6 to the immunological synapse. J Immunol. (2007) 178:4351–61. doi: 10.4049/jimmunol.178.7.4351
71. Alonso R, Huerta V, de Leon J, Piedra P, Puchades Y, Guirola O, et al. Towards the definition of a chimpanzee and human conserved CD6 domain 1 epitope recognized by T1 monoclonal antibody. Hybridoma (2008) 27:291–301. doi: 10.1089/hyb.2008.0007
72. Hernández P, Moreno E, Aira LE, Rodríguez PC. Therapeutic targeting of CD6 in autoimmune diseases: a review of cuban clinical studies with the antibodies IOR-T1 and itolizumab. Curr Drug Targets (2016) 17:666–77. doi: 10.2174/1389450117666160201114308
73. Rodriguez PC, Torres-Moya R, Reyes G, Molinero C, Prada D, Lopez AM, et al. A clinical exploratory study with itolizumab, an anti-CD6 monoclonal antibody, in patients with rheumatoid arthritis. Results Immunol. (2012) 2:204–11. doi: 10.1016/j.rinim.2012.11.001
74. Krupashankar DS, Dogra S, Kura M, Saraswat A, Budamakuntla L, Sumathy TK, et al. Efficacy and safety of itolizumab, a novel anti-CD6 monoclonal antibody, in patients with moderate to severe chronic plaque psoriasis: results of a double-blind, randomized, placebo-controlled, phase-III study. J Am Acad Dermatol. (2014) 71:484–92. doi: 10.1016/j.jaad.2014.01.897
75. Aira LE, López-Requena A, Fuentes D, Sánchez L, Pérez T, Urquiza A, et al. Immunological and histological evaluation of clinical samples from psoriasis patients treated with anti-CD6 itolizumab. MAbs (2014) 6:783–93. doi: 10.4161/mabs.28376
76. Garner LI, Hartland A, Breuning J, Brown MH. CD6 monoclonal antibodies differ in epitope, kinetics and mechanism of action. Immunology (2018) 155:273–82. doi: 10.1111/imm.12952
77. Chappell PE, Garner LI, Yan J, Metcalfe C, Hatherley D, Johnson S, et al. Structures of CD6 and its ligand CD166 give insight into their interaction. Structure (2015) 23:1426–36. doi: 10.1016/j.str.2015.05.019
78. Singer NG, Mitra R, Lialios F, Richardson BC, Marks RM, Pesando JM, et al. CD6 dependent interactions of T cells and keratinocytes: functional evidence for a second CD6 ligand on gamma-interferon activated keratinocytes. Immunol Lett. (1997) 58:9–14. doi: 10.1016/S0165-2478(97)02707-7
Keywords: inhibitory receptors, CD5, CD6, signalosome, T lymphocytes
Citation: Gonçalves CM, Henriques SN, Santos RF and Carmo AM (2018) CD6, a Rheostat-Type Signalosome That Tunes T Cell Activation. Front. Immunol. 9:2994. doi: 10.3389/fimmu.2018.02994
Received: 31 July 2018; Accepted: 04 December 2018;
Published: 18 December 2018.
Edited by:
Bernard Malissen, INSERM U1104 Centre d'Immunologie de Marseille-Luminy, FranceReviewed by:
Renaud Lesourne, INSERM U1043 Centre de Physiopathologie de Toulouse Purpan, FranceJacques A. Nunes, INSERM U1068 Centre de Recherche en Cancérologie de Marseille, France
Copyright © 2018 Gonçalves, Henriques, Santos and Carmo. This is an open-access article distributed under the terms of the Creative Commons Attribution License (CC BY). The use, distribution or reproduction in other forums is permitted, provided the original author(s) and the copyright owner(s) are credited and that the original publication in this journal is cited, in accordance with accepted academic practice. No use, distribution or reproduction is permitted which does not comply with these terms.
*Correspondence: Alexandre M. Carmo, acarmo@ibmc.up.pt