- 1Immunology and General Pathology Laboratory, Department of Biotechnology and Life Sciences, University of Insubria, Varese, Italy
- 2UOC Cell Biology, IRCCS Ospedale Policlinico San Martino, Genoa, Italy
- 3Vascular Biology and Angiogenesis Laboratory, Scientific and Technologic Park, IRCCS MultiMedica, Milan, Italy
- 4UOSD Molecular Oncology and Angiogenesis Unit, IRCCS Ospedale Policlinico San Martino, Genoa, Italy
- 5UOC Immunology Unit, IRCCS Ospedale Policlinico San Martino, Genoa, Italy
Antibody-cytokine fusion proteins (immunocytokine) exert a potent anti-cancer effect; indeed, they target the immunosuppressive tumor microenvironment (TME) due to a specific anti-tumor antibody linked to immune activating cytokines. Once bound to the target tumor, the interleukin-2 (IL-2) immunocytokines composed of either full antibody or single chain Fv conjugated to IL-2 can promote the in situ recruitment and activation of natural killer (NK) cells and cytotoxic CD8+ T lymphocytes (CTL). This recruitment induces a TME switch toward a classical T helper 1 (Th1) anti-tumor immune response, supported by the cross-talk between NK and dendritic cells (DC). Furthermore, some IL-2 immunocytokines have been largely shown to trigger tumor cell killing by antibody dependent cellular cytotoxicity (ADCC), through Fcγ receptors engagement. The modulation of the TME can be also achieved with immunocytokines conjugated with a mutated form of IL-2 that impairs regulatory T (Treg) cell proliferation and activity. Preclinical animal models and more recently phase I/II clinical trials have shown that IL-2 immunocytokines can avoid the severe toxicities of the systemic administration of high doses of soluble IL-2 maintaining the potent anti-tumor effect of this cytokine. Also, very promising results have been reported using IL-2 immunocytokines delivered in combination with other immunocytokines, chemo-, radio-, anti-angiogenic therapies, and blockade of immune checkpoints. Here, we summarize and discuss the most relevant reported studies with a focus on: (a) the effects of IL-2 immunocytokines on innate and adaptive anti-tumor immune cell responses as well as immunosuppressive Treg cells and (b) the approaches to circumvent IL-2-mediated severe toxic side effects.
Introduction
Viewing the tumor microenvironment (TME) like a critical orchestrator in tumor biology has been a central paradigm shift of the cancer field during the past two decades. Within this time, the distinct role of tissue-residing cells in promoting or suppressing tumor growth, metastasis and resistance to therapy has been gradually elucidated. Among the host-dependent biological features of the tumor, the hallmarks defined by Hanahan and Weinberg, such as the “evading immune destruction”, the “tumor-promoting inflammation”, and the “immune orchestration of angiogenesis” point out the key role of the immune system in neoplastic disease (1). Therefore, diverse cells both from innate or adaptive immunity, as a consequence of their plasticity, have been reported to acquire an altered phenotype and functions upon TME interaction; indeed, the cross-talk between TME and immune system leads to (a) attenuation of targeting and killing of tumor cells, (b) generation of tolerogenic/immunosuppressive behavior, and (c) acquisition of pro-angiogenic activities (1–5). As soon as the immune checkpoint inhibitors entered the clinic showing important and long lasting responses, the immune system gained greater attention in cancer biology.
Cytokines are molecular messengers, allowing immune cells to communicate with each other and with the TME compartments. Growing interest has been focused in exploiting the immune system to eradicate cancer using different cytokines. Nonetheless, toxicity and dual ambivalent activities of some cytokines (tumor promoting vs. tumor inhibiting) still remain relevant issues. In this context, immunotherapy approaches, and cytokine therapy, has been a promising strategy for the treatment of cancer (6).
IL-2 cytokine displays multiple immunological effects and acts by binding to the IL-2 receptor (IL-2R). The association of IL-2Rα (CD25), IL-2Rβ (CD122), and IL-2Rγ (CD132) subunits results in the trimeric high affinity IL-2Rαβγ. CD25 confers high affinity binding to IL-2, whereas the β and γ subunits (expressed on natural killer (NK) cells, monocytes, macrophages and resting CD4+ and CD8+ T cells) mediate signal transduction (7, 8). It appears that the expression of CD25 is essential for the expansion of immunosuppressive regulatory T cells (Treg); on the other hand, cytolytic CD8+ T and NK cells can proliferate and kill target cells responding to IL-2 by the IL-2Rβγ engagement in the absence of CD25 (9). The IL-2 cytokine acts as a master activation factor for helper/regulatory T cell and NK cell proliferation, differentiation and acts as a relevant mediator for pro- and anti-inflammatory immune responses (10). Treatment with IL-2 has been associated with stable and curative regressions in patients with metastatic melanoma, renal cancer and advanced non-Hodgkin's lymphomas, representing the first effective immunotherapeutic agent (11). Generally, IL-2 can evoke some mild common side effects, such as a flu-like syndrome, fever, asthenia, nausea, and vomiting. These side effects are more frequent and more relevant when IL-2 administration is associated with chemotherapy. However, the very rare observation of potential life-threatening clinical toxicities, such as vascular leakage syndrome (VLS), severe flu-like symptoms and coma has recommended that the IL-2 be employed under the supervision of the oncologist in a hospital setting. IL-2 immunocytokines have been developed both to avoid these undesired clinical side effects, and to target cancer cells with specific anti-tumor antibodies fused with IL-2 that can elicit a potent anti-tumor immunological response within the immunosuppressive TME.
Here, according to the literature reviewing, we summarized and discussed: (a) the effects of IL-2 immunocytokines on innate and adaptive anti-tumor immune response and (b) their use in combination with other immunocytokines, chemio- and radio-therapy, immune checkpoint blockade, and immunotherapies.
IL-2-Targeted Anti-Cancer Therapies
IL-2 was identified in 1976 as a T cell growth factor and later approved for treatment of patients with metastatic melanoma and renal cell carcinoma with beneficial results in a subset of patients (11, 12). Administration of high-dose IL-2 can be associated with relevant adverse effects that include the VLS, fever, chills, malaise, hypotension, organ dysfunction and cytopenia, well-reviewed previously (13–17). Low-doses of IL-2 lead to the preferential expansion of Treg cells; this is an unwanted effect in anti-cancer immunotherapy (18). To overcome the toxicity related to the systemic administration of IL-2 at high-dose, diverse IL-2 immunocytokines composed of IL-2 fused to antibodies directed against tumor-associated antigens (TAAs) have been tested in preclinical models with promising results (10, 19–48). Indeed, some IL-2 immunocytokines are currently in phases I–II of several clinical trials, in combination with other therapeutics (49–56) (https://www.clinicaltrials.gov). These immunocytokines showed beneficial effects for a wide range of tumor types with manageable and reversible side effects and toxicities (49–51, 53, 54, 57–60). IL-2 immunocytokines-targeted proteins are represented by either surface membrane TAAs, such as disialoganglioside 2 (GD2), epithelial cell adhesion molecule (EpCAM), carcinoembryonic antigen (CEA), CD20, and CD30 or proteins belonging to tumor extracellular matrix (ECM) like extra domain A (ED-A) and B (ED-B) of Fibronectin A-FN and B-FN, respectively and tenascin-C. The targeting of proteins expressed on the cell surface of tumor cells presents some limitations, such as the transitory expression of TAAs, the rapid immunocytokine internalization, localization and degradation into the lysosomal compartment, determining failure of the expected therapeutic effect. The tumor ECM proteins have been proposed as good targets due to the over-expression of isoforms absent or barely expressed in the ECM of normal tissues (61, 62). An overview of IL-2-immunocytokines in preclinical and clinical development for treatment of cancer is summarized in Table 1 and some examples will be discussed here.
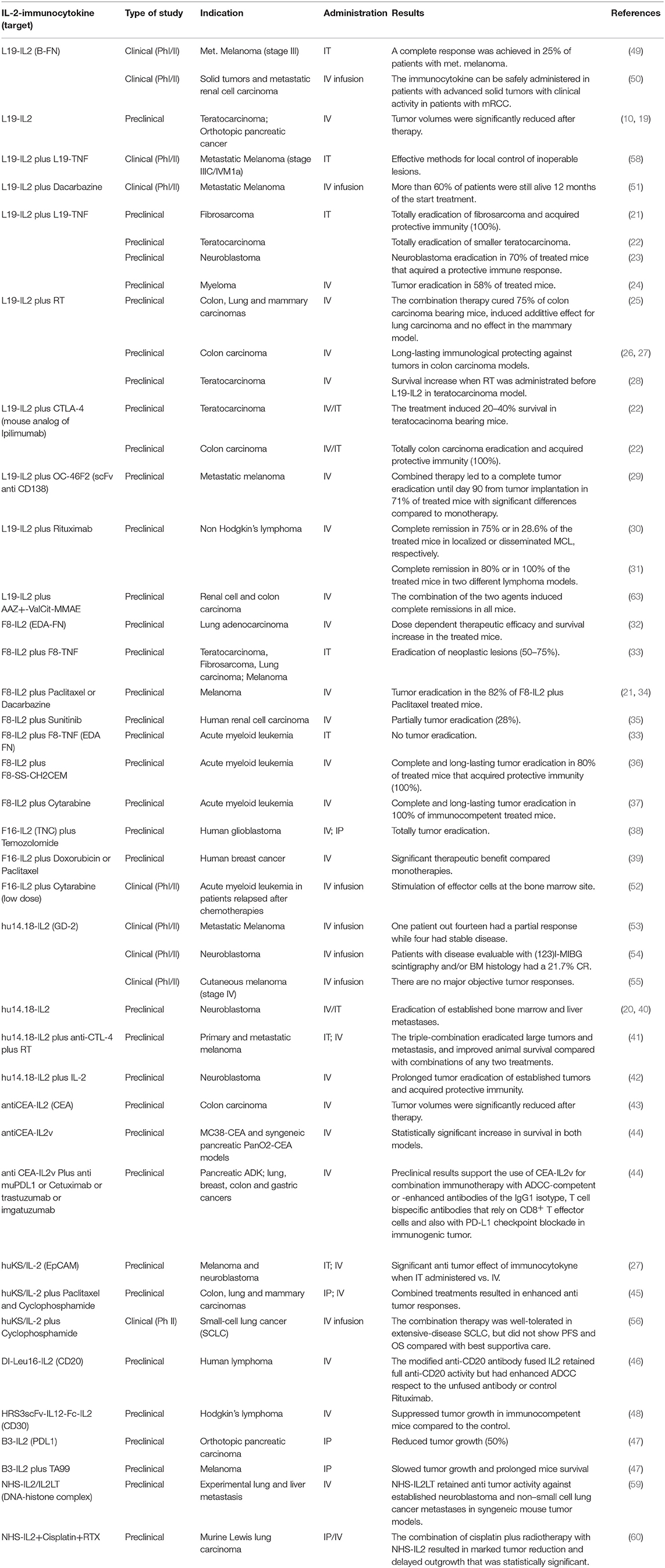
Table 1. IL-2-immunocytokines in preclinical and clinical development for treatment of various types of cancer.
Among the IL-2 immunocytokines directed to ECM, the first and most studied was L19-IL-2. L19-IL-2 is specific for the angiogenesis-associated B-FN isoform selectively accumulated on tumor neovasculature; L19-IL-2 showed a good anti-tumor activity in preclinical models both in solid and hematological tumors (Table 1). A complete tumor eradication was reported when L19-IL-2 was administered in combination with CTLA-4 blockade in two syngeneic immunocompetent mouse models of teratocarcinoma and colon carcinoma; in the latter model, responder mice to this combination treatment were resistant to tumor re-challenge (22). Complete remissions of established localized lymphomas were induced when L19-IL-2 was co-administered with the anti-CD20 antibody rituximab (31). Recently, similar preclinical results were observed in renal cell carcinoma and colorectal cancer models when L19-IL-2 was co-administered with a small molecule-drug conjugate, capable of selective homing to tumor cells expressing surface carbonic anhydrase IX (63). Rekers et al. have recently reported that radiotherapy (RT) combined to systemic administration of L19-IL-2, resulted in a long-lasting immunological protection against tumors in mouse colon carcinoma. These authors hypothesize that the IL-2 immunocytokine and RT could elicit an immune-mediated abscopal effect with tumor regression far from the irradiated tumor field (25–28). Preclinical data have shown that a combination of L19-IL-2 and L19-TNF-α induced complete remission when administered as a single intratumoral injection in two immunocompetent mouse models of melanoma and sarcoma (21). These promising results led to multicenter phase II trials with the intralesional application of L19-IL-2 as single agent or in combination with L19-TNF-α in stage IIIB/IIIC and IVM1 melanoma patients as recently reviewed by Weide et al. (64).
Cancer immunotherapy holds promising synergistic potential when combined with chemotherapy and anti-angiogenic therapy. However, to our knowledge, the combined therapy of L19-IL-2 with anti-angiogenic drugs, such as bevacizumab, has not been reported until now. It is known the pro-angiogenic role of the cell surface proteoglycan syndecan-1 (CD138) whose ectodomain is a target of ADAM17 sheddase activity. The soluble ectodomain of syndecan-1 binds several matrix effectors (e.g., VEGF, FGF-2, or cytokines) and presents them to the corresponding cell surface receptors promoting angiogenesis and tumor growth (65, 66). We have recently reported that blocking syndecan-1 activity via the specific antibody OC-46F2 leads to an anti-tumor effect by inhibiting vascular maturation and tumor growth in experimental models of human melanoma and ovarian carcinoma (67). Furthermore, we have shown that OC-46F2, in combination with the L19-IL-2 resulted in complete inhibition of melanoma growth until day 90 from tumor implantation in 71% of treated mice with a significant increase of their tumor free survival (29).
The hu14.18-IL-2 immunocytokine containing a humanized anti-GD2 mAb linked to IL-2 was mainly studied as mono-therapy in neuroblastoma and in combination with anti-CTLA-4 plus RT in primary and metastatic melanoma. The triple-combination therapy eradicated large tumors and metastasis improving animal survival (41). A still ongoing phase II clinical trial using hu14.18-IL-2, reported stable disease in four patients and a partial response in one patient out of fourteen in metastatic melanoma (53) while not significant results were shown by the other two phase I/II trials already completed (54, 55).
Recently, it has been described as a novel class of monomeric tumor-targeted immunocytokines in which a single engineered IL-2 variant (IL-2v) with abolished CD25 binding is fused to the C-terminus of an antibody against the CEA or fibroblast activation protein-α (FAP). CEA-IL-2v and FAP-IL-2v demonstrated superior safety, pharmacokinetics and tumor targeting, while lacking preferential induction of Treg cells due to abolished CD25 binding. At the same time, these constructs showed monovalency and high-affinity tumor targeting as compared to classical IL-2 based immunocytokines. They retain the capacity to activate and expand NK and CD8+ effector T cells through IL-2Rγβ in the periphery and in the TME (Klein C.; 1st Immunotherapy of Cancer Conference (ITOC1) Munich, Germany, 2014) (44, 68).
Both in MC38-CEA and syngeneic pancreatic PanO2-CEA models, animals treated with CEA-IL2v monotherapy showed a statistically significant increase in median survival compared to untreated animals. Moreover, CEA-IL2v treatment resulted in a superior efficacy when administered in combination with PD-L1 checkpoint blockade or with ADCC competent antibodies, such as trastuzumab and cetuximab (44). In the syngeneic PancO2 model, similar results were recently described using FAP-IL2v associated with a CD40 agonistic and PD-L1 inhibitory checkpoint antibodies as reported by Nicolini V. et al. at AACR Annual Meeting 2018; Chicago, IL (https://www.clinicaltrials.gov, NCT02627274; NCT03386721).
Although it is not an immunocytokine, it is important to analyze the effects of OMCP-mutIL-2, a mutated form of IL-2 (mutIL-2) linked to a high-affinity NKG2D ligand (OMCP), which is directed to cytotoxic immune effector cells rather than tumor cells. This targeted therapy resulted in preferential binding to and activation of NK cells rather than Treg cells and a significant decrease of tumor growth was obtained after treatment with OMCP-mutIL-2 in mouse models of Lewis lung carcinoma (LLC) (69).
Outstanding Relevance of Triggering NK Cell Activity in Therapeutic Effect of IL-2 Immunocytokines
It is well-established that IL-2 can trigger T lymphocytes to expand and acquire a therapeutic anti-tumor activity (70). Noteworthy, cytotoxic CD8+ T cells can be activated with IL-2 mainly by IL-2Rβγ, whereas regulatory T cells can efficiently respond to IL-2 through the IL-2Rαβγ complex (71–75). These peculiar features of CD8+ T cells have been used to design unique IL-2 molecules and favor the expansion of cytotoxic anti-tumor rather than regulatory T lymphocytes (72–75). Likewise, NK cells can respond efficiently to IL-2 through the IL-2Rβγ in the absence of IL-2Rαβγ heterotrimer (18, 70, 71, 76). Since NK cell can kill their target without prior sensitization or priming, they may represent a good candidate to respond to in vivo during administration of immunocytokines composed of IL-2 (20, 38, 70, 77). This is the case for the hu14.18-IL-2 immunocytokine, where depletion of NK cells resulted in the abrogation of the anti-tumor response detected in vivo in preclinical murine model of NXS2 neuroblastoma (20). Furthermore, the effect of hu14.18-IL-2 immunocytokine was strongly enhanced when combined with poly I:C or recombinant mouse IFN-γ which can be considered potent NK cell stimulating factors (20). Impressively, only NK cells, but not CD8+ T cells, isolated from these mice exerted a detectable cytolytic activity against the NK cell target YAC-1. This would indicate that in this murine model system NK cells can cure from neuroblastoma. It is not clear whether this effect is dependent only on IL-2-mediated activation of NK cells, or other cytolytic effector cells, such as NK-like T and/or γδ T cells not expressing CD8. In addition, both poly I:C and IFN-γ can be potent stimulators of antigen presenting cells (APC) as monocytes and monocyte-derived dendritic cells (mDC) (20, 78, 79). More importantly, APC can produce IL-12 (79), a strong inducer of NK cell cytotoxicity, and it is still to be defined whether poly I:C and IFN-γ can exert both direct and indirect effect on NK cell activation. We can speculate that the crosstalk between NK and DC, further reinforced by the triggering with poly I:C and IFN-γ of both NK and DC, could generate a positive loop to produce high IL-12 and amplify NK cell response (80, 81); this could eventually generate a Th1 microenvironment favoring anti-tumor adaptive immune response (Figure 1A).
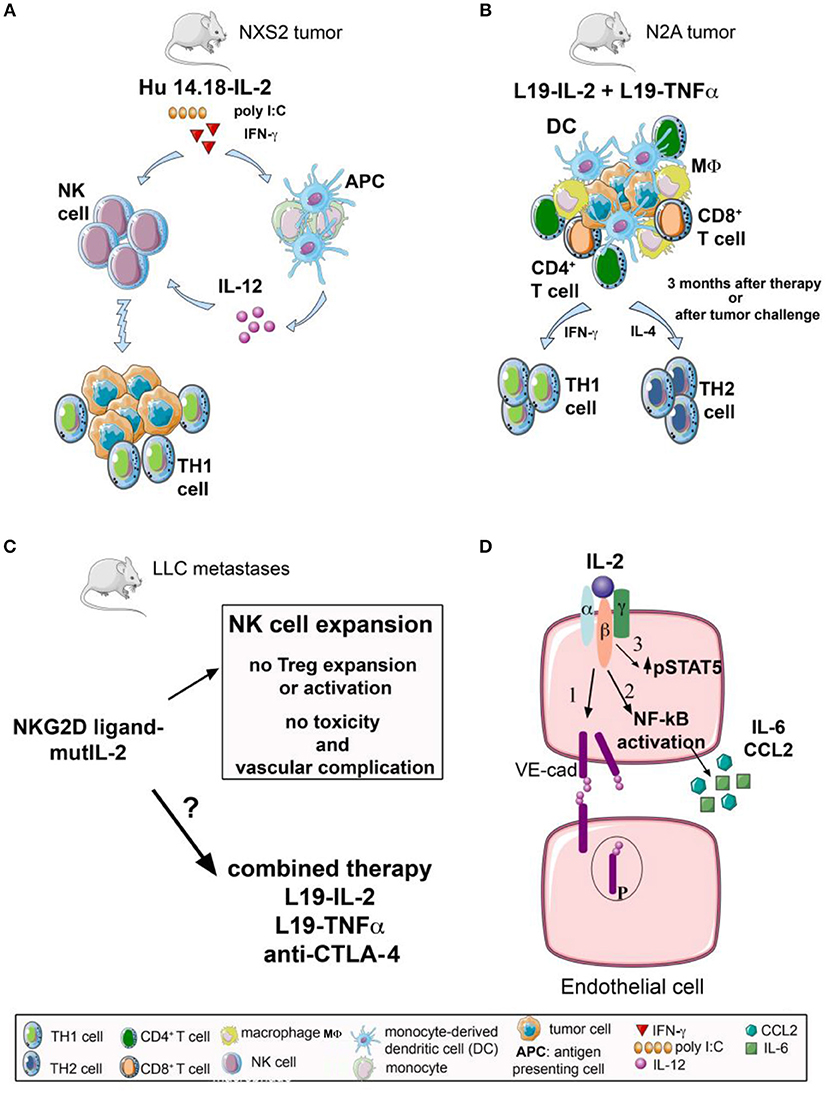
Figure 1. Effects on innate and adaptive immune response of IL-2 immunocytokines and IL-2 fusion protein either alone or in combination with other therapeutic approaches, and IL-2 mediated modulation of endothelial cells. (A) The NK cell stimulating effect of hu14.18-IL2 immunocytokine, containing a humanized anti-GD2 mAb linked to IL-2, is strongly enhanced when combined with poly I:C or recombinant mouse IFN-γ. Poly I:C and IFN-γ can be potent stimulators of antigen presenting cells (APC) as monocytes and monocyte-derived dendritic cells (mDC) that can produce IL-12, a strong inducer of NK cell cytotoxicity. This mechanism could eventually generate a Th1 microenvironment favoring anti-tumor adaptive immune response. (B) L19-IL-2 in combination with another immunocytokine, L19-TNF-α, shows therapeutic synergistic effects in neuroblastoma N2A murine model. 70% of systemically treated mice result in a specific long-lasting anti-tumor immune memory, with efficient priming of CD4+ T helper cells and CD8+ CTL effectors, massive tumor infiltration of CD4+, CD8+ T cells, macrophages and dendritic cells, accompanied by a mixed Th1/Th2 response. (C) The use of a fusion protein consisting in a mutated form of IL-2 targeting NKG2D-positive cells (OMCP-mutIL2) is employed as a monotherapy, in a preclinical model of Lewis lung carcinoma (LLC). This protocol is highly efficient in stimulating anti-tumor NK cells and their cytotoxicity with no involvement of Treg cells and in absence of vascular-related toxicity. It is still to be investigated if OMCP-mutIL2 can display a synergistic effect in those combination therapies which trigger the anti-tumor adaptive T cell response. (D) IL-2 is able to interact with IL-2R complex (IL-2Rβ and IL-2Rγ) on brain microvascular endothelial cells (BMEC) inducing: (1) destabilization of adherent junctions through an increase in VE-cadherin (VE-cad) phosphorylation and internalization accompanied by NF-kB activation, and (2) release of pro-inflammatory mediators, such as CCL2 and IL-6, resulting in brain oedema. Moreover, (3) IL-2 binds directly to CD25+ lung endothelial cells with an increase of STAT5 phosphorylation inducing pulmonary oedema.
Regarding the therapeutic efficiency of NK cells under administration of IL-2 immunocytokines, it is relevant to analyze the role of major histocompatibility complex antigens (82). It is well-established that NK cells in allogeneic hematopoietic stem cell transplant (HSCT) can show the so called “killer immunoglobulin-like receptor (KIR)/KIR-ligand incompatibility”. An improvement of leukemia control is related to a difference in HLA-I between the donor and recipient because the corresponding KIR expressed on NK cell does not recognize the HLA-I antigen (83). Thus, due to the KIR/KIR-ligand mismatch the KIR on NK cell donor does not deliver a signal in NK cell leading to inhibition of NK-cell mediated killing of residual leukemia cells present in the recipient. Importantly, the KIR/KIR-ligand mismatch can happen also in an autologous setting (84, 85). In relapsed/refractory neuroblastoma patients, hu14.18-IL-2 immunocytokine administered to the cohort with the KIR/KIR-ligand mismatch showed a better anti-tumor response to that of the matched cohort patients (82). Thus, the KIR/KIR-ligand mismatch analysis should be associated with the immunocytokine therapy to further improve the NK cell response in anti-neuroblastoma activity (82). The involvement of NK cells in the therapeutic effect of IL-2 immunocytokine has been further confirmed in targeting the tumor stroma with the F16-IL-2 immunocytokine (52). In point of fact, F16-IL-2 treatment of acute myeloid leukemia (AML) relapsed patients after HSCT led to a massive accumulation of lymphocytes in the bone marrow and CD56+CD16+ NK cells represented the most prominent increment, besides γδT and CD8+ T cells (52). In addition, lymphocytes appeared in contact with clusters of leukemic blasts suggesting that a recognition of tumor cells and formation of immunological synapses have been clearly established in F16-IL-2 treated patients.
Role of Adaptive T-cell Responses in the Anti-tumor Therapeutic Efficacy in IL-2 Immunocytokines Treatments
L19-IL-2 has been largely studied in different tumor preclinical mouse models, indicating a powerful action of this compound in the ability to induce a pro-inflammatory reaction and a tumor influx of lymphocytes together with an IFN-γ response and NK and/or T cell responses. Interestingly, in the CT26 colon carcinoma murine model, L19-IL2 as well as anti-CTLA-4 mAb treatments have been shown to be active as single agents; importantly, the combination of the IL-2 immunocytokine and the immune checkpoint blocker determined an enhanced anti-tumor therapeutic effect and a prolonged survival (22). In this tumor model, treated and cured mice have been protected following tumor re-challenging, indicating memory of anti-tumor immunity. But there was no synergy between L19-IL-2 and anti-PD-1 checkpoint blockade with increased survival of CT26-tumor bearing mice; this would suggest that to obtain with this IL-2 immunocytokine a synergistic anti-tumor effect a distinctive immune checkpoint blocker should be targeted. Intratumoral L19-IL-2 in combination with L19-TNF-α immunocytokine led to a complete cure of 100% treated F9 teratocarcinoma-bearing mice. By contrast, in athymic mice, tumor rejection capacity elicited by the combination of these immunocytokines was impaired; only a delayed tumor growth in comparison to the control immunocompetent mice was observed; this points out the relevance of T cell response for the complete eradication of tumors (22). Synergy between L19-IL-2 and L19-TNF-α has been also documented in neuroblastoma models (Figure 1B) (23). L19-TNF-α is also considered a crucial element in combined anti-tumor immunotherapeutic strategies, showing encouraging results in both preclinical (86–88) and clinical studies (58).
Intratumoral injections with hu14.18-IL-2 in neuroblastoma NXS2 murine model showed an enhanced inhibition of tumor growth and prolonged survival compared with controls; this therapeutic effect involved both NK and T cells localized in situ and peripheral blood (40). Interestingly, after intratumoral injection, an enhanced proportion of both NK and T cells expressing NKG2A/C/E antigens in comparison to control mice and intravenous-treated tumor-bearing mice was detected. Moreover, this therapeutic approach induced a remarkable tumor infiltration of CD8+ CTLs, CD4+ T cells as well as macrophages. In in vivo immune cell subsets depletion assays demonstrated a key role for CD4+, CD8+ T and NK cells. Remarkably, the hu14.18-IL-2 immunocytokine has been shown to synergize with local RT and systemic checkpoint blockade (anti-CTLA-4 mAb) to eradicate large tumor and metastases in different tumor murine models (38). An important issue derived from these studies was that the cooperative effect was mediated, at least in part, by NK cells through ADCC and that the use of tumor-specific IL-2 immunocytokine led to memory T-cell responses (38).
The F8-IL-2 immunocytokine when used as monotherapy in a metastatic adenocarcinoma lung mouse model resulted in strong tumor infiltration of both CD3+ T and NK cells but not of Treg cells and F4/80+CD11b+ macrophages. Of note, in this model, TILs also contained an enhanced percentage of intratumoral proliferating Ki67+ Granzyme B+ CD8+ T cells (32).
Finally, monotherapy with OMCP-mutIL2, demonstrated a strong NK cell-mediated anti-tumor effect but no involvement of adaptive immune response, at least in the LLC model (66). It is still to be elucidated if OMCP-mutIL2 could have additive or synergistic anti-tumor effects in association with therapies that can trigger T-cell responses (Figure 1C).
Could IL-2 be Directly Involved in the Tumor Vessels Destruction?
As mentioned above, one of the major and potentially fatal side effects upon administration of high-dose IL-2 is the VLS. This syndrome is characterized by the accumulation of fluid in the extravascular space in multiple organs, such as heart, lung, kidney, and brain. IL-2 can induce VLS acting either indirectly or directly on endothelial cells. Indeed, VLS is caused by the release of pro-inflammatory cytokines, such as TNF-α from IL-2–activated NK cells (89); in turn, this TNF-α alters the vascular permeability. Furthermore, IL-2 is able to induce both pulmonary and brain oedema binding directly CD25 expressed on lung and brain microvascular endothelial cells (BMEC); this engagement leads to disruption of the integrity of lung vascular permeability and blood-brain barrier (BBB) (90, 91).
Moreover, IL-2 interacts on BMEC with intermediate affinity IL-2Rβγ complex inducing destabilization of adherent junctions through an increase in VE-cadherin phosphorylation and internalization accompanied by NF-kB activation; this results in the release of pro-inflammatory mediators, such as CCL2 and IL-6 (Figure 1D) (91, 92). Thus, it is essential that the targeted therapy with IL-2 immunocytokines should avoid, or at least reduce, the VLS in healthy organs. Importantly, recent results from tumor-targeting IL-2 immunocytokines composed of variant forms of IL-2 lacking vascular effects and low or absent Treg cell stimulation have shown promising new avenues for IL-2 applications (44, 69, 90, 93). In addition, the molecular and biochemical mechanisms of IL-2-mediated activation of endothelial cells could be investigated in vitro and in vivo on several types of tumor-associated cells (e.g., tumor-associated endothelial cell and tumor-associated fibroblast) expressing the different chains of IL-2R; this could be potentially exploited for the treatment of tumors.
Conclusion
IL-2 therapy can lead to durable responses in cancer patients but it is associated with significant toxicity and even life-threatening syndromes. IL-2 immunocytokines, alone or in combination with other immunocytokines, checkpoint blockade, chemio-, radio- and/or immunotherapies showed cooperative anti-tumor effects without relevant toxicities; indeed, the vast majority of preclinical tumor models have shown a strong therapeutic response to IL-2 immunocytokine. This is the firm starting point to employ IL-2 immunocytokine to improve the patients' survival and to treat metastatic cancers as well.
Author Contributions
LM, EB, AB, AP, PO, and BC planned, organized, wrote and revised the manuscript, and prepared the figure and table.
Funding
This work was supported by the University of Insubria intramural grant FAR 2017–2019 and the MIUR National Fund for Basic Research grant FFABR 2017 to LM, by the 5xmille 2014 and 5xmille 2015 from Italian Ministry of Health to AP; by 5xmille 2014 and 5xmille 2015 from Italian Ministry of Health to PO. PO is recipient of a researcher contract funded by Italian Ministry of Health RF-2013, GR-2013-02356568.
Conflict of Interest Statement
The authors declare that the research was conducted in the absence of any commercial or financial relationships that could be construed as a potential conflict of interest.
References
1. Hanahan D, Weinberg RA. Hallmarks of cancer: the next generation. Cell (2011) 144:646–74. doi: 10.1016/j.cell.2011.02.013
2. Balkwill FR, Capasso M, Hagemann T. The tumor microenvironment at a glance. J Cell Sci. (2012) 125:5591–6. doi: 10.1242/jcs.116392
3. Bruno A, Ferlazzo G, Albini A, Noonan DM. A think tank of TINK/TANKs: tumor-infiltrating/tumor-associated natural killer cells in tumor progression and angiogenesis. J Natl Cancer Institute (2014) 106:dju200. doi: 10.1093/jnci/dju200
4. Noonan DM, De Lerma Barbaro A, Vannini N, Mortara L, Albini A. Inflammation, inflammatory cells and angiogenesis: decisions and indecisions. Cancer Metastasis Rev. (2008) 27:31–40. doi: 10.1007/s10555-007-9108-5
5. Sica A, Mantovani A. Macrophage plasticity and polarization: in vivo veritas. J Clin Invest. (2012) 122:787–95. doi: 10.1172/JCI59643
6. Dougan M, Dranoff G. Immune therapy for cancer. Annu Rev Immunol. (2009) 27:83–117. doi: 10.1146/annurev.immunol.021908.132544
7. Waldmann TA. The biology of interleukin-2 and interleukin-15: implications for cancer therapy and vaccine design. Nat Rev Immunol. (2006) 6:595–601. doi: 10.1038/nri1901
8. Boyman O, Sprent J. The role of interleukin-2 during homeostasis and activation of the immune system. Nat Rev Immunol. (2012) 12:180–90. doi: 10.1038/nri3156
9. Spolski R, Li P, Leonard WJ. Biology and regulation of IL-2: from molecular mechanisms to human therapy. Nat Rev Immunol. (2018) 18:648–59. doi: 10.1038/s41577-018-0046-y
10. Carnemolla B, Borsi L, Balza E, Castellani P, Meazza R, Berndt A, et al. Enhancement of the antitumor properties of interleukin-2 by its targeted delivery to the tumor blood vessel extracellular matrix. Blood (2002) 99:1659–65. doi: 10.1182/blood.V99.5.1659
11. Rosenberg SA. IL-2: the first effective immunotherapy for human cancer. J Immunol. (2014) 192:5451–8. doi: 10.4049/jimmunol.1490019
12. Morgan DA, Ruscetti FW, Gallo R. Selective in vitro growth of T lymphocytes from normal human bone marrows. Science (1976) 193:1007–8.
13. Pachella LA, Madsen LT, Dains JE. The Toxicity and Benefit of Various Dosing Strategies for Interleukin-2 in metastatic melanoma and renal cell carcinoma. J Adv Pract Oncol. (2015) 6:212–21. doi: 10.6004/jadpro.2015.6.3.3
14. West WH, Tauer KW, Yannelli JR, Marshall GD, Orr DW, Thurman GB, et al. Constant-infusion recombinant interleukin-2 in adoptive immunotherapy of advanced cancer. N Engl J Med. (1987) 316:898–905. doi: 10.1056/NEJM198704093161502
15. Dranoff G. Cytokines in cancer pathogenesis and cancer therapy. Nat Rev Cancer (2004) 4:11–22. doi: 10.1038/nrc1252
16. Atkins MB, Lotze MT, Dutcher JP, Fisher RI, Weiss G, Margolin K, et al. High-dose recombinant interleukin 2 therapy for patients with metastatic melanoma: analysis of 270 patients treated between 1985 and 1993. J Clin Oncol. (1999) 17:2105–16. doi: 10.1200/JCO.1999.17.7.2105
17. McDermott DF, Atkins MB. Application of IL-2 and other cytokines in renal cancer. Expert Opin Biol Ther. (2004) 4:455–68. doi: 10.1517/14712598.4.4.455
18. Mitra S, Leonard WJ. Biology of IL-2 and its therapeutic modulation: Mechanisms and strategies. J Leukoc Biol. (2018) 103:643–55. doi: 10.1002/JLB.2RI0717-278R
19. Wagner K, Schulz P, Scholz A, Wiedenmann B, Menrad A. The targeted immunocytokine L19-IL2 efficiently inhibits the growth of orthotopic pancreatic cancer. Clin Cancer Res. (2008) 14:4951–60. doi: 10.1158/1078-0432.CCR-08-0157
20. Lode HN, Xiang R, Dreier T, Varki NM, Gillies SD, Reisfeld RA. Natural killer cell-mediated eradication of neuroblastoma metastases to bone marrow by targeted interleukin-2 therapy. Blood (1998) 91:1706–15.
21. Pretto F, Elia G, Castioni N, Neri D. Preclinical evaluation of IL2-based immunocytokines supports their use in combination with dacarbazine, paclitaxel and TNF-based immunotherapy. Cancer Immunol Immunother. (2014) 63:901–10. doi: 10.1007/s00262-014-1562-7
22. Schwager K, Hemmerle T, Aebischer D, Neri D. The immunocytokine L19-IL2 eradicates cancer when used in combination with CTLA-4 blockade or with L19-TNF. J Invest Dermatol. (2013) 133:751–8. doi: 10.1038/jid.2012.376
23. Balza E, Carnemolla B, Mortara L, Castellani P, Soncini D, Accolla RS, et al. Therapy-induced antitumor vaccination in neuroblastomas by the combined targeting of IL-2 and TNFalpha. Int J Cancer (2010) 127:101–10. doi: 10.1002/ijc.25018
24. Menssen HD, Harnack U, Erben U, Neri D, Hirsch B, Durkop H. Antibody-based delivery of tumor necrosis factor (L19-TNFalpha) and interleukin-2 (L19-IL2) to tumor-associated blood vessels has potent immunological and anticancer activity in the syngeneic J558L BALB/c myeloma model. J Cancer Res Clin Oncol. (2018) 144:499–507. doi: 10.1007/s00432-017-2564-6
25. Zegers CM, Rekers NH, Quaden DH, Lieuwes NG, Yaromina A, Germeraad WT, et al. Radiotherapy combined with the immunocytokine L19-IL2 provides long-lasting antitumor effects. Clin Cancer Res. (2015) 21:1151–60. doi: 10.1158/1078-0432.CCR-14-2676
26. Rekers NH, Olivo Pimentel V, Yaromina A, Lieuwes NG, Biemans R, Zegers CML, et al. The immunocytokine L19-IL2: An interplay between radiotherapy and long-lasting systemic anti-tumour immune responses. Oncoimmunology (2018) 7:e1414119. doi: 10.1080/2162402X.2017.1414119
27. Rekers NH, Zegers CM, Germeraad WT, Dubois L, Lambin P. Long-lasting antitumor effects provided by radiotherapy combined with the immunocytokine L19-IL2. Oncoimmunology (2015) 4:e1021541. doi: 10.1080/2162402X.2015.1021541
28. Rekers NH, Zegers CM, Yaromina A, Lieuwes NG, Biemans R, Senden-Gijsbers BL, et al. Combination of radiotherapy with the immunocytokine L19-IL2: Additive effect in a NK cell dependent tumour model. Radiother Oncol. (2015) 116:438–42. doi: 10.1016/j.radonc.2015.06.019
29. Orecchia P, Conte R, Balza E, Pietra G, Mingari MC, Carnemolla B. Targeting Syndecan-1, a molecule implicated in the process of vasculogenic mimicry, enhances the therapeutic efficacy of the L19-IL2 immunocytokine in human melanoma xenografts. Oncotarget (2015) 6:37426–42. doi: 10.18632/oncotarget.6055
30. Borschel N, Schwoppe C, Zerbst C, Angenendt L, Kessler T, Klapper W, et al. Potentiating the activity of rituximab against mantle cell lymphoma in mice by targeting interleukin-2 to the neovasculature. Leukemia Res. (2015) 39:739–48. doi: 10.1016/j.leukres.2015.04.005
31. Schliemann C, Palumbo A, Zuberbuhler K, Villa A, Kaspar M, Trachsel E, et al. Complete eradication of human B-cell lymphoma xenografts using rituximab in combination with the immunocytokine L19-IL2. Blood (2009) 113:2275–83. doi: 10.1182/blood-2008-05-160747
32. Wieckowski S, Hemmerle T, Prince SS, Schlienger BD, Hillinger S, Neri D, et al. Therapeutic efficacy of the F8-IL2 immunocytokine in a metastatic mouse model of lung adenocarcinoma. Lung Cancer (2015) 88:9–15. doi: 10.1016/j.lungcan.2015.01.019
33. Ziffels B, Pretto F, Neri D. Intratumoral administration of IL2- and TNF-based fusion proteins cures cancer without establishing protective immunity. Immunotherapy (2018) 10:177–88. doi: 10.2217/imt-2017-0119
34. Moschetta M, Pretto F, Berndt A, Galler K, Richter P, Bassi A, et al. Paclitaxel enhances therapeutic efficacy of the F8-IL2 immunocytokine to EDA-fibronectin-positive metastatic human melanoma xenografts. Cancer Res. (2012) 72:1814–24. doi: 10.1158/0008-5472.CAN-11-1919
35. Frey K, Schliemann C, Schwager K, Giavazzi R, Johannsen M, Neri D. The immunocytokine F8-IL2 improves the therapeutic performance of sunitinib in a mouse model of renal cell carcinoma. J Urol. (2010) 184:2540–8. doi: 10.1016/j.juro.2010.07.030
36. Gutbrodt KL, Casi G, Neri D. Antibody-based delivery of IL2 and cytotoxics eradicates tumors in immunocompetent mice. Mol Cancer Therapeut. (2014) 13:1772–6. doi: 10.1158/1535-7163.MCT-14-0105
37. Gutbrodt KL, Schliemann C, Giovannoni L, Frey K, Pabst T, Klapper W, et al. Antibody-based delivery of interleukin-2 to neovasculature has potent activity against acute myeloid leukemia. Sci Transl Med. (2013) 5:201ra118. doi: 10.1126/scitranslmed.3006221
38. Pedretti M, Verpelli C, Marlind J, Bertani G, Sala C, Neri D, et al. Combination of temozolomide with immunocytokine F16-IL2 for the treatment of glioblastoma. Br J Cancer (2010) 103:827–36. doi: 10.1038/sj.bjc.6605832
39. Marlind J, Kaspar M, Trachsel E, Sommavilla R, Hindle S, Bacci C, et al. Antibody-mediated delivery of interleukin-2 to the stroma of breast cancer strongly enhances the potency of chemotherapy. Clin Cancer Res. (2008) 14:6515–24. doi: 10.1158/1078-0432.CCR-07-5041
40. Yang RK, Kalogriopoulos NA, Rakhmilevich AL, Ranheim EA, Seo S, Kim K, et al. Intratumoral treatment of smaller mouse neuroblastoma tumors with a recombinant protein consisting of IL-2 linked to the hu14.18 antibody increases intratumoral CD8+ T and NK cells and improves survival. Cancer Immunol Immunother. (2013) 62:1303–13. doi: 10.1007/s00262-013-1430-x
41. Morris ZS, Guy EI, Francis DM, Gressett MM, Werner LR, Carmichael LL, et al. In situ tumor vaccination by combining local radiation and tumor-specific antibody or Immunocytokine Treatments. Cancer Res. (2016) 76:3929–41. doi: 10.1158/0008-5472.CAN-15-2644
42. Neal ZC, Yang JC, Rakhmilevich AL, Buhtoiarov IN, Lum HE, Imboden M, et al. Enhanced activity of hu14.18-IL2 immunocytokine against murine NXS2 neuroblastoma when combined with interleukin 2 therapy. Clin Cancer Res. (2004) 10:4839–47. doi: 10.1158/1078-0432.CCR-03-0799
43. Xu X, Clarke P, Szalai G, Shively JE, Williams LE, Shyr Y, et al. Targeting and therapy of carcinoembryonic antigen-expressing tumors in transgenic mice with an antibody-interleukin 2 fusion protein. Cancer Res. (2000) 60:4475–84.
44. Klein C, Waldhauer I, Nicolini VG, Freimoser-Grundschober A, Nayak T, Vugts DJ, et al. Cergutuzumab amunaleukin (CEA-IL2v), a CEA-targeted IL-2 variant-based immunocytokine for combination cancer immunotherapy: Overcoming limitations of aldesleukin and conventional IL-2-based immunocytokines. Oncoimmunology (2017) 6:e1277306. doi: 10.1080/2162402X.2016.1277306
45. Holden SA, Lan Y, Pardo AM, Wesolowski JS, Gillies SD. Augmentation of antitumor activity of an antibody-interleukin 2 immunocytokine with chemotherapeutic agents. Clin Cancer Res. (2001) 7:2862–9.
46. Gillies SD, Lan Y, Williams S, Carr F, Forman S, Raubitschek A, et al. An anti-CD20-IL-2 immunocytokine is highly efficacious in a SCID mouse model of established human B lymphoma. Blood (2005) 105:3972–8. doi: 10.1182/blood-2004-09-3533
47. Dougan M, Ingram JR, Jeong HJ, Mosaheb MM, Bruck PT, Ali L, et al. Targeting cytokine therapy to the pancreatic tumor microenvironment Using PD-L1-Specific VHHs. Cancer Immunol Res. (2018) 6:389–401. doi: 10.1158/2326-6066.CIR-17-0495
48. Jahn T, Zuther M, Friedrichs B, Heuser C, Guhlke S, Abken H, et al. An IL12-IL2-antibody fusion protein targeting Hodgkin's lymphoma cells potentiates activation of NK and T cells for an anti-tumor attack. PLoS ONE (2012) 7:e44482. doi: 10.1371/journal.pone.0044482
49. Weide B, Eigentler TK, Pflugfelder A, Zelba H, Martens A, Pawelec G, et al. Intralesional treatment of stage III metastatic melanoma patients with L19-IL2 results in sustained clinical and systemic immunologic responses. Cancer Immunol Res. (2014) 2:668–78. doi: 10.1158/2326-6066.CIR-13-0206
50. Johannsen M, Spitaleri G, Curigliano G, Roigas J, Weikert S, Kempkensteffen C, et al. The tumour-targeting human L19-IL2 immunocytokine: preclinical safety studies, phase I clinical trial in patients with solid tumours and expansion into patients with advanced renal cell carcinoma. Eur J Cancer (2010) 46:2926–35. doi: 10.1016/j.ejca.2010.07.033
51. Eigentler TK, Weide B, de Braud F, Spitaleri G, Romanini A, Pflugfelder A, et al. A dose-escalation and signal-generating study of the immunocytokine L19-IL2 in combination with dacarbazine for the therapy of patients with metastatic melanoma. Clin Cancer Res. (2011) 17:7732–42. doi: 10.1158/1078-0432.CCR-11-1203
52. Schliemann C, Gutbrodt KL, Kerkhoff A, Pohlen M, Wiebe S, Silling G, et al. Targeting interleukin-2 to the bone marrow stroma for therapy of acute myeloid leukemia relapsing after allogeneic hematopoietic stem cell transplantation. Cancer Immunol Res. (2015) 3:547–56. doi: 10.1158/2326-6066.CIR-14-0179
53. Albertini MR, Hank JA, Gadbaw B, Kostlevy J, Haldeman J, Schalch H, et al. Phase II trial of hu14.18-IL2 for patients with metastatic melanoma. Cancer Immunol Immunother. (2012) 61:2261–71. doi: 10.1007/s00262-012-1286-5
54. Shusterman S, London WB, Gillies SD, Hank JA, Voss SD, Seeger RC, et al. Antitumor activity of hu14.18-IL2 in patients with relapsed/refractory neuroblastoma: a Children's Oncology Group (COG) phase II study. J Clin Oncol. (2010) 28:4969–75. doi: 10.1200/JCO.2009.27.8861
55. Ribas A, Kirkwood JM, Atkins MB, Whiteside TL, Gooding W, Kovar A, et al. Phase I/II open-label study of the biologic effects of the interleukin-2 immunocytokine EMD 273063 (hu14.18-IL2) in patients with metastatic malignant melanoma. J Transl Med. (2009) 7:68. doi: 10.1186/1479-5876-7-68
56. Gladkov O, Ramlau R, Serwatowski P, Milanowski J, Tomeczko J, Komarnitsky PB, et al. Cyclophosphamide and tucotuzumab (huKS-IL2) following first-line chemotherapy in responding patients with extensive-disease small-cell lung cancer. Anticancer Drugs (2015) 26:1061–8. doi: 10.1097/CAD.0000000000000281
57. Danielli R, Patuzzo R, Ruffini PA, Maurichi A, Giovannoni L, Elia G, et al. Armed antibodies for cancer treatment: a promising tool in a changing era. Cancer Immunol Immunother. (2015) 64:113–21. doi: 10.1007/s00262-014-1621-0
58. Danielli R, Patuzzo R, Di Giacomo AM, Gallino G, Maurichi A, Di Florio A, et al. Intralesional administration of L19-IL2/L19-TNF in stage III or stage IVM1a melanoma patients: results of a phase II study. Cancer Immunol Immunother. (2015) 64:999–1009. doi: 10.1007/s00262-015-1704-6
59. Gillies SD, Lan Y, Hettmann T, Brunkhorst B, Sun Y, Mueller SO, et al. A low-toxicity IL-2-based immunocytokine retains antitumor activity despite its high degree of IL-2 receptor selectivity. Clin Cancer Res. (2011) 17:3673–85. doi: 10.1158/1078-0432.CCR-10-2921
60. van den Heuvel MM, Verheij M, Boshuizen R, Belderbos J, Dingemans AM, De Ruysscher D, et al. NHS-IL2 combined with radiotherapy: preclinical rationale and phase Ib trial results in metastatic non-small cell lung cancer following first-line chemotherapy. J Transl Med. (2015) 13:32. doi: 10.1186/s12967-015-0397-0
61. Raave R, van Kuppevelt TH, Daamen WF. Chemotherapeutic drug delivery by tumoral extracellular matrix targeting. J Control Release (2018) 274:1–8. doi: 10.1016/j.jconrel.2018.01.029
62. Kaspar M, Zardi L, Neri D. Fibronectin as target for tumor therapy. Int J Cancer (2006) 118:1331–9. doi: 10.1002/ijc.21677
63. Cazzamalli S, Ziffels B, Widmayer F, Murer P, Pellegrini G, Pretto F, et al. Enhanced therapeutic activity of non-internalizing small molecule-drug conjugates targeting carbonic Anhydrase IX in combination with targeted interleukin-2. Clin Cancer Res. (2018) 24:3656–67. doi: 10.1158/1078-0432.CCR-17-3457
64. Weide B, Neri D, Elia G. Intralesional treatment of metastatic melanoma: a review of therapeutic options. Cancer Immunol Immunother. (2017) 66:647–56. doi: 10.1007/s00262-016-1952-0
65. Purushothaman A, Uyama T, Kobayashi F, Yamada S, Sugahara K, Rapraeger AC, et al. Heparanase-enhanced shedding of syndecan-1 by myeloma cells promotes endothelial invasion and angiogenesis. Blood (2010) 115:2449–57. doi: 10.1182/blood-2009-07-234757
66. Gharbaran R. Advances in the molecular functions of syndecan-1 (SDC1/CD138) in the pathogenesis of malignancies. Crit Rev Oncol Hematol. (2015) 94:1–17. doi: 10.1016/j.critrevonc.2014.12.003
67. Orecchia P, Conte R, Balza E, Petretto A, Mauri P, Mingari MC, et al. A novel human anti-syndecan-1 antibody inhibits vascular maturation and tumour growth in melanoma. Eur J Cancer (2013) 49:2022–33. doi: 10.1016/j.ejca.2012.12.019
68. Arenas-Ramirez N, Woytschak J, Boyman O. Interleukin-2: Biology, Design and Application. Trends Immunol. (2015) 36:763–77. doi: 10.1016/j.it.2015.10.003
69. Ghasemi R, Lazear E, Wang X, Arefanian S, Zheleznyak A, Carreno BM, et al. Selective targeting of IL-2 to NKG2D bearing cells for improved immunotherapy. Nat Commun. (2016) 7:12878. doi: 10.1038/ncomms12878
71. Abbas AK, Trotta E, D RS, Marson A, Bluestone JA. Revisiting IL-2: Biology and therapeutic prospects. Sci Immunol. (2018) 3:eaat1482. doi: 10.1126/sciimmunol.aat1482
72. Tran E, Robbins PF, Rosenberg SA. ‘Final common pathway' of human cancer immunotherapy: targeting random somatic mutations. Nat Immunol. (2017) 18:255–62. doi: 10.1038/ni.3682
73. Yang JC, Rosenberg SA. Adoptive T-Cell therapy for cancer. Adv Immunol. (2016) 130:279–94. doi: 10.1016/bs.ai.2015.12.006
74. Charych D, Khalili S, Dixit V, Kirk P, Chang T, Langowski J, et al. Modeling the receptor pharmacology, pharmacokinetics, and pharmacodynamics of NKTR-214, a kinetically-controlled interleukin-2 (IL2) receptor agonist for cancer immunotherapy. PLoS ONE (2017) 12:e0179431. doi: 10.1371/journal.pone.0179431
75. Charych DH, Hoch U, Langowski JL, Lee SR, Addepalli MK, Kirk PB, et al. NKTR-214, an engineered cytokine with Biased IL2 Receptor Binding, increased tumor exposure, and marked efficacy in mouse tumor models. Clin Cancer Res. (2016) 22:680–90. doi: 10.1158/1078-0432.CCR-15-1631
76. Waldmann TA. The shared and contrasting roles of IL2 and IL15 in the life and death of normal and neoplastic lymphocytes: implications for cancer therapy. Cancer Immunol Res. (2015) 3:219–27. doi: 10.1158/2326-6066.CIR-15-0009
77. Johnson EE, Lum HD, Rakhmilevich AL, Schmidt BE, Furlong M, Buhtoiarov IN, et al. Intratumoral immunocytokine treatment results in enhanced antitumor effects. Cancer Immunol Immunother. (2008) 57:1891–902. doi: 10.1007/s00262-008-0519-0
78. Yang RK, Kalogriopoulos NA, Rakhmilevich AL, Ranheim EA, Seo S, Kim K, et al. Intratumoral hu14.18-IL-2 (IC) induces local and systemic antitumor effects that involve both activated T and NK cells as well as enhanced IC retention. J Immunol. (2012) 189:2656–64. doi: 10.4049/jimmunol.1200934
79. Oth T, Habets T, Germeraad WTV, Zonneveld MI, Bos GMJ, Vanderlocht J. Pathogen recognition by NK cells amplifies the pro-inflammatory cytokine production of monocyte-derived DC via IFN-gamma. BMC Immunol. (2018) 19:8. doi: 10.1186/s12865-018-0247-y
80. Trinchieri G. Interleukin-12: a proinflammatory cytokine with immunoregulatory functions that bridge innate resistance and antigen-specific adaptive immunity. Annu Rev Immunol. (1995) 13:251–76. doi: 10.1146/annurev.iy.13.040195.001343
81. Gerosa F, Baldani-Guerra B, Nisii C, Marchesini V, Carra G, Trinchieri G. Reciprocal activating interaction between natural killer cells and dendritic cells. J Exp Med. (2002) 195:327–33. doi: 10.1084/jem.20010938
82. Delgado DC, Hank JA, Kolesar J, Lorentzen D, Gan J, Seo S, et al. Genotypes of NK cell KIR receptors, their ligands, and Fcgamma receptors in the response of neuroblastoma patients to Hu14.18-IL2 immunotherapy. Cancer Res. (2010) 70:9554–61. doi: 10.1158/0008-5472.CAN-10-2211
83. Ruggeri L, Capanni M, Casucci M, Volpi I, Tosti A, Perruccio K, et al. Role of natural killer cell alloreactivity in HLA-mismatched hematopoietic stem cell transplantation. Blood (1999) 94:333–9.
84. Leung W, Handgretinger R, Iyengar R, Turner V, Holladay MS, Hale GA. Inhibitory KIR-HLA receptor-ligand mismatch in autologous haematopoietic stem cell transplantation for solid tumour and lymphoma. Br J Cancer (2007) 97:539–42. doi: 10.1038/sj.bjc.6603913
85. Venstrom JM, Zheng J, Noor N, Danis KE, Yeh AW, Cheung IY, et al. KIR and HLA genotypes are associated with disease progression and survival following autologous hematopoietic stem cell transplantation for high-risk neuroblastoma. Clin Cancer Res. (2009) 15:7330–4. doi: 10.1158/1078-0432.CCR-09-1720
86. Balza E, Mortara L, Sassi F, Monteghirfo S, Carnemolla B, Castellani P, et al. Targeted delivery of tumor necrosis factor-alpha to tumor vessels induces a therapeutic T cell-mediated immune response that protects the host against syngeneic tumors of different histologic origin. Clin Cancer Res. (2006) 12:2575–82. doi: 10.1158/1078-0432.CCR-05-2448
87. Mortara L, Balza E, Sassi F, Castellani P, Carnemolla B, De Lerma Barbaro A, et al. Therapy-induced antitumor vaccination by targeting tumor necrosis factor alpha to tumor vessels in combination with melphalan. Eur J Immunol. (2007) 37:3381–92. doi: 10.1002/eji.200737450
88. Balza E, Zanellato S, Poggi A, Reverberi D, Rubartelli A, Mortara L. The therapeutic T-cell response induced by tumor delivery of TNF and melphalan is dependent on early triggering of natural killer and dendritic cells. Eur J Immunol. (2017) 47:743–53. doi: 10.1002/eji.201646544
89. Boyman O, Surh CD, Sprent J. Potential use of IL-2/anti-IL-2 antibody immune complexes for the treatment of cancer and autoimmune disease. Expert Opin Biol Ther. (2006) 6:1323–31. doi: 10.1517/14712598.6.12.1323
90. Krieg C, Letourneau S, Pantaleo G, Boyman O. Improved IL-2 immunotherapy by selective stimulation of IL-2 receptors on lymphocytes and endothelial cells. Proc Natl Acad Sci USA. (2010) 107:11906–11. doi: 10.1073/pnas.1002569107
91. Wylezinski LS, Hawiger J. Interleukin 2 activates brain microvascular endothelial cells resulting in destabilization of adherens junctions. J Biol Chem. (2016) 291:22913–23. doi: 10.1074/jbc.M116.729038
92. Kim DW, Zloza A, Broucek J, Schenkel JM, Ruby C, Samaha G, et al. Interleukin-2 alters distribution of CD144 (VE-cadherin) in endothelial cells. J Transl Med. (2014) 12:113. doi: 10.1186/1479-5876-12-113
Keywords: anti-tumor therapy, IL-2, targeting immunotherapy, chemotherapy, T-cell responses, NK cells
Citation: Mortara L, Balza E, Bruno A, Poggi A, Orecchia P and Carnemolla B (2018) Anti-cancer Therapies Employing IL-2 Cytokine Tumor Targeting: Contribution of Innate, Adaptive and Immunosuppressive Cells in the Anti-tumor Efficacy. Front. Immunol. 9:2905. doi: 10.3389/fimmu.2018.02905
Received: 13 September 2018; Accepted: 27 November 2018;
Published: 18 December 2018.
Edited by:
Patrik Andersson, Harvard Medical School, United StatesReviewed by:
William L. Redmond, Earle A. Chiles Research Institute, United StatesOnur Boyman, University of Zurich, Switzerland
Copyright © 2018 Mortara, Balza, Bruno, Poggi, Orecchia and Carnemolla. This is an open-access article distributed under the terms of the Creative Commons Attribution License (CC BY). The use, distribution or reproduction in other forums is permitted, provided the original author(s) and the copyright owner(s) are credited and that the original publication in this journal is cited, in accordance with accepted academic practice. No use, distribution or reproduction is permitted which does not comply with these terms.
*Correspondence: Lorenzo Mortara, bG9yZW56by5tb3J0YXJhQHVuaW5zdWJyaWEuaXQ=