- 1US Food and Drug Administration, Division of Bacterial Allergenic and Parasitic Diseases, Center for Biologics Evaluation and Research, Silver Spring, MD, United States
- 2Emory Vaccine Center, Yerkes National Primate Research Center, Emory University, Atlanta, GA, United States
- 3Division of Infectious Diseases, Department of Medicine, Emory University School of Medicine, Atlanta, GA, United States
The delay in parasite-specific B cell development leaves people in malaria endemic areas vulnerable to repeated Plasmodium infections. Here, we investigated the role of transmembrane activator and calcium-modulator and cyclophilin ligand interactor (TACI), a molecule involved in the generation of antigen-specific antibody secreting cells, in host response to non-lethal Plasmodium yoelii infection. We found that TACI deficiency not only resulted in higher peak parasitemia levels in P. yoelii challenged mice, but also led to a delay in parasite clearance and anti-P. yoelii Merozoite Surface Protein 1(C-terminal 19-kDa fragment [rMSP-119]) protein and anti-rMSP-119 and anti-P. yoelii IgG antibody development. There was also a delay in the generation of splenic high affinity antibody secreting cells that recognize rMSP-119 protein as compared to wild-type mice. Interestingly, coinciding with the delay in parasite clearance there was a delay in the resolution of T follicular helper (TFH) cell and germinal center (GC) B cell responses in TACI -/- mice. The persistence of TFH and GC B cells is likely a result of enhanced interaction between TFH and GC B cells because inducible costimulator ligand (ICOSL) expression was significantly higher on TACI -/- GC B cells than wild-type cells. The difference in the kinetics of GC reaction appeared to also impact the emergence of plasma cells (PC) because there was a delay in the generation of TACI -/- mice PC. Nevertheless, following the recovery from P. yoelii infection, TACI -/- and wild-type mice were both protected from a rechallenge infection. Establishment of protective B cell response was responsible for the resolution of parasitemia because B cells purified from recovered TACI -/- or wild-type mice were equally protective when introduced to naïve wild-type mice prior to P. yoelii challenge. Thus, despite the increased susceptibility of TACI -/- mice to P. yoelii infection and a delay in the development of protective antibody levels, TACI -/- mice are able to clear the infection and resist rechallenge infection.
Introduction
Children under the age of 5 years (1), especially those who are less than 1 year of age, are highly vulnerable to Plasmodium infections (2). While antibodies play a critical role in controlling parasitemia burden and illness (3), protective humoral immunity to malaria occurs only after repeated exposure to parasites (4). Shortcomings of immunological response that can control Plasmodium parasites have been attributed to the diversity of the malarial antigens, the rapid disappearance of anti-malarial antibodies and an insufficient long-lived plasma cell (PC) pool (4). Despite the recognition of these B cell insufficiencies, molecular and cellular events that prevent the host's ability to mount optimal B cell responses are poorly understood.
In this study, we examined the role of transmembrane activator and calcium modulator and cyclophilin ligand interactor (TACI) in host resistance to malaria infection. TACI is a receptor for B cell activating factor belonging to TNF family (BAFF) and a proliferation-inducing ligand (APRIL) (5). Together with two other receptors, BAFF receptor (BAFF-R) and B cell maturation antigen (BCMA), these molecules are crucial in maintaining B cell homeostasis, and TACI is involved in immunoglobulin isotype switching and antibody secretion, PC maintenance and macrophage polarization (6–10). TACI is also important in controlling T follicular helper (TFH) cell responses as immunization or infection of TACI deficient mouse results with augmented TFH development (11, 12). However, while immunization of TACI -/- mice with a T cell dependent antigen elicited reduced antibody responses and short lived PC as compared to wild-type mice (11), TACI -/- mice controlled Citrobacter rodentium infection better than the wild-type mice most likely because of an increase in antibody secreting cells and development of high affinity antibodies directed against C. rodentium (12). Measurement of elevated circulating BAFF and increased BAFF-R on B cells in humans experimentally challenged with Plasmodium falciparum suggest an involvement of these molecules in host response to malaria (13, 14). Whether TACI participates in BAFF-induced host responses during malaria infection has not been explored.
We found that Plasmodium yoelii challenged TACI -/- mice manifested significantly higher levels of parasitemia than wild-type mice, which persisted longer. The increased susceptibility of TACI -/- mice appeared to be the result of a delay in anti-parasite antibody development. Analysis of TFH cell development and germinal center (GC) formation suggested that altered kinetics of GC reaction may be responsible for the delay in the PC development and antibody production in infected TACI -/- mice. Nevertheless, despite late parasite clearance, not only were the TACI -/- mice protected from a second P. yoelii challenge, but also, B cells from TACI -/- mice were sufficient to prevent P. yoelii infection when transferred to naïve wild-type mice. In the absence of TACI, host control of parasitemia is delayed compared to wild-type mice. However, once the parasitemia is cleared, B cell mediated immunity renders TACI -/- mice resistant to a second infection.
Materials and Methods
Mice
C57BL/6 mice (6–8 weeks old) were purchased from the Jackson Laboratories (Bar Harbor, ME). TACI -/- mice on a C57BL/6 background were described previously (15, 16). The experimental procedures were approved by the Institutional Animal Care and Use Committee of the Center for Biologics Evaluation and Research (Protocol-2008-08).
Parasites
Nonlethal P. yoelii strain 17XNL was used in mouse challenge experiments (17). Frozen stocks of P. yoelii 17XNL-infected erythrocytes were intraperitoneally (i.p.) injected to C57BL/6 mice to generate donor mice. When 8 to 10% parasitemia was detected, blood was collected by cardiac puncture, diluted in PBS and used to i.p. infect experimental animals with 1 × 106 P. yoelii parasites in 200 μl of PBS. The percent parasitemia [parasitized red blood cells (RBCs)/total RBCsx100] after infection was determined by Giemsa-stained thin blood smears.
Anti-Plasmodium Antibody Detection
Serum samples were pooled or not from 3 C57BL/6 and 3 TACI -/- mice per time point at 8, 16, 22, 28, and 71 days post P. yoelii infection. Serum antibody levels against an extended version of the P. yoelii Merozoite Surface Protein 1(C-terminal 19-kDa fragment [rMSP-119]) (18) and whole P. yoelii 17XNL extract were measured by ELISA. ELISA plates were coated with 70 ng/well of rMSP-119 or sonicated P. yoelii infected RBCs in coating buffer (15 mM Na2CO3 and 35 mM NaHCO3). After washing with PBS/0.05%Tween-20, plates were blocked with 5% milk/PBS. Next, 100 μl of 1:50 to 1:51200 titrated sera were added. After 2 h, plates were incubated with 1:3500 diluted goat anti-mouse IgG-HRP antibody (Southern Biotechnology Associates, Birmingham, AL). Plates were read on a VERSA max microplate reader after adding ABTS substrate (Molecular Devices, Sunnydale, CA).
Adoptive Transfer of B Cells
Splenic B cells of mice that had cleared P. yoelii infection 4 months earlier were isolated by using B220 magnetic beads (Miltenyi Biotec, San Diego, CA). The purity of B220+CD19+ B cells was > 95%. Plasmodium yoelii immune B-cells were then adoptively transferred by intravenous (i.v.) injection of 3 × 107 immune cells per mouse. Two hours after the B cell transfer, mice were injected with P. yoelii infected RBCs. The percent parasitemia (parasitized RBCs/total RBCs × 100) after infection was determined by examining Giemsa-stained thin blood smears.
Flow Cytometry
Single cell suspensions prepared from spleen and dead cells were excluded after staining with fixable efluor 780 (Affymatrix, Santa Clare, CA). For TFH analysis, splenocytes were stained in 2%FBS/0.5 EDTA/PBS buffer with anti-CD4-PercpCy5.5 (clone GK1.55, Affymatrix), PD-1-PE (clone 29F.1A12, BioLegend, San Diego, CA), CD44-Alexa Fluor 700 (clone IM7, BD Biosciences, San Jose, CA), CXCR5-biotin (clone 2G8, BD Biosciences), and ICOSL-biotin (clone HK5.3, Biolegend) antibodies. Biotin was detected with streptavidin-BV421 (BioLegend). For GC B cell and PC analysis, B220-BV605 (clone RA3-6B2, BioLegend), FAS-APC (clone J02, BioLegend), T/B cell activating antigen-FITC (clone GL-7, BioLegend), B220-APC (clone RA3-6B2, BioLegend), CD138-PE (clone 281-2 from BioLegend), and IgD-BV605 (clone 11-26c.2a, BD Biosciences) antibodies were used. For intracellular staining, samples were fixed with the Foxp3 Fix/Perm buffer set, following the manufacturer's instructions (eBioscience). Samples were then intracellularly stained with α-Foxp3 (BioLegend, 150D, 1:100) antibody. 'Fluorescence minus one' (FMO) controls were used to gate the cells for each antibody. Data were acquired on LSRII flow cytometer (BD Biosciences) and analyzed using FlowJo software v10 (FlowJo, Ashland, OR).
Immunofluorescence Microscopy
OCT-embedded spleens were snap-frozen by floating on liquid nitrogen-cooled isopentane. Frozen tissues were cut into 10 μm sections using a cryostat and mounted on poly-lysine-coated slides. Sections were allowed to air dry at room temperature for 10 min and fixed with pre-cooled Acetone and Methanol (1:1 vol) for 10 min, followed by washing three times with PBS containing 0.5% Tween-20. Sections were first blocked with 5% goat serum for 1 h, and then stained overnight at 4°C with anti-B220 (Rat IgG, 1:50, eBioscience), anti-GL-7 (Rat IgM, 1:50, BioLegend) antibodies. Following three washing steps with PBS containing 0.5% Tween-20, sections were stained for 1 h at room temperature with the following secondary antibodies: goat anti-rat IgG-AF488, goat anti-rat IgM-AF647 (1:200, Invitrogen, Carlsbad, CA). Samples were imaged on Leica DMI6000 Inverted Light Microscope. The tile scan function was used to stitch individual 10x images together.
Elispot Assay
rMSP-119-specific antibody secreting cells (ASC) were quantified by direct ex vivo ELISPOT assay. Ninety-six-well nitrocellulose plates (Multiscreen-HA; Millipore, Bedford, MA) were coated overnight at 4°C with rMSP-119 at 10 μg/ml concentration and incubated in 15 μM 2-mercaptoethanol/10%FBS/RPMI for 1 h. Splenocytes or bone marrow cells (106 cells/well/100 μl) were incubated at 37°C, 5% CO2 for 5 h in RPMI medium. Plates were washed, and bound rMSP-119-specific IgG-producing cells were stained with goat anti-mouse IgG-HRP (Bethyl Laboratories, Montgomery, TX) antibody. HRP was developed with 3-aminoethyl carbazole, AEC peroxidase substrate kit (Vector Laboratories, Burlingame, CA). Spots were counted using an AID ELISPOT analyzer (Autoimmun Diagnostika, Germany).
Antibody Avidity Measurement
Serum samples collected from 5 C57BL/6 mice and 5 TACI -/- mice 71 days after P. yoelii infection were pooled. The avidity of the antibodies was evaluated using guanidine hydrochloride (GuHCl) as a dissociative agent (19). The ELISA plates were coated, blocked and serum titrations were prepared as described in ELISA method. Triplicate serum samples were added to each well. After 2 h of incubation and washing steps, “avidity samples” were incubated with 100 μl of 0.1 M GuHCl (Sigma, Darmstadt, Germany) while triplicate “control samples” were incubated in washing buffer. After incubation for 10 min and 5 washes, wells were exposed to goat anti-mouse IgG-HRP antibody (Southern Biotechnology Associates) for 1 h. Following washing steps, ABTS substrate was added and the plates were read on a VERSA max microplate reader (Molecular Devices). Antibody avidity was calculated using the method described by Perciani et al. (20). Optical densities from each titration were graphed using GraphPad Prism software (La Jolla, CA) and the area under the curve (AUC) was measured for both the GuHCl and control-treated samples for each serum pool. The formula (AUC of guanidine treated samples)/(AUC of control-treated samples) was used to calculate the avidity index ratio.
Statistical Analyses
The parasitemia data were evaluated using unpaired Student's t-test. Unpaired Student's t-test was also used for the comparison of cell numbers measured in flow cytometry and ELISPOT analysis. Values of p < 0.05 were considered statistically significant. The avidity ELISA data was evaluated using AUC with a baseline value of 0, and the means compared using a Mann-Whitney non-parametric test.
Results
Elevated Parasitemia and Delayed Clearance of P. yoelii in TACI -/- Mice
To assess whether TACI participates in resistance to Plasmodium infection, we challenged C57BL/6 and TACI -/- mice with P. yoelii. Parasitemia levels were monitored by counting the percentage of P. yoelii infected RBCs until the resolution of parasitemia. The wild-type mice developed a typical self-limiting course of parasitemias that characterize infections with the non-lethal P. yoelii strain 17XNL (21), with peak parasitemia on day 11 post-infection, and parasitemia resolution by day 21 post-infection (Figure 1A). In contrast, parasitemia levels were significantly higher in TACI -/- mice starting at day 11 post-P. yoelii infection when compared to the wild-type mice. Parasitemias continued to increase in TACI -/- mice after day 11 post-infection, until reaching a peak at day 21 post-infection. In contrast, C57BL/6 mice had 0% parasitemia at day 21 post-infection. Thus, in the absence of TACI, malaria parasite load was increased, and parasitemia resolution was markedly delayed.
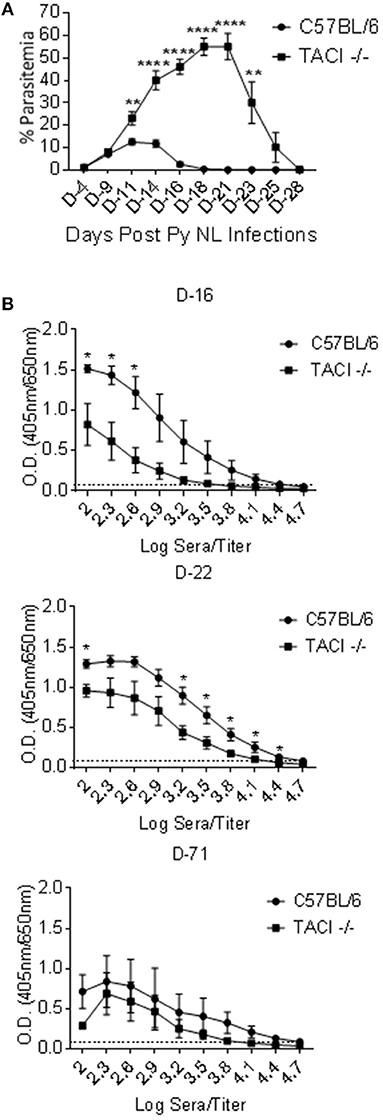
Figure 1. P. yoelii infection clearance and antibody development are delayed in TACI -/- mice. (A) TACI -/- and C57BL/6 mice were infected (i.p.) with 1 × 106 P. yoelii (Py 17XNL) parasites. Parasitemia levels were evaluated by blood smears at indicated days post challenge until parasitemia clearance. Results are expressed as mean % parasitemia ± SEM for 10 mice per group. One representative experiment out of three with similar infection outcome is shown. (B) TACI -/- and C57BL/6 mice (9 per group) were infected (i.p.) with 1 × 106 P. yoelii parasites. Individual serum was collected from three mice of each group (per time point) on days 16, 22, and 71 post parasite challenge and were tested in ELISA for anti-rMSP-119 IgG antibody measurement (Please see Supplementary Figure 1A for days 8 and 28 ELISA results). Average OD values ± SEM from three samples on each time point were plotted. Dotted line indicates ELISA negative control. Unpaired student's t-test was used for statistical evaluation. *indicates p < 0.05, ** < 0.01; ****p < 0.0001. Experiment was performed once.
Anti-Plasmodium Antibody Responses are Delayed in TACI -/- Mice
Antibodies to Plasmodium are involved in reducing parasite load and clearance in both mice and humans (3, 22). To assess whether antibody development was altered in parallel to the delay of parasitemia resolution in TACI -/- mice, serum antibodies against rMSP-119, a surface exposed fraction of P. yoelii MSP-1 protein that is a target of protective antibodies (18), were measured over the course of the infection. Both strains had low levels of anti-rMSP-119 IgG antibodies on day 8 post-infection (Supplementary Figure 1A). Although anti-rMSP-119 IgG levels increased thereafter in both strains, wild-type mice levels were markedly higher on days 16 and 22 as compared to those of TACI -/- mice (Figure 1B). TACI -/- mice antibody levels reached comparable levels to those elicited in the wild-type mice only on day 28 (Supplementary Figure 1A) and remained at levels comparable to those of wild-type mice at 71 days post infection (Figure 1B). Similar trends in antibody responses were observed when sera were tested for IgG antibodies against P yoelii parasites (Supplementary Figure 1B). Since the peak anti-P. yoelii antibody development coincided with the parasitemia resolution time-point in TACI -/- mice, this delayed antibody response is likely responsible for the magnitude and the delay in the resolution of parasitemia.
TACI Deficiency Extends Formation and Resolution Kinetics of the GC Response
TFH cells provide soluble and contact-dependent signals to B cells for somatic hypermutation and affinity maturation of antibodies in the GC (23). Following TFH help, activated B cells leave the GC as plasmablasts and memory B cells (24). Moreover, the formation of TFH cells and their communication with GC B cells is required for the development of parasite-specific B cells during Plasmodium infection (11, 25). The discovery of delayed kinetics of anti-malarial antibody development in P. yoelii infected TACI -/- mice led us to investigate the TFH development and GC formation in infected mice spleens. In wild-type mice, the number of CD4+CD44+CXCR5highPD-1high TFH cells (Supplementary Figure 2A) were highest at day 10 post-infection, which steadily declined on days 15 and 23 (Figures 2A,B). The percentage of TFH cells in wild-type mice followed kinetics similar to the number of TFH cells (Supplementary Figure 2B). Compared to TFH cells, wild-type mice B220+GL7+FAS+ (Supplementary Figure 2A) GC B cell-percentages and numbers peaked slightly later (day 15) (Figures 2C,D). In TACI -/- mice, day 10 TFH cell-numbers were comparable to those of wild-type mice on the same day (Figures 3A,B). However, instead of declining thereafter as in wild-type mice, TACI -/- TFH cell-numbers persisted until day 15. The kinetics of TACI -/- mice TFH cell-percentage was similar to the changes in TFH cell numbers (Supplementary Figure 2B). This delayed resolution of TFH cells coincided with a significantly more GC B cell numbers in TACI -/- mice than wild-type mice on day 15 (Figures 2C,D). Both mouse strains had lower numbers of GC B cells on day 23 than on day 15, but TACI -/- mice cell numbers were still significantly more than those of wild-type mice. Similar to the number of GC measured on day 15, the percentage of GC B cells on the same day were significantly higher in TACI -/- mice than wild-type mice, but the difference between the two mouse strains vanished on day 23 (Supplementary Figure 2C).
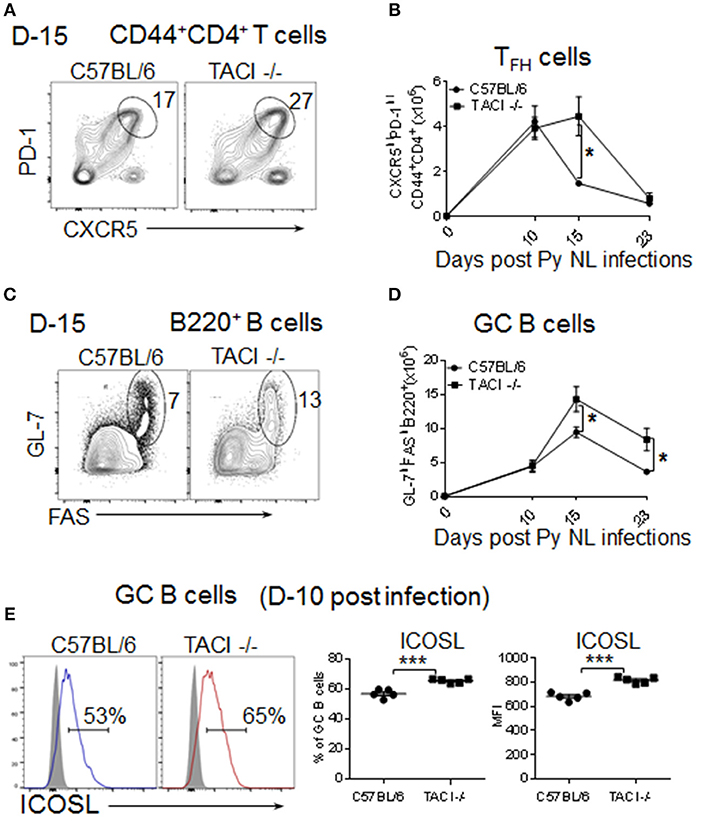
Figure 2. TACI deficiency extends formation and resolution kinetics of TFH and GC response. TACI -/- and C57BL/6 mice were infected (i.p.) with 1 × 106 P. yoelii (Py 17XNL strain) parasites. (A) Representative dot plots depict the percentage of splenic PD-1highCXCR5high (TFH) cell on CD44+CD4+ pre-gated T cells at day 15 post-infection. (B) Formation and resolution kinetics of TFH cells presented as number of TFH cells per spleen. (C) Representative dot plots depict the percentage of and GL-7highFAShigh (GC B cells) on B220+ pre-gated B cells at day 15 post-infection. (D) Formation and resolution ki“netics of GC B cells presented as number of GC B cells per spleen. Total splenic B cell (E) Day 10 post-infection ICOSL expression levels were measured on B220+GL-7highFAShigh gated splenic GC B cells. Representative histograms as well as frequencies of ICOSL expressing cells and ICOSL MFI for each mouse strain are shown. Unpaired Student's t-test was used for statistical evaluation. Results are expressed as mean ± SEM (n = 5) from one representative experiment out of three with similar results. *p < 0.05 and ***p < 0.001 for TACI -/- vs. C57BL/6 comparison. GC, germinal center.
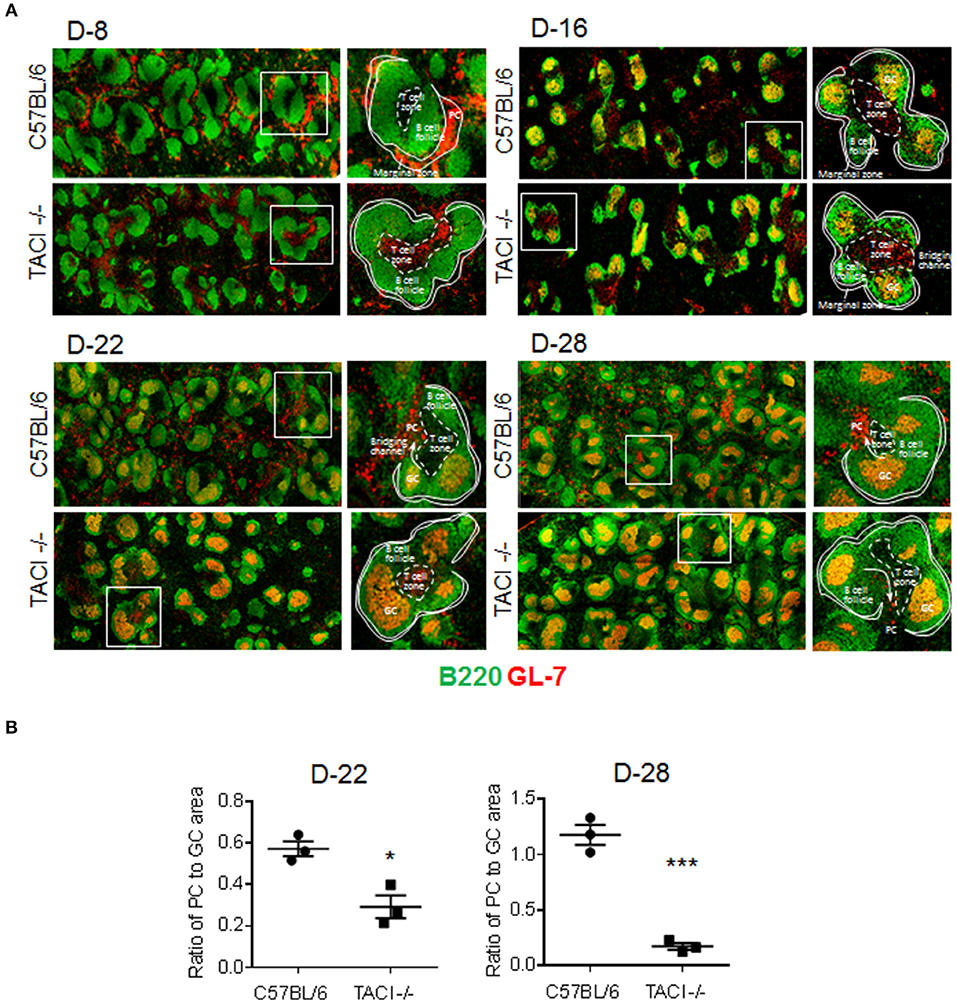
Figure 3. PC output from GC is limited in TACI -/- mice. TACI -/- and C57BL/6 mice were infected (i.p.) with 1 × 106 P. yoelii (Py 17XNL) parasites. Splenic GC and PC were examined by microscopy on indicated days after infection. (A) Histologic sections of spleens were stained with anti-B220 (green) and anti-GL-7 (red) antibodies to visualize B-cell follicles (green), GC (B220+GL-7+, yellow), and PC (B220lowGL-7+, orange). T-cell independent PC are located in the marginal zone and T-cell dependent PC in red pulp of the spleen, adjacent to the “Bridging channels.” T cell zones were defined as central location where tightly packed follicles are distributed around and the activated T cells (B220−GL-7+, red) are located. Arrows indicate the plasmablast pathway to extrafollicular areas through the “Bridging channels.” (B) PC output was calculated by ratio of PC foci to GCs in half spleen. Each dot represents one mouse. Magnification is 10x. *p < 0.05 and ***p < 0.001 for TACI -/- vs. C57BL/6 comparison. Experiment was performed once.
Although the CD4+CD44+CXCR5highPD-1high cells were initially designated as TFH cells that promote B cell responses in the GC (23), more recent studies showed that a Foxp3-expressing regulatory subset of TFH cells (TFR) is inhibitory to the GC reaction and B cell responses (26, 27). The function of these cells can be assessed by analyzing the ratio of TFR to TFH (TFR:TFH) cells, which predicts the influence of TFR cells on antibody responses (28). Since we measured lower anti-parasite antibody levels despite the persistent of TFH and GC in TACI -/- mice, higher TFR:TFH ratio could be responsible for the ablated antibody responses. However, this possibility was ruled out because we measured comparable TFR:TFH ratios between the mouse strains at 15 day time point (Supplementary Figures 3A–C).
Interaction of ICOSL on B cells with ICOS on pre-TFH cells is essential for early stage TFH cell formation (29). In nitrophenyl-chicken gamma globulin immunized TACI -/- mice, the elevated ICOSL expression has been implicated in expanded TFH and GC formation (11, 12). Reminiscent of immunized and C. rodentium infected TACI -/- mice, we also detected significantly higher ICOSL expression on TACI -/- B cells as compared to wild-type mice at 10 days post-infection (Figure 2E). Thus, the elevated ICOSL on GC B cells likely contributes to the persistent TFH and GC B cell interaction in P. yoelii infected TACI -/- mice.
The Emergence of PC Is Delayed in TACI -/- Mice
Although an increase in ICOSL expression on B cells has been detected in both immunized and C. rodentium infected TACI -/- mice, the consequence of elevated ICOSL on PC development and antibody response was different in the two studies (11, 12). In immunized TACI -/- mice elevated ICOSL resulted in impaired PC development and reduced antibody responses (11). In contrast, C. rodentium infection of TACI -/- mice resulted in higher avidity antibodies that cleared the infection faster than wild-type mice did (12). To assess whether the persistence of GC for an extended duration together with increased ICOSL expression on TACI -/- GC B cells impacted the development of PC exiting the GC, we analyzed the kinetics of PC formation and GC development in the spleens of TACI -/- and wild-type mice following P. yoelii infection. As expected, both mice were free from GC occupying B cell follicules on day 8 post-infection (Figure 3A). We observed more PC (B220lowGL-7+) located in the marginal zones of wild-type mice spleens than the marginal zones of TACI -/- mice, which suggested the initiation of T-cell independent response (30) as early as day 8 in wild-type mice because, together with B1 cells, marginal zone B cells are responsible for T-cell independent responses (31). The absence of PC (B220lowGL-7+) in TACI -/- mice marginal zones is consistent with the well-established role for TACI in mediating T-cell independent responses (16, 32). At the same time, TACI -/- mice T cell zone was already populated with activated GL-7+ cells (33), suggesting that T cells were activated faster in TACI -/- than in wild-type mice (Figure 3A). Both strains had well-formed GC (B220+GL-7+) (34, 35) in B cell follicules as well as activated (GL-7+) cells occupying the T cell zone on day 16. By day 22, activated T cells were relocated to GC in the wild-type mice leaving the T cell-zones empty of GL-7+ T cells. Also on day 22, B220lowGL-7+ PC began exiting GC from the “Bridging channels” (36) in wild-type mice (Figures 3A,B). In TACI -/- mice however, not only the population of “Bridging channels” by B220lowGL-7+ PC was sparse on day 22, but also the T cell zone was still occupied with activated GL-7+ T cells in addition to the persistence of GCs in the follicules (Figures 3A,B). By day 28, activated T cells in the T cell zones were not present in TACI -/- spleens any longer. On the same day, the B220lowGL-7+ PC in the wild-type mice “Bridging channels” were more abundant and remained significantly more than that of TACI -/- mice (Figures 3A,B). In addition to the analysis of spleens in microscopy, we also assessed the frequency of splenic IgD−CD138+ PC in P. yoelii infected mice in flow cytometry. Supporting the microscopy results, we found that the frequencies of PC on days 15 and 23 were significantly lower in TACI -/- mice than wild-type mice (Figure 4).
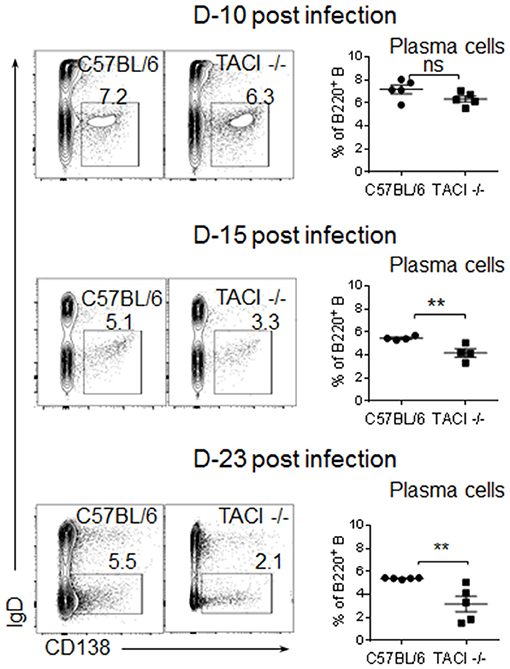
Figure 4. Development of PC in infected TACI -/- mice. TACI -/- and C57BL/6 mice were infected (i.p.) with 1 × 106 P. yoelii (Py 17XNL strain) parasites. Representative dot blots of splenic B220 gated IgD-CD138+ plasma cells on indicated time points are shown. Mean frequencies of PC as percentages of B cells are plotted for each time point. Each data point represents a mouse. Unpaired Student's t-test is used for statistical evaluation. Results are expressed as mean ± SEM (n = 5) from one representative experiment out of two with similar results. **p < 0.01 indicates TACI -/- vs. C57BL/6 mice. ns, not significant.
Despite a Delay in PC Response, TACI -/- Mice Develop Parasite Specific ASC and High Affinity Antibodies Following the Clearance of Infection
The discovery of significantly lower numbers of PC in TACI -/- mice as compared to wild-type mice at the time of parasite clearance (day 25), prompted us to measure Plasmodium-specific ASC during and after the infection. Reflecting the difference in anti-P. yoelii antibody levels between the two strains, we found significantly lower numbers of ASC recognizing the rMSP-119 protein in the spleen and bone marrow of TACI -/- mice 2 weeks post-infection (Figure 5). At the 2-month time point, TACI -/- mouse cells were still less than the wild-type cells but only the bone marrow ASC were statistically significantly lower in TACI -/- mice. In addition to aiding in the expansion of antibody secreting GC B cells, TFH cells also promote antibody affinity maturation. At 71 days post-infection, we found no difference in the avidity of antibodies directed against rMSP-119 protein between the mouse strains (Supplementary Figure 4). These observations suggested that anti-P. yoelii antibodies secreted from splenic ASC may be responsible for the resolution of infection in TACI -/- mice.
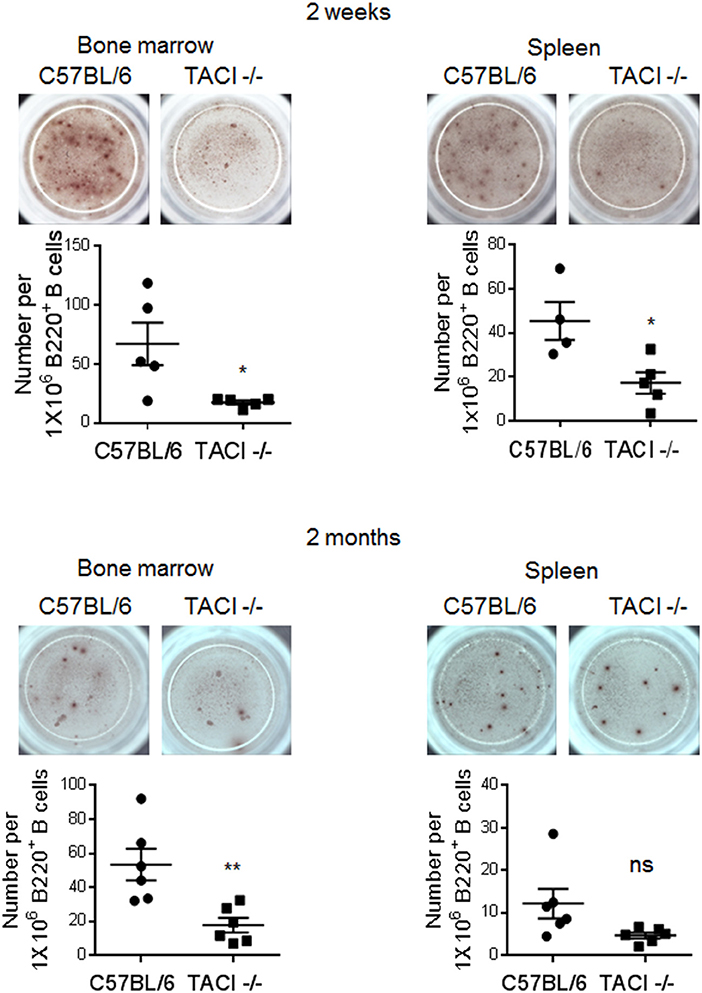
Figure 5. TACI deficiency causes delayed generation of rMSP-119-specific ASC. Spleens and bone marrow cells were harvested 2 weeks and 2 months post-infection to examine the frequency of antigen-specific IgG antibody secreting cells (ASC) in ELISPOT assay. The number of rMSP-119-specific ASC per 1 × 106 B220+ B cells were quantified and plotted. Each data point represents one mouse. Unpaired Student's t-test is used for statistical evaluation. Results are expressed as mean ± SEM (n = 4–6) from one representative experiment out of two with similar results. *p < 0.05 and **p < 0.01 indicate TACI -/- vs. C57BL/6 mice. ns, not significant.
TACI -/- Mice Were Protected From Re-infection With P. yoelii Parasites
B cells are crucial for the development of protective immunity against the erythrocytic stages of Plasmodium (37, 38). Since TACI -/- mice eventually cleared the infection and elicited parasite specific ASC and antibodies with affinities comparable to those of wild-type mice, we assessed whether they were resistant to a second challenge as has been shown for wild-type mice (39). TACI -/- and C57BL/6 mice were infected a second time with 1 × 106 P. yoelii 11 months after the clearance of the first infection. Naïve mice were also infected as a control. As expected, higher and delayed peak parasitemia, as well as delayed parasitemia resolution (day 29 post-infection) was observed in 1X infected TACI -/- mice as compared to wild-type mice (Figure 6A). Also, naïve wild-type mice parasitemia peaked on day 11 post-infection and was cleared by day 18. TACI -/- and C57BL/6 mice that had been infected previously were protected from a second P. yoelii infection, indicating a sustained long-term immunity. In these experimental groups, parasitemia levels at day 4 post-infection were 0.025% and 0.023% for TACI -/- and C57BL/6 mice, respectively, while no parasites were detected at day 11-time point.
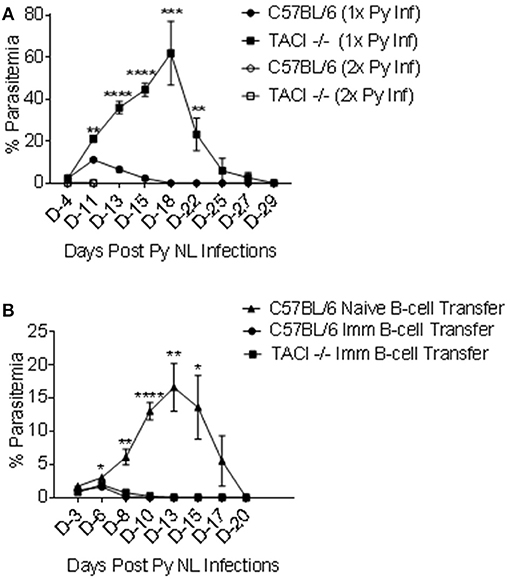
Figure 6. TACI -/- mice are resistant to re-infection with P. yoelii and P. yoelii experienced TACI -/- mice B cells are protective. (A) TACI -/- and C57BL/6 mice were re-infected (i.p.) with 1 × 106 P. yoelii (Py 17XNL) parasites 11 months after the first infection (2x Py inf). A second group of naïve TACI -/- and C57BL/6 mice was infected for the first time to recapture the first infection parasitemia (1x Py inf). Parasitemia levels were evaluated by blood smears at indicated days post challenges. Results are expressed as mean % parasitemia ± SEM for 10 mice per group for first challenge, and for 5 mice per group for the second challenge. Unpaired student's t-test was used for statistical evaluation. *indicates p < 0.05, **p < 0.01;***p < 0.001; and ****p < 0.0001 when comparing 1x Py NL and 2x Py infected TACI -/- mice. Experiment was performed once. (B) B cells from naive C57BL/6 or P. yoelii 17XNL infected C57BL/6 and TACI -/- mice were adoptively transferred into 3 to 4 naïve C57BL/6 mice. Two hours after B-cell transfer, mice were challenged (i.p.) with 1 × 106 P. yoelii parasites and parasitemia were assessed on indicated days. Unpaired student's t test was used for statistical evaluation. Results are expressed as mean % parasitemia ± SEM. *indicates p < 0.05, **p < 0.01;***p < 0.001; and ****p < 0.0001 when comparing the naïve B cell transfer and the TACI -/- B cell transfer groups. Experiment was performed once.
Adoptive Transfer of P. Yoelii Immune B-Cells Afford Protection
The association between antibody development and parasite clearance in both the strains suggested that the resistance to a second infection is likely a result of the persistence of B cell memory and/or PC. In a cerebral malaria model, CD19+ B cells from Plasmodium berghei recovered mice confers protection in naïve mice (40). To assess the role of parasite experienced B cells in the protection of TACI -/- mice against P. yoelii infection, we performed adoptive transfer experiments. Naïve B cells from C57BL/6 mice or B cells from previously infected C57BL/6 and TACI -/- mice (4 months earlier) were used as donor cells. Thirty million B cells were adoptively transferred into each naïve C57BL/6 mouse two hours before the experimental challenge with 1 × 106 P. yoelii parasites. In mice receiving naïve B cells, peak parasitemia level and time-point (day 13) (Figure 6B) were comparable to those of naïve mice receiving P. yoelii for the first time (Figure 1A). In contrast, C57BL/6 mice that were adoptively transferred with P. yoelii experienced cells from either strain had very low peak parasitemia levels (day 6), which resolved by day 10 post-infection in both mouse strains (Figure 6B). Thus, the B cell immunity developed after P. yoelii infection of TACI -/- mice is transferable and sufficient to render naïve mice resistant to P. yoelii challenge.
Discussion
Infant susceptibility to malaria is likely compounded by the unique features of their immune system. Both the qualitative and quantitative antibody responses to malaria are tightly associated with the age of infected infants (41–43). Incomplete understanding of the underlying molecular and cellular requirements supporting the development and maintenance of long lasting anti-malarial memory B cell and PC responses in infants hamper the development of effective malaria vaccines in children. In this study, we investigated the role of TACI in resistance to malaria infection because TACI is important for the development and maintenance of PC (5, 8–10, 15).
Both human and mouse studies suggested a role for the TACI ligand BAFF in host response to malaria infection. Elevated serum BAFF has been measured in adults experimentally infected with P. falciparum and in children with acute malaria (13, 14). The increase in serum BAFF levels is accompanied by a decrease in BAFF-R and an increase in TACI and BCMA on B cells of malaria-infected children (13). Despite these reports, the contribution of TACI in malaria infection has not been explored. We have previously shown that low TACI expression in neonatal B cells is responsible for the inability of this age group to respond to polysaccharide vaccines (15). We hypothesized that low TACI expression might also promote the suboptimal anti-malarial humoral immune response observed in infants. Significantly elevated levels and the delayed resolution of parasitemia in P. yoelii infected TACI -/- mice indicated that TACI is important for controlling malaria. Elicited protective humoral immunity was likely responsible for the clearance of Plasmodium in TACI -/- mice because the delayed clearance of P. yoelii coincided with the late emergence of anti-malaria antibodies, and B cells transferred from P. yoelii immune TACI -/- mice afforded protection in naïve wild-type mice, and TACI -/- mice rechallenged with P. yoelii resisted infection. Moreover, recovered TACI -/- mouse spleens contained P. yoelii specific ASC with comparable antibody avidity to those secreted from wild-type mice. Both T-cell dependent and independent mechanisms are involved in the development of anti-malaria antibodies (30). Since TACI expression is required for the development of T cell independent antibody responses, impairment in the antibodies targeting malarial T cell independent antigens likely have contributed to delayed parasitemia resolution. Indeed, we detected the appearance of GL7+ PC in the splenic marginal zones of wild-type mice as early as day 8 after infection while the TACI -/- mice marginal zone PC response was absent. Additional association between TACI expression and TI B cell responses to malaria may be related to the effect of toll-like receptor (TLR) 9 on TACI expression. We have previously shown that the TLR9 agonist CpG boosts BAFF and APRIL mediated PC generation by strongly upregulating the expression of TACI on B cells (44). Since, TLR9 deficiency compromises host control of parasitemia (45), TACI deficiency may also be negating the beneficial effect mediated by malaria TLR9 agonists (46).
Previous reports have shown that the formation of TFH and GC is amplified in TACI -/- mice after immunization (11) and infection (12). However, while the increased TFH and GC development was beneficial in eliciting high affinity antibodies that helped resist C. rodentium infection (12), nitrophenyl-chicken gamma globulin immunized TACI -/- mice generated lower levels of nitrophenyl-specific antibodies with diminished affinities (11). The immunization study also demonstrated an ablated ASC development in TACI -/- mice, which was attributed to the decrease in PC survival, a likely consequence of the absence of TACI mediated survival signals (11). Diminished ASC maintenance was reported in another study where influenza infected TACI -/- mice developed lower levels of virus-specific ASC compared to wild-type mice (10). Like the previous reports (11, 12), we observed exaggerated magnitude and kinetics in TACI -/- mice TFH and GC formation after Plasmodium infection. Despite the augmented TFH and GC B responses, TACI -/- mice were not able to control the parasitemia partly due to the delay in the emergence of PC from GC and the development of parasite specific ASC. Although the increased expression of ICOSL may be contributing to the persistence of Tfh and GC B cells, it can also be a result of persistent stimulation with parasite antigens as has been shown in a model where boosting with peptide stimulates TFH response without increasing B cell response (47).
Supporting the clinical observations where high level of BAFF is measured in Plasmodium infected individuals (13, 14), stimulation of monocytes with soluble Plasmodium molecules, soluble schizont fraction of Plasmodium falciparum antigen (sPFAg) and hemozoin (HZ) both induce the expression of BAFF (48). Although these studies suggested a possible involvement of increased serum BAFF in the activation of B cells and generation of antibody secreting cells in malaria, by analyzing P. yoelii infected mice, Liu et al detected a decrease in the number of dendritic cells (DCs) expressing membrane BAFF after malaria challenge (49). Since multimeric BAFF, but not trimeric serum BAFF, is able to promote PC by engaging TACI (50), the authors proposed a possible link between the disappearance of malaria specific ASC with the decrease in BAFF expressing DCs in malaria endemic regions. It remains to be seen whether the decrease in BAFF expressing DCs is accompanied by a decrease in the other TACI ligand, APRIL, and if not, whether APRIL can or cannot compensate for the diminished BAFF expressing DCs in sustaining the survival of ASC. Regardless of the significance of the changes in BAFF and APRIL expression, our study highlights the importance of TACI mediated development of ASC in controlling malaria infection. Interestingly, despite the delay in the generation of malaria specific antibodies and recovery from infection, TACI deficient mice remained resistant to second malaria challenge even after 11 months. Malaria specific B cells elicited in TACI -/- mice were not only able to clear the infection but also could render naïve mice resistant to malaria challenge.
Our study highlights the importance of TACI mediated control of TFH and GC response, and ASC development during malaria infection. These findings may have implications in understanding the immunobiological bases of infant-susceptibility to malaria since TACI expression is severely reduced in neonatal B cells in mice and in humans (15, 51). Moreover, as in TACI -/- mice, the development of humoral immune response is delayed in children from malaria endemic area (4, 52). Further work is needed in infant populations and in murine models of neonatal malaria to elucidate the impact of low TACI expression on the phenotype of TFH cells during malaria infection and vaccine response.
Author Contributions
MA, MP, and JY: designed the study and wrote the manuscript; MP, JY, MW, SD, AY, TS, and BS: performed the experiments; MP, JY, MW, SD, AY, TS, BS, AM, and MA: analyzed data.
Conflict of Interest Statement
The authors declare that the research was conducted in the absence of any commercial or financial relationships that could be construed as a potential conflict of interest.
Supplementary Material
The Supplementary Material for this article can be found online at: https://www.frontiersin.org/articles/10.3389/fimmu.2018.02612/full#supplementary-material
References
3. Cohen S, Mc GI, Carrington S. Gamma-globulin and acquired immunity to human malaria. Nature (1961) 192:733–7. doi: 10.1038/192733a0
4. Portugal S, Pierce SK, Crompton PD. Young lives lost as B cells falter: what we are learning about antibody responses in malaria. J Immunol. (2013) 190:3039–46. doi: 10.4049/jimmunol.1203067
5. Mackay F, Browning JL. BAFF: a fundamental survival factor for B cells. Nat Rev Immunol. (2002) 2:465–75. doi: 10.1038/nri844
6. Allman WR, Dey R, Liu L, Siddiqui S, Coleman AS, Bhattacharya P, et al. TACI deficiency leads to alternatively activated macrophage phenotype and susceptibility to Leishmania infection. Proc Natl Acad Sci USA. (2015) 112:E4094–103. doi: 10.1073/pnas.1421580112
7. Castigli E, Wilson SA, Scott S, Dedeoglu F, Xu S, Lam KP, et al. TACI and BAFF-R mediate isotype switching in B cells. J Exp Med. (2005) 201:35–9. doi: 10.1084/jem.20032000
8. Mantchev GT, Cortesao CS, Rebrovich M, Cascalho M, Bram RJ. TACI is required for efficient plasma cell differentiation in response to T-independent type 2 antigens. J Immunol. (2007) 179:2282–8. doi: 10.4049/jimmunol.179.4.2282
9. Tsuji S, Cortesao C, Bram RJ, Platt JL, Cascalho M. TACI deficiency impairs sustained Blimp-1 expression in B cells decreasing long-lived plasma cells in the bone marrow. Blood (2011) 118:5832–9. doi: 10.1182/blood-2011-05-353961
10. Wolf AI, Mozdzanowska K, Quinn WJ 3rd, Metzgar M, Williams KL, Caton AJ, et al. Protective antiviral antibody responses in a mouse model of influenza virus infection require TACI. J Clin Invest. (2011) 121:3954–64. doi: 10.1172/JCI57362
11. Ou X, Xu S, Lam KP. Deficiency in TNFRSF13B (TACI) expands T-follicular helper and germinal center B cells via increased ICOS-ligand expression but impairs plasma cell survival. Proc Natl Acad Sci USA. (2012) 109:15401–6. doi: 10.1073/pnas.1200386109
12. Tsuji S, Stein L, Kamada N, Nunez G, Bram R, Vallance BA, et al. TACI deficiency enhances antibody avidity and clearance of an intestinal pathogen. J Clin Invest. (2014) 124:4857–66. doi: 10.1172/JCI74428
13. Nduati E, Gwela A, Karanja H, Mugyenyi C, Langhorne J, Marsh K, et al. The plasma concentration of the B cell activating factor is increased in children with acute malaria. J Infect Dis. (2011) 204:962–70. doi: 10.1093/infdis/jir438
14. Scholzen A, Teirlinck AC, Bijker EM, Roestenberg M, Hermsen CC, Hoffman SL, et al. BAFF and BAFF receptor levels correlate with B cell subset activation and redistribution in controlled human malaria infection. J Immunol. (2014) 192:3719–29. doi: 10.4049/jimmunol.1302960
15. Kanswal S, Katsenelson N, Selvapandiyan A, Bram RJ, Akkoyunlu M. Deficient TACI expression on B lymphocytes of newborn mice leads to defective Ig secretion in response to BAFF or APRIL. J Immunol. (2008) 181:976–90. doi: 10.4049/jimmunol.181.2.976
16. von Bulow GU, van Deursen JM, Bram RJ. Regulation of the T-independent humoral response by TACI. Immunity (2001) 14:573–82. doi: 10.1016/S1074-7613(01)00130-3
17. Parra M, Derrick SC, Yang A, Tian J, Kolibab K, Oakley M, et al. Malaria infections do not compromise vaccine-induced immunity against tuberculosis in mice. PLoS ONE (2011) 6:e28164. doi: 10.1371/journal.pone.0028164
18. Singh B, Cabrera-Mora M, Jiang J, Galinski M, Moreno A. Genetic linkage of autologous T cell epitopes in a chimeric recombinant construct improves anti-parasite and anti-disease protective effect of a malaria vaccine candidate. Vaccine (2010) 28:2580–92. doi: 10.1016/j.vaccine.2010.01.019
19. Ibison F, Olotu A, Muema DM, Mwacharo J, Ohuma E, Kimani D, et al. Lack of avidity maturation of merozoite antigen-specific antibodies with increasing exposure to Plasmodium falciparum amongst children and adults exposed to endemic malaria in Kenya. PLoS ONE (2012) 7:e52939. doi: 10.1371/journal.pone.0052939
20. Perciani CT, Peixoto PS, Dias WO, Kubrusly FS, Tanizaki MM. Improved method to calculate the antibody avidity index. J Clin Lab Anal. (2007) 21:201–6. doi: 10.1002/jcla.20172
21. Parra M, Liu X, Derrick SC, Yang A, Tian J, Kolibab K, et al. Molecular analysis of non-specific protection against murine malaria induced by BCG vaccination. PLoS ONE (2013) 8:e66115. doi: 10.1371/journal.pone.0066115
22. Jayawardena AN, Targett GA, Leuchars E, Davies AJ. The immunological response of CBA mice to P. yoelii. II. The passive transfer of immunity with serum and cells. Immunology (1978) 34:157–65.
23. Vinuesa CG, Linterman MA, Yu D, MacLennan IC. Follicular helper T cells. Annu Rev Immunol. (2016) 34:335–68. doi: 10.1146/annurev-immunol-041015-055605
24. De Silva NS, Klein U. Dynamics of B cells in germinal centres. Nat Rev Immunol. (2015) 15:137–48. doi: 10.1038/nri3804
25. Hansen DS, Obeng-Adjei N, Ly A, Ioannidis LJ, Crompton PD. Emerging concepts in T follicular helper cell responses to malaria. Int J Parasitol. (2017) 47:105–10. doi: 10.1016/j.ijpara.2016.09.004
26. Chung Y, Tanaka S, Chu F, Nurieva RI, Martinez GJ, Rawal S, et al. Follicular regulatory T cells expressing Foxp3 and Bcl-6 suppress germinal center reactions. Nat Med. (2011) 17:983–8. doi: 10.1038/nm.2426
27. Linterman MA, Pierson W, Lee SK, Kallies A, Kawamoto S, Rayner TF, et al. Foxp3+ follicular regulatory T cells control the germinal center response. Nat Med. (2011) 17:975–82. doi: 10.1038/nm.2425
28. Sage PT, Sharpe A H. T follicular regulatory cells. Immunol Rev. (2016) 271:246–59. doi: 10.1111/imr.12411
29. Choi YS, Kageyama R, Eto D, Escobar TC, Johnston RJ, Monticelli L, et al. ICOS receptor instructs T follicular helper cell versus effector cell differentiation via induction of the transcriptional repressor Bcl6. Immunity (2011) 34:932–46. doi: 10.1016/j.immuni.2011.03.023
30. Krishnamurty AT, Thouvenel CD, Portugal S, Keitany GJ, Kim KS, Holder A, et al. Somatically hypermutated plasmodium-specific IgM(+) memory B cells are rapid, plastic, early responders upon malaria rechallenge. Immunity (2016) 45:402–14. doi: 10.1016/j.immuni.2016.06.014
31. Martin F, Oliver AM, Kearney JF. Marginal zone and B1 B cells unite in the early response against T-independent blood-borne particulate antigens. Immunity (2001) 14:617–29. doi: 10.1016/S1074-7613(01)00129-7
32. Yan M, Wang H, Chan B, Roose-Girma M, Erickson S, Baker T, et al. Activation and accumulation of B cells in TACI-deficient mice. Nat Immunol. (2001) 2:638–43. doi: 10.1038/89790
33. Kerfoot SM, Yaari G, Patel JR, Johnson KL, Gonzalez DG, Kleinstein SH, et al. Germinal center B cell and T follicular helper cell development initiates in the interfollicular zone. Immunity (2011) 34:947–60. doi: 10.1016/j.immuni.2011.03.024
34. Han S, Dillon SR, Zheng B, Shimoda M, Schlissel MS, Kelsoe G. V(D)J recombinase activity in a subset of germinal center B lymphocytes. Science (1997) 278:301–5. doi: 10.1126/science.278.5336.301
35. Han S, Zheng B, Schatz DG, Spanopoulou E, Kelsoe G. Neoteny in lymphocytes: Rag1 and Rag2 expression in germinal center B cells. Science (1996) 274:2094–7. doi: 10.1126/science.274.5295.2094
36. Gatto D, Brink R. The germinal center reaction. J Allergy Clin Immunol. (2010) 126:898–907; quiz 908–9. doi: 10.1016/j.jaci.2010.09.007
37. von der Weid T, Honarvar N, Langhorne J. Gene-targeted mice lacking B cells are unable to eliminate a blood stage malaria infection. J Immunol. (1996) 156:2510–6.
38. Langhorne J, Cross C, Seixas E, Li C, von der Weid T. A role for B cells in the development of T cell helper function in a malaria infection in mice. Proc Natl Acad Sci USA. (1998) 95:1730–4. doi: 10.1073/pnas.95.4.1730
39. Fahey JR, Spitalny GL. Rapid parasite multiplication rate, rather than immunosuppression, causes the death of mice infected with virulent Plasmodium yoelii. Infect Immun. (1987) 55:490–3.
40. Bao LQ, Huy NT, Kikuchi M, Yanagi T, Senba M, Shuaibu MN, et al. CD19(+) B cells confer protection against experimental cerebral malaria in semi-immune rodent model. PLoS ONE (2013) 8:e64836. doi: 10.1371/journal.pone.0064836
41. Branch OH, Oloo AJ, Nahlen BL, Kaslow D, Lal A. Anti-merozoite surface protein-1 19-kDa IgG in mother-infant pairs naturally exposed to Plasmodium falciparum: subclass analysis with age, exposure to asexual parasitemia, and protection against malaria. V. The Asembo Bay Cohort Project. J Infect Dis. (2000) 181:1746–52. doi: 10.1086/315424
42. Branch OH, Udhayakumar V, Hightower AW, Oloo AJ, Hawley WA, Nahlen BL, et al. A longitudinal investigation of IgG and IgM antibody responses to the merozoite surface protein-1 19-kiloDalton domain of Plasmodium falciparum in pregnant women and infants: associations with febrile illness, parasitemia, and anemia. Am J Trop Med Hyg. (1998) 58:211–9. doi: 10.4269/ajtmh.1998.58.211
43. Tongren JE, Drakeley CJ, McDonald SL, Reyburn HG, Manjurano A, Nkya WM, et al. Target antigen, age, and duration of antigen exposure independently regulate immunoglobulin G subclass switching in malaria. Infect Immun. (2006) 74:257–64. doi: 10.1128/IAI.74.1.257-264.2006
44. Katsenelson N, Kanswal S, Puig M, Mostowski H, Verthelyi D, Akkoyunlu M. Synthetic CpG oligodeoxynucleotides augment BAFF- and APRIL-mediated immunoglobulin secretion. Eur J Immunol. (2007) 37:1785–95. doi: 10.1002/eji.200636800
45. Gowda NM, Wu X, Gowda DC. TLR9 and MyD88 are crucial for the development of protective immunity to malaria. J Immunol. (2012) 188:5073–85. doi: 10.4049/jimmunol.1102143
46. Coban C, Horii T, Akira S, Ishii KJ. TLR9 and endogenous adjuvants of the whole blood-stage malaria vaccine. Expert Rev Vaccines (2010) 9:775–84. doi: 10.1586/erv.10.60
47. Deenick EK, Chan A, Ma CS, Gatto D, Schwartzberg PL, Brink R, et al. Follicular helper T cell differentiation requires continuous antigen presentation that is independent of unique B cell signaling. Immunity (2010) 33:241–53. doi: 10.1016/j.immuni.2010.07.015
48. Kumsiri R, Potup P, Chotivanich K, Petmitr S, Kalambaheti T, Maneerat Y. Blood stage Plasmodium falciparum antigens induce T cell independent immunoglobulin production via B cell activation factor of the TNF family (BAFF) pathway. Acta Trop. (2010) 116:217–26. doi: 10.1016/j.actatropica.2010.08.012
49. Liu XQ, Stacey KJ, Horne-Debets JM, Cridland JA, Fischer K, Narum D, et al. Malaria infection alters the expression of B-cell activating factor resulting in diminished memory antibody responses and survival. Eur J Immunol. (2012) 42:3291–301. doi: 10.1002/eji.201242689
50. Bossen C, Cachero TG, Tardivel A, Ingold K, Willen L, Dobles M, et al. TACI, unlike BAFF-R, is solely activated by oligomeric BAFF and APRIL to support survival of activated B cells and plasmablasts. Blood (2008) 111:1004–12. doi: 10.1182/blood-2007-09-110874
51. Kaur K, Chowdhury S, Greenspan NS, Schreiber JR. Decreased expression of tumor necrosis factor family receptors involved in humoral immune responses in preterm neonates. Blood (2007) 110:2948–54. doi: 10.1182/blood-2007-01-069245
Keywords: Plasmodium yoelii, TACI, T follicular helper cell, Germinal center, B cell, antibody
Citation: Parra M, Yang J, Weitner M, Derrick S, Yang A, Schmidt T, Singh B, Moreno A and Akkoyunlu M (2018) TACI Contributes to Plasmodium yoelii Host Resistance by Controlling T Follicular Helper Cell Response and Germinal Center Formation. Front. Immunol. 9:2612. doi: 10.3389/fimmu.2018.02612
Received: 28 June 2018; Accepted: 23 October 2018;
Published: 09 November 2018.
Edited by:
Daniel M. Altmann, Imperial College London, United KingdomReviewed by:
Christian Engwerda, Queensland Children's Medical Research Institute, AustraliaKevin Couper, University of Manchester, United Kingdom
Copyright © 2018 Parra, Yang, Weitner, Derrick, Yang, Schmidt, Singh, Moreno and Akkoyunlu. This is an open-access article distributed under the terms of the Creative Commons Attribution License (CC BY). The use, distribution or reproduction in other forums is permitted, provided the original author(s) and the copyright owner(s) are credited and that the original publication in this journal is cited, in accordance with accepted academic practice. No use, distribution or reproduction is permitted which does not comply with these terms.
*Correspondence: Mustafa Akkoyunlu, TXVzdGFmYS5Ba2tveXVubHVAZmRhLmhocy5nb3Y=
†These authors have contributed equally to this work