- 1Discipline of Pharmacology, Faculty of Medicine and Health, School of Medicine, The University of Sydney, Camperdown, NSW, Australia
- 2Medlab Clinical Ltd., Sydney, NSW, Australia
Post-birth there is a bacterial assault on all mucosal surfaces. The intestinal microbiome is an important participant in health and disease. The pattern of composition and concentration of the intestinal microbiome varies greatly. Therefore, achieving immunological tolerance in the first 3–4 years of life is critical for maintaining health throughout a lifetime. Probiotic bacteria are organisms that afford beneficial health effects to the host and in certain instances may protect against the development of disease. The potential benefits of modifying the composition of the intestinal microbial cohort for therapeutic benefit is evident in the use in high risks groups such as premature infants, children receiving antibiotics, rotavirus infections in non-vaccinated children and traveler's diarrhea in adults. Probiotics and prebiotics are postulated to have immunomodulating capabilities by influencing the intestinal microbial cohort and dampening the activity of pathobiont intestinal microbes, such as Klebsiella pneumonia and Clostridia perfringens. Lactobacilli and Bifidobacteria are examples of probiotics found in the large intestine and so far, the benefits afforded to probiotics have varied in efficacy. Most likely the efficacy of probiotic bacteria has a multifactorial dependency, namely on a number of factors that include agents used, the dose, the pattern of dosing, and the characteristics of the host and the underlying luminal microbial environment and the activity of bacteriophages. Bacteriophages, are small viruses that infect and lyse intestinal bacteria. As such it can be posited that these viruses display an effective local protective control mechanism for the intestinal barrier against commensal pathobionts that indirectly may assist the host in controlling bacterial concentrations in the gut. A co-operative activity may be envisaged between the intestinal epithelia, mucosal immunity and the activity of bacteriophages to eliminate pathobiots, highlighting the potential role of bacteriophages in assisting with maintaining intestinal homeostasis. Hence bacteriophage local control of inflammation and immune responses may be an additional immunological defense mechanism that exploits bacteriophage–mucin glycoprotein interactions that controls bacterial diversity and abundance in the mucin layers of the gut. Moreover, and importantly the efficacy of probiotics may be dependent on the symbiotic incorporation of prebiotics, and the abundance and diversity of the intestinal microbiome encountered. The virome may be an important factor that determines the efficacy of some probiotic formulations.
Historical Perspective—Microorganisms and Immunity
The mid seventeenth century ushered the beginning of a new era in medicine with the discovery of a microscopic world of bacteria and fungi (1) that in the twenty-first century these entities have been demonstrated to play a crucial role in shaping human immunity and maintaining immunological and metabolic tolerance throughout a lifetime (2, 3).
The history of health assertions relative to live microorganisms present in food is extensive and predates antiquity. Particularly, that involving lactic acid producing bacteria as described in the Old Testament from Persian scriptures where it was alluded to, that Abraham's consumption of sour milk provided a health and longevity benefit (4). Furthermore, writings from the Roman historian Plinius from the first-century, suggested that the administration of fermented milk foods could be used to treat gastroenteritis (4). Microbiological observations (5–7) and Metchnikoff (7) are credited with promoting the extensive health effects and restorative shifts in the balance of the gastrointestinal microbial cohort with the consumption of lactic acid bacteria. Metchnikoff who was at the Pasteur Institute, suggested that an extension of the life span of Bulgarian workers resulted from the ingestion of fermented milk foods (7). The consequence of consuming Lactobacilli inoculated yogurts could lead to a significant decrease of intestinal toxin-producing bacteria and that this effect then led to an increase in longevity. Moreover, Tissier (5, 8) reported that Bifidobacteria predominated in the intestines of breast-fed infants (5) and subsequently endorsed the administration of Bifidobacteria to infants distressed with diarrhea, and then further suggested that Bifidobacteria superseded the putrefactive gut pathobionts that would cause disease (8).
The early studies on lactic acid bacteria became recognized as the link between microbiology and the foundation of immunology (9). Metchnikoff's immunological studies credit his discovery of phagocytosis by macrophages and microphages as the elucidative step in host-defense mechanisms that established innate immunity; simultaneously Ehrlich defined the side-chain theory of antibody formation and the immunological pathways of how antibodies counteract toxins that encourage bacterial lysis. Furthermore, Erhlich also documented on how with the participation of complement, it led to an enhanced understanding of humoral adaptive immunity (9).
The early studies on lactic acid bacteria became recognized as the link between microbiology and the foundation of immunology (10). Metchnikoff's immunological studies associated with phagocytosis with macrophages and microphages resulted as an important discovery step in host-defense mechanisms. These studies established the foundation of cell innate immunity. Simultaneously Ehrlich explained the side-chain model of antibody formation and the immunological pathways of how antibodies counteract systemic toxins and encourage bacterial lysis. Furthermore, Ehrlich proposed that the participation of complement enhances the understanding of humoral adaptive immunity (10). Recent advances describe an overview of immunity as divided into two predominant systems that are determined by the speed and specificity of the reactions that occur (11). That is innate immunity describes chemical, physical, and microbiological barriers that usually encompass immune system elements of neutrophils, monocytes, macrophages, and the complement network of cytokine proteins and other acute phase proteins that provide an immediate response to an infective insult, a response that is imperative for survival. Cell mediated immunity is the immune characteristic exhibited by higher animals that comprises antigen specific responses through T and B lymphocytes, a slow but precise response to an infective agent (12). Moreover, a link has been recognized to exist between the innate and cell mediated immunity systems that is necessitated to complete the immune response and that is the role of complement that effectively bridges both systems in order to neutralize an infective bacterial, viral or other insult (13). The human microbiome project (14) has redefined the role of bacteria that live on and within humans especially in the intestinal tract, from one that was considered to be a site of toxic waste and pathogenic bacteria to a site with important immunological activity (15). Recently, reviews have described how the role of probiotics enhancing immunological functions in the intestines to restore local and extra-intestinal mucosal and innate immunity equilibrium have gained significant support (16, 17).
Dysbiosis
A historical perspective on the origin of the term “dysbiosis” has been recently documented (18), with Haene (19) a German microecologist, credited with popularizing the term (18). Moreover, Haenel was also instrumental in contrasting dysbiosis with eubiosis which he referred to this latter term as the “normal state” (19).
Dysbiosis usually refers to an imbalance of intestinal bacteria that occupy the lumen of the gut that refers to adverse microbiome pattern shifts that have been associated with disease development and progression (18). Further, dysbiosis has been reported to be the subject of multiple explicit and semi-explicit delineations (18).
The chronic diseases that have been associated with intestinal microbiome dysbiosis include intestinal inflammatory diseases (e.g., Ulcerative colitis, Irritable Bowel Syndrome), auto-immune diseases (e.g., multiple sclerosis, asthma), metabolic diseases (e.g., diabetes), neuro-degenerative diseases (e.g., Parkinson's Disease, Dementias), and cancer (20). The community of bacteria that inhabit the intestines has been investigated from multi-dimensional studies with samples from different geographical areas. The samples were constructed from variations observed in the concentration of three dominant/conserved bacterial genera namely, Bacteroides (enterotype 1); Prevotella (enterotype 2); and Ruminococcus (enterotype 3) (21, 22). The topographical view of the intestinal microbiome has provided the basis to further understand the bacterial phyla configurations associated with dysbiotic shifts that have been correlated with disease states. As for example, when comparing the intestinal microbiome of obese to lean subjects studies have shown increased phyla abundances in Firmicutes and decreased Bacteriodetes (23).
Intestinal Bacteria and Immunity
The human microbiome project has largely changed the way bacteria have been viewed in the large bowel; from a collection of waste products and pathogens to a more pragmatic view concerned with early immunological and metabolic development and to sustain a stable equilibrium. The mucosal surfaces of the intestines provides a large complex and interactive surface area between the commensal bacterial cohort and the intestinal epithelia (24). Specifically, the complex nature of the intestinal architecture is evident from the components of the differentiated epithelial cell types that are encountered such as enterocytes (approximate turnover of 3–5 days which migrate out of the aberrant crypt), enteroendocrine cells, goblet cells, tuft cells, and Paneth cells (approximate turnover of 30 days which do not migrate out of the aberrant crypt) (25, 26). The cross-talk that has evolved between bacteria and host gut tissue has spanned millennia (16). In particular, Paneth cells synthesize and secrete proteins and antimicrobial peptides (i.e., α/β defensins; cathelicidin; 14β-glycosidases; C-type lectins; ribonuclease), activities that emanate from various external and internal stimuli (e.g., intestinal bacterial milieu such as bacterial surface components) and toll-like receptor activity (27).
Experiments with gnotobiotic murine models have shown that when colonizing the animals with a single bacterium that adhered to the surface of the intestinal epithelia encouraged the growth and activity of a set of genes in the host animal, that were involved in immune function, and the elaboration of proteins that protected against the deterioration of the gut epithelia [Figure 1; (28, 29)] Additional experiments have also reported on the interactions between commensal bacteria and host immune cells and have shown that macrophages that are in close proximity to the base of the intestinal epithelia participate in antigen recognition and responses (30). That is, macrophages/dendritic cells take up antigen and produce cytokines and depending on which cytokine is produced, a response is elicited such as anti-inflammatory. Research has advanced the idea that antigens from the intestines or from the environment are transcytosed (31, 32) by specialized enterocytes namely, Microfold-cells into the sub-epithelial dome region of the Peyer's patch mucosa, an area rich in macrophages/dendritic cells (33–35) where these antigen-presenting cells reside and progress to collect the trancytosed bacteria and macromolecular antigens (36). In the lamina propria macrophages have been demonstrated to sample antigens, entero-pathobionts and commensal bacteria through trans-epithelial dendrites. Intestine-resident macrophages (CX3 CR1hi+) that are resultant from blood monocytes (Ly6C+) do persist in close physical proximity whilst maintaining the integrity of the intestinal epithelial cells and barrier function. Experiments investigating specific effects with the human and animal commensal bacillus, Clostridium butyricum, have demonstrated that in a murine model the spore forming anaerobe induced IL-10 producing macrophages that suppressed an acute experimental inflammatory outcome (37). The explicit effect triggered by Clostrodium butyricum treatment showed that the outcome was not associated with cytokine IL-10 production by Treg cells but that instead the anti-inflammatory effect was due to IL-10-producing F4/80+CD11b+CD11cint macrophages through the TLR2/MyD88 pathway and subsequently observed to have accumulated in the inflamed mucosa. The posit that macrophage sampling eliciting and then escalating an antigen specific immune response has recently been insightfully progressed (38, 39). Man et al. (38) in a murine study with Salmonella typhimurium demonstrated that there also exist protective mechanisms in the intestines that hinder the access of bacteria to the intestinal epithelia that thereby block pathogen penetration across the intestinal barrier. Specifically, intestinal epithelium cell emerging signals, trigger the intraluminal migration of CX3CR1+ cells forming an intricate network of cellular defense. This protective effect presents an additional barrier to the copious amounts of mucus and Immunoglobulin A that are constantly produced to maintain local homeostasis, when these first line of defense are breached the complex network of intraluminal migration prevents early local infectivity from progressing.
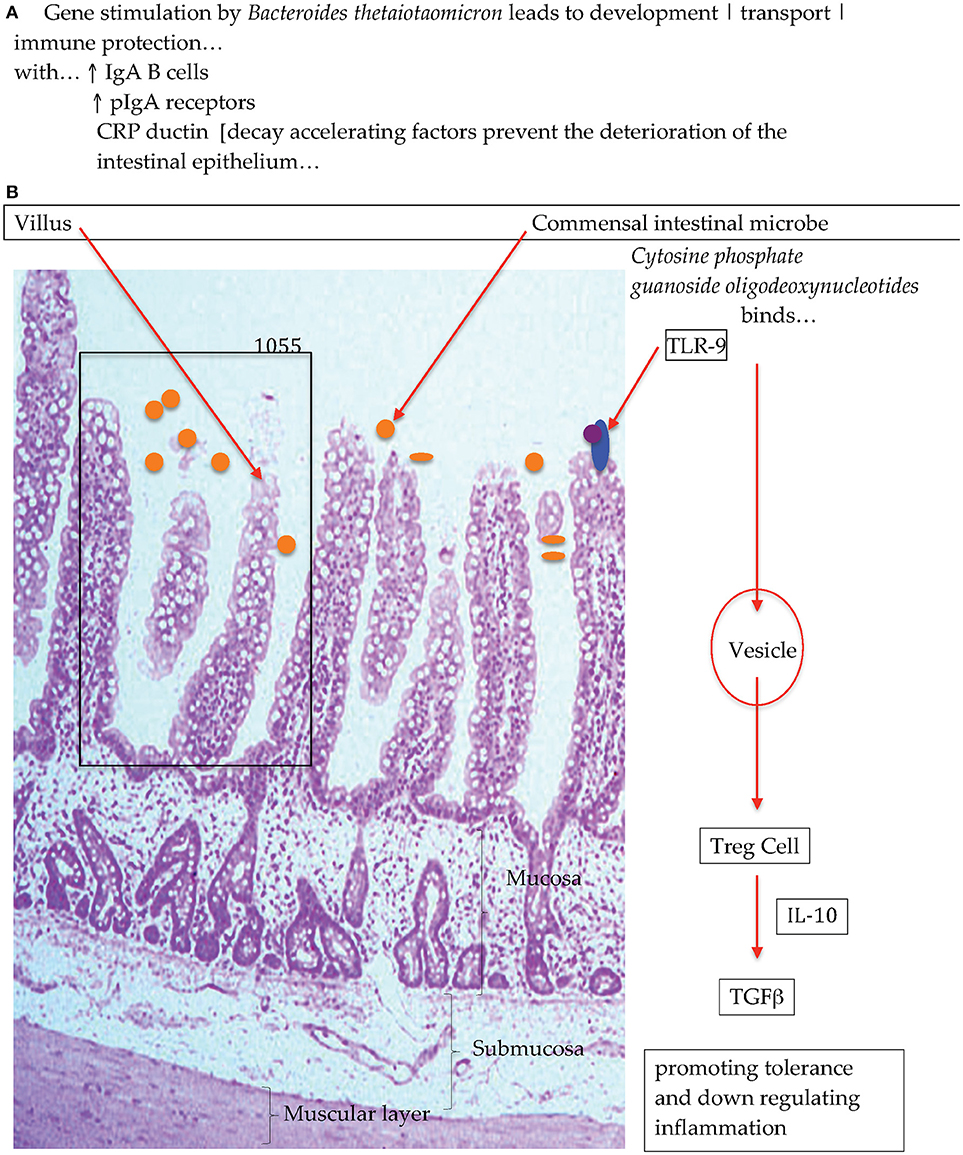
Figure 1. Diagrammatic representation of (A) bacterial colonization stimulates intestinal gene activation and (B) commensal bacteria interactions with Toll-Like Receptors to promote immunological equilibrirum (28).
It is widely acknowledged and published in the scientific literature that food antigens do indeed affect immunological responses (40). In the intestinal induction of Tregs and Th17 is characteristic of the gut immune network (41). Consequently, the Th17 cells strengthen the mucosal barrier and concomitantly encourage intestinal epithelial cells to produce antimicrobial peptides, with the overall effect centered on maintaining local homeostasis (42). Regulatory T cells that promote tolerance do so to reduce reactivity to dietary and environmental antigens by decreasing intestinal inflammation. Strikingly this regulated pro-inflammatory to anti-inflammatory activity has been demonstrated to be a cooperative crosstalk between commensal bacteria, the host intestinal epithelia and the mucosal immune network to reduce the risk of inflammatory responses by down regulating the effect of dietary and or bacterial antigens that in turn maintains local immunity in equilibrium (43). For example the mucosal accumulation of Th17 cells has been shown to be subject to stimulation by commensal bacteria (i.e., Segmented Filamentous Bacterium) (44) indicating that commensal bacteria very much promote the accumulation of Th17 cells in the intestinal lamina propria (45, 46).
As a consequence, specific bacterial species have been shown to significantly influence immune tissue subsets of cells and maturation of innate immunity in health and disease [Table 1; (70, 71)]. Probiotic bacteria, in one instance, were posited to induce a re-regulation of the nuclear translocation of NFκB in intestinal epithelial cells by shaping the release of TNF-α that links this molecular action to a significant decrease in epithelial permeability and susceptibility to an inflammatory Crohn-like ileitis in the SAMP1/YitFc murine model that spontaneously progress to an inflammatory disease (72).
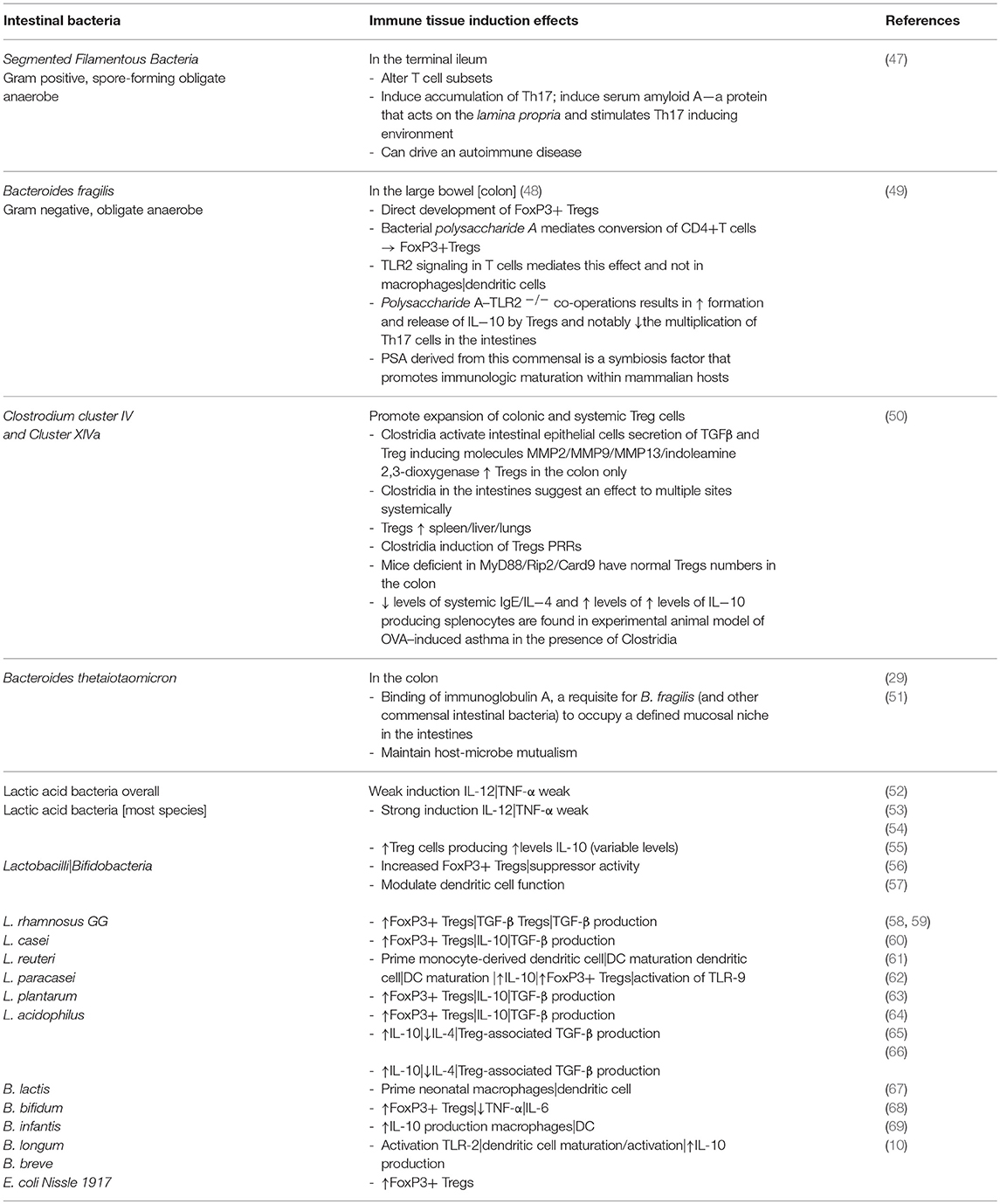
Table 1. Commensal intestinal bacteria and effects on the development of tolerogenic macrophages|dendritic cells; induction of Treg cells; and stimulation of TLRs.
Among all the microbial metabolites expressed in the intestinal lumen the most abundant in the colon are SCFAs (73). Recently, probiotic species from the Lactobacilli and Bifidobacteria genera were demonstrated to increase the levels of fecal SCFAs (74). These chemical species exhibit multi-faceted regulatory roles in the local mucosal immune system in the gut. That is (i) SCFAs are a major energy source of intestinal epithelia significantly influencing gene expression (i.e., epigenetics) that is an important pre-requisite for maintaining the coherence of the epithelial lining and epithelium to epithelium tight junctions and mechanisms of defense. Hence it is envisaged that SCFAs can also (ii) regulate local mucosal derived innate immune cells such as macrophages or dendritic cells as well as neutrophils. Moreover, SCFAs also (iii) are involved in a bi-directional regulation of specific antigen-triggered adaptive immunity activities that are mediated by T and B lymphocytic cells.
Probiotics
Probiotics were originally defined as live micro-organisms that could be added to fermented foods that in such a matrix could be advantageous to health by establishing an overall improved stability to the intestinal microbial cohort (75) and then modified by the FAO/WHO (76). Micro-organisms predominantly utilized as probiotics include various members of the Lactobacilli or Bifidobacteria species which are administered either individually or combinations of various formulations. The non-pathogenic yeast, Saccharomyces boulardii, has also been designated as a probiotic following its administration in both animal studies and human clinical trials. Further, the inability of probiotics to permanently colonize the intestines has let to posits that they be dispensed in sufficient quantities to maintain high amounts in the colon; and that probiotic species be of human origin.
Probiotics: in vitro and Laboratory Animal Data
The critical and important purported health-recommended effect of probiotics is the ability of these bacteria to enhance mucosal immune defenses (77). The gut associated lymphoid tissue can be distributed according to anatomical sites, with lymphocytes disseminated throughout (i) intestinal epithelia regions in contact with the lamina propria and (ii) structured lymphoid tissue sites, that includes Peyer's patches and mesenteric lymph nodes (35).
Studies with germ-free animals (gnotobiotic) clearly demonstrate that in the absence of significant intestinal microbial colonization, the effective component of the mucosal immune system remains immature, an outcome that enhances the host's susceptibility to bacterial infections by pathobionts (78). Moreover, general mechanisms for the function of probiotics have been associated with protective effects provided against pathobiont microbial colonization and translocation within the intestines (79). It has been postulated that mechanisms include production of antibiotic type substances (i.e., reuterin) (80) and competition for receptor sites on the mucosal intestinal surface (81). Other mechanisms such as heightening immune defenses of the host that produce adjuvant effects, amplified immunoglobulin A production and cytokine stimuli, as well as competition with pathogenic organisms for intraluminal nutrients (82, 83). Studies have also suggested that probiotics that include non-immune intestinal host defenses could strengthen tight junctions of the gut mucosa, increase mucous secretions, enhance motility, and produce amino acid by-products including arginine and glutamine, and SCFAs, that could secondarily function as protective foods for the gut (84–87).
A series of basic laboratory studies have highlighted the influence that probiotic bacteria and commensal bacteria may have on the maturation of intestinal macrophages/dendritic cells and the production of various cytokines (Table 2).
In vitro and in vivo studies have appraised the effects of probiotics on the prevention/development of large bowel cancer (i.e., specifically in the colon) (96). Epidemiological studies have associated large bowel cancers with genetic, environmental risk factors, including diet and the nature of the intestinal bacterial cohort (97). Probiotics used in animal models have been shown to reduce the occurrence of precancerous lesions observed in aberrant crypts (98, 99). In vitro experiments with probiotics have further suggested that the administration of these beneficial bacteria could reduce hypertension and lower serum cholesterol (100, 101). Furthermore, animal studies have also suggested that Helicobactor pylori infection in germ free murine models could be averted with the administration of Lactobacilli to dislodge H. pylori from the stomach and that cell attachment and invasion by enteropathogenic bacteria such as Escherichia coli and other gram-negative pathobionts can be inhibited with the use of Lactobacillus acidophilus containing probiotics (102). Interestingly, in a murine model of experimental uremia (103) intestinal macrophages were skewed toward a pro-inflammatory phenotype with reduced phagocytic activity with resultant bacterial translocation that triggered a local inflammatory response. The administration of a Lactobacilli LB probiotic reduced bacterial translocation by improving macrophage phagocytic activity.
Probiotics Human Studies
The extensive body of clinical evidence that supports the use of probiotics in the prevention or treatment of gastrointestinal diseases have been administered in clinical trials with pediatric and adult patients (104–107). Formulations with probiotic bacteria include members from the bacterial genera, Lactobacilli, Bifidobacteria, and Streptoccocus (i.e., Streptococcus thermophiles) or the yeast, S. boulardii (108). Numerous studies report that probiotics provide efficacy for reducing the risk of developing Clostridium-Difficile-Associated-Diarrhea (109); preventing antibiotic associated diarrhea (110, 111); and Traveler's diarrhea (112, 113). Moreover in a systematic review study of outpatients, S. boulardii was reported efficacious in preventing antibiotic associated diarrhea (110). Hence probiotics can have beneficial effects on diarrheal conditions and related gastrointestinal symptoms. Further, evidence-based probiotic formulations can be administered to either prevent or reduce the severity of pathogenic bacteria triggered intestinal inflammations. Consequently, inflammatory bowel diseases (IBDs) can present with major clinical inflammatory associated complications. Anti-inflammatory pharmaceutical agents have been used extensively to ameliorate the chronic inflammatory responses that occur associated with IBDs. In vivo laboratory studies have reported success with probiotics used to prevent or reduce the inflammatory response associated with colitis (114). Although encouraging, additional studies are needed and warrants further focused research to make conclusive inferences on the efficacy of probiotics for ulcerative colitis, Crohn's Disease, and liver diseases (e.g., NAFLD) (115).
Mechanistically, it has been posited that formulas supplemented with probiotic bacteria could induce changes in the stool pattern that is bifidogenic and that it could mimic that observed with breast-fed infants (116, 117). Alternatively, in an early study with undernourished Peruvian infants, especially among non-breast fed children with a high encumbrance of diarrheal disease, the administration of Lactobacilli GG was reported associated with significantly fewer episodes of diarrhea (118). Clinical efficacy with probiotic formulations have been reported to reduce the number of episodes of diarrhea and rotavirus shedding among chronically infected infants admitted to hospital, young children as well as adults (119). Studies specifically with Lactobacillus GG administered as a treatment modality, during acute rotavirus infections with diarrhea has been reported and associated with higher titres of polymeric immunoglobulin A to the infections with rotavirus (119, 120). Additionally, significant experimental and clinical studies have reported the efficacy for reducing the incidence of neonatal necrotizing enterocolitis with the administration of Lactobalcilli and Bifidobacteria (121, 122). Increased efficacy of probiotics have recently been reported to significantly decrease the risk of developing clinical complications related with necrotizing enterocolitis and sepsis; decrease mortality and length of hospital stay; as well as promote neonatal weight increases in very low birth weight infants (123). Further that probiotics were more efficatious when administered with breast milk and or an infant formula, and consumed for <6 weeks, provided in a dose of ~109 CFU/day and the probiotic formulation included multiple strains (123).
Prebiotics
Prebiotics, have been defined as non-digestible food components (i.e., non-digestible carbohydrates), are important functional foods that potentiate the action of commensal/beneficial bacteria in the intestines [Table 2; (124)]. Hence the effectiveness of prebiotics is largely dependent on these substances eluding hydrolysis and absorption in the proximal small intestines so as to reach the large bowel (125). Once in the large bowel to be utilized selectively by the commensal group of bacteria (124). These include, Fructooligosaccharide (FOS), Galactooligosaccharide (GOS), inulin, dietary carbohydrates, and Xylooligosaccharide (XOS) are among the most commonly studied prebiotics in clinical studies (126, 127). Interestingly, oligosaccharides in human breast milk have been reported to represent the quintessential prebiotic, as they can readily facilitate the favored growth of the Bifidobacteria and Lactobacilli genera in the large bowel of neonates that have been exclusively breast-fed (128).
The chemical structures of prebiotics prevents metabolism and absorption in the small bowel and leads to bacterial fermentation reactions in the large bowel (i.e., specifically the colon) to form combustible gases, lactate, and SCFAs (i.e., acetate, propionate, butyrate) (129) that have been associated with health benefits (130).
Prebiotics: in vitro and Animal Experimental Data
Strong clinical evidence pertaining to the potential health benefits of prebiotics, result from in vitro and in vivo study models (131–133) in vitro studies have shown that individual specific bacterial species from the Bifidobacteria and Lactobacilli genera will ferment selected prebiotics as defined by the production of SCFAs in an acid environment (134). The mechanism of this selective activity involves factors that include the lowering of colonic pH and the production of metabolites that can inhibit pathobiont growth while simultaneously promoting the growth of probiotic bacteria and the production of antimicrobial effects (135, 136). Studies have shown the preferential administration of prebiotics over probiotics for the selected growth of bacteria in the large bowel (137, 138). For example, the incorporation of oligosaccharides in doses of 5–7 g/day may lead to the proliferation of certain types of bacteria that are generally considered to be beneficial (i.e., Bifidobacteria, Lactobacilli, non-pathogenic E. coli while decreasing Bacteroidaceae) to the detriment of pathobionts; this re-equilibration or intestinal homeostasis of the colonic biotope has been designated as the prebiotic effect (139).
The effect of prebiotics on the proliferation of specific bacterial classes is complex. The interactions with the intestinal microbiome cannot be easily explained by prebiotic compounds acting as exclusive substrates (140). Exploiting intestinal bacterial communities through the introduction of prebiotics has the capacity to indirectly influence beneficial immune responses (141). The production of SCFAs the major products of bacterial fermentation of prebiotics in the absence of oxygen modulates the concentration of SCFAs by down regulating pro-inflammatory mediators by intestinal macrophages (142).
SCFAs such as propionic acid and butyric acid have been reported to inhibit molecular induced expression of adhesion molecules, chemokine formation with concomitant suppression of monocyte/macrophage immune activities and neutrophil recruitment that in combination suggest an anti-inflammatory effect (142). This effect is articulated by butyrate, which is reported to suppress lipopolysaccharide and cytokine-promoted production of pro-inflammatory mediators including TNF-α, IL-6 and nitric oxide while enhancing the release of IL-10 an anti-inflammatory cytokine (143–145). Interestingly SCFAs have been documented to decrease the in vitro adherence of monocytes and lymphocytes to human umbilical vein endothelial cells (146). Furthermore, butyric acid interactions with monocytes, has also been shown to reduce the constitutive and IFN-γ-induced expression of lymphocyte function-associated antigen 3 and intercellular adhesion molecule-1 (147). In addition SCFAs regulate several functions expressed by leukocytes and these include production of a number of cytokines (i.e., TNF-α, IL-2, IL-6, and IL-10), eicosanoids and chemokines (e.g., macrophage chemo-attractant protein-1 and cytokine induce neutrophil chemo-attractant-2) (148).
SCFAs have also been reported to modulate the production of prostaglandin E2 (PGE2) and that this activity stimulates the in vitro production of PGE2 by human monocytes (149). This eicosanoid has been shown to suppress T cell receptor signaling and may play an important role in the resolution of inflammatory responses toward equilibrium (150), redefining it as an anti-inflammatory prostanoid by attenuating the formation of IL-1β and TNF-α by macrophages and Th1 differentiation (151). SCFAs such as acetic acid and propionic acid have been reported to reduce TNF-α formation induced by LPS that have been stimulated by human neutrophils (152). Moreover, reports relative the effects of propionate and butyrate demonstrate an inhibitory expression profile of pro-inflammatory mediators (i.e., TNF-α, CINC-2αβ, NO) from rat neutrophils through the attenuation of NF-kB activation (153). Prebiotics such as FOS, GOS, inulin and resistant starch have been demonstrated to affect microbial genera (indigenous Bifidobacteria, Lactobacilli, Faecalibacteria) (154, 155) that progress the up-regulation of Tregs by different mechanistic pathways and in turn re-regulate pro-inflammatory activity (94).
Prebiotics influencing immune modulation by exploiting the metabolic activities of commensal intestinal bacteria where experimental murine models suggest that such activity can significantly reduce the precancerous colonic lesions present as aberrant crypt foci (156). Other studies have documented that prebiotics augment the bioavailability and absorption of minerals such as calcium and may affect the metabolism of other minerals namely, magnesium, iron, and zinc (157); stimulating the reduction of endogenous carcinogens such as sialomucin (158); and reducing the growth of tumors in murine models of carcinogenesis (159).
Prebiotics and Human Studies
Clinical studies have confirmed that FOS has a bifidogenic effect on the human large bowel (i.e., colon) and the endogenous intestinal microbiome (160, 161). Moreover, a number of clinical studies have also demonstrated the bifidogenic effect for inulin-type fructans (162). These studies have effectively demonstrated the growth promoting activity of prebiotics and the targeting of the bacterial genera Lactobacilli and Bifidobacteria (163). The overall implications from the clinical data is that the proposed bifidogenic effect is not simply attributed to prebiotics as preferential substrates for the commensal bacterial cohort, rather that prebiotic substances can interact with other commensal bacteria and may be subject to associations with environmental fluctuations including variations in luminal pH, and other unknown factors in order to achieve a net bifidogenic effect in the large bowel (164). In a clinical study with children attending day care daily supplementation with oligofructose (dose: 2 g/day over 3 weeks) was associated with significantly fewer episodes of diarrhea, flatulence, vomiting and fever, and with reductions in the level of pathobionts from the Clostridia and Staphylococci genera and with increases in Bifidobacteria genus (165). Infants supplemented with formula that included a prebiotic mixture (dose: 8 g/L) achieved normal growth and stool features that were more similar to those of breast-fed infants and in comparison with infants fed an un-supplemented formula (166). Others have reported no effect for oligofructose-supplemented infant cereal (167). Studies on the effect prebiotics have on the intestinal microbiome have reported that the consumption, daily, of whole-grain-wheat was observed to provide a pronounced prebiotic effect on the composition of the intestinal microbiota, positing that this activity may contribute toward beneficial physiological effects (168). Other studies have reported that prebiotics in the form of a whole-grain-maize augmented cereal (dose: 48/day) induced a bifidogenic modulatory effect on the intestinal microbiota (169). No significant changes were observed in serum lipid profiles, blood glucose levels or fecal output measures (169). Celiac disease is an autoimmune inflammatory problem characterized by the interplay between the host's genetic factors and gluten as the environmental trigger (170).
Clinical studies have posited and reported that the concentration of SCFAs in blood and more importantly in the intestines may predispose to or prevent pathological conditions such as IBD (171), cancer and obesity (172), diabetes (173), and symptoms such as diarrhea (174). Interestingly, in a study with healthy physically active subjects a synbiotic supplement, increased fecal Lactobacillus paracasei with no appreciable effect on mucosal immunity (175). This latter outcome is not surprising given that in healthy individuals mucosal immunity should be in equilibrium.
Clinical studies administering prebiotics to encourage intestinal microbiome shifts toward the production of increased levels of SCFAs remains contentious though with clinical studies, showing a benefit with beneficial microbial shifts and immune function (94, 176, 177) and others not (178).
Bacteriophages
Bacteriophages are ancient dependent infective agents that have existed on this planet for millennia, were discovered about a century ago (179), and have been posited to participate in immunological activities of the host (180). The predominant intestinal phage load harbored by adult healthy individuals has been reported to be a member of the order Caudovirales, a double stranded DNA bacteriophage (from the families Podoviridae, Siphoviridae, and Myoviridae), single stranded DNA bacteriophages (from the families Microviridae and Inoviridae) and RNA viruses (181–184). Viruses that reside in the intestines are comprised predominantly of bacteriophages (including prophages) and to a lesser degree eukaryotic viruses, reported to be stably integrated into bacterial genomes (parasitized phase) and lytic phages which can infect and lyse bacteria and hence release virus particles (185). Bacteriophages can hence influence the bacterial structure of the microbiome through a parasitic or lytic phase of bacterial cells and show the greatest abundance and diversity in the intestines of healthy adults (186).
Recent reports show that the neonate is exposed to a diverse range of bacteriophages at birth (187), an exposure that together with bacteria could herald a further consideration of how immunological and metabolic tolerance is achieved in early life. Bacteriophage directed control of behaviors of how bacteria colonize and survive in different anatomical sites encourage developmental properties that helps establish commensal populations that reduces the risk of disease (182). Studies report that bacteriophages may be involved in important functions in human immunity by defending the intestinal epithelial barrier and mucosal tissue from infections by pathobionts (188).
Bacteriophages influencing the stability of the intestinal microbiome cohort indirectly shape the immunological and metabolic functions of intestinal immunity, especially as reported for the direct action on T and B cells (189). Bacteriophages have been reported to adhere to the mucus layer of the intestines, and that the interaction with glycan residues from mucin glycoproteins affords the viruses a niche that is in close proximity to the intestinal epithelial layer and mucosal surfaces (190). Furthermore, it is reported that when phages exhibit muco-adherent properties, such actions can influence the activity of the innate and cell mediated immune systems (191). Moreover, temperate phages that positively influence host immunity could do so by selective screening of the commensal bacterial cohort (191).
In concert with peptides that exhibit antimicrobial properties in the mucus layer there is demonstrated a control over the density of the commensal bacteria that can occupy the mucus layer. This effective local control provides a protective barrier against commensal pathobionts while establishing a symbiotic relationship with the host; an immunological defense mechanism not derived from the host.
In the pre-antibiotic era, the use of bacteriophages to treat infections was an early example of the immunogenicity effect that was attributed to bacteriophages (192). A number of studies have documented the immune-modulatory effects of bacteriophages. These have included, (i) an anti-bacteriophage immune response, whereby exposure to bacteriophages in the circulation have induced strong anti-bacteriophage humoral responses resulting in swift and proficient neutralization and clearance of the phage on subsequent exposures to the virus (193, 194); (ii) bacteriophage-mediated re-regulation of the over-production of reactive oxygen species by phagocytes is a critical immunological effect that significantly contributed to the favorable effects of bacteriophage therapy in patients diagnosed with a life-threatening condition such as sepsis (193); and iii) a chemically modified phage with high affinity ligands for cell specific receptors has been reported to induce humoral and cellular immune responses regressing solid tumors in murine models (193, 194).
Certain Eastern European countries with bacteriophage treatment centers, routinely administer phage therapies for the prophylactic and therapeutic treatment of bacterial infections for ulcers/wounds, septicemia, UTIs, MRSA and others (195). Reports note though that the state of the immune system can determine what type of an effect the virome may have that then determines the interactions that ensue with host immunity. This is particularly relevant with inflammatory diseases such Crohn's Disease and Ulcerative colitis where the intestinal virome has been deemed to be abnormally altered in terms of increased bacteriophage richness and then correlated to decreased bacterial diversity (196). Moreover the bacteriophage changes were Inflammatory Bowel Disease (IBD) specific. Such specific effects have been consistent with studies that have reported decreases in diversity and abundance of the bacterial phyla (197). Specifically decreased Bacteroidetes and Firmicutes phyla from the fecal samples, were reported associated with IBD (197).
It is hence very probable that bacteriophages may control and cull the bacterial population in the intestines with substantial turnover that as such significantly influences bacterial diversity/abundance and metabolism in the intestines. Furthermore, increases in the intestinal virome due to decreases in commensal bacterial diversity and abundance could also explain the inefficacy that has been reported with certain probiotic treatments for IBD, especially in patients diagnosed with Crohn's Disease.
Summary
The commensal microbial cohort functions to develop and establish the host's immune system (i.e., mucosal and cell mediated) in order to promote immunological and metabolic tolerance. The microbiome complement effect is mediated by bacteriological factors that stimulate cells of the host; and these factors operate across a diverse set of host receptors and cellular molecular targets that are expressed on the surface or within the cells of the host. The host receptors that interact with microbial factors include numerous operators such as pattern recognition receptors (i.e., TLRs), receptors such as C-type lectin and nucleotide oligomerization and RIG-1-like receptors, which can sense important microbial macromolecular constituents such as nucleic acids (i.e., DNA, RNA), proteins and cell wall components (198).
Specifically, probiotics and prebiotics are reported to have positive immuno-equilibrium restorative effects. An increasingly supported posit is that bacteria such as those from the probiotic genera of Bifidobacteria and Lactobacilli can participate in immune-regulation and do so by inducing regulatory T cells (199, 200). The beneficial immune-modulatory effects are elicited across several molecules, that include microbial cell walls, peptidoglycan, and exopolysaccharides, through interactions with specific host cell receptors (i.e., Toll-Like Receptor (TLR)-2 and TLR-4) (200). Relative to prebiotics, these compounds encourage the intestinal microbiome production of SCFAs, which have a central role in intestinal immunogenicity (201). It is generally accepted that SCFAs such as acetate propionate and butyrate can interact with local intestinal epithelial and mucosal immune tissues as well as having significant epigenetic effects (202), and are essential participants in health and disease. Bacteriophages seem to have a two-way role in the intestines that balances health and disease; from determinants of decreased abundance and diversity of the commensal microbiome to the control of bacterial diversity and abundance as a necessary factor to control pathobiont insults that balances the risk of disease and health. Bacteriophages, it would seem display immune-suppressive characteristics in the intestines across involvement in a number of immune related areas such as the control of inflammation and autoimmune reactions (189).
Author Contributions
LV conception and design of the manuscript. LV, GV, and SH read, amended, and approved the final version of the manuscript.
Conflict of Interest Statement
LV has received National Institute of Complementary Medicine and National Health and Medical Research Council of Australia competitive funding and Industry support for research into probiotics. LV and SH participate in research on probiotics in Medlab Clinical's research laboratory facility in Sydney, Australia.
The remaining author declares that the research was conducted in the absence of any commercial or financial relationships that could be construed as a potential conflict of interest.
References
1. Gest H. The discovery of microorganisms by Robert Hooke and Antoni Van Leeuwenhoek, fellows of the Royal Society. Notes Rec R Soc Lond. (2004) 58:187–201. doi: 10.1098/rsnr.2004.0055
2. Lewis KL, Reizis B. Dendritic cells: arbiters of immunity and immunological tolerance. Cold Spring Harbor Perspect Biol. (2012) 4:a007401.
3. Probst HC, Muth S, Schild H. Regulation of the tolerogenic function of steady-state DCs. Eur J Immunol. (2014) 44:927–33. doi: 10.1002/eji.201343862
4. Hart AL, Stagg AJ, Frame M, Graffner H, Glise H, Falk P, et al. The role of the gut flora in health and disease, and its modification as therapy. Aliment Pharmacol Ther. (2002) 16:1383–93. doi: 10.1046/j.1365-2036.2002.01310.x
5. Tissier H. Le bacterium coli et la reaction chromophile d'Escherich. Crit Rev Soc Biol. (1899) 51:943–945.
6. Funkhouser LJ, Bordenstein SR. Mom knows best: the universality of maternal microbial transmission. PLoS Biol. (2013) 11:e1001631. doi: 10.1371/journal.pbio.1001631
7. Mackowiak PA. Recycling metchnikoff: probiotics, the intestinal microbiome and the quest for long life. Front Pub Health (2013) 1:52. doi: 10.3389/fpubh.2013.00052
8. Tissier H. Traitement des infections intestinales par la methode de la flore bacterienne de l'intestin. Crit Rev Soc Biol. (1906) 60:359–361.
9. Kaufmann SH. Immunology's foundation: the 100-year anniversary of the Nobel Prize to Paul Ehrlich and Elie Metchnikoff. Nat Immunol. (2008) 9:705–12. doi: 10.1038/ni0708-705
10. Aumeunier A, Grela F, Ramadan A, Pham Van L, Bardel E, Gomez Alcala A, et al. Systemic Toll-like receptor stimulation suppresses experimental allergic asthma and autoimmune diabetes in NOD mice. PLoS ONE (2010) 5:e11484. doi: 10.1371/journal.pone.0011484
11. Parkin J, Cohen B. An overview of the immune system. Lancet (2001) 357:1777–89.doi: 10.1016/S0140-6736(00)04904-7
12. Delves PJ, Roitt IM. The immune system. Second of two parts. N Engl J Med. (2000) 343:108–17. doi: 10.1056/NEJM200007133430207
13. Freeley S, Kemper C, Le Friec G. The “ins and outs” of complement-driven immune responses. Immunol Rev. (2016) 274:16–32. doi: 10.1111/imr.12472
14. Cong J, Zhang X. How human microbiome talks to health and disease. Eur J Clin Microbiol Infect Dis. (2018) 37:1595–601. doi: 10.1007/s10096-018-3263-1
15. McDermott AJ, Huffnagle GB. The microbiome and regulation of mucosal immunity. Immunology (2014) 142:24–31. doi: 10.1111/imm.12231
16. Vitetta L, Hall S, Linnane AW. Live probiotic cultures and the gastrointestinal tract: symbiotic preservation of tolerance whilst attenuating pathogenicity. Front Cell Infect Microbiol. (2014) 4:143. doi: 10.3389/fcimb.2014.00143
17. Vitetta L, Saltzman ET, Thomsen M, Nikov T, Hall S. Adjuvant probiotics and the intestinal microbiome: enhancing vaccines and immunotherapy outcomes. Vaccines (2017) 5: E50. doi: 10.3390/vaccines5040050
18. Hooks KB, O'Malley MA. Dysbiosis and its discontents. MBio (2017) 8:e01492–17. doi: 10.1128/mBio.01492-17
19. Haenel H. Some rules in the ecology of the intestinal microflora of man. J Appl Bacteriol. (1961) 24:242–51.
20. Stecher B, Maier L, Hardt WD. 'Blooming' in the gut: how dysbiosis might contribute to pathogen evolution. Nat Revi Microbiol. (2013) 11:277–84. doi: 10.1038/nrmicro2989
21. Arumugam M, Raes J, Pelletier E, Le Paslier D, Yamada T, Mende DR, et al. Enterotypes of the human gut microbiome. Nature (2011) 473:174–80. doi: 10.1038/nature09944
22. Costea PI, Hildebrand F, Arumugam M, Backhed F, Blaser MJ, Bushman FD, et al. Enterotypes in the landscape of gut microbial community composition. Nat Microbiol. (2018) 3:8–16. doi: 10.1038/s41564-017-0072-8
23. Ley RE, Turnbaugh PJ, Klein S, Gordon JI. Microbial ecology: human gut microbes associated with obesity. Nature (2006) 444:1022–3. doi: 10.1101/cshperspect.a007401
24. Srikanth CV, McCormick BA. Interactions of the intestinal epithelium with the pathogen and the indigenous microbiota: a three-way crosstalk. Interdisc Perspect Infect Dis. (2008) 2008:626827. doi: 10.1155/2008/626827
25. Porter EM, Bevins CL, Ghosh D, Ganz T. The multifaceted Paneth cell. Cell Mol Life Scie. (2002) 59:156–70. doi: 10.1007/s00018-002-8412-z
26. Tan DW, Barker N. Intestinal stem cells and their defining niche. Curr Top Develop Biol. (2014) 107:77–107. doi: 10.1016/B978-0-12-416022-4.00003-2
27. Rumio C, Sommariva M, Sfondrini L, Palazzo M, Morelli D, Vigano L, et al. Induction of Paneth cell degranulation by orally administered Toll-like receptor ligands. J Cell Physiol. (2012) 227:1107–13. doi: 10.1002/jcp.22830
28. Hooper LV, Gordon JI. Commensal host-bacterial relationships in the gut. Science (2001) 292:1115–8. doi: 10.1126/science.1058709
29. Peterson DA, Planer JD, Guruge JL, Xue L, Downey-Virgin W, Goodman AL, et al. Characterizing the interactions between a naturally primed immunoglobulin A and its conserved Bacteroides thetaiotaomicron species-specific epitope in gnotobiotic mice. J Biol Chem. (2015) 290:12630–49. doi: 10.1074/jbc.M114.633800
30. Morhardt TL, Hayashi A, Kao JY, Kamada N. Regional control of regulatory immune cells in the intestine. Curr Pathobiol Rep. (2018) 6:29–34. doi: 10.1007/s40139-018-0156-z
31. Hase K, Kawano K, Nochi T, Pontes GS, Fukuda S, Ebisawa M, et al. Uptake through glycoprotein 2 of FimH(+) bacteria by M cells initiates mucosal immune response. Nature (2009) 462:226–30. doi: 10.1038/nature08529
32. Kimura S. Molecular insights into the mechanisms of M-cell differentiation and transcytosis in the mucosa-associated lymphoid tissues. Anatom Sci Int. (2018) 93:23–34. doi: 10.1007/s12565-017-0418-6
33. Corr SC, Gahan CC, Hill C. M-cells: origin, morphology and role in mucosal immunity and microbial pathogenesis. FEMS Immunol Med Microbiol. (2008) 52:2–12. doi: 10.1111/j.1574-695X.2007.00359.x
34. Kulkarni N, Pathak M, Lal G. Role of chemokine receptors and intestinal epithelial cells in the mucosal inflammation and tolerance. J Leukoc Biol. (2017) 101:377–94. doi: 10.1189/jlb.1RU0716-327R
35. Mowat AM. Anatomical basis of tolerance and immunity to intestinal antigens. Nat Rev Immunol. (2003) 3:331–41. doi: 10.1038/nri1057
36. Neutra MR, Mantis NJ, Kraehenbuhl JP. Collaboration of epithelial cells with organized mucosal lymphoid tissues. Nat Immunol. (2001) 2:1004–9. doi: 10.1038/ni1101-1004
37. Hayashi A, Sato T, Kamada N, Mikami Y, Matsuoka K, Hisamatsu T, et al. A single strain of Clostridium butyricum induces intestinal IL-10-producing macrophages to suppress acute experimental colitis in mice. Cell Host Microb. (2013) 13:711–22. doi: 10.1016/j.chom.2013.05.013
38. Man AL, Gicheva N, Regoli M, Rowley G, De Cunto G, Wellner N, et al. CX3CR1+ Cell-mediated salmonella exclusion protects the intestinal mucosa during the initial stage of infection. J Immunol. (2017) 198:335–43. doi: 10.4049/jimmunol.1502559
39. Regoli M, Bertelli E, Gulisano M, Nicoletti C. The multifaceted personality of intestinal CX3CR1(+) macrophages. Trends Immunol. (2017) 38:879–87. doi: 10.1016/j.it.2017.07.009
40. Maslowski KM, Mackay CR. Diet, gut microbiota and immune responses. Nat Immunol. (2011) 12:5–9. doi: 10.1038/ni0111-5
41. Atarashi K, Umesaki Y, Honda K. Microbiotal influence on T cell subset development. Sem Immunol. (2011) 23:146–53. doi: 10.1016/j.smim.2011.01.010
42. Hachimura S, Totsuka M, Hosono A. Immunomodulation by food: impact on gut immunity and immune cell function. Biosci Biotechnol Biochem. (2018) 82:584–99. doi: 10.1080/09168451.2018.1433017
43. Kurashima Y, Kiyono H. Mucosal ecological network of epithelium and immune cells for gut homeostasis and tissue healing. Ann Rev Immunol. (2017) 35:119–47. doi: 10.1146/annurev-immunol-051116-052424
44. Gaboriau-Routhiau V, Rakotobe S, Lecuyer E, Mulder I, Lan A, Bridonneau C, et al. The key role of segmented filamentous bacteria in the coordinated maturation of gut helper T cell responses. Immunity (2009) 31:677–89. doi: 10.1016/j.immuni.2009.08.020
45. Atarashi K, Nishimura J, Shima T, Umesaki Y, Yamamoto M, Onoue M, et al. ATP drives lamina propria T(H)17 cell differentiation. Nature (2008) 455:808–12. doi: 10.1038/nature07240
46. Ivanov, II, Frutos Rde L, Manel N, Yoshinaga K, Rifkin DB, Sartor RB, et al. Specific microbiota direct the differentiation of IL-17-producing T-helper cells in the mucosa of the small intestine. Cell Host Microb. (2008) 4:337–49. doi: 10.1016/j.chom.2008.09.009
47. Wu HJ, Ivanov, II, Darce J, Hattori K, Shima T, Umesaki Y, et al. Gut-residing segmented filamentous bacteria drive autoimmune arthritis via T helper 17 cells. Immunity (2010) 32:815–27. doi: 10.1016/j.immuni.2010.06.001
48. Abeles SR, Pride DT. Molecular bases and role of viruses in the human microbiome. J Mol Biol. (2014) 426:3892–906. doi: 10.1016/j.jmb.2014.07.002
49. Telesford KM, Yan W, Ochoa-Reparaz J, Pant A, Kircher C, Christy MA, et al. A commensal symbiotic factor derived from Bacteroides fragilis promotes human CD39(+)Foxp3(+) T cells and Treg function. Gut Microb. (2015) 6:234–42. doi: 10.1080/19490976.2015.1056973
50. Lopetuso LR, Scaldaferri F, Petito V, Gasbarrini A. Commensal clostridia: leading players in the maintenance of gut homeostasis. Gut Pathog. (2013) 5:23. doi: 10.1186/1757-4749-5-23
51. Donaldson GP, Ladinsky MS, Yu KB, Sanders JG, Yoo BB, Chou WC, et al. Gut microbiota utilize immunoglobulin A for mucosal colonization. Science (2018) 360:795–800. doi: 10.1126/science.aaq0926
52. Mohamadzadeh M, Olson S, Kalina WV, Ruthel G, Demmin GL, Warfield KL, et al. Lactobacilli activate human dendritic cells that skew T cells toward T helper 1 polarization. Proc Natl Acad Sci USA. (2005) 102:2880–5. doi: 10.1073/pnas.0500098102
53. Fink LN, Zeuthen LH, Ferlazzo G, Frokiaer H. Human antigen-presenting cells respond differently to gut-derived probiotic bacteria but mediate similar strain-dependent NK and T cell activation. FEMS Immunol Med Microbiol. (2007) 51:535–46. doi: 10.1111/j.1574-695X.2007.00333.x
54. Young SL, Simon MA, Baird MA, Tannock GW, Bibiloni R, Spencely K, et al. Bifidobacterial species differentially affect expression of cell surface markers and cytokines of dendritic cells harvested from cord blood. Clin Diagnost Lab Immunol. (2004) 11:686–90. doi: 10.1128/CDLI.11.4.686-690.2004
55. Zeuthen LH, Christensen HR, Frokiaer H. Lactic acid bacteria inducing a weak interleukin-12 and tumor necrosis factor alpha response in human dendritic cells inhibit strongly stimulating lactic acid bacteria but act synergistically with gram-negative bacteria. Clin Vaccine Immunol. (2006) 13:365–75. doi: 10.1128/CVI.13.3.365-375.2006
56. Borchers AT, Selmi C, Meyers FJ, Keen CL, Gershwin ME. Probiotics and immunity. J Gastroenterol. (2009) 44:26–46. doi: 10.1007/s00535-008-2296-0
57. Smits HH, Engering A, van der Kleij D, de Jong EC, Schipper K, van Capel TM, et al. Selective probiotic bacteria induce IL-10-producing regulatory T cells in vitro by modulating dendritic cell function through dendritic cell-specific intercellular adhesion molecule 3-grabbing nonintegrin. J Allergy Clin Immunol. (2005) 115:1260–7. doi: 10.1016/j.jaci.2005.03.036
58. Niers LE, Hoekstra MO, Timmerman HM, van Uden NO, de Graaf PM, Smits HH, et al. Selection of probiotic bacteria for prevention of allergic diseases: immunomodulation of neonatal dendritic cells. Clin Exp Immunol. (2007) 149:344–52. doi: 10.1111/j.1365-2249.2007.03421.x
59. Niers LE, Timmerman HM, Rijkers GT, van Bleek GM, van Uden NO, Knol EF, et al. Identification of strong interleukin-10 inducing lactic acid bacteria which down-regulate T helper type 2 cytokines. Clin Exp Allergy (2005) 35:1481–9. doi: 10.1111/j.1365-2222.2005.02375.x
60. Braat H, van den Brande J, van Tol E, Hommes D, Peppelenbosch M, van Deventer S. Lactobacillus rhamnosus induces peripheral hyporesponsiveness in stimulated CD4+ T cells via modulation of dendritic cell function. Am J Clin Nutrit. (2004) 80:1618–25. doi: 10.1093/ajcn/80.6.1618
61. Rigby RJ, Knight SC, Kamm MA, Stagg AJ. Production of interleukin (IL)-10 and IL-12 by murine colonic dendritic cells in response to microbial stimuli. Clin Exp Immunol. (2005) 139:245–56. doi: 10.1111/j.1365-2249.2004.02674.x
62. Weise C, Zhu Y, Ernst D, Kuhl AA, Worm M. Oral administration of Escherichia coli Nissle 1917 prevents allergen-induced dermatitis in mice. Exp Dermatol. (2011) 20:805–9. doi: 10.1111/j.1600-0625.2011.01326.x
63. Kim JY, Choi YO, Ji GE. Effect of oral probiotics (Bifidobacterium lactis AD011 and Lactobacillus acidophilus AD031) administration on ovalbumin-induced food allergy mouse model. J Microbiol Biotechnol. (2008) 18:1393–400.
64. So JS, Lee CG, Kwon HK, Yi HJ, Chae CS, Park JA, et al. Lactobacillus casei potentiates induction of oral tolerance in experimental arthritis. Mol Immunol. (2008) 46:172–80. doi: 10.1016/j.molimm.2008.07.038
65. Hacini-Rachinel F, Gheit H, Le Luduec JB, Dif F, Nancey S, Kaiserlian D. Oral probiotic control skin inflammation by acting on both effector and regulatory T cells. PLoS ONE (2009) 4:e4903. doi: 10.1371/journal.pone.0004903
66. Feleszko W, Jaworska J, Rha RD, Steinhausen S, Avagyan A, Jaudszus A, et al. Probiotic-induced suppression of allergic sensitization and airway inflammation is associated with an increase of T regulatory-dependent mechanisms in a murine model of asthma. Clin Exp Allergy (2007) 37:498–505. doi: 10.1111/j.1365-2222.2006.02629.x
67. Karimi K, Inman MD, Bienenstock J, Forsythe P. Lactobacillus reuteri-induced regulatory T cells protect against an allergic airway response in mice. Am J Respir Critic Care Med. (2009) 179:186–93. doi: 10.1164/rccm.200806-951OC
68. Hoarau C, Lagaraine C, Martin L, Velge-Roussel F, Lebranchu Y. Supernatant of Bifidobacterium breve induces dendritic cell maturation, activation, and survival through a Toll-like receptor 2 pathway. J Allergy Clin Immunol. (2006) 117:696–702. doi: 10.1016/j.jaci.2005.10.043
69. Forsythe P, Inman MD, Bienenstock J. Oral treatment with live Lactobacillus reuteri inhibits the allergic airway response in mice. Am J Respir Critic Care Med. (2007) 175:561–9. doi: 10.1164/rccm.200606-821OC
70. Kim CH. Immune regulation by microbiome metabolites. Immunology (2018) 154:220–9. doi: 10.1111/imm.12930
71. Rooks MG, Garrett WS. Gut microbiota, metabolites and host immunity. Nat Rev Immunol. (2016) 16:341–52. doi: 10.1038/nri.2016.42
72. Pagnini C, Saeed R, Bamias G, Arseneau KO, Pizarro TT, Cominelli F. Probiotics promote gut health through stimulation of epithelial innate immunity. Proc Natl Acad Sci USA. (2010) 107:454–9. doi: 10.1073/pnas.0910307107
73. Morrison DJ, Preston T. Formation of short chain fatty acids by the gut microbiota and their impact on human metabolism. Gut Microb. (2016) 7:189–200. doi: 10.1080/19490976.2015.1134082
74. Hemalatha R, Ouwehand AC, Saarinen MT, Prasad UV, Swetha K, Bhaskar V. Effect of probiotic supplementation on total Lactobacilli, bifidobacteria and short chain fatty acids in 2-5-year-old children. Microb Ecol Health Dis. (2017) 28:1298340. doi: 10.1080/16512235.2017.1298340
76. Morelli L, Capurso L. FAO/WHO guidelines on probiotics: 10 years later. J Clin Gastroenterol. (2012) 46(Suppl.):S1–2. doi: 10.1097/MCG.0b013e318269fdd5
77. Hidalgo-Cantabrana C, Delgado S, Ruiz L, Ruas-Madiedo P, Sanchez B, Margolles A. Bifidobacteria and their health-promoting effects. Microbiol Spectr. (2017) 5. doi: 10.1128/microbiolspec.BAD-0010-2016
78. Insoft RM, Sanderson IR, Walker WA. Development of immune function in the intestine and its role in neonatal diseases. Pediatr Clin North Am. (1996) 43:551–71. doi: 10.1016/S0031-3955(05)70420-X
79. Nowak A, Paliwoda A, Blasiak J. Anti-proliferative, pro-apoptotic and anti-oxidative activity of Lactobacillus and Bifidobacterium strains: a review of mechanisms and therapeutic perspectives. Critic Rev Food Sci Nutr. (2018). doi: 10.1080/10408398.2018.1494539. [Epub ahead of print].
80. Mu Q, Tavella VJ, Luo XM. Role of Lactobacillus reuteri in human health and diseases. Front Microbiol. (2018) 9:757. doi: 10.3389/fmicb.2018.00757
81. Christensen HR, Frokiaer H, Pestka JJ. Lactobacilli differentially modulate expression of cytokines and maturation surface markers in murine dendritic cells. J Immunol. (2002) 168:171–8. doi: 10.4049/jimmunol.168.1.171
82. Hardy H, Harris J, Lyon E, Beal J, Foey AD. Probiotics, prebiotics and immunomodulation of gut mucosal defences: homeostasis and immunopathology. Nutrients (2013) 5:1869–912. doi: 10.3390/nu5061869
83. Llewellyn A, Foey A. Probiotic modulation of innate cell pathogen sensing and signaling events. Nutrients (2017) 9:E1156. doi: 10.3390/nu9101156
84. Briskey D, Heritage M, Jaskowski LA, Peake J, Gobe G, Subramaniam VN, et al. Probiotics modify tight-junction proteins in an animal model of nonalcoholic fatty liver disease. Ther Adv Gastroenterol. (2016) 9:463–72. doi: 10.1177/1756283X16645055
85. Goldin BR, Gorbach SL. Clinical indications for probiotics: an overview. Clin Infect Dis. (2008) 46(Suppl. 2):S96–100; discussion: S144–51. doi: 10.1086/523333
86. Vanderhoof JA, Young RJ. The role of probiotics in the treatment of intestinal infections and inflammation. Curr Opin Gastroenterol. (2001) 17:58–62.
87. Vitetta L, Briskey D, Alford H, Hall S, Coulson S. Probiotics, prebiotics and the gastrointestinal tract in health and disease. Inflammopharmacology (2014) 22:135–54. doi: 10.1007/s10787-014-0201-4
88. Bringiotti R, Ierardi E, Lovero R, Losurdo G, Di Leo A, Principi M. Intestinal microbiota: the explosive mixture at the origin of inflammatory bowel disease? World J Gastroint Pathophysiol. (2014) 5:550–9. doi: 10.4291/wjgp.v5.i4.550
89. Wasilewski A, Zielinska M, Storr M, Fichna J. Beneficial effects of probiotics, prebiotics, synbiotics, and psychobiotics in inflammatory bowel disease. Inflamm Bowel Dis. (2015) 21:1674–82. doi: 10.1097/MIB.0000000000000364
90. Vulevic J, Drakoularakou A, Yaqoob P, Tzortzis G, Gibson GR. Modulation of the fecal microflora profile and immune function by a novel trans-galactooligosaccharide mixture (B-GOS) in healthy elderly volunteers. Am J Clin Nutr. (2008) 88:1438–46. doi: 10.3945/ajcn.2008.26242
91. Depeint F, Tzortzis G, Vulevic J, I'Anson K, Gibson GR. Prebiotic evaluation of a novel galactooligosaccharide mixture produced by the enzymatic activity of Bifidobacterium bifidum NCIMB 41171, in healthy humans: a randomized, double-blind, crossover, placebo-controlled intervention study. Am J Clin Nutr. (2008) 87:785–91. doi: 10.1093/ajcn/87.3.785
92. Moro G, Arslanoglu S, Stahl B, Jelinek J, Wahn U, Boehm G. A mixture of prebiotic oligosaccharides reduces the incidence of atopic dermatitis during the first six months of age. Archiv Dis Child. (2006) 91:814–9. doi: 10.1136/adc.2006.098251
93. de Moura PN, Rosario Filho NA. The use of prebiotics during the first year of life for atopy prevention and treatment. Immun Inflamm Dis. (2013) 1:63–9. doi: 10.1002/iid3.8
94. Bassaganya-Riera J, DiGuardo M, Viladomiu M, de Horna A, Sanchez S, Einerhand AW, et al. Soluble fibers and resistant starch ameliorate disease activity in interleukin-10-deficient mice with inflammatory bowel disease. J Nutr. (2011) 141:1318–25. doi: 10.3945/jn.111.139022
95. Ye MB, Lim BO, Dietary pectin regulates the levels of inflammatory cytokines and immunoglobulins in interleukin-10 knockout mice. J Agric Food Chem. (2010) 58:11281–6. doi: 10.1021/jf103262s
96. Kumar R, Dhanda S. Mechanistic insight of probiotics derived anticancer pharmaceuticals: a road forward for cancer therapeutics. Nutr Cancer (2017) 69:375–80. doi: 10.1080/01635581.2017.1267773
97. Konstantinov SR, Kuipers EJ, Peppelenbosch MP. Functional genomic analyses of the gut microbiota for CRC screening. Nat Rev Gastroenterol Hepatol. (2013) 10:741–5. doi: 10.1038/nrgastro.2013.178
98. Capurso G, Marignani M, Delle Fave G. Probiotics and the incidence of colorectal cancer: when evidence is not evident. Digest Liver Dis. (2006) 38(Suppl. 2):S277–82. doi: 10.1016/S1590-8658(07)60010-3
99. Pool-Zobel BL. Inulin-type fructans and reduction in colon cancer risk: review of experimental and human data. Br J Nutr. (2005) 93(Suppl. 1):S73–90. doi: 10.1079/BJN20041349
100. Antza C, Stabouli S, Kotsis V. Gut microbiota in kidney disease and hypertension. Pharmacol. Res. (2018) 130:198–203. doi: 10.1016/j.phrs.2018.02.028
101. He J, Zhang F, Han Y. Effect of probiotics on lipid profiles and blood pressure in patients with type 2 diabetes: a meta-analysis of RCTs. Medicine (2017) 96:e9166. doi: 10.1097/MD.0000000000009166
102. Hungin APS, Mitchell CR, Whorwell P, Mulligan C, Cole O, Agreus L, et al. Systematic review: probiotics in the management of lower gastrointestinal symptoms - an updated evidence-based international consensus. Aliment Pharmacol Ther. (2018) 47:1054–1070. doi: 10.1111/apt.14539
103. Sun L, Liu H, Jiang H, Wei M, Liang S, Wang M, et al. Macrophages are involved in gut bacterial translocation and reversed by lactobacillus in experimental uremia. Digest Dis Sci. (2016) 61:1534–44. doi: 10.1007/s10620-015-3950-z
104. Goldenberg JZ, Yap C, Lytvyn L, Lo CK, Beardsley J, Mertz D, et al. Probiotics for the prevention of Clostridium difficile-associated diarrhea in adults and children. Cochr Database Syst Rev. (2017) 12:Cd006095. doi: 10.1002/14651858.CD006095.pub3
105. Hassan H, Rompola M, Glaser AW, Kinsey SE, Phillips RS. Systematic review and meta-analysis investigating the efficacy and safety of probiotics in people with cancer. Support Care Cancer (2018) 26:2503–9. doi: 10.1007/s00520-018-4216-z
106. Hojsak I, Fabiano V, Pop TL, Goulet O, Zuccotti GV, Cokugras FC, et al. Guidance on the use of probiotics in clinical practice in children with selected clinical conditions and in specific vulnerable groups. Acta Paediatr. (2018) 107:927–37. doi: 10.1111/apa.14270
107. Laursen RP, Hojsak I. Probiotics for respiratory tract infections in children attending day care centers—a systematic review. Eur J Pediatr (2018) 177:979–94. doi: 10.1007/s00431-018-3167-1.
108. Markowiak P, Slizewska K. Effects of probiotics, prebiotics, and synbiotics on human health. Nutrients (2017) 9:1021. doi: 10.3390/nu9091021
109. Lau CS, Chamberlain RS. Probiotics are effective at preventing Clostridium difficile-associated diarrhea: a systematic review and meta-analysis. Int J Gen Med. (2016) 9:27–37. doi: 10.2147/IJGM.S98280
110. Blaabjerg S, Artzi DM, Aabenhus R. Probiotics for the prevention of antibiotic-associated diarrhea in outpatients-A systematic review and meta-analysis. Antibiotics (2017) 6:E21. doi: 10.3390/antibiotics6040021
111. Goldenberg JZ, Lytvyn L, Steurich J, Parkin P, Mahant S, Johnston BC. Probiotics for the prevention of pediatric antibiotic-associated diarrhea. Cochr Database Syst Rev. (2015) Cd004827. doi: 10.1002/14651858.CD004827.pub2
112. Lomax AR, Calder PC. Probiotics, immune function, infection and inflammation: a review of the evidence from studies conducted in humans. Curr Pharmac Des. (2009) 15:1428–518. doi: 10.1017/S0007114508055608
113. Szajewska H, Kolodziej M. Systematic review with meta-analysis: Lactobacillus rhamnosus GG in the prevention of antibiotic-associated diarrhoea in children and adults. Aliment Pharmacol Ther. (2015) 42:1149–57. doi: 10.1111/apt.13404
114. Abraham BP, Quigley EMM. Probiotics in inflammatory bowel disease. Gastroenterol Clin North Am. (2017) 46:769–82. doi: 10.1016/j.gtc.2017.08.003
115. Parker EA, Roy T, D'Adamo CR, Wieland LS. Probiotics and gastrointestinal conditions: an overview of evidence from the Cochrane Collaboration. Nutrition (2018) 45:125–134.e11. doi: 10.1016/j.nut.2017.06.024
116. Clancy R. Immunobiotics and the probiotic evolution. FEMS Immunol Med Microbiol. (2003) 38:9–12. doi: 10.1016/S0928-8244(03)00147-0
117. Schiffrin EJ, Donnet A, Blum S. How can we impact the immune system with pre- and probiotics? Nestle nutrition workshop series. Clin Perform Program. (2005) 10:203–13; discussion: 213–7.
118. Oberhelman RA, Gilman RH, Sheen P, Taylor DN, Black RE, Cabrera L, et al. A placebo-controlled trial of Lactobacillus GG to prevent diarrhea in undernourished Peruvian children. J Pediatr. (1999) 134:15–20. doi: 10.1016/S0022-3476(99)70366-5
119. Guandalini S. Probiotics for prevention and treatment of diarrhea. J Clin Gastroenterol. (2011) 45(Suppl. ):S149–53. doi: 10.1097/MCG.0b013e3182257e98
120. Szajewska H, Kotowska M, Mrukowicz JZ, Armanska M, Mikolajczyk W. Efficacy of Lactobacillus GG in prevention of nosocomial diarrhea in infants. J Pediatr. (2001) 138:361–5. doi: 10.1067/mpd.2001.111321
121. Athalye-Jape G, Rao S, Patole S. Effects of probiotics on experimental necrotizing enterocolitis: a systematic review and meta-analysis. Pediatr Res. (2018) 83:16–22. doi: 10.1038/pr.2017.218
122. Robinson J. Cochrane in context: probiotics for prevention of necrotizing enterocolitis in preterm infants. Evid Based Child Health (2014) 9:672–4. doi: 10.1002/ebch.1977
123. Sun J, Marwah G, Westgarth M, Buys N, Ellwood D, Gray PH. Effects of probiotics on necrotizing enterocolitis, sepsis, intraventricular hemorrhage, mortality, length of hospital stay, and weight gain in very preterm infants: a meta-analysis. Adv Nutr. (2017) 8:749–763. doi: 10.3945/an.116.014605
124. Geurts L, Neyrinck AM, Delzenne NM, Knauf C, Cani PD. Gut microbiota controls adipose tissue expansion, gut barrier and glucose metabolism: novel insights into molecular targets and interventions using prebiotics. Benef Microb. (2014) 5:3–17. doi: 10.3920/BM2012.0065
125. Corzo N, Alonso JL, Azpiroz F, Calvo MA, Cirici M, Leis R, et al. Prebiotics: concept, properties and beneficial effects. Nutricion Hospital. (2015) 31(Suppl. 1):99–118. doi: 10.3305/nh.2015.31.sup1.8715
126. Nawaz A, Bakhsh Javaid A, Irshad S, Hoseinifar SH, Xiong H. The functionality of prebiotics as immunostimulant: evidences from trials on terrestrial and aquatic animals. Fish Shellf Immunol. (2018) 76:272–8. doi: 10.1016/j.fsi.2018.03.004
127. Vieira AT, Teixeira MM, Martins FS. The role of probiotics and prebiotics in inducing gut immunity. Front Immunol. (2013) 4:445. doi: 10.3389/fimmu.2013.00445
128. Le Doare K, Holder B, Bassett A, Pannaraj PS. Mother's milk: a purposeful contribution to the development of the infant microbiota and immunity. Front Immunol. (2018) 9:361. doi: 10.3389/fimmu.2018.00361
129. Holscher HD. Dietary fiber and prebiotics and the gastrointestinal microbiota. Gut Microb. (2017) 8:172–84. doi: 10.1080/19490976.2017.1290756
130. Gibson GR, Hutkins R, Sanders ME, Prescott SL, Reimer RA, Salminen SJ, et al. Expert consensus document: the International Scientific Association for Probiotics and Prebiotics (ISAPP) consensus statement on the definition and scope of prebiotics. Nat Rev Gastroenterol Hepatol. (2017) 14:491–502. doi: 10.1038/nrgastro.2017.75
131. Blaut M. Relationship of prebiotics and food to intestinal microflora. Eur J Nutr. (2002) 41(Suppl. 1):I11–6. doi: 10.1007/s00394-002-1102-7
132. Gibson GR. Dietary modulation of the human gut microflora using the prebiotics oligofructose and inulin. J Nutrit. (1999) 129:1438s−41.
133. Manning TS, Gibson GR. Microbial-gut interactions in health and disease. Prebiotics (2004) 18:287–98. doi: 10.1016/j.bpg.2003.10.008
134. Goh YJ, Klaenhammer TR. Genetic mechanisms of prebiotic oligosaccharide metabolism in probiotic microbes. Annu Rev Food Sci Technol. (2015) 6:137–56. doi: 10.1146/annurev-food-022814-015706
135. Slavin J. Fiber and prebiotics: mechanisms and health benefits. Nutrients (2013) 5:1417–35. doi: 10.3390/nu5041417
136. Verbeke KA, Boobis AR, Chiodini A, Edwards CA, Franck A, Kleerebezem M, et al. Towards microbial fermentation metabolites as markers for health benefits of prebiotics. Nutr Res Rev. (2015) 28:42–66. doi: 10.1017/S0954422415000037
137. Kolida S, Gibson GR. Prebiotic capacity of inulin-type fructans. J Nutr. (2007) 137:2503s−6. doi: 10.1093/jn/137.11.2503S
138. Roberfroid MB. Prebiotics: preferential substrates for specific germs? Am J Clin Nutr. (2001) 73:406s−9. doi: 10.1093/ajcn/73.2.406s
139. Gibson GR, Roberfroid MB. Dietary modulation of the human colonic microbiota: introducing the concept of prebiotics. The Journal of nutrition (1995) 125:1401–12.
140. van Zanten GC, Knudsen A, Roytio H, Forssten S, Lawther M, Blennow A, et al. The effect of selected synbiotics on microbial composition and short-chain fatty acid production in a model system of the human colon. PLoS One (2012) 7e47212. doi: 10.1371/journal.pone.0047212
141. Schley PD, Field CJ. The immune-enhancing effects of dietary fibres and prebiotics. Br J Nutr. (2002) 87(Suppl. 2) S221–30. doi: 10.1079/BJNBJN/2002541
142. Vinolo MA, Rodrigues HG, Nachbar RT, Curi R. Regulation of inflammation by short chain fatty acids. Nutrients (2011) 3:858–76. doi: 10.3390/nu3100858
143. Chakravortty D, Koide N, Kato Y, Sugiyama T, Mu MM, Yoshida T, et al. The inhibitory action of butyrate on lipopolysaccharide-induced nitric oxide production in RAW 264.7 murine macrophage cells. J Endot Res. (2000) 6:243–7. doi: 10.1177/09680519000060030501
144. Hodin R. Maintaining gut homeostasis: the butyrate-NF-kappaB connection. Gastroenterology (2000) 118:798–801. doi: 10.1016/S0016-5085(00)70150-8
145. Park JS, Lee EJ, Lee JC, Kim WK, Kim HS. Anti-inflammatory effects of short chain fatty acids in IFN-gamma-stimulated RAW 264.7 murine macrophage cells: involvement of NF-kappaB and ERK signaling pathways. Int Immunopharmacol. (2007) 7:70–7. doi: 10.1016/j.intimp.2006.08.015
146. Menzel T, Luhrs H, Zirlik S, Schauber J, Kudlich T, Gerke T, et al. Butyrate inhibits leukocyte adhesion to endothelial cells via modulation of VCAM-1. Inflamm Bowel Dis. (2004) 10:122–8. doi: 10.1097/00054725-200403000-00010
147. Bohmig GA, Krieger PM, Saemann MD, Wenhardt C, Pohanka E, Zlabinger GJ. n-butyrate downregulates the stimulatory function of peripheral blood-derived antigen-presenting cells: a potential mechanism for modulating T-cell responses by short-chain fatty acids. Immunology (1997) 92:234–43.
148. Asarat M, Apostolopoulos V, Vasiljevic T, Donkor O. Short-chain fatty acids regulate cytokines and Th17/Treg cells in human peripheral blood mononuclear cells in vitro. Immunol Investig. (2016) 45:205–22. doi: 10.3109/08820139.2015.1122613
149. Cox MA, Jackson J, Stanton M, Rojas-Triana A, Bober L, Laverty M, et al. Short-chain fatty acids act as antiinflammatory mediators by regulating prostaglandin E(2) and cytokines. World J Gastroenterol. (2009) 15:5549–57. doi: 10.3748/wjg.15.5549
150. Wiemer AJ, Hegde S, Gumperz JE, Huttenlocher A. A live imaging cell motility screen identifies prostaglandin E2 as a T cell stop signal antagonist. J Immunol. (2011) 187:3663–70. doi: 10.4049/jimmunol.1100103
151. Birrell MA, Maher SA, Dekkak B, Jones V, Wong S, Brook P, et al. Anti-inflammatory effects of PGE2 in the lung: role of the EP4 receptor subtype. Thorax (2015) 70:740–7. doi: 10.1136/thoraxjnl-2014-206592
152. Tedelind S, Westberg F, Kjerrulf M, Vidal A. Anti-inflammatory properties of the short-chain fatty acids acetate and propionate: a study with relevance to inflammatory bowel disease. World J Gastroenterol. (2007) 13:2826–32. doi: 10.3748/wjg.v13.i20.2826
153. Vinolo MA, Rodrigues HG, Hatanaka E, Sato FT, Sampaio SC, Curi R. Suppressive effect of short-chain fatty acids on production of proinflammatory mediators by neutrophils. J Nutr Biochem. (2011) 22:849–55. doi: 10.1016/j.jnutbio.2010.07.009
154. Flint HJ, Scott KP, Duncan SH, Louis P, Forano E. Microbial degradation of complex carbohydrates in the gut. Gut Microb. (2012) 3:289–306. doi: 10.4161/gmic.19897
155. Porter NT, Martens EC. The critical roles of polysaccharides in gut microbial ecology and physiology. Ann Rev Microb. (2017) 71:349–69. doi: 10.1146/annurev-micro-102215-095316
156. Pool-Zobel BL, Sauer J. Overview of experimental data on reduction of colorectal cancer risk by inulin-type fructans. J Nutr. (2007) 137:2580s−84. doi: 10.1093/jn/137.11.2580S
157. Whisner CM, Castillo LF. Prebiotics, bone and mineral metabolism. Calcif Tissue Int. (2018) 102:443–479. doi: 10.1007/s00223-017-0339-3
158. Satchithanandam S, Cassidy MM, Kharroubi AT, Calvert RJ, Leeds AR, Vahouny GV. Alterations in rat intestinal mucin patterns following luminal infusion of acetylsalicylic acid and prostaglandin derivatives. Digest Dis Sci. (1990) 35:1518–27. doi: 10.1007/BF01540570
159. Rivera-Huerta M, Lizarraga-Grimes VL, Castro-Torres IG, Tinoco-Mendez M, Macias-Rosales L, Sanchez-Bartez F, et al. Functional effects of prebiotic fructans in colon cancer and calcium metabolism in animal models. BioMed Res Int. (2017) 2017:9758982. doi: 10.1155/2017/9758982
160. Ducatelle R, Eeckhaut V, Haesebrouck F, Van Immerseel F. A review on prebiotics and probiotics for the control of dysbiosis: present status and future perspectives. Animal (2015) 9:43–8. doi: 10.1017/S1751731114002584
161. Floch MH. The role of prebiotics and probiotics in gastrointestinal disease. Gastroenterol Clin North Am. (2018) 47:179–91. doi: 10.1016/j.gtc.2017.09.011
162. Meyer D, Stasse-Wolthuis M. The bifidogenic effect of inulin and oligofructose and its consequences for gut health. Eur J Clin Nutr. (2009) 63:1277–89. doi: 10.1038/ejcn.2009.64
163. Shokryazdan P, Faseleh Jahromi M, Navidshad B, Liang JB. Effects of prebiotics on immune system and cytokine expression. Med Microbiol Immunol. (2017) 206:1–9. doi: 10.1007/s00430-016-0481-y
164. Roberfroid M, Gibson GR, Hoyles L, McCartney AL, Rastall R, Rowland I, et al. Prebiotic effects: metabolic and health benefits. Br J Nutr. (2010) 104(Suppl. 2):S1–63. doi: 10.1017/S0007114510003363
165. Waligora-Dupriet AJ, Campeotto F, Nicolis I, Bonet A, Soulaines P, Dupont C, et al. Effect of oligofructose supplementation on gut microflora and well-being in young children attending a day care centre. Int J Food Microbiol. (2007) 113:108–13. doi: 10.1016/j.ijfoodmicro.2006.07.009
166. Ziegler E, Vanderhoof JA, Petschow B, Mitmesser SH, Stolz SI, Harris CL, et al. Term infants fed formula supplemented with selected blends of prebiotics grow normally and have soft stools similar to those reported for breast-fed infants. J Pediat Gastroenterol Nutrit. (2007) 44:359–64. doi: 10.1097/MPG.0b013e31802fca8c
167. Duggan C, Penny ME, Hibberd P, Gil A, Huapaya A, Cooper A, et al. Oligofructose-supplemented infant cereal: 2 randomized, blinded, community-based trials in Peruvian infants. Am J Clin Nutr. (2003) 77:937–42. doi: 10.1093/ajcn/77.4.937
168. Costabile A, Klinder A, Fava F, Napolitano A, Fogliano V, Leonard C, et al. Whole-grain wheat breakfast cereal has a prebiotic effect on the human gut microbiota: a double-blind, placebo-controlled, crossover study. Br J Nutr. (2008) 99:110–20. doi: 10.1017/S0007114507793923
169. Carvalho-Wells AL, Helmolz K, Nodet C, Molzer C, Leonard C, McKevith B, et al. Determination of the in vivo prebiotic potential of a maize-based whole grain breakfast cereal: a human feeding study. Br J Nutr. (2010) 104:1353–6. doi: 10.1017/S0007114510002084
170. Sanz Y. Microbiome and gluten. Ann Nutr Metab (2015) 67(Suppl. 2):28–41. doi: 10.1159/000440991
171. Huda-Faujan N, Abdulamir AS, Fatimah AB, Anas OM, Shuhaimi M, Yazid AM, et al. The impact of the level of the intestinal short chain Fatty acids in inflammatory bowel disease patients versus healthy subjects. Open Biochem J. (2010) 4:53–8. doi: 10.2174/1874091X01004010053
172. Nicolucci AC, Hume MP, Martinez I, Mayengbam S, Walter J, Reimer RA. Prebiotics reduce body fat and alter intestinal microbiota in children who are overweight or with obesity. Gastroenterology (2017) 153:711–22. doi: 10.1053/j.gastro.2017.05.055
173. Ho J, Reimer RA, Doulla M, Huang C. Effect of prebiotic intake on gut microbiota, intestinal permeability and glycemic control in children with type 1 diabetes: study protocol for a randomized controlled trial. Trials 17 (2016):347. doi: 10.1186/s13063-016-1486-y
174. Krokowicz L, Kaczmarek BF, Krokowicz P, Stojcev Z, Mackiewicz J, Walkowiak J, et al. Sodium butyrate and short chain fatty acids in prevention of travellers' diarrhoea: a randomized prospective study. Travel Med Infect Dis. (2014) 12:183–8. doi: 10.1016/j.tmaid.2013.08.008
175. West NP, Pyne DB, Cripps AW, Christophersen CT, Conlon MA, Fricker PA. Gut Balance, a synbiotic supplement, increases fecal Lactobacillus paracasei but has little effect on immunity in healthy physically active individuals. Gut microbes (2012) 3:221–7. doi: 10.4161/gmic.19579
176. Lindsay JO, Whelan K, Stagg AJ, Gobin P, Al-Hassi HO, Rayment N, et al. Clinical, microbiological, and immunological effects of fructo-oligosaccharide in patients with Crohn's disease. Gut (2006) 55:348–55. doi: 10.1136/gut.2005.074971
177. Vulevic J, Juric A, Walton GE, Claus SP, Tzortzis G, Toward RE, et al. Influence of galacto-oligosaccharide mixture (B-GOS) on gut microbiota, immune parameters and metabonomics in elderly persons. Br J Nutr. (2015) 114:586–95. doi: 10.1017/S0007114515001889
178. Benjamin JL, Hedin CR, Koutsoumpas A, Ng SC, McCarthy NE, Hart AL, et al. Randomised, double-blind, placebo-controlled trial of fructo-oligosaccharides in active Crohn's disease. Gut (2011) 60:923–9. doi: 10.1136/gut.2010.232025
179. Wittebole X, De Roock S, Opal SM. A historical overview of bacteriophage therapy as an alternative to antibiotics for the treatment of bacterial pathogens. Virulence (2014) 5:226–35. doi: 10.4161/viru.25991
180. Criscuolo E, Spadini S, Lamanna J, Ferro M, Burioni R. Bacteriophages and their immunological applications against infectious threats. J Immunol Res. (2017) 2017:3780697. doi: 10.1155/2017/3780697
181. Breitbart M, Rohwer F. Here a virus, there a virus, everywhere the same virus? Trends Microbiol. (2005) 13:278–84. doi: 10.1016/j.tim.2005.04.003
182. Carding SR, Davis N, Hoyles L. Review article: the human intestinal virome in health and disease. Aliment Pharmacol Ther. (2017) 46:800–15. doi: 10.1111/apt.14280
183. Kim MS, Park EJ, Roh SW, Bae JW. Diversity and abundance of single-stranded DNA viruses in human feces. Appl Environ Microbiol. (2011) 77:8062–70. doi: 10.1128/AEM.06331-11
184. Manrique P, Bolduc B, Walk ST, van der Oost J, de Vos WM, Young MJ. Healthy human gut phageome. Proc Natl Acad Sci USA. (2016) 113:10400–5. doi: 10.1073/pnas.1601060113
185. Duerkop BA, Hooper LV. Resident viruses and their interactions with the immune system. Nat Immunol. (2013) 14:654–9. doi: 10.1038/ni.2614
186. Breitbart M, Hewson I, Felts B, Mahaffy JM, Nulton J, Salamon P, et al. Metagenomic analyses of an uncultured viral community from human feces. J Bacteriol. (2003) 185:6220–3. doi: 10.1128/JB.185.20.6220-6223.2003
187. Duranti S, Lugli GA, Mancabelli L, Armanini F, Turroni F, James K, et al. Maternal inheritance of bifidobacterial communities and bifidophages in infants through vertical transmission. Microbiome (2017) 5:66. doi: 10.1186/s40168-017-0282-6
188. Chhibber S, Bansal S, Kaur S. Disrupting the mixed-species biofilm of Klebsiella pneumoniae B5055 and Pseudomonas aeruginosa PAO using bacteriophages alone or in combination with xylitol. Microbiology (2015) 161:1369–77. doi: 10.1099/mic.0.000104
189. Gorski A, Miedzybrodzki R, Borysowski J, Dabrowska K, Wierzbicki P, Ohams M, et al. Phage as a modulator of immune responses: practical implications for phage therapy. Adv Virus Res. (2012) 83:41–71. doi: 10.1016/B978-0-12-394438-2.00002-5
190. Barr JJ, Auro R, Furlan M, Whiteson KL, Erb ML, Pogliano J, et al. Bacteriophage adhering to mucus provide a non-host-derived immunity. Proc Natl Acad Sci USA. (2013) 110:10771–6. doi: 10.1073/pnas.1305923110
191. Barr JJ, Youle M, Rohwer F. Innate and acquired bacteriophage-mediated immunity. Bacteriophage (2013) 3:e25857. doi: 10.4161/bact.25857
192. Clark JR, March JB. Bacteriophages and biotechnology: vaccines, gene therapy and antibacterials. Trends Biotechnol. (2006) 24:212–8. doi: 10.1016/j.tibtech.2006.03.003
193. Kurzepa A, Dabrowska K, Skaradzinski G, Gorski A. Bacteriophage interactions with phagocytes and their potential significance in experimental therapy. Clin Exp Med. (2009) 9:93–100. doi: 10.1007/s10238-008-0027-8
194. Molenaar TJ, Michon I, de Haas SA, van Berkel TJ, Kuiper J, Biessen EA. Uptake and processing of modified bacteriophage M13 in mice: implications for phage display. Virology (2002) 293:182–91. doi: 10.1006/viro.2001.1254
195. Hill C, Mills S, Ross RP. Phages & antibiotic resistance: are the most abundant entities on earth ready for a comeback? Future Microbiol. (2018) 13:711–26. doi: 10.2217/fmb-2017-0261
196. Norman JM, Handley SA, Baldridge MT, Droit L, Liu CY, Keller BC, et al. Disease-specific alterations in the enteric virome in inflammatory bowel disease. Cell (2015) 160:447–60. doi: 10.1016/j.cell.2015.01.002
197. Lucas Lopez R, Grande Burgos MJ, Galvez A, Perez Pulido R. The human gastrointestinal tract and oral microbiota in inflammatory bowel disease: a state of the science review. APMIS (2017) 125:3–10. doi: 10.1111/apm.12609
198. Lebeer S, Vanderleyden J, De Keersmaecker SC. Host interactions of probiotic bacterial surface molecules: comparison with commensals and pathogens. Nat Rev Microbiol. (2010) 8:171–84. doi: 10.1038/nrmicro2297
199. Konieczna P, Akdis CA, Quigley EM, Shanahan F, O'Mahony L. Portrait of an immunoregulatory Bifidobacterium. Gut Microb. (2012) 3:261–6. doi: 10.4161/gmic.20358
200. Laino J, Villena J, Kanmani P, Kitazawa H. Immunoregulatory effects triggered by lactic acid bacteria exopolysaccharides: new insights into molecular interactions with host cells. Microorganisms (2016) 4:E27. doi: 10.3390/microorganisms403002
201. Dwivedi M, Kumar P, Laddha NC, Kemp EH. Induction of regulatory T cells: a role for probiotics and prebiotics to suppress autoimmunity. Autoimmun Rev. (2016) 15:379–92. doi: 10.1016/j.autrev.2016.01.002
Keywords: immunological tolerance, probiotics, prebiotics, Lactobacilli, Bifidobacteria, inflammation, bacteriophages
Citation: Vitetta L, Vitetta G and Hall S (2018) Immunological Tolerance and Function: Associations Between Intestinal Bacteria, Probiotics, Prebiotics, and Phages. Front. Immunol. 9:2240. doi: 10.3389/fimmu.2018.02240
Received: 01 June 2018; Accepted: 10 September 2018;
Published: 09 October 2018.
Edited by:
Javier Ochoa-Reparaz, Eastern Washington University, United StatesReviewed by:
Claudio Nicoletti, Università degli Studi di Firenze, ItalyPaolo Riccio, University of Basilicata, Italy
Copyright © 2018 Vitetta, Vitetta and Hall. This is an open-access article distributed under the terms of the Creative Commons Attribution License (CC BY). The use, distribution or reproduction in other forums is permitted, provided the original author(s) and the copyright owner(s) are credited and that the original publication in this journal is cited, in accordance with accepted academic practice. No use, distribution or reproduction is permitted which does not comply with these terms.
*Correspondence: Luis Vitetta, luis.vitetta@sydney.edu.au; luis_vitetta@medlab.co