- 1Department of Radiation Sciences, University of Umeå, Umeå, Sweden
- 2Department of Clinical Microbiology & Laboratory of Molecular Infection Medicine Sweden, Umeå University, Umeå, Sweden
Neutrophils are crucial for the human innate immunity and constitute the majority of leukocytes in circulation. Thus, blood neutrophil counts serve as a measure for the immune system's functionality. Hematological patients often have low neutrophil counts due to disease or chemotherapy. To increase neutrophil counts and thereby preventing infections in high-risk patients, recombinant G-CSF is widely used as adjunct therapy to stimulate the maturation of neutrophils. In addition, G-CSF is utilized to recruit stem cells (SCs) into the peripheral blood of SC donors. Still, the actual functionality of neutrophils resulting from G-CSF treatment remains insufficiently understood. We tested the ex vivo functionality of neutrophils isolated from blood of G-CSF-treated healthy SC donors. We quantified chemotaxis, oxidative burst, and phagocytosis before and after treatment and detected significantly reduced chemotactic activity upon G-CSF treatment. Similarly, in vitro treatment of previously untreated neutrophils with G-CSF led to reduced chemotactic activity. In addition, we revealed that this effect persists in the allogeneic SC recipients up to 4 weeks after neutrophil engraftment. Our data indicates that neutrophil quantity, as a sole measure of immunocompetence in high-risk patients should be considered cautiously as neutrophil functionality might be affected by the primary treatment.
Introduction
Neutrophils are the most common leukocytes in blood and constitute 50–70% of the white blood cells corresponding to an absolute neutrophil count of 1.8-7.7 × 109/l blood (1). As part of the first line of defense they immediately migrate to the site of an infection following gradients of chemoattractants released by tissue-dwelling, pathogen sensing cells (2). Like macrophages, neutrophils are professional phagocytes. However, unlike macrophages, they are loaded with preformed granules containing antimicrobial effector molecules (3). This allows neutrophils to kill invading microbes very efficiently. During phagocytosis, neutrophils engulf microbes into the phagosome that subsequently fuses with granules to form an antimicrobial environment that toxifies microorganisms by reactive oxygen species (ROS), antimicrobial peptides and proteins (4, 5). To eradicate microbes in the extracellular milieu neutrophils either degranulate antimicrobial effectors into the extracellular milieu (6) or deliver their effectors embedded into a web-like structure, known as neutrophil extracellular traps (2, 7). Clinically relevant neutropenia and severe neutropenia are defined by neutrophil counts below 1.5–2 × 109/l or 0.5 × 109/l, respectively (1, 8). Neutropenia occurs transiently or chronically in a variety of diseases and disorders and renders individuals more susceptible to infections (1, 9–13). A major group at risk for neutropenia are patients treated for hematological malignancies: Chemo- and radiation therapy often affect hematopoiesis, thereby reducing neutrophil production (8). Furthermore, immunosuppressive drugs such as azathioprine or mycophenolate can depress neutrophil counts (14, 15). Accordingly, persistent neutropenia is a known risk factor for invasive bacterial and fungal infections after receiving a hematopoietic stem cell (HSC) transplant (16, 17). Today, the granulocyte colony-stimulating factor (G-CSF) is widely used as a prophylactic treatment to counteract neutropenia and to prevent infections, for instance, in patients undergoing systemic chemotherapy (18, 19). However, studies on lymphoma patients revealed that G-CSF given as primary prophylaxis could not increase overall survival or reduce infection-related mortality (18). Another study on patients undergoing HSC transplantation while receiving G-CSF treatment showed a slightly decreased infection rate but no difference in treatment-related mortality (20). HSCs are transplanted either by autologous or allogeneic routes into patients suffering from different malignant and non-malignant hematopoetic disorders, for instance, acute myeloid leukemia, myeloma, or thalassemia major (8). To increase the number of pluripotent cells in peripheral blood, the donors receive G-CSF prior to the process of harvesting HSCs from peripheral blood (21). Concomitantly, the numbers of circulating neutrophils increase. Naturally, the cytokine G-CSF is a major factor controlling the development and function of neutrophils (22, 23). G-CSF regulates proliferation, maturation, survival, and functional activation of these cells (24). During the course of an infection, serum levels of G-CSF increase which in turn enhances proliferation of granulocytic precursor cells and an increased level of circulating neutrophils (23). In our study we aimed to analyze the quality of neutrophils resulting from G-CSF-treatment. We investigated key neutrophil functions in cells isolated from blood of healthy individuals recruited as HSC donors before and after G-CSF treatment as well as from allogeneic HSC recipients at different time points after receiving the HSC graft from G-CSF-treated donors. While oxidative burst and phagocytosis were unaffected in all these samples, chemotaxis was significantly reduced upon treatment with G-CSF. Strikingly, impaired chemotaxis was detectable in neutrophils from HSC recipients up to 4 weeks post engraftment.
Materials and Methods
Ethical Statement
Ethical approval (register number 09-210M, project number CFU-12/09) for the study was obtained from the Regional Ethical Review Board, Umeå, Sweden. All parts of the study were performed according to the Declaration of Helsinki. The patients were included after informed written consent.
Subjects and Treatment Time Line
Blood samples were collected between 2010 and 2017 at Umeå University Hospital, Sweden. The first cohort consisted of healthy individuals selected by Hematology Unit clinicians to donate for bone marrow transplantation (Table 1). For approval, SC donors underwent general health examination. The first blood sample from donors of this study was collected shortly after initial health check and before receiving the first dose of G-CSF (Figure S1A). Before donating HSCs, donors received 10 μg G-CSF/kg/day (“Nivestim™”, filgrastim, Pfizer) over 4 days to be able to donate HSCs by a continuous flow apheresis procedure. The second donor blood sample was collected on the day of HSC harvest, thus at day 5 after 4 days of G-CSF treatment, immediately before the apheresis procedure started (Figure S1A).
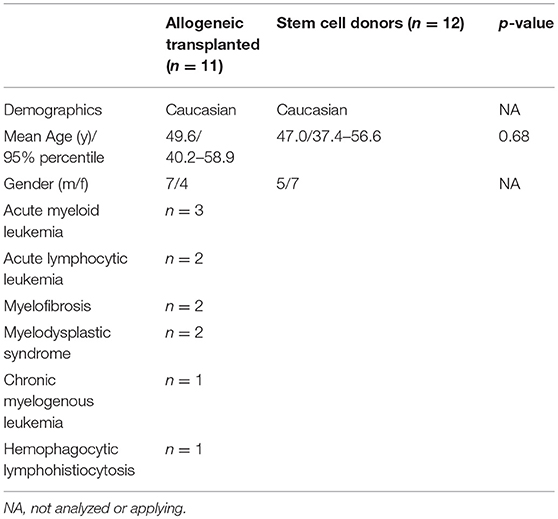
Table 1. Age and gender distribution of the cohorts: HSC donors and allogeneic transplant recipients.
The second cohort consisted of patients undergoing allogeneic HSC transplantation (Table 1). Blood samples were taken from the patients 2 weeks after successful neutrophil engraftment which was defined by absolute neutrophil count of >1.0 × 109/l (Figure S1B). Depending on the individual patient, one additional sample was taken either 4 or 8 weeks post engraftment (Figure S1B). When comparing the two groups (HSC donors vs allogeneic transplant recipients) there was no statistical significant difference between the groups according to age (mean 47.0 y vs. 49.6 y, respectively) (Table 1). To control for maturity of circulating neutrophils, blood of 4 additional HSC donors (not included before in table 1) was collected prior and post G-CSF treatment and neutrophils were isolated using the same isolation procedure. In addition, we used blood from healthy volunteers not involved in any clinical treatment for in vitro cell stimulation analyses.
Neutrophil Isolation and Maturity Assessment
Peripheral venous blood was collected in EDTA-containing tubes and neutrophils were isolated using a Histopaque-1119 separation followed by a Percoll gradient, as previously described (25, 26). Viable cells were counted in a Neubauer chamber using trypan blue staining and subsequently diluted according to assay protocols. As demonstrated earlier, this procedure leads to a neutrophils purity of > 93% (27). To assess neutrophil maturity, full blood as well as isolated neutrophil fractions were analyzed for their exact cell composition. We prepared blood smears on glass slides as well as smears from neutrophil isolations, which were resuspended in plasma from the same sample. These smears were fixed and Giemsa stained. Trained personnel used these smears to perform a comprehensive blood differentiation analysis at the Unit of Laboratory Medicine at the Norrland University Hospital in Umeå (project number FFLA01). All samples were analyzed in a blinded fashion. Band cells were considered immature, cells containing a segmented nucleus were considered mature (28, 29).
Media and Incubation
If not stated otherwise, neutrophil functionality assays were performed in RPMI medium without phenol-red (Lonza) and incubation occurred under standard cell culture conditions: 37°C, 5% CO2, humid atmosphere.
Chemotaxis
Neutrophils chemotaxis was quantified using a transwell setup similar to previous description (30, 31). Briefly, 5 × 106 neutrophils/ml were stained with 3.3 μM bis-2-carboxyethyl-5-[and-6]-carboxyfluorescein-AM (Sigma-Aldrich) for 20 min at room temperature, washed, and resuspended in medium with 0.05% human serum albumin. Fluorescence-impermeable transwell inserts (BD Falcon, HTS FluoroBlok, 3 μm pore size, PET membrane) were loaded with 5 × 105 cells each and placed into wells of 24-well plates containing 600 μl RPMI, with or without 10 nM formyl-methionyl-leucyl-phenylalanine (fMLF). Fluorescence intensity (FI) with excitation 485 nm and emission 520 nm was detected in a plate reader (FLUOstar OMEGA; BMG Labtech) with 5% CO2 and 37°C over the course of 30 min. Medium only served as blank and 5 × 105 stained cells seeded directly into the lower compartment served as 100% FI reference. If indicated, we added 10 ng/ml or 50 ng/ml G-CSF (human recombinant G-CSF, Sigma-Aldrich) to the respective neutrophil sample. Subsequently, cells were washed in medium and used as described. Thus, if not stated otherwise, neutrophils are unstimulated prior to the analysis. Migration was calculated as percent of 100% reference Migration of unstimulated cells was subtracted from the fMLF-induced migration. Half migration time was determined as time required for 50% of maximum migration. Velocity at half migration time was calculated as Δ%/min (see Figure S2).
Reactive Oxygen Species Production
Oxidative burst was quantified by luminol-based chemiluminescence (26). Briefly, 5 × 104 neutrophils per well were seeded in white 96-well plates with 50 μM luminol and 1.2 U/ml horseradish peroxidase (both Sigma-Aldrich). Neutrophils were stimulated with 100 nM phorbol 12-myristate 13-acetate (PMA) or left untreated. Chemiluminescence as a measure of hydrogen peroxide production was detected every 2 min for 3 h in an Infinite 200 luminometer (Tecan). ROS were quantified by the area under the curve (AUC) calculated for the time given (3 h) and reduced by background of unstimulated cells.
Phagocytosis
Phagocytosis was assessed using pH rodo beads (pHrodoTM Red S. aureus bioparticles conjugated for phagocytosis, Lifetechnologies) that change their FI pH-dependently. Briefly, 5 × 104 neutrophils per well were added into a black 96-well plate with 100 μg beads/well. Beads put directly into a buffer with pH 4 served as 100% FI control. Beads in medium with pH 7.4 served as blank. Neutrophils pre-treated with 10 μM cytochalasin D for 15 min to block phagocytosis served as negative control. FI (540/580 nm) was measured every 60 min for 3 h (FLUOstar Omega plate reader, BMG Labtech).
Plasma Cytokine Quantification
Along with neutrophil isolation from HSC donors, 1.5 ml of plasma were collected and immediately stored at−80°C. These samples were used to analyze levels of human G-CSF and interleukin 8 (IL-8) using Luminex ELISA assays (Bio-Rad, Bio-Tech, respectively). Assays were performed according to manufacturer's protocol using a magnetic beads washer. For G-CSF quantification, the samples (Luminex, Bio-Rad) were diluted 1:4 in sample diluent. For IL-8 quantification in plasma from HSC donors, a high-sensitive Luminex assay (BioTech) was used with standard range 0.78–3200 pg/ml. Samples were diluted 1:2 in calibrator diluent RD6-40 and added to plates for subsequent analysis with a Bio-Plex reader (Bio-Plex 200 system, Bio-Rad). Plasma samples were used for the analysis of C-reactive protein (CRP) which was quantified using the CRPL3 cobas® assay (Roche Diagnostics Scandinavia) performed by the Clinical Chemistry Laboratory at Umeå University Hospital according to the manufacturer's recommendations and the standard ISO/IEC 17025:2005 with continuous evaluations by the Swedish Board for Accreditation and Conformity Assessment.
In vitro Cytokine Release
To analyze IL-8 release upon G-CSF stimulation, neutrophils were isolated from healthy volunteers, and 5 × 105 neutrophils were treated with 50 ng/ml G-CSF. After 30 min, 1 μg/ml LPS was added as a co-stimulant for 6 h. As control, cells were treated with medium only. After 6 h, supernatants were collected and directly frozen at −80°C. The IL-8 concentration was quantified according to manufacturer's protocol (BioLegend Human IL-8 ELISA Max standard set).
Quantification of Cell Surface fMLP Receptor
G-CSF treated as well as untreated neutrophils were stained with labeled monoclonal anti-fMLP receptor antibody coupled to PE (PE, MACS, Miltenyi Biotec) in PBS supplemented with 3% FCS in a final dilution of 1:10 and incubated for 30 min at 8°C. Cells were washed 3 times in PBS with 3% FCS followed by flow cytometry analysis (BD Biosciences LSR II). The population of neutrophils were identified using FSC-A and SSC-A. At least 104 cells were analyzed per sample and all samples were run in duplicates.
Statistics
We used Graph-Pad Prism V 5.04 for statistical analysis. Data was analyzed using Students t-test, and one-way ANOVA with Tukey's multiple comparison tests. Mann-Whitney U-, Wilcoxon signed-rank and Kruskal-Wallis tests were used when data was not normally distributed. A p-value below 0.05 was considered significant.
Results
G-CSF Treatment in Healthy HSC Donors Reduces Neutrophil ex vivo Chemotaxis
We determined chemotaxis of neutrophils isolated from peripheral blood of G-CSF-treated HSC donors (Figure S1). As expected, the total neutrophil count increased in the blood of these individuals ~ 9-fold upon treatment (Figure 1A). When analyzing the neutrophils ex vivo, we detected that neutrophils isolated from HSC donors after G-CSF-treatment displayed significantly increased half migration time and a reduced velocity at half migration time compared to neutrophils before treatment (Figures 1B,C). In contrast, spontaneous, unstimulated migration of neutrophils was similar using neutrophils from donors before and after G-CSF treatment (Figure S2) as determined by half migration time and velocity at half migration time (Figure S3). In summary, chemotaxis of neutrophils from HSC donors after G-CSF-treatment was reduced compared to neutrophils from the same individuals before treatment. This suggests that G-CSF administration in healthy HSC donors could reduce the ability of circulating neutrophils to migrate upon chemotactic stimulation. Of note, the number of immature neutrophils, so-called band cells, was negligibly small below 2% before and after G-CSF treatment in both, full blood and neutrophil isolation fractions (Figures 1D,E) as determined by a complete blood differentiation analysis. Further, the neutrophil isolation strategy chosen for this study proved to be efficient in enriching the neutrophil content to > 95%.
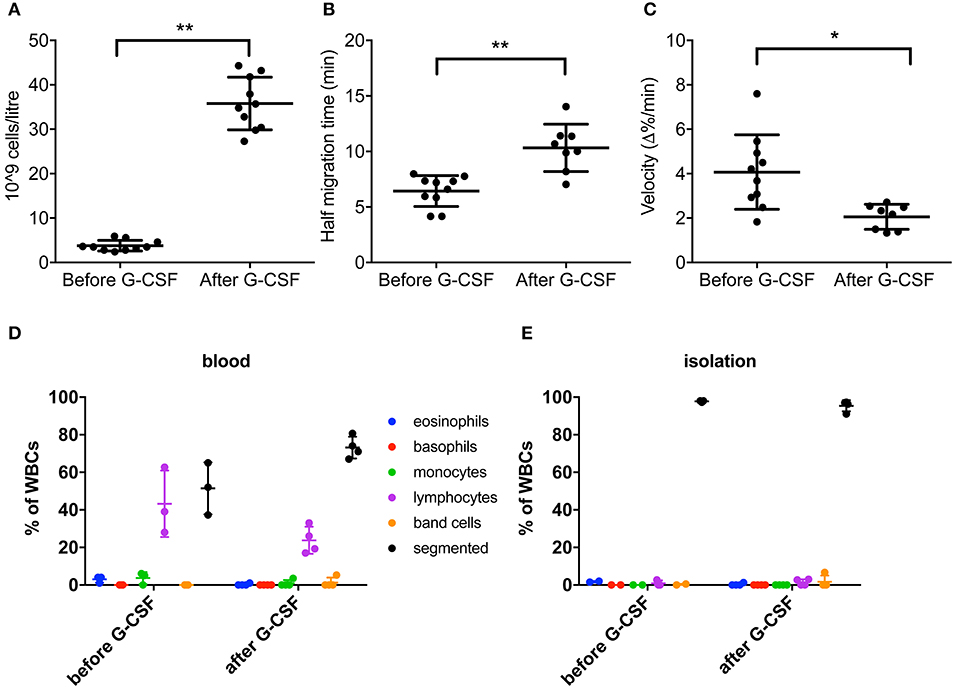
Figure 1. G-CSF treatment affects neutrophil migration. Stem cell donors before and after treatment were analyzed for neutrophil function. (A) Neutrophil count measured before and after G-CSF-treatment in the stem cell donor group (n = 10). Revealed a significant increase in the number of circulating neutrophils after G-CSF treatment. (B) Distribution of half migration time during chemotaxis within the stem cell donor group before and after G-CSF-treatment. Half migration time was defined as the time required for half-maximal fluorescence signal indicative for migration toward 10 nM fMLP. (C) The distribution of velocity during chemotaxis before and after G-CSF-treatment is indicated. Velocity was measured as Δ %/min at half migration time indicating the speed of the neutrophils when they have reached half maximal fluorescence in the bottom well. Blood cell differentiation analysis (D) before and (E) after purification of neutrophils from stem cell donors before (n = 3) and after (n = 4) GCSF treatment confirmed that the purification enriched mature neutrophils >95% independent of previous G-CSF treatment. Statistical analysis was performed using a Wilcoxon signed-rank test (A–C). *p < 0.05, **p < 0.01.
We next investigated whether G-CSF had a similar effect on neutrophil chemotaxis if directly applied to neutrophils in vitro. For this purpose, isolated neutrophils from peripheral blood of eight healthy volunteers were pre-incubated with 10 and 50 ng/ml G-CSF prior to the migration assay. Indeed, we observed that high concentrations of G-CSF decreased the chemotactic ability of isolated neutrophils (Figures 2A,B). With 50 ng/ml G-CSF, chemotaxis was significantly impaired as determined by half migration time and velocity at half migration time. This demonstrates that G-CSF can directly decrease migration activity of isolated neutrophils ex vivo although to a smaller extent. To test whether this effect could result from a removal of the fMLF-specific G-protein coupled receptor, we quantified its surface expression by flow cytometry. We incubated purified neutrophils with 10 and 50 ng/ml G-CSF and quantified surface exposure of the receptor by staining with antibodies directed against fMLF receptor (Figure 2C). We could not observe any differences between treated and untreated neutrophils suggesting that surface exposure of the fMLF receptor on neutrophils was not affected by G-CSF treatment.
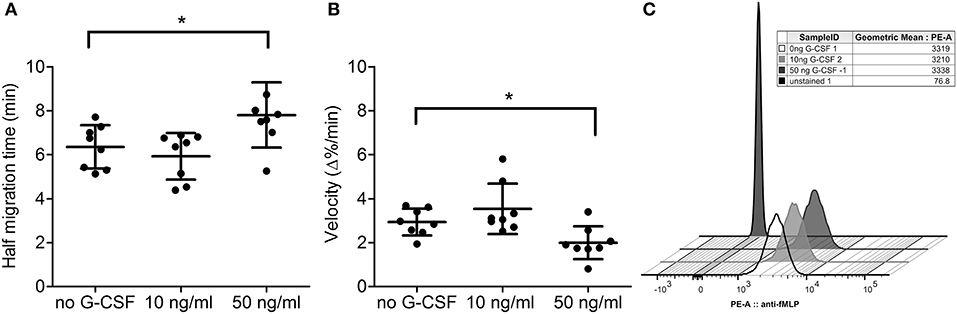
Figure 2. Systemic G-CSF treatment impairs chemotaxis in neutrophils from healthy donors. Purified neutrophils from peripheral blood of healthy, untreated donors (n = 8) were incubated with 0, 10, or 50 ng/ml human recombinant G-CSF. Thereafter, a chemotaxis assay was performed using fMLP as chemoattractant and half migration time (A) as well as velocity at half migration time (B) was determined. Neutrophils pre-treated with 0, 10, and 50 ng/ml of G-CSF respectively were stained with a PE-labeled anti-fMLP receptor antibody. The histogram is showing a representative experiment of three similar replications (C). Statistical analysis was performed with a Kruskal-Wallis test for (A) (p = 0.0098) and (B) (p = 0.0093), complemented with Mann-Whitney U-tests showing that samples 0 and 50 ng/ml are significantly different for (A,B) with *p < 0.05.
G-CSF Administration to Healthy HSC Donors Alters Levels of IL-8 and CRP in Plasma
Next, we investigated potential mechanisms for the reduced chemotaxis of G-CSF-treated neutrophils. Interleukin 8 (IL-8) is a central chemokine for neutrophil recruitment contributing to the extravasation of neutrophils to the site of infection (32). C-reactive protein (CRP), an acute phase serum protein of hepatic origin, has been previously implicated to suppress neutrophil chemotaxis in vitro (33). We therefore, analyzed plasma samples from HSC donors, taken before and after G-CSF treatment, for IL-8 and CRP levels. To reveal possible correlations, we also quantified plasma levels of G-CSF. After treatment, G-CSF concentrations were significantly increased, 1042 pg/ml compared to 8 pg/ml before treatment (Figure 3A). Notably, we observed a significant decrease of IL-8 in plasma of G-CSF-treated individuals as compared to the samples taken before treatment (Figure 3B). After treatment, IL-8 levels were at detection limit (0.72 pg/ml), whereas before treatment all obtained values for IL-8 were within detection range. Despite this observation in plasma, in vitro priming of neutrophils from healthy volunteers with 50 μg/ml G-CSF did not affect the release of IL-8 upon stimulation with endotoxin lipopolysaccharide from Escherichia coli (Figure 3C). In contrast to IL-8, acute phase protein CRP levels in plasma significantly increased upon G-CSF treatment (Figure 3D).
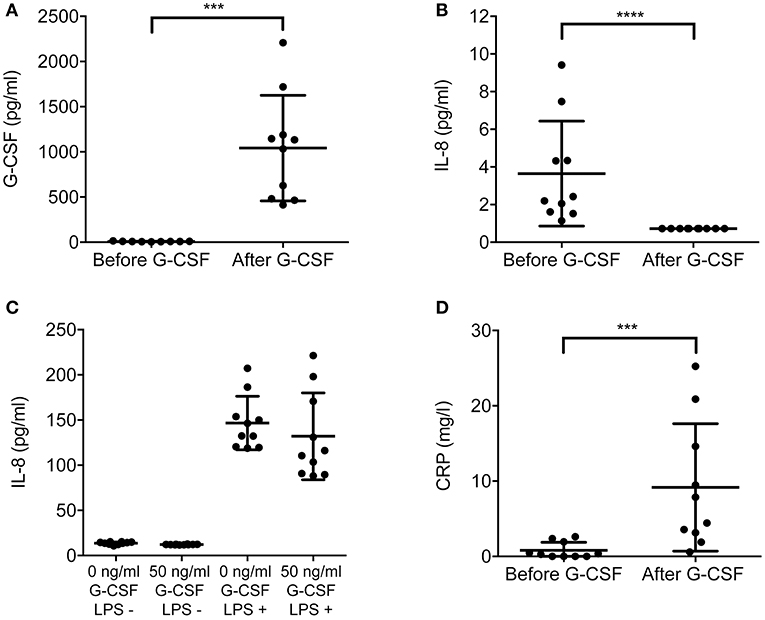
Figure 3. Plasma from G-CSF-treated individuals contains lower amount of IL-8 than untreated controls. G-CSF (A), IL-8 (B), and CRP (D) concentrations in plasma obtained from stem cell donors (n = 10) before and after G-CSF-treatment. Each value was determined from a mean of four technical replicates for G-CSF and IL-8. For IL-8 (B) all the samples after G-CSF treatment were below the range of the assay (0.72 pg/ml). To be able to perform statistical analysis the values were extrapolated to 0.72 pg/ml. (C) Levels of IL-8 secreted by neutrophils in vitro after G-CSF (50 ng/ml) or mock treatment for 30 min and subsequently either stimulated with LPS (1 μg/ml, labeled as LPS +) or left untreated (labeled as LPS -). The levels of IL-8 were measured by ELISA after 6 h stimulation. The CRP values (D) were measured as single measurements for each donor before and after G-CSF treatment. Statistical analysis was performed using a paired students t-test (C) and Mann-Whitney U-test (A,B,D) when data was not normally distributed. ***p < 0.001 and ****p < 0.0001.
Neutrophil Chemotaxis Remains Reduced in Allogeneic Transplant Recipients
We compared neutrophil chemotaxis from controls and allogeneic transplant recipients 2 weeks after neutrophil engraftment (Figure S1) and observed significant differences. Half migration time is increased (Figure 4A) and velocity at half migration time is decreased for the group of allogeneic transplant recipients (Figure 4B) similarly to the G-CSF-treated healthy HSC donors (Figures 1B,C). This indicates that the G-CSF-mediated effects on neutrophil chemotaxis remained in the recipient 2 weeks after neutrophil engraftment. Healthy HSC donors prior to G-CSF treatment serving as controls were homogenous for age, but not for gender when compared to the transplant recipients. However, evaluating our data in a gender-separated fashion we do not find differences between the sexes (Figure S4). This indicates that our findings do not correlate with differences in gender or age between the groups.
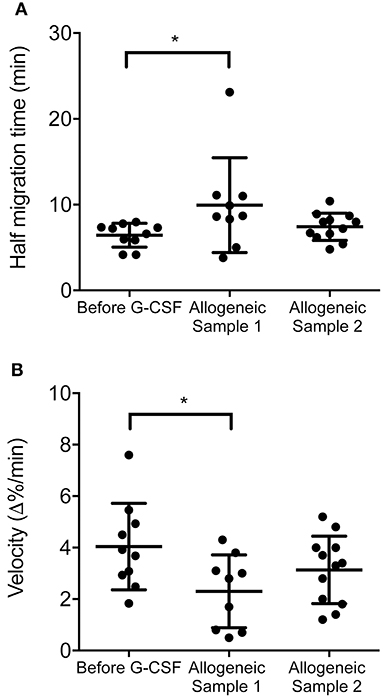
Figure 4. The chemotactic activity remains impaired in allogeneic transplant recipients. (A) Distribution of half migration time in the stem cell donors before G-CSF treatment (n = 10), serving as controls, and samples from patients undergoing allogeneic stem cell transplantation at a medium of 48.9 days (n = 9), indicated as sample 1, and 80.2 days (n = 12), indicated as sample 2, after transplant, respectively. The half migration time is measured as the time it takes to reach half of max fluorescent and is a measure of neutrophil speed in the process of chemotaxis. (B) The distribution of velocity during chemotaxis comparing stem cell donors before G-CSF with samples from patients undergoing allogeneic stem cell transplantation as described above. The evaluation shows that the velocity at half max migration goes down early after allogeneic stem cell transplantation which confirms that the chemotaxis in neutrophils is slower early after stem cell transplantation. Statistical analysis was performed with a Kruskal–Wallis test for (A) (p = 0.0466) and (B) (p = complemented with Mann–Whitney U-tests showing that samples “before G-CSF treatment” and “allogeneic sample 1” are significantly different for (A,B) with *p < 0.05.
Analysis of neutrophils sampled 4 weeks after neutrophil engraftment and beyond (Figure S1) showed that this effect was transient. Neutrophil chemotaxis was not significantly different from those of the untreated controls (Figures 4A,B). Thus, our data implies that there might be a window of increased risk of infection for the transplant recipients after engraftment (30 days in average) in which neutrophil numbers are high, but they might still have functional limitations (Table 1).
ROS Production and Phagocytosis Unaffected by G-CSF Treatment
ROS, produced by the NADPH oxidase complex in phagocytes, are important mediators of cell signaling and very powerful effectors of antimicrobial killing. Thus, we investigated whether G-CSF treatment alters total ROS production in neutrophils stimulated with PMA for 3 h. We could not detect a difference in ROS production by neutrophils from HSC donors before and after G-CSF-treatment (Figure 5A). Similarly, neutrophils from allogeneic transplant recipients did not display a significantly altered ROS production when comparing sample 1 and sample 2 (Figure 5B). This implies that the neutrophil's potential to produce ROS was unaffected by G-CSF treatment.
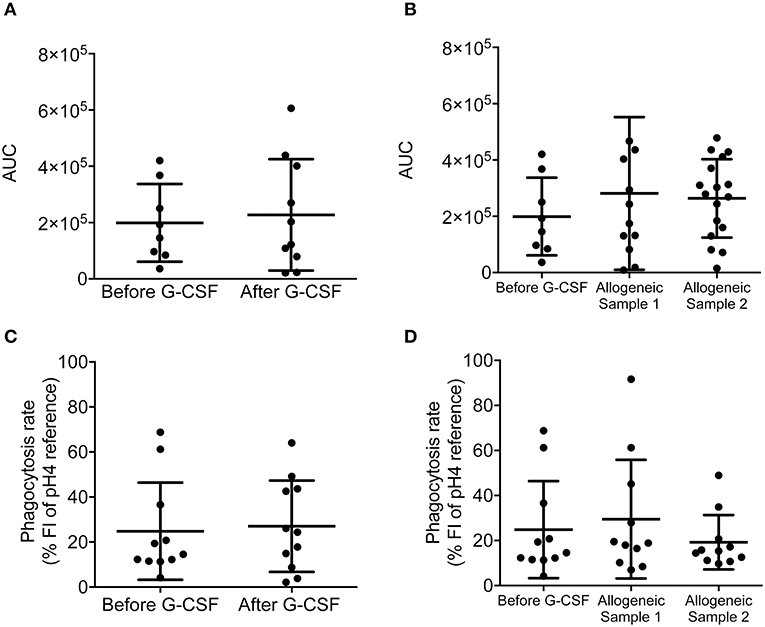
Figure 5. ROS production and phagocytosis are not affected by G-CSF in stem cell donors and neither in allogeneic transplant recipients. (A) Production of ROS by neutrophils was measured as area under the curve (AUC) in the stem cell donor group before and after G-CSF-treatment (n = 10). Statistical analysis was performed using an unpaired students t-test. (B) Production of ROS measured as AUC in the samples from patients undergoing allogeneic stem cell transplantation using the samples from the stem cell donors before G-CSF-treatment as a control. Statistical analysis was performed using a Kruskal-Wallis test due to skewness of data. (C,D) Phagocytosis measured as the uptake of pH-sensitive pHrodo beads that triggered phagocytosis by neutrophil isolated from (C) stem cell donor group before and after G-CSF-treatment (n = 10) and from (D) patients undergoing allogeneic stem cell transplantation using the samples from the stem cell donors before G-CSF-treatment as a control. Phagocytosis rate was calculated as percent out of 100 percent control. Statistical analysis was performed using a One-way ANOVA with Tukey's multiple comparison tests. Statistical analyses in (A-D) did not reveal significance p < 0.05.
As professional phagocytes, engulfment of microbes is a key mode of action of neutrophils. We therefore quantified phagocytic uptake using fluorescently-labeled beads tagged with surface molecules from Staphylococcus aureus. Upon engulfment, these beads increase fluorescence intensity in the acidic milieu of the mature phagosome. To confirm actual phagocytosis, we included neutrophils treated with cytochalasin D as a negative control. Cytochalasin D inhibits cytoskeleton rearrangements and thus allows discrimination between adherence and phagocytosis. When comparing the phagocytic uptake by neutrophils from HSC donors before and after G-CSF treatment as well as the neutrophils from the allogeneic transplant recipients at different time points of harvest we could not observe any significant difference (Figures 5C,D). Of note, we observed that neutrophils from G-CSF-treated individuals could engulf beads even in the presence of the cytoskeleton inhibitor cytochalasin D, while phagocytic uptake of neutrophils from HSC donors prior to G-CSF treatment was blocked as expected (Figure S5). This may indicate that G-CSF renders neutrophils less susceptible against cytochalasin D. Notably, pH of surrounding medium was regularly controlled and did not change during the course of the experiments. In conclusion, ROS production and phagocytosis were not affected by G-CSF treatment.
Discussion
Using G-CSF to mobilize HSCs has revolutionized the treatment of blood malignancies as it often replaces the classical bone marrow transplant (34). Instead of harvesting bone marrow surgically from the pelvis bone, which requires, e.g., general anesthesia, the cells of interest are collected from the peripheral blood. Yet again, there is growing evidence that G-CSF treatment alters more physiological functions than the blood cell count (22, 35). Our study analyzed key neutrophil functions, i.e., chemotaxis, ROS production, and phagocytosis, in isolated neutrophils from the peripheral blood of HSC donors and allogeneic transplant recipients by using well-established in vitro stimuli and assays. The individuals included in our study received standard dosages of G-CSF (10 μg/kg/d) and the resulting plasma concentrations were in the expected ranges (34, 35). As expected, G-CSF treatment substantially increased the number of neutrophils in the circulation. When analyzing the functionality of these neutrophils, we revealed that if derived from healthy HSC donors treated with G-CSF, the cells showed reduced ex vivo chemotaxis toward fMLF. Other key functions tested, i.e., ROS production and phagocytosis, seemed to remain unaltered. Strikingly, the chemotactic impairment observed in neutrophils from HSC donors persisted in the group of allogeneic transplant recipients. Even after transplantation and novel granulopoiesis within the transplant recipient, newly emerging neutrophils showed the same migration defect as the neutrophils from the HSC donors. Of note, the recipients themselves received no prophylactic G-CSF. According to our data, it took several weeks until normal neutrophil activity was regained. Notably, we observed the chemotactic impairment even though the allogeneic transplant recipient group was heterogeneously composed with diversity regarding the underlying disease. Nevertheless, these patients frequently receive immunosuppressive therapy, such as steroids or calcineurin inhibitors, which in turn were shown to impair neutrophil functions. However, the effects of these type of drugs are usually pleiotropic and do not specifically target neutrophil chemotaxis. For instance, calcineurin inhibitors can impair chemokinesis of neutrophils on vitronectin (36), reduce oxidative burst induced by fMLF (37) and diminish phagocytic activity (38). Further, the content of immature neutrophils was <2% in both, full blood and neutrophil isolation fractions—even after G-CSF treatment.
Our results confirm previous studies describing impaired neutrophil functionality in allogeneic transplant recipients. Sosa et al. reported that neutrophil chemotaxis is impaired up to 4 months after HSC transplantation (39) and Katoh et al. observed a decrease in chemotactic activity for up to 12 months (40). Azzara et al. even pointed toward a reduced motility of neutrophils from chemotherapy patients treated with G-CSF (41). Still, these studies were performed with neutrophils from cancer patients while we also included otherwise healthy HSC donors yet observed similar abnormalities. This implies that G-CSF treatment in general affects neutrophils and is supported by Kerst et al. who described phenotypically and functionally altered neutrophils upon G-CSF treatment in healthy donors (42). Few studies addressed the impact of G-CSF administration on neutrophils in healthy individuals, in our case HSC donors. Dale and coworkers investigated the combined impact of G-CSF and corticosteroids on neutrophil function (43), whereas Höglund and colleagues similar to our study investigated the singular effect of G-CSF (44). The authors observed a reduced migratory activity of neutrophils after several doses of G-CSF and the transient nature of this effect, as it disappeared several weeks after treatment. Our study confirms and extends on these findings, since we directly combine investigations of healthy HSC donors with allogeneic transplant recipients as well as linking our observations to CRP and IL-8 levels and direct in vitro effects as discussed in more detail below. Interestingly, in vitro studies triggering neutrophils directly with G-CSF observed a rapid stimulation of random mobility that declined within about half an hour (45). In our experiments showing reduced mobility due to G-CSF treatment, neutrophils were incubated with G-CSF for that same amount of time and subsequently challenged with fMLF to induce active movement. Taken together, these observations suggest a possible “exhaustion” of chemotactic potential due to random mobility if neutrophils are directly challenged with G-CSF. Notably, in our study, reduced in vitro chemotaxis did unlikely result from decreased surface exposure of the fMLF receptor.
More strikingly, we demonstrate that reduced neutrophil chemotaxis persisted within the allogeneic transplant recipients at 2 weeks post HSC engraftment. The average life span of neutrophils in circulation is relatively short; it is estimated to be not longer than a few days (46–48). So, in these patients, the neutrophils analyzed did not stem from the first round of newly generated cells, but more likely from a following generation of neutrophils. Our findings are in good agreement with a study investigating neutrophil functions in autologous bone marrow transplantation recipients (49). Humphreys et al. report decreased chemotaxis of neutrophils upon G-CSF treatment, which persisted until 2 weeks post engraftment (49). Yet again, the effects observed were detected for neutrophils derived from HSCs from patients with a hematological disorder, and not, like in our case, from healthy HSC donors.
In addition to reduced chemotaxis, we show that IL-8 plasma levels of HSC donors are reduced compared to untreated controls after G-CSF treatment. That decrease is contradictory to a study by Watanabe et al. where the authors reported elevated levels of IL-8 after G-CSF-treatment even though the G-CSF treatment regimen was virtually identical to our donors (50). Moreover, this study showed a correlation between the IL-8 levels and the amount of harvested CD34+ cells that are used as a measure for HSC mobilization. Again, this is in contrast with our findings: We could not detect any correlation between IL-8 levels and amount of harvested CD34+ cells or the neutrophil count in circulation. Since IL-8 is pivotal for neutrophil chemotaxis, we conclude that the significant reduction of IL-8 plasma levels might contribute also to reduced chemotaxis of neutrophils. However, it remains unclear, which cells are responsible for IL-8 production under these conditions.
Neutrophils are crucial for the innate immunity. G-CSF is therefore used in neutropenic patients as a preventive treatment to avoid infections. Still, a study with transplant recipients reported that preventive G-CSF treatment did not alter infection-related mortality in these patients (51). While this supports the relevance of reduced neutrophil functionality, i.e., the ability to reach the site of infection in vivo by chemotaxis, others described an unchanged infection risk in G-CSF-treated HSC donors (52). Taken together, this suggests a potential compensation by number if neutrophil functionality is reduced.
Previously, it was shown that CRP levels increased upon G-CSF treatment (53). Our data confirms this finding. Moreover, our observations provide a possible link between increased CRP plasma levels in G-CSF-treated individuals and a negative effect on neutrophil chemotaxis. Of note, CRP reduced the chemotactic ability of neutrophils in vitro (33).
To judge whether reduced chemotaxis in neutrophils is a direct effect of the G-CSF present in blood, we have detected G-CSF plasma levels. Even in treated HSC donors, G-CSF plasma levels ranged around 1 ng/ml. Other studies have described slightly higher plasma concentrations ranging from 4 to > 15 ng/ml (35). In our in vitro experiments with purified neutrophils, 10 ng/ml G-CSF had no effect on neutrophil chemotaxis, only 50 ng/ml G-CSF impacted neutrophil chemotaxis. Taking this into consideration, our findings indicate that lower IL-8 and higher CRP concentrations in combination with a direct effect of G-CSF might render neutrophils less motile in G-CSF-treated individuals. Reduced IL-8 levels in plasma of G-CSF treated individuals could stem from non-neutrophil IL-8-sequestering cells.
In our cohorts, ROS production and phagocytosis were not affected by systemic G-CSF treatment in healthy HSC donors or in allogeneic recipients. Oxidative burst and phagocytosis remained unchanged in all cohorts and at all time points of sampling. This specificity suggests that the reduced chemotaxis in our cohorts is not a result of general immaturity of neutrophils mediated by G-CSF as immaturity of neutrophils is usually accompanied by the impairment of other functions as well (54, 55). It should be mentioned that other studies investigating neutrophil function of allogeneic recipients as reviewed by Ramaprasad et al. reported effects on ROS production or phagocytosis (56). Scholl and coworkers, for instance, observed an impaired oxidative burst in neutrophils from allogeneic recipients when stimulating with E. coli, but not when stimulating with PMA, confirming our findings (57). Macey and colleagues described impaired phagocytic ability of neutrophils after transplantation, however these patients received additional G-CSF treatment, which is in contrast to our study (58).
Allogeneic transplant recipients are susceptible to infections (59). With a weakened immune system, often combined with immunosuppressive treatments, an infection can often be a fatal complication. To judge the immune status of patients at risk, it is still common practice to rely on the neutrophil count in blood. To the best of our knowledge, there is no clinical tool available to evaluate the functionality of innate immune cells. In our study, we show that neutrophils recruited by G-CSF treatment in healthy HSC donors displayed decreased functionality in chemotaxis. Notably, this decrease persisted temporarily in allogeneic transplant recipients after neutrophil engraftment—even though these patients did not receive G-CSF themselves. Therefore, we conclude that it is possible that G-CSF could have a similar effect on neutrophils in blood cancer patients undergoing G-CSF treatment. Our study provides evidence that this regimen might require reconsideration that is more thorough. Henceforth, our findings imply that chemotaxis is a sensitive neutrophil function, which seems to be impaired early on during treatment and disease. From another point of view, our results provide evidence that chemotaxis could serve as reporter for neutrophil functionality in the clinical setting. With this, our study serves as a promising starting point for future prospective studies on chemotaxis as functional marker related to infection risk in patients with hematological diseases and other patient groups receiving G-CSF as prophylactic treatment.
Author Contributions
DE, AW, CA, MN, and CU designed the research study. AW, FÅ, and AT recruited and took care of the donors and patients. AT, MN, AH, DE, MS, and MR performed the experiments. AT, MN, AH, MS, and CU analyzed the data. AT, MN, DE, and CU wrote the paper.
Funding
This work was supported by grants for CFU from the Cancer Research Foundation in Northern Sweden (AMP 11-684 & LP 10-1833), the Västerbotten County Council (VLL-ALF), Laboratory for Molecular Infection Medicine Sweden (MIMS) and the Medical Faculty Umeå University (316-886-10).
Conflict of Interest Statement
The authors declare that the research was conducted in the absence of any commercial or financial relationships that could be construed as a potential conflict of interest.
Acknowledgments
We thank Dr. Dorothea Kretschmer and Prof. Andreas Peschel (both University of Tübingen) for kindly sharing their expertise on chemotaxis assessment, Dr. Niklas Aehling (University Hospital Leipzig) for carefully revising our manuscript and Erik Gillenius for helping with experiments.
Supplementary Material
The Supplementary Material for this article can be found online at: https://www.frontiersin.org/articles/10.3389/fimmu.2018.01968/full#supplementary-material
References
1. Mcclatchey KD. Clinical Laboratory Medicine. Philadelphia, PA: Lippincott Williams and Wilkins (2002).
2. Mayadas TN, Cullere X, Lowell CA. The multifaceted functions of neutrophils. Annu Rev Pathol. (2014) 9:181–218. doi: 10.1146/annurev-pathol-020712-164023
3. Leliefeld PH, Koenderman L, Pillay J. How neutrophils shape adaptive immune responses. Front Immunol. (2015) 6:471. doi: 10.3389/fimmu.2015.00471
4. Borregaard N, Sorensen OE, Theilgaard-Monch K. Neutrophil granules: a library of innate immunity proteins. Trends Immunol. (2007) 28:340–5. doi: 10.1016/j.it.2007.06.002
5. Nordenfelt P, Tapper H. Phagosome dynamics during phagocytosis by neutrophils. J Leukoc Biol. (2011) 90:271–84. doi: 10.1189/jlb.0810457
6. Cowland JB, Borregaard N. Granulopoiesis and granules of human neutrophils. Immunol Rev. (2016) 273:11–28. doi: 10.1111/imr.12440
7. Brinkmann V, Reichard U, Goosmann C, Fauler B, Uhlemann Y, Weiss DS, et al. Neutrophil extracellular traps kill bacteria. Science (2004) 303:1532–5. doi: 10.1126/science.1092385
8. Henig I, Zuckerman T. Hematopoietic stem cell transplantation-50 years of evolution and future perspectives. Rambam Maimonides Med J. (2014) 5:e0028. doi: 10.5041/RMMJ.10162
9. Boxer LA, Yokoyama M, Wiebe RA. Autoimmune neutropenia associated with chronic active hepatitis. Am J Med. (1972) 52:279–82. doi: 10.1016/0002-9343(72)90076-9
10. Pagano L, Tacconelli E, Tumbarello M, Laurenti L, Ortu-La Barbera E, Antinori A, et al. Bacteremia in patients with hematological malignancies. Analysis of risk factors, etiological agents and prognostic indicators Haematologica (1997) 82:415–9.
11. Andres E, Maloisel F. Idiosyncratic drug-induced agranulocytosis or acute neutropenia. Curr Opin Hematol. (2008) 15:15–21. doi: 10.1097/MOH.0b013e3282f15fb9
12. Andres E, Mourot-Cottet R. Non-chemotherapy drug-induced neutropenia - an update. Expert Opin Drug Saf. (2017) 16:1235–42. doi: 10.1080/14740338.2017.1376645
13. Donadieu J, Beaupain B, Fenneteau O, Bellanne-Chantelot C. Congenital neutropenia in the era of genomics: classification, diagnosis, and natural history. Br J Haematol. (2017) 179:557–74. doi: 10.1111/bjh.14887
14. Konidari A, Matary WE. Use of thiopurines in inflammatory bowel disease: safety issues. World J Gastrointest Pharmacol Ther. (2014) 5:63–76. doi: 10.4292/wjgpt.v5.i2.63
15. Omair MA, Alahmadi A, Johnson SR. Safety and effectiveness of mycophenolate in systemic sclerosis. A systematic review PLoS ONE (2015) 10:e0124205. doi: 10.1371/journal.pone.0124205
16. Omrani AS, Almaghrabi RS. Complications of hematopoietic stem cell transplantation: bacterial infections. Hematol Oncol Stem Cell Ther. (2017) 10:228–32. doi: 10.1016/j.hemonc.2017.05.018
17. Omrani AS, Almaghrabi RS. Complications of hematopoietic stem transplantation: fungal infections. Hematol Oncol Stem Cell Ther. (2017) 10:239–44. doi: 10.1016/j.hemonc.2017.05.013
18. Kansara R, Kumar R, Seftel M. Is primary prophylaxis with granulocyte colony stimulating factor (G-CSF) indicated in the treatment of lymphoma? Transfus Apher Sci. (2013) 49:51–5. doi: 10.1016/j.transci.2013.05.026
19. Lyman GH, Allcott K, Garcia J, Stryker S, Li Y, Reiner MT, et al. The effectiveness and safety of same-day versus next-day administration of long-acting granulocyte colony-stimulating factors for the prophylaxis of chemotherapy-induced neutropenia: a systematic review. Support Care Cancer (2017) 25:2619–29. doi: 10.1007/s00520-017-3703-y
20. Dekker A, Bulley S, Beyene J, Dupuis LL, Doyle JJ, Sung L. Meta-analysis of randomized controlled trials of prophylactic granulocyte colony-stimulating factor and granulocyte-macrophage colony-stimulating factor after autologous and allogeneic stem cell transplantation. J Clin Oncol. (2006) 24:5207–15. doi: 10.1200/JCO.2006.06.1663
21. Takeyama K, Ohto H. PBSC mobilization. Transfus Apher Sci. (2004) 31:233–43. doi: 10.1016/j.transci.2004.09.007
22. Roberts AW. G-CSF: a key regulator of neutrophil production, but that's not all!. Growth Factors (2005) 23:33–41. doi: 10.1080/08977190500055836
23. Panopoulos AD, Watowich SS. Granulocyte colony-stimulating factor: molecular mechanisms of action during steady state and ‘emergency’ hematopoiesis. Cytokine (2008) 42:277–88. doi: 10.1016/j.cyto.2008.03.002
24. Bartels M, Murphy K, Rieter E, Bruin M. Understanding chronic neutropenia: life is short. Br J Haematol. (2016) 172:157–69. doi: 10.1111/bjh.13798
25. Aga E, Katschinski DM, Van Zandbergen G, Laufs H, Hansen B, Muller K, et al. Inhibition of the spontaneous apoptosis of neutrophil granulocytes by the intracellular parasite Leishmania major. J Immunol. (2002) 169:898–905. doi: 10.4049/jimmunol.169.2.898
26. Hosseinzadeh A, Urban CF. Novel insight into neutrophil immune responses by dry mass determination of Candida albicans morphotypes. PLoS ONE (2013) 8:e77993. doi: 10.1371/journal.pone.0077993
27. Niemiec MJ, Grumaz C, Ermert D, Desel C, Shankar M, Lopes JP, et al. Dual transcriptome of the immediate neutrophil and Candida albicans interplay. BMC Genomics (2017) 18:696. doi: 10.1186/s12864-017-4097-4
28. Yokoyama Y, Suzuki T, Sakata-Yanagimoto M, Kumano K, Higashi K, Takato T, et al. Derivation of functional mature neutrophils from human embryonic stem cells. Blood (2009) 113:6584–92. doi: 10.1182/blood-2008-06-160838
29. Pillay J, Tak T, Kamp VM, Koenderman L. Immune suppression by neutrophils and granulocytic myeloid-derived suppressor cells: similarities and differences. Cell Mol Life Sci. (2013) 70:3813–27. doi: 10.1007/s00018-013-1286-4
30. Dürr MC, Kristian SA, Otto M, Matteoli G, Margolis PS, Trias J, et al. Neutrophil chemotaxis by pathogen-associated molecular patterns–formylated peptides are crucial but not the sole neutrophil attractants produced by Staphylococcus aureus. Cell Microbiol. (2006) 8:207–17. doi: 10.1111/j.1462-5822.2005.00610.x
31. Kretschmer D, Rautenberg M, Linke D, Peschel A. Peptide length and folding state govern the capacity of staphylococcal beta-type phenol-soluble modulins to activate human formyl-peptide receptors 1 or 2. J Leukoc Biol. (2015) 97:689–97. doi: 10.1189/jlb.2A0514-275R
32. Pichert A, Schlorke D, Franz S, Arnhold J. Functional aspects of the interaction between interleukin-8 and sulfated glycosaminoglycans. Biomatter (2012) 2:142–8. doi: 10.4161/biom.21316
33. Zhong W, Zen Q, Tebo J, Schlottmann K, Coggeshall M, Mortensen RF. Effect of human C-reactive protein on chemokine and chemotactic factor-induced neutrophil chemotaxis and signaling. J Immunol. (1998) 161:2533–40.
34. Holig K. G-CSF in healthy allogeneic stem cell donors. Transfus Med Hemother. (2013) 40:225–35. doi: 10.1159/000354196
35. Hatfield KJ, Melve GK, Bruserud O. Granulocyte colony-stimulating factor alters the systemic metabolomic profile in healthy donors. Metabolomics (2017) 13:2. doi: 10.1007/s11306-016-1139-x
36. Hendey B, Klee CB, Maxfield FR. Inhibition of neutrophil chemokinesis on vitronectin by inhibitors of calcineurin. Science (1992) 258:296–9. doi: 10.1126/science.1384129
37. Wenzel-Seifert K, Grunbaum L, Seifert R. Differential inhibition of human neutrophil activation by cyclosporins A, D, and H. Cyclosporin H is a potent and effective inhibitor of formyl peptide-induced superoxide formation J Immunol. (1991) 147:1940–6.
38. Thorat SP, Thatte UM, Pai N, Dahanukar SA. Inhibition of phagocytes by cyclosporin in vitro. Q J Med. (1994) 87:311–4.
39. Sosa R, Weiden PL, Storb R, Syrotuck J, Thomas ED. Granulocyte function in human allogenic marrow graft recipients. Exp Hematol. (1980) 8:1183–9.
40. Katoh M, Takada M, Nakayama M, Shikoshi K, Umeda M, Tsuji A. [Combined deficiency in neutrophil functions after bone marrow transplantation and the in vitro effect of granulocyte colony-stimulating factor]. Rinsho Ketsueki (1997) 38:566–71.
41. Azzara A, Carulli G, Rizzuti-Gullaci A, Minnucci S, Capochiani E, Ambrogi F. Motility of rhG-CSF-induced neutrophils in patients undergoing chemotherapy: evidence for inhibition detected by image analysis. Br J Haematol. (1996) 92:161–8. doi: 10.1046/j.1365-2141.1996.00295.x
42. Kerst JM, De Haas M, Van Der Schoot CE, Slaper-Cortenbach IC, Kleijer M, Von Dem Borne AE, et al. Recombinant granulocyte colony-stimulating factor administration to healthy volunteers: induction of immunophenotypically and functionally altered neutrophils via an effect on myeloid progenitor cells. Blood (1993) 82:3265–72.
43. Dale DC, Liles WC, Llewellyn C, Rodger E, Price TH. Neutrophil transfusions: kinetics and functions of neutrophils mobilized with granulocyte-colony-stimulating factor and dexamethasone. Transfusion (1998) 38:713–21. doi: 10.1046/j.1537-2995.1998.38898375509.x
44. Hoglund M, Hakansson L, Venge P. Effects of In vivo administration of G-CSF on neutrophil functions in healthy volunteers. Eur J Haematol. (1997) 58:195–202. doi: 10.1111/j.1600-0609.1997.tb00947.x
45. Nakamae-Akahori M, Kato T, Masuda S, Sakamoto E, Kutsuna H, Hato F, et al. Enhanced neutrophil motility by granulocyte colony-stimulating factor: the role of extracellular signal-regulated kinase and phosphatidylinositol 3-kinase. Immunology (2006) 119:393–403. doi: 10.1111/j.1365-2567.2006.02448.x
46. Pillay J, Den Braber I, Vrisekoop N, Kwast LM, De Boer RJ, Borghans JA, et al. In vivo labeling with 2H2O reveals a human neutrophil lifespan of 5.4 days. Blood (2010) 116:625–7. doi: 10.1182/blood-2010-01-259028
47. Amulic B, Cazalet C, Hayes GL, Metzler KD, Zychlinsky A. Neutrophil function: from mechanisms to disease. Annu Rev Immunol. (2011) 30:459–89. doi: 10.1146/annurev-immunol-020711-074942
48. Tofts PS, Chevassut T, Cutajar M, Dowell NG, Peters AM. Doubts concerning the recently reported human neutrophil lifespan of 5.4 days. Blood (2011) 117:6050–2; author reply 6053–4. doi: 10.1182/blood-2010-10-310532
49. Humphreys JM, Rugman FP, Davies JM, Mimnagh P, Hart CA, Edwards SW. Effects of recombinant human granulocyte colony-stimulating factor on neutrophil function in vitro and In vivo following chemotherapy and autologous bone marrow transplantation. J Clin Lab Immunol. (1991) 34:55–61.
50. Watanabe T, Kawano Y, Kanamaru S, Onishi T, Kaneko S, Wakata Y, et al. Endogenous interleukin-8 (IL-8) surge in granulocyte colony-stimulating factor-induced peripheral blood stem cell mobilization. Blood (1999) 93:1157–63.
51. Baron F, Nagler A. Novel strategies for improving hematopoietic reconstruction after allogeneic hematopoietic stem cell transplantation or intensive chemotherapy. Expert Opin Biol Ther. (2017) 17:163–74. doi: 10.1080/14712598.2017.1269167
52. Hölig K, Kramer M, Kroschinsky F, Bornhäuser M, Mengling T, Schmidt AH, et al. Safety and efficacy of hematopoietic stem cell collection from mobilized peripheral blood in unrelated volunteers: 12 years of single-center experience in 3928 donors. Blood (2009) 114:3757–63. doi: 10.1182/blood-2009-04-218651
53. Ilhan Ç, Suyani E, Sucak GT, Paşaoglu Ö, Aki S, Paşaoglu H. Inflammatory markers, oxidative stress, and antioxidant capacity in healthy allo-HSCT donors during hematopoietic stem cell mobilization. J Clin Apher. (2015) 30:197–203. doi: 10.1002/jca.21361
54. Taneja R, Sharma AP, Hallett MB, Findlay GP, Morris MR. Immature circulating neutrophils in sepsis have impaired phagocytosis and calcium signaling. Shock (2008) 30:618–22. doi: 10.1097/SHK.0b013e318173ef9c
55. Drifte G, Dunn-Siegrist I, Tissières P, Pugin J. Innate immune functions of immature neutrophils in patients with sepsis and severe systemic inflammatory response syndrome. Crit Care Med. (2013) 41:820–32. doi: 10.1097/CCM.0b013e318274647d
56. Ramaprasad C, Pouch S, Pitrak DL. Neutrophil function after bone marrow and hematopoietic stem cell transplant. Leuk Lymphoma (2010) 51:756–67. doi: 10.3109/10428191003695678
57. Scholl S, Hanke M, Hoffken K, Sayer HG. Distinct reconstitution of neutrophil functions after allogeneic peripheral blood stem cell transplantation. J Cancer Res Clin Oncol. (2007) 133:411–5. doi: 10.1007/s00432-006-0187-4
58. Macey MG, Sangster J, Kelsey SM, Newland AC. Pilot study: effects of G-CSF on neutrophil ex-vivo function post bone marrow transplantation. Clin Lab Haematol. (1993) 15:79–85. doi: 10.1111/j.1365-2257.1993.tb00130.x
Keywords: neutrophil, granulocyte colony stimulating factor (G-CSF), allogeneic transplant, chemotaxis, hematopoietic stem cell donor
Citation: Thunström Salzer A, Niemiec MJ, Hosseinzadeh A, Stylianou M, Åström F, Röhm M, Ahlm C, Wahlin A, Ermert D and Urban CF (2018) Assessment of Neutrophil Chemotaxis Upon G-CSF Treatment of Healthy Stem Cell Donors and in Allogeneic Transplant Recipients. Front. Immunol. 9:1968. doi: 10.3389/fimmu.2018.01968
Received: 30 May 2018; Accepted: 10 August 2018;
Published: 11 September 2018.
Edited by:
Ulrike Koehl, Hannover Medical School, GermanyReviewed by:
Nina Worel, Universitätsklinik für Blutgruppenserologie und Transfusionsmedizin, Medizinische Universität Wien, AustriaMichael Uhlin, Karolinska Institutet (KI), Sweden
Copyright © 2018 Thunström Salzer, Niemiec, Hosseinzadeh, Stylianou, Åström, Röhm, Ahlm, Wahlin, Ermert and Urban. This is an open-access article distributed under the terms of the Creative Commons Attribution License (CC BY). The use, distribution or reproduction in other forums is permitted, provided the original author(s) and the copyright owner(s) are credited and that the original publication in this journal is cited, in accordance with accepted academic practice. No use, distribution or reproduction is permitted which does not comply with these terms.
*Correspondence: David Ermert, david.ermert@med.lu.se
Constantin F. Urban, constantin.urban@umu.se
† These authors share first authorship
‡Present Address: Maria J. Niemiec, Leibniz Institute for Natural Product Research and Infection Biology, Hans Knöll Institute & Center for Sepsis Control and Care, Jena, Germany
Marios Stylianou, School of Science and Technology, University of Örebro, Örebro, Sweden
David Ermert, Department of Translational Medicine, Division of Medical Protein Chemistry, Lund University, Malmö, Sweden
§ These authors have contributed equally to this work and share senior authorship