- 1Department of Immunopathology, Sanquin Research and Landsteiner Laboratory of the Academic Medical Centre, University of Amsterdam, Amsterdam, Netherlands
- 2Department of Pediatric Hematology, Immunology and Infectious Diseases, Emma Children’s Hospital, Academic Medical Centre, Amsterdam, Netherlands
- 3Sanquin Diagnostic Services, Amsterdam, Netherlands
- 4Department of Blood Cell Research, Sanquin Research and Landsteiner Laboratory of the Academic Medical Centre, University of Amsterdam, Amsterdam, Netherlands
Complement is activated as part of the innate immune defense against invading pathogens. Also, it helps to remove apoptotic debris and immune complexes from the circulation. Impaired complement function due to aberrant plasma levels of complement proteins may be indicative for complement-mediated diseases or can be involved in susceptibility for infections. To determine whether plasma levels are abnormal, reference intervals (RIs) are used from adult healthy donors. Since many complement-mediated diseases have an onset during childhood, it is important to know whether these RIs can be extrapolated to children. RIs of Factor H (FH), the crucial fluid-phase regulator, and the FH-related proteins (FHRs), its homologous counterparts, are unknown in healthy children. While FH is measured to diagnose and monitor therapy of patients with atypical hemolytic uremic syndrome, recent studies also implicated increased plasma levels of FHRs in disease. Here, we investigated the levels of FH and FHRs in healthy children using recently developed specific ELISAs. We found that levels of FH, FHR-2, and FHR-3 were equal to those found in healthy adults. Levels of FHR-4A and FHR-5 were lower in children than in adults. However, only the FHR-5 levels associated with age. The RIs of these FH family proteins now serve to support the interpretation of plasma levels in prospective and retrospective studies that can be used for routine diagnostic and monitoring purposes including pediatric patient samples.
Introduction
Complement is part of innate immunity, comprising a powerful cascade of proteins able to eradicate invading pathogens and is important for removal of apoptotic debris and immune complexes from the circulation. Complement activation is tightly controlled and regulator proteins make sure that bystander damage to healthy host cells is kept to a minimum. Within the population, there is variation in the expression levels of these proteins and other complement components, leading to different steady-state complement activities in healthy individuals (1). Assessment of abnormal circulating levels can help to diagnose complement-mediated diseases such as atypical hemolytic uremic syndrome (aHUS) and differences in expression levels can help in understanding the susceptibility for infectious diseases as described in retrospective studies (2).
To discriminate between normal and abnormal levels, and to interpret retrospective studies, clinical laboratory reference intervals (RIs) are needed. As many complement-mediated diseases can have their onset during childhood, it is important to know whether adult levels can be extrapolated toward pediatric patients. For proteins such as C3 and C4, it has been determined that the normal ranges can be different in childhood compared to adults and between different ethnicities, and as such, adjusted RIs may be used (3–6). No pediatric RIs are known of Factor H (FH) and the FH-related proteins (FHRs), of which their plasma levels associate with various diseases.
Factor H is a crucial regulator of the alternative complement pathway and protects human host cells from unwanted complement activation. Genetic variants in complement regulator FH are associated with multiple diseases. Such variants can either alter protein functionality or induce variation in levels of expression. Many have been described to associate with aHUS or age-related macular degeneration, affecting the regulating function of FH (7, 8). However, some genetic variants result in lower (insufficient) circulating levels of FH (9–11). Differences in steady-state FH protein levels are associated with susceptibility for meningococcal disease and have recently been implicated as a marker of cardiovascular risk in chronic Chagas disease (12, 13). In general, low expression of complement regulators, such as FH, would make an individual more prone for chronic inflammation but more protected against infectious diseases, while high expression rather associates with risk of infectious diseases but less chronic inflammation (14).
Apart from FH, the FH protein family also includes the short splice variant of FH, FH-like-1 (FHL-1), and the FH-related (FHR) proteins, named FHR-1, FHR-2, FHR-3, FHR-4, and FHR-5, all of which are encoded by their own gene. FHR-4A and FHR-4B are the two splice variants of CFHR4, but FHR-4A is the only circulating variant found in human serum (15). While FHRs share homology with FH in its surface binding domains, they lack domains similar to SCR1-4 in FH and FHL-1, and for that reason are believed to have no complement-regulatory activity (16). Although limited data are available on the in vivo function of FHRs, many have shown associations of complement-mediated diseases with these FHR genes due to their copy number variations (17, 18), internal duplications (19–21), fusion proteins (22–26), or polymorphisms (27–30).
In addition, recent developments in the determination of circulating FHR levels in adults have led to the discovery of new associations with disease. FHR-1 levels were shown to be increased during IgA nephropathy (31, 32), although the authors report much higher levels than we and others have published (33, 34). FHR-3 levels were shown to be elevated during sepsis (35) and in systemic lupus erythematosus, rheumatoid arthritis, and polymyalgia rheumatica (36). Although Schäfer et al. did not find increased FHR-3 levels in aHUS patients, a recent study demonstrated increased levels in a larger, well-characterized cohort (36, 37). FHR-2 and FHR-4A levels have, so far, not been studied except in healthy donors, although FHR-2 and FHR-4A are implicated in the acute phase of bacterial infections (van Beek et al., manuscript in preparation) (15, 33, 38). FHR-5 levels were shown to be decreased in patients with C3 glomerulonephropathy (C3G) (39) and was recently identified as an independent risk factor for IgA nephropathy (32, 40). In summary, assessment of FHR protein levels contributes to the understanding of various diseases.
To investigate whether different RIs should be used for FH and the FHRs in children, we assessed the circulating levels in a cohort of healthy Dutch children and adolescents (all referred to as children), covering various age categories. These RIs now serve to support the interpretation of plasma levels in retrospective studies that include children. Moreover, they can be used for routine diagnostic and monitoring purposes in pediatric patient samples.
Materials and Methods
Samples
Serum samples were obtained from anonymous, healthy children from a previous study, in accordance with Dutch regulations and approved by the Sanquin Ethical Advisory Board in accordance with the Declaration of Helsinki (41). Samples from adult healthy donors (n = 124 for FH and FHR-3, n = 120 for FHR-1, 2, 4A, and 5) were collected and measured during previous studies (15, 33, 35).
ELISAs
All ELISAs were performed as previously described for an adult healthy donor cohort (15, 33, 35). Briefly, the FH ELISA uses anti-FH.16, a monospecific mAb directed against SCR16-17, as a coat and goat anti-human-FH antiserum as detection. FHR-1/1 homodimers were measured using anti-FH.02 (directed against SCR20 of FH and cross-reactive to SCR5 of FHR-1) both as catching and detecting mAb. FHR-1/2 homodimers were also caught by anti-FH.02, but detected with a commercially available anti-FHR-2 (R&D Systems). FHR-2/2 homodimer levels, as well as total levels of FHR-1 and FHR-2 were calculated based on the observed levels of FHR-1/1 and FHR-1/2 dimers. The FHR-3 ELISA uses anti-FHR-3.1 (cross-reactive to FHR-4A) as a coating mAb and anti-FHR-3.4 (cross-reactive to FH) as a detecting mAb. FHR-4A was measured by catching with the monospecific mAb anti-FHR-4A.04 and detecting with rabbit anti-FHR-3 antiserum. FHR-5 homodimers were measured using two monospecific mAbs, anti-FHR-5.1 and anti-FHR-5.4. Two control sera were included in each plate to ensure limited inter assay variation.
Statistics
GraphPad Prism software v7 was used to analyze data and perform statistics (GraphPad Software, La Jolla, CA, USA). Significant differences were assessed by unpaired t-test. Correlations were assessed with a parametric Pearson’s correlation test.
Results
With this study, we obtained more insight in the normal ranges of FH and the FHRs in children. For this, we used a cohort of 110 healthy children, of which 53% were females (Table 1). The subjects were evenly distributed across the age categories, aged 7 months up to 251 months (20.9 years) (41). We compared the levels in children to the levels that we previously found in adult healthy Dutch donors (15, 33, 35).
We investigated the plasma levels of FH and the FHRs in these healthy children (Figure 1). We observed that the levels of FH and FHR-3 were similar between the two genders and independent of age (Figures 1A,G). Indeed, the levels are equal to those previously found in adult healthy Dutch donors (Table 2) (35).
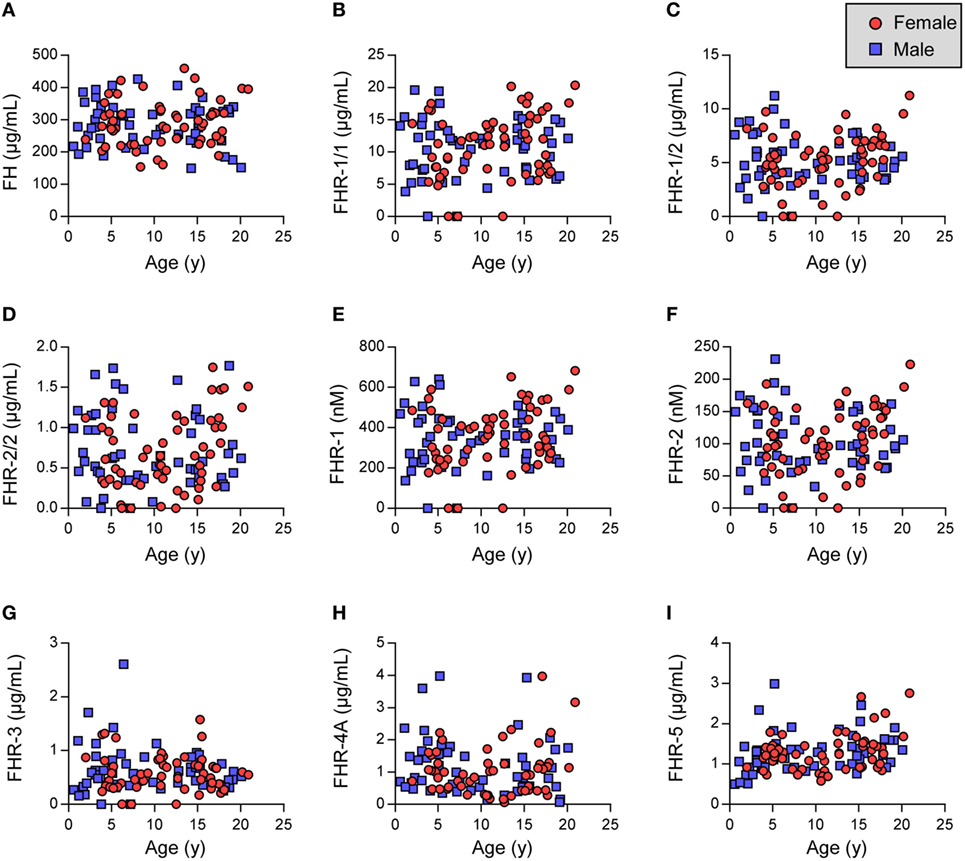
Figure 1. Factor H (FH) family proteins in healthy children. (A–C,G–I) Show FH and FHRs as assessed by in-house ELISA. (D–F) Indicate calculated FHR-1 and FHR-2 levels. (D,F) Samples lacking FHR-1 (likely CFHR1 deficient) were excluded. Females are indicated by red circles, males by blue squares. Data were analyzed using Pearson’s correlation and unpaired t-tests (Table 2).
Next, FHR-1 and FHR-2 were assessed using dimer-specific ELISAs (Figures 1B,C). The levels of FHR-1/1 homodimers were independent of age and gender, although we did find a minor, but significant, difference when comparing the FHR-1/1 levels to adults (Table 2, difference between means = 1.2 µg/mL) (33). FHR-1/2 heterodimers and FHR-2/2 homodimers were also found to be independent of age and gender but were similar to the adult healthy donors (Figure 1D; Table 2). This implied that only the FHR-1 plasma levels differed from the adults. Indeed, when we calculated the concentrations of total FHR-1 and FHR-2 monomers, only FHR-1 levels were significantly lower [difference between means = 1.3 µg/mL (33 nM)] than the healthy adults (Figures 1E,F; Table 2).
We recently demonstrated that FHR-4A is the only circulating form of FHR-4 and that no FHR-4B could be observed in serum (15). Therefore, we measured only FHR-4A in the children and found that FHR-4A levels were lower than expected based on levels found in adult healthy donors (Figure 1H; Table 2). It would, therefore, be expected that the levels showed an association with age. Surprisingly, the FHR-4A levels did neither show an association with age, nor with gender, in the children.
Last, we assessed the levels of FHR-5/5 homodimers. Similar to the other FHR proteins, FHR-5 levels were independent of gender. However, the levels did increase with age (Figure 1I, Table 2), being approximately 0.5 µg/mL lower in the youngest children than in the oldest children. While the younger children indeed showed significantly lower levels, the older children presented with levels equal to the adult healthy donors.
As the CFHR genes originated as part of segmental duplications of the CFH gene, it would be possible that protein expression is similarly regulated (42). Therefore, we investigated whether FH plasma levels associated with plasma levels of the FHRs. We saw an association between FH and FHR-1/1 homodimer levels in adult donors, when they carry two copies of CFHR1 (r = 0.62, P < 0.0001), in contrast to those who carry only 1 copy of CFHR1 (r = 0.09, P = 0.67) (33, 35). Children who most likely carry two copies of CFHR1 [expressing > 10.1 µg/mL FHR-1/1 homodimers, as determined by ROC analysis (area under the curve = 0.97)] showed a similar association (r = 0.49, P < 0.0001) (33). No association between FH and other FHR levels was noted. As a general conclusion, we observed no remarkable differences compared to adult circulating levels of FH family proteins.
Discussion
We have determined RIs for FH and FHR-1 to 5 in Dutch healthy children. We were able to interpret the circulating levels of these FH family proteins in relation to adult healthy donors, which we have previously assessed (15, 33, 35). We found differences in some but not all of these proteins in the healthy children when compared with adults.
In contrast to FHR-1, FHR-4A, and FHR-5, no remarkable observations were made when analyzing the circulating levels of FH, FHR-2, and FHR-3. The three proteins were independent of age and gender, confirming a previous study on FH in Brazilian children (43). FH levels were previously found to be low in neonates, suggesting that plasma levels reach adult ranges within the first 6 months after birth (44, 45). Unfortunately, no sera were available from children below the age of 6 months. Future studies should test cord blood and plasma of neonates for the presence of FHRs at birth and early infancy to investigate these protein levels in more detail.
FHR-1 levels were independent of age and gender. We did observe lower FHR-1 levels than previously seen in adults, although the biological relevance may be disputed. FHR-3 levels were also trending toward significance, indicating that a minor difference in the copy number variation in CFHR3/CFHR1 between the two cohorts might be affecting the results (33, 35).
We found lower FHR-4A levels in children than in adults, even though FHR-4A did not associate with age of the children. Our group demonstrated previously that FHR-4A is stable up to at least 10 freeze-thaw cycles (15). However, we cannot exclude the possibility that long-term storage of these samples may have suffered from breakdown of FHR-4A when kept at −30°C (15). New studies on more recent samples are needed to confirm or disprove this possible explanation.
For FHR-5, we observed an increase with age, indicating that normal ranges for FHR-5 are low in the youngest children and that RIs may need to be adjusted accordingly. As FHR-5 levels positively associated with severity of IgA nephropathy in adults (32), and as IgA nephropathy is the main nephropathy in children (46), measurements of FHR-5 in a pediatric cohort will be highly informative to further study the role of FHR-5 in this nephropathy.
This study represents the most complete assessment of FH family proteins to date in a cohort of healthy children providing RIs. These RIs can now be used to interpret serum levels in prospective and retrospective studies that include children and used for routine diagnostic and monitoring purposes in pediatric patient samples. Ideally, each laboratory should adapt these RIs for their own assays.
Ethics Statement
Serum samples were obtained from anonymous, healthy children from a previous study, in accordance with Dutch regulations and approved by the Sanquin Ethical Advisory Board in accordance with the Declaration of Helsinki.
Author Contributions
AB, DW, TK, and KG designed research. AB, AK, SK, and EN performed research. AB, IJ, TR, TK, and KG analyzed data and wrote the paper. All authors critically reviewed the manuscript, gave final approval of the version to be published, and agreed to be accountable for all aspects of the work in ensuring that questions related to the accuracy or integrity of any part of the work are appropriately investigated and resolved.
Conflict of Interest Statement
The authors declare that the research was conducted in the absence of any commercial or financial relationships that could be construed as a potential conflict of interest.
Funding
Research leading to these results has received funding from the European Union’s seventh Framework program under EC-GA no. 279185 (EUCLIDS; www.euclids-project.eu). The funders had no role in study design, data collection and analysis, decision to publish, or preparation of the manuscript.
References
1. Harris CL, Heurich M, Rodriguez de Cordoba S, Morgan BP. The complotype: dictating risk for inflammation and infection. Trends Immunol (2012) 33:513–21. doi:10.1016/j.it.2012.06.001
2. Davila S, Wright VJ, Khor CC, Sim KS, Binder A, Breunis WB, et al. Genome-wide association study identifies variants in the CFH region associated with host susceptibility to meningococcal disease. Nat Genet (2010) 42:772–6. doi:10.1038/ng.640
3. Jolliff CR, Cost KM, Stivrins PC, Grossman PP, Nolte CR, Franco SM, et al. Reference intervals for serum IgG, IgA, IgM, C3, and C4 as determined by rate nephelometry. Clin Chem (1982) 28:126–8.
4. Roach B, Kim Y, Jerome E, Michael AF. Influence of age and sex on serum complement components in children. Am J Dis Child (1981) 135:918–20.
5. Kardar GA, Oraei M, Shahsavani M, Namdar Z, Kazemisefat GE, Haghi Ashtiani MT, et al. Reference intervals for serum immunoglobulins IgG, IgA, IgM and complements C3 and C4 in Iranian healthy children. Iran J Public Health (2012) 41:59–63.
6. Norman ME, Gall EP, Taylor A, Laster L, Nilsson UR. Serum complement profiles in infants and children. J Pediatr (1975) 87:912–6. doi:10.1016/S0022-3476(75)80904-8
7. Kavanagh D, Goodship TH, Richards A. Atypical hemolytic uremic syndrome. Semin Nephrol (2013) 33:508–30. doi:10.1016/j.semnephrol.2013.08.003
8. Toomey CB, Johnson LV, Bowes Rickman C. Complement factor H in AMD: bridging genetic associations and pathobiology. Prog Retin Eye Res (2018) 62:38–57. doi:10.1016/j.preteyeres.2017.09.001
9. Triebwasser MP, Roberson EDO, Yu Y, Schramm EC, Wagner EK, Raychaudhuri S, et al. Rare variants in the functional domains of complement factor H are associated with age-related macular degeneration. Investig Opthalmol Vis Sci (2015) 56:6873. doi:10.1167/iovs.15-17432
10. Dragon-Durey MA, Frémeaux-Bacchi V, Loirat C, Blouin J, Niaudet P, Deschenes G, et al. Heterozygous and homozygous factor H deficiencies associated with hemolytic uremic syndrome or membranoproliferative glomerulonephritis: report and genetic analysis of 16 cases. J Am Soc Nephrol (2004) 15:787–95. doi:10.1097/01.ASN.0000115702.28859.A7
11. Ansari M, McKeigue PM, Skerka C, Hayward C, Rudan I, Vitart V, et al. Genetic influences on plasma CFH and CFHR1 concentrations and their role in susceptibility to age-related macular degeneration. Hum Mol Genet (2013) 22:4857–69. doi:10.1093/hmg/ddt336
12. Haralambous E, Dolly SO, Hibberd ML, Litt DJ, Udalova IA, O’dwyer C, et al. Factor H, a regulator of complement activity, is a major determinant of meningococcal disease susceptibility in UK Caucasian patients. Scand J Infect Dis (2006) 38:764–71. doi:10.1080/00365540600643203
13. Lidani KCF, Sandri TL, Andrade FA, Bavia L, Nisihara R, Messias-Reason IJ. Complement Factor H as a potential atherogenic marker in chronis Chagas disease. Parasite Immunol (2018) 40(7):e12537. doi:10.1111/pim.12537
14. Heurich M, Martinez-Barricarte R, Francis NJ, Roberts DL, Rodriguez de Cordoba S, Morgan BP, et al. Common polymorphisms in C3, factor B, and factor H collaborate to determine systemic complement activity and disease risk. Proc Natl Acad Sci U S A (2011) 108:8761–6. doi:10.1073/pnas.1019338108
15. Pouw RB, Brouwer MC, Van Beek AE, Józsi M, Barlow PN, Sim RB, et al. Complement factor H-related protein 4A is the dominant circulating splice variant of CFHR4. Front Immunol (2018) 9:729. doi:10.3389/fimmu.2018.00729
16. Medjeral-Thomas N, Pickering MC. The complement factor H-related proteins. Immunol Rev (2016) 274:191–201. doi:10.1111/imr.12477
17. Kubista KE, Tosakulwong N, Wu Y, Ryu E, Roeder JL, Hecker LA, et al. Copy number variation in the complement factor H-related genes and age-related macular degeneration. Mol Vis (2011) 17:2080–92.
18. Cantsilieris S, White SJ, Richardson AJ, Guymer RH, Baird PN. Comprehensive analysis of copy number variation of genes at chromosome 1 and 10 loci associated with late age related macular degeneration. PLoS One (2012) 7:e35255. doi:10.1371/journal.pone.0035255
19. Tortajada A, Yébenes H, Abarrategui-garrido C, Anter J, García-fernández JM, Martínez-barricarte R, et al. C3 glomerulopathy–associated CFHR1 mutation alters FHR oligomerization and complement regulation. J Clin Invest (2013) 123:2434–46. doi:10.1172/JCI68280
20. Gale DP, de Jorge EG, Cook HT, Martinez-Barricarte R, Hadjisavvas A, McLean AG, et al. Identification of a mutation in complement factor H-related protein 5 in patients of Cypriot origin with glomerulonephritis. Lancet (2010) 376:794–801. doi:10.1016/S0140-6736(10)60670-8
21. Medjeral-Thomas N, Malik TH, Patel MP, Toth T, Cook HT, Tomson C, et al. A novel CFHR5 fusion protein causes C3 glomerulopathy in a family without Cypriot ancestry. Kidney Int (2014) 85:933–7. doi:10.1038/ki.2013.348
22. Xiao X, Ghossein C, Tortajada A, Zhang Y, Meyer N, Jones M, et al. Familial C3 glomerulonephritis caused by a novel CFHR5-CFHR2 fusion gene. Mol Immunol (2016) 77:89–96. doi:10.1016/j.molimm.2016.07.007
23. Chen Q, Wiesener M, Eberhardt HU, Hartmann A, Uzonyi B, Kirschfink M, et al. Complement factor H-related hybrid protein deregulates complement in dense deposit disease. J Clin Invest (2014) 124:145–55. doi:10.1172/JCI71866
24. Malik TH, Lavin PJ, Goicoechea de Jorge E, Vernon KA, Rose KL, Patel MP, et al. A hybrid CFHR3-1 gene causes familial C3 glomerulopathy. J Am Soc Nephrol (2012) 23:1155–60. doi:10.1681/ASN.2012020166
25. Valoti E, Alberti M, Tortajada A, Garcia-Fernandez J, Gastoldi S, Besso L, et al. A novel atypical hemolytic uremic syndrome-associated hybrid CFHR1/CFH gene encoding a fusion protein that antagonizes factor H-dependent complement regulation. J Am Soc Nephrol (2015) 26:209–19. doi:10.1681/ASN.2013121339
26. Togarsimalemath SK, Sethi SK, Duggal R, Le Quintrec M, Jha P, Daniel R, et al. A novel CFHR1-CFHR5 hybrid leads to a familial dominant C3 glomerulopathy. Kidney Int (2017) 92(4):876–87. doi:10.1016/j.kint.2017.04.025
27. Westra D, Vernon Ka, Volokhina EB, Pickering MC, van de Kar NC, van den Heuvel LP. Atypical hemolytic uremic syndrome and genetic aberrations in the complement factor H-related 5 gene. J Hum Genet (2012) 57:459–64. doi:10.1038/jhg.2012.57
28. Abrera-Abeleda Ma, Nishimura C, Smith JLH, Sethi S, McRae JL, Murphy BF, et al. Variations in the complement regulatory genes factor H (CFH) and factor H related 5 (CFHR5) are associated with membranoproliferative glomerulonephritis type II (dense deposit disease). J Med Genet (2006) 43:582–9. doi:10.1136/jmg.2005.038315
29. Bernabéu-Herrero ME, Jiménez-Alcázar M, Anter J, Pinto S, Sánchez Chinchilla D, Garrido S, et al. Complement factor H, FHR-3 and FHR-1 variants associate in an extended haplotype conferring increased risk of atypical hemolytic uremic syndrome. Mol Immunol (2015) 67:276–86. doi:10.1016/j.molimm.2015.06.021
30. Monteferrante G, Brioschi S, Caprioli J, Pianetti G, Bettinaglio P, Bresin E, et al. Genetic analysis of the complement factor H related 5 gene in haemolytic uraemic syndrome. Mol Immunol (2007) 44:1704–8. doi:10.1016/j.molimm.2006.08.004
31. Tortajada A, Gutiérrez E, Goicoechea de Jorge E, Anter J, Segarra A, Espinosa M, et al. Elevated factor H-related protein 1 and factor H pathogenic variants decrease complement regulation in IgA nephropathy. Kidney Int (2017) 92(4):953–63. doi:10.1016/j.kint.2017.03.041
32. Medjeral-thomas NR, Lomax-browne HJ, Beckwith H, Willicombe M, Mclean AG, Brookes P, et al. Circulating complement factor H-related proteins 1 and 5 correlate with disease activity in IgA nephropathy. Kidney Int (2017) 92:942–52. doi:10.1016/j.kint.2017.03.043
33. van Beek AE, Pouw RB, Brouwer MC, van Mierlo G, Geissler J, Ooijevaar-De Heer P, et al. Factor H-related (FHR)-1 and FHR-2 form homo- and heterodimers, while FHR-5 circulates only as homodimer in human plasma. Front Immunol (2017) 8:1328. doi:10.3389/fimmu.2017.01328
34. Kopczynska M, Zelek W, Touchard S, Gaughran F, Di Forti M, Mondelli V, et al. Complement system biomarkers in first episode psychosis. Schizophr Res (2017). doi:10.1016/j.schres.2017.12.012
35. Pouw RB, Brouwer MC, Geissler J, van Herpen LV, Zeerleder SS, Wuillemin WA, et al. Complement factor H-related protein 3 serum levels are low compared to factor H and mainly determined by gene copy number variation in CFHR3. PLoS One (2016) 11:e0152164. doi:10.1371/journal.pone.0152164
36. Schäfer N, Grosche A, Reinders J, Hauck S, Pouw RB, Kuijpers TW, et al. Complement regulator FHR-3 is elevated either locally or systemically in a selection of autoimmune diseases. Front Immunol (2016) 7:1–16. doi:10.3389/fimmu.2016.00542
37. Pouw RB, Delgado IG, Lera AL, Rodríguez de Córdoba S, Wouters D, Kuijpers TW, et al. High complement factor H-related (FHR)-3 levels are associated with the atypical hemolytic-uremic syndrome-risk allele CFHR3*B. Front Immunol (2018) 9:848. doi:10.3389/fimmu.2018.00848
38. Hebecker M, Józsi M. Factor H-related protein 4 activates complement by serving as a platform for the assembly of alternative pathway C3 convertase via its interaction with C3b protein. J Biol Chem (2012) 287:19528–36. doi:10.1074/jbc.M112.364471
39. Vernon Ka, Goicoechea de Jorge E, Hall AE, Fremeaux-Bacchi V, Aitman TJ, Cook HT, et al. Acute presentation and persistent glomerulonephritis following streptococcal infection in a patient with heterozygous complement factor H-related protein 5 deficiency. Am J Kidney Dis (2012) 60:121–5. doi:10.1053/j.ajkd.2012.02.329
40. Zhu L, Guo W, Shi S, Liu L, Lv J, Medjeral-thomas NR, et al. Circulating complement factor H-related protein 5 levels contribute to development and progression of IgA nephropathy. Kidney Int (2018) 94(1):150–8. doi:10.1016/j.kint.2018.02.023
41. Lepage N, Huang SHS, Nieuwenhuys E, Filler G. Pediatric reference intervals for immunoglobulin G and its subclasses with Siemens immunonephelometric assays. Clin Biochem (2010) 43:694–6. doi:10.1016/j.clinbiochem.2010.02.003
42. Cantsilieris S, Nelson BJ, Huddleston J, Baker C, Harshman L, Penewit K. Recurrent structural variation, clustered sites of selection, and disease risk for the complement factor H (CFH) gene family. Proc Natl Acad Sci U S A (2018) 115(19):E4433–42. doi:10.1073/pnas.1717600115
43. De Paula PF, Barbosa JE, Junior PR, Ferriani VPL, Latorre MRDO, Nudelman V, et al. Ontogeny of complement regulatory proteins – concentrations of factor H, factor I, C4b-binding protein, properdin and vitronectin in healthy children of different ages and in adults. Scand J Immunol (2003) 58:572–7. doi:10.1046/j.1365-3083.2003.01326.x
44. Sonntag J, Brandenburg U, Polzehl D, Strauss E, Vogel M, Dudenhausen JW, et al. Complement system in healthy term newborns: reference values in umbilical cord blood. Pediatr Dev Pathol (1998) 1:131–5. doi:10.1007/s100249900016
45. Davis CA, Vallota EH, Forristal J. Serum complement levels in infancy: age related changes. Pediatr Res (1979) 13:1043–6. doi:10.1203/00006450-197909000-00019
Keywords: normal ranges, complement, complement factor H, factor H-related proteins, pediatrics, diagnostics, reference intervals
Citation: van Beek AE, Kamp A, Kruithof S, Nieuwenhuys EJ, Wouters D, Jongerius I, Rispens T, Kuijpers TW and Gelderman KA (2018) Reference Intervals of Factor H and Factor H-Related Proteins in Healthy Children. Front. Immunol. 9:1727. doi: 10.3389/fimmu.2018.01727
Received: 28 May 2018; Accepted: 12 July 2018;
Published: 02 August 2018
Edited by:
Thomas Vorup-Jensen, Aarhus University, DenmarkReviewed by:
Michael Kirschfink, Universität Heidelberg, GermanyGunnar Houen, State Serum Institute (SSI), Denmark
Copyright: © 2018 van Beek, Kamp, Kruithof, Nieuwenhuys, Wouters, Jongerius, Rispens, Kuijpers and Gelderman. This is an open-access article distributed under the terms of the Creative Commons Attribution License (CC BY). The use, distribution or reproduction in other forums is permitted, provided the original author(s) and the copyright owner(s) are credited and that the original publication in this journal is cited, in accordance with accepted academic practice. No use, distribution or reproduction is permitted which does not comply with these terms.
*Correspondence: Kyra A. Gelderman, ay5nZWxkZXJtYW5Ac2FucXVpbi5ubA==
†Present address: Diana Wouters, Centre for Infectious Disease Control, National Institute for Public Health and the Environment, Bilthoven, Netherlands