- 1Department of Parasitology, Zhongshan School of Medicine, Sun Yat-sen University, Guangzhou, China
- 2Key Laboratory of Tropical Disease Control of Ministry of Education, Sun Yat-sen University, Guangzhou, China
- 3School of Stomatology, Jinan University, Guangzhou, China
Toxoplasmic encephalitis (TE), an opportunistic infection, is a severe health problem in immunocompromised patients. Previous studies have revealed that C57BL/6 mice are susceptible and BALB/c mice are resistant to TE. To investigate the mechanisms involved in the immunopathogenesis of TE in susceptible C57BL/6 and resistant BALB/c mice, both strains of mice were perorally infected with the Prugniuad (Pru) strain of Toxoplasma gondii. Our results showed that compared with BALB/c mice, C57BL/6 mice infected with T. gondii Pru strain had more severe brain histopathological damage, and higher mRNA expression levels of tachyzoite-specific surface antigen 1, bradyzoite-specific antigen 1, interferon gamma (IFNγ), interleukin (IL)-10, arginase1 (Arg1) (M2 marker), galectin (Gal)-3, Gal-9, T. gondii microneme protein 1 (TgMIC1), TgMIC4, and TgMIC6 during the course of infection by using quantitative real-time reverse transcription-polymerase chain reaction. Further analysis displayed that BALB/c mice showed higher numbers of microglial cells and higher levels of IL-1β, inducible nitric oxide synthase (iNOS) (M1 marker), and chitinase-3-like protein 3 (Ym1) (M2 marker) in the early infective stage [at day 14 or 35 post infection (p.i.)] compared with C57BL/6 mice, whereas C57BL/6 mice showed higher numbers of microglial cells and higher levels of IL-10, iNOS (M1 marker), and Ym1 (M2 marker) at days 35, 50, or 70 p.i. compared with BALB/c mice. Correlation analysis showed that significant positive correlations existed between Gal-3 and IL-4/IL-10/iNOS/Ym1 and between Gal-9 and IL-4/Ym1 in C57BL/6 mice; between Gal-3 and IFNγ/Arg1 and between Gal-9 and IFNγ/Arg1 in BALB/c mice. Together, our data demonstrated that different Gal-3 and Gal-9 expressions as well as different positive correlations were found between Gal-3 and T helper 1 (Th1)/Th2/M1/M2 cytokines or between Gal-9 and Th1/Th2/M2 cytokines in the brains of T. gondii Pru strain-infected C57BL/6 and BALB/c mice.
Introduction
Toxoplasma gondii, a pathogen of medical and veterinary importance, is an obligate intracellular protozoan parasite that has a global distribution and can infect almost any warm-blooded vertebrate (1). T. gondii infection in the immunocompetent individual is effectively controlled by a vigorous immune response (2); however, the infection can cause toxoplasmic encephalitis (TE), a life-threatening disease in immunocompromised patients (3). Although all mice lineages develop a strong T helper 1 (Th1) immune response to T. gondii infection (4), the immune response to the parasite infection in the brains can be drastically different between genetically resistant mice (e.g., BALB/c mice) and that of susceptible mice (e.g., C57BL/6 mice) (5). During the late stage of infection, resistant mouse strain establishes a latent chronic infection, while susceptible strain spontaneously develops necrotizing TE (6). So far, the mechanisms behind the differences between the two strains of mice during the development of TE are not fully understood.
It has been proposed that T. gondii utilizes innate immune cells such as macrophages to migrate to immunoprivileged sites such as the central nervous system (CNS) to establish chronic infection (7). Macrophages are generally categorized into two distinct subsets as either classically activated (M1) or alternatively activated (M2). M1 type macrophages, characterized by CD86 expression, can release high levels of pro-inflammatory markers such as monocyte chemotactic protein-1β, inducible nitric oxide synthase (iNOS), interleukin (IL)-6, and tumor necrosis factor alpha (TNFα) (8). M2 macrophages can produce a large amount of IL-10, chitinase-3-like protein 3 (Ym1), macrophage and granulocyte inducer-form 1, and arginase1 (Arg1) and play important roles in the protection of the host by decreasing inflammation and promoting tissue repair (9, 10). During T. gondii infection, Th1 cells produce cytokines such as interferon gamma (IFNγ) to activate macrophages and cytotoxic T lymphocytes, while Th2 cells secrete cytokines such as IL-4 to induce humoral type immune responses (11, 12). IFNγ-activated microglial cells significantly upregulate iNOS and produce nitric oxide (NO), which can inhibit intracellular T. gondii replication (13).
Galectins belong to the family of β-galactoside-binding lectins, which are known to regulate a number of pathways that involve in apoptosis (14), immune tolerance, inflammation (15), and cell adhesion (16). Currently, 15 members of the galectin family have been identified in mammals; some members are widely distributed in different cells and tissue types, while others are more selectively expressed (17). The major galectins expressed in the CNS are galectin (Gal)-1, Gal-3, Gal-4, Gal-8, and Gal-9 (18). Under normal physiological conditions, galectins maintain CNS homeostasis, while in neuronal diseases and experimental neuroinflammatory disease models, galectins may serve as extracellular mediators or intracellular regulators in controlling the inflammatory response or conferring the remodeling capacity in damaged CNS tissues (18). So far, the roles of galectins in TE remains poorly understood.
Apicomplexan parasites such as T. gondii and Plasmodium spp. utilize apical complex organelles consisting of dense granules, rhoptries, and micronemes to deploy for the release (egress), attachment, and invasion of host cells, as well as the establishment of the parasitophorous vacuole (19). T. gondii microneme proteins (TgMICs) are secreted by micronemes upon contact with host cells and play important roles in T. gondii motility, invasion, intracellular survival, and egress from host cells (20). TgMIC6 and TgMIC8 genes are expressed in the rapidly dividing tachyzoites, whereas TgMIC7 and TgMIC9 genes are predominantly expressed in the slowly dividing encysted bradyzoites (21). TgMIC1 and TgMIC4 can bind to host cells, while TgMIC6 serves as an escorter for two soluble adhesins TgMIC1 and TgMIC4 and along with adhesins can establish a molecular bridge between the host and parasites (22). So far, limited data are available about the role of TgMICs in the immune response to T. gondii infection.
Based on the relationship between galectins and brain diseases, this study was designed to compare the expressions of galectins, microglial activation markers (M1 and M2 phenotypes), TgMICs, and Th1 and Th2 cytokines between C57BL/6 and BALB/c mice infected with T. gondii Pru strain. We found that significant positive correlations existed between Gal-3 and Th1/Th2/M1/M2 cytokines as well as between Gal-9 and Th1/Th2/M2 cytokines in C57BL/6 or BALB/c mice after T. gondii Pru strain infection.
Materials and Methods
Mice, Parasites, and Experimental Infections
This experimental study and all administrations were reviewed and approved by the Ethical Committee of Animal Experiments at Sun Yat-sen University.
Female 6- to 8-week-old C57BL/6 and BALB/c mice were purchased from the Experimental Animal Center at Sun Yat-sen University (Guangzhou, China), and 20 mice were used per each group. All animals were housed under specific-pathogen-free conditions in the animal facility at Sun Yat-sen University. Mice were infected via oral route with eight cysts of T. gondii Pru strain prepared from the brain of chronically infected mice. To establish a chronic infection by controlling the proliferation of tachyzoites during acute stage, mice were treated with sulfadiazine (Sigma-Aldrich, Shanghai, China) in the drinking water as described previously (23).
Histopathology
Mice infected with T. gondii Pru strain were euthanatized by CO2 asphyxiation at 14, 35, 50, and 70 days post infection (p.i.), and their brains were harvested and immediately fixed in 10% buffered natural formaldehyde (Guangzhou Chemical Reagent Factory, China) for over 48 h. The paraffin-embedded tissues from each mouse were sectioned at 5 µm and prepared for hematoxylin and eosin (Sigma-Aldrich, Shanghai, China) staining. The histopathological changes of brains from each group were determined under 200× magnification in three noncontiguous sections from four mice, and histopathological scores were given based on previously described criteria (23) with some modifications. In brief, the histological changes were scored semi-quantitatively as 1, 2, 3, and 4 (e.g., normal, mild inflammation, moderate inflammation and necrosis, and severe inflammation and necrosis, respectively).
Immunohistochemical Staining
The paraffin-embedded brain sections (6-µm) were deparaffinized and rehydrated in distilled water. Heat-induced antigen retrieval was carried out in an 800-W microwave oven for 30 min. Sections were treated with 3% hydrogen peroxide in methanol for 10 min at 37°C, and then incubated in 10% normal goat serum with 1% bovine serum albumin (Sigma-Aldrich, Shanghai, China) in PBS (pH 7.4) for 10 min at room temperature to block nonspecific binding. After washing with PBS, sections were incubated with rabbit anti-Iba1 (1:200 dilutions) (Wako Pure Chemical Industries, Osaka, Japan), rabbit anti-Gal-9 (1:200 dilutions) (Boster Biological Technology, Wuhan, China), and mouse anti-Gal-3 (1:200 dilutions) (R&D Systems, Minneapolis, MN, USA) overnight at 4°C. Those sections incubated with secondary antibodies alone were used as isotype controls. Immunohistochemical staining was then performed with a streptavidin–biotin–peroxidase complex kit and developed with diaminobenzidine tetrahydrochloride (Zhongshan Golden Bridge Technology, Beijing, China). The sections were counterstained with hematoxylin and positive cells were identified by dark-brown staining under light microscopy.
Morphometric Analysis
Serial sections from the brains were immunostained with anti-Iba1. A total of three mice were analyzed in each time point, and four sections per animal were selected for counting of positive cells. In every brain section, the microglial cells expressing Iba1 markers were captured with a digital microscopy under 400× magnification and the numbers of Iba1-positive cells in the brains (0.015066 mm2 tissue section) were determined by Image-Pro Plus (Image Z1 software, Media Cybernetics, MD, USA), and the density of positive cells was expressed as the number of cells per square millimeter.
Selection of Galectins
Gal-1, Gal-3, Gal-7, Gal-8, and Gal-9 are known to be relevant to brain diseases (18). Therefore, in this study, the specific expression pattern of these five galectins was examined.
Determination of mRNA Expression Using Quantitative Real-Time Reverse Transcription-Polymerase Chain Reaction (qRT-PCR)
Total RNA was extracted from about 100 mg of mouse brain tissues from each group using a RNA Extraction Kit (TaKaRa, Shiga, Japan) as per the manufacturer’s protocol. RNA amount was determined by measuring the ratio of absorbance at 260 and 280 nm using a NanoDrop ND-1000 spectrophotometer (NanoDrop Technologies). First-strand cDNA was constructed from 1.0 µg of total RNA with oligo (dT) as primers using a PrimeScript 1st Strand cDNA Synthesis Kit (TaKaRa, Shiga, Japan). To determine tissue mRNA levels of cytokines (IL-1β, IFNγ, IL-4, IL-10, iNOS, Arg1, and Ym-1), galectins (Gal-1, Gal-3, Gal-4, Gal-8, and Gal-9), TgMICs (TgMIC1, TgMIC4, TgMIC6, TgMIC3, and TgMIC8), β-actin, actin of the ME49 strain of T. gondii, T. gondii tachyzoite-specific surface antigen 1 (SAG1), and T. gondii bradyzoite-specific antigen 1 (BAG1), qRT-PCR measurements were performed using SYBR Green QPCR Master Mix (TaKaRa, Shiga, Japan). Primers are listed in Table 1. Briefly, a total of 10 µl reaction mixture contained 5.0 µl of SYBR® Premix Ex TaqTM (2×), 0.5 µl of each primer (10 pM), 3.0 µl of dH2O, and 1.0 µl of cDNA (0.2 µg/µl). Amplification was pre-denaturized for 30 s at 95°C, followed by 43 cycles of 5 s at 95°C and 20 s at 60°C with a LightCycler® 480 instrument (Roche Diagnostics, USA). The mRNA expression levels of cytokines, SAG1, and BAG1 were normalized to that of mouse housekeeping gene, β-actin, and the mRNA levels of TgMICs were normalized to that of T. gondii housekeeping gene (actin of T. gondii ME49 strain). The results were expressed as fold change compared with uninfected controls.
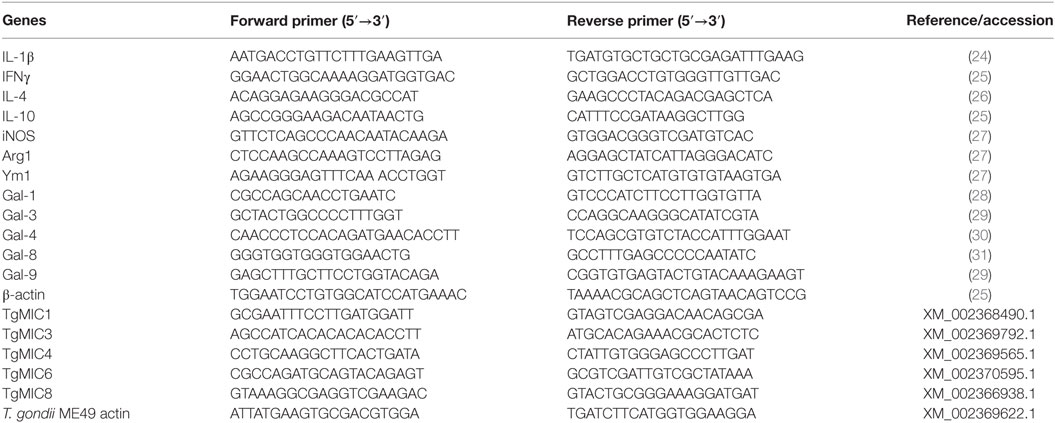
Table 1. Primer sequences of genes used for quantitative real-time reverse transcription-polymerase chain reaction assays.
Statistical Analysis
Results of experimental studies were reported as mean ± SD. Statistical analysis of the data was performed by the Wilcoxon rank sum test and one-way ANOVA followed by Bonferroni’s multiple comparison tests using SPSS software for windows (version 19.0; SPSS, Inc., IL, USA). Pearson’s correlation coefficient was used to analyze correlations between the levels of cytokines and galectins. All graphs were performed using GraphPad Prism software (version 5.0). A value of P < 0.01 was considered significant for correlation analysis, while a value of P < 0.05 was considered significant for other statistical analysis.
Results
Comparison of Histopathology and Parasite Burdens in the Brains of T. gondii-Susceptible C57BL/6 and T. gondii-Resistant BALB/c Mice
Histological observation showed that control sections of the brains from uninfected C57BL/6 and BALB/c mice had no obvious inflammations or structural abnormalities. The brains of T. gondii Pru strain-infected C57BL/6 mice showed moderate-to-severe inflammation, diffuse inflammatory cellular infiltration, necrotic focus, and tissue structural damages at days 14, 35, 50, and 70 p.i., while the brains of infected BALB/c mice showed limited infiltration of inflammatory cells at the aforementioned times (Figure 1A). Semi-quantitative analysis of the severity of inflammation and necrosis in the brain sections of the two strains of mice were performed. Compared with uninfected controls, the pathological severity scores of brains were significantly increased in both C57BL/6 and BALB/c mice at days 14, 35, 50, and 70 p.i. Compared with BALB/c mice, the histopathological scores in the brains of C57BL/6 mice were significantly higher at days 35 (P < 0.05), 50 (P < 0.01), and 70 (P < 0.01) p.i. (Figure 1B).
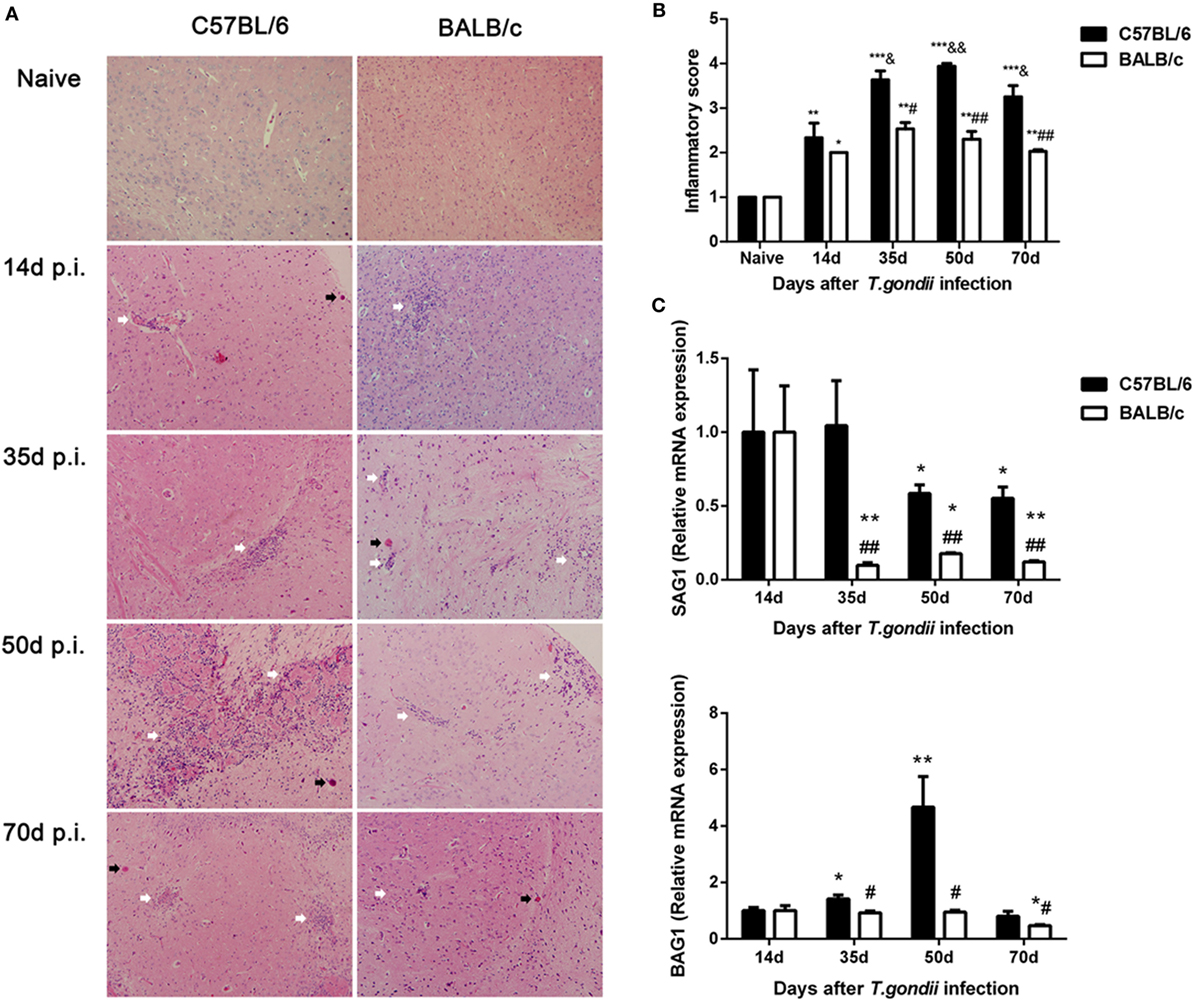
Figure 1. Histopathological changes and parasite burdens in the brains of Toxoplasma gondii Pru strain-infected C57BL/6 and BALB/c mice. (A) Histopathological changes in the brains at days 14, 35, 50, and 70 post infection (p.i.). Cysts were indicated with black arrow heads and inflammatory cell infiltrates were indicated with white arrow heads. Original magnification 200×; hematoxylin and eosin stain. (B) Histopathological score analysis at days 14, 35, 50, and 70 p.i. Data are represented as mean ± SEM. Significant differences between groups are analyzed by the Wilcoxon rank sum test. *P < 0.05, **P < 0.01, and ***P < 0.001 vs naive; &P < 0.05 and &&P < 0.01 vs 14 days p.i.; #P < 0.05 and ##P < 0.01 vs C57BL/6 mice. (C) Parasite burdens in the brains at days 14, 35, 50, and 70 p.i. Relative mRNA expressions of surface antigen 1 (SAG1) and bradyzoite-specific antigen 1 (BAG1) were detected by using quantitative real-time reverse transcription-polymerase chain reaction. Transcript level at day 14 p.i. was taken as 1. Values are means from triplicate measurements, and data are presented as mean ± SD. *P < 0.05 and **P < 0.01 vs naive; #P < 0.05 and ##P < 0.01 vs C57BL/6 mice. There were four mice per group. The data shown are representative of those from two different experiments.
Stage conversion between tachyzoite and bradyzoite forms is associated with stage specific antigen expression. In this study, the mRNA expression levels of tachyzoite-specific SAG1 and bradyzoite-specific BAG1 in the brains of C57BL/6 and BALB/c mice infected with T. gondii Pru strain were detected by using qRT-PCR and the transcript. Levels of SAG1 and BAG1 were relative to day 14 p.i. (e.g., the relative transcript level at day 14 p.i. = 1.0). Compared with day 14 p.i., the SAG1 levels in the brains of both C57BL/6 and BALB/c mice were significantly decreased at days 35, 50, and 70 p.i. The BAG1 levels in the brains of C57BL/6 mice were significantly elevated at days 35 and 70 p.i., while the BAG1 level in BALB/c mice was significantly reduced at day 70 p.i. Compared with BALB/c mice, both SAG1 and BAG1 levels were significantly higher in the brains of C57BL/6 mice at days 35, 50, and 70 p.i. (P < 0.01 and P < 0.05, respectively) (Figure 1C).
Comparison of Microglial Cells in the Brains of T. gondii-Susceptible C57BL/6 and T. gondii-Resistant BALB/c Mice
A few Iba1-positive microglial cells were observed in the sections of brains of uninfected C57BL/6 and BALB/c mice. However, a large number of activated microglial cells were observed in the brains of both T. gondii Pru strain-infected C57BL/6 and BALB/c mice; the majority of activated microglial cells were ameboid shape with thickened and retracted branches (Figure 2A). Quantitative analysis of Iba1 staining showed that, compared with uninfected controls, the numbers of Iba1-positive microglial cells in the brains of both C57BL/6 and BALB/c mice were significantly increased at days 14, 35, 50, and 70 p.i. However, compared with BALB/c mice, the microglial cell numbers in the brains of C57BL/6 mice were significantly higher at days 35 (P < 0.001), 50 (P < 0.001), and 70 (P < 0.01) p.i. (Figure 2B).
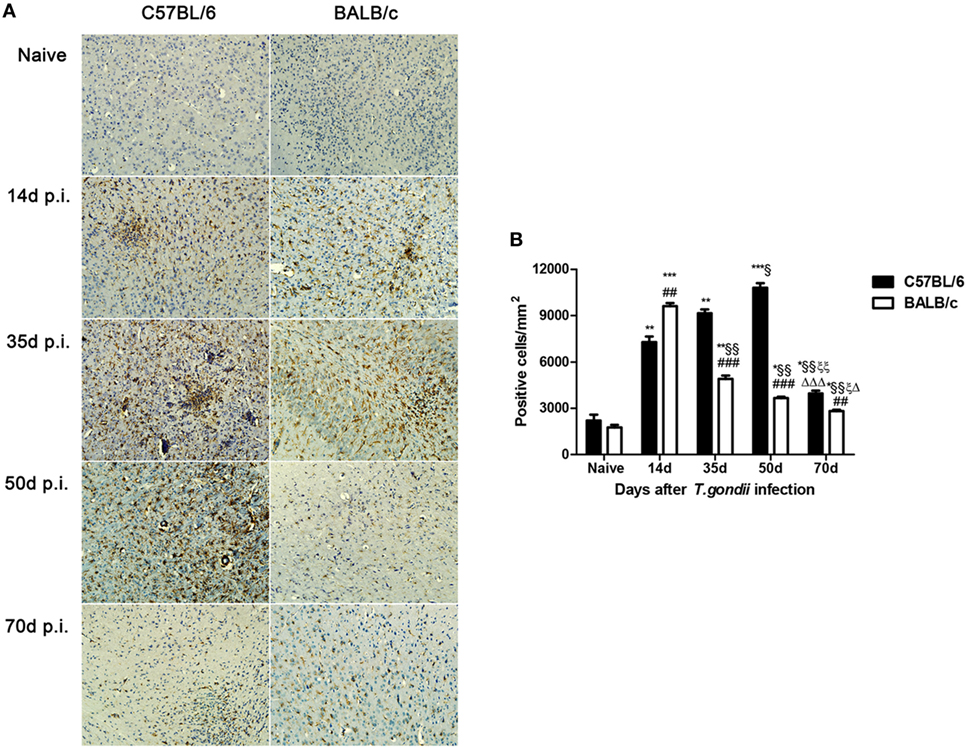
Figure 2. Expression of activated microglial marker Iba1 in the brains of Toxoplasma gondii Pru strain-infected C57BL/6 and BALB/c mice. (A) Immunohistochemistry for Iba1 in the brains of uninfected mice, and mice infected with T. gondii Pru strain at days 14, 35, 50, and 70 post infection (p.i.). Original magnification 200×. (B) Quantitative analysis of Iba1-positive microglia. The density of positive cells was expressed as the number of cells per square millimeter. Data are presented as means ± SD; experiments were performed with three mice per group. *P < 0.05, **P < 0.01, and ***P < 0.001 vs Naive; §P < 0.05 and §§P < 0.01 vs 14 days p.i.; ξP < 0.05 and ξξP < 0.01 vs 35 days p.i.; ΔP < 0.05 and ΔΔΔP < 0.001 vs 50 days p.i.; ##P < 0.01 and ###P < 0.001 vs C57BL/6 mice.
Comparison of mRNA Levels of M1/M2 Markers in the Brains of T. gondii-Susceptible C57BL/6 and T. gondii-Resistant BALB/c Mice
The mRNA levels of M1 marker (iNOS) and M2 marker (Arg1 and Ym1) in the brains of T. gondii Pru strain-infected C57BL/6 and BALB/c mice were examined. Compared with uninfected controls, iNOS levels were significantly increased in the brains of both C57BL/6 and BALB/c mice at days 14, 35, 50, and 70 p.i.; Arg1 and Ym1 levels were significantly increased in C57BL/6 mice at days 14, 35, 50, and 70 p.i., and significantly increased in BALB/c mice at days 14 and 35 p.i. (Figure 3A). Compared with BALB/c mice, there were significantly lower iNOS levels at days 14 and 35 p.i. (P < 0.01); while there were significantly higher iNOS levels at days 50 and 70 p.i. (P < 0.001), significantly higher Arg1 levels at days 14 (P < 0.05), 35 (P < 0.01), 50 (P < 0.001), and 70 (P < 0.01) p.i., and significantly lower Ym1 level at day 14 p.i. (P < 0.001) and significantly higher Ym1 levels at days 35 (P < 0.01), 50 (P < 0.001), and 70 (P < 0.001) p.i. in the brains of C57BL/6 mice (Figure 3B).
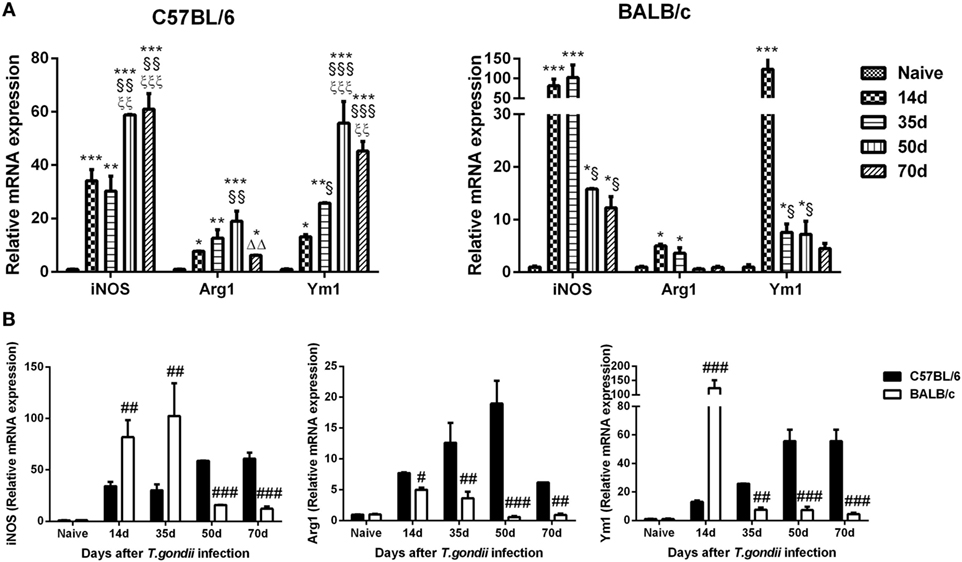
Figure 3. Relative mRNA expressions of inducible nitric oxide synthase (iNOS), arginase1 (Arg1), and Ym1 in the brain tissues of Toxoplasma gondii Pru strain-infected C57BL/6 and BALB/c mice were detected by using quantitative real-time reverse transcription-polymerase chain reaction. (A) iNOS, Arg1, and Ym1 expressions in the brains of C57BL/6 and BALB/c mice. (B) Comparison of iNOS, Arg1, and Ym1 levels in the brains of C57BL/6 and BALB/c mice. Values are means from triplicate measurements, and data are presented as mean ± SD. There were four mice per group. The data shown are representative of those from two different experiments. *P < 0.05, **P < 0.01, and ***P < 0.001 vs naive; §P < 0.05; §§P < 0.01, and §§§P < 0.001 vs 14 days post infection (p.i.); ξξP < 0.01 and ξξξP < 0.001 vs 35 days p.i.; ΔΔP < 0.01 vs 50 days p.i.; #P < 0.05, ##P < 0.01, and ###P < 0.001 vs C57BL/6 mice.
Comparison of mRNA Levels of Th1/Th2 Cytokines in the Brains of T. gondii-Susceptible C57BL/6 and T. gondii-Resistant BALB/c Mice
Compared with uninfected controls, the levels of IL-1β, IFNγ, and IL-10 were significantly increased in the brains of both C57BL/6 and BALB/c mice at days 14, 35, 50, and 70 p.i.; IL-4 levels were significantly increased in both T. gondii Pru strain-infected C57BL/6 and BALB/c mice at days 35, 50, and 70 p.i. (Figure 4A). Compared with BALB/c mice, significantly lower IL-1β level at day 35 p.i. (P < 0.05), significantly higher IL-10 level at day 50 p.i. (P < 0.01), and significantly higher IFNγ levels at days 14 (P < 0.05), 35 (P < 0.05), 50 (P < 0.01), and 70 (P < 0.01) p.i. were detected in the brains of C57BL/6 mice (Figure 4B).
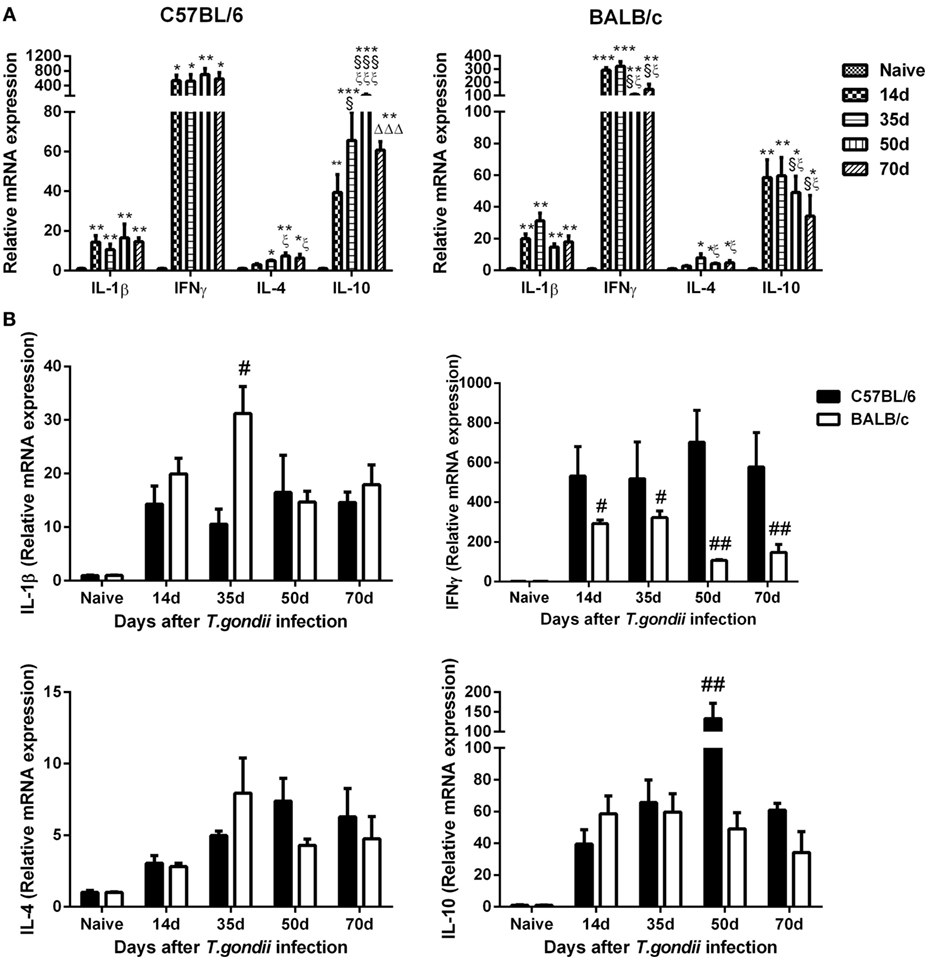
Figure 4. Relative mRNA expressions of interleukin (IL)-1β, interferon gamma (IFNγ), IL-4, and IL-10 in the brain tissues of Toxoplasma gondii Pru strain-infected C57BL/6 and BALB/c mice were detected by using quantitative real-time reverse transcription-polymerase chain reaction. (A) IL-1β, IFNγ, IL-4, and IL-10 expressions in the brains of C57BL/6 and BALB/c mice. (B) Comparison of IL-1β, IFNγ, IL-4, and IL-10 levels in the brains of C57BL/6 and BALB/c mice. Values are means from triplicate measurements, and data are presented as mean ± SD. There were four mice per group. The data shown are representative of those from two different experiments. *P < 0.05, **P < 0.01, and ***P < 0.001 vs naive; §P < 0.05 and §§§P < 0.001 vs 14 days post infection (p.i.); ξP < 0.05 and ξξξP < 0.001 vs 35 days p.i.; ΔΔΔP < 0.001 vs 50 days p.i.; #P < 0.05 and ##P < 0.01 vs C57BL/6 mice.
Comparison of mRNA Levels of Galectins in the Brains of T. gondii-Susceptible C57BL/6 and T. gondii-Resistant BALB/c Mice
Compared with uninfected controls, Gal-3 expression levels were significantly increased in the brains of both T. gondii Pru strain-infected C57BL/6 and BALB/c mice at days 14, 35, 50, and 70 p.i.; Gal-9 levels were significantly increased in both C57BL/6 and BALB/c mice at days 14, 35, and 50 p.i., and significantly increased in C57BL/6 mice at day 70 p.i. (Figure 5A). Compared with BALB/c mice, there were significantly higher levels of Gal-3 and Gal-9 in the brains of C57BL/6 mice at days 35 (P < 0.05), 50 (P < 0.01 and P < 0.05, respectively), and 70 (P < 0.01) p.i. (Figure 5B).
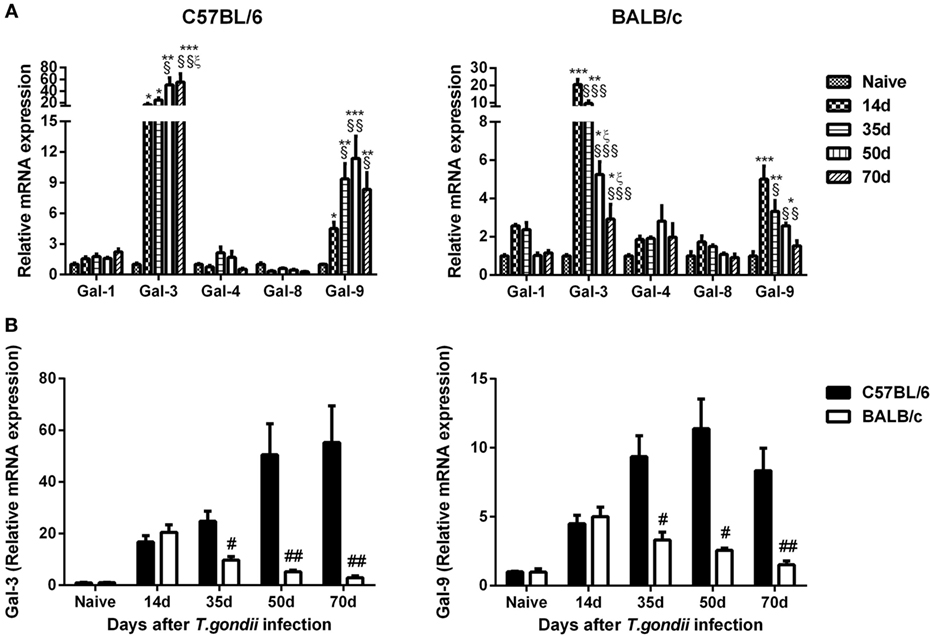
Figure 5. Relative mRNA expressions of Gal-1, Gal-3, Gal-4, Gal-8, and Gal-9 in the brain tissues of Toxoplasma gondii Pru strain-infected C57BL/6 and BALB/c mice were detected by using quantitative real-time reverse transcription-polymerase chain reaction. (A) Galectin expressions in the brains of C57BL/6 and BALB/c mice. (B) Comparison of Gal-3 and Gal-9 levels in the brains of C57BL/6 and BALB/c mice. Values are means from triplicate measurements, and data are presented as mean ± SD. There were four mice per group. The data shown are representative of those from two different experiments. *P < 0.05, **P < 0.01, and ***P < 0.001 vs naive; §P < 0.05, §§P < 0.01, and §§§P < 0.001 vs 14 days post infection (p.i.); ξP < 0.05 vs 35 days p.i.; #P < 0.05 and ##P < 0.01 vs C57BL/6 mice.
Comparison of mRNA Levels of TgMICs in the Brains of T. gondii-Susceptible C57BL/6 and T. gondii-Resistant BALB/c Mice
Compared with day 14 p.i., TgMIC1 levels were significantly decreased in the brains of both T. gondii Pru strain-infected C57BL/6 and BALB/c mice at days 35, 50, and 70 p.i.; TgMIC3 levels were significantly increased in C57BL/6 mice at days 35, 50, and 70 p.i.; TgMIC4 levels were significantly increased in C57BL/6 mice at days 50 and 70 p.i. and significantly increased in BALB/c mice at day 70 p.i. TgMIC6 levels were significantly decreased in BALB/c mice at days 35, 50, and 70 p.i., and TgMIC8 levels were significantly decreased in both C57BL/6 and BALB/c mice at days 35, 50, and 70 p.i. (Figure 6A). Compared with BALB/c mice, there were significantly higher levels of TgMIC1 at days 35 and 70 p.i. (P < 0.05) and significantly higher levels of TgMIC4 and TgMIC6 at days 35, 50, and 70 p.i. in the brains of C57BL/6 mice (P < 0.05) (Figure 6B).
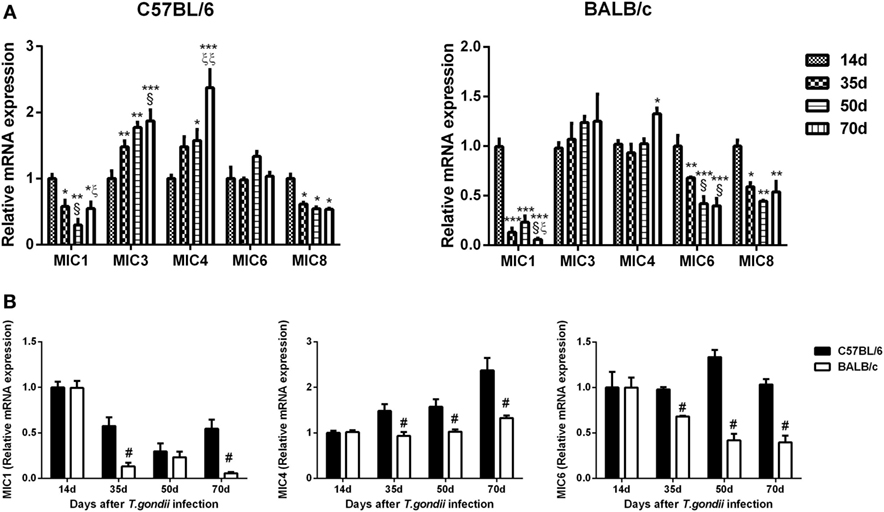
Figure 6. Relative mRNA expressions of TgMIC1, TgMIC4, TgMIC6, TgMIC3, and TgMIC8 in the brain tissues of Toxoplasma gondii Pru strain-infected C57BL/6 and BALB/c mice were detected by using quantitative real-time reverse transcription-polymerase chain reaction. (A) TgMICs expressions in the brains of C57BL/6 and BALB/c mice. (B) Comparison of TgMIC1, TgMIC4, and TgMIC6 levels in the brains of C57BL/6 and BALB/c mice. Transcript level at day 14 post infection (p.i.) was taken as 1. Values are means from triplicate measurements, and data are presented as mean ± SD. There were four mice per group, and data are representative of those from two different experiments. *P < 0.05, **P < 0.01, and ***P < 0.001 vs 14 days p.i.; §P < 0.05 vs 35 days p.i.; ξP < 0.05 and ξξP < 0.05 vs 50 days p.i.; #P < 0.05 vs C57BL/6 mice.
Correlations Between Gal-3/Ga-9 and Th1/Th2/M1/M2 Cytokines in the Brains of T. gondii-Resistant BALB/c and T. gondii-Susceptible C57BL/6 Mice
The correlations between mRNA levels of Gal-3/Gal-9 and Th1/Th2/M1/M2 in the brains of T. gondii Pru strain-infected C57BL/6 and BALB/c mice were evaluated, herein only significant correlations were shown. There were significant correlations between the mRNA levels of Gal-3 and IL-4 (r = 0.8424, P = 0.0002), Gal-3 and IL-10 (r = 0.6996, P = 0.0037), Gal-3 and iNOS (r = 0.7344, P = 0.0018), Gal-3 and Ym1 (r = 0.6866, P = 0.0047), Gal-9 and IL-4 (r = 0.8293, P = 0.0002), and Gal-9 and Ym1 (r = 0.6714, P = 0.0061) in T. gondii Pru strain-infected C57BL/6 mice (Figure 7A). However, there were significant correlations between the mRNA levels of Gal-3 and IFNγ (r = 0.6993, P = 0.0078), Gal-3 and Arg1 (r = 0.8099, P = 0.0004), Gal-9 and IFNγ (r = 0.7378, P = 0.0040), and Gal-9 and Arg1 (r = 0.7963, P = 0.0007) in T. gondii Pru strain-infected BALB/c mice (Figure 7B). Taken together, in C57BL/6 mice, significant positive correlations existed between Gal-3 and IL-4/IL-10/iNOS/Ym1 as well as between Gal-9 and IL-4/Ym1; whereas in BALB/c mice, significant positive correlations existed between Gal-3 and IFNγ/Arg1 as well as between Gal-9 and IFNγ/Arg1.
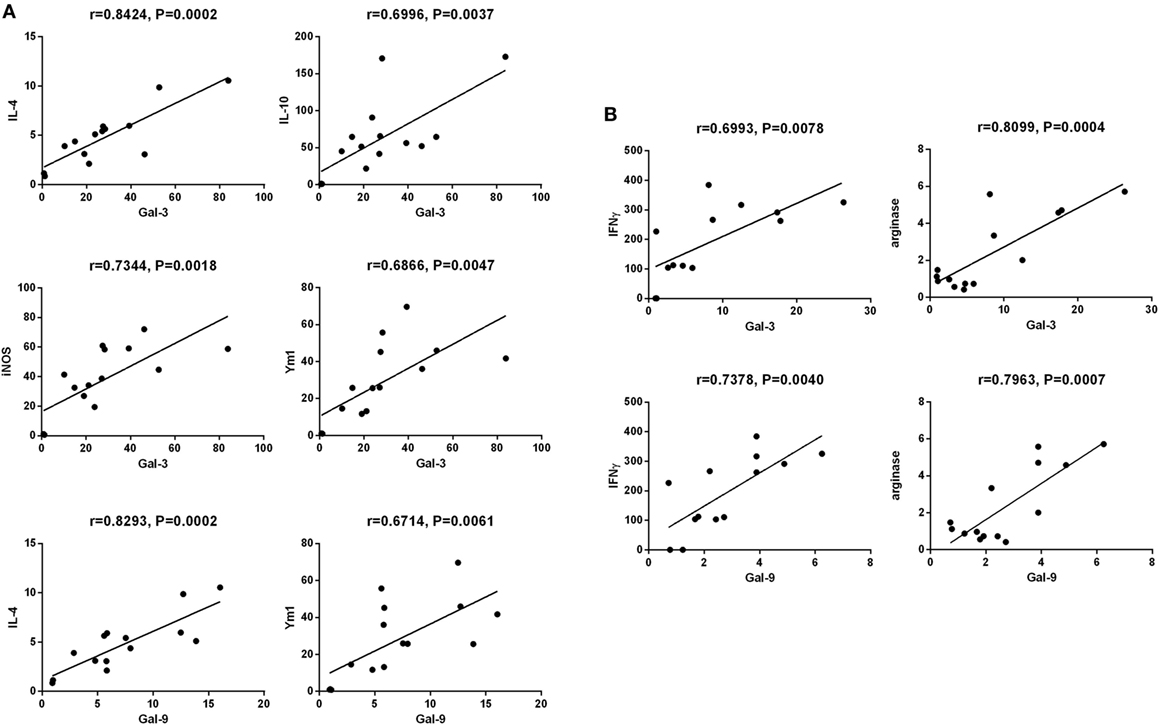
Figure 7. Correlation analysis between Gal-3 and T helper 1 (Th1)/Th2/M1/M2 cytokines as well as between Gal-9 and Th1/Th2/M2 cytokines in the brain tissues of Toxoplasma gondii Pru strain-infected C57BL/6 and BALB/c mice (n = 16). (A) Significant correlations between Gal-3 and IL-4/IL-10/iNOS/Ym1 as well as between Gal-9 and IL-4/Ym1 existed in the brains of T. gondii-infected C57BL/6 mice. (B) Significant correlations between Gal-3 and IFNγ/Arg1 as well as between Gal-9 and IFNγ/Arg1 existed in the brains of T. gondii-infected BALB/c mice. The r value generates for the theoretical line of best fit, and the P value indicates the significance of the correlation.
Discussion
When T. gondii parasites infect the host, the cysts can exist predominantly in the brain tissue for lifetime, and an immunocompetent host will establish a strong and persistent Th1-biased cell-mediated immunity to resist cyst reactivation and the consequences of TE (4). However, there is a remarkable difference in susceptibility to the infection of T. gondii among inbred strains of mice. After peroral infection with T. gondii ME49 strain, C57BL/6 mice all died whereas BALB/c mice all survived (32). So far, the immune responses differing between TE-resistant and TE-susceptible hosts are not fully understood. In this study, genetically susceptible C57BL/6 and resistant BALB/c mice were perorally infected with T. gondii Pru strain, and significantly more severe histopathological damage (inflammation and necrosis) were found in the brains of C57BL/6 mice in comparison of those of BALB/c mice at all the times during the observations (e.g., at days 14, 35, 50, and 70 p.i.). The levels of mRNA transcripts of both tachyzoite-specific SAG1 and bradyzoite-specific BAG1 genes were significantly higher in the brains of C57BL/6 mice than those of BALB/c mice at days 35, 50, and 70 p.i. It has been reported that following T. gondii ME49 strain infection, C57BL/6 mice showed an intense and progressive inflammatory alteration in the CNS, while BALB/c mice showed slight inflammatory reaction in the CNS (33). After infection with low virulent T. gondii DX strain, C57BL/6 mice presented higher tachyzoite and bradyzoite loads than those of BALB/c mice (34). Our data were in accordance with the previous studies.
Microglia activation is recognized as the hallmark of neuroinflammation. Microglial cells are the primary source for inflammatory mediators. Resident microglial cells play a critical role in TE, producing essential pro- and anti-inflammatory cytokines such as IL-1β, IL-10, TNFα, IL-12, and IL-15 (35–37). In this study, we found that microglial cell numbers in the brains of C57BL/6 mice were significantly higher than those of BALB/c mice at days 35, 50, and 70 p.i.; however, the number of microglial cells was significantly higher in BALB/c mice than that of C57BL/6 mice at day 14 p.i. Our data suggest that resident microglia are activated earlier in BALB/c mice, which may be essential for control of the parasite in the early infective stage in T. gondii-resistant BALB/c mice; whereas increased microglial activation remains longer in C57BL/6 mice, which may be required for establishing chronic TE in T. gondii-susceptible C57BL/6 mice.
It has been reported that activated microglial cells range from the pro-inflammatory M1 phenotype to the alternative/M2 phenotype and play neuroprotective or neurodetrimental roles (38). Therefore, identifying microglia phenotypes is critical for understanding the role of microglia in the pathogenesis of TE. In this study, we found that alterations in M1 and M2 phenotypes differed between the two models. In T. gondii Pru strain-infected BALB/c mice, both M1 (iNOS) and M2 (Ym1) phenotypic markers were significantly increased in the early infective stage (at day 14 or 35 p.i.); while in C57BL/6 mice, both M1 (iNOS) and M2 (Ym1) phenotypic markers were significantly increased in the late infective stage (at days 50 and 70 p.i.) and M2 marker (Arg1) was significantly increased at all the times during the study. M1 macrophages are critical for host defense against intracellular pathogens and have roles in antitumor immunity and autoimmune inflammation, whereas M2 macrophages are protective against helminth parasites and are important regulators of the wound healing response, tissue homeostasis, and adiposity (39). Inhibition of iNOS exacerbates chronic TE in T. gondii-susceptible C57BL/6 mice but does not lead to reactivation of latent TE in T. gondii-resistant BALB/c mice (34). CBA/Ca mice are susceptible to the development of TE. An in vitro study demonstrated that microglia from CBA/Ca mice show decreased production of NO and decreased inhibition of T. gondii replication after stimulation with lipopolysaccharide or IFNγ plus TNFα compared with microglia from BALB/c mice (40). Our data demonstrated that both M1 (iNOS) and M2 (Ym1 and Arg1) responses may play a role during chronic TE in T. gondii-susceptible C57BL/6 mice.
Toxoplasma gondii infection induces Th1-biased immune response, which is critical for the prevention of reactivation of TE (41). In this study, although the mRNA levels of Th1-associated cytokines (IFNγ and IL-1β) and Th2-associated cytokines (IL-4 and IL-10) were increased in the brain tissues of both C57BL/6 and BALB/c mice infected with T. gondii Pru strain, susceptible C57BL/6 mice presented a dominant Th1 response characterized by high expression of IFNγ at all the times after infection, accompanied by stronger neuroinflammatory outcomes. Our data suggested that the delayed M1 and M2 microglial activation and increased IFNγ expression in C57BL/6 mice after T. gondii Pru strain infection may be a part of the reason that C57BL/6 mice are more susceptible than BALB/c mice during TE.
Galectins have recently been demonstrated to play vital roles in host–pathogen interaction (42). Galectins are important modulators participating in homeostasis of the CNS and neuroinflammation; the major galectins expressed in the CNS are Gal-1, Gal-3, Gal-4, Gal-8, and Gal-9 (18). In this study, we compared the dynamic gene expressions of Gal-1, Gal-3, Gal-4, Gal-8, and Gal-9 in the brains between C57BL/6 and BALB/c mice infected with T. gondii Pru strain, only Gal-3 and Gal-9 were highly expressed in the brains of both C57BL/6 and BALB/c mice. C57BL/6 mice presented significantly higher mRNA expressions of Gal-3 and Gal-9 than those of BALB/c mice at days 35, 50, and 70 p.i. Gal-3 and Gal-9 are known pro-inflammatory mediators and regulators of apoptosis (29). Gal-9 is produced by activated astrocytes (43), functions as an astrocyte–microglia communication signal and promotes cytokine production, such as TNF, from microglia (44). After infection with ME49 strain of T. gondii, gal3−/− mice exhibits a higher parasite burden, delayed inflammatory response in the CNS, and significantly higher concentrations of IL-12p40 and IFNγ in the sera compared with those of gal3+/+ mice (45). Gal-3 is required for resident microglia activation and proliferation in response to ischemic injury in a mouse model (46). Our data demonstrated that both Gal-3 and Gal-9 are important factors in TE-susceptible C57BL/6 and TE-resistant BALB/c mice infected with T. gondii Pru strain. In addition, the inflammatory response is more pronounced in the brains of C57BL/6 mice, which are corresponded well with the increased numbers of Iba1-positive resident microglia as well as increased Gal-3 and Gal-9 expressions in C57BL/6 mice. Furthermore, we evaluated the correlations between the gene expressions of Gal-3/Gal-9 and the levels of Th1 and Th2 cytokines, and M1- and M2-associated cytokines in the brains after T. gondii Pru strain infection. Positive correlations were found in the mRNA levels between Gal-3 and IL-4/IL-10/iNOS/Ym1 as well as between Gal-9 and IL-4/Ym1 in C57BL/6 mice; whereas positive correlations were found between Gal-3 and IFNγ/Arg1 as well as between Gal-9 and IFNγ/Arg1 in BALB/c mice. These data suggest that Gal-3 is related to Th2 and M1/M2 immune responses while Gal-9 is related to Th2 and M2 immune responses in T. gondii-infected C57BL/6 mice. Indeed, both Gal-3 and Gal-9 are related to Th1 and M2 immunity in BALB/c mice with chronic T. gondii infection. Our data suggested that Gal-3 and Gal-9 may involve in different immune responses to T. gondii Pru strain infection in the two lineages of mice.
Proteins secreted from apicomplexan MICs play important roles in the parasite adhesion and invasion of the host cells (47). MICs, which have been identified with lectin domains, support several key cellular processes including gliding motility, active cell invasion and migration through cells, biological barriers, and tissues (47). Our data showed that C57BL/6 mice expressed significantly higher levels of TgMIC1, TgMIC4, and TgMIC6 at days 35, 50, or 70 p.i. than those of BALB/c mice after T. gondii Pru strain infection. Therefore, TgMICs may be expressed differently in the two strains of mice with different genetic background. TgMIC1–4–6 complex contributes to host cell recognition and attachment via the action of TgMIC1 as well as contributes to the virulence of T. gondii in mice (48). Our data indicate that the different expression levels of TgMIC1, TgMIC4, and TgMIC6 in the two strains of mice may be associated with the different outcomes in T. gondii Pru strain-infected C57BL/6 and BALB/c mice.
In conclusion, this study has provided evidences that Gal-3 and Gal-9 may play a critical role in the regulation of M1, M2, Th1, and Th2 cytokines in the hosts with TE. Our data demonstrated that significant different mRNA expressions of Gal-3 and Gal-9 as well as microglial activation markers, cytokines, and TgMICs were found between C57BL/6 and BALB/c mice after T. gondii Pru strain infection. Whether these differences are related to the phenomenon that C57BL/6 mice are susceptible while BALB/c mice are resistant to the development of TE needs to be further investigated.
Ethics Statement
This study was carried out in accordance with the recommendations of the requirements of the Animal Ethics Committee at Sun Yat-sen University. The protocol was approved by the Animal Ethics Committee at Sun Yat-sen University.
Author Contributions
FL designed experiments, wrote, and edited the manuscript. JL conducted experiments, analyzed data, and wrote the manuscript draft. SH revised and edited the manuscript.
Conflict of Interest Statement
The authors declare that the research was conducted in the absence of any commercial or financial relationships that could be construed as a potential conflict of interest.
Funding
This work was supported by the Natural Science Foundation of China (no. 81471973) and the 2016 Medical Education Research Project of Chinese Medical Association Medical Education Branch and China Higher Education Society of Medical Education Professional Committee (2016B-KY013).
References
1. Dubey JP. The history of Toxoplasma gondii – the first 100 years. J Eukaryot Microbiol (2008) 55(6):467–75. doi:10.1111/j.1550-7408.2008.00345.x
2. Weiss LM, Dubey JP. Toxoplasmosis: a history of clinical observations. Int J Parasitol (2009) 39(8):895–901. doi:10.1016/j.ijpara.2009.02.004
3. Connolly MP, Goodwin E, Schey C, Zummo J. Toxoplasmic encephalitis relapse rates with pyrimethamine-based therapy: systematic review and meta-analysis. Pathog Glob Health (2017) 111(1):31–44. doi:10.1080/20477724.2016.1273597
4. Denkers EY, Gazzinelli RT. Regulation and function of T-cell-mediated immunity during Toxoplasma gondii infection. Clin Microbiol Rev (1998) 11(4):569–88.
5. Suzuki Y, Kang H, Parmley S, Lim S, Park D. Induction of tumor necrosis factor-alpha and inducible nitric oxide synthase fails to prevent toxoplasmic encephalitis in the absence of interferon-gamma in genetically resistant BALB/c mice. Microbes Infect (2000) 2(5):455–62. doi:10.1016/S1286-4579(00)00318-X
6. Suzuki Y. Immunopathogenesis of cerebral toxoplasmosis. J Infect Dis (2002) 186(Suppl 2):S234–40. doi:10.1086/344276
7. Da Gama LM, Ribeiro-Gomes FL, Guimaraes U Jr, Arnholdt AC. Reduction in adhesiveness to extracellular matrix components, modulation of adhesion molecules and in vivo migration of murine macrophages infected with Toxoplasma gondii. Microbes Infect (2004) 6(14):1287–96. doi:10.1016/j.micinf.2004.07.008
8. Sica A, Mantovani A. Macrophage plasticity and polarization: in vivo veritas. J Clin Invest (2012) 122(3):787–95. doi:10.1172/JCI59643
9. Galvan-Pena S, O’Neill LA. Metabolic reprograming in macrophage polarization. Front Immunol (2014) 5:420. doi:10.3389/fimmu.2014.00420
10. Martinez FO, Helming L, Gordon S. Alternative activation of macrophages: an immunologic functional perspective. Annu Rev Immunol (2009) 27:451–83. doi:10.1146/annurev.immunol.021908.132532
11. Constant SL, Bottomly K. Induction of Th1 and Th2 CD4+ T cell responses: the alternative approaches. Annu Rev Immunol (1997) 15:297–322. doi:10.1146/annurev.immunol.15.1.297
12. Kalinski P, Moser M. Consensual immunity: success-driven development of T-helper-1 and T-helper-2 responses. Nat Rev Immunol (2005) 5(3):251–60. doi:10.1038/nri1569
13. Chao CC, Anderson WR, Hu S, Gekker G, Martella A, Peterson PK. Activated microglia inhibit multiplication of Toxoplasma gondii via a nitric oxide mechanism. Clin Immunol Immunopathol (1993) 67(2):178–83. doi:10.1006/clin.1993.1062
14. Hernandez JD, Baum LG. Ah, sweet mystery of death! Galectins and control of cell fate. Glycobiology (2002) 12(10):127r–36r. doi:10.1093/glycob/cwf081
15. Rabinovich GA, Toscano MA. Turning “sweet” on immunity: galectin-glycan interactions in immune tolerance and inflammation. Nat Rev Immunol (2009) 9(5):338–52. doi:10.1038/nri2536
16. Perillo NL, Marcus ME, Baum LG. Galectins: versatile modulators of cell adhesion, cell proliferation, and cell death. J Mol Med (Berl) (1998) 76(6):402–12. doi:10.1007/s001090050232
17. Liu FT, Rabinovich GA. Galectins: regulators of acute and chronic inflammation. Ann N Y Acad Sci (2010) 1183:158–82. doi:10.1111/j.1749-6632.2009.05131.x
18. Chen HL, Liao F, Lin TN, Liu FT. Galectins and neuroinflammation. Adv Neurobiol (2014) 9:517–42. doi:10.1007/978-1-4939-1154-7_24
19. Child MA, Garland M, Foe I, Madzelan P, Treeck M, van der Linden WA, et al. Toxoplasma DJ-1 regulates organelle secretion by a direct interaction with calcium-dependent protein kinase 1. MBio (2017) 8(1):e02189-16. doi:10.1128/mBio.02189-16
20. Liu Q, Li FC, Zhou CX, Zhu XQ. Research advances in interactions related to Toxoplasma gondii microneme proteins. Exp Parasitol (2017) 176:89–98. doi:10.1016/j.exppara.2017.03.001
21. Meissner M, Reiss M, Viebig N, Carruthers VB, Toursel C, Tomavo S, et al. A family of transmembrane microneme proteins of Toxoplasma gondii contain EGF-like domains and function as escorters. J Cell Sci (2002) 115(Pt 3):563–74.
22. Reiss M, Viebig N, Brecht S, Fourmaux MN, Soete M, Di Cristina M, et al. Identification and characterization of an escorter for two secretory adhesins in Toxoplasma gondii. J Cell Biol (2001) 152(3):563–78. doi:10.1083/jcb.152.3.563
23. Dunay IR, Heimesaat MM, Bushrab FN, Muller RH, Stocker H, Arasteh K, et al. Atovaquone maintenance therapy prevents reactivation of toxoplasmic encephalitis in a murine model of reactivated toxoplasmosis. Antimicrob Agents Chemother (2004) 48(12):4848–54. doi:10.1128/AAC.48.12.4848-4854.2004
24. Dang Y, Xu Y, Wu W, Li W, Sun Y, Yang J, et al. Tetrandrine suppresses lipopolysaccharide-induced microglial activation by inhibiting NF-kappaB and ERK signaling pathways in BV2 cells. PLoS One (2014) 9(8):e102522. doi:10.1371/journal.pone.0102522
25. Jones LA, Roberts F, Nickdel MB, Brombacher F, McKenzie AN, Henriquez FL, et al. IL-33 receptor (T1/ST2) signalling is necessary to prevent the development of encephalitis in mice infected with Toxoplasma gondii. Eur J Immunol (2010) 40(2):426–36. doi:10.1002/eji.200939705
26. Jash A, Kwon HK, Sahoo A, Lee CG, So JS, Kim J, et al. Topical application of porcine placenta extract inhibits the progression of experimental contact hypersensitivity. J Ethnopharmacol (2011) 133(2):654–62. doi:10.1016/j.jep.2010.10.054
27. Zhang AM, Shen Q, Li M, Xu XC, Chen H, Cai YH, et al. Comparative studies of macrophage-biased responses in mice to infection with Toxoplasma gondii ToxoDB #9 strains of different virulence isolated from China. Parasit Vectors (2013) 6(1):308. doi:10.1186/1756-3305-6-308
28. Zhou X, Xu Y, Yang G, Li F. Increased galectin-1 expression in muscle of Astragalus polysaccharide-treated type 1 diabetic mice. J Nat Med (2011) 65(3–4):500–7. doi:10.1007/s11418-011-0527-9
29. Bellac CL, Coimbra RS, Simon F, Imboden H, Leib SL. Gene and protein expression of galectin-3 and galectin-9 in experimental pneumococcal meningitis. Neurobiol Dis (2007) 28(2):175–83. doi:10.1016/j.nbd.2007.07.005
30. Markova V, Smetana K Jr, Jenikova G, Lachova J, Krejcirikova V, Poplstein M, et al. Role of the carbohydrate recognition domains of mouse galectin-4 in oligosaccharide binding and epitope recognition and expression of galectin-4 and galectin-6 in mouse cells and tissues. Int J Mol Med (2006) 18(1):65–76. doi:10.3892/ijmm.18.1.65
31. Tribulatti MV, Mucci J, Cattaneo V, Aguero F, Gilmartin T, Head SR, et al. Galectin-8 induces apoptosis in the CD4highCD8high thymocyte subpopulation. Glycobiology (2007) 17(12):1404–12. doi:10.1093/glycob/cwm104
32. Liesenfeld O, Kosek J, Remington JS, Suzuki Y. Association of CD4+ T cell-dependent, interferon-gamma-mediated necrosis of the small intestine with genetic susceptibility of mice to peroral infection with Toxoplasma gondii. J Exp Med (1996) 184(2):597–607. doi:10.1084/jem.184.2.597
33. Silva NM, Manzan RM, Carneiro WP, Milanezi CM, Silva JS, Ferro EA, et al. Toxoplasma gondii: the severity of toxoplasmic encephalitis in C57BL/6 mice is associated with increased ALCAM and VCAM-1 expression in the central nervous system and higher blood-brain barrier permeability. Exp Parasitol (2010) 126(2):167–77. doi:10.1016/j.exppara.2010.04.019
34. Schluter D, Deckert-Schluter M, Lorenz E, Meyer T, Rollinghoff M, Bogdan C. Inhibition of inducible nitric oxide synthase exacerbates chronic cerebral toxoplasmosis in Toxoplasma gondii-susceptible C57BL/6 mice but does not reactivate the latent disease in T. gondii-resistant BALB/c mice. J Immunol (1999) 162(6):3512–8.
35. Schluter D, Kaefer N, Hof H, Wiestler OD, Deckert-Schluter M. Expression pattern and cellular origin of cytokines in the normal and Toxoplasma gondii-infected murine brain. Am J Pathol (1997) 150(3):1021–35.
36. Schluter D, Meyer T, Strack A, Reiter S, Kretschmar M, Wiestler OD, et al. Regulation of microglia by CD4+ and CD8+ T cells: selective analysis in CD45-congenic normal and Toxoplasma gondii-infected bone marrow chimeras. Brain Pathol (2001) 11(1):44–55. doi:10.1111/j.1750-3639.2001.tb00380.x
37. Fischer HG, Nitzgen B, Reichmann G, Hadding U. Cytokine responses induced by Toxoplasma gondii in astrocytes and microglial cells. Eur J Immunol (1997) 27(6):1539–48. doi:10.1002/eji.1830270633
38. Jones RS, Lynch MA. How dependent is synaptic plasticity on microglial phenotype? Neuropharmacology (2015) 96(Pt A):3–10. doi:10.1016/j.neuropharm.2014.08.012
39. Murray PJ, Wynn TA. Protective and pathogenic functions of macrophage subsets. Nat Rev Immunol (2011) 11(11):723–37. doi:10.1038/nri3073
40. Freund YR, Zaveri NT, Javitz HS. In vitro investigation of host resistance to Toxoplasma gondii infection in microglia of BALB/c and CBA/Ca mice. Infect Immun (2001) 69(2):765–72. doi:10.1128/IAI.69.2.765-772.2001
41. Suzuki Y, Remington JS. The effect of anti-IFN-gamma antibody on the protective effect of Lyt-2+ immune T cells against toxoplasmosis in mice. J Immunol (1990) 144(5):1954–6.
42. Shi W, Xue C, Su XZ, Lu F. The roles of galectins in parasitic infections. Acta Trop (2018) 177:97–104. doi:10.1016/j.actatropica.2017.09.027
43. Burman J, Svenningsson A. Cerebrospinal fluid concentration of galectin-9 is increased in secondary progressive multiple sclerosis. J Neuroimmunol (2016) 292:40–4. doi:10.1016/j.jneuroim.2016.01.008
44. Steelman AJ, Li J. Astrocyte galectin-9 potentiates microglial TNF secretion. J Neuroinflammation (2014) 11:144. doi:10.1186/s12974-014-0144-0
45. Bernardes ES, Silva NM, Ruas LP, Mineo JR, Loyola AM, Hsu DK, et al. Toxoplasma gondii infection reveals a novel regulatory role for galectin-3 in the interface of innate and adaptive immunity. Am J Pathol (2006) 168(6):1910–20. doi:10.2353/ajpath.2006.050636
46. Lalancette-Hebert M, Swarup V, Beaulieu JM, Bohacek I, Abdelhamid E, Weng YC, et al. Galectin-3 is required for resident microglia activation and proliferation in response to ischemic injury. J Neurosci (2012) 32(30):10383–95. doi:10.1523/JNEUROSCI.1498-12.2012
47. Carruthers VB, Tomley FM. Microneme proteins in apicomplexans. Subcell Biochem (2008) 47:33–45. doi:10.1007/978-0-387-78267-6_2
Keywords: toxoplasmic encephalitis, galectins, microglial M1/M2 markers, T. gondii microneme proteins, mice
Citation: Liu J, Huang S and Lu F (2018) Galectin-3 and Galectin-9 May Differently Regulate the Expressions of Microglial M1/M2 Markers and T Helper 1/Th2 Cytokines in the Brains of Genetically Susceptible C57BL/6 and Resistant BALB/c Mice Following Peroral Infection With Toxoplasma gondii. Front. Immunol. 9:1648. doi: 10.3389/fimmu.2018.01648
Received: 07 January 2018; Accepted: 04 July 2018;
Published: 31 July 2018
Edited by:
Xun Suo, China Agricultural University, ChinaReviewed by:
Hridayesh Prakash, All India Institute of Medical Sciences, IndiaMarisa Mariel Fernandez, Instituto de Estudios de la Inmunidad Humoral (IDEHU), Argentina
Copyright: © 2018 Liu, Huang and Lu. This is an open-access article distributed under the terms of the Creative Commons Attribution License (CC BY). The use, distribution or reproduction in other forums is permitted, provided the original author(s) and the copyright owner(s) are credited and that the original publication in this journal is cited, in accordance with accepted academic practice. No use, distribution or reproduction is permitted which does not comply with these terms.
*Correspondence: Shiguang Huang, dGhzaGdAMTI2LmNvbQ==;
Fangli Lu, ZmFuZ2xpbHVAeWFob28uY29t