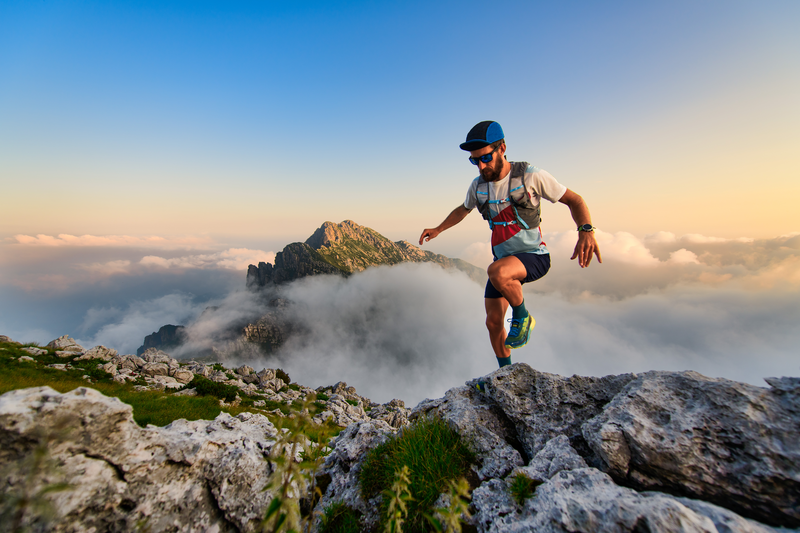
94% of researchers rate our articles as excellent or good
Learn more about the work of our research integrity team to safeguard the quality of each article we publish.
Find out more
ORIGINAL RESEARCH article
Front. Immunol. , 09 July 2018
Sec. T Cell Biology
Volume 9 - 2018 | https://doi.org/10.3389/fimmu.2018.01579
This article is part of the Research Topic Molecular Determinants of γδ T cell Selection, Maintenance and Function View all 15 articles
T lymphocytes expressing the γδ T cell receptor (γδ TCR) can recognize antigens expressed by tumor cells and subsequently kill these cells. γδ T cells are indeed used in cancer immunotherapy clinical trials. The anti-CD3ε antibody UCHT1 enhanced the in vitro tumor killing activity of human γδ T cells by an unknown molecular mechanism. Here, we demonstrate that Fab fragments of UCHT1, which only bind monovalently to the γδ TCR, also enhanced tumor killing by expanded human Vγ9Vδ2 γδ T cells or pan-γδ T cells of the peripheral blood. The Fab fragments induced Nck recruitment to the γδ TCR, suggesting that they stabilized the γδ TCR in an active CD3ε conformation. However, blocking the Nck-CD3ε interaction in γδ T cells using the small molecule inhibitor AX-024 neither reduced the γδ T cells’ natural nor the Fab-enhanced tumor killing activity. Likewise, Nck recruitment to CD3ε was not required for intracellular signaling, CD69 and CD25 up-regulation, or cytokine secretion by γδ T cells. Thus, the Nck-CD3ε interaction seems to be dispensable in γδ T cells.
T cells are part of the adaptive immune system and can be divided into αβ and γδ T cells, depending on the T cell antigen receptor (TCR) they express. Whereas most αβ TCRs recognize peptides presented by MHC molecules, γδ TCRs recognize stress-induced self-antigens (1, 2), lipids or pyrophosphates that are secreted by microbes or overproduced in tumor cells (3–7).
The main subset of γδ T cells in human blood is Vγ9Vδ2, which accounts for 2–10% of all T cells. The Vγ9Vδ2 TCR recognizes self and foreign non-peptidic phosphorylated small organic compounds of the isoprenoid pathway, collectively termed phosphoantigens (8–12). It is known that Vγ9Vδ2 T cells are also stimulated by certain tumor cells, such as the Daudi B cell lymphoma (13), which most likely expresses high levels of phosphoantigens (14). Alternatively, γδ T cells recognize cell surface molecules that are differentially expressed on transformed solid tumors or lymphomas and leukemias (7, 15). An enhanced production of phosphoantigens in transformed cells can be further increased by therapeutically administered nitrogen-containing bisphosphonates, such as zoledronate, which inhibit the farnesyl pyrophosphate synthase of the isoprenoid pathway (16, 17). Several studies demonstrated that a repetitive stimulation of γδ T cells in vivo is necessary to reduce tumor growth (18–20). While sustained stimulation of Vγ9Vδ2 γδ T cells by phosphoantigens or nitrogen-containing bisphosphonates often leads to their exhaustion, bispecific antibodies provide a newly tool to target γδ T cells to antigens expressed by tumor cells and enhanced their cytotoxic activity (19, 21–23). Although the exact molecular mechanism leading to phosphoantigen recognition is a matter of debate (24, 25), this recognition is clearly mediated by cognate interaction with the Vγ9Vδ2 TCR.
T cell antigen receptors consist of a clonotypic TCRαβ or TCRγδ heterodimer, and the CD3δε, CD3γε, and CD3ζζ dimers. TCRαβ and TCRγδ bind to the antigen and the CD3 chains transduce the signal of antigen binding into the cell by phosphorylation of the tyrosines in their cytoplasmic tails by Src-family kinases. Consequently, the tyrosine kinase ZAP70 can bind to phosphorylated CD3 and the signal of ligand binding is transmitted further to intracellular signaling cascades, such as Ca2+ influx and the Ras/Erk pathway, ultimately resulting in the activation of the T cell. This includes the execution of the cytotoxic activity to kill infected or tumor cells, up-regulation of CD69 and CD25, as well as secretion of cytokines.
How antigen binding to the TCR is communicated to the cytosolic tails of CD3 is not well understood. The αβ TCR is in equilibrium between two reversible conformations: the antigen-stabilized active CD3 conformation and the resting conformation adopted by non-engaged TCRs (26–28). The active CD3 conformation is stabilized by peptide-MHC or anti-CD3 antibody binding to the αβ TCR (29, 30), and it is absolutely required (but not sufficient) for TCR activation (27, 30–32). This active CD3 conformation is defined by the exposure of a proline-rich sequence (PRS) in CD3ε that then binds to the SH3.1 domain of the adaptor protein Nck [SH3.1(Nck)] (26, 33). Blocking the CD3ε–Nck interaction by the small molecule inhibitor AX-024 or by other means diminished ligand-induced CD3 phosphorylation and downstream signaling events (34–36). Shifting to the active CD3 conformation is necessary for αβ TCR triggering, however, it is not sufficient (30, 37). Fab fragments of anti-CD3 antibodies stabilize the active conformation, but are unable to elicit biochemical signals leading to T cell activation (30, 38, 39). In addition, antigen-induced αβ TCR clustering and/or phosphatase exclusion are required, most likely to elicit stable phosphorylation of the ITAMs and thus, T cell activation (30, 37, 40).
How antigen binding to the γδ TCR is transmitted to the cytosolic tails of CD3 is even more obscure. Antigen binding to TCRγδ failed to expose the CD3ε’s PRS, in sharp contrast to the αβ TCR, but efficiently activated the γδ T cell (41). Artificial induction of the active conformation by binding the anti-CD3ε antibody UCHT1 to the γδ TCR enhanced the cytotoxic activity of human γδ T cells against a pancreatic tumor cell line (41). Whether Nck is recruited to γδ TCRs in the natural or the UCHT1 enhanced activity and whether this plays a role in the increased tumor killing is to date unknown.
Here, we used expanded γδ T cells from human peripheral blood of healthy donors and show that UCHT1 and Fab fragments of UCHT1 lead to the recruitment of Nck to the γδ TCR. Further, we stimulated the γδ T cells with B cell lymphomas and demonstrate that UCHT1 Fab fragments increase the tumor killing by the γδ T cells and that Nck binding to the γδ TCR is not involved in this tumor killing.
Informed consent for the performed studies was obtained from the donors in accordance with the Declaration of Helsinki and Institutional Review Board approval from the University of Freiburg Ethics Committee (412/9). Human peripheral blood mononuclear cells were isolated from healthy donors by using a Ficoll–Hypaque gradient. Cells were adjusted to 106 cells/ml and cultured in RPMI 1640 supplemented with 10% fetal calf serum (FCS) and antibiotics.
To expand Vγ9Vδ2 γδ T cells, cells were stimulated with 2.5 µM zoledronate and 50 U/ml rIL-2 (Novartis). Additionally, rIL-2 was added every 2 days over a culture period of 21 days. After 14 days the purity of expanded γδ T cells was analyzed by flow cytometry and was >95% Vγ9Vδ2 T cells.
To expand different γδ T cell subsets, cells were stimulated with 1 µg/ml concanavalin A and rIL-2 and rIL-4 (both 100 U/ml) were added to the cell suspensions. Additionally, rIL-2 and IL-4 were added every 3–4 days over a culture period of 21 days. After 14 days γδ T cells were enriched by negative isolation using the human TCRγ/δ+ T Cell Isolation Kit (Miltenyi Biotech). Cultures were composed of 20–40% Vδ1+ and 60–80% Vδ2+ T cells and the purity of the enriched γδ T cells was evaluated by flow cytometry. Cultures with a purity >95% were used for the experiments.
Daudi or Raji tumor cells (5,000 cells/well in a 96-well plate) were incubated with 50 µl 51Cr for 1 h at 37°C. After labeling, cells were washed two times and the cell concentration was adjusted to 5 × 104 cells/ml in RPMI 1640 supplemented with 10% FCS, antibiotics, and 50 U/ml rIL-2 (Novartis). The assay was performed in U bottom 96-well plate, with an effector (γδ T cell) to target (Daudi or Raji cells) ratio of 12.5:1, in triplicates and with six replicates for spontaneous (51Cr release from target cells in medium alone) and maximum release (51Cr release from target cells lysed with the detergent Trition X-100). The required amount of effector cells (6.25 × 104 cells/well) was harvested and the concentration was adjusted to 1.25 × 106 cells/ml in RPMI 1640 supplemented with 10% FCS and 50 U/ml rIL-2 (Novartis). To the labeled tumor cells the expanded γδ T cells were added, and left untreated (−) or treated with 5 µg/ml UCHT1, 3.33 µg/ml Fab, or 3.33 µg/ml Fabred (to obtain equimolar amounts). Additionally, DMSO or different concentrations of the inhibitor AX-024 (36) solubilized in DMSO were added. Cells were incubated at 37°C and 5% CO2 for 5 h. After incubation, 50 µl of the supernatant was transferred into 51Cr filter plates (Lumaplate). The plates were measured in a microplate scintillation γ-ray counter and data were acquired in counts per minute (cpm). Cytotoxicity was calculated according to the formula: specific lysis (%) = (experimental cpm − spontaneous cpm): (maximum cpm − spontaneous cpm) × 100.
Zoledronate-expanded γδ T cells were starved for 1 h at 37°C in RPMI 1640 without FCS and left unstimulated or stimulated with 5 µg/ml anti-CD3 antibody UCHT1. Cells were lysed in lysis buffer containing 20 mM Tris–HCl pH 8, 137 mM NaCl, 2 mM EDTA, 10% glycerol, protease inhibitor cocktail, and 0.3% Brij96V. Subsequently, insoluble material was removed by centrifugation. Postnuclear fractions were incubated with glutathione beads coupled to GST-SH3.1(Nck) at 4°C as described (26). Beads, including the bound proteins, were washed and proteins separated by non-reducing SDS-PAGE. Western blotting was performed with anti-CD3ζ and anti-GST antibodies.
UCHT1 Fab fragments were prepared using the Pierce® Fab Micro Preparation Kit from Thermo Fisher Scientific, which uses the enzyme papain to cleave the complete UCHT1 antibody and protein A coupled beads to purify the Fab fragments. The purified Fab fragments were analyzed by SDS-PAGE and Coomassie staining. To generate reduced Fab fragments (Fabred) the purified Fab fragment was incubated with 10 mM dithiothreitol for 30 min at room temperature. Afterward, 1 mM iodoacetamide was added and incubated for further 30 min at room temperature. The Fabred were then immediately used. Purity of Fab and Fabred was further tested by their inability to induce Ca2+ influx in T cells.
Concanavalin A expanded γδ T cells were incubated with 5 µg/ml UCHT1, 3.33 µg/ml Fab, or 3.33 µg/ml Fabred or left untreated at 37°C and 5% CO2 for 5 h. Cells were washed two times and stained with APC-labeled anti-mouse IgG antibody on ice (Southern Biotech). The labeled cell suspension was analyzed by the Gallios™ flow cytometer and the data were analyzed with FlowJo software.
Jurkat and G8 γδ TCR-expressing Jurkat cells were stained with APC-labeled anti-mouse γδ TCR (GL3; eBioscience). Cells were analyzed as described above.
Five million cells were resuspended in 1 ml RPMI 1640 medium supplemented with 1% FCS in the presence or absence of 10 nM AX-024, antibiotics, and labeled in the dark with 0.1% pluronic acid, 2.6 µM Fluo-3 AM, and 5.5 µM Fura Red AM (Life Technologies) for 45 min at 37°C. The stained cells were washed and kept on ice in the dark until the measurement. For calcium influx, cells were diluted 1:20 with pre-warmed medium and maintained at 37°C during the event collection on a CyAn ADP flow cytometer (Beckman Coulter). Baseline fluorescence was monitored for 1.5 min, then the stimuli were added as indicated (the Fab and Fabred fragments were added 30 s earlier to ensure binding to the γδ TCR when the other stimuli were added). The stimulation was recorded for further 5 min. Data were analyzed with the FlowJo software.
Peripheral blood mononuclear cells were isolated from healthy donors using a Ficoll–Hypaque gradient. T cells were obtained by negative isolation using the Pan T cell Isolation Kit (Miltenyi Biotech). Cells were taken in RPMI 1640 supplemented with 10% FCS and were rested for 1 h at 37°C in the presence or absence of different concentrations of the inhibitor AX-024 (36). Cells were left unstimulated or stimulated with 10 µg/ml anti-CD3 antibody UCHT1 for 2 min or 5 min and were fixed with 2% paraformaldehyde for 30 min on ice. Subsequently, cells were permeabilized with 87.7% methanol for 30 min on ice and stained with rabbit anti-phospho-ZAP70 (Cell Signaling) or rabbit phospho-Erk (Cell Signaling) overnight. Next, cells were stained with biotin-labeled anti-CD3 (UCHT1; BioLegend), PE-labeled anti-γ/δTCR antibodies (Life Technologies), and DyLight-labeled anti-rabbit IgG (Thermo Scientific) and subsequently with eFluor 450-labeled Streptavidin (eBioscience). Cells were measured by flow cytometry and gated on CD3- and TCRγδ-positive cells for analysis.
Cells were grown on diagnostic microscopic slides (Thermo Scientific). They were left unstimulated, stimulated with 5 µg/ml UCHT1, 3.33 µg/ml Fab, or 3.33 µg/ml Fabred and simultaneously treated with 10 nM AX-024 at 37°C for 5 min. Cells were then fixed with 4% paraformaldehyde, permeabilized with 0.5% saponin, and blocked with blocking solution. Subsequently, cells were co-incubated with the goat anti-CD3ε (M20ε, Santacruz) and a rabbit anti-Nck1 antibody (Cell Signaling). PLA between the CD3ε and Nck1 molecules was performed with the Duolink kit according to the manufacturer’s instructions (Olink Bioscience), resulting in red fluorescence signals. Cell nuclei were stained with DAPI. A confocal microscope (C2, Nikon) was used for imaging and analysis. The number of the PLA signal dots was scored with the BlobFinder program (Uppsala University).
To each well of a U bottom 96-well plate 2 × 104 Daudi or Raji tumor cells, 2.5 × 105 expanded γδ T cells, and 5 µg/ml UCHT1, 3.33 µg/ml Fab, 3.33 µg/ml Fabred, or medium (unstimulated) were given. In addition, the cells were left untreated or treated with different concentrations of AX-024. The plate was incubated at 37°C and 5% CO2 for 18 h. After incubation, the supernatants were kept at −80°C to quantify the amounts of TNFα and IFNγ (see below). Cells were stained with APC-labeled anti-CD25 (eBioscience) or APC-labeled anti-CD69 (Life Technologies) together with PE-labeled anti-γ/δTCR antibodies (Life Technologies). Cells were analyzed by flow cytometry gating on γδTCR-positive cells.
The concentrations of TNFα and IFNγ in the culture supernatants were measured by standard enzyme linked immunosorbent assay (ELISA).
Data are represented as mean ± SEM or ± SD. All differences between experimental groups were analyzed with the Student’s t-test. Significant differences were considered when the p values were less than 0.05. (n.s. = non significant, *p < 0.05, **p < 0.01, ***p < 0.001, and ****p < 0.0001). n refers to the number of independently performed experiments.
Human short-term expanded Vγ9Vδ2 γδ T cells can kill pancreatic ductal adenocarcinoma Panc89 cells in vitro (21, 23), which was enhanced by co-incubation with the anti-CD3ε antibody UCHT1 (41). In order to test target cells from different origins, we used now the 51Cr-labeled human B cell lymphoma lines Daudi or Raji (13) and incubated them with zoledronate-expanded peripheral blood human γδ T cells in the presence or absence of UCHT1. Release of 51Cr to the cell culture supernatant is a measure for the lysis of the tumor cells and was quantified with a scintillation γ-ray counter. Approximately 40% of the Daudi and 5% of the Raji cells were specifically killed by the γδ T cells in the absence of exogenously added antibody (Figure 1). The addition of the UCHT1 antibody strongly enhanced tumor killing by the γδ T cells reaching 75% of specific killing for both cell lines (Figure 1).
Figure 1. UCHT1 increased tumor killing by γδ T cells. 51Cr-labeled Daudi and Raji cells were incubated with zoledronate-expanded human γδ T cells without (−) or with the addition of 5 µg/ml UCHT1 using an effector to target (T cell to tumor cell) ratio of 12.5:1. After 5 h of co-culture, the amount of released 51Cr was measured in the supernatant by γ-ray spectroscopy. Data represent mean ± SD of triplicate wells (n = 3). Significance was determined by unpaired t-test, two-tailed between untreated γδ T cells and treated samples.
UCHT1 stabilizes the γδ TCR in the active CD3 conformation, in which the CD3ε PRS is exposed (41). Since only the exposed PRS binds to SH3.1(Nck), PRS exposure can be measured with the SH3.1(Nck) pull down assay (Figure 2A). To test whether the UCHT1-bound γδ TCR can bind to SH3.1(Nck) in our zoledronate-expanded γδ T cells, we incubated the cells with or without UCHT1 and lysed them. Performing a pull down assay using SH3.1(Nck)-coupled beads, we found that UCHT1 increased the amount of γδ TCR that bound to the beads (Figures 2B,C). This indicated that SH3.1(Nck) can bind to the γδ TCR and that UCHT1 stabilizes the γδ TCR in the active CD3 conformation.
Figure 2. Using its SH3.1(Nck) domain Nck binds to the UCHT1-stimulated γδ T cell antigen receptor (TCR). (A) Schematic of the SH3.1(Nck) pull down assay. (B) Zoledronate-expanded γδ T cells were left untreated (−) or stimulated with 5 µg/ml UCHT1 at 37°C for 5 min. The cellular lysates were incubated with SH3.1(Nck)-coupled beads and bound proteins separated by SDS-PAGE along with aliquots of the cellular lysate. Western blotting was done using anti-CD3ζ and anti-GST antibodies. (C) The normalized ratio of the band intensities of CD3ζ to GST in the SH3.1(Nck) pull down is plotted. Data of three independent experiments were used to calculate the mean ± SD; statistics was done by two-tailed t-test. (D) Schematic of the in situ proximity ligation assay (PLA) using anti-CD3ε and anti-Nck antibodies. (E) Close proximity between the TCR and Nck was detected by in situ PLA. Zoledronate-expanded γδ T cells were either left untreated (−) or treated with 5 µg/ml UCHT1 in the absence or presence of 10 nM AX-024 at 37°C for 5 min. After fixation and permeabilization, PLA was performed using the primary antibodies goat anti-CD3ε (M20) and rabbit anti-Nck1, and the corresponding secondary antibodies. Nuclei were stained with DAPI. (F) The corresponding quantification of the red PLA dots and the mean ± SEM is displayed; statistics was done by two-tailed t-test. For each condition 500 cells were analyzed. Three independent experiments were performed (n = 3).
To date it is unknown whether endogenous Nck is recruited to the γδ TCR upon stimulation. To test this, we used the in situ PLA. PLA is a technique that allows visualization of the close proximity between endogenous proteins in fixed cells by a red fluorescent dot (42). Recently, we established the PLA to quantify the proximity of Nck with the αβ TCR using anti-CD3ε and anti-Nck antibodies (33). Here we applied this assay to γδ T cells. To this end, we incubated expanded human γδ T cells with UCHT1 and performed PLA (Figure 2D). Indeed, CD3ε–Nck proximity was increased in UCHT1-stimulated cells compared to unstimulated cells (Figures 2E,F) suggesting that Nck is indeed recruited to the γδ TCR upon UCHT1 binding.
To test whether endogenous Nck uses its SH3.1 domain to bind to the CD3ε PRS in the γδ TCR, we made use of the small molecule inhibitor AX-024. In αβ T cells AX-024 was shown to specifically bind to SH3.1(Nck) and hence block the SH3.1(Nck)–PRS interaction (36). Using αβ T cells as a control, we made sure that PLA is a suitable assay to test the inhibition of the SH3.1(Nck)–PRS interaction mediated by AX-024 (Figure S1 in Supplementary Material). Next, we incubated the γδ T cells with UCHT1 in the absence or presence of 10 nM AX-024. Indeed, the CD3ε–Nck proximity was reduced to background levels after AX-024 treatment (Figures 2E,F), indicating that the PRS–SH3.1 interaction is necessary for the recruitment of Nck to the γδ TCR upon UCHT1 binding. This finding was corroborated with a PLA experiment in which CD3 phosphorylation was blocked using the Src kinase inhibitor PP2 (Figure S2 in Supplementary Material). Indeed, Nck was recruited to the γδ TCR in the presence of PP2, suggesting that an SH2(Nck)-phospho-CD3 interaction was not required.
Complete anti-CD3ε antibodies bind bivalently to the TCR leading to T cell activation, whereas Fab fragments of the same antibodies bind monovalently and fail to activate T cells (30, 37–39). The use of complete anti-CD3ε antibodies in therapeutical settings might have the drawback of unspecific polyclonal T cell activation. Thus, we aimed here to investigate whether UCHT1 Fab fragments that only bind monovalently to the TCR might enhance tumor killing by human γδ T cells in the absence of undesired unspecific T cell activation. To this end, we generated pure Fab fragments by cleaving UCHT1 antibodies with papain and subsequently purifying the Fab fragments using the Pierce Fab Micro Preparation kit. The complete UCHT1 antibody before and after digestion and the purified Fab fragments were analyzed by reducing SDS-PAGE and Coomassie staining (Figure 3A). We found that the complete immunoglobulin heavy chain (HC) at 50 kDa was not detectable in the Fab fragments, but the light chain (LC) was traceable at 24 kDa (lane 4). As the variable and CH1 parts of the HC have the same size as the LC, they are not visible as an additional band in SDS-PAGE (Figure 3A, Fab).
Figure 3. Preparation of UCHT1 Fab fragments. (A) The anti-CD3ε antibody UCHT1 was digested with papain using a kit from Thermo Fisher Scientific. The Fab fragments were purified with protein A coupled beads. The complete antibody (lane 1), the first and second digestions (lanes 2 and 3), and the purified Fab fragment (lane 4) were separated by reducing SDS-PAGE followed by Coomassie staining (n > 3). (B) Jurkat Vγ9Vδ2 cells were labeled with Fluo-3 AA and Fura Red AM and the baseline of cellular Ca2+ was measured by flow cytometry for 1 min. The indicated reagents were then added and Ca2+ levels were measured for additional 6 min (black lines). The gray lines represent baseline Ca2+ level without addition of any stimuli (n > 3). (C) Concanavalin A expanded γδ T cells were stained with UCHT1, Fab, Fabred, or left untreated, followed by an APC-labeled anti-mouse IgG antibody as a secondary reagent. Fluorescence intensities were quantified by flow cytometry (n > 3).
We found earlier that very small amounts of F(ab′)2 fragments might contaminate the Fab preparation. They are not detectable by SDS-PAGE and Coomassie staining, but due to their cross-linking ability they can lead to TCR activation in functional assays (30). F(ab′)2 fragments can be reduced to Fab fragments by the use of dithiothreitol (DTT) followed by quenching of DTT with iodoacetamide (30). To test for the presence of small amounts of contaminating UCHT1 or UCHT1 F(ab′)2 fragments in the Fab preparation, we incubated our reagents with TCRαβ-negative Jurkat T cells expressing the human Vγ9Vδ2 TCR (43, 44) and measured calcium influx into the cytosol as a read-out for TCR activity. The unreduced Fab fragments either did not induce any or just a very small increase in cytosolic calcium (Figure 3B, upper panel), indicating that some preparations were contaminated with tiny amounts of F(ab′)2. However, the reduced Fab fragments never induced any calcium response (Figure 3B, second panel). As a control, the reduced Fab fragment cross-linked by anti-κ LC antibodies, resulted in calcium influx, indicating that the reduced Fab fragments were functional. Treatment of the cells with the complete UCHT1 antibody resulted in the strongest calcium response (Figure 3B, lowest panel). From now on, UCHT1 Fab fragments will be called “Fab” and the reduced fragments “Fabred.”
By using staining and flow cytometry, we show that Fab and Fabred can equally well bind to human γδ T cells (Figure 3C).
In conclusion, Fabred preparations contained functional Fab fragments that are purely monovalent and failed to crosslink and activate the TCR.
Next, we tested whether Fab and Fabred enhance tumor killing by γδ T cells. To this end, we co-cultured zoledronate-expanded γδ T cells with Daudi or Raji cells as in Figure 1. Both, Fab and Fabred increased killing of the tumor cells (Figure 4A). In Daudi cells, which were more susceptible to the cytotoxic activity of the γδ T cells, Fab, and Fabred were almost as active as UCHT1. In Raji cells, which were only killed to 5% by γδ T cells, Fab and Fabred enhanced the cytotoxic activity, so that 30% of the cells were killed.
Figure 4. Fab and Fabred fragments enhanced tumor killing by γδ T cells. 51Cr-labeled Daudi and Raji cells were incubated with zoledronate (A) or concanavalin A (B) expanded γδ T cells in triplicates without (−) or with 5 µg/ml UCHT1, 3.33 µg/ml Fab, or 3.33 µg/ml Fabred (these concentrations were chosen to obtain equimolar amounts of the different reagents). The effector to target ratio was 12.5:1. After 5 h of co-culture, the amount of released 51Cr was measured in the supernatant by γ-ray spectroscopy (n = 3). (C) Concanavalin A expanded γδ T cells from four different donors (HD1, 2, 3, and 4) were used in a chromium-release assay using Daudi cells as target as in (A) (n = 1). Data represent mean ± SD of triplicate wells. Significance was determined by unpaired t-test, two-tailed between untreated γδ T cells and UCHT1, Fab or Fabred-treated samples.
Zoledronate and IL-2 specifically expand human Vγ9Vδ2 γδ T cells (16, 23). γδ T cells can also be expanded from human peripheral blood using the lectin concanavalin A, IL-4, and IL-2, resulting in cultures containing both, Vδ1 and Vδ2 γδ T cells (45). Concanavalin A, IL-4, and IL-2 expanded γδ T cells (here called concanavalin A expanded γδ T cells) also killed Daudi and Raji cells, and this activity was increased by UCHT1, Fab, and Fabred (Figure 4B). This effect was not donor-specific, since the enhanced tumor killing mediated by UCHT1, Fab, and Fabred was also observed when using concanavalin A expanded γδ T cell from blood of four different healthy donors (HD, Figure 4C).
Fab and Fabred do not cross-link the γδ TCR, but might enhance tumor killing by stabilizing the active CD3 conformation of the γδ TCR—just as the complete UCHT1 antibody does (Figure 2). Hence, we used PLA to test whether Fab and Fabred induced the recruitment of Nck to the γδ TCR. Indeed, treatment of the γδ T cells with Fab and Fabred resulted in an increase in TCR–Nck proximity (Figures 5A,B). Again, the incubation with the small molecule inhibitor AX-024 reduced Nck binding to the γδ TCR to background levels. This finding suggests that binding of Fab and Fabred to the γδ TCR artificially stabilizes the active CD3 conformation and results in the recruitment of Nck to the γδ TCR using the SH3.1(Nck) domain.
Figure 5. UCHT1 Fab induced the recruitment of Nck to the γδ T cell antigen receptor. (A) The proximity ligation assay as in Figure 2 was performed using 5 µg/ml UCHT1, 3.33 µg/ml Fab, and 3.33 µg/ml Fabred in the absence or presence of 10 nM AX-024. (B) The data of (A) were analyzed as in Figure 2F; 700 cells were analyzed per condition. Two independent experiments were performed (n = 2).
We next analyzed whether Nck recruitment to the γδ TCR upon UCHT1, Fab, or Fabred treatment was involved in γδ T cell activation mediated by tumor cells, and for the cytotoxic activity of γδ T cells. Concanavalin A expanded γδ T cells (Figure 6A) or zoledronate-expanded γδ T cells (Figure 6B) were incubated with Daudi cells in the presence or absence of UCHT1, Fab, or Fabred with or without AX-024 to block the PRS–SH3.1 interaction. Two concentrations of AX-024 were used, 10 nM AX-024 as in Figure 5 and 100 nM AX-024. All three anti-CD3 reagents significantly enhanced tumor killing by the γδ T cells. AX-024 did not disturb the γδ T cell-mediated “basal” tumor cell killing (without anti-CD3 reagents). Importantly, AX-024 did also not influence the enhanced killing activity in the presence of the anti-CD3 reagents for the concanavalin A expanded γδ T cells (Figure 6A). In case of the zoledronate-expanded γδ T cells (Figure 6B) very small reductions with 10 nM, but not with 100 nM AX-024, can be detected. Hence, we concluded that AX-024 did also not diminish tumor killing for the zoledronate-expanded γδ T cells.
Figure 6. Tumor killing by γδ T cells is independent of Nck recruitment to the γδ T cell antigen receptor. 51Cr-labeled Daudi cells were incubated with concanavalin A (A) or zoledronate (B) expanded γδ T cells without (−) or with 5 µg/ml UCHT1, 3.33 µg/ml Fab, or 3.33 µg/ml Fabred. In addition, 10 or 100 nM of AX-024 was included or not. The effector to target ratio was 12.5:1. After 5 h of co-culture, the amount of released 51Cr was measured in the supernatant by γ-ray spectroscopy. Data represent mean ± SD of triplicates (n = 3). Significance was determined by unpaired t-test, two-tailed between untreated samples and samples treated with AX-024.
These findings indicate that Nck recruitment to the γδ TCR is dispensable for the cytotoxic activity of γδ T cells stimulated by tumor cells.
In addition to cytotoxic activity, γδ T cell activation involves up-regulation of the expression of the high affinity IL-2 receptor CD25 and of the activation marker CD69 (46). Next, we sought to analyze whether Fab can also enhance these activation events in γδ T cells and whether Nck binding to the γδ TCR was required for that. Zoledronate-expanded γδ T cells were stimulated with Daudi or Raji cells in the presence or absence of UCHT1, Fab, or Fabred with or without AX-024. As with tumor cell killing, all three anti-CD3 reagents enhanced CD25 (Figures 7A,B) or CD69 (Figure 7C) up-regulation by the γδ T cells. Blocking the γδ TCR–Nck interaction with AX-024 did neither affect the expression of CD25 nor of CD69 independently of the anti-CD3 reagents (Note that the decrease of UCHT1 enhanced CD69 expression by AX-024 was not seen in other experiments).
Figure 7. The up-regulation of activation markers and cytokines is independent of Nck recruitment to the γδ T cell antigen receptor (TCR). Daudi and Raji cells were incubated with zoledronate-expanded γδ T cells without (−) or with 5 µg/ml UCHT1, 3.33 µg/ml Fab, or 3.33 µg/ml Fabred. In addition, 10 nM AX-024 was included or not. The γδ T cell to target cell ratio was 12.5:1. The cells were co-cultured at 37°C and 5% CO2 for 18 h. Subsequently, cells were either stained with anti-CD25 and anti-γ/δTCR (A,B) or with anti-CD69 and anti-γ/δTCR (C) antibodies. Fluorescence intensities were quantified by flow cytometry (A) and the mean fluorescence intensity is displayed (B,C). Data represent mean ± SD of triplicates (n = 3). Significance was determined by unpaired t-test, two-tailed between untreated samples and samples treated with AX-024. (D,E) The experiments were performed as in (A). After co-culturing of the cells for 18 h, the supernatants were harvested. TNFα (D) and IFNγ (E) concentrations were quantified by enzyme linked immunosorbent assay.
We have also tested whether AX-024 impacts on CD69 up-regulation in fresh, naive γδ T cells from human blood. As with the expanded cells, AX-024 did not change the extent of CD69 expression when the cells were stimulated with UCHT1 (Figure S3 in Supplementary Material).
Furthermore, γδ T cells secrete pro-inflammatory cytokines, such as TNFα and IFNγ, upon activation by tumor cells (47). Finally, we show that TNFα and IFNγ production induced by Daudi or Raji cells was enhanced by UCHT1, Fab, or Fabred (Figures 7D,E). AX-024 neither diminished TNFα and IFNγ secretion in the absence nor in the presence of the anti-CD3 reagents.
Together, our data indicate that UCHT1, Fab, or Fabred binding to the γδ TCR enhances the tumor cell-induced activation of γδ T cells. Although Nck is recruited to the anti-CD3 bound γδ TCR, this recruitment seems to be dispensable for γδ T cell activation events, such as cytotoxicity, CD25, and CD69 up-regulation, as well as TNFα and IFNγ secretion.
If Nck recruitment to the γδ TCR is not involved in tumor killing and the up-regulation of activation markers, it might also not be required for the γδ TCR induced induction of intracellular signaling. To test this, we stimulated fresh human γδ T cells with UCHT1 in the absence or presence of AX-024 and measured the phosphorylation of the kinases ZAP70 and Erk by flow cytometry (Figures S4A,B in Supplementary Material). As expected, γδ TCR stimulation increased the amount of phospho-ZAP70 and phospho-Erk. Importantly, treatment of the cells with AX-024 did not influence the extent of ZAP70 or Erk phosphorylation, indicating that recruitment of Nck to the γδ TCR was not required for the induction of signaling by the UCHT1-stimulated γδ TCR.
Next we asked whether Fab fragments would also increase signaling by an antigen-triggered γδ TCR. In order to use a cognate ligand for the γδ TCR, we switched to a different γδ TCR system, namely the G8 γδ TCR where a clearly defined ligand, namely MHC class I-like T22, can be used (48, 49). In fact, stimulation of G8 γδ TCR-expressing cells with soluble T22 tetramers leads to T cell stimulation without stabilizing the CD3 conformational change at the γδ TCR (41). Here, we expressed the G8 γδ TCR in Jurkat T cells, similar to as we did with a chimeric γδ TCR in a Jurkat-derived cell (27). Indeed, the G8 γδ TCR was expressed on the surface of the Jurakt cells (Figure 8A).
Figure 8. Calcium signaling is enhanced by Fab fragments and does not require the Nck-γδ T cell antigen receptor (TCR) interaction. (A) G8 γδ TCR-expressing Jurkat cells were stained with the γδ TCR-specific antibody GL3 and analyzed by flow cytometry. As controls, the GL3-stained parental Jurkat cells and unstained G8 γδ TCR-expressing cells are shown (n > 3). (B) Jurkat cells expressing the G8 γδ TCR were stimulated with the G8 ligand T22 tetramers (T22t), UCHT1 Fab, or a combination of both T22t and Fab. Intracellular calcium was measured using the dyes Fluo-3 and Fura-red by flow cytometry. Calcium influx is depicted as the median ratio of Fluo-3 to Fura-red fluorescence over time. As a negative control, steptavidin was added to the cells. The arrow indicates addition of the stimuli (n = 3). (C) The experiment was performed as in (C) with the difference that cells without and with 10 nM AX-024 were stimulated with the combination of T22t and Fab. As a control, PBS was added to the cells (n = 3).
Next, we stimulated the G8 γδ TCR-expressing Jurkat cells with soluble T22 tetramers and measured the amount of intracellular calcium as a signaling read-out. As expected, the Fab fragments hardly induced calcium signaling downstream of the γδ TCR, whereas T22 tetramers did (Figure 8B). Importantly, adding Fab to the T22 tetramers augmented the calcium response, most likely by stabilizing the γδ TCR in the active CD3 conformation. The same result was obtained when using Fabred fragments (Figure S5A in Supplementary Material).
To test whether the enhanced calcium response was sensitive to the recruitment of Nck to the γδ TCR, we stimulated the G8 γδ TCR-expressing Jurkat cells with the T22 tetramers and Fab in the absence or presence of AX-024. Clearly, AX-024 treatment did reduce the calcium response (Figure 8C), suggesting that the Nck–γδ TCR interaction was not required for the enhanced calcium signaling. The same result was obtained when using Fabred fragments (Figure S5B in Supplementary Material).
Together our data suggest that Fab fragments enhance signaling via the γδ TCR by stabilizing the active CD3 conformation, independent of the Nck–γδ TCR interaction.
The potential use of cytotoxic γδ T cells in immunotherapy against cancer is particularly attractive. Since γδ T cells are independent of antigen presentation by MHC class I and of the presence of mutated epitopes, they are ideal effectors against tumors with low mutation loads. Cytotoxic γδ T cells display afferent responses and have been recognized as the best favorable prognosis marker for solid tumors when infiltrated immune cells were analyzed (50). This suggested that γδ T cells play an important role in the defense against tumors in human patients. Therefore, γδ T cells are currently tested as cellular reagents in cancer immunotherapy clinical trials (51–53).
We have previously shown that the tumor killing activity of γδ T cells can be increased by addition of the anti-CD3ε antibody UCHT1. However, UCHT1 is a potent T cell activating agent due to its intrinsic capability to induce cross-linking of TCRs and to stabilize the active CD3 conformation (27, 41). Thus, UCHT1 activates all T cells (αβ and γδ), regardless of their specificity, possibly resulting in an unspecific polyclonal T cell response and a potential life-threatening cytokine storm. These drawbacks profoundly limit the use of UCHT1 as a therapeutical agent to enhance tumor cell killing by cytotoxic γδ T cells that recognize tumor antigens by their γδ TCR. Here, we aimed to explore the use of UCHT1 Fab fragments as an alternative to enhance tumor killing by γδ T cells. UCHT1 Fab fragments do not activate αβ TCRs by themselves due to their monovalent binding (30, 38, 39) and here we show that this is also the case with the γδ TCR. Importantly, we demonstrate that UCHT1 Fab fragments significantly boosted tumor cell killing by γδ T cells, suggesting the use of UCHT1 Fab fragments as specific co-stimulation agents in γδ T cell immunotherapy approaches.
All three ligands that were previously tested for the γδ TCR (Daudi and phosphoantigens for the human Vγ9Vδ2 TCR and T22 tetramers for the murine G8 TCR) did not stabilize the active CD3 conformation, as defined by the exposure of the CD3ε PRS (41). In contrast, UCHT1 did stabilize the γδ TCR in its active conformation (41). In addition, UCHT1 binds simultaneously to two TCRs and thereby crosslinks γδ TCRs, leading to γδ TCR and T cell activation. Thus, by using UCHT1, it is not possible to distinguish whether cross-linking of TCRs or stabilization of the CD3 active conformation is the event enhancing tumor killing by γδ T cells. To answer this mechanistic question, we used here monovalent UCHT1 Fab fragments, which only have one binding site per molecule and, therefore, do not cross-link TCRs. Our data show that UCHT1 Fab fragments also enhanced the cytotoxic activity of human γδ T cells. Thus, a cross-linking activity is not required to boost γδ T cell cytotoxic activity. We next tested whether UCHT1 Fab fragments also stabilize the active γδ CD3 conformation and thereby, induce the recruitment of Nck to the CD3ε PRS. The treatment of the γδ T cells with the Fab fragments or with UCHT1 led to the recruitment of Nck to the γδ TCR. This induced recruitment was abrogated in the presence of the inhibitor AX-024, which blocks the interaction of the CD3ε PRS with SH3.1(Nck) (36). And indeed SH3.1(Nck) can bind to the CD3ε PRS in γδ TCRs [(41) and this study]. These data provide strong mechanistic evidence demonstrating that Nck binds to the γδ TCR via the CD3ε PRS upon stabilization of the active CD3 conformation, like in the αβ TCR (26, 33).
Human Vγ9Vδ2 γδ T cells modestly killed the B cell lymphoma lines Daudi and Raji. This killing was enhanced in the presence of UCHT1 Fab. Daudi and other tumor cells express high levels of phosphoantigens (14), which together with butyrophilin 3A1 most likely constitute (part of) the ligand for the Vγ9Vδ2 TCR (24, 25). Thus, the γδ TCR was likely to be bound to the natural phosphoantigen/butyrophilin 3A1 ligand in our experimental settings. This potential binding stimulated the γδ TCR, but without stabilizing the γδ TCR in its active CD3 conformation (41). Here, we show that, in addition to stimulation by the natural ligands, the γδ TCR was stabilized in its active conformation when we used the UCHT1 Fab fragments. This treatment not only enhance the tumor cell killing, but also the activation of γδ T cells as seen by augmented CD69, CD25, IFNγ, and TNFα expression. Enhanced up-regulation of CD69 and CD25 was also seen with the complete UCHT1 antibody ( (41) and this study). However, increased production of IFNγ and TNFα by UCHT1 was not seen earlier (41) and this could be due to differences in the cells used (expanded γδ T cells versus a γδ T cell clone). Our data thus support the idea that enforcing the γδ TCR to adopt the active CD3 conformation in the presence of its natural ligand might generate a quantitatively or/and qualitatively distinct set of activation signals that ultimately enhance γδ T cell activation.
One mechanism to promote such distinct signals might have been the recruitment of Nck to the γδ TCR. We found, however, that Nck recruitment was dispensable for the enhanced activation of the γδ T cells in the presence of the Fab fragments or the complete antibody. This suggests that a so far unknown effect of the active CD3 conformation in the γδ TCR increases γδ T cell activity. One possibility might be enhanced phosphorylation of the CD3 tails on tyrosines in analogy to the αβ TCR (27, 28), which might lead to a stronger T cell stimulation. Indeed, the kinase ZAP70 that binds to doubly phosphorylated CD3 subunits (54) and is recruited to the γδ TCR (55) was phosphorylated upon stimulation of γδ T cells with UCHT1. Since this phosphorylation was independent of Nck recruitment, it might be a downstream effect of the active CD3 conformation that we were looking for.
ZAP70 is important to trigger signaling cascades in αβ T cells, such as the Erk and calcium pathways (54), and these pathways are also triggered by stimulation of the Vγ9Vδ2 TCR by phosphoantigens or anti-CD3 antibodies (41, 55–59). Here, we show that calcium influx stimulated by the cognate ligand–γδ TCR interaction was increased upon stabilization of the active CD3 conformation. This is in line with our earlier finding that trapping the γδ TCR in the resting CD3 conformation by using CD3ε mutants [CD3εK76T and CD3εC80G (31, 32)] reduced γδ TCR calcium signaling (41). Similarly UCHT1 enhanced phosphorylation of Erk [(41) and this study], and this again was independent of Nck recruitment to the γδ TCR. Since the Erk pathway is involved in the antitumor activity of Vγ9Vδ2 T cells (56), an increase in phospho-Erk might explain the enhanced tumor killing when the active CD3 conformation was stabilized. In conclusion, stabilization of the active CD3 conformation in a ligand-triggered γδ TCR leads to enhanced downstream signaling.
Taken together, this study might help to design therapeutical agents, such as the Fab fragments of UCHT1, to specifically enhance tumor cell killing by γδ T cells while preventing unspecific activation of all T cells.
Informed consent for the performed studies was obtained from the donors in accordance with the Declaration of Helsinki and Institutional Review Board approval from the University of Freiburg Ethics Committee (412/9).
CJ performed the killing assays as well as the CD69 and CD25 up-regulation experiments. CJ and AM prepared and tested the Fab fragments and performed the signaling assays. CJ, KR, H-HO, DW, and AM expanded human γδ T cells. PW, CJ, and FH performed the PLA experiments and JH performed the ELISA. PF, SM, SP, and WS conceived the experiments. CJ, WS, PW, DW, PF, SM, and SP wrote or edited the manuscript.
The authors declare that the research was conducted in the absence of any commercial or financial relationships that could be construed as a potential conflict of interest.
We thank the BIOSS toolbox for preparing antibodies for this work and for running the robotic platform (INST 39/899-1 FUGG) to conduct the calcium equipments as well as Sylvia Kock for technical assistance. We thank Balbino Alarcon, Damia Tormo, and Andy Gagete from Artax Biopharma for providing the AX-024 inhibitor and Erin J. Adams and Andrew Sandstrom for the T22 tetramers. This work was funded by the Deutsche Forschungsgemeinschaft (DFG) through EXC294 (the Center for Biological Signaling Studies, BIOSS, WWS), GSC-4 (Spemann Graduate School, AM and KR), SFB1160 (PF, SM), SFB850 (SM), and MI1942/2-1 (SM). FAH was supported by the Elite programme of the Baden-Württemberg Stiftung to SM. JH was supported by a Ph.D. fellowship from the Deutscher Akademischer Austauschdienst (DAAD) and PW by the Royal Golden Jubilee Ph.D. programme of the Thailand Research Fund (TRF). WS was supported by the German Federal Ministry of Education and Research (BMBF 01EO1303). SP received research grants from Naresuan University (R2560B044) and the TRF (RSA5880009).
The Supplementary Material for this article can be found online at https://www.frontiersin.org/article/10.3389/fimmu.2018.01579/full#supplementary-material.
1. Groh V, Steinle A, Bauer S, Spies T. Recognition of stress-induced MHC molecules by intestinal epithelial γδ T cells. Science (1998) 279:1737–40. doi:10.1126/science.279.5357.1737
2. Uldrich AP, Le Nours J, Pellicci DG, Gherardin NA, McPherson KG, Lim RT, et al. CD1d-lipid antigen recognition by the γδ TCR. Nat Immunol (2013) 14:1137–45. doi:10.1038/ni.2713
3. Vantourout P, Hayday A. Six-of-the-best: unique contributions of γδ T cells to immunology. Nat Rev Immunol (2013) 13:88–100. doi:10.1038/nri3384
4. Chien Y, Konigshofer Y. Antigen recognition by γδ T cells. Immunol Rev (2007) 215:46–58. doi:10.1111/j.1600-065X.2006.00470.x
5. Bonneville M, O’Brien RL, Born WK. γδ T cell effector functions: a blend of innate programming and acquired plasticity. Nat Rev Immunol (2010) 10:467–78. doi:10.1038/nri2781
6. Zou C, Zhao P, Xiao Z, Han X, Fu F, Fu L. γδ T cells in cancer immunotherapy. Oncotarget (2017) 8:8900–9. doi:10.18632/oncotarget.13051
7. Chitadze G, Oberg HH, Wesch D, Kabelitz D. The ambiguous role of γδ T lymphocytes in antitumor immunity. Trends Immunol (2017) 38:668–78. doi:10.1016/j.it.2017.06.004
8. Bukowski JF, Morita CT, Band H, Brenner MB. Crucial role of TCR γ chain junctional region in prenyl pyrophosphate antigen recognition by γδ T cells. J Immunol (1998) 161:286–93.
9. Bukowski JF, Morita CT, Tanaka Y, Bloom BR, Brenner MB, Band H. Vγ2Vδ2 TCR-dependent recognition of non-peptide antigens and Daudi cells analyzed by TCR gene transfer. J Immunol (1995) 154:998–1006.
10. Constant P, Davodeau F, Peyrat MA, Poquet Y, Puzo G, Bonneville M, et al. Stimulation of human γδ T cells by nonpeptidic mycobacterial ligands. Science (1994) 264:267–70. doi:10.1126/science.8146660
11. Tanaka Y, Morita CT, Tanaka Y, Nieves E, Brenner MB, Bloom BR. Natural and synthetic non-peptide antigens recognized by human γδ T cells. Nature (1995) 375:155–8. doi:10.1038/375155a0
12. Espinosa E, Belmant C, Pont F, Luciani B, Poupot R, Romagne F, et al. Chemical synthesis and biological activity of bromohydrin pyrophosphate, a potent stimulator of human γδ T cells. J Biol Chem (2001) 276:18337–44. doi:10.1074/jbc.M100495200
13. Fisch P, Meuer E, Pende D, Rothenfusser S, Viale O, Kock S, et al. Control of B cell lymphoma recognition via natural killer inhibitory receptors implies a role for human Vγ9/Vδ2 T cells in tumor immunity. Eur J Immunol (1997) 27:3368–79. doi:10.1002/eji.1830271236
14. Gober HJ, Kistowska M, Angman L, Jenö P, Mori L, De Libero G. Human T cell receptor γδ cells recognize endogenous mevalonate metabolites in tumor cells. J Exp Med (2003) 197:163–8. doi:10.1084/jem.20021500
15. Silva-Santos B, Serre K, Norell H. γδ T cells in cancer. Nat Rev Immunol (2015) 15:683–91. doi:10.1038/nri3904
16. Roelofs AJ, Jauhiainen M, Monkkonen H, Rogers MJ, Monkkonen J, Thompson K. Peripheral blood monocytes are responsible for γδ T cell activation induced by zoledronic acid through accumulation of IPP/DMAPP. Br J Haematol (2009) 144:245–50. doi:10.1111/j.1365-2141.2008.07435.x
17. Benzaid I, Monkkonen H, Bonnelye E, Monkkonen J, Clezardin P. In vivo phosphoantigen levels in bisphosphonate-treated human breast tumors trigger Vgamma9Vdelta2 T-cell antitumor cytotoxicity through ICAM-1 engagement. Clin Cancer Res (2012) 18:6249–59. doi:10.1158/1078-0432.CCR-12-0918
18. Kabelitz D, Wesch D, Pitters E, Zoller M. Characterization of tumor reactivity of human Vγ9Vδ2 γδ T cells in vitro and in SCID mice in vivo. J Immunol (2004) 173:6767–76. doi:10.4049/jimmunol.173.11.6767
19. Dieli F, Vermijlen D, Fulfaro F, Caccamo N, Meraviglia S, Cicero G, et al. Targeting human γδ T cells with zoledronate and interleukin-2 for immunotherapy of hormone-refractory prostate cancer. Cancer Res (2007) 67:7450–7. doi:10.1158/0008-5472.CAN-07-0199
20. Meraviglia S, Eberl M, Vermijlen D, Todaro M, Buccheri S, Cicero G, et al. In vivo manipulation of Vγ9Vδ2 T cells with zoledronate and low-dose IL-2 for immunotherapy of advanced breast cancer patients. Clin Exp Immunol (2010) 161:290–7. doi:10.1111/j.1365-2249.2010.04167.x
21. Oberg HH, Grage-Griebenow E, dam-Klages SA, Jerg E, Peipp M, Kellner C, et al. Monitoring and functional characterization of the lymphocytic compartment in pancreatic ductal adenocarcinoma patients. Pancreatology (2016) 16:1069–79. doi:10.1016/j.pan.2016.07.008
22. Oberg HH, Kellner C, Gonnermann D, Peipp M, Peters C, Sebens S, et al. γδ T cell activation by bispecific antibodies. Cell Immunol (2015) 296:41–9. doi:10.1016/j.cellimm.2015.04.009
23. Oberg HH, Peipp M, Kellner C, Sebens S, Krause S, Petrick D, et al. Novel bispecific antibodies increase γδ T-cell cytotoxicity against pancreatic cancer cells. Cancer Res (2014) 74:1349–60. doi:10.1158/0008-5472.CAN-13-0675
24. Vavassori S, Kumar A, Wan GS, Ramanjaneyulu GS, Cavallari M, El Daker S, et al. Butyrophilin 3A1 binds phosphorylated antigens and stimulates human γδ T cells. Nat Immunol (2013) 14:908–16. doi:10.1038/ni.2665
25. Sandstrom A, Peigne CM, Leger A, Crooks JE, Konczak F, Gesnel MC, et al. The intracellular B30.2 domain of butyrophilin 3A1 binds phosphoantigens to mediate activation of human Vγ9Vδ2 T cells. Immunity (2014) 40:490–500. doi:10.1016/j.immuni.2014.03.003
26. Gil D, Schamel WW, Montoya M, Sanchez-Madrid F, Alarcon B. Recruitment of Nck by CD3 epsilon reveals a ligand-induced conformational change essential for T cell receptor signaling and synapse formation. Cell (2002) 109:901–12. doi:10.1016/S0092-8674(02)00799-7
27. Swamy M, Beck-Garcia K, Beck-Garcia E, Hartl FA, Morath A, Yousefi OS, et al. A cholesterol-based allostery model of T cell receptor phosphorylation. Immunity (2016) 44:1091–101. doi:10.1016/j.immuni.2016.04.011
28. Schamel WW, Alarcon B, Hofer T, Minguet S. The allostery model of TCR regulation. J Immunol (2017) 198:47–52. doi:10.4049/jimmunol.1601661
29. Risueno RM, Gil D, Fernandez E, Sanchez-Madrid F, Alarcon B. Ligand-induced conformational change in the T-cell receptor associated with productive immune synapses. Blood (2005) 106:601–8. doi:10.1182/blood-2004-12-4763
30. Minguet S, Swamy M, Alarcon B, Luescher IF, Schamel WW. Full activation of the T cell receptor requires both clustering and conformational changes at CD3. Immunity (2007) 26:43–54. doi:10.1016/j.immuni.2006.10.019
31. Martínez-Martín N, Risueño RM, Morreale A, Zaldívar I, Fernández-Arenas E, Herranz F, et al. Cooperativity between T cell receptor complexes revealed by conformational mutants of CD3epsilon. Sci Signal (2009) 2:ra43. doi:10.1126/scisignal.2000402
32. Blanco R, Borroto A, Schamel WW, Pereira P, Alarcon B. Conformational changes in the T cell receptor differentially determine T cell subset development in mice. Sci Signal (2014) 7:ra115. doi:10.1126/scisignal.2005650
33. Paensuwan P, Hartl FA, Yousefi OS, Ngoenkam J, Wipa P, Beck-Garcia E, et al. Nck binds to the T cell antigen receptor using its SH3.1 and SH2 domains in a cooperative manner, promoting TCR functioning. J Immunol (2016) 196:448–58. doi:10.4049/jimmunol.1500958
34. Borroto A, Arellano I, Dopfer EP, Prouza M, Suchanek M, Fuentes M, et al. Nck recruitment to the TCR required for ZAP70 activation during thymic development. J Immunol (2013) 190:1103–12. doi:10.4049/jimmunol.1202055
35. Borroto A, Arellano I, Blanco R, Fuentes M, Orfao A, Dopfer EP, et al. Relevance of Nck-CD3epsilon interaction for T cell activation in vivo. J Immunol (2014) 192:2042–53. doi:10.4049/jimmunol.1203414
36. Borroto A, Reyes-Garau D, Jimenez MA, Carrasco E, Moreno B, Martinez-Pasamar S, et al. First-in-class inhibitor of the T cell receptor for the treatment of autoimmune diseases. Sci Transl Med (2016) 8:370ra184. doi:10.1126/scitranslmed.aaf2140
37. Minguet S, Schamel WWA. A permissive geometry model for TCR-CD3 activation. Trends Biochem Sci (2008) 33:51–7. doi:10.1016/j.tibs.2007.10.008
38. Chang TW, Kung PC, Gingras SP, Goldstein G. Does OKT3 monoclonal antibody react with an antigen-recognition structure on human T cells? Proc Natl Acad Sci U S A (1981) 78:1805–8. doi:10.1073/pnas.78.3.1805
39. Kaye J, Janeway CA Jr. The Fab fragment of a directly activating monoclonal antibody that precipitates a disulfide-linked heterodimer from a helper T cell clone blocks activation by either allogeneic Ia or antigen and self-Ia. J Exp Med (1984) 159:1397–412. doi:10.1084/jem.159.5.1397
40. Cordoba SP, Choudhuri K, Zhang H, Bridge M, Basat AB, Dustin ML, et al. The large ectodomains of CD45 and CD148 regulate their segregation from and inhibition of ligated T-cell receptor. Blood (2013) 121:4295–302. doi:10.1182/blood-2012-07-442251
41. Dopfer EP, Hartl FA, Oberg HH, Siegers GM, Yousefi OS, Kock S, et al. The CD3 conformational change in the γδ T cell receptor is not triggered by antigens but can be enforced to enhance tumor killing. Cell Rep (2014) 7:1704–15. doi:10.1016/j.celrep.2014.04.049
42. Soderberg O, Gullberg M, Jarvius M, Ridderstrale K, Leuchowius KJ, Jarvius J, et al. Direct observation of individual endogenous protein complexes in situ by proximity ligation. Nat Methods (2006) 3:995–1000. doi:10.1038/nmeth947
43. Alibaud L, Arnaud J, Llobera R, Rubin B. On the role of CD3δ chains in TCRγδ/CD3 complexes during assembly and membrane expression. Scand J Immunol (2001) 54:155–62. doi:10.1046/j.1365-3083.2001.00938.x
44. Siegers GM, Swamy M, Fernandez-Malave E, Minguet S, Rathmann S, Guardo AC, et al. Different composition of the human and the mouse γδ T cell receptor explains different phenotypes of CD3γ and CD3δ-immunodeficiencies. J Exp Med (2007) 204:2537–44. doi:10.1084/jem.20070782102207c
45. Siegers GM, Ribot EJ, Keating A, Foster PJ. Extensive expansion of primary human γδ T cells generates cytotoxic effector memory cells that can be labeled with Feraheme for cellular MRI. Cancer Immunol Immunother (2012) 62:571–83. doi:10.1007/s00262-012-1353-y
46. Wesch D, Marx S, Kabelitz D. Comparative analysis of alpha beta and γδ T cell activation by Mycobacterium tuberculosis and isopentenyl pyrophosphate. Eur J Immunol (1997) 27:952–6. doi:10.1002/eji.1830270422
47. Kondo M, Sakuta K, Noguchi A, Ariyoshi N, Sato K, Sato S, et al. Zoledronate facilitates large-scale ex vivo expansion of functional γδ T cells from cancer patients for use in adoptive immunotherapy. Cytotherapy (2008) 10:842–56. doi:10.1080/14653240802419328
48. Crowley MP, Fahrer AM, Baumgarth N, Hampl J, Gutgemann I, Teyton L, et al. A population of murine γδ T cells that recognize an inducible MHC class Ib molecule. Science (2000) 287:314–6. doi:10.1126/science.287.5451.314
49. Adams EJ, Chien YH, Garcia KC. Structure of a γδ T cell receptor in complex with the nonclassical MHC T22. Science (2005) 308:227–31. doi:10.1126/science.1106885
50. Gentles AJ, Newman AM, Liu CL, Bratman SV, Feng W, Kim D, et al. The prognostic landscape of genes and infiltrating immune cells across human cancers. Nat Med (2015) 21:938–45. doi:10.1038/nm.3909
51. Deniger DC, Moyes JS, Cooper LJ. Clinical applications of γδ T cells with multivalent immunity. Front Immunol (2014) 5:636. doi:10.3389/fimmu.2014.00636
52. Fournie JJ, Sicard H, Poupot M, Bezombes C, Blanc A, Romagne F, et al. What lessons can be learned from γδ T cell-based cancer immunotherapy trials? Cell Mol Immunol (2013) 10:35–41. doi:10.1038/cmi.2012.39
53. Paul S, Lal G. Regulatory and effector functions of γδ T cells and their therapeutic potential in adoptive cellular therapy for cancer. Int J Cancer (2016) 139:976–85. doi:10.1002/ijc.30109
54. Courtney AH, Lo WL, Weiss A. TCR signaling: mechanisms of initiation and propagation. Trends Biochem Sci (2018) 43:108–23. doi:10.1016/j.tibs.2017.11.008
55. Lafont V, Liautard J, Sable-Techene M, Sainte-Marie Y, Favero J. Isopentenyl pyrophosphate, a mycobacterial non-peptidic antigen, triggers delayed and highly sustained signaling in human γδ T lymphocytes without inducing down-modulation of T cell antigen receptor. J Biol Chem (2001) 276:15961–7. doi:10.1074/jbc.M008684200
56. Correia DV, d’Orey F, Cardoso BA, Lanca T, Grosso AR, deBarros A, et al. Highly active microbial phosphoantigen induces rapid yet sustained MEK/Erk- and PI-3K/Akt-mediated signal transduction in anti-tumor human γδ T-cells. PLoS One (2009) 4:e5657. doi:10.1371/journal.pone.0005657
57. Nedellec S, Sabourin C, Bonneville M, Scotet E. NKG2D costimulates human V gamma 9V delta 2 T cell antitumor cytotoxicity through protein kinase C theta-dependent modulation of early TCR-induced calcium and transduction signals. J Immunol (2010) 185:55–63. doi:10.4049/jimmunol.1000373
58. Thedrez A, Sabourin C, Gertner J, Devilder MC, Allain-Maillet S, Fournie JJ, et al. Self/non-self discrimination by human γδ T cells: simple solutions for a complex issue? Immunol Rev (2007) 215:123–35. doi:10.1111/j.1600-065X.2006.00468.x
Keywords: γδ T cells, T cell antigen receptor, tumor, Nck, activation, signaling, Fab fragments, AX-024
Citation: Juraske C, Wipa P, Morath A, Hidalgo JV, Hartl FA, Raute K, Oberg H-H, Wesch D, Fisch P, Minguet S, Pongcharoen S and Schamel WW (2018) Anti-CD3 Fab Fragments Enhance Tumor Killing by Human γδ T Cells Independent of Nck Recruitment to the γδ T Cell Antigen Receptor. Front. Immunol. 9:1579. doi: 10.3389/fimmu.2018.01579
Received: 13 January 2018; Accepted: 26 June 2018;
Published: 09 July 2018
Edited by:
Daniel J. Pennington, Queen Mary University of London, United KingdomReviewed by:
Christopher E. Rudd, Université de Montréal, CanadaCopyright: © 2018 Juraske, Wipa, Morath, Hidalgo, Hartl, Raute, Oberg, Wesch, Fisch, Minguet, Pongcharoen and Schamel. This is an open-access article distributed under the terms of the Creative Commons Attribution License (CC BY). The use, distribution or reproduction in other forums is permitted, provided the original author(s) and the copyright owner(s) are credited and that the original publication in this journal is cited, in accordance with accepted academic practice. No use, distribution or reproduction is permitted which does not comply with these terms.
*Correspondence: Wolfgang W. Schamel, d29sZmdhbmcuc2NoYW1lbEBiaW9sb2dpZS51bmktZnJlaWJ1cmcuZGU=
Disclaimer: All claims expressed in this article are solely those of the authors and do not necessarily represent those of their affiliated organizations, or those of the publisher, the editors and the reviewers. Any product that may be evaluated in this article or claim that may be made by its manufacturer is not guaranteed or endorsed by the publisher.
Research integrity at Frontiers
Learn more about the work of our research integrity team to safeguard the quality of each article we publish.