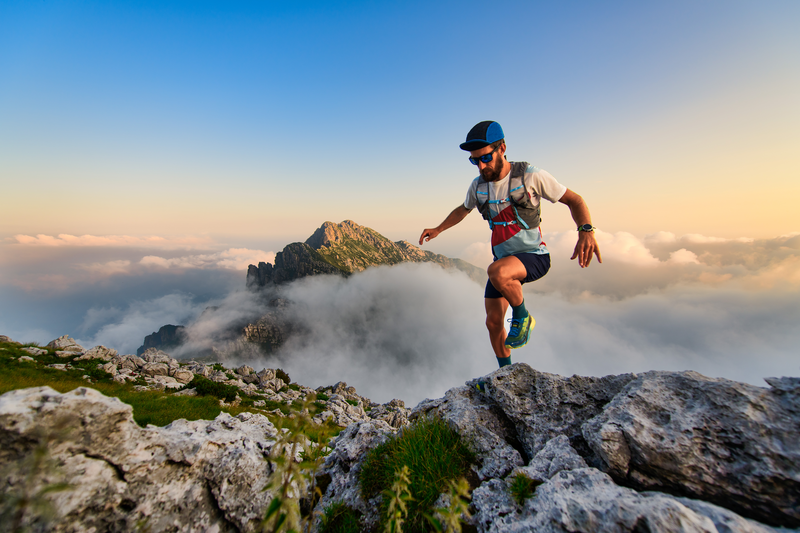
94% of researchers rate our articles as excellent or good
Learn more about the work of our research integrity team to safeguard the quality of each article we publish.
Find out more
ORIGINAL RESEARCH article
Front. Immunol. , 22 June 2018
Sec. Inflammation
Volume 9 - 2018 | https://doi.org/10.3389/fimmu.2018.01445
IL-1 family regulatory cytokine IL-37b can suppress innate immunity and inflammatory activity in inflammatory diseases. In this study, IL-37b showed remarkable in vitro suppression of inflammatory tumor necrosis factor-α, IL-1β, IL-6, CCL2, and CXCL8 production in the coculture of human primary eosinophils and human bronchial epithelial BEAS-2B cells with the stimulation of bacterial toll-like receptor-2 ligand peptidoglycan, while antagonizing the activation of intracellular nuclear factor-κB, PI3K–Akt, extracellular signal-regulated kinase 1/2, and suppressing the gene transcription of allergic inflammation-related PYCARD, S100A9, and CAMP as demonstrated by flow cytometry, RNA-sequencing, and bioinformatics. Results therefore elucidated the novel anti-inflammation-related molecular mechanisms mediated by IL-37b. Using the house dust mite (HDM)-induced humanized asthmatic NOD/SCID mice for preclinical study, intravenous administration of IL-37b restored the normal plasma levels of eosinophil activators CCL11 and IL-5, suppressed the elevated concentrations of Th2 and asthma-related cytokines IL-4, IL-6, and IL-13 and inflammatory IL-17, CCL5, and CCL11 in lung homogenate of asthmatic mice. Histopathological results of lung tissue illustrated that IL-37b could mitigate the enhanced mucus, eosinophil infiltration, thickened airway wall, and goblet cells. Together with similar findings using the ovalbumin- and HDM-induced allergic asthmatic mice further validated the therapeutic potential of IL-37b in allergic asthma. The above results illustrate the novel IL-37-mediated regulation of intracellular inflammation mechanism linking bacterial infection and the activation of human eosinophils and confirm the in vivo anti-inflammatory activity of IL-37b on human allergic asthma.
According to the Global Burden of Diseases Study 2015, it was estimated that about 358 million people worldwide were suffering from asthma, with 0.40 million deaths in 2015 (1). The prevalence of asthma has continued to rise, with a higher annual incidence in children than in adults. Asthma can be classified into allergic asthma and non-allergic asthma. Most children and half of adult patients suffer from allergic asthma which is distinguished from non-allergic asthma, due to the presence of elevated serum immunoglobulin E (IgE, total and specific) and provocation by inhaled or ingested allergens such as pollen, dust mite, mold, animal dander, and peanut (2, 3).
Among one of the most prevalent chronic respiratory diseases of the conducting airways, allergic asthma is clinically characterized by reversible bronchial constriction and airway hyper-responsiveness (AHR), often associated with elevated plasma IgE concentration. Increased differentiated CD4+ T helper type (Th)2 lymphocytes originated from bronchial tissues and draining lymph nodes secrete allergy-related Th2 pro-inflammatory cytokines such as IL-4, IL-5, and IL-13, thereby contributing to the pathogenesis and exacerbation of allergic asthma (4–6). It has been shown that respiratory bacterial and viral infection can provoke allergic inflammation in allergic asthma (7, 8). The innate immune system actually recognizes infection through pattern-recognition receptors, including toll-like receptors (TLRs), NOD-like receptors, and RIG-I-like receptor, which detect conserved microbial components called pathogen-associated molecular patterns (PAMPs) including TLR2 ligand peptidoglycan (PGN) (9).
Eosinophils are crucial effector cells of allergic inflammation that accumulate and infiltrate in local inflammatory tissues upon the mediation of the specific eosinophil chemokine CCL11, and intercellular adhesion molecule-1 on epithelial cells (10). Allergen-reactive Th2 lymphocytes are important for the induction and maintenance of allergic inflammation (11). Cytokines and chemokines released by Th2 cells (IL-4, IL-5, IL-6, IL-9, IL-10, and IL-13) and those induced by other cell types in response to Th2 cytokines (CCL11, transforming growth factor/TGF-β, and IL-11) mediate most of the pathophysiological mechanisms in allergic reaction such as the recruitment, activation, and delayed apoptosis of eosinophils, and degranulation to release granular toxic proteins from eosinophils (11). In allergic airway inflammation, activated eosinophils release cytotoxic cationic proteins such as eosinophilic cationic protein (ECP) to cause tissue damage and airway hyper-reactivity (12). Our previous publications have elucidated the underlying intracellular signaling mechanisms by which the interaction of eosinophils and epithelial cells can induce the secretion of inflammatory cytokines, cysteinyl leukotrienes, and eosinophilic degranulation for ECP release in allergic asthma (13–16).
Regulatory cytokine IL-37, the seventh interleukin factor of IL-1 family (IL-1F7), can downregulate systemic and local inflammation by suppressing the production of pro-inflammatory mediators in both innate and adaptive immunity (17). IL-37 can be expressed in various human tissues such as skin, tonsils, esophagus, placenta, breast, prostate, and colon (18), and induced by TLR ligands and inflammatory cytokines IL-1β, tumor necrosis factor (TNF)-α, and interferon-γ in peripheral blood mononuclear cells (PBMCs) and dendritic cells (DCs) (19). IL-37 can suppress the production of various pro-inflammatory cytokines, including IL-1α, IL-1β, IL-6, IL-12, granulocyte colony-stimulating factor, granulocyte-macrophage colony-stimulating factor, and TNF-α, probably by forming an intracellular functional complex with transcription factorSmad-3 to regulate relevant gene transcription (17). Moreover, IL-37 can be expressed by regulatory T (Treg) cells to enhance the expression of anti-inflammatory TGF-β and IL-10, Foxp3, and cytotoxic T-lymphocyte associated antigen-4 to promote the immunosuppressive activity of human Treg cells (20). In addition, extracellular IL-37 can bind to IL-18-binding protein, IL-18Rα, and β-chain, thereby inhibiting the pro-inflammatory activity of IL-18 (17, 21). Apart from being a natural inhibitor of innate immunity, IL-37 can inhibit DC activation to regulate adaptive immunity (17, 22, 23). IL-37 binds to the IL-18 receptor but recruits the orphan IL-1R8 to act as an inhibitor to exhibit its multifaceted anti-inflammatory activity, via the regulation of cellular adhesion and migration, and intracellular mechanism in murine splenic lymphocytes (17, 24). IL-37 can also ameliorate experimental asthma by Th2 suppression that is independent of IL-18 signaling (25). Although no mouse homolog has yet been found, human IL-37 has been reported to be an in vivo suppressor of inflammation in mice (17). Using murine experiments, IL-37 has been shown to play immunoregulatory roles in myocardial infarction, rheumatoid arthritis (RA), diabetes, allergic asthma, and fungal infections (26–30). Clinical studies have demonstrated the downregulated production of IL-37 of human PBMC in allergic asthmatics and decreased IL-37 level in induced sputum with negative correlation with disease severity of asthma (29, 31). The ex vivo lipopolysaccharide (LPS)-induced release of pro-inflammatory cytokines including TNF-α, IL-6, and IL-1β from asthma sputum cells was abrogated by IL-37. Therefore, IL-37 is important in modulating asthma by suppressing production of pro-inflammatory cytokines (31).
Together, the expression and activities of IL-37 are important in the maintenance of immune homeostasis. It is therefore hypothesized that IL-37 is a natural inhibitor of allergic airway inflammation via suppressing eosinophil activation. In an attempt to further evaluate the anti-inflammatory mechanism of IL-37 in allergic asthma, in vitro intracellular signaling mechanisms of TLR2-activated human eosinophils and the in vivo immunoregulatory activities of IL-37 in house dust mite (HDM)-humanized allergic asthmatic mice, ovalbumin (OVA)/HDM-induced allergic asthmatic mice have been investigated. Among five different IL-37 splice variants, IL-37b is the largest and best characterized variant (32). This study was therefore performed using recombinant human IL-37b.
Recombinant human IL-37b protein was purchased from R&D Systems, Inc., Minneapolis, MN, USA. PGN was bought from Invivogen Inc., San Diego, CA, USA. Albumin from chicken egg white (OVA) was purchased from Sigma-Aldrich Corp., St. Louis, MO, USA. HDM (Dermatophagoides pteronyssinus) was obtained from Greerlabs Inc., Lenoir, NC, USA.
Fresh human buffy coat was obtained from healthy volunteers of Hong Kong Red Cross Blood Transfusion Service for the purification of primary human eosinophils. The experimental procedure using human eosinophils purified from buffy coats was approved by Clinical Research Ethics Committee, The Chinese University of Hong Kong-New Territories East Cluster Hospitals, according to the 1964 Declaration of Helsinki and its later amendments and informed written consent was obtained from all subjects.
Inbred male BALB/c mice (6–8 weeks old) and female non-obese diabetic/severe combined immunodeficiency (NOD/SCID) (5–6 weeks old) were bred under specific pathogen-free conditions and kept at Laboratory Animal Services Center, The Chinese University of Hong Kong. All animal experimentations were performed in accordance with the principles outlined in the Animal Experimentation Ethics Committee (AEEC) guide for the Care and Use of Laboratory Animals, with the approval of the AEEC of The Chinese University of Hong Kong.
Allergic asthmatic patients aged 30–40 years with HDM sensitization (n = 4) and sex- and age-matched non-atopic healthy control subjects (n = 4) were recruited from The Prince of Wales Hospital, Hong Kong. EDTA anticoagulated blood (50 ml) obtained from each subject was diluted (1:1 v/v) with cold phosphate-buffered saline (PBS) and PBMC were then harvested by using Ficoll density gradient centrifugation (GE Healthcare Life Sciences, Piscataway, NJ, USA). Purified PBMC (2 × 107) were used for intraperitoneal injection (i.p.) into SCID mouse to construct humanized mice. All recruited subjects were ethnic Chinese. The above clinical protocol were approved by the Clinical Research Ethics Committee, The Chinese University of Hong Kong-New Territories East Cluster Hospitals, and informed written consent was signed by all subjects or their parents in accordance with the 1964 Declaration of Helsinki and its later amendments.
Phosphate-buffered saline diluted fresh human blood buffy coat was centrifuged using the 1.082 g/ml isotonic Percoll solution (GE Healthcare Life Sciences) for 20 min at 900 g. After RBC lysis, the obtained granulocyte fraction was collected to isolate eosinophils by anti-CD16 antibody-coated magnetic beads (Miltenyi Biotec, Bergisch Gladbach, Germany), followed by the depletion of CD16-positive cells by loading the cells onto a LS + column (Miltenyi Biotec) within a magnetic field. The drop-through fraction containing eosinophils with purity of at least 99% assessed by Hemacolor rapid blood smear stain (E Merck Diagnostica, Darmstadt, Germany) were collected (33).
The human bronchial epithelial cell line BEAS-2B cells (American Type Culture Collections, Manassas, VA, USA) were maintained in LHC-8 medium (Thermo Fisher Scientific, Rockford, IL, USA). For the experiment of coculture, the medium was changed to RPMI 1640 supplemented with 10% fetal bovine serum (FBS, Gibco Invitrogen Corp., Carlsbad, CA, USA). Eosinophils (3 × 105) and BEAS-2B cells (1 × 105) were cocultured with or without IL-37b (100 or 200 ng/ml) pre-treatment for 10 min, followed by PGN (10 µg/ml) stimulation for further 20 h.
Cocultured cells were pre-treated with IL-37b (200 ng/ml) for 10 min and stimulated with TLR2 ligand PGN (10 µg/ml) for 20 min. BEAS-2B cells and eosinophils were collected and fixed with fixation buffer (BioLegend Inc., San Diego, CA, USA) for 30 min at room temperature, followed with Intracellular Staining Permeabilization Wash Buffer (BD Biosciences, San Jose, CA, USA) at 4°C for 30 min. Cells were then stained with fluorescence dye-conjugated antibodies of mouse anti-human phosphorylated IκBα, Akt, extracellular signal-regulated kinase (ERK)1/2, or corresponding isotypic control antibody (BD Biosciences). After washing, cells were analyzed with FACSCalibur flow cytometer (BD Biosciences).
RNA isolation, library preparation for transcriptome sequencing and clustering and sequencing were performed by Novogene Co., Ltd., Beijing, China. Briefly, BEAS-2B cells and eosinophils were cocultured with different treatments, including IL-37b (200 ng/ml), PGN (10 µg/ml), PGN (10 µg/ml) plus IL-37b (200 ng/ml), with untreated cocultured cells as normal control, for 20 h before harvest. Total RNA was extracted from cocultured eosinophils using QIAzol reagent (Qiagen, Valencia, CA, USA). RNA (1 µg per sample) was applied as starting material for the RNA sample preparation. Sequencing libraries were created using NEBNext® Ultra™ RNA Library Prep Kit for Illumina® (NEB, USA) and index codes were used to attribute sequence to each sample. The clustering of the index-coded samples was analyzed on a cBot Cluster Generation System with TruSeq PE Cluster Kit v3-cBot-HS (Illumina, Inc., San Diego, CA, USA). After cluster generation, the library preparations were sequenced on an Illumina Hiseq 4000 platform to generate 150 bp paired-end reads. By clearing low quality reads from raw data, clean data/reads were obtained and were subsequently mapped to human genome assembly by HISAT (version 2.0.4). Fragments per kilobase of transcript per million mapped reads (FPKM) were calculated using HTSeq (version 0.6.1) to analyze gene expression levels. Differential expression analysis of four treatments was performed by the DESeq (version 1.10.1, Bioconductor). Genes with P < 0.05 were stated as differentially expressed. Gene ontology (GO) enrichment was assessed by DAVID Bioinformatics Resources 6.8 (National Institute of Allergy and Infectious Diseases, NIH). GO terms with Benjamini–Hochberg corrected P < 0.05 were defined to be significantly enriched by differentially expressed genes (DEG). RNA sequence data have been deposited at NCBI Sequence Read Archive under SRP study accession number SRP138008.
Total RNA was reverse transcribed into first-strand complementary DNA by using PrimeScript™ RT Master Mix (Takara Bio Inc., Shiga, Japan). The expression of gene TNF-α, IL-1β, IL-6, CCL2, and CXCL8 in cocultured eosinophils and BEAS-2B cells, as well as nine target genes screened from RNA-seq result were quantified using SYBR® Premix Ex Taq™ (Takara Bio Inc., Shiga, Japan), with corresponding primers (Table S1 in Supplementary Material). The GAPDH housekeeping gene was used as an internal reference and 2−ΔCt (Ct, target gene-Ct, GAPDH) was used to calculate the relative gene expression.
Humanized asthmatic NOD/SCID (non-obese diabetic/severe combined immunodeficiency) mice were developed as our previously described (34). PBMCs (2 × 107) from allergic asthmatic patients with HDM sensitization or sex- and age-matched healthy control subjects were i.p. transplanted into NOD/SCID mice on day 1. HDM (50 µg) was intratracheally instilled on days 2, 4, and 8. Recombinant human IL-37b (1 µg) or 200 μl PBS was i.v. injected on days 1, 2, 4, 8, 12, 16, and 19. The humanized SCID mice were sacrificed for analysis on day 20.
The concentrations of human CCL2 and CXCL8 in culture supernatant were analyzed using ELISA kit (BioLegend). Human TNF-α, IL-1β, and IL-6 and murine IL-4, IL-5, IL-6, IL-13, IL-17, CCL5, and CCL11 in plasma or lung homogenate were quantified with Human or Mouse Cytokine Milliplex MAP assay kit (Millipore Corporation, Billerica MA, USA) on Bio-Plex 200 system (Bio-Rad Laboratories, Hercules, CA, USA).
Hematoxylin and eosin (H&E) staining and periodic acid-Schiff (PAS) staining were performed as previously reported (35). Briefly, lung tissues were obtained, fixed with 4% paraformaldehyde, and embedded in paraffin. Sections (5 µm) were stained with H&E staining kit (Beyotime Inc., Jiangsu, China) for assessing the general morphology and inflammatory cells infiltration. For the detection of goblet cells in the bronchia, the lung sections were stained with PAS staining kit (Sigma-Aldrich) followed with hematoxylin staining (Beyotime). After H&E and PAS staining, lung sections were dehydrated, mounted, and examined under Leica DM6000 B microscope (Leica Microsystems Inc., Buffalo Grove, IL, USA).
All data were analyzed on Statistical Package for the Social Sciences statistical software for Mac OS, version 22. Differences between groups were evaluated by one-way ANOVA. Data were presented as mean ± SD. Differences with P < 0.05 were considered statistically significant.
For the in vitro study of suppressing allergic inflammation, eosinophils and BEAS-2B cells were cocultured to mimic the microenvironment in the asthmatic lung tissue in which bronchial epithelial cells interact with eosinophils (36). Since IL-18Rα and IL-1R8 are receptors for mediating the anti-inflammatory activities of IL-37b (24, 29), both IL-18Rα and IL-1R8 were detected on the surface of eosinophils and BEAS-2B cells by flow cytometry (Figures S1A,B in Supplementary Material). MTT assay and 7-AAD viability staining assay were performed on BEAS-2B cells and eosinophils, respectively, to delineate the non-cytotoxic dosage range of IL-37b for the subsequent in vitro study. Both assays demonstrated that IL-37b (10–1,000 ng/ml) did not produce any remarkable effect (P > 0.05) on both cell proliferation and viability (Figures S2A,B in Supplementary Material).
Our previous studies have shown the production of cytokines and chemokines in the coculture of eosinophils and BEAS-2B cells significantly increased when compared with culture of eosinophils or BEAS-2B cells alone, and their expression could be further upregulated upon the stimulation by NOD2 ligand MDP (13, 37, 38). In this in vitro study, bacterial TLR2 ligand PGN was used as the stimulus because TLR2 recognizes the bacterial cell wall components to initiate the subsequent innate immune response and antigen-specific Th2 immunity in allergic asthma (39, 40). As shown in Figure 1, PGN (10 µg/ml) significantly increased inflammatory cytokines TNF-α, IL-1β, and IL-6 production and chemokines CCL2 and CXCL-8 release in eosinophils or BEAS-2B cells alone as well as coculture, except for nearly undetectable TNF-α and IL-1β production in BEAS-2Bs alone. The PGN-induced TNF-α release in eosinophils alone and eosinophils-BEAS-2B cells coculture were downregulated by IL-37b (100 and 200 ng/ml, all P < 0.05, Figure 1A). Moreover, the PGN-induced IL-1β release from eosinophils alone but not eosinophils-BEAS-2B cells coculture could also be significantly suppressed by IL-37b (P < 0.05, Figure 1B). The in vitro upregulated production of inflammatory cytokine IL-6 and chemokines CCL2 and CXCL-8 could also be notably suppressed by IL-37b (200 ng/ml, all P < 0.05, Figures 1C–E). The gene expression of corresponding cytokines and chemokines showed similar results with protein levels (Figures 1F,G).
Figure 1. Effect of IL-37b on cytokine/chemokine release in human eosinophil-BEAS-2B coculture upon stimulation by TLR2 ligand PGN. Human eosinophils (3 × 105) and BEAS-2B cells (1 × 105) were cultured either together or separately with or without IL-37b (100 and 200 ng/ml) pre-treatment for 10 min, followed by stimulation with PGN (10 µg/ml) for 20 h. Release of TNF-α (A), IL-1β (B), IL-6 (C), CCL2 (D), and CXCL8 (E) were determined. The mRNA expression of gene TNF-α, IL-1β, IL-6, CCL2, and CXCL8 in cocultured eosinophils (F) and BEAS-2B (G) were analyzed by qPCR. Abbreviations: EOS, eosinophils; TLR2, toll-like receptor-2; PGN, peptidoglycan; TNF, tumor necrosis factor. Results are shown as mean ± SD of triplicate experiments. *P < 0.05, **P < 0.01, and ***P < 0.001 when compared between the denoted groups.
We have previously shown that bacterial PGN and TLR2 ligation could activate eosinophils by eliciting the activation of well-characterized downstream signaling mechanisms including NF-κB,PI3K–Akt, and ERK1/2 pathway, thereby accounting for the production of the inflammatory cytokines and chemokines within 30 min (41). The expressions of signaling molecules were analyzed by flow cytometry. The mixed eosinophils and BEAS-2B cells could be distinguished and analyzed separately by forward scatter and side scatter (Figure 2A). Figure 2 illustrates the underlying intracellular mechanism of immunosuppressive IL-37b in which upregulated levels of phosphorylated IκBα, Akt, and ERK1/2 in response to PGN were all markedly suppressed by IL-37b in both BEAS-2Bs and eosinophils in coculture (all P < 0.05, Figures 2B–E).
Figure 2. Inhibition of PGN-induced IκBα, PI3K–Akt, and ERK1/2 activation in eosinophil-BEAS-2B coculture under IL-37b treatment. Human eosinophils (1.2 × 106) and BEAS-2B cells (4 × 105) were cocultured with or without IL-37b (200 ng/ml) pre-treatment for 10 min followed by stimulation with PGN (10 µg/ml) for 20 min prior to fixation and permeabilization. (A) Eosinophils and BEAS-2B cells could be distinguished through FSC and SSC by flow cytometry. (B) Representative flow cytometric histograms from triplicate experiments of phosphorylated signaling molecules in cocultured eosinophils and BEAS-2B cells. Intracellular levels of phosphorylated IκBα (C), phosphorylated Akt (D), and phosphorylated ERK1/2 (E) in cells were measured by intracellular staining with specific antibodies and analyzed using flow cytometry. Results are expressed as fold of change (%) comparing with corresponding control groups and shown as mean ± SD, n = 3–6 per group. *P < 0.05, **P < 0.01, and ***P < 0.001 when compared between the denoted groups. Abbreviations: FSC, forward scatter; SSC, side scatter; EOS, eosinophils; coEOS, eosinophils in coculture; coBEAS-2B, BEAS-2B cells in coculture; ERK, extracellular signal-regulated kinase; PGN, peptidoglycan.
RNA-seq data were collected from the analysis of eosinophils cocultured with BEAS-2B cells after different treatments. The suspended cocultured eosinophils were separated from adhesive BEAS-2B cells. According to hemacolor rapid blood smear staining, the purity of eosinophils was more than 99% (Figure S3 in Supplementary Material). DEG were screened from IL-37b treated PGN sample versus untreated PGN sample. Heatmap analysis is shown in Figure 3A. According to the criteria of log2 (fold-change) < 0 and P < 0.05, 25 downregulated DEG were identified. Excluding pseudogenes and uncategorized genes, the functions of protein coding genes BOLA2B, CAMP, DPM3, ELOB, C4ORF48, S100A9, TFF3, NPIPB15, and PYCARD were annotated (Table 1). GO enrichment analysis was performed for the genes mentioned above. Only extracellular region, a cellular component GO term, was significantly overrepresented with Benjamini–Hochberg corrected P < 0.05. However, we found that all other GO terms with P < 0.05 belonged to biological process GO term, and were mainly related to innate immune response, cytokines secretion, and signal transcription activation which may give an elucidation of the mechanism of IL-37b on eosinophils (Figure 3B; Table 2). Heatmap of the downregulated DEG was constructed using log2 (fpkm fold-change) to present gene expression level. As shown in Figure 3C, the upregulated expression of target genes by PGN was suppressed upon IL-37b treatment. Target genes were further validated by real-time qPCR in three biological replicates and the result was coincident with RNA-seq (Figure 3D). Also, the expression differences of BOLA2B, CAMP, S100A9, and PYCARD between PGN and PGN + IL-37b were statistically significant (Figure 3D). Upregulated DEG of IL-37b treated PGN sample versus untreated PGN sample were sorted out with the criteria of log2 (fold-change) > 0 and P < 0.05, and then proceeded to GO enrichment analysis. In total, 408 genes were categorized into 171 functional groups, in which 9 groups were significantly overrepresented with Benjamini–Hochberg corrected (P < 0.05) and mainly belonged to molecular function and cellular component GO terms. However, no inflammation or immune-related terms were found in upregulated DEG (Figure 4).
Figure 3. Identification of IL-37b target genes through transcriptional profiling. Human eosinophils and BEAS-2B cells were cocultured with or without IL-37b (200 ng/ml) pre-treatment for 10 min followed by stimulation with PGN (10 µg/ml) for 20 h. Total RNA of eosinophils was extracted and transcriptome analysis was performed to identify IL-37b target genes. (A) Heatmap of DEG between IL-37b-treated PGN sample and untreated PGN sample is shown. (B) Gene ontology (GO) term enrichment analysis of downregulated DEG, *P < 0.05 indicates significantly enriched GO term after Benjamini–Hochberg correction. (C) Fold of change for downregulated DE genes was depicted on a log2 scale heatmap, with a yellow-to-red gradient indicating downregulation to upregulation. (D) Real-time qPCR validation of RNA-seq result. The GAPDH housekeeping gene was used as the internal reference and the relative gene expression was calculated using 2−ΔCt (Ct, target gene-Ct, GAPDH). Results are shown as mean ± SD, n = 3 per group, *P < 0.05 when compared between the denoted groups. Abbreviations: DEG, differentially expressed genes; PGN, peptidoglycan.
Table 1. The summary of downregulated DE genes for IL-37b-treated peptidoglycan (PGN) sample versus untreated PGN sample.
Figure 4. GO term enrichment analysis of upregulated DEG. Upregulated DEG for IL-37b treated PGN sample versus untreated PGN sample were sorted out with the criteria of log2 (fold-change) > 0 and P < 0.05, and then proceeded to GO enrichment analysis. Total 408 genes were categorized into 171 functional groups, in which 9 groups were significantly overrepresented with Benjamini–Hochberg corrected P < 0.05. Abbreviations: DEG, differentially expressed gene; GO, gene ontology; PGN, peptidoglycan.
Humanized allergic asthmatic NOD/SCID mice were applied as preclinical murine model to further evaluate the therapeutic potential of IL-37b for human allergic asthma (Figure 5A). Human CD45+ cells were assessed just before termination to ensure human PBMCs existed stably in the blood of human PBMC NOD/SCID mice (Figure 5B).
Figure 5. In vivo effects of human recombinant IL-37b on the development of asthmatic features in humanized allergic asthmatic mice. (A) Timeline protocol of asthmatic human-PBMC NOD/SCID mice and IL-37b administration. (B) Human CD45+ cells were assessed just before termination on day 19 to ensure human PBMCs existed stably in the blood of human-PBMC NOD/SCID mice. The concentrations of (C) IL-5 and CCL11 in plasma, and (D) protein expression of IL-4, IL-6, IL-13, IL-17, CCL5, and CCL11 in lung homogenate were analyzed. (E) Representative H&E and PAS staining of lung sections (200× magnification) with essentially similar results are shown. The black, red, green, and blue arrows denote increased mucus, eosinophils infiltration, thickened airway wall, and goblet cells, respectively. Results are shown as mean ± SD, n = 4 per group. *P < 0.05, **P < 0.01, and ***P < 0.001 when compared between the denoted groups. Data are representative from two independent experiments with essentially similar results. Abbreviations: PBMCs, peripheral blood mononuclear cells; NOD/SCID, non-obese diabetic/severe combined immunodeficiency; PAS, periodic acid-Schiff; H&E, hematoxylin and eosin.
IL-37b treated group resulted in a remission of the airway inflammation. The levels of eosinophil activators CCL11 and IL-5 were significantly increased in plasma of humanized asthma control group, and CCL11 restored to normal level upon IL-37b administration (all P < 0.05, Figure 5C). Moreover, IL-37b could significantly suppress the induced concentrations of Th2 and asthma-related cytokines IL-4, IL-6, and IL-13 in lung homogenate of asthmatic human PBMC NOD/SCID mice (all P < 0.05, Figure 5D). Reductions were also observed on IL-17, CCL5, and CCL11 upon the treatment of IL-37b (Figure 5D). Similarly, histopathological results of lung tissue illustrated that IL-37b could mitigate the enhanced mucus, eosinophil infiltration, thickened airway wall, and goblet cells in the lung tissue of humanized allergic asthmatic mice (Figure 5E). Regarding inflammatory cell infiltration of lymphocytes, macrophages, eosinophils, neutrophils, as well as total cells in BALF, cell number of macrophages, eosinophils, and total inflammatory cells was 8.80 × 105, 2.41 × 105, and 11.42 × 105, respectively, which were significantly increased in asthmatic mice when compared with normal control mice. The cell number decreased to 7.04 × 105, 1.22 × 105, and 8.44 × 105, respectively, after IL-37b treatment. Similar IL-37-mediated anti-allergic inflammatory activities, such as the suppression of inflammatory cytokine/chemokine expression, specific IgE, inflammatory cell infiltration, thickened airway wall, collagen deposition, mucus and lung fibrosis together with upregulated Treg in lung tissue, etc., were shown in OVA-induced allergic asthmatic mice (Figure S4 in Supplementary Material) and HDM-induced allergic asthmatic mice (Figure S5 in Supplementary Material).
IL-37 has been shown to play immunoregulatory roles in animal models of myocardial infarction, RA, diabetes, fungal infection, septic shock, colitis, hepatitis, contact-hypersensitivity, psoriasis, and fibrosarcoma (22, 26–30, 42–46). Protective functions have also been reported for IL-37b on allergic airway inflammation induced by OVA, probably via IL-18Rα and the orphan receptor IL-1R8 (29, 47). Eosinophilia is a prominent characteristic of allergic asthma and related with asthma severity. However, the underlying cellular mechanisms by which IL-37b regulates human eosinophils in allergic asthma have not been clearly investigated. In this study, we illustrated that IL-37b could suppress transcriptional levels of PYCARD, S100A9, and CAMP genes, and antagonize the activation of NF-κB, PI3K–Akt, and ERK1/2 pathways in eosinophils, which elucidate the intracellular signaling cascade to suppress the bacterial TLR2-mediated activation in eosinophils upon interaction with human bronchial epithelial cells, and ameliorate the exacerbated allergic airway inflammation in HDM-humanized asthmatic mice.
Cascade reactions amplified by Th2 cells promote the recruitment of eosinophils into the lung tissue, with subsequent mucus production, bronchial inflammation, and AHR and remodeling which are characteristics of allergic asthma (3, 48). During the development of asthma, eosinophils are accessible to human bronchial epithelial cells in bronchus, and their interaction upon the stimulation by allergen activates both cells to induce allergic inflammation. In our study, BEAS-2B cells, which share similar pattern for the expression of cell surface adhesion molecules and production of inflammatory cytokines and chemokines with primary human bronchial epithelial cells as demonstrated in our previous studies (49, 50), were used to coculture with human eosinophils followed by stimulation with TLR2 ligand PGN to mimic the microenvironment upon infection in the bronchus of allergic asthmatic patients. Results in Figure 1 showed the capability of IL-37b for relieving the activation of TLR2 pathway in both eosinophils and BEAS-2B cells, via specific signaling pathways and transcriptional regulation (Figures 2 and 3).
Using murine splenic macrophages and DCs of wild-type IL-37-transgenic mice and IL-37-transgenic with IL-1R8-deficient mice, a previous report has revealed the signaling pathway under LPS stimulation that IL-37b binds to its receptors IL-1R8 and IL-18Rα and exhibits anti-inflammatory properties through signaling molecules such as STAT3 and p62 (dok) to inhibit the kinases Fyn, TAK1, and the transcription factor NF-κB, as well as mitogen-activated protein kinase (MAPK) (24). This finding therefore elucidated the regulatory mechanism of IL-37b on murine splenic antigen-presenting cells in LPS-induced inflammation. Given that the mechanisms of IL-37b may be different in different species and cell types, the phosphorylation levels of cell signaling molecules IκB, ERK1/2, and Akt in human eosinophils upon coculture with BEAS-2B and stimulation by PGN were quantified using flow cytometry in our study. We illustrated the involvement of distinct NF-κB, ERK1/2, and Akt pathways in IL-37b suppressing the activation of eosinophils and BEAS-2B cells (Figure 2). With regard of the central roles of pro-inflammatory gene transcription for the NF-κB pathway (51), reductions of TNF-α, IL-1β, IL-6, CCL2, and CXCL-8 release in in vitro coculture by IL-37b may partly rely on the suppressed transcriptional activity of NF-κB. Activation of ERK1/2 and Akt pathways has been reported to involve in lung inflammation and alveolar remodeling (52, 53). Consequently, IL-37b-attenuated phosphorylation of ERK1/2 and Akt in eosinophils and BEAS-2B cells, at least in part, can account for the diminished airway thickness in asthmatic mice.
To further investigate the molecular mechanisms mediated by IL-37b, RNA-sequencing, bioinformatics, and real-time qPCR validation were performed on cocultured eosinophils. Genes PYCARD (apoptosis-associated speck-like protein containing a CARD), S100A9, and CAMP (cathelicidin antimicrobial peptide LL-37) were suggested to play pivotal roles in IL-37b anti-allergic inflammatory activities. PYCARD is well known for its function as an adapter in diverse types of inflammasomes to activate caspase-1 and lead to the maturation of pro-inflammatory cytokines (54). PYCARD also acts on inflammasome independently with subsequent regulation on MAPK activity, NF-κB activation, and cytokines/chemokines expression (55, 56). S100A9 protein plays a vital role in inflammation and immune response (57–59). The concentration of S100A9 was elevated in BALF of asthmatic patients and correlated with plasma IgE concentration (60, 61). It has been shown that S100A9 triggers the production of mucin protein MUC5AC to contribute to mucin hyper-production in airway and activates NF-κB pathway to induce pro-inflammatory cytokines and chemokines (62). CAMP is the unique cathelicidin-family antimicrobial peptide LL-37 found in human (63–65). Our recent study has demonstrated that LL-37 promotes asthma deterioration (33). Furthermore, PYCARD, S100A9, and CAMP are annotated to inflammation and immune-related GO terms, which further illustrate their contributions in inflammation and immune response (all P < 0.05, Table 2; Figure 3B). Therefore, downregulated transcriptional level of these genes, at least partly, is responsible for the amelioration of allergic airway inflammation in IL-37b-treated mice. The IL-37-mediated intracellular signaling cascades in eosinophils for the suppression of allergic inflammation are illustrated in Figure 6.
Figure 6. IL-37-mediated intracellular signaling cascades in eosinophils for the suppression of allergic airway inflammation. Interaction of toll-like receptor-2 (TLR2) with bacterial cell wall component leads to the activation of intracellular signaling cascades in eosinophils with subsequent intercellular interaction with airway epithelial cells. Signaling molecules Akt, extracellular signal-regulated kinase (ERK)1/2, and IκB are phosphorylated to initiate the downstream transcription of target genes for cell survival and pro-inflammatory cytokines and chemokines which deteriorate allergic inflammation. Furthermore, transcriptional level of CAMP, PYCARD, and S100A9 is upregulated in allergic inflammation. CAMP (LL37) protein is capable of triggering TLR3 on bronchial epithelial cells, which also activates signaling molecules and results in asthma deterioration. PYCARD functions as an adapter in diverse types of inflammasomes, such as AIM2 inflammasome, NLRC4 inflammasome, NLRP3 inflammasome, to activate caspase-1 and lead to the maturation of pro-inflammatory cytokine such as IL-1β. S100A9 triggers the production of mucin protein MUC5AC in airway epithelial cells, which contributes to mucin hyper-production in airway, and activates nuclear factor-κB pathway to induce pro-inflammatory cytokines and chemokines, which result in exacerbation of airway inflammation. IL-37 is capable of attenuating phosphorylation of Akt, ERK1/2, and IκB in eosinophils, with subsequent downregulating transcriptional level of CAMP, PYCARD, and S100A9 in eosinophils, thereby ameliorating allergic airway inflammation.
Adopting our established humanized murine mice (34), chimeric allergic asthmatic mice with humanized peripheral blood cells were used to mimic human allergic asthma. Results from the humanized mice provide more clinical relevant biochemical basis for future therapeutic application of IL-37b on the treatment of allergic asthma. In OVA-induced allergic airway inflammation mice (Figure S4 in Supplementary Material) and HDM-induced allergic asthmatic mice (Figure S5 in Supplementary Material), amelioration on asthma exacerbation was indicated in IL-37b-treated groups, which is in concordance with the result of previous publications (66, 67). Noteworthy, IL-37b administrated at challenge stage showed higher efficacy on ameliorating asthma exacerbation when compared with that of sensitization stage. This finding indicates that IL-37b may be beneficial for therapeutic treatment, but less effective in prophylactic treatment. Nevertheless, further studies are needed to estimate the feasibility of IL-37b for clinical application, including efficacy and safety, and more in-depth mechanistic study is on-going.
A recently study reported the inhibition of IL-37b on HDM-induced pulmonary eosinophilia and AHR, without affecting Th2-related cytokine production (67). This discrepancy comparing with the suppression of local and systemic Th2 cytokines in our present study (Figures S4 and S5 in Supplementary Material) may be due to different administration route and dosage. In Lv et al. study, mice was intranasally (i.n.) administrated with IL-37b (200 ng). Nasal mucosa is a barrier for protein that can decrease the nasal bioavailability of high molecular weight protein ((1,000 Da) (68, 69). Therefore, most intranasal administrated IL-37b (MW = 19.4 kDa) may enter respiratory tract to interact with local pro-inflammatory cells and mediators. Together with the mucociliary clearance and enzymatic degradation in nasal mucosa (70, 71), the bioavailability of intranasal IL-37b can be further decreased, resulting in short half-life. Similar decreasing activity of i.n. administrated IL-37b (500 ng) was also observed in our study (data not shown). Therefore, our present i.v. systemic administration of IL-37b not only benefits local pulmonary inflammation but also downregulates inflammatory cells migration from circulation into pulmonary vasculature and airway submucosa, together with suppression of Th2 and inflammatory cytokine and chemokine production.
In conclusion, our study has demonstrated novel intracellular mechanism of IL-37b on human eosinophil axis by which IL-37b can suppress bacterial TLR2-mediated activation in eosinophils upon interacting with human bronchial epithelial cells, thereby contributing to the remission of allergic airway inflammation. Together with the result of preclinical studies using humanized mice, IL-37b may be used as a novel treatment modality for allergic asthmatic patients, with least side effects comparing with conventional steroid treatment. Given the pivotal role of eosinophils in many other allergic diseases such as atopic dermatitis, IL-37b may also be a potential therapeutic agent for various eosinophilic disorders.
The experimental procedure using human eosinophils purified from buffy coats was approved by Clinical Research Ethics Committee of The Chinese University of Hong Kong-New Territories East Cluster Hospitals according to the 1964 Declaration of Helsinki and its later amendments and informed written consent was obtained from all subjects. All recruited subjects were ethnic Chinese. The above protocol were approved by the Clinical Research Ethics Committee of The Chinese University of Hong Kong-New Territories East Cluster Hospitals, and informed written consent was obtained from all subjects or their parents in accordance with the 1964 Declaration of Helsinki and its later amendments. All animal experimentations were conducted in accordance with the principles outlined in the Animal Experimentation Ethics Committee (AEEC) guide for the Care and Use of Laboratory Animals, as approved by the AEEC of The Chinese University of Hong Kong.
JZ, JD, PJ, TL, LN, DJ, CL, and C-KW contributed to the conception and design of the study. JZ, JD, LJ, DL, MT, and DJ performed experiment and analyzed data. JZ, CL, and C-KW drafted and revised the manuscript.
The authors declare that the research was conducted in the absence of any commercial or financial relationships that could be construed as a potential conflict of interest.
The authors would like to give their thanks to Ida Chu and Elvis Koon, Department of Chemical Pathology, The Chinese University of Hong Kong, who helped for blood taking from studied subjects, and all allergic asthmatic patients and healthy subjects who participated in the study. We also appreciate Ben Chan, Institute of Chinese Medicine, The Chinese University of Hong Kong, for supporting necessary materials for the murine study.
This work was supported by Research Grant from Hong Kong Institute of Allergy, Hong Kong, 2016.
The Supplementary Material for this article can be found online at https://www.frontiersin.org/articles/10.3389/fimmu.2018.01445/full#supplementary-material.
1. GBD 2015 Chronic Respiratory Disease Collaborators. Global, regional, and national deaths, prevalence, disability-adjusted life years, and years lived with disability for chronic obstructive pulmonary disease and asthma, 1990-2015: a systematic analysis for the Global Burden of Disease Study 2015. Lancet Respir Med (2017) 5(9):691–706. doi:10.1016/S2213-2600(17)30293-X
2. Romanet-Manent S, Charpin D, Magnan A, Lanteaume A, Vervloet D; EGEA Cooperative Group. Allergic vs nonallergic asthma: what makes the difference? Allergy (2002) 57(7):607–13. doi:10.1034/j.1398-9995.2002.23504.x
3. Lambrecht BN, Hammad H. The immunology of asthma. Nat Immunol (2015) 16(1):45–56. doi:10.1038/ni.3049
4. Herrick CA, Bottomly K. To respond or not to respond: T cells in allergic asthma. Nat Rev Immunol (2003) 3(5):405–12. doi:10.1038/nri1084
5. Oettgen HC, Geha RS. IgE regulation and roles in asthma pathogenesis. J Allergy Clin Immunol (2001) 107(3):429–40. doi:10.1067/mai.2001.113759
6. Galli SJ, Tsai M, Piliponsky AM. The development of allergic inflammation. Nature (2008) 454(7203):445–54. doi:10.1038/nature07204
7. Schroder NW, Crother TR, Naiki Y, Chen S, Wong MH, Yilmaz A, et al. Innate immune responses during respiratory tract infection with a bacterial pathogen induce allergic airway sensitization. J Allergy Clin Immunol (2008) 122(3):595–602.e5. doi:10.1016/j.jaci.2008.06.038
8. Ritchie AI, Farne HA, Singanayagam A, Jackson DJ, Mallia P, Johnston SL. Pathogenesis of viral infection in exacerbations of airway disease. Ann Am Thorac Soc (2015) 12(Suppl 2):S115–32. doi:10.1513/AnnalsATS.201503-151AW
9. Takeuchi O, Akira S. Pattern recognition receptors and inflammation. Cell (2010) 140(6):805–20. doi:10.1016/j.cell.2010.01.022
10. Fulkerson PC, Rothenberg ME. Targeting eosinophils in allergy, inflammation and beyond. Nat Rev Drug Discov (2013) 12(2):117–29. doi:10.1038/nrd3838
11. Romagnani S. The role of lymphocytes in allergic disease. J Allergy Clin Immunol (2000) 105(3):399–408. doi:10.1067/mai.2000.104575
12. Murphy K, Travers P, Walport M, Janeway C. Janway’s Immunobiology. 8th ed. USA: Garland Science (2012). xix, 868 p.
13. Wong CK, Wang CB, Ip WK, Tian YP, Lam CW. Role of p38 MAPK and NF-kB for chemokine release in coculture of human eosinophils and bronchial epithelial cells. Clin Exp Immunol (2005) 139(1):90–100. doi:10.1111/j.1365-2249.2005.02678.x
14. Wang CB, Wong CK, Ip WK, Li ML, Tian YP, Lam CW. Induction of IL-6 in co-culture of bronchial epithelial cells and eosinophils is regulated by p38 MAPK and NF-kappaB. Allergy (2005) 60(11):1378–85. doi:10.1111/j.1398-9995.2005.00884.x
15. Cheung PF, Wong CK, Ho AW, Hu S, Chen DP, Lam CW. Activation of human eosinophils and epidermal keratinocytes by Th2 cytokine IL-31: implication for the immunopathogenesis of atopic dermatitis. Int Immunol (2010) 22(6):453–67. doi:10.1093/intimm/dxq027
16. Wong CK, Hu S, Leung KM, Dong J, He L, Chu YJ, et al. NOD-like receptors mediated activation of eosinophils interacting with bronchial epithelial cells: a link between innate immunity and allergic asthma. Cell Mol Immunol (2013) 10(4):317–29. doi:10.1038/cmi.2012.77
17. Nold MF, Nold-Petry CA, Zepp JA, Palmer BE, Bufler P, Dinarello CA. IL-37 is a fundamental inhibitor of innate immunity. Nat Immunol (2010) 11(11):1014–22. doi:10.1038/ni.1944
18. Kumar S, Hanning CR, Brigham-Burke MR, Rieman DJ, Lehr R, Khandekar S, et al. Interleukin-1F7B (IL-1H4/IL-1F7) is processed by caspase-1 and mature IL-1F7B binds to the IL-18 receptor but does not induce IFN-gamma production. Cytokine (2002) 18(2):61–71. doi:10.1006/cyto.2002.0873
19. Bufler P, Gamboni-Robertson F, Azam T, Kim SH, Dinarello CA. Interleukin-1 homologues IL-1F7b and IL-18 contain functional mRNA instability elements within the coding region responsive to lipopolysaccharide. Biochem J (2004) 381(Pt 2):503–10. doi:10.1042/BJ20040217
20. Shuai X, Wei-min L, Tong YL, Dong N, Sheng ZY, Yao YM. Expression of IL-37 contributes to the immunosuppressive property of human CD4+CD25+ regulatory T cells. Sci Rep (2015) 5:14478. doi:10.1038/srep14478
21. Bufler P, Azam T, Gamboni-Robertson F, Reznikov LL, Kumar S, Dinarello CA, et al. A complex of the IL-1 homologue IL-1F7b and IL-18-binding protein reduces IL-18 activity. Proc Natl Acad Sci U S A (2002) 99(21):13723–8. doi:10.1073/pnas.212519099
22. Luo Y, Cai X, Liu S, Wang S, Nold-Petry CA, Nold MF, et al. Suppression of antigen-specific adaptive immunity by IL-37 via induction of tolerogenic dendritic cells. Proc Natl Acad Sci U S A (2014) 111(42):15178–83. doi:10.1073/pnas.1416714111
23. Lunding L, Schroder A, Wegmann M. Allergic airway inflammation: unravelling the relationship between IL-37, IL-18Ralpha and Tir8/SIGIRR. Expert Rev Respir Med (2015) 9(6):739–50. doi:10.1586/17476348.2015.1109452
24. Nold-Petry CA, Lo CY, Rudloff I, Elgass KD, Li S, Gantier MP, et al. IL-37 requires the receptors IL-18Ralpha and IL-1R8 (SIGIRR) to carry out its multifaceted anti-inflammatory program upon innate signal transduction. Nat Immunol (2015) 16(4):354–65. doi:10.1038/ni.3103
25. Schröder A, Lunding L, Webering S, Vock C, Raedler D, Schaub B, et al. IL-37 ameliorates experimental asthma via a mechanism that is independent from IL-18 signaling. Pneumologie (2016) 70:422. doi:10.1055/s-0036-1572306
26. Wu B, Meng K, Ji Q, Cheng M, Yu K, Zhao X, et al. Interleukin-37 ameliorates myocardial ischaemia/reperfusion injury in mice. Clin Exp Immunol (2014) 176(3):438–51. doi:10.1111/cei.12284
27. Ye L, Jiang B, Deng J, Du J, Xiong W, Guan Y, et al. IL-37 alleviates rheumatoid arthritis by suppressing IL-17 and IL-17-triggering cytokine production and limiting Th17 cell proliferation. J Immunol (2015) 194(11):5110–9. doi:10.4049/jimmunol.1401810
28. Ballak DB, van Diepen JA, Moschen AR, Jansen HJ, Hijmans A, Groenhof GJ, et al. IL-37 protects against obesity-induced inflammation and insulin resistance. Nat Commun (2014) 5:4711. doi:10.1038/ncomms5711
29. Lunding L, Webering S, Vock C, Schroder A, Raedler D, Schaub B, et al. IL-37 requires IL-18Ralpha and SIGIRR/IL-1R8 to diminish allergic airway inflammation in mice. Allergy (2015) 70(4):366–73. doi:10.1111/all.12566
30. Moretti S, Bozza S, Oikonomou V, Renga G, Casagrande A, Iannitti RG, et al. IL-37 inhibits inflammasome activation and disease severity in murine aspergillosis. PLoS Pathog (2014) 10(11):e1004462. doi:10.1371/journal.ppat.1004462
31. Charrad R, Berraies A, Hamdi B, Ammar J, Hamzaoui K, Hamzaoui A. Anti-inflammatory activity of IL-37 in asthmatic children: correlation with inflammatory cytokines TNF-alpha, IL-beta, IL-6 and IL-17A. Immunobiology (2016) 221(2):182–7. doi:10.1016/j.imbio.2015.09.009
32. Boraschi D, Lucchesi D, Hainzl S, Leitner M, Maier E, Mangelberger D, et al. IL-37: a new anti-inflammatory cytokine of the IL-1 family. Eur Cytokine Netw (2011) 22(3):127–47. doi:10.1684/ecn.2011.0288
33. Jiao D, Wong CK, Tsang MS, Chu IM, Liu D, Zhu J, et al. Activation of eosinophils interacting with bronchial epithelial cells by antimicrobial peptide LL-37: implications in allergic asthma. Sci Rep (2017) 7(1):1848. doi:10.1038/s41598-017-02085-5
34. Dong J, Wong CK, Cai Z, Jiao D, Chu M, Lam CW. Amelioration of allergic airway inflammation in mice by regulatory IL-35 through dampening inflammatory dendritic cells. Allergy (2015) 70(8):921–32. doi:10.1111/all.12631
35. Fischer AH, Jacobson KA, Rose J, Zeller R. Hematoxylin and eosin staining of tissue and cell sections. CSH Protoc (2008) 2008:pdb.prot4986. doi:10.1101/pdb.prot4986
36. Rosenberg HF, Dyer KD, Foster PS. Eosinophils: changing perspectives in health and disease. Nat Rev Immunol (2013) 13(1):9–22. doi:10.1038/nri3341
37. Wong CK, Wang CB, Li ML, Ip WK, Tian YP, Lam CW. Induction of adhesion molecules upon the interaction between eosinophils and bronchial epithelial cells: involvement of p38 MAPK and NF-kappaB. Int Immunopharmacol (2006) 6(12):1859–71. doi:10.1016/j.intimp.2006.08.003
38. Wong CK, Dong J, Lam CW. Molecular mechanisms regulating the synergism between IL-32gamma and NOD for the activation of eosinophils. J Leukoc Biol (2014) 95(4):631–42. doi:10.1189/jlb.0813452
39. Phipps S, Lam CE, Foster PS, Matthaei KI. The contribution of toll-like receptors to the pathogenesis of asthma. Immunol Cell Biol (2007) 85(6):463–70. doi:10.1038/sj.icb.7100104
40. Kawai T, Akira S. The role of pattern-recognition receptors in innate immunity: update on toll-like receptors. Nat Immunol (2010) 11(5):373–84. doi:10.1038/ni.1863
41. Wong CK, Cheung PF, Ip WK, Lam CW. Intracellular signaling mechanisms regulating toll-like receptor-mediated activation of eosinophils. Am J Respir Cell Mol Biol (2007) 37(1):85–96. doi:10.1165/rcmb.2006-0457OC
42. Kumar S, McDonnell PC, Lehr R, Tierney L, Tzimas MN, Griswold DE, et al. Identification and initial characterization of four novel members of the interleukin-1 family. J Biol Chem (2000) 275(14):10308–14. doi:10.1074/jbc.275.14.10308
43. Liu W, Deng L, Chen Y, Sun C, Wang J, Zhou L, et al. Anti-inflammatory effect of IL-37b in children with allergic rhinitis. Mediators Inflamm (2014) 2014:746846. doi:10.1155/2014/746846
44. Sharma S, Kulk N, Nold MF, Graf R, Kim SH, Reinhardt D, et al. The IL-1 family member 7b translocates to the nucleus and down-regulates proinflammatory cytokines. J Immunol (2008) 180(8):5477–82. doi:10.4049/jimmunol.180.8.5477
45. McNamee EN, Masterson JC, Jedlicka P, McManus M, Grenz A, Collins CB, et al. Interleukin 37 expression protects mice from colitis. Proc Natl Acad Sci U S A (2011) 108(40):16711–6. doi:10.1073/pnas.1111982108
46. Bulau AM, Fink M, Maucksch C, Kappler R, Mayr D, Wagner K, et al. In vivo expression of interleukin-37 reduces local and systemic inflammation in concanavalin A-induced hepatitis. ScientificWorldJournal (2011) 11:2480–90. doi:10.1100/2011/968479
47. Lunding L, Webering S, Vock C, Schroder A, Raedler D, Schaub B, et al. Effect of IL-37 on allergic airway inflammation. Ann American Thorac Soc (2016) 13(Suppl 1):S95–6. doi:10.1513/AnnalsATS.201506-380MG
48. Kumar V, Abbas AK, Aster JC, Robbins SL. Robbins Basic Pathology. 9th ed. Philadelphia, PA: Elsevier/Saunders (2013). xii, 910 p.
49. Wong CK, Cao J, Yin YB, Lam CW. Interleukin-17A activation on bronchial epithelium and basophils: a novel inflammatory mechanism. Eur Respir J (2010) 35(4):883–93. doi:10.1183/09031936.00088309
50. Qiu HN, Wong CK, Chu IM, Hu S, Lam CW. Muramyl dipeptide mediated activation of human bronchial epithelial cells interacting with basophils: a novel mechanism of airway inflammation. Clin Exp Immunol (2013) 172(1):81–94. doi:10.1111/cei.12031
51. Tak PP, Firestein GS. NF-kappaB: a key role in inflammatory diseases. J Clin Invest (2001) 107(1):7–11. doi:10.1172/JCI11830
52. Lee PJ, Zhang X, Shan P, Ma B, Lee CG, Homer RJ, et al. ERK1/2 mitogen-activated protein kinase selectively mediates IL-13-induced lung inflammation and remodeling in vivo. J Clin Invest (2006) 116(1):163–73. doi:10.1172/JCI25711
53. Rao SS, Mu Q, Zeng Y, Cai PC, Liu F, Yang J, et al. Calpain-activated mTORC2/Akt pathway mediates airway smooth muscle remodelling in asthma. Clin Exp Allergy (2017) 47(2):176–89. doi:10.1111/cea.12805
54. Franklin BS, Bossaller L, De Nardo D, Ratter JM, Stutz A, Engels G, et al. The adaptor ASC has extracellular and ‘prionoid’ activities that propagate inflammation. Nat Immunol (2014) 15(8):727–37. doi:10.1038/ni.2913
55. Taxman DJ, Holley-Guthrie EA, Huang MT, Moore CB, Bergstralh DT, Allen IC, et al. The NLR adaptor ASC/PYCARD regulates DUSP10, mitogen-activated protein kinase (MAPK), and chemokine induction independent of the inflammasome. J Biol Chem (2011) 286(22):19605–16. doi:10.1074/jbc.M111.221077
56. Hasegawa M, Imamura R, Motani K, Nishiuchi T, Matsumoto N, Kinoshita T, et al. Mechanism and repertoire of ASC-mediated gene expression. J Immunol (2009) 182(12):7655–62. doi:10.4049/jimmunol.0800448
57. Lagasse E, Clerc RG. Cloning and expression of two human genes encoding calcium-binding proteins that are regulated during myeloid differentiation. Mol Cell Biol (1988) 8(6):2402–10. doi:10.1128/MCB.8.6.2402
58. Gebhardt C, Nemeth J, Angel P, Hess J. S100A8 and S100A9 in inflammation and cancer. Biochem Pharmacol (2006) 72(11):1622–31. doi:10.1016/j.bcp.2006.05.017
59. Nacken W, Roth J, Sorg C, Kerkhoff C. S100A9/S100A8: myeloid representatives of the S100 protein family as prominent players in innate immunity. Microsc Res Tech (2003) 60(6):569–80. doi:10.1002/jemt.10299
60. Kim DH, Choi E, Lee JS, Lee NR, Baek SY, Gu A, et al. House dust mite allergen regulates constitutive apoptosis of normal and asthmatic neutrophils via toll-like receptor 4. PLoS One (2015) 10(5):e0125983. doi:10.1371/journal.pone.0125983
61. Sharma M, Mehla K, Batra J, Ghosh B. Association of a chromosome 1q21 locus in close proximity to a late cornified envelope-like proline-rich 1 (LELP1) gene with total serum IgE levels. J Hum Genet (2007) 52(4):378–83. doi:10.1007/s10038-007-0118-5
62. Kang JH, Hwang SM, Chung IY. S100A8, S100A9 and S100A12 activate airway epithelial cells to produce MUC5AC via extracellular signal-regulated kinase and nuclear factor-kappaB pathways. Immunology (2015) 144(1):79–90. doi:10.1111/imm.12352
63. Durr UH, Sudheendra US, Ramamoorthy A. LL-37, the only human member of the cathelicidin family of antimicrobial peptides. Biochim Biophys Acta (2006) 1758(9):1408–25. doi:10.1016/j.bbamem.2006.03.030
64. Kuroda K, Okumura K, Isogai H, Isogai E. The human cathelicidin antimicrobial peptide LL-37 and mimics are potential anticancer drugs. Front Oncol (2015) 5:144. doi:10.3389/fonc.2015.00144
65. Piktel E, Niemirowicz K, Wnorowska U, Watek M, Wollny T, Gluszek K, et al. The role of cathelicidin LL-37 in cancer development. Arch Immunol Ther Exp (2016) 64(1):33–46. doi:10.1007/s00005-015-0359-5
66. Huang N, Liu K, Liu J, Gao X, Zeng Z, Zhang Y, et al. Interleukin-37 alleviates airway inflammation and remodeling in asthma via inhibiting the activation of NF-kappaB and STAT3 signalings. Int Immunopharmacol (2018) 55:198–204. doi:10.1016/j.intimp.2017.12.010
67. Lv J, Xiong Y, Li W, Cui X, Cheng X, Leng Q, et al. IL-37 inhibits IL-4/IL-13-induced CCL11 production and lung eosinophilia in murine allergic asthma. Allergy (2018). doi:10.1111/all.13395
68. McMartin C, Hutchinson LE, Hyde R, Peters GE. Analysis of structural requirements for the absorption of drugs and macromolecules from the nasal cavity. J Pharm Sci (1987) 76(7):535–40. doi:10.1002/jps.2600760709
69. O’Hagan DT, Illum L. Absorption of peptides and proteins from the respiratory tract and the potential for development of locally administered vaccine. Crit Rev Ther Drug Carrier Syst (1990) 7(1):35–97.
70. Romeo VD, deMeireles J, Sileno AP, Pimplaskar HK, Behl CR. Effects of physicochemical properties and other factors on systemic nasal drug delivery. Adv Drug Deliv Rev (1998) 29(1–2):89–116. doi:10.1016/S0169-409X(97)00063-X
Keywords: allergic asthma, eosinophils, interleukin-37, signal transduction, transcriptional profile
Citation: Zhu J, Dong J, Ji L, Jiang P, Leung TF, Liu D, Ng LG, Tsang MS-M, Jiao D, Lam CW-K and Wong C-K (2018) Anti-Allergic Inflammatory Activity of Interleukin-37 Is Mediated by Novel Signaling Cascades in Human Eosinophils. Front. Immunol. 9:1445. doi: 10.3389/fimmu.2018.01445
Received: 01 February 2018; Accepted: 11 June 2018;
Published: 22 June 2018
Edited by:
Deirdre R. Coombe, Curtin University, AustraliaReviewed by:
David Dombrowicz, Institut National de la Santé et de la Recherche Médicale (INSERM), FranceCopyright: © 2018 Zhu, Dong, Ji, Jiang, Leung, Liu, Ng, Tsang, Jiao, Lam and Wong. This is an open-access article distributed under the terms of the Creative Commons Attribution License (CC BY). The use, distribution or reproduction in other forums is permitted, provided the original author(s) and the copyright owner are credited and that the original publication in this journal is cited, in accordance with accepted academic practice. No use, distribution or reproduction is permitted which does not comply with these terms.
*Correspondence: Chun-Kwok Wong, Y2std29uZ0BjdWhrLmVkdS5oaw==
Disclaimer: All claims expressed in this article are solely those of the authors and do not necessarily represent those of their affiliated organizations, or those of the publisher, the editors and the reviewers. Any product that may be evaluated in this article or claim that may be made by its manufacturer is not guaranteed or endorsed by the publisher.
Research integrity at Frontiers
Learn more about the work of our research integrity team to safeguard the quality of each article we publish.