- Division of Oncology, Department of Medicine, Stanford University School of Medicine, Stanford, CA, United States
Metastasis is the ultimate consequence of cancer progression and the cause of patients’ death across different cancer types. Patients with initial diagnosis of distant disease have a worst 5-year survival compared to patients with localized disease. Therapies that target primary tumors fail to eradicate distant dissemination of cancer. Recently, immunotherapies have improved the survival of patients with metastatic disease, such as melanoma and lung cancer. However, only a fraction of patients responds to immunotherapy modalities that target the host immune system. The need to identify new druggable targets that inhibit or prevent metastasis is, therefore, much needed. Tetraspanins have emerged as key players in regulating cell migration, invasion, and metastasis. By serving as molecular adaptors that cluster adhesion receptors, signaling molecules, and cell surface receptors; tetraspanins are involved in all steps of the metastatic cascade. They regulate cell proliferation, participate in EMT transition, modulate integrin-mediated cell adhesion, and participate in angiogenesis and invasion processes. Tetraspanins have also been shown to modulate metastasis indirectly through exosomes and by regulating cellular interactions in the immune system. Importantly, targeting individual tetraspanin with antibodies has impacted tumor progression. This review will focus on the contribution of tetraspanins to the metastatic process and their potential as therapeutic tumor targets.
Introduction
Malignant transformation of healthy tissues gives rise to cancer, this disease affects millions of people worldwide. Moreover, metastases; the dissemination of cancer cells is still the greatest cause of death. Patients diagnosed with localized disease have a better 5-year survival than patients with distant disease at the time of diagnosis (1). Therefore, treatments that prevent or diminish metastatic lesions are much needed, such as identifying new druggable targets involved in the metastatic cascade.
Monoclonal antibodies (mAbs) are the preferred immunotherapeutic tools to either target the host immune system or to target the tumor (2). The most common tumor targets are cell surface molecules, such as EGFR, HER2, Mesothelin, CD19, or CD20 whose cell membrane localization, and sometimes tumor-specific expression, or overexpression in comparison to healthy tissues, makes them suitable for antibody-based therapy (2). More recent approaches to immunotherapy of cancer do not target antigens expressed on tumor cells—they unleash the host immune checkpoint blockade, and have improved the survival of patients with metastatic cancers (3).
Tetraspanins are a family of conserved proteins in eukaryotic cells that spans the membrane four times. In humans, 33 members have been identified with different tissue distribution. Expression of some tetraspanins such as CD37 and CD53 is restricted to hematopoietic cells, whereas others, such as CD9, CD81, and CD151 are more broadly expressed. Tetraspanins serve as membrane scaffolds that bring together surface molecules, such as integrins and cell-specific receptors, additional growing evidence shows that engagement of tetraspanins leads to recruiting signaling molecules thereby activating downstream pathways (4). This plethora of interacting partners allows tetraspanins to function in different cellular processes under physiological conditions but also in disease. Multiple studies have shown that tetraspanins regulate tumor growth, cell adhesion, invasion, and migration of tumor cells, reviewed in Ref. (5, 6). Importantly, targeting some tetraspanins with mAbs has proven to be efficient in eliminating tumor cells and in preventing metastasis in preclinical models (7). Here, we will give an overview of tetraspanins as prognostic markers in tumors, their role in invasion and metastasis, and discuss recent studies aimed at antibody-based targeting of these molecules in cancer.
Tetraspanins as Prognostic Markers of Cancer Progression
Among the human tetraspanins, Tspan8 and 12, CD9, CD37, CD63, CD81, CD82, and CD151 play a role in cancer progression (5, 6). Down or upregulation of these tetraspanins on tumors has been correlated with either good or bad prognosis in different types of cancers. Historically, several tetraspanins were identified by studies that compared normal and malignant tissues. Tspan8 was originally identified as a tumor-associated antigen by an antibody (CO-029) (8), CD63 by a melanoma-associated antigen (ME491) (9), CD151 was re-identified by an antimetastatic antibody (10), and CD82/KAI1 as a metastasis suppressor gene (11).
More recent studies have demonstrated that loss of CD82/KAI1 expression is correlated with poor prognosis of several cancers, reviewed in Ref. (12). Interestingly, presence of a specific splice variant of CD82 that removes exon 7 increases tumor progression and invasion (13). Similarly, loss of CD37 expression in patients with diffuse large B-cell lymphoma showed significant correlation with decreased survival after R-CHOP therapy (14). And lack of CD37 in mice increased the development of germinal center derived B cell lymphomas (15).
By contrast, CD151 is expressed in different types of cancer and high expression correlates with poor prognosis (16). It is of note that expression of CD151 in the host contributes to cancer progression—CD151 knockout (KO) mice have fewer skin, melanoma, lung, and prostate cancers than their wild type (WT) counterparts (17–20). Similarly, upregulated CD81 expression in melanoma was found to contribute to an enhanced metastatic phenotype (21, 22). Additionally, expression of CD81 in the host contributes to tumor progression; CD81KO mice have fewer metastases of breast and lung tumors in syngeneic mouse models (23). Importantly, expression of CD81 in immune suppressive cells contributes to tumor progression (24). A recent study showed that expression of CD151 in human is associated with a hyper-proliferative T cell phenotype (25), it would be interesting to know if CD151 expressed in mouse immune cells plays a role in tumor progression. Tspan8 is another tetraspanin whose upregulation is correlated with ovarian cancer progression (26). More recently, the presence of Tspan8 mRNA in the blood was shown to be a sensitive marker for colorectal cancer detection (27). Reduced CD9 expression has been correlated with poor prognosis in several types of cancers, including melanoma, lung, breast, colon, prostate, pancreatic ovarian, and prostate, reviewed in Ref. (28). However, this is not the case for esophageal squamous cell carcinomas that express higher CD9 levels than normal esophageal tissues (29). Lack of CD9 in mice that develop spontaneous prostate cancer mirrors the former human studies, namely, CD9 deficiency increased liver metastases, although it had no effect on onset of primary tumors, nor on lung metastases (30).
Thus, tetraspanins are important players during cancer progression, some tetraspanins are upregulated in certain cancer types while others are downregulated. Clearly, tetraspanins have been used as prognostic markers in cancer.
Interactions of Tetraspanins with Partner Proteins Regulate Invasion and Metastasis
We now know that tetraspanin-enriched microdomains (TEMs) incorporate partner proteins, such as integrins, cell surface receptors, and metalloproteases (MMPs) that contribute to cellular invasion and metastasis (Figure 1). Biochemically, very few of these complexes are held together when solubilized by harsh detergents, the majority only withstand mild detergents (31). The association of CD151 with laminin-binding integrins α3β1, α6β1, and α6β4 (32) is strong, stoichiometric, and occurs early in biosynthesis (33). Silencing CD151 in epithelial carcinoma cells disrupts α3β1 association with TEMS and impairs cell migration (34). In addition, CD151 ablation reduces cell migration, invasion, spreading, and signaling in an integrin-dependent manner (35). CD9/CD81 also regulate α3β1 integrin, loss of these two tetraspanins impairs breast cancer spreading, motility, and disrupts its association with PKCα in a CD151-independent manner (36). Tspan8 was also shown to modulate invasion of melanoma in vitro and in vivo through a β1 integrin by affecting integrin-linked kinase signaling and its downstream target AKT (37).
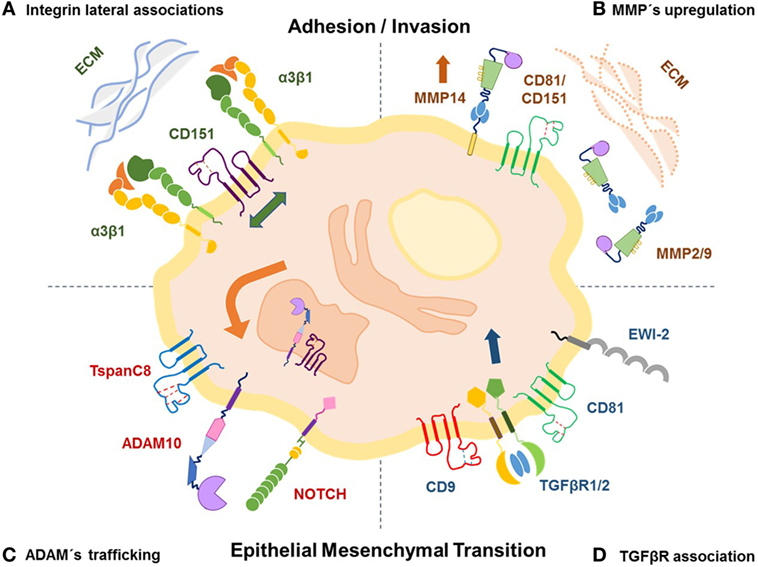
Figure 1. Tetraspanins modulate invasion and metastases by regulating the activity of their partners. (A) Tetraspanins form stable lateral association with integrins in tetraspanin-enriched microdomains (TEMS) favoring spreading, cell adhesion, and migration through the extracellular matrix (ECM); on the other hand, (B) tetraspanins regulate ECM degradation during cell invasion by modulating expression and activity of metalloproteases, such as MMP-2, MMP-9, and MMP-14. (C) The TspanC8 subgroup is known to promote trafficking and cell localization of ADAM10 and its sheddase activity thereby regulating substrates, such as NOTCH receptor, to favor epithelial to mesenchymal transition (EMT). (D) Association of CD9/CD81 with EWI-2 affects TGFβ signaling modulating EMT, invasion, and metastases of melanoma.
While integrins serve as receptors to extracellular matrix (ECM) components, matrix metalloproteinases (MMPs) degrade ECM components. Several tetraspanins associate with MMP-14 (MT1-MMP) during biosynthesis and prevent its degradation enabling cell surface expression. And this association also involves another known tetraspanin partner, EWI-2 (38). However, a knockdown of a single tetraspanin, such as CD9 or CD81 had no effect on MMP-14-dependent fibronectin degradation, but a CD9/CD81 double knockdown clearly affected degradation (39). Interestingly, overexpression of CD81 in a human melanoma cell line upregulated MT1-MMP expression and activity with a consequent increased invasion and metastases in vitro and in vivo (21). Similarly, overexpression of CD9 on fibrosarcoma cells increased MMP-9 production and activity, resulting in a more invasive phenotype in vitro (40); however, transfection of CD9 into small cell lung cancer cells inhibited transcription of MMP-2 and MMP-14 (41). CD151 was shown to be a link between MMP-14 and integrin α3β1 (42); and to mediate tumor progression by affecting expression and function of MMP-9 in hepatocellular carcinoma (43), melanoma (44), and pancreatic adeno carcinoma (43, 45). Finally, silencing CD63 reduced the levels of β-catenin protein and its downstream target MMP-2 inhibiting metastatic lung colonization of ovarian and melanoma tumors (46).
A disintegrin and metalloproteases (ADAMs) also interact with tetraspanins (47). ADAMs are a family of proteases, classified as sheddases because they can cleave extracellular portions of transmembrane proteins regulating cell functions such as cell invasion and motility. The tetraspanin subgroup TspanC8 (Tspan 5, 10, 14, 15, 17, and 33) mediates trafficking, maturation, and compartmentalization of ADAM10, thereby influencing its function (48, 49). Interestingly, TspanC8 members expressed in Drosophila and C. elegans were found to regulate ADAM10 levels and to modulate Notch functions that promote epithelial to mesenchymal transition (EMT) in cancer cells (50, 51). Another tetraspanin family member, Tetraspanin-8, is also linked to cancer progression by inducing ADAM12 upregulation in esophageal carcinoma promoting metastases (52).
Transforming growth factor β (TGF-β1) is also regulated by specific tetraspanins. It was shown that absence of CD151 in breast cancer cells affected the compartmentalization of TGFβ receptor 1 thereby disturbing TGF-β1-induced activation of p38, which correlated with reduced lung adhesion and decreased metastases (53). Similarly, CD9/CD81 were shown to regulate TGF-β1 signaling in melanoma by providing critical support for TGFβR2- TGFβR1 association, which in turns favors EMT-like changes, invasion, and metastases (22). However, TGF-β1 signaling is negatively regulated by EWI-motif containing protein 2 (EWI-2), which when present, sequesters CD9/CD81 thereby dissociating EWI-2 interaction with TGF receptors (22). Indeed, lack of EWI-2 in melanoma cells was associated with increased invasion and metastasis in vitro and in vivo (12).
Tetraspanins clearly regulate tumor progression at different levels by interacting with a plethora of partners, which are implicated in tumor initiation, promotion of an EMT phenotype, invasion, and migration that ultimately lead to metastasis. To better understand the mechanisms of how individual tetraspanin members and their partners contribute to cancer progression in vivo, numerous in vitro studies have used specific anti-tetraspanins mAbs.
Targeting Tetraspanins with mAbs
At the cellular level, mAbs that target individual tetraspanin members have been used to study signaling pathways, to disrupt molecular associations, to analyze the dynamics of cell surface partitioning, and to probe the tetraspanin web (54). Because tetraspanins regulate cell adhesion, invasion, and metastases, a strategy that prevents any of these cell functions seems reasonable (Figure 2). Indeed, several anti-tetraspanins’ mAbs inhibit tumor cell invasion and migration (7, 55). However, not all antibodies that target the same individual tetraspanin share the same properties, suggesting that engagement of specific epitopes in the tetraspanin molecule could result in different outcomes. Thus, an anti-CD9 mAb (PAINS-13) that disrupts the association of CD9 with β1 integrin (56) inhibited the growth of a human colon carcinoma cell line xenograft more effectively than another anti-CD9 mAb (VJI/20) or integrin mAbs (57). Yet, all tested anti-CD9 mAbs (VJI/10, VJI/20, and GR2110) inhibited the trans-endothelial migration of melanoma cells (58). An additional anti-CD9 antibody (ABL6) (59) induced apoptosis (60) and reduced the growth of a human gastric cancer cell line in a xenograft model (61).
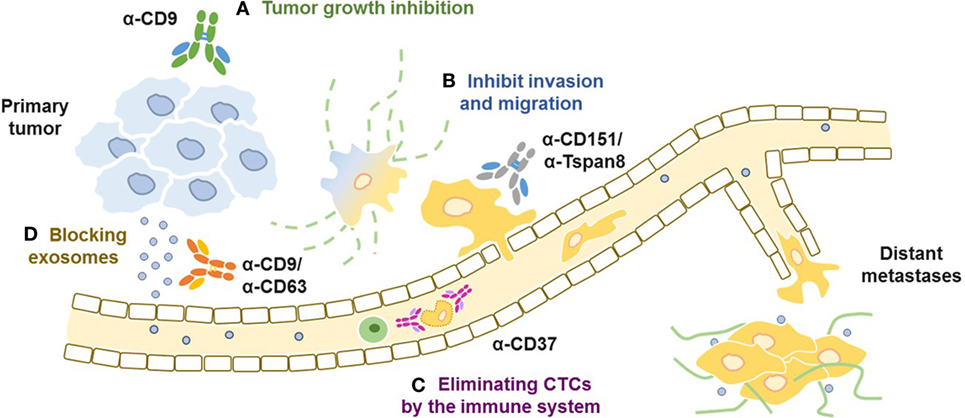
Figure 2. Targeting tetraspanins at different stages of tumor progression. (A) Anti-CD9 antibodies have been shown to inhibit proliferation of human gastric tumors (B) anti-Tspan8 and anti-CD151 antibodies which inhibit adhesion, migration, and extravasation of tumor cells in different types of cancer in vitro and in vivo. (C) Anti-CD37 antibodies eliminates circulating chronic lymphocytic leukemia cells by recruiting and activating the immune system, (D) anti-CD9 and anti-CD63 antibodies block exosomes and enhance their clearance from circulation through macrophage-dependent mechanisms.
The disruption of the interaction with integrins has been studied even in more details for anti-CD151 mAbs whose epitope map to the QRD sequence in the large extracellular loop (LEL) (33). Anti-CD151 mAbs that disrupt the interaction with integrins prevent prostate cancer metastases, in contrast to anti-CD151 mAbs that bind to integrin-associated CD151 (62–64). Indeed, anti-CD151 mAb (1A5) blocked metastases in SCID mice bearing Hep-3 tumors by inhibiting invasion and migration, although the antibody did not inhibit primary tumor growth (65). However, a study using a different monoclonal antibody (SFA1-2B4) that co-immunoprecipitated CD151 with α3β1 integrin prevented lung metastases of human colon cancer and fibrosarcoma cell lines (66). TEMs in endothelial cell junctions include CD151, CD81, and CD9, a study comparing migration of endothelial cells demonstrated that anti-CD151 and CD81, as well as anti-integrin mAbs inhibited migration (67).
More recent studies have shown that mAb targeting Tspan8 (Ts29.2) inhibited the growth of two human colorectal cancer cell lines when injected into nude mice, interestingly the antibody did not induce direct toxicity nor the inhibition of the previously reported angiogenic properties of Tspan8 (68). Moreover, a mAb reacting with the LEL of Tspan8 inhibited tumor invasion in vitro and diminished incidence of ovarian metastases in vivo (26, 69). Recently, a radiolabeled anti-Tspan8 mAb, labeled with lutetium-177 was effective against colorectal cancer in a xenograft model, showing a significantly reduced tumor growth (70).
Taken together, anti-tetraspanin mAbs have shown significant anti-tumor effects in vitro and in mouse models, but because of expression in both tumor and host, off-target effects are still of major concerns. Strategies to reduce the risk of off-target effects could include the use of bispecific mAbs that target both the individual tetraspanin and its interacting partner, for example, CD81/CD19 in B cells.
Exploiting Tetraspanin Function for Immunotherapy
Indeed, a bispecific antibody was engineered to target CD63 on one arm thereby enabling efficient internalization of an anti-HER2 arm that targets the tumor (71). This bispecific construct allows targeting a drug-conjugated tumor binding-antigen, HER2, to the lysosomal pathway by CD63. This novel approach resulted in an improved survival and delayed tumor growth in a xenograft model of ovarian cancer. Mice treated with the bispecific bsHER2xCD63-Duostatin-3 conjugate increased HER2 internalization, this effect was not observed with the monovalent antibodies targeting only HER2 or CD63. This interesting approach of exploiting CD63 for cancer immunotherapy is based on its role in intracellular trafficking and abundance in exosomes (72).
Targeting tetraspanins in exosomes for cargo delivery has been reviewed extensively (5, 73). Exosomes gained attention due to their important function during cellular communication, in addition to tetraspanins they contain a variety of different molecules; receptors, integrins, lipids, mRNAs, and miRNA, all of which have an effect in their target cells (74). Importantly exosomes released from cancer cells have been shown to support metastases. Thus, blocking exosomes from tumor cells with antibodies could be used as a therapeutic strategy to prevent metastases, as shown for cancer stem cells (75). Although tetraspanins (i.e., Tspan8, CD81, and CD63) were originally used as exosome markers it is now clear that they play an active role in exosome cargo-loading and delivery (5, 73). Proteomic analysis using tetraspanins c-terminal domains to pull-down interacting proteins showed a significant overlap with proteins found in exosomes, suggesting that tetraspanins might regulate protein cargo (76). In addition, it has been shown that several tetraspanins regulate protein trafficking to the membrane and intracellular compartments of several receptors (48, 49). Indeed, deleting CD81 revealed a differential protein cargo in exosomes lacking CD81 (76). Moreover, deleting CD81 in endothelial-producing exosome cells but not tetraspanins CD63 or CD82 reduced breast cancer motility and metastasis (77). It is, therefore, likely that individual tetraspanins might regulate protein loading in exosomes, and that such specificity could potentially be exploited to confer selective exosome cargo and/or delivery. For example, the preferential interaction of Tspan8 with α4β4 in exosomes (78). Such preferential interaction could render uptake-specificity of exosome by endothelial and pancreatic cells, and possibly facilitate the use of exosomes for drug delivery, reviewed in Ref. (79).
Proteomic profiling of extracellular vesicles of 60 cancer cell lines (NCI-60) revealed CD81 expression in all 60, while CD9 and CD63 were expressed in about 40 of these cell lines (80). Moreover, clinically relevant exosomes isolated with anti-CD9 or anti-CD63 antibodies and then detected with anti-HER2 revealed a 14–35% tumor-specific exosomes from breast cancer patient serums, which potentially could be used as non-invasive diagnostic method or even used to detect disease progression (81). A study that used anti-CD9 and anti-CD63 antibodies to deplete tumor-derived exosomes in a xenograft model showed a significant reduction in metastases to different organs but had no effect on growth of primary tumors (82). That study showed that exosome depletion from blood was macrophage-mediated. However, in a xenograft model only the tumor tetraspanins are targeted by the antibodies, whereas in human the targeted tetraspanins are expressed both in the host and in the tumors.
From Bench to Bedside
Several tetraspanins used as therapeutic targets show promise in preclinical models of tumor progression and metastases (7, 28, 55, 83). However, CD37 is the only tetraspanin target that has moved forward into the clinic (84). CD37 is predominantly and abundantly expressed on mature B cell malignancies, but not on solid tumors. B cells serve as especially suitable targets for immunotherapy because of the ability of the antibodies to mediate both direct and indirect immune responses. This therapy is a promising tool, especially in those cases where other immunotherapies have failed. Different anti-CD37 antibodies that better mediate antibody-dependent cell cytotoxicity, improve complement activation, or are conjugated to a cytotoxic drugs (83), have recruited patients to clinical trials (clinicalTrials.gov).
Concluding Remarks
Tetraspanins regulate cancer progression and metastases; yet, their broad tissue distribution presents an impediment for cancer immunotherapy, due to possible off-target effects. However, with current exponential advances in immunotherapy, these limitations could be overcome. Examples include bispecific antibodies that confer tumor selectivity or shielding antibodies with tumor-specific proteases (85). Lastly, understanding the function of tetraspanins and their molecular partners, both in the tumor and in the host, will ultimately develop new therapies for cancer treatment.
Author Contributions
FVC ans SL reviewed literature and wrote the manuscript. FVC prepared the figures. All authors approved the manuscript for publication.
Conflict of Interest Statement
The authors declare that the research was conducted in the absence of any commercial or financial relationships that could be construed as a potential conflict of interest.
The handling Editor declared a past co-authorship with one of the authors SL.
Funding
This work was supported by the Breast Cancer Research program from the Department of Defense grant W81XWH-14-1-0397 and The Wallace H. Coulter Translational Research Program Grant at Stanford. FVC was supported by The American Society of Immunology through Careers in Immunology fellowship.
References
2. Scott AM, Wolchok JD, Old LJ. Antibody therapy of cancer. Nat Rev Cancer (2012) 12(4):278–87. doi:10.1038/nrc3236
3. Pardoll DM. The blockade of immune checkpoints in cancer immunotherapy. Nat Rev Cancer (2012) 12(4):252–64. doi:10.1038/nrc3239
4. Levy S, Shoham T. The tetraspanin web modulates immune-signalling complexes. Nat Rev Immunol (2005) 5(2):136–48. doi:10.1038/nri1548
5. Zoller M. Tetraspanins: push and pull in suppressing and promoting metastasis. Nat Rev Cancer (2009) 9(1):40–55. doi:10.1038/nrc2543
6. Hemler ME. Tetraspanin proteins promote multiple cancer stages. Nat Rev Cancer (2014) 14(1):49–60. doi:10.1038/nrc3640
7. Hemler ME. Targeting of tetraspanin proteins – potential benefits and strategies. Nat Rev Drug Discov (2008) 7(9):747–58. doi:10.1038/nrd2659
8. Szala S, Kasai Y, Steplewski Z, Rodeck U, Koprowski H, Linnenbach AJ. Molecular cloning of cDNA for the human tumor-associated antigen CO-029 and identification of related transmembrane antigens. Proc Natl Acad Sci U S A (1990) 87(17):6833–7. doi:10.1073/pnas.87.17.6833
9. Hotta H, Ross AH, Huebner K, Isobe M, Wendeborn S, Chao MV, et al. Molecular cloning and characterization of an antigen associated with early stages of melanoma tumor progression. Cancer Res (1988) 48(11):2955–62.
10. Testa JE, Brooks PC, Lin JM, Quigley JP. Eukaryotic expression cloning with an antimetastatic monoclonal antibody identifies a tetraspanin (PETA-3/CD151) as an effector of human tumor cell migration and metastasis. Cancer Res (1999) 59(15):3812–20.
11. Dong JT, Lamb PW, Rinker-Schaeffer CW, Vukanovic J, Ichikawa T, Isaacs JT, et al. KAI1, a metastasis suppressor gene for prostate cancer on human chromosome 11p11.2. Science (1995) 268(5212):884–6. doi:10.1126/science.7754374
12. Feng J, Huang C, Wren JD, Wang DW, Yan J, Zhang J, et al. Tetraspanin CD82: a suppressor of solid tumors and a modulator of membrane heterogeneity. Cancer Metastasis Rev (2015) 34(4):619–33. doi:10.1007/s10555-015-9585-x
13. Lee JH, Seo YW, Park SR, Kim YJ, Kim KK. Expression of a splice variant of KAI1, a tumor metastasis suppressor gene, influences tumor invasion and progression. Cancer Res (2003) 63(21):7247–55.
14. Xu-Monette ZY, Li L, Byrd JC, Jabbar KJ, Manyam GC, Maria de Winde C, et al. Assessment of CD37 B-cell antigen and cell of origin significantly improves risk prediction in diffuse large B-cell lymphoma. Blood (2016) 128(26):3083–100. doi:10.1182/blood-2016-05-715094
15. de Winde CM, Veenbergen S, Young KH, Xu-Monette ZY, Wang XX, Xia Y, et al. Tetraspanin CD37 protects against the development of B cell lymphoma. J Clin Invest (2016) 126(2):653–66. doi:10.1172/JCI81041
16. Zeng P, Wang YH, Si M, Gu JH, Li P, Lu PH, et al. Tetraspanin CD151 as an emerging potential poor prognostic factor across solid tumors: a systematic review and meta-analysis. Oncotarget (2017) 8(3):5592–602. doi:10.18632/oncotarget.13532
17. Takeda Y, Li Q, Kazarov AR, Epardaud M, Elpek K, Turley SJ, et al. Diminished metastasis in tetraspanin CD151-knockout mice. Blood (2011) 118(2):464–72. doi:10.1182/blood-2010-08-302240
18. Copeland BT, Bowman MJ, Ashman LK. Genetic ablation of the tetraspanin CD151 reduces spontaneous metastatic spread of prostate cancer in the TRAMP model. Mol Cancer Res (2013) 11(1):95–105. doi:10.1158/1541-7786.MCR-12-0468
19. Li Q, Yang XH, Xu F, Sharma C, Wang HX, Knoblich K, et al. Tetraspanin CD151 plays a key role in skin squamous cell carcinoma. Oncogene (2013) 32(14):1772–83. doi:10.1038/onc.2012.205
20. Sachs N, Secades P, van Hulst L, Song JY, Sonnenberg A. Reduced susceptibility to two-stage skin carcinogenesis in mice with epidermis-specific deletion of CD151. J Invest Dermatol (2014) 134(1):221–8. doi:10.1038/jid.2013.280
21. Hong IK, Byun HJ, Lee J, Jin YJ, Wang SJ, Jeoung DI, et al. The tetraspanin CD81 protein increases melanoma cell motility by up-regulating metalloproteinase MT1-MMP expression through the pro-oncogenic Akt-dependent Sp1 activation signaling pathways. J Biol Chem (2014) 289(22):15691–704. doi:10.1074/jbc.M113.534206
22. Wang HX, Sharma C, Knoblich K, Granter SR, Hemler ME. EWI-2 negatively regulates TGF-beta signaling leading to altered melanoma growth and metastasis. Cell Res (2015) 25(3):370–85. doi:10.1038/cr.2015.17
23. Vences-Catalan F, Rajapaksa R, Srivastava MK, Marabelle A, Kuo CC, Levy R, et al. Tetraspanin CD81 promotes tumor growth and metastasis by modulating the functions of T regulatory and myeloid-derived suppressor cells. Cancer Res (2015) 75(21):4517–26. doi:10.1158/0008-5472.CAN-15-1021
24. Vences-Catalan F, Rajapaksa R, Srivastava MK, Marabelle A, Kuo CC, Levy R, et al. Tetraspanin CD81, a modulator of immune suppression in cancer and metastasis. Oncoimmunology (2016) 5(5):e1120399. doi:10.1080/2162402X.2015.1120399
25. Seu L, Tidwell C, Timares L, Duverger A, Wagner FH, Goepfert PA, et al. CD151 expression is associated with a hyperproliferative T cell phenotype. J Immunol (2017) 199(9):3336–47. doi:10.4049/jimmunol.1700648
26. Park CS, Kim TK, Kim HG, Kim YJ, Jeoung MH, Lee WR, et al. Therapeutic targeting of tetraspanin8 in epithelial ovarian cancer invasion and metastasis. Oncogene (2016) 35(34):4540–8. doi:10.1038/onc.2015.520
27. Rodia MT, Ugolini G, Mattei G, Montroni I, Zattoni D, Ghignone F, et al. Systematic large-scale meta-analysis identifies a panel of two mRNAs as blood biomarkers for colorectal cancer detection. Oncotarget (2016) 7(21):30295–306. doi:10.18632/oncotarget.8108
28. Murayama Y, Oritani K, Tsutsui S. Novel CD9-targeted therapies in gastric cancer. World J Gastroenterol (2015) 21(11):3206–13. doi:10.3748/wjg.v21.i11.3206
29. Huan J, Gao Y, Xu J, Sheng W, Zhu W, Zhang S, et al. Overexpression of CD9 correlates with tumor stage and lymph node metastasis in esophageal squamous cell carcinoma. Int J Clin Exp Pathol (2015) 8(3):3054–61.
30. Copeland BT, Bowman MJ, Boucheix C, Ashman LK. Knockout of the tetraspanin Cd9 in the TRAMP model of de novo prostate cancer increases spontaneous metastases in an organ-specific manner. Int J Cancer (2013) 133(8):1803–12. doi:10.1002/ijc.28204
31. Hemler ME. Tetraspanin functions and associated microdomains. Nat Rev Mol Cell Biol (2005) 6(10):801–11. doi:10.1038/nrm1736
32. Sincock PM, Fitter S, Parton RG, Berndt MC, Gamble JR, Ashman LK. PETA-3/CD151, a member of the transmembrane 4 superfamily, is localised to the plasma membrane and endocytic system of endothelial cells, associates with multiple integrins and modulates cell function. J Cell Sci (1999) 112(Pt 6):833–44.
33. Kazarov AR, Yang X, Stipp CS, Sehgal B, Hemler ME. An extracellular site on tetraspanin CD151 determines alpha 3 and alpha 6 integrin-dependent cellular morphology. J Cell Biol (2002) 158(7):1299–309. doi:10.1083/jcb.200204056
34. Winterwood NE, Varzavand A, Meland MN, Ashman LK, Stipp CS. A critical role for tetraspanin CD151 in alpha3beta1 and alpha6beta4 integrin-dependent tumor cell functions on laminin-5. Mol Biol Cell (2006) 17(6):2707–21. doi:10.1091/mbc.e05-11-1042
35. Yang XH, Richardson AL, Torres-Arzayus MI, Zhou P, Sharma C, Kazarov AR, et al. CD151 accelerates breast cancer by regulating alpha 6 integrin function, signaling, and molecular organization. Cancer Res (2008) 68(9):3204–13. doi:10.1158/0008-5472.CAN-07-2949
36. Gustafson-Wagner E, Stipp CS. The CD9/CD81 tetraspanin complex and tetraspanin CD151 regulate alpha3beta1 integrin-dependent tumor cell behaviors by overlapping but distinct mechanisms. PLoS One (2013) 8(4):e61834. doi:10.1371/journal.pone.0061834
37. El Kharbili M, Robert C, Witkowski T, Danty-Berger E, Barbollat-Boutrand L, Masse I, et al. Tetraspanin 8 is a novel regulator of ILK-driven beta1 integrin adhesion and signaling in invasive melanoma cells. Oncotarget (2017) 8(10):17140–55. doi:10.18632/oncotarget.15084
38. Schroder HM, Hoffmann SC, Hecker M, Korff T, Ludwig T. The tetraspanin network modulates MT1-MMP cell surface trafficking. Int J Biochem Cell Biol (2013) 45(6):1133–44. doi:10.1016/j.biocel.2013.02.020
39. Lafleur MA, Xu D, Hemler ME. Tetraspanin proteins regulate membrane type-1 matrix metalloproteinase-dependent pericellular proteolysis. Mol Biol Cell (2009) 20(7):2030–40. doi:10.1091/mbc.E08-11-1149
40. Herr MJ, Kotha J, Hagedorn N, Smith B, Jennings LK. Tetraspanin CD9 promotes the invasive phenotype of human fibrosarcoma cells via upregulation of matrix metalloproteinase-9. PLoS One (2013) 8(6):e67766. doi:10.1371/journal.pone.0067766
41. Saito Y, Tachibana I, Takeda Y, Yamane H, He P, Suzuki M, et al. Absence of CD9 enhances adhesion-dependent morphologic differentiation, survival, and matrix metalloproteinase-2 production in small cell lung cancer cells. Cancer Res (2006) 66(19):9557–65. doi:10.1158/0008-5472.CAN-06-1131
42. Yanez-Mo M, Barreiro O, Gonzalo P, Batista A, Megias D, Genis L, et al. MT1-MMP collagenolytic activity is regulated through association with tetraspanin CD151 in primary endothelial cells. Blood (2008) 112(8):3217–26. doi:10.1182/blood-2008-02-139394
43. Shi GM, Ke AW, Zhou J, Wang XY, Xu Y, Ding ZB, et al. CD151 modulates expression of matrix metalloproteinase 9 and promotes neoangiogenesis and progression of hepatocellular carcinoma. Hepatology (2010) 52(1):183–96. doi:10.1002/hep.23661
44. Hong IK, Jin YJ, Byun HJ, Jeoung DI, Kim YM, Lee H. Homophilic interactions of tetraspanin CD151 up-regulate motility and matrix metalloproteinase-9 expression of human melanoma cells through adhesion-dependent c-Jun activation signaling pathways. J Biol Chem (2006) 281(34):24279–92. doi:10.1074/jbc.M601209200
45. Yue S, Mu W, Zoller M. Tspan8 and CD151 promote metastasis by distinct mechanisms. Eur J Cancer (2013) 49(13):2934–48. doi:10.1016/j.ejca.2013.03.032
46. Seubert B, Cui H, Simonavicius N, Honert K, Schafer S, Reuning U, et al. Tetraspanin CD63 acts as a pro-metastatic factor via beta-catenin stabilization. Int J Cancer (2015) 136(10):2304–15. doi:10.1002/ijc.29296
47. Yanez-Mo M, Sanchez-Madrid F, Cabanas C. Membrane proteases and tetraspanins. Biochem Soc Trans (2011) 39(2):541–6. doi:10.1042/BST0390541
48. Matthews AL, Szyroka J, Collier R, Noy PJ, Tomlinson MG. Scissor sisters: regulation of ADAM10 by the TspanC8 tetraspanins. Biochem Soc Trans (2017) 45(3):719–30. doi:10.1042/BST20160290
49. Saint-Pol J, Eschenbrenner E, Dornier E, Boucheix C, Charrin S, Rubinstein E. Regulation of the trafficking and the function of the metalloprotease ADAM10 by tetraspanins. Biochem Soc Trans (2017) 45(4):937–44. doi:10.1042/BST20160296
50. Dunn CD, Sulis ML, Ferrando AA, Greenwald I. A conserved tetraspanin subfamily promotes Notch signaling in Caenorhabditis elegans and in human cells. Proc Natl Acad Sci U S A (2010) 107(13):5907–12. doi:10.1073/pnas.1001647107
51. Dornier E, Coumailleau F, Ottavi JF, Moretti J, Boucheix C, Mauduit P, et al. TspanC8 tetraspanins regulate ADAM10/kuzbanian trafficking and promote Notch activation in flies and mammals. J Cell Biol (2012) 199(3):481–96. doi:10.1083/jcb.201201133
52. Fang T, Lin J, Wang Y, Chen G, Huang J, Chen J, et al. Tetraspanin-8 promotes hepatocellular carcinoma metastasis by increasing ADAM12m expression. Oncotarget (2016) 7(26):40630–43. doi:10.18632/oncotarget.9769
53. Sadej R, Romanska H, Kavanagh D, Baldwin G, Takahashi T, Kalia N, et al. Tetraspanin CD151 regulates transforming growth factor beta signaling: implication in tumor metastasis. Cancer Res (2010) 70(14):6059–70. doi:10.1158/0008-5472.CAN-09-3497
54. Zuidscherwoude M, Gottfert F, Dunlock VM, Figdor CG, van den Bogaart G, van Spriel AB. The tetraspanin web revisited by super-resolution microscopy. Sci Rep (2015) 5:12201. doi:10.1038/srep12201
55. Haeuw JF, Goetsch L, Bailly C, Corvaia N. Tetraspanin CD151 as a target for antibody-based cancer immunotherapy. Biochem Soc Trans (2011) 39(2):553–8. doi:10.1042/BST0390553
56. Gutierrez-Lopez MD, Ovalle S, Yanez-Mo M, Sanchez-Sanchez N, Rubinstein E, Olmo N, et al. A functionally relevant conformational epitope on the CD9 tetraspanin depends on the association with activated beta1 integrin. J Biol Chem (2003) 278(1):208–18. doi:10.1074/jbc.M207805200
57. Ovalle S, Gutierrez-Lopez MD, Olmo N, Turnay J, Lizarbe MA, Majano P, et al. The tetraspanin CD9 inhibits the proliferation and tumorigenicity of human colon carcinoma cells. Int J Cancer (2007) 121(10):2140–52. doi:10.1002/ijc.22902
58. Longo N, Yanez-Mo M, Mittelbrunn M, de la Rosa G, Munoz ML, Sanchez-Madrid F, et al. Regulatory role of tetraspanin CD9 in tumor-endothelial cell interaction during transendothelial invasion of melanoma cells. Blood (2001) 98(13):3717–26. doi:10.1182/blood.V98.13.3717
59. Boucheix C, Soria C, Mirshahi M, Soria J, Perrot JY, Fournier N, et al. Characteristics of platelet aggregation induced by the monoclonal antibody ALB6 (acute lymphoblastic leukemia antigen p 24). Inhibition of aggregation by ALB6Fab. FEBS Lett (1983) 161(2):289–95. doi:10.1016/0014-5793(83)81027-8
60. Murayama Y, Miyagawa J, Oritani K, Yoshida H, Yamamoto K, Kishida O, et al. CD9-mediated activation of the p46 Shc isoform leads to apoptosis in cancer cells. J Cell Sci (2004) 117(Pt 15):3379–88. doi:10.1242/jcs.01201
61. Nakamoto T, Murayama Y, Oritani K, Boucheix C, Rubinstein E, Nishida M, et al. A novel therapeutic strategy with anti-CD9 antibody in gastric cancers. J Gastroenterol (2009) 44(9):889–96. doi:10.1007/s00535-009-0081-3
62. Geary SM, Cambareri AC, Sincock PM, Fitter S, Ashman LK. Differential tissue expression of epitopes of the tetraspanin CD151 recognised by monoclonal antibodies. Tissue Antigens (2001) 58(3):141–53. doi:10.1034/j.1399-0039.2001.580301.x
63. Yamada M, Tamura Y, Sanzen N, Sato-Nishiuchi R, Hasegawa H, Ashman LK, et al. Probing the interaction of tetraspanin CD151 with integrin alpha 3 beta 1 using a panel of monoclonal antibodies with distinct reactivities toward the CD151-integrin alpha 3 beta 1 complex. Biochem J (2008) 415(3):417–27. doi:10.1042/BJ20071625
64. Palmer TD, Martinez CH, Vasquez C, Hebron KE, Jones-Paris C, Arnold SA, et al. Integrin-free tetraspanin CD151 can inhibit tumor cell motility upon clustering and is a clinical indicator of prostate cancer progression. Cancer Res (2014) 74(1):173–87. doi:10.1158/0008-5472.CAN-13-0275
65. Zijlstra A, Lewis J, Degryse B, Stuhlmann H, Quigley JP. The inhibition of tumor cell intravasation and subsequent metastasis via regulation of in vivo tumor cell motility by the tetraspanin CD151. Cancer Cell (2008) 13(3):221–34. doi:10.1016/j.ccr.2008.01.031
66. Kohno M, Hasegawa H, Miyake M, Yamamoto T, Fujita S. CD151 enhances cell motility and metastasis of cancer cells in the presence of focal adhesion kinase. Int J Cancer (2002) 97(3):336–43. doi:10.1002/ijc.1605
67. Yanez-Mo M, Alfranca A, Cabanas C, Marazuela M, Tejedor R, Ursa MA, et al. Regulation of endothelial cell motility by complexes of tetraspan molecules CD81/TAPA-1 and CD151/PETA-3 with alpha3 beta1 integrin localized at endothelial lateral junctions. J Cell Biol (1998) 141(3):791–804. doi:10.1083/jcb.141.3.791
68. Ailane N, Greco C, Zhu Y, Sala-Valdes M, Billard M, Casal I, et al. Effect of an anti-human Co-029/tspan8 mouse monoclonal antibody on tumor growth in a nude mouse model. Front Physiol (2014) 5:364. doi:10.3389/fphys.2014.00364
69. Kim TK, Park CS, Jeoung MH, Lee WR, Go NK, Choi JR, et al. Generation of a human antibody that inhibits TSPAN8-mediated invasion of metastatic colorectal cancer cells. Biochem Biophys Res Commun (2015) 468(4):774–80. doi:10.1016/j.bbrc.2015.11.031
70. Maisonial-Besset A, Witkowski T, Navarro-Teulon I, Berthier-Vergnes O, Fois G, Zhu Y, et al. Tetraspanin 8 (TSPAN 8) as a potential target for radio-immunotherapy of colorectal cancer. Oncotarget (2017) 8(13):22034–47. doi:10.18632/oncotarget.15787
71. de Goeij BE, Vink T, Ten Napel H, Breij EC, Satijn D, Wubbolts R, et al. Efficient payload delivery by a bispecific antibody-drug conjugate targeting HER2 and CD63. Mol Cancer Ther (2016) 15(11):2688–97. doi:10.1158/1535-7163.MCT-16-0364
72. Pols MS, Klumperman J. Trafficking and function of the tetraspanin CD63. Exp Cell Res (2009) 315(9):1584–92. doi:10.1016/j.yexcr.2008.09.020
73. Andreu Z, Yanez-Mo M. Tetraspanins in extracellular vesicle formation and function. Front Immunol (2014) 5:442. doi:10.3389/fimmu.2014.00442
74. Lu J, Li J, Liu S, Wang T, Ianni A, Bober E, et al. Exosomal tetraspanins mediate cancer metastasis by altering host microenvironment. Oncotarget (2017) 8(37):62803–15. doi:10.18632/oncotarget.19119
75. Wang J, Zheng Y, Zhao M. Exosome-based cancer therapy: implication for targeting cancer stem cells. Front Pharmacol (2016) 7:533. doi:10.3389/fphar.2016.00533
76. Perez-Hernandez D, Gutierrez-Vazquez C, Jorge I, Lopez-Martin S, Ursa A, Sanchez-Madrid F, et al. The intracellular interactome of tetraspanin-enriched microdomains reveals their function as sorting machineries toward exosomes. J Biol Chem (2013) 288(17):11649–61. doi:10.1074/jbc.M112.445304
77. Luga V, Zhang L, Viloria-Petit AM, Ogunjimi AA, Inanlou MR, Chiu E, et al. Exosomes mediate stromal mobilization of autocrine Wnt-PCP signaling in breast cancer cell migration. Cell (2012) 151(7):1542–56. doi:10.1016/j.cell.2012.11.024
78. Rana S, Yue S, Stadel D, Zoller M. Toward tailored exosomes: the exosomal tetraspanin web contributes to target cell selection. Int J Biochem Cell Biol (2012) 44(9):1574–84. doi:10.1016/j.biocel.2012.06.018
79. Gilligan KE, Dwyer RM. Engineering exosomes for cancer therapy. Int J Mol Sci (2017) 18(6):E1122. doi:10.3390/ijms18061122
80. Hurwitz SN, Rider MA, Bundy JL, Liu X, Singh RK, Meckes DG Jr. Proteomic profiling of NCI-60 extracellular vesicles uncovers common protein cargo and cancer type-specific biomarkers. Oncotarget (2016) 7(52):86999–7015. doi:10.18632/oncotarget.13569
81. Sina AA, Vaidyanathan R, Dey S, Carrascosa LG, Shiddiky MJ, Trau M. Real time and label free profiling of clinically relevant exosomes. Sci Rep (2016) 6:30460. doi:10.1038/srep30460
82. Nishida-Aoki N, Tominaga N, Takeshita F, Sonoda H, Yoshioka Y, Ochiya T. Disruption of circulating extracellular vesicles as a novel therapeutic strategy against cancer metastasis. Mol Ther (2017) 25(1):181–91. doi:10.1016/j.ymthe.2016.10.009
83. Beckwith KA, Byrd JC, Muthusamy N. Tetraspanins as therapeutic targets in hematological malignancy: a concise review. Front Physiol (2015) 6:91. doi:10.3389/fphys.2015.00091
84. de Winde CM, Elfrink S, van Spriel AB. Novel insights into membrane targeting of B cell lymphoma. Trends Cancer (2017) 3(6):442–53. doi:10.1016/j.trecan.2017.04.006
Keywords: cancer, immunotherapy, monoclonal antibodies, bench, bedside
Citation: Vences-Catalán F and Levy S (2018) Immune Targeting of Tetraspanins Involved in Cell Invasion and Metastasis. Front. Immunol. 9:1277. doi: 10.3389/fimmu.2018.01277
Received: 09 March 2018; Accepted: 22 May 2018;
Published: 12 June 2018
Edited by:
María Yáñez-Mó, Universidad Autónoma de Madrid, SpainReviewed by:
Andries Zijlstra, Vanderbilt University, United StatesHsi-Hsien Lin, Chang Gung University, Taiwan
Copyright: © 2018 Vences-Catalán and Levy. This is an open-access article distributed under the terms of the Creative Commons Attribution License (CC BY). The use, distribution or reproduction in other forums is permitted, provided the original author(s) and the copyright owner are credited and that the original publication in this journal is cited, in accordance with accepted academic practice. No use, distribution or reproduction is permitted which does not comply with these terms.
*Correspondence: Shoshana Levy, c2xldnlAc3RhbmZvcmQuZWR1