- 1Department of Dermatology/Allergology, University Medical Center Utrecht, Utrecht University, Utrecht, Netherlands
- 2Laboratory of Translational Immunology, University Medical Center Utrecht, Utrecht, Netherlands
- 3Division of Pharmacology, Faculty of Science, Utrecht Institute for Pharmaceutical Sciences, Utrecht University, Utrecht, Netherlands
- 4Nutricia Research, Immunology, Utrecht, Netherlands
Background: Dietary non-digestible oligosaccharides (NDOs) have a protective effect against allergic manifestations in children at risk. Dietary intervention with NDOs promotes the colonization of beneficial bacteria in the gut and enhances serum galectin-9 levels in mice and atopic children. Next to this, NDOs also directly affect immune cells and low amounts may reach the blood. We investigated whether pre-incubation of whole blood from peanut-allergic patients with NDOs or galectin-9 can affect basophil degranulation.
Methods: Heparinized blood samples from 15 peanut-allergic adult patients were pre-incubated with a mixture of short-chain galacto-oligosaccharides and long-chain fructo-oligosaccharides (scGOS/lcFOS), scFOS/lcFOS, or galectin-9 (1 or 5 µg/mL) at 37°C in the presence of IL-3 (0.75 ng/mL). After 2, 6, or 24 h, a basophil activation test was performed. Expression of FcεRI on basophils, plasma cytokine, and chemokine concentrations before degranulation were determined after 24 h.
Results: Pre-incubation with scGOS/lcFOS, scFOS/lcFOS, or galectin-9 reduced anti-IgE-mediated basophil degranulation. scFOS/lcFOS or 5 µg/mL galectin-9 also decreased peanut-specific basophil degranulation by approximately 20%, mainly in whole blood from female patients. Inhibitory effects were not related to diminished FcεRI expression on basophils. Galectin-9 was increased in plasma after pre-incubation with scGOS/lcFOS, and both NDOs and 5 µg/mL galectin-9 increased MCP-1 production.
Conclusion and clinical relevance: The prebiotic mixture scFOS/lcFOS and galectin-9 can contribute to decreased degranulation of basophils in vitro in peanut-allergic patients. The exact mechanism needs to be elucidated, but these NDOs might be useful in reducing allergic symptoms.
Introduction
In Westernized countries, the prevalence of food allergies has increased over the years and is still increasing (1, 2). The prevalence of food allergy is currently estimated between 6 and 10% (3). Among children, less is known about the prevalence, although peanut allergy is one of the most common food allergies. Allergic reactions develop as a result of a hampered tolerance mechanism toward harmless antigens (4). When patients are sensitized, B cells start to produce antigen-specific IgE molecules that can sensitize the high-affinity FcεRI on mast cell or basophils (5). Upon a second encounter with the specific allergen, these IgE molecules can crosslink and will induce degranulation of mast cells and basophils, leading to clinical symptoms due to the release of histamine and other mediators. Currently, there is no curative treatment available to re-establish tolerance against these harmless food antigens, although progress is made in terms of immunotherapy and dietary adjunct therapy with, for example, probiotics that can improve the efficacy of immunotherapy (6).
Previous research has indicated a role of prebiotic non-digestible oligosaccharides (NDOs) in decreasing the incidence of atopic dermatitis in children at risk of developing allergy (7–9). The exact mechanism of action of these NDOs is not fully understood, however, it is known that they can promote the colonization of beneficial bacteria in the gut, similar as human milk oligosaccharides (HMOs) in breast milk (10, 11). Whey-allergic mice receiving oral immunotherapy in combination with a diet of short- and long-chain fructo-oligosaccharides (scFOS/lcFOS) experienced enhanced serum galectin-9 levels (12). Galectin-9 is a soluble type lectin which can, among others, be released by intestinal epithelial cells. It can bind to carbohydrate moieties located on the heavy chains of IgE (13, 14), hereby suppressing degranulation of mast cells and basophils by the inhibition of the formation of the IgE-allergen complex, which could be abrogated in the presence of lactose (14, 15). In addition, galectin-9 can support tolerance via the induction of Tregs (15, 16). Next to induction of galectin-9, these NDOs might also have a direct effect on immune cells. Earlier research indicated that HMOs (normally present in concentrations of 5–23 g/L in human milk) could be traced with 13C labeling, HPLC, and other techniques in plasma and urine (17–20). Approximately 0.05–0.1% of these oligosaccharides could be traced back to plasma, while 4% was traced back in urine (18–21). In addition, a study with fructo-oligosaccharides demonstrated that FOS, and hereby most likely more prebiotic structures, could reach the plasma compartment and were excreted in the urine (21).
For this research, we were interested in both the direct and indirect (galectin-9) effects of NDOs on basophil degranulation in whole blood of peanut-allergic patients. Therefore, two different mixtures of NDOs were tested; short-chain galacto-oligosaccharides and long-chain fructo-oligosaccharides (scGOS/lcFOS) and scFOS/lcFOS. In addition, the effects of galectin-9 on basophil degranulation were assessed. Next to determining the effects on basophil degranulation, the expression of FcεRI on basophils and mediator release in whole blood exposed to NDOs or galectin-9 was determined.
Materials and Methods
Study Design and Study Population
Fifteen peanut-allergic patients (6 male and 9 female; age 18–50; mean 32) were recruited from the Department of Dermatology/Allergology at the University Medical Center Utrecht. Inclusion criteria consisted of a type I allergic reaction to peanut, previously confirmed by a positive double-blind placebo-controlled food challenge and serum peanut-specific IgE (Table 1). Exclusion criteria were pregnancy or the continuous use of systemic immune-suppressants, such as prednisone. Total IgE levels were measured by ELISA (Euroimmun, Lübeck). In addition, average basophil percentages are shown per patient (normal range 0–2%). All patients gave written informed consent before enrollment in the study. The study was reviewed and approved by the Ethics Committee of the University Medical Center Utrecht (NL51606.041.15).
Basophil Activation Test (BAT)
Whole heparinized blood was obtained from 15 peanut-allergic patients. Blood was incubated for 2, 6, or 24 h at 37°C with galectin-9 [1 µg/mL (~28 nM) or 5 µg/mL (~140 nM), R&D Systems, Minneapolis, MN, USA], 0.05% (w/v) of a 9:1 mixture of scGOS (Vivinal GOS, Borculo Domo, the Netherlands) and lcFOS (scGOS/lcFOS) (Raftiline HP, Orafti) or 0.05% of a 9:1 mixture of scFOS (Raftilose P95, Orafti) and lcFOS (scFOS/lcFOS). To maintain basophil viability, 0.75 ng/mL IL-3 (R&D Systems) was added to the blood during pre-incubation and control samples were included. After different pre-incubation periods, a BAT was performed. Basophils in the different blood samples were stimulated for 30 min with increasing concentrations of anti-IgE (0.1, 0.3, and 1 µg/mL, Vector Laboratories, Burlingame, CA, USA) or crude peanut extract (0.1, 0.3, 1, 3, 10, 100, and 1,000 ng/mL) in RPMI 1640 medium (Gibco, Life Technologies) supplemented with 1 ng/mL IL-3. Control samples included RMPI + IL-3 and formyl-methionyl-leucyl-phenylalanine (1 µM fMLP, Sigma-Aldrich). Leukocytes were stained with an antibody cocktail of CD45-PO (Life Technologies), CD123-FITC (BioLegend), HLA-DR-PB (BioLegend), CD63-PE (Monosan), CD41-PE-Cy7 (Beckman Coulter), and CD203c (BioLegend). Basophils were defined as CD45+ CD203c+ CD123+ and HLA-DR− CD41−, and degranulation was determined as CD63+ cells. Results are expressed as percentage of CD63+ cells. Per patient, the dose of peanut allergen or anti-IgE that induced maximal degranulation in the control sample was used to normalize the data.
Determination of FcεRI on the Cell Surface of Basophils
FcεRI expression was quantified in seven random patients using a QIFIKIT (Dako) according to the manufacturer’s protocol. In short, a small sample of blood was first incubated with a primary mouse monoclonal against FcεRI (clone CRA-1), or an isotype. CRA-1 binds to both the open and occupied FcεRI receptor (22), Next, the blood samples, set-up and calibration beads were labeled with an FITC-conjugated anti-mouse secondary antibody, followed by further staining of the samples with an antibody cocktail to identify basophils (CD45, CD123, HLA-DR, CD203c, and CD41). FcεRI expression was quantified based on the calibration curve from the calibration beads by Graphpad Prism 7.0. Values were calculated relative to the control sample.
Determination of Cytokines and Chemokines in Plasma Pre-Incubated Samples
Residual plasma was collected after pre-incubation of the blood samples. Mediators secreted by basophils or involved in basophil activation or degranulation were measured (IL-4 (23), IL-5 (24), GM-CSF (25), MCP-1 (26), MDC (27), and TARC [upregulated by basophil derived IL-4 and IL-13 (28)]). Chemoattractants or mediators in basophil degranulation Eotaxin-3 (29), RANTES (30), galectin-3 (31, 32), and galectin-9 (14) were measured in these plasma samples with a luminex assay, performed by the luminex facility located in the UMC Medical Center, Utrecht. Values were calculated relative to the control sample.
Statistical Analysis
Data are expressed as mean ± SEM. Statistical significance was analyzed with GraphPad Prism 7.0 software (GraphPad Software, San Diego, CA, USA). Normally distributed data were analyzed with a one-way repeated measures ANOVA followed by Bonferroni post hoc analysis. Data were considered significant when P < 0.05. Demographic data were analyzed with the non-parametric Mann–Whitney test. Correlation was determined with Pearson’s correlation coefficients.
Results
Time-Dependent Kinetics Basophil Degranulation Influenced by NDOs and Galectin-9
In a pilot experiment, kinetics of the effects of basophil degranulation pre-incubated by NDOs and galectin-9 were determined. A time curve was performed in 1 mL whole blood of three patients. Whole blood samples were pre-incubated for 2, 6, or 24 h at 37°C, with 0.05% scFOS/lcFOS, 1 µg/mL galectin-9 or were left untreated as control. Figure 1A shows the relative IgE-mediated degranulation of basophils of three patients. After 2 and/or 6 h, some decrease in IgE-mediated basophil degranulation and peanut-specific (Figure 1B) was observed in the pre-incubated samples. After 24 h, the differences between the pre-incubated and untreated control sample were most pronounced, therefore we continued with the 24 h pre-incubation.
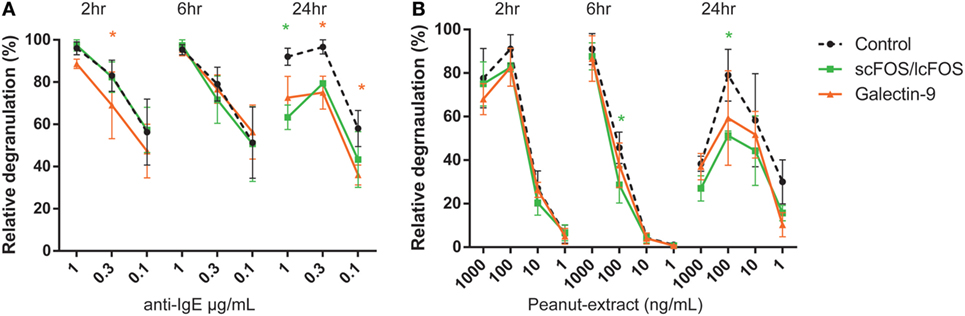
Figure 1. Time-dependent kinetics of non-digestible oligosaccharides or galectin-9 on anti-IgE or CPE induced basophil degranulation of a representative donor. Blood of peanut-allergic patients (n = 3) was pre-incubated for three different time-periods with short- and long-chain fructo-oligosaccharides (scFOS/lcFOS) or 1 µg/mL galectin-9 either in the aspecific (A) or peanut-specific (B) basophil activation test. Data represent n = 3 peanut-allergic patients. Significance is indicated compared to the control sample. *P < 0.05 by two-way ANOVA. Green: control compared to scFOS/lcFOS, orange: control compared to galectin-9.
Pre-Incubation of Blood With NDOs or Galectin-9 Decreases Basophil Degranulation in Peanut-Allergic Patients
Blood samples pre-incubated with NDOs or galectin-9 showed a reduced basophil degranulation after the IgE-mediated BAT compared to the controls after 24 h (Figure 2A). Pre-incubation with scGOS/lcFOS or scFOS/lcFOS resulted in an average decrease in anti-IgE-mediated basophil degranulation of 11 ± 3.5 or 13 ± 4%, respectively. Pre-incubation with 1 µg/mL galectin-9 resulted in an average decrease of 16 ± 6%, while the highest concentration of galectin-9 could reduce basophil degranulation on average with 30 ± 7%. In the peanut-specific BAT (Figure 2D) only scFOS/lcFOS and the highest dose of galectin-9 reduced basophil degranulation significantly compared to the control sample, with an average reduction of 20 ± 6% (scFOS/lcFOS) and 17 ± 9% (galectin-9). Not all patients were responsive to all pre-incubations, and also differences in response between the aspecific (anti-IgE) and peanut-specific BAT were observed in blood samples of individual patients. In addition, the oligosaccharides did not alter the fMLP-mediated degranulation, indicating an IgE-specific inhibition rather than a general inhibition (data not shown).
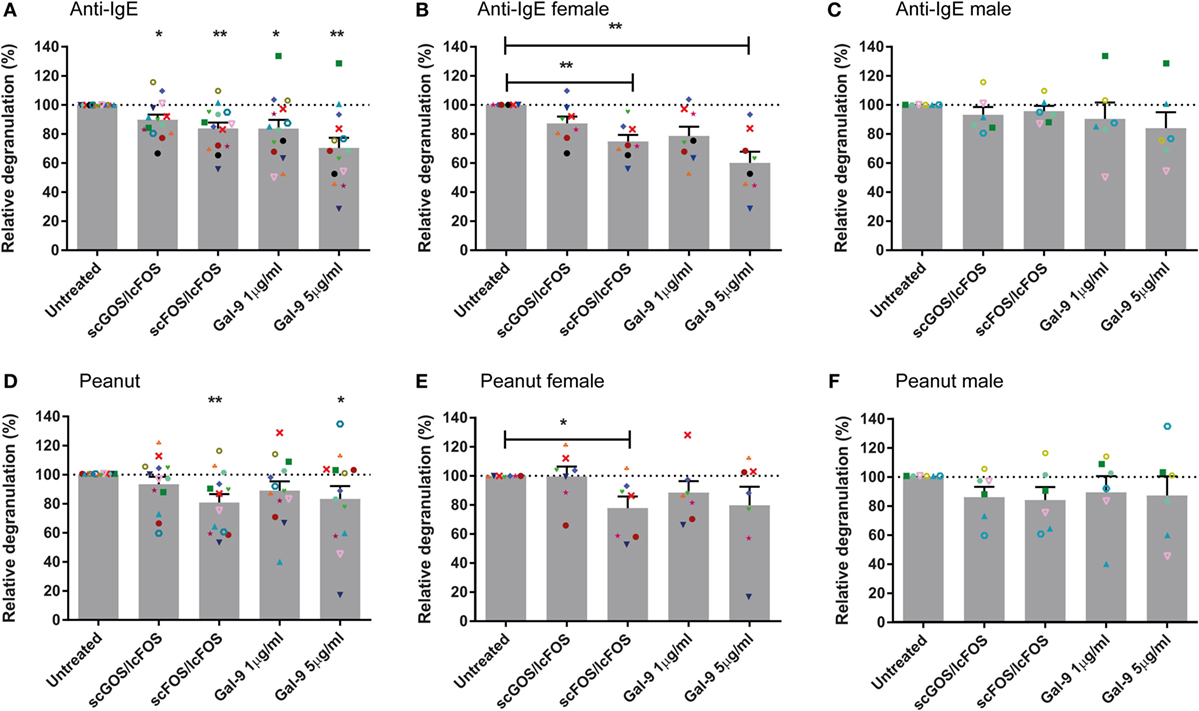
Figure 2. Reduced basophil degranulation after pre-incubation with non-digestible oligosaccharides (NDOs) or galectin-9. Pre-incubation of whole blood for 24 h with NDOs of galectin-9 resulted in a decrease in IgE-mediated basophil degranulation (A–C). Pre-incubation with short- and long-chain fructo-oligosaccharides (scFOS/lcFOS) and 5 µg/mL galectin-9 reduced peanut-specific basophil degranulation (D–F). Females tended to have a higher decrease in basophil degranulation than males after pre-incubation (B,C,E,F). Data represent the mean ± SEM of n = 15 peanut-allergic patients, two patients were unresponsive (<5% basophil degranulation) in the peanut-specific basophil activation test (BAT), one of these two patients was also non-responsive in the IgE-mediated BAT. *P < 0.05, **P < 0.01 by one-way ANOVA.
Pre-Incubation of Blood With NDOs or Galectin-9 Reduces Basophil Degranulation More Effective in Female Patients and Does Not Correlate With FcεRI Expression
Next to the role of pre-incubation or the specificity of the BAT (anti-IgE or peanut) on basophil degranulation, differences in degranulation were observed between male and female blood samples. Female blood samples pre-incubated with NDOs or galectin-9 showed less basophil degranulation compared to their control samples than males. This was observed in both the peanut-specific and the anti-IgE BAT (Figures 2B,C,E,F). In the IgE-mediated BAT, significant differences were observed between the female and male samples for scFOS/lcFOS (P < 0.01) and a similar trend for galectin-9, 5 µg/mL (P < 0.1). In addition, other demographic variables as indicated in Table 2 could not explain the differences between males and females, as they were not statistically different.
To determine whether scFOS/lcFOS or galectin-9 suppressed basophil degranulation via directly or indirectly affecting FcεRI expression, these expression levels on basophils were determined in blood samples of seven patients relative to the expression of this receptor on untreated positive control samples of the same donor (Figure 3). First, anti-IgE (Figure 3A) and peanut-specific basophil degranulation (Figure 3B) of blood pre-incubated with either scFOS/lcFOS or galectin-9 was determined as described earlier. In addition, quantitative expression of FcεRI on basophils was determined before basophil degranulation (Figure 3C). No correlation was observed between the difference in expression of FcεRI and the corresponding difference in basophil degranulation of the pre-incubated samples (Figure 3D). Therefore, the mechanism of action of NDOs and galectin-9 cannot be ascribed to effects on expression of FcεRI on the basophil cell surface.
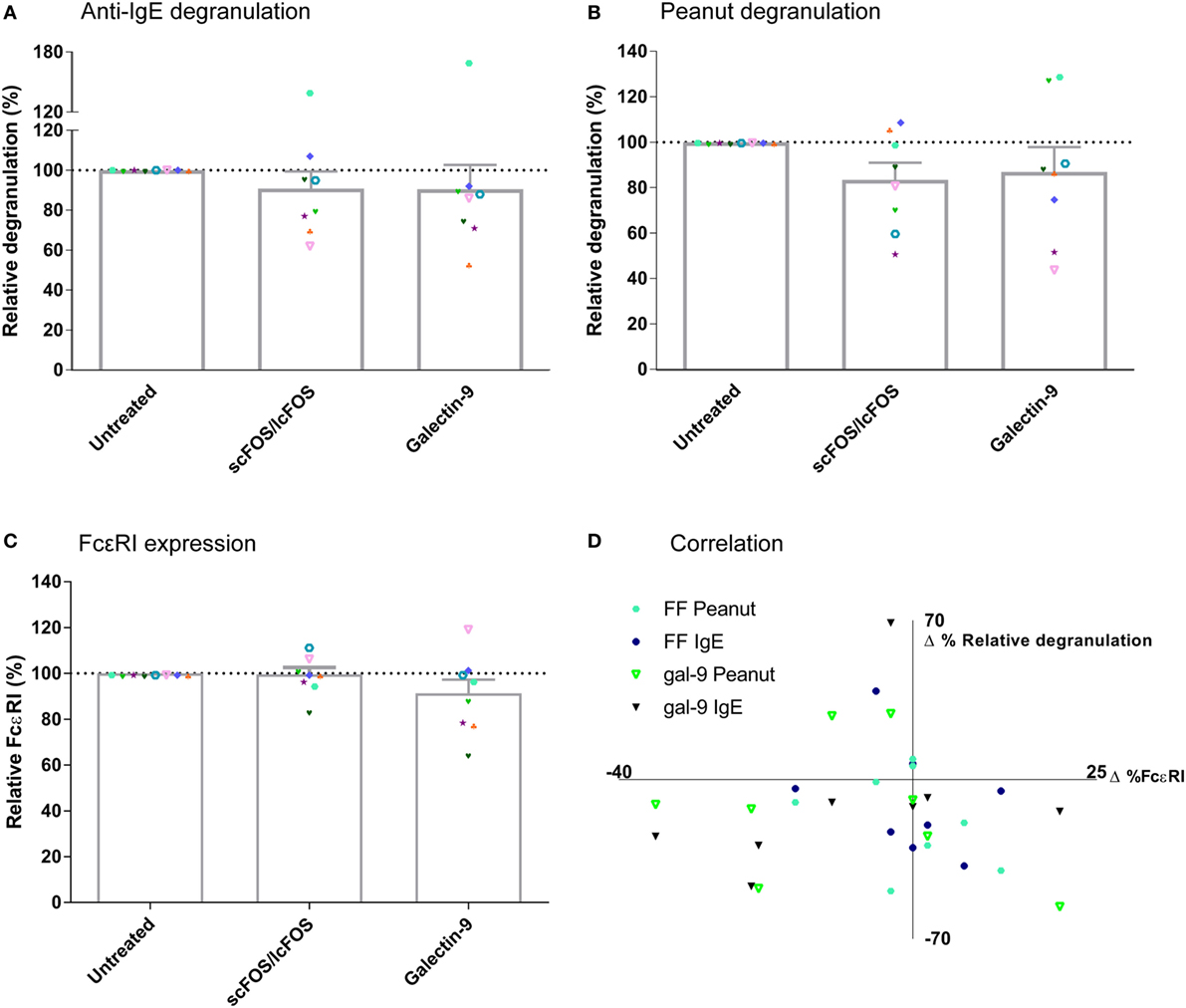
Figure 3. Correlation relative expression of FcεRI and basophil degranulation. Anti-IgE mediated and peanut-specific basophil degranulation after 24 h of pre-incubation with short- and long-chain fructo-oligosaccharides (scFOS/lcFOS) or galectin-9 (A,B). FcεRI expression on basophils was not decreased after pre-incubation with scFOS/lcFOS or 1 µg/mL galectin-9 (C). Δ% relative degranulation was calculated based on panels (A,B), whereas Δ%FcεRI was calculated based on panel (C), both relative to the control samples. No correlation was found between expression of ΔFcεRI on the cell surface and the corresponding Δbasophil degranulation (D). (FF = scFOS/lcFOS). Data represent n = 8 patients. Correlation was tested with Pearson correlation coefficient.
Luminex Analysis Plasma Samples
To determine whether NDO or galectin-9 pre-incubation of blood affects mediator secretion by cells, remaining plasma samples after the pre-incubation were collected and were analyzed on cytokine and chemokines levels. Table 3 indicates the mean baseline levels per mediator and the mean levels ± SEM when blood samples were pre-incubated with scGOS/lcFOS, scFOS/lcFOS, or galectin-9. IL-5 and GM-CSF were for several samples extrapolated from the standard curve or below the detection limit of the luminex assay, therefore no conclusions can be drawn on the contribution of these mediators in the observed decrease in basophil degranulation. Significant differences compared to the untreated control were found for galectin-9 and MCP-1 (CCL2) levels (Figure 4). Galectin-9 levels in blood pre-incubated with scGOS/lcFOS increased relative to the untreated control sample which was set to 100%, while pre-incubation with scFOS/lcFOS did not result in an increase (Figure 4A). Galectin-9 levels in the galectin-9 pre-incubated samples were above the detection limit of the luminex and are, therefore, not displayed. MCP-1 (CCL2) levels were increased in plasma samples after pre-incubation with scGOS/lcFOS, scFOS/lcFOS, and the highest dose of galectin-9 relative to untreated controls (Figure 4B). However, no correlations were found between levels of galectin-9, MCP-1, and anti-IgE or peanut-specific basophil degranulation (Figures 4C–F). Only a trend between galectin-9 and IgE-mediated basophil degranulation was observed for the control sample and after pre-incubation with scFOS/lcFOS (Figure 4C).
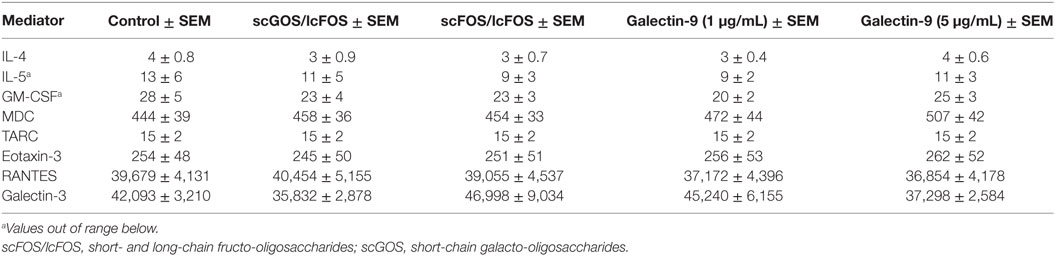
Table 3. Average concentration mediators (pg/mL) in blood plasma after 24 h of pre-incubation with non-digestible oligosaccharides.
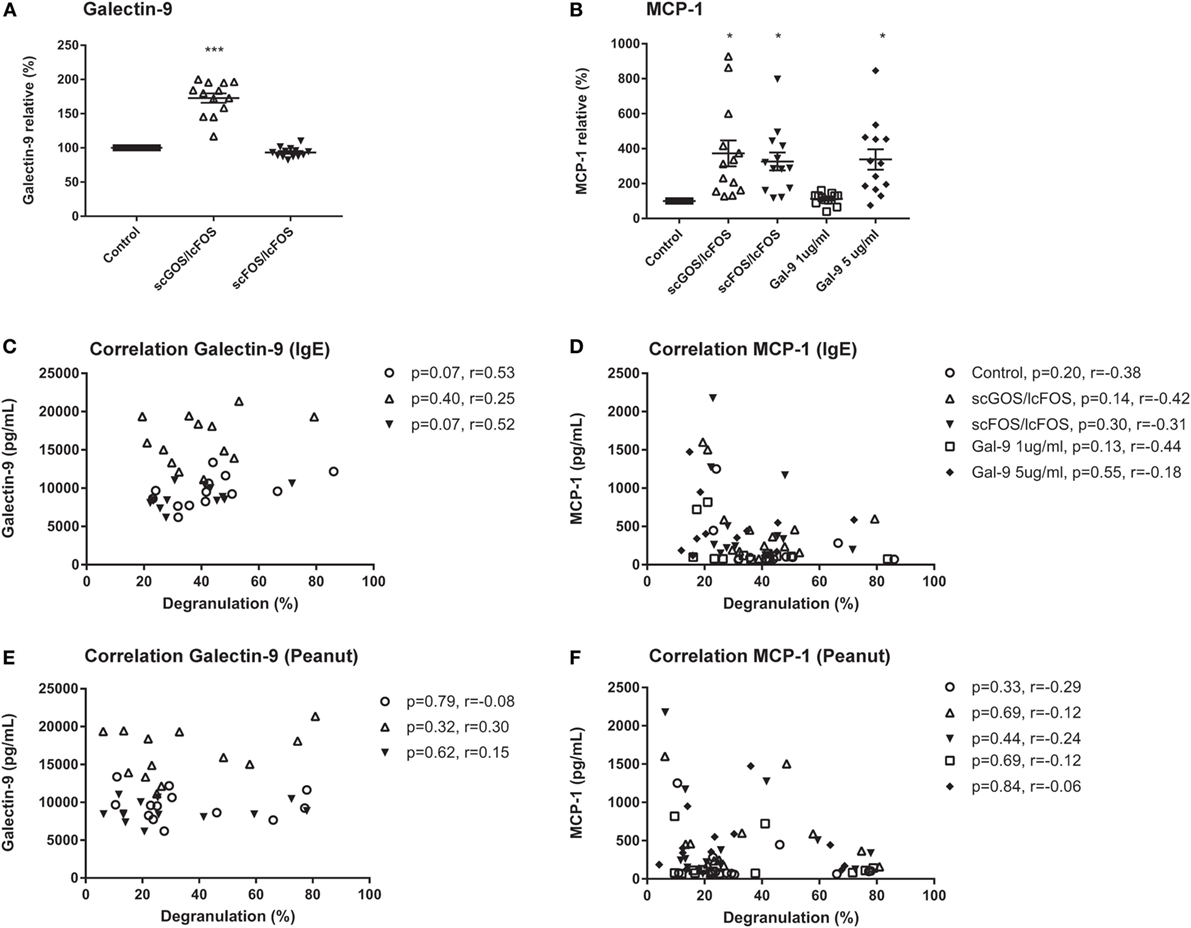
Figure 4. No correlation between relative basophil degranulation and galectin-9 or MCP-1 production. Galectin-9 increased in short-chain galacto-oligosaccharides and long-chain fructo-oligosaccharides (scGOS/lcFOS) pre-incubated samples, and were above the detection limit for galectin-9 treated samples (A). MCP-1 (CCL2) was increased after pre-incubation with both non-digestible oligosaccharides and 5 µg/mL galectin-9 (B). This was not correlated with anti-IgE or peanut-specific basophil degranulation (C–F). Data are represented as mean ± SEM of n = 15 patients. *P < 0.05, ***P < 0.001 by one-way ANOVA. Correlation was tested with Pearson correlation coefficient.
Discussion
Basophil degranulation is an important event in allergic reactions. This study demonstrates that IgE-mediated and peanut-specific basophil degranulation can be reduced by pre-incubation of blood with NDOs scGOS/lcFOS and scFOS/lcFOS, but also by their indirect product galectin-9, which can be enhanced amongst others under influence of NDOs (33). These effects on basophil degranulation were mainly observed in female peanut-allergic subjects. The reduction in basophil degranulation was not correlated with altered FcεRI expression levels on the basophil cell surface. The differences between males and females could not be related to other demographic variables. An explanation for differences between males and females might be hormone-related. While the exact working mechanism of hormones is not elucidated, estrogen receptors for instance are found on several immune subsets and are described to have effects on the allergic sensitization pathway including promotion of basophil degranulation (34–37). More differences in innate and adaptive immune responses between males and females have been studied and described, indicating that sex is an important parameter to consider for the observed differences in basophil degranulation (38). No other relations between the effects of NDOs and other demographic variables, for example age, were observed. However, we cannot exclude this, due to the limited number of patients and their inhomogeneous age distribution.
When blood was pre-incubated with scGOS/lcFOS, an increase in galectin-9 was observed. Soluble type lectin galectin-9 is a small (36 kDa) glycoprotein that can bind to glycans containing galactose and derivatives (39). The carbohydrate recognition domains (CRDs) can only fit galactose residues; other sugar residues do not fit into these CRDs due to steric hindrance (40). Interactions between galactose residues and galectin-9 can result in the formation of so-called lattices which play an important role in the regulation of immune responses (41). We hypothesize that pre-incubation with scGOS/lcFOS stimulates cells in blood, such as T-cells, B-cells, eosinophils, or basophils (14) to release galectin-9. This may result in the reduced basophil degranulation that was observed, since galectin-9 may have bound to IgE on the basophil cell surface, hereby hindering the formation of an IgE-antigen complex. Similar effects of IgE-binding capacities of (recombinant) galectin-9 have previously been described, and here galectin-9 was able to reduce mast cell degranulation in RBL-2H3 cells or HMC-1 cells, which was also explained by steric hindrance by galectin-9 (14, 42). In the allergen-specific BAT, basophil degranulation was not significantly decreased upon pre-incubation with scGOS/lcFOS and the lowest dose of galectin-9. This might be explained by the difference in size between anti-IgE (~150 kDa) and peanut allergens (~15–60 kDa). Peanut-allergens are smaller and have multiple IgE-binding epitopes (43), and therefore may circumvent the steric hindrance caused by galectin-9. The higher concentration of galectin-9 might overcome this steric hindrance in the peanut-specific BAT, while scFOS/lcFOS probably acts via a different mechanism than scGOS/lcFOS and galectins.
Although the concentration of galectin-9 was upregulated by scGOS/lcFOS to a concentration of 16 ng/mL, it is relatively low when compared to the recombinant galectin-9 used in this study (1 and 5 µg/mL). No data are available whether such low concentrations of galectin-9 could influence basophil degranulation. Studies that have been performed used higher concentrations, and indicated a dose-response curve for galectin-9 and subsequent degranulation (14, 42). However, these concentrations are always much higher than physiological galectin-9 concentrations in serum of healthy controls (5–12 ng/mL) (44). In addition, most studies performed on basophil degranulation are using recombinant galectin-9, which may also generate different responses than natural galectin-9 that was induced by scGOS/lcFOS. In summary, galectin-9 might be a contributing factor in the observed decrease in degranulation after pre-treatment with scGOS/lcFOS, but since there was no correlation between galectin-9 and degranulation and not all patients were responsive to scGOS/lcFOS, other factors are probably involved.
scFOS/lcFOS was most effective in reducing basophil degranulation in both the IgE-mediated and the peanut-specific BAT, with a similar reduction as 5 µg/mL galectin-9 (approximately 20%). In contrast to scGOS/lcFOS, scFOS/lcFOS did not affect plasma galectin-9 concentrations. This indicates that scFOS/lcFOS may exert its functions in a different manner than scGOS/lcFOS. One of the possible mechanisms by which scFOS/lcFOS may exert its effect is via modulation of FcεRI expression. However, no correlation was observed between scFOS/lcFOS induced effects on basophil degranulation and relative FcεRI expression on these cells.
In addition to differences in galectin-9 levels, changes in MCP-1 chemokine levels after pre-incubation were observed. MCP-1 is described in literature as a potent activator for basophil degranulation at concentrations of 3–10 nM and can be produced by various cell types (26, 45). This increase in MCP-1 is in contrast to our findings, since basophil degranulation was reduced in the pre-incubated blood samples. Both NDO mixtures and the highest concentration of galectin-9 increased MCP-1 levels in the blood plasma. However, these concentrations of MCP-1 are not elevated enough to induce basophil degranulation, since they are approximately 0.02–0.05 nM. In addition, no correlation was observed between MCP-1 levels and basophil degranulation, and no spontaneous CD63 release was observed after 2, 6, and 24 h of pre-incubation with NDOs or galectin-9 (data not shown). This might indicate that NDOs and galectin-9 can have direct effects on basophils. A previous study investigating the effects of galectin-9 on degranulation of HMC-1, a mast cell line that does not express FcεRI, also indicated an increase of MCP-1, which was dose-dependently correlated to increased galectin-9 levels (42). In this study, galectin-9 reduced PMA and ionomycin-induced degranulation of HMC-1 and induced the phosphorylation of the ERK1/2 pathway. Based on these results, it would be interesting to investigate whether activation of this ERK1/2 signaling pathway is also involved in the reduction in degranulation like we observed in human basophils.
This study was an in vitro model, and it is therefore important that the effects observed in this study are validated in vivo in humans. For future research, it would be interesting to focus on the effects of NDOs and other components on other cells subsets, such as eosinophils, that play an important role in allergy and also have degranulation capacities. In addition, this study was performed in whole blood and results shown may be caused by either a direct or indirect effect of NDOs. To be able to investigate the mechanism of action of these NDOs and galectin-9 on basophil degranulation, and to disseminate between these paths, it would be interesting to perform these experiments on isolated basophils of allergic patients, to exclude the involvement of surrounding cells.
In conclusion, this study indicated that NDOs can decrease basophil degranulation in an in vitro model. Indirect mediator galectin-9, which may be released by different kinds of cell types in response to exposure to NDOs, was also able to decrease basophil degranulation. No modification of the FcεRI receptor expression, cytokines, or chemokines was found in relation to this effect. The exact mechanism of action by which these NDOs can exert their immunomodulatory effects still needs to be elucidated, and in vivo validation of these results is necessary. However, it indicates that these NDOs are interesting dietary immunomodulatory agents, and might be useful as adjunct therapy in allergen-specific immunotherapy.
Ethics Statement
All patients gave written informed consent before enrollment in the study. The study was reviewed and approved by the Ethics Committee of the University Medical Center Utrecht (NL51606.041.15).
Author Contributions
SH, LW, and HO designed the experiments; AK assisted in recruitment of peanut-allergic patients; SH and CJ performed the experimental procedures. SH interpreted and collected data and drafted the manuscript. EK, JG, AK, LW, and HO contributed to data interpretation and critically revised the manuscript.
Conflict of Interest Statement
JG is partly employed by Danone Nutricia Research. SH, CJ, AK, EK, LW, and HO: no conflict of interest.
Funding
This research was funded by the STW/NWO “Open Technology Program,” project number 12652 NUTRALL (Nutrition-based approach to support antigen-specific immunotherapy for food allergies).
References
1. Prescott SL, Macaubas C, Smallacombe T, Holt BJ, Sly PD, Holt PG. Development of allergen-specific T-cell memory in atopic and normal children. Lancet (1999) 353(9148):196–200. doi:10.1016/S0140-6736(98)05104-6
2. Tang ML, Mullins RJ. Food allergy: is prevalence increasing? Intern Med J (2017) 47(3):256–61. doi:10.1111/imj.13362
3. Renz H, Allen KJ, Sicherer SH, Sampson HA, Lack G, Beyer K, et al. Food allergy. Nat Rev Dis Primers (2018) 4:17098. doi:10.1038/nrdp.2017.98
4. Perrier C, Corthesy B. Gut permeability and food allergies. Clin Exp Allergy (2011) 41(1):20–8. doi:10.1111/j.1365-2222.2010.03639.x
5. Valenta R, Hochwallner H, Linhart B, Pahr S. Food allergies: the basics. Gastroenterology (2015) 148(6):1120–31.e4. doi:10.1053/j.gastro.2015.02.006
6. Nurmatov U, Dhami S, Arasi S, Pajno GB, Fernandez-Rivas M, Muraro A, et al. Allergen immunotherapy for IgE-mediated food allergy: a systematic review and meta-analysis. Allergy (2017) 72(8):1133–47. doi:10.1111/all.13124
7. Arslanoglu S, Moro GE, Boehm G. Early supplementation of prebiotic oligosaccharides protects formula-fed infants against infections during the first 6 months of life. J Nutr (2007) 137(11):2420–4. doi:10.1093/jn/137.11.2420
8. Moro G, Arslanoglu S, Stahl B, Jelinek J, Wahn U, Boehm G. A mixture of prebiotic oligosaccharides reduces the incidence of atopic dermatitis during the first six months of age. Arch Dis Child (2006) 91(10):814–9. doi:10.1136/adc.2006.098251
9. van der Aa LB, van Aalderen WM, Heymans HS, Henk Sillevis Smitt J, Nauta AJ, Knippels LM, et al. Synbiotics prevent asthma-like symptoms in infants with atopic dermatitis. Allergy (2011) 66(2):170–7. doi:10.1111/j.1398-9995.2010.02416.x
10. Boehm G, Lidestri M, Casetta P, Jelinek J, Negretti F, Stahl B, et al. Supplementation of a bovine milk formula with an oligosaccharide mixture increases counts of faecal bifidobacteria in preterm infants. Arch Dis Child Fetal Neonatal Ed (2002) 86(3):F178–81. doi:10.1136/fn.86.3.F178
11. Boehm G, Moro G. Structural and functional aspects of prebiotics used in infant nutrition. J Nutr (2008) 138(9):1818S–28S. doi:10.1093/jn/138.9.1818S
12. Vonk MM, Diks MAP, Wagenaar L, Smit JJ, Pieters RHH, Garssen J, et al. Improved efficacy of oral immunotherapy using non-digestible oligosaccharides in a murine cow’s milk allergy model: a potential role for Foxp3+ regulatory T cells. Front Immunol (2017) 8:1230. doi:10.3389/fimmu.2017.01230
13. Baenziger J, Kornfeld S, Kochwa S. Structure of the carbohydrate units of IgE immunoglobulin. I. Over-all composition, glycopeptide isolation, and structure of the high mannose oligosaccharide unit. J Biol Chem (1974) 249(6):1889–96.
14. Niki T, Tsutsui S, Hirose S, Aradono S, Sugimoto Y, Takeshita K, et al. Galectin-9 is a high affinity IgE-binding lectin with anti-allergic effect by blocking IgE-antigen complex formation. J Biol Chem (2009) 284(47):32344–52. doi:10.1074/jbc.M109.035196
15. de Kivit S, Kraneveld AD, Knippels LM, van Kooyk Y, Garssen J, Willemsen LE. Intestinal epithelium-derived galectin-9 is involved in the immunomodulating effects of nondigestible oligosaccharides. J Innate Immun (2013) 5(6):625–38. doi:10.1159/000350515
16. Hu CC, Jeng WJ, Chen YC, Fang JH, Huang CH, Teng W, et al. Memory regulatory T cells increase only in inflammatory phase of chronic hepatitis B infection and related to galectin-9/Tim-3 interaction. Sci Rep (2017) 7(1):15280. doi:10.1038/s41598-017-15527-x
17. Ruhaak LR, Stroble C, Underwood MA, Lebrilla CB. Detection of milk oligosaccharides in plasma of infants. Anal Bioanal Chem (2014) 406(24):5775–84. doi:10.1007/s00216-014-8025-z
18. Obermeier S, Rudloff S, Pohlentz G, Lentze MJ, Kunz C. Secretion of 13C-labelled oligosaccharides into human milk and infant’s urine after an oral [13C]galactose load. Isotopes Environ Health Stud (1999) 35(1–2):119–25. doi:10.1080/10256019908234084
19. Goehring KC, Kennedy AD, Prieto PA, Buck RH. Direct evidence for the presence of human milk oligosaccharides in the circulation of breastfed infants. PLoS One (2014) 9(7):e101692. doi:10.1371/journal.pone.0101692
20. Rudloff S, Obermeier S, Borsch C, Pohlentz G, Hartmann R, Brösicke H, et al. Incorporation of orally applied (13)C-galactose into milk lactose and oligosaccharides. Glycobiology (2006) 16(6):477–87. doi:10.1093/glycob/cwj092
21. Prieto P. In vitro and clinical experiences with a human milk oligosaccharide, lacto-N-neoTetraose, and fructooligosaccharides. Foods Food Ingredients J Jpn (2005) 210(11):1018–30.
22. Suzukawa M, Hirai K, Iikura M, Nagase H, Komiya A, Yoshimura-Uchiyama C, et al. IgE- and FcepsilonRI-mediated migration of human basophils. Int Immunol (2005) 17(9):1249–55. doi:10.1093/intimm/dxh301
23. Motomura Y, Morita H, Moro K, Nakae S, Artis D, Endo TA, et al. Basophil-derived interleukin-4 controls the function of natural helper cells, a member of ILC2s, in lung inflammation. Immunity (2014) 40(5):758–71. doi:10.1016/j.immuni.2014.04.013
24. Bischoff SC, Brunner T, De Weck AL, Dahinden CA. Interleukin 5 modifies histamine release and leukotriene generation by human basophils in response to diverse agonists. J Exp Med (1990) 172(6):1577–82. doi:10.1084/jem.172.6.1577
25. Hirai K, Morita Y, Miyamoto T. Hemopoietic growth factors regulate basophil function and viability. Immunol Ser (1992) 57:587–600.
26. Bischoff SC, Krieger M, Brunner T, Dahinden CA. Monocyte chemotactic protein 1 is a potent activator of human basophils. J Exp Med (1992) 175(5):1271–5. doi:10.1084/jem.175.5.1271
27. Watanabe M, Satoh T, Yamamoto Y, Kanai Y, Karasuyama H, Yokozeki H. Overproduction of IgE induces macrophage-derived chemokine (CCL22) secretion from basophils. J Immunol (2008) 181(8):5653–9. doi:10.4049/jimmunol.181.8.5653
28. Borriello F, Longo M, Spinelli R, Pecoraro A, Granata F, Staiano RI, et al. IL-3 synergises with basophil-derived IL-4 and IL-13 to promote the alternative activation of human monocytes. Eur J Immunol (2015) 45(7):2042–51. doi:10.1002/eji.201445303
29. Petkovic V, Moghini C, Paoletti S, Uguccioni M, Gerber B. Eotaxin-3/CCL26 is a natural antagonist for CC chemokine receptors 1 and 5. A human chemokine with a regulatory role. J Biol Chem (2004) 279(22):23357–63. doi:10.1074/jbc.M309283200
30. Bischoff SC, Krieger M, Brunner T, Rot A, von Tscharner V, Baggiolini M, et al. RANTES and related chemokines activate human basophil granulocytes through different G protein-coupled receptors. Eur J Immunol (1993) 23(3):761–7. doi:10.1002/eji.1830230329
31. Bambouskova M, Polakovicova I, Halova I, Goel G, Draberova L, Bugajev V, et al. New regulatory roles of galectin-3 in high-affinity IgE receptor signaling. Mol Cell Biol (2016) 36(9):1366–82. doi:10.1128/MCB.00064-16
32. Blankestijn MA, Blom WM, Otten HG, Baumert JL, Taylor SL, Bruijnzeel-Koomen CA, et al. Specific IgE to Jug r 1 has no additional value compared with extract-based testing in diagnosing walnut allergy in adults. J Allergy Clin Immunol (2017) 139(2):688–90.e4. doi:10.1016/j.jaci.2016.07.026
33. de Kivit S, Saeland E, Kraneveld AD, van de Kant HJ, Schouten B, van Esch BC, et al. Galectin-9 induced by dietary synbiotics is involved in suppression of allergic symptoms in mice and humans. Allergy (2012) 67(3):343–52. doi:10.1111/j.1398-9995.2011.02771.x
34. van Esch BC, Abbring S, Diks MA, Dingjan GM, Harthoorn LF, Vos AP, et al. Post-sensitization administration of non-digestible oligosaccharides and Bifidobacterium breve M-16V reduces allergic symptoms in mice. Immun Inflamm Dis (2016) 4(2):155–65. doi:10.1002/iid3.101
35. Cai Y, Zhou J, Webb DC. Estrogen stimulates Th2 cytokine production and regulates the compartmentalisation of eosinophils during allergen challenge in a mouse model of asthma. Int Arch Allergy Immunol (2012) 158(3):252–60. doi:10.1159/000331437
36. Zaitsu M, Narita S, Lambert KC, Grady JJ, Estes DM, Curran EM, et al. Estradiol activates mast cells via a non-genomic estrogen receptor-alpha and calcium influx. Mol Immunol (2007) 44(8):1977–85. doi:10.1016/j.molimm.2006.09.030
37. Narita S, Goldblum RM, Watson CS, Brooks EG, Estes DM, Curran EM, et al. Environmental estrogens induce mast cell degranulation and enhance IgE-mediated release of allergic mediators. Environ Health Perspect (2007) 115(1):48–52. doi:10.1289/ehp.9378
38. Klein SL, Flanagan KL. Sex differences in immune responses. Nat Rev Immunol (2016) 16(10):626–38. doi:10.1038/nri.2016.90
39. Grigorian A, Torossian S, Demetriou M. T-cell growth, cell surface organization, and the galectin-glycoprotein lattice. Immunol Rev (2009) 230(1):232–46. doi:10.1111/j.1600-065X.2009.00796.x
40. Lobsanov YD, Gitt MA, Leffler H, Barondes SH, Rini JM. X-ray crystal structure of the human dimeric S-Lac lectin, L-14-II, in complex with lactose at 2.9-A resolution. J Biol Chem (1993) 268(36):27034–8.
41. Rabinovich GA, Toscano MA, Jackson SS, Vasta GR. Functions of cell surface galectin-glycoprotein lattices. Curr Opin Struct Biol (2007) 17(5):513–20. doi:10.1016/j.sbi.2007.09.002
42. Kojima R, Ohno T, Iikura M, Niki T, Hirashima M, Iwaya K, et al. Galectin-9 enhances cytokine secretion, but suppresses survival and degranulation, in human mast cell line. PLoS One (2014) 9(1):e86106. doi:10.1371/journal.pone.0086106
43. Shreffler WG, Lencer DA, Bardina L, Sampson HA. IgE and IgG4 epitope mapping by microarray immunoassay reveals the diversity of immune response to the peanut allergen, Ara h 2. J Allergy Clin Immunol (2005) 116(4):893–9. doi:10.1016/j.jaci.2005.06.033
44. He XW, Li WL, Li C, Liu P, Shen YG, Zhu M, et al. Serum levels of galectin-1, galectin-3, and galectin-9 are associated with large artery atherosclerotic stroke. Sci Rep (2017) 7:40994. doi:10.1038/srep40994
Keywords: basophil degranulation, food allergy, galectin-9, immunomodulation, non-digestible oligosaccharides
Citation: Hayen SM, den Hartog Jager CF, Knulst AC, Knol EF, Garssen J, Willemsen LEM and Otten HG (2018) Non-Digestible Oligosaccharides Can Suppress Basophil Degranulation in Whole Blood of Peanut-Allergic Patients. Front. Immunol. 9:1265. doi: 10.3389/fimmu.2018.01265
Received: 05 April 2018; Accepted: 22 May 2018;
Published: 11 June 2018
Edited by:
Caroline Elizabeth Childs, University of Southampton, United KingdomReviewed by:
Matteo A. Russo, IRCCS San Raffaele Pisana, ItalyClaudio Nicoletti, Università degli Studi di Firenze, Italy
Copyright: © 2018 Hayen, den Hartog Jager, Knulst, Knol, Garssen, Willemsen and Otten. This is an open-access article distributed under the terms of the Creative Commons Attribution License (CC BY). The use, distribution or reproduction in other forums is permitted, provided the original author(s) and the copyright owner are credited and that the original publication in this journal is cited, in accordance with accepted academic practice. No use, distribution or reproduction is permitted which does not comply with these terms.
*Correspondence: Simone M. Hayen, cy5tLmhheWVuLTImI3gwMDA0MDt1bWN1dHJlY2h0Lm5s