- 1Institute for Microbiology and Hygiene, Charité – Universitätsmedizin Berlin, Berlin, Germany
- 2Septomics Research Center, Jena University Hospital, Jena, Germany
- 3International Leibniz Research School for Microbial and Biomolecular Interactions, Leibniz Institute for Natural Product Research and Infection Biology/Hans Knöll Institute, Jena, Germany
Fungi, usually present as commensals, are a major cause of opportunistic infections in immunocompromised patients. Such infections, if not diagnosed or treated properly, can prove fatal. However, in most cases healthy individuals are able to avert the fungal attacks by mounting proper antifungal immune responses. Among the pattern recognition receptors (PRRs), C-type lectin receptors (CLRs) are the major players in antifungal immunity. CLRs can recognize carbohydrate ligands, such as β-glucans and mannans, which are mainly found on fungal cell surfaces. They induce proinflammatory immune reactions, including phagocytosis, oxidative burst, cytokine, and chemokine production from innate effector cells, as well as activation of adaptive immunity via Th17 responses. CLRs such as Dectin-1, Dectin-2, Mincle, mannose receptor (MR), and DC-SIGN can recognize many disease-causing fungi and also collaborate with each other as well as other PRRs in mounting a fungi-specific immune response. Mutations in these receptors affect the host response and have been linked to a higher risk in contracting fungal infections. This review focuses on how CLRs on various immune cells orchestrate the antifungal response and on the contribution of single nucleotide polymorphisms in these receptors toward the risk of developing such infections.
Introduction
Fungi are ubiquitously present in the environment and as commensals in humans; therefore, innate immunity needs to continuously work against the constant exposure. Pattern recognition receptors (PRRs) found on cell surfaces and as soluble forms in body fluids can recognize microbe-specific molecules; the so-called pathogen-associated molecular patterns (PAMPs). PRRs are expressed on immune cells and also on epithelial cells. The interaction of PRRs with PAMPs induces cell- and receptor-specific cellular host responses involving both, the innate and the acquired immune system. There are mainly four different kinds of PRR families, including the toll-like receptors, Nod-like receptors, C-type lectin receptors (CLRs), and RIG-I-like receptors (1). CLRs can recognize carbohydrates by virtue of having a C-type lectin-like domain (2). The domain consists of a conserved double loop structure and a long, structurally, and evolutionarily flexible loop which is involved in Ca2+-dependent carbohydrate binding (3). The characteristic fungal cell wall feature is its richness in carbohydrates and, therefore, they serve as the candidate targets for recognition by CLRs. It is widely accepted that CLRs play major role in antifungal immunity compared to other PRRs (1, 4).
C-type lectin receptors can be found as soluble forms in the serum and other body fluids or as transmembrane receptors on various immune cells, such as macrophages, dendritic cells (DCs), neutrophils, and various other cell types (Table 1) (5). Although CLRs have been divided based on their domain organization and phylogenetic features (3, 6), a broader classification of transmembrane receptors is possible based on the type of signaling mechanisms employed by them (7). One such mechanism is the signaling of CLRs via immunoreceptor tyrosine-based activation motifs (ITAMs). The ITAM motif (consensus sequence YxxL/I) recruits and phosphorylates Syk kinase on receptor ligation. Signaling via Syk typically leads to NF-κB activation via the complex consisting of caspase recruitment domain-containing protein 9 (CARD9) singalosome, a trimeric CARD9, B cell lymphoma/leukemia 10, and the mucosa-associated lymphoid tissue lymphoma translocation protein 1. Syk activation ultimately induces subsequent proinflammatory responses, as well as other responses, such as phagocytosis and reactive oxygen species (ROS) and reactive nitrogen species (RNS) production (8, 9). Some CLRs do not have their own cytoplasmic ITAMs. Such receptors couple with ITAM containing adaptor molecules like FcRγ to emanate signaling (10, 11). Dectin-1 is another non-classical CLR bearing a hemITAM motif (consensus sequence YxxL) and the ligand binding is Ca2+ independent (12). A second signaling mechanism with contrary effects to those elicited by ITAM signaling is employed by CLRs containing a cytoplasmic immunoreceptor tyrosine-based inhibitory motif (ITIM). Here, receptor ligation leads to the phosphorylation of tyrosine within the ITIM motif (consensus sequence I/V/L/SxYxxI/L/V) and the recruitment of SHP-1, SHP-2, and/or SHIP-1 phosphatases which exert an inhibitory effect by dampening the proinflammatory response (13, 14). Finally, some CLRs do not contain any known signaling motifs and, therefore, only little is known about their signaling mechanisms, such as LOX-1, MR, and langerin.
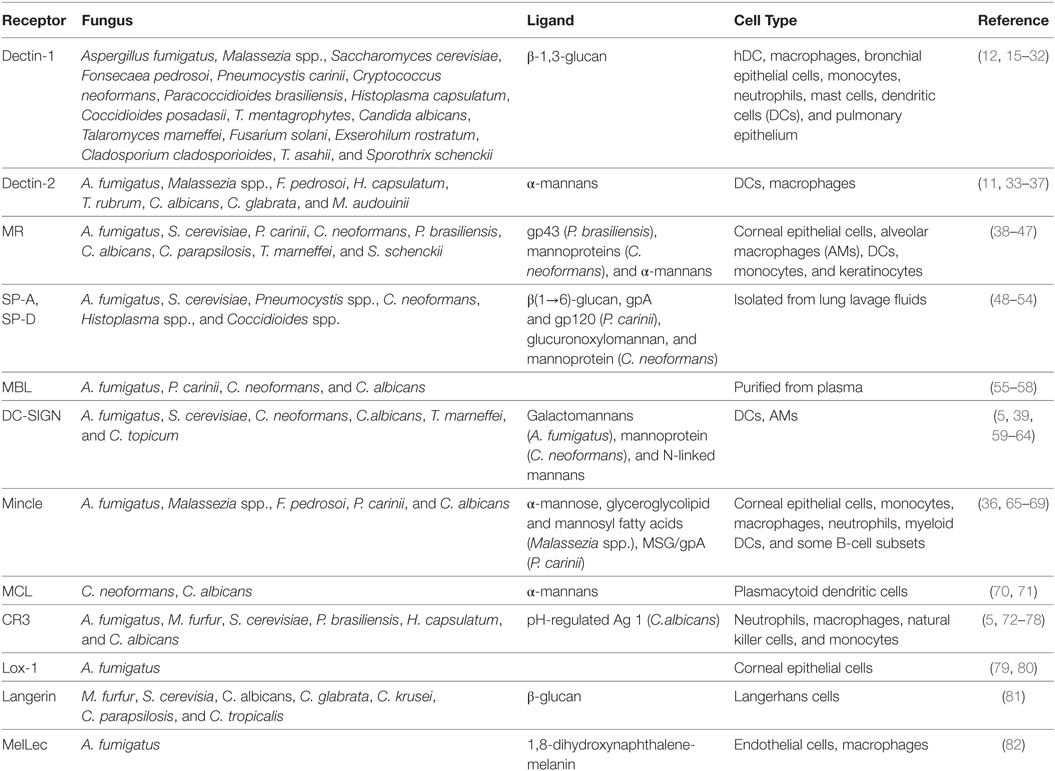
Table 1. C-type lectin receptors and their respective ligands involved in fungal recognition by different human cell types.
Fungal pathogens have a huge influence on human life, since they can infect the human body and cause various diseases from superficial infections to invasive and systemic infections. Infections of the skin and nails are the most common fungal diseases which affect ~25% of the general population worldwide (83). Invasive fungal infections have a lower incidence than superficial infections; however, they are of greater concern because they are associated with high morbidity and mortality. They are mostly caused by opportunistic fungal pathogens that take advantage of a debilitated immune system to proliferate in the human host and cause disease (84). Among the fungal species, only several 100 species are associated with human fungal diseases and just a minor number of species cause the most common invasive infections in immunocompromised individuals (85). The most notorious genera that are responsible for more than 90% of all reported fungal-related deaths are Cryptococcus, Candida, Aspergillus, and Pneumocystis (84). This increased prevalence of fungal infections has motivated the study of host–pathogen interactions in order to understand the protective and nonprotective mechanisms of antifungal immune responses in the human body. Investigation of the fungal recognition by the innate immune system led to the discovery of CLRs, the best-characterized PRRs for fungi. CLRs recognize carbohydrate polymers (mannan, glucans, and chitins) present in the fungal cell wall, resulting in the induction of innate and adaptive immunity to clear the pathogen (Figure 1; Table 1) (86).
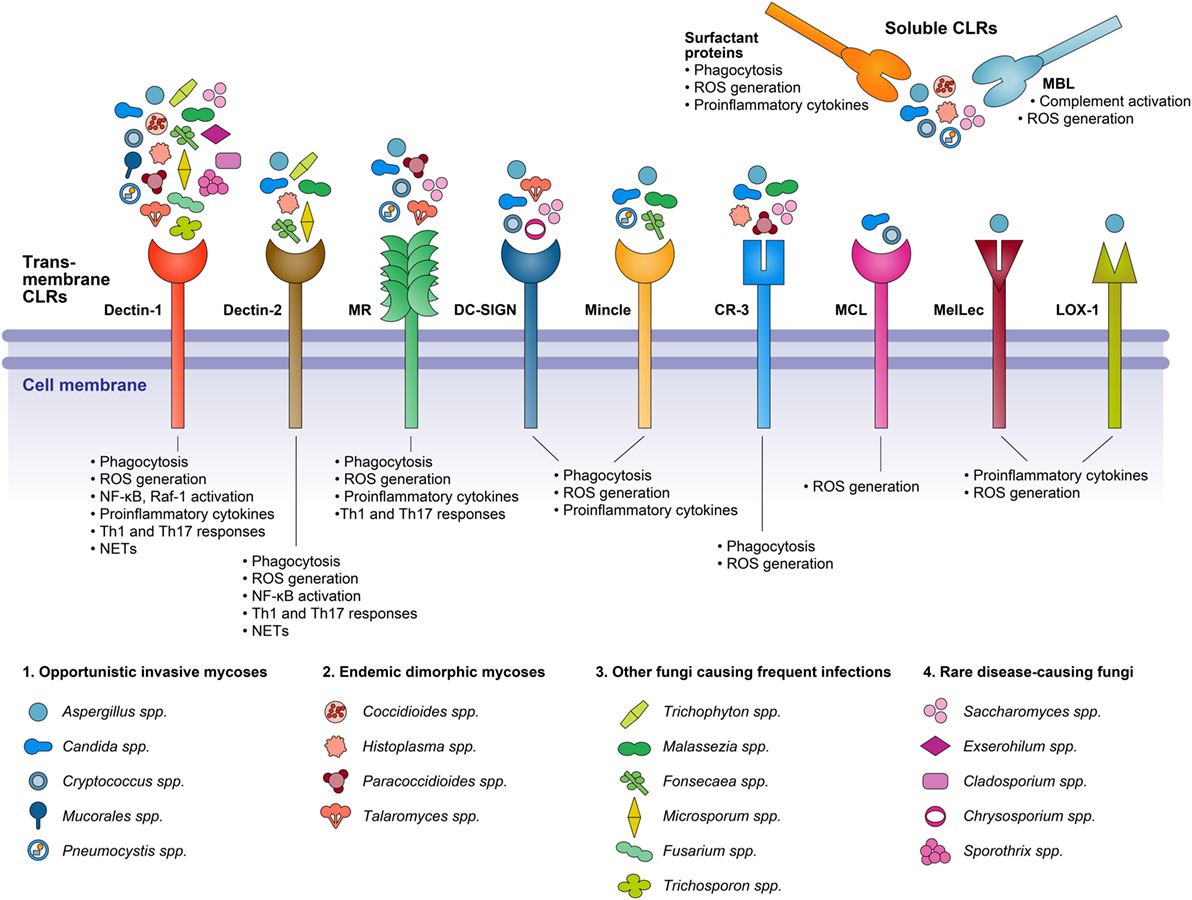
Figure 1. A diagrammatic representation of C-type lectin receptors (CLRs) involved in the recognition of various fungal species, and the resepective cellular responses triggered on receptor–ligand binding.
In the following sections, we will summarize the current knowledge about the interaction of important human pathogenic fungi with CLRs. We further include information on CLR-associated single nucleotide polymorphisms (SNPs) and their effect on the susceptibility to fungal infections.
Opportunistic Invasive Mycoses
Aspergillus spp.
Aspergillus species (Aspergillus spp.) are ubiquitous molds commonly found in the soil. They produce a large number of conidia, which are released and dispersed into the air by wind leading to a deep penetration into the respiratory tract upon inhalation (87). These conidia are effectively cleared from the lungs of immunocompetent individuals. However, patients with a compromised immunity are at risk of developing an acute invasive aspergillosis (AIA). AIA is characterized by hyphal invasion of lung tissues and even dissemination to other organs (87). Aspergillus fumigatus (A. fumigatus) accounts for about 65% of all invasive infections in humans and is the most frequently encountered Aspergillus spp. in pulmonary infections. A. flavus, A. niger, A. terreus, and A. nidulans are less frequent causes of infections (87). The primary innate immune response is mediated mainly by macrophages, DCs, and neutrophils, taking place after Aspergillus spp. encounters these cells. Several of the Aspergillus cell wall components, such as β-glucans, chitins, and mannans act as ligands that are recognized by CLRs. Ligation results in the activation of cellular immune responses, such as phagocytosis, extracellular trap formation, conidial killing, and the production of proinflammatory and anti-inflammatory cytokines, such as TNF-α, IFN-α, IL-6, and IL-18 (88–91). Fungal recognition by specific CLRs can depend on morphological changes of Aspergillus spp., since different growth forms expose diverse PAMPs at variable amounts on their surface. For example, the surface of the Aspergillus dormant conidia does not present β-glucan, but is accessible for receptor recognition after the loss of hydrophobic cell wall components (outer layer of rodlets/hydrophobins and melanin) during the swelling of conidia and the development of germ tubes (89, 92, 93). Several CLRs are involved in the recognition of Aspergillus spp. such as the transmembrane receptors Dectin-1, Dectin-2, MR, DC-SIGN, and the soluble collectins MBL and the lung surfactant proteins (SP) SP-A and SP-D (94) (Table 1). The most studied Aspergillus receptor is Dectin-1. It is present on the surface of myeloid cells recognizing β-1,3-glucan, a common component of the cell wall of several fungi (15). However, another Aspergillus cell wall-associated polysaccharide, the galactosaminogalactan, has also been identified as a ligand and prevents host inflammatory responses in vitro and in vivo, in part by avoiding cell wall β-glucans recognition by Dectin-1 (95). Several observations suggest a significant role for Dectin-1 in protective immunity against A. fumigatus (96–98). A. fumigatus also induces the expression of cytokines (TNF-α and IL-12) and genes related to fungal recognition and phagocytosis in immature human DCs (99). The transcription of Dectin-1 in response to A. fumigatus likely occurs via granulocyte-macrophage colony stimulating factor (GM-CSF)/PU.1, where GM-CSF potentiates the expression of PU.1, which carries out transcription of Dectin-1 augmenting Dectin-1 protein expression and responsiveness in THP-1 cells (100, 101). In HEK293T cells, the activation of AP-1 by heat-killed swollen conidia was inhibited by treatment with Syk inhibitor, indicating that the Syk signaling pathway is required for AP-1 activation in a Dectin-1-dependent manner (102). Silencing of Dectin-1 in murine macrophages resulted in a reduced expression of proinflammatory cytokines, and an observed inhibition of phagocytosis (103). Likewise, A. fumigatus conidia and germ tubes stimulated NF-κB activation, mediated the secretion of proinflammatory cytokines involved in the recruitment of neutrophils, and led to ROS production by human monocyte-derived macrophages, murine macrophages, and alveolar macrophages (AMs) (89, 92, 93). Clinical studies showed that individuals who developed AIA during the course of chemotherapy often displayed a defective expression of Dectin-1. The frequency of Dectin-1-expressing monocytes was reduced in patients with AIA compared to controls (65.6 vs. 87.5%) (104). This important role of Dectin-1 was confirmed by transfecting murine AMs with a vector encoding full-length Dectin-1 (105). Results demonstrated that Dectin-1 overexpression enhanced the generation of proinflammatory cytokines TNF-α and IL-1β, and enhanced the killing ability of macrophages during A. fumigatus exposure (105). The epithelial lining of human airways is another important spot for host-pathogen interactions, and Dectin-1 is also expressed in lung tissues (12). One study found that A. fumigatus induces the expression of Dectin-1 via TLR-2 in human bronchial epithelial cells, resulting in the stimulation of proinflammatory responses and ROS generation in response to A. fumigatus indicating its important role in the innate immune response in non-phagocytic cells (106). Furthermore, some findings indicate that the pulmonary infection of mice with A. fumigatus induces concurrent Th1 and Th17 responses that depend on Dectin-1 (107). With regards to Aspergillus-induced fungal keratitis, recent findings demonstrated that Dectin-1 is expressed in the cornea of rat and mice, where it is involved in the detection of invading fungi (108–110). Also Dectin-2 triggers a response to A. fumigatus infection. Human plasmacytoid dendritic cells (pDCs) recognize A. fumigatus hyphae via Dectin-2, resulting in cytokine release and extracellular trap (pET) formation (88). The noticeable Dectin-2 expression of AMs in human lung during A. fumigatus invasion suggests a prominent contribution to antifungal defenses in pulmonary aspergillosis (90). Moreover, Dectin-2 ligation leads to NF-κB activation and ROS production in response to A. fumigatus infection in human macrophages (111). An A. fumigatus-specific ligand has not been described until now, but Dectin-2 binds to high-mannose structures distributed in several fungal species, including Aspergillus spp. (33). Collectins, such as SP-A, SP-D, and MBL also bind to A. fumigatus (55, 112). One study about the contribution of MBL in the antifungal defense in invasive pulmonary aspergillosis (IPA) showed that in murine models of IPA, rhMBL-treated (recombinant human MBL) mice showed 80% survival compared to untreated IPA mice. A clear increase of TNF-α and IL-1α in treated IPA mice and a significant decrease in pulmonary fungal hyphae and IL-10 could be observed (113). In vitro, there was an enhanced uptake of A. fumigatus conidia by polymorphonuclear neutrophil (PMNs) in the presence of rhMBL, indicating a protective role of this receptor during IPA, possibly through MBL-mediated lectin complement activation (113). SP-A and SP-D also enhanced agglutination and binding of conidia to AMs and neutrophils and increased the phagocytosis, oxidative burst, and killing of A. fumigatus conidia by human neutrophils and AMs (91). The SP-D-mediated protective mechanism is dependent on calcium-activated protein phosphatase calcineurin (114) and include enhanced phagocytosis by recruited macrophages and neutrophils and enhanced local production of the Th1 cytokines TNF-α and IFN-γ in the supernatant from mice lung cell suspension (115). Corneal epithelial cells also express SP-D and in the setting of fungal keratitis A. fumigatus may induce these cells to express inflammatory cytokines via the SP-D and NF-κB pathway (116, 117). Since β(1→6)-glucan is a ligand for SP-D and since many fungi, including Aspergillus spp., have this carbohydrate structure in their cell wall compositions, it is expected that SP-D recognizes all Aspergillus spp. (48).
Several other CLRs ligate Aspergillus spp., but for each only a few data are available. DC-SIGN, another transmembrane receptor of the CLR family expressed on the surface of DCs, contributes to the binding of A. fumigatus conidia in human DC (59). However, DC-SIGN is also expressed in AMs and lung tissue, suggesting a contribution of DC-SIGN in the initial stages and in fungal spreading during AIA (60). Galactomannans appear to be the main DC-SIGN ligand on the cell wall of A. fumigatus conidia (60). Additionally, CR3 influences adaptive responses to Aspergillus. Blocking of CR3 significantly reduced Aspergillus-induced Th1 and Th17 responses independently from complement activation, demonstrating that CR3 might play a significant role in the adaptive host defense against A. fumigatus (72).
In fungal keratitis models, LOX-1 was increased in A. fumigatus infected corneas of C57BL/6 mice and human corneal epithelial cells, indicating a possible role of this receptor in controlling the infection (79, 80). In addition, Mincle and MR may play a role in the early innate immune response of the corneal resistance, since their expression increased significantly during the initial period of A. fumigatus infection, along with an increased expression of TNF-α and IL-1β in human and rat cornea (38, 65). A. fumigatus-specific ligands for these receptors have not been described up to now.
Recently, the CLR Clec1a, also called melanin-sensing C-type lectin receptor (MelLec), has been decribed to play an important role in the detection of A. fumigatus through recognition of the naphthalene-diol unit of 1,8-dihydroxynaphthalene-melanin in conidial spores of A. fumigatus. MelLec is ubiquitously expressed by CD31+ endothelial cells in mice and is required for protection against disseminated infection with A. fumigatus. MelLec is also expressed by myeloid cells in humans and a SNP within the coding region of this receptor (rs 2306894) was identified that significantly increased the susceptibility of stem-cell transplant recipients to AIA (82). AIA is of great interest for immunogenetic studies due to its high prevalence. A moderately large number of studies have investigated the association of SNPs and other genetic variations of different CLRs in order to get some benefit for preventive strategies. For Dectin-1, the CLEC7A rs3901533 (T/T) and rs7309123 (G/G) genotypes and the presence of Y238X (rs16910526) polymorphism resulted in a significantly increased risk of AIA in a Caucasian population (118–120). Two SNPs of CD209 encoding DC-SIGN (rs735239 and rs735240) are associated with a higher susceptibility to fungal keratitis in the northern Han Chinese population (121). Association analysis revealed that carriers the CD209 rs4804800 (G), rs11465384 (T), rs7248637 (A), and rs7252229 (C) alleles and the variant CD209-139A/G (rs2287886) in the Caucasian population had a significantly increased risk of contracting IPA (118, 122).
Several studies show that distinct alleles, genotypes, and genotype arrangements of SFTPA2 and MBL2 may contribute to a susceptibility of the host to aspergillosis. A significant association of SFTPA2 1649G and SFTPA2 1660G and MBL2 1011A alleles with allergic bronchopulmonary aspergillosis patients suggests that defects in these innate immune molecules may lead to an increased genetic susceptibility to allergic airway inflammation and asthma (123–126). Another study implies that the presence of the T allele and CT genotype at position 868 of MBL2, the CC genotype at position 1649 of SFTPA2, and its combination with the CC or CT genotype on position 868 of MBL gene increases susceptibility specifically to chronic cavitary pulmonary aspergillosis in the Caucasian population (127). It was demonstrated that the presence of the codon 52 mutation (W/M52) within the MBL gene was particularly common in patients with chronic necrotizing pulmonary aspergillosis. Since the mutation results in changes in the protein structure, it is likely that a reduced amount of active protein is available for pathogen clearance (128).
Overall, Dectin-1 plays an important role in the local immune response during aspergillosis by inducing the expression of proinflammatory cytokines. Dectin-1 is the best-characterized CLR for the recognition of A. fumigatus, since it recognizes β-1,3-glucan, which is a major component of the inner cell wall of this fungus. Even a single polymorphism results in a significantly increased risk of contracting AIA, indicating the importance of this receptor in the contribution to antifungal defenses. With regards to the other CLRs recognizing Aspergillus spp., more studies are required in order to establish a concrete role of them during an AIA.
Candida spp.
The most common species of Candida responsible for causing human diseases is Candida albicans. It is an opportunistic pathogen that commensally colonizes not only the skin but also the gastro-intestinal and urino-genital mucosal surfaces mostly in yeast form in healthy individuals. In cases of immunosuppression or weakening, the yeast forms can convert into virulent hyphae that can cause either muco-cutaneous infection or disseminate to internal organs causing candidaemia (129). In addition to phenotypic switching between yeast and hyphal forms, C. albicans virulence factors include adhesion properties, secreted lipases, and aspartyl proteases (130). Other clinically relevant Candida species include C. krusei, C. glabrata, C. tropicalis, C. parapsilosis, and others (131). Among the Candida species-recognizing CLRs are Dectin-1, Dectin-2, MCL, Mincle, MR, DC-SIGN, CR3, MBL, and Langerin.
Dectin-1 is a type II transmembrane receptor expressed on several antigen-presenting cells of myeloid origin, including macrophages, monocytes, neutrophils, mast cells, DCs, as well as pulmonary epithelium (12, 16). Like A. fumigatus and other fungi, Dectin-1 also recognizes C. albicans by binding to β-1,3-glucan (Table 1) (132).The binding of β-1,3-glucan to Dectin-1 is Ca2+-independent (133). Notably, Dectin-1 recognition of the yeast form of C. albicans induces responses such as phagocytosis and oxidative burst in mouse phagocytes, ultimately resulting in the clearance of the yeast cells; in contrast, filamentous forms may mask the β-glucans by mannans and affect certain β-glucan-mediated responses (17, 134, 135). However, C. albicans germ tubes can be resognized by Dectin-1 in Syk-dependent mechanism and initiate Th-17 response (136).
Dectin-1 recognition of C. albicans or its ligand β-1,3-glucan initiates several distinct immune responses. β-1,3-glucan-Dectin-1 binding leads to NFκB-mediated ROS production and proinflammatory cytokine release, such as IL-12, TNFα, and IL-6, via the Syk-CARD9 pathway in mouse DCs and macrophages, as well as in human intestinal cells. The response is enhanced in co-operation with TLR-2 (137–141). Interestingly, C. albicans activation of Dectin-1 can also result in anti-inflammatory responses, like IL-10 release by macrophages and peripheral blood mononuclear cells (PBMCs) or the production of IL-1 receptor antagonist (IL-1Ra) (142–144). Furthermore, ligation of Dectin-1 on APC by C. albicans, but also by other fungi and even the endogenous ligand galectin-9 drives T cell differentiation into a TH2/TH17 response (145–148). PKCδ is essential for CARD9-dependent NFκB activation (149). C. albicans also induces mast cell activation in rat and mice that leads to a differential cytokine production depending upon the fungal morphology, and induces phagocytosis and nitric oxide production in a TLR-2 and Dectin-1-dependent manner (150, 151). A similar co-operation of Dectin-1 with TLR-2 and TLR-4 can be observed in human mononuclear cells, PBMCs, and macrophages on stimulation with β-1,3-glucan (152, 153). The Dectin-1-Syk-CARD-9 pathway can also activate IRF5 to produce IFN-β (154) or ERK to generate proinflammatory responses against C. albicans (155). Moreover, Dectin-1 also mediates the β-1,3-glucan-medidated opsonization-independent phagocytosis by human neutrophils and retinal microglia (156, 157). The Syk-dependent pathway is also involved in β-1,3-glucan-containing phagosome maturation and recruitment of TLR-9 in RAW cells (158). Additionally, Dectin-1 involvement with C. albicans activates many other signaling pathways. Dectin-1 binding with C. albicans can activate NFAT transcription factors induce IL-2, IL-10, and IL-p70 release in collaboration with TLR-2 in mouse DCs (15, 159). β-1,3-glucan-induced human DCs activate NFκB via Syk as well as Raf-1 in vitro. In fact, Raf-1 activation represses Syk-induced RelB activity, although not completely, and increases p65 transactivation activity to induce IL-12p40 and IL-1β production (160). Several studies based on human and mouse cell lines have demonstrated that Dectin-1 is important in activating the inflammasomes such as the noncanonical caspase-8 inflammasome that promotes Th-17 responses which are essential for antifungal immunity (136, 161–166). Th17 responses are important against cutaneous infection, while Th1 responses are directed against systemic infection (134). Dectin-1 also co-operates with other CLRs such as SIGNR1 in mouse macrophages enhancing the oxidative burst against C. albicans (167). Some studies have also demonstrated a Dectin-1-dependent CR3 activation on mouse neutrophils and subsequent killing of C. albicans by these cells (168, 169). Human neutrophils release neutrophil extracellular traps in response to C. albicans in vitro, triggered by the ROS production on recognition of β-glucan by Dectin-1 and CR-3 (170, 171). Interestingly, Dectin-1 stimulation with C. albicans or β-1,3-glucan can also generate certain immunomodulatory responses, e.g., IL-10 production and reduction in ROS production via SHIP-1 activation in mouse GM-CSF-derived bone marrow cells (172). Additionally, C. albicans-Dectin-1 engagement induces human as well as mouse granulocytic myeloid-derived suppressor cells to dampen the pathogenic hyperinflammatory NK and Th17 responses (173). Moreover, a recent study has also demonstrated a role of Dectin-1 in adaptive immunity by controlling CD4+ T cell responses in the murine gut (174). All these results indicate how C. albicans can influence the immune responses by engaging the same receptor on different cell types.
Mouse knockout studies have shown contrasting results. Dectin-1 deficient mice display defective macrophage activation with impaired subsequent inflammatory responses and present enhanced fungal burden and dissemination after C. albicans infection (163, 175). However, further mouse studies implied that Dectin-1 deficiency probably plays a minor role in systemic Candida infection but may control the mucosal infections (176). The differences in the results may be attributed to different mouse and C. albicans strains used in experiments (177). Dectin-1-deficient mice also are more susceptible to C. glabrata infections and show impaired inflammatory responses (178).
Polymorphisms in Dectin-1 have been studied with respect to Candida infections. The first study to report an early-stop-codon mutation Y238X in a family with recurrent vulvovaginal candidiasis (RVVC) among four women demonstrated that the monocytes and neutrophils from homozygotes lack Dectin-1 expression and are defective in cytokine production such as IL-17 upon C. albicans stimulation in vitro. However, phagocytosis and killing of fungi is normal (179, 180). Another report demonstrated that heterozygotes for Y238X receiving hematopoietic stem cell transplantation display an increased incidence of gastrointestinal Candida colonization. The monocytes of homozygotes show less IL-1β production and lack of TLR-2-Dectin-1 synergism complementing the previous in vivo and in vitro studies describing the role of Dectin-1 in Candida infections (181). In an HIV-infected African population, a mutation I223S has been associated with a lower IFNγ response to C. albicans stimulation of whole blood and tends to provide protection against oropharyngeal candidiasis (182).
The hyphal form of C. albicans can be recognized by Dectin-2, which binds to high-mannose structures such as α-mannans in a cation-dependent manner and is expressed predominantly on macrophages and DCs (Table 1) (11, 33). Several studies in mouse DCs and macrophages have demonstrated that Dectin-2 mediates its signaling via the ITAM-bearing adapter FcRγ and the Syk-CARD9 pathway but the subsequent responses seem to differ depending upon the cell type, fungal morphology, and methodologies (11, 34, 183, 184). Nevertheless, C. albicans recognition by Dectin-2 can induce phagocytosis, proinflammatory cytokine production, such as IL-6, IL-23, TNFα, and IL-12 as well as protective Th-17 responses (34, 183). In addition, Dectin-2-deficient mice show decreased survival and high kidney fungal burden after 10 days of infection with C. albicans (34). Indeed, it was later shown in two independent studies that Dectin-2-deficient mice are also susceptible to C. albicans and C. glabrata systemic infections, showing high fungal burdens in kidneys and reduced neutrophilic phagocytosis (185, 186). Similar to Dectin-1, Dectin-2 can also induce type I IFN responses in mouse macrophages and DCs by activating IRF5 in response to C. albicans (154). PLCγ2 is essential for Dectin-2-mediated NF-κB, MAPK and ROS activation in mouse macrophages when infected with hyphal C. albicans (187). Zhu et al. showed that Dectin-2 and MCL (Dectin-3) heterodimers recognize C. albicans α-mannans more effectively than either receptor alone and that MCL-deficient mice are highly susceptible to systemic candidiasis (70). However, most of these studies have been performed in mouse models and provide a picture of Dectin-2 and MCL roles in murine Candida infections, and studies regarding their role in the human host and the impact of mutations in the human receptors will be needed in order to complete the picture.
Mannose receptor is a mannan-binding lectin found on phagocytic cell surfaces and recognizes C. albicans α-mannans (Table 1) (39). Early studies have demonstrated the involvement of MR in cytokine release, non-opsonic phagocytosis, and killing of Candida spp. by phagocytic cells (Figure 1) (40, 188, 189). Human DCs phagocytose and kill Candida via MR leading to subsequent responses such as Th1 immunity and ROS production (39, 190–192). Dectin-1 engagement with C. albicans on mouse macrophages induces surface MR shedding which could be the reason for downregulation of MR surface expression observed on rat macrophages after C. albicans ingestion (193, 194). Dectin-1 was later shown to be the main phagocytic receptor, while MR is recruited to phagosomes in mouse macrophages in later stages and mediates the secretion of immunomodulators such as TNFα and MCP-1 (195). Indeed, MR-deficient mouse macrophages are able to take up and phagocytose C. albicans normally (195, 196). However, in human phagocytic cells, MR induces Th17 responses upon stimulation with C. albicans in vitro by inducing IL-1β and prostaglandin E2 production, which is enhanced by Dectin-1/TLR-2 synergism (162, 197–199). Neumann et al. reported the formation of unique MR-induced pseudopodial protrusions called fungipods in human monocyte-derived DCs in response to C. albicans yeast, which may have role in fungal phagocytosis. This response is species-specific with C. parapsilosis showing stronger fungipod formation compared to C. albicans and C. tropicalis (41). In fact, the innate immune recognition of C. parapsilosis complex and C. albicans by human PBMCs differ with respect to the receptors involved and the induced cytokine production; for example, MR is important for TNFα and IL-1β production upon C. parapsilosis stimulation (200). Interestingly, IFNγ stimulation of human monocyte-derived DCs and macrophages increases the candidacidal activity of these cells by increasing non-opsonic phagocytosis and ROS production which is related to a reduced expression of MR (201, 202). So far, no genetic studies have been performed to understand the significance of MR polymorphisms in Candida infections.
Candida albicans yeast and hyphae are also recognized by the collectin MBL (56, 203). Several studies have demonstrated the binding of Candida spp. to MBL followed by activation of the complement system and subsequent opsonophagocytosis of fungi by phagocytic cells in vitro (204–206). Li et al. demonstrated MBL-dependent opsonophagocytosis of C. albicans by human neutrophils but without complement activation. This response was coupled with intracellular Dectin-1-dependent ROS production (207). Parenteral administration of MBL increased the resistance of mice in a model of disseminated candidiasis (56). In fact, mice deficient in MBL-A and MBL-C (mice homologs to human MBL) are more susceptible to systemic Candida infection (208). MBL is expressed in the mouse gut and its blocking or elimination leads to increased C. albicans colonization (209). MBL can also modulate the C. albicans-triggered TLR-generated proinflammatory signals by THP-1 cells (210). MBL concentrations are greatly affected by promoter polymorphisms in the MBL2 gene and the resulting lower MBL levels are linked to the risk to develop several infectious diseases (128, 211, 212). Reduced levels of MBL were observed in the cervicovaginal lavage of RVVC patients, while the levels were higher in VVC patients compared to healthy controls (213–215). Moreover, MBL deficiency is also associated with the development of abdominal yeast infection in peritonitis patients (216). However, MBL serum levels and genotypes were not associated with intra-abdominal candidiasis in a Swiss cohort (217). The RVVC patients also have a higher frequency of MBL2 mutations compared to both VVC and healthy groups (214). Furthermore, the MBL2 codon 54 allele B is associated with a higher susceptibility to RVVC as observed in Belgian, Latvian, and Brazilian women (215, 218, 219). A recent meta-analysis of five different studies also concluded the correlation of allele B of codon 54 to be associated with both RVVC and VVC (220). Only a couple of studies have addressed the polymorphisms in components of MBL complement pathway in development of invasive fungal infections (221, 222). The polymorphisms in MBL complement pathway components have been associated with other infectious diseases as well, such as tuberculosis and leprosy (223, 224) and further studies exploring the effects of mutations in complement proteins on the pathogenesis of fungal infections are still needed.
Another CLR, DC-SIGN, can recognize the N-linked mannans in the C. albicans cell wall (39, 225). It mediates the internalization of conidia by human DCs, which are abundantly present in mucosal tissue (Table 1) (61), although human DCs exhibit less-efficient phagocytic activity compared to monocytes and macrophages (226). Gringhuis et al. showed that C. albicans stimulation can modulate TLR-dependent pathways by Raf-1 activation in human DCs. There is also evidence for possible anti-inflammatory effects upon DC-SIGN ligation. However, data so far are derived from non-fungal ligands (227). The fungus-induced IL-10 production is mediated through coactivation of DC-SIGN and TLR signaling pathways (228). The mouse homolog of DC-SIGN, SIGNR1 works in co-operation with Dectin-1 and TLR-2 in mouse macrophages to induce responses such as oxidative burst and TNFα production in vitro (167, 229). Knockout mouse studies and genetic studies may enlighten us more regarding the importance of this CLR in these fungal infections.
Candida albicans is also recognized by Mincle, another CLR expressed on several immune cells (Table 1), although the related ligand has not yet been discovered (230). An in vivo study demonstrated a non-redundant role of Mincle against Candida infection as Mincle-deficient mice were highly susceptible to systemic candidiasis. Mincle induces TNFα production in mouse macrophages upon C. albicans hyphae stimulation in vitro but is not important for phagocytosis, indicating that Mincle has a role in initial macrophage binding and early responses to the fungus (66). A similar effect was observed in human monocytes where the stimulation with C. albicans yeast leads to TNFα production but is related to poor yeast uptake. However, human neutrophils expressing Mincle show a fungicidal activity correlated with phagocytosis of the yeast (231). Taken together, more studies are needed to discover the C. albicans ligand(s) for Mincle and the related pathways as well as in vitro and in vivo immune responses and further work is necessary to elucidate the role of Mincle polymorphisms in fungal infections.
CR3 is an important CLR expressed on neutrophils, macrophages, NK cells, and monocytes and is involved in adhesion and phagocytosis of C. albicans (5, 73, 232). CR3 has a role in C. albicans hyphae recognition as the human lymphocyte adhesion of C. albicans hyphae was abrogated upon blocking CR3 with monoclonal antibodies (233, 234). Soloviev et al. showed that C. albicans releases a soluble CR3-binding mannoprotein called pH-regulated Ag 1 (Pra1), which mediates CR3-dependent adhesion and migration of THP-1 cells and neutrophils toward C. albicans (74, 235). Pra1 is expressed highly and exclusively on C. albicans hyphae (236). Soluble Pra1 was found to be beneficial for fungal survival as it inhibits the human neutrophil activation upon stimulation with Pra1 overexpressing C. albicans hyphae (235). Additionally, a CR3 knockout mouse study demonstrated that these mice show an increased susceptibility toward C. albicans systemic infection (168, 237) and their neutrophils display impaired adhesion, migration, and oxidative burst when challenged with C. albicans in vitro (237). Several studies have shown β-glucan to be another potential ligand for CR3 (238–240). Dectin-1 and CR3 co-localize on yeast C. albicans phagocytic cups in mouse peritoneal macrophages (195). Further studies support the interaction of Dectin-1 and CR3 in C. albicans infection as mentioned earlier. Dectin-1 activates CR3 for recognition of C. albicans yeast components and together they induce neutrophil cytotoxic responses in mice (168, 169).
Langerhans cells (LCs), found in epidermis and mucosal linings, express Langerin, which can bind to β-glucans and recognizes Candida spp., including C. albicans, C. glabrata, C. parapsilosis, C. tropicalis, and C. krusei, among others, in vitro (81, 241, 242). Some studies have shed light on the roles of LCs in Candida infections (243, 244), but specific studies regarding the role of Langerin are lacking.
Taken together, Candida is recognized by a number of CLRs, each of which is able to generate fungal-specific immune responses. While Dectin-2 and CR3 can recognize and respond to the fungal hyphae, Dectin-1, MR, and DC-SIGN mainly recognize the conidial forms. For Mincle, MCL and Langerin little is known and they need to be further investigated for their roles in Candida infections. Moreover, more studies need to focus on the genetic component of the effect of CLRs on Candida infections.
Cryptococcus spp.
Cryptococcosis is a worldwide distributed and invasive fungal infection that is caused by species of the genus Cryptococcus. Nearly 100 species have been described within this genus so far, but Cryptococcus neoformans and C. gattii species are considered to be the only disease-causing fungi (245). Although cryptococcosis is predominantly a disease of immunocompromised patients (AIDS-defining illness), a recent outbreak showed the capacity of some lineages of the fungus to act as primary pathogens in healthy individuals (246). Within the lung, Cryptococcus spp. can cause pneumonia in immunosuppressed patients, and the latent infection can then disseminate to other tissues, most particularly the central nervous system (CNS), where this fungus causes an infection of the meninges accompanied by elevated intracranial pressure and without a rapid treatment it becomes fatal (246).
The interaction of Cryptococcus with CLRs is poorly understood. Initial data demonstrated that C. neoformans binds to soluble collectin MBL and the ingestion of the acapsular form is inhibited by both soluble mannan and β-1,3-glucan, showing that ingestion of acapsular C. neoformans takes place via mannose and β-glucan receptors in murine macrophages (247).
The role of Dectin-1 is still controversial, since no significant differences were observed in the clinical course and cytokine production between Dectin-1-deficient and control mice in a cryptococcosis model (248), but another study found that C. neoformans spores are phagocytosed by murine AMs via Dectin-1 (18). The role of Dectin-2 is also poorly understood. Some results show that it may not be required for the production of Th1 and Th17 responses, proinflammatory cytokines or for the clearance of C. neoformans in Dectin-2 knockout mice (249). Dectin-3 seems to have a role as it was demonstrated that human and murine pDCs have a direct Dectin-3-dependent anti-cryptococcal activity by inhibiting the growth of C. neoformans via ROS production (71). A Cryptococcus-specific ligand for this receptor has not been described yet.
Mannose receptor also has a role in Cryptococcus infection. After a pulmonary infection with C. neoformans, MR knockout mice died significantly earlier than wild-type mice and had higher lung fungal burdens (250). This receptor was required for the presentation of C. neoformans antigens to T lymphocytes by primary DCs, since blocking this receptor reduced both uptake of C. neoformans and lymphocyte proliferation (251). Some data suggest that mannoproteins, secreted by C. neoformans, might be the ligands for MR, as T cell stimulation is inhibited either by competitive blockade of MR in APCs or by removal of carbohydrate residues from mannoproteins. These results imply a capacity of mannoproteins to bind MR and to be processed by APCs to stimulate primary T cells (42). However, multiple receptors on DC could recognize this ligand, since DC-SIGN was also determined to have an affinity for mannoproteins. Further, MR and DC-SIGN both colocalize with mannoproteins, supporting a role for each in mannoprotein capture (62).
The pulmonary surfactant proteins, SP-A and SP-D bind to both encapsulated and acapsular C. neoformans (49, 252) and SP-D binds to the high-molecular weight polysaccharide glucuronoxylomannan and mannoproteins on the fungal cell wall (50). However, some data suggest that these receptors actually increase the susceptibility to C. neoformans infection. SP-A inhibits the IgG-dependent phagocytosis of C. neoformans by AMs and SP-A−/− mice exhibit wild-type vulnerability to C. neoformans; SP-D−/− mice are even protected during C. neoformans infection and display decreased fungal burden compared to wild-type mice. SP-D−/− AMs also demonstrate an enhanced ability to kill C. neoformans cells (253, 254). Indeed, SP-D increases vulnerability to C. neoformans infection by stimulating C. neoformans-driven pulmonary IL-5 and eosinophil infiltration (255). SP-D may also play a role in protecting C. neoformans cells during the early stages of infection by opsonization. It was found that SP-D increases phagocytosis of hypocapsular C. neoformans by murine macrophages and enhances fungal survival allowing to gain access to specific intracellular compartments where it can grow (256). Another study reports that both, the presence of capsules and a wild-type cell wall design, prevent MBL binding to C. neoformans (257).
Last but not the least, complement activation by Cryptococcus spp. was demonstrated in the presence of MBL in vitro (57). A Cryptococcus-specific ligand for this receptor has not been described up to now.
Given that Dectin-1, Dectin-2, SP-A, and SP-D studies showed controversial results and their interactions with Cryptococcus are poorly understood, further studies are necessary.
Mucorales spp.
Mucormycosis is the second most-common form of invasive mold infections. The disease is characterized by vessel thrombosis and tissue necrosis resulting from extensive angioinvasion and further dissemination (258). The members of the Mucorales order of Zygomycetes are among the leading causes of mucormycosis in immunocompromised individuals apart from more common fungal genera, such as Candida or Aspergillus, with mortality rates ranging from 50 to 100% (259). Among Mucorales spp., although rare, Rhizopus oryzae accounts for 70% of mucormycosis infections. Mainly phagocytotic cells play an important role in restricting the infection (260). The studies on mechanistic details of fungal recognition by CLRs and their role in pathogenesis are still lacking. One study reported that patients with mucormycosis showed reduced expression of Dectin-1 on monocytes compared to healthy controls (104). However, further investigations into the role of C-type lectins and their polymorphisms in this infection are needed.
Pneumocystis spp.
The genus Pneumocystis includes a variety of ubiquitous fungi that colonize and infect several mammalian host species. The species P. jirovecii particularly infects humans, whereas Pneumocystis carinii (P. carinii) and P. murina are associated with rats and mice, respectively. In the immunocompromised host, Pneumocystis pneumonia (PCP) is fatal if untreated. However, infection of an immunocompetent host can result in a self-limited mild or subclinical lower respiratory tract infection (261).
The first studies demonstrated an interaction of Pneumocystis cell wall isolates with macrophage β-glucan receptors, which induced a potent stimulation of TNF-α release in rat AMs in response to P. carinii (262, 263). During P. carinii infection, the expression of Dectin-1 is upregulated in macrophages of immunocompetent rat models (264). According to some studies, Dectin-1 is required for the protection against P. carinii infection, since Dectin-1-knockout mice are more sensitive to infection than infected wild-type mice, and production of ROS is completely abolished in Dectin-1-knockout macrophages incubated with P. carinii (176). Probably, the expression levels of Dectin-1 in AMs are under the control of the transcription factor PU.1 during a PCP infection, where the GM-CSF appears to play a major role in the regulation of PU.1 expression (265). Phagocytosis of P. carinii and generation of hydrogen peroxide by murine AMs is mediated by Dectin-1, since the blockage of Dectin-1 inhibits the binding and killing of P. carinii (266). The binding of Dectin-1 to Pneumocystis was tested by creating recombinant Dectin-Fc fusion proteins which bind P. carinii and enhance murine macrophage-dependent killing. These findings demonstrate that Dectin-1 binds β-glucan from Pneumocystis, enhancing host recognition and clearance of P. carinii (19). P. carinii β-glucan cell wall component challenge of rat alveolar epithelial cells resulted in a prominent nuclear translocation of p65 NF-κB with a subsequent increase in MIP-2 and TNF-α mRNA production. However, rat alveolar epithelial cells do not require Dectin-1 for MIP-2 production, which rather involves the participation of the alternative lactosylceramide β-glucan receptor (267, 268).
Pneumocystis carinii also enhances soluble MR production in human and murine macrophages (269). In human AMs, phagocytosis of Pneumocystis is mediated through MR and depends on Cdc42 and especially RhoB activation (270). Pneumocystis also stimulates NF-κB nuclear translocation in human AMs, which is mediated primarily through MR (43). A recombinant soluble MR-Fc fusion protein binds P. carinii and leads to an increased uptake by hPMNs (271). The role of MR was confirmed by the fact that binding and uptake of cultured P. carinii by human and rat AMs is reduced 90% by using competitive inhibitors of MR, emphasizing the role of the AMs in the first-line host defense (272). Other studies suggest that a reduced AM MR-mediated binding of P. carinii may contribute to the susceptibility of HIV-infected individuals to this pathogen (273). However, it was demonstrated that IL-8 release by human AMs following the stimulation with Pneumocystis requires the co-expression of MR and TLR-2, since the IL-8 release is reduced significantly upon blocking of TLR-2 and silencing of MR gene (274). These results support the idea that MR on human AMs may suppress the production of proinflammatory cytokines and may serve to regulate the innate inflammatory responses to Pneumocystis infection in the lungs (275). A Pneumocystis-specific ligand for MR has not been described up to now.
Some results indicate that SP-A and SP-D can modulate the virulence of P. murina and P. carinii during development of infection in SP-D- and SP-A-deficient and immunosuppressed mice. They attenuate the production of proinflammatory cytokines and ROS and RNS, indicating that both receptors are local effector molecules in the lung host defense against Pneumocystis in vivo (276–280). These results are supported by the fact that SP-A and SP-D can bind P. carinii, acting as opsonins and enhancing their phagocytosis by AMs (281–284). However, some data suggest that the increased SP-A and SP-D mediated aggregation of P. carinii fungal particles interferes with AM recognition and thus the SPs may contribute to the pathogenesis of P. carinii pneumonia (285–287). This view is supported by the fact that SP-A in immunosuppressed mice acts as a therapeutic agent in the beginning of Pneumocystis infection, but not in the middle or late stages of the infection (288). SP-D strongly interacts with gpA, the main glycoprotein antigen on the surface of P. carinii. The interaction of SP-D with P. carinii gpA is mediated by the carbohydrate recognition domain (CRD) of this collectin (51, 289). Similarly, the CRD of SP-A mediates binding to the main surface glycoprotein gp120 of P. carinii (52, 290).
Binding of MBL to P. carinii is followed by the activation of the respiratory burst, indicating that the MBL in serum has opsonizing properties and might contribute in controlling fungal spread from the lungs (58). A Pneumocystis-specific ligand has not been described up to now for this receptor.
Mincle binds whole P. carinii and a surface glycoprotein called MSG/gpA, a Pneumocystis cell wall component, which is expressed at enhanced levels during infection (67). Moreover, Mincle−/− mice exhibit significantly higher P. murina burdens with elevated levels of TNF-α, IL-6, and IL-1Ra during infection, indicating that Mincle functions as an important signaling receptor in host defense against Pneumocystis infection (67).
Little is known about polymorphisms affecting Pneumocystis recognition, however, one study analyzed 53 HIV patients having CD4 counts <200 μL, in order to find a correlation between MBL and PCP. Of these 53 patients, 30 had PCP at admission, and 23 did not. Genotypes related with a low production of MBL were significantly more common in the PCP group than in the non-PCP group. Serum MBL levels were significantly higher in the non-PCP group. Genetic variations influencing MBL production also affect the susceptibility to PCP in HIV-advanced infection patients, and may be considered as a risk factor for PCP (291).
Overall, CLRs seem to be of importance for orchestrating the Pneumocystis-induced immune response. However, Pneumocystis cannot easily be propagated in culture, which has delayed the understanding of its pathobiology. Efforts to study Pneumocystis have been greatly limited by the inability to maintain ex vivo culture of the organism. Early attempts to isolate and propagate P. jirovecii, have been moderately successful, however, none of these models garnered sufficient recognition to become a standard method for the isolation of Pneumocystis (292). Nonetheless, studies of organisms isolated directly from the infected lung of patients or immunosuppressed research animals still allow for some insight into the pathobiology of Pneumocystis (293).
Endemic Dimorphic Mycoses
Coccidioides spp.
There are two species of Coccidioides (C. immitis and Coccidioides posadasii) that cause human disease. They have similar phenotypes and pathogenicities, but differ in genotype and geographic distribution. They are the etiologic agents of coccidioidomycosis, which ranges from asymptomatic infections to pneumonia and severe disseminated disease. These organisms are found in the soil, especially in low-moisture environments, so preventing exposure can be difficult due to the ubiquitous risk of dust inhalation by individuals living in endemic areas. The pathogenesis of coccidioidomycosis is complex and can be asymptomatic but also cause extrapulmonary dissemination (294).
The reasons of the complexity of the pathogenesis of coccidioidomycosis are not well understood; however, some data suggest the main involvement of Dectin-1. Some results suggest that an alternative splicing of the Dectin-1 gene enhances the susceptibility of C57BL/6 mice to coccidioidomycosis, regulating the cytokine responses of macrophages and mDCs to spherules, the pathognomonic structure of this fungus (295). RAW 264.7 macrophages overexpressing Dectin-1 produced more TNF-α than control macrophages in respond to C. posadasii spherules. Also, macrophages overexpressing Dectin-1 and activated with purified β-glucan from C. posadasii spherules produced a significantly higher level of TNF-α than control macrophages, indicating a role of β-glucan from C. posadasii as a ligand for Dectin-1 (20). Moreover, Dectin-1 activation is essential to leading the adaptive immune response toward Th1 and Th17 pathways, thus leading to the resolution of infections in mice (35, 296).
Other results suggest that there is an association between low serum MBL levels and symptomatic coccidioidomycosis, but in order to understand the role of MBL in the pathogenesis of this fungal disease, further studies are necessary (297). SP-A and SP-D also bind coccidioidal antigens (53). Deficiencies of MR and Dectin-2, either alone or in combination, affect cellular responses to formalin-killed spherules in vitro but do not make C57BL/6 mice more vulnerable to pulmonary coccidioidomycosis (298). A Coccidioides-specific ligand for these receptors has not been described up to now.
In conclusion, further studies are necessary to elucidate interactions of Coccidioides with CLTRs, since few receptors and no ligands have been studied. However, Dectin-1 seems to have an important role in this infection.
Histoplasma spp.
Fungi of the genus Histoplasma cause histoplasmosis and are found throughout the world, but are most common in North America and Central America. Histoplasma capsulatum is a member of this group of fungal pathogens that cause respiratory and disseminated disease in mammals. It grows as a saprobic conidia-producing mycelium in the environment, and when the aerosolized mycelium fragments and conidia are inhaled, they reach the lower respiratory tract causing disease even in immunocompetent hosts (299).
Little is known about the receptors recognizing Histoplasma and its signaling response. However some CLRs, such as Dectin-1, Dectin-2, and some collectins are involved in Histoplasma immunity (Table 1). Dectin-1 and Dectin-2 exert several contributions to the development of antifungal Th1 and Th17 cells and vaccine resistance in mice against H. capsulatum (35, 300). CR3 and Dectin-1 act together to induce murine macrophages to TNF and IL-6 responses through a Syk-JNK-AP-1-dependent mechanism (75). Some data show that CR3 participates in phagocytosis and cytokine responses, but Dectin-1 takes part in cytokine production only on murine macrophage (21). Histoplasma pathogenic yeast cells secrete Eng1, a β-glucanase that hydrolyzes β-(1,3)-glycosyl linkages, which reduces levels of surface-exposed β-glucans on yeast cells, thereby enabling Histoplasma yeasts to escape detection by Dectin-1. Histoplasma yeasts deficient for Eng1 show an enhanced binding to Dectin-1 and an increased TNF-α and IL-6 production in murine macrophages and DCs (301, 302). Also, SP-A and SP-D demonstrate potent antifungal properties, since they cause a dose-dependent decrement in yeast viability, which is associated with an increase in the permeability of the yeast cells. Mice lacking SP-A manifest a modestly higher fungal burden in lungs than wild-type littermates (54). A Histoplasma-specific ligand for these receptors has not been described up to now.
Together, these studies indicate minor roles for CLRs in the control of Histoplasma infections, but further studies are needed to understand the significance of CLRs in Histoplasma infections.
Paracoccidioides spp.
Paracoccidioides spp. is the causal agent of paracoccidioidomycosis (PCM), a systemic mycosis endemic to Latin America. It comprises two species: Paracoccidioides brasiliensis and the recently described P. lutzii (303). Manifestations of PCM include subclinical or asymptomatic infection. The symptomatic disease causes an acute/subacute or a chronic form, the latter involving the lungs as well as other organs. PCM is acquired after inhalation of infectious propagules in the environment, leading to a primary pulmonary infection (303).
Few studies have investigated the role of CLRs on Paracoccidioides infection. Human monocytes display a decrease in Dectin-1 expression as soon as 30 min after stimulation with P. brasiliensis (22). There is a trend toward an increased Dectin-1 mRNA expression in response to P. brasiliensis and this receptor is able to induce a balanced production of TNF-α, IFN-γ, IL-12, and IL-10 in human neutrophils and monocytes (22, 304, 305). By binding to Dectin-1, P. brasiliensis induces neutrophil extracellular trap (NET) release that is responsible for trapping yeast cells, promoting their immobilization, as well as contributing to their extracellular killing (306). Moreover, the fungal infection of Dectin-1−/− mice results in enhanced tissue pathology and mortality rates. The deficiency of Dectin-1 has also reduced the production of Th1, Th2, and Th17 cytokines and the activation and migration of T cells to the site of infection (307). Altogether, these results suggest the participation of Dectin-1 in P. brasiliensis recognition, internalization, and consequent activation of the immune response against the fungus. A Paracoccidioides-specific ligand for Dectin-1 has not been described up to now, however, it is known that Dectin-1 binds glucan structures that are distributed in a wide range of fungal species.
The gp43 glycoprotein is the main antigenic component secreted by P. brasiliensis. gp43 binds to TLR2, TLR4, and MR receptors and all three receptors influenced a high production of IL-10 and TNF-α in human monocytes (44). The specific blockade of MR and CR3 impaired fungal recognition and modified the production of cytokines (308). The CR3 receptor may participate in phagocytosis of P. brasiliensis conidia through both opsonic and non-opsonic mechanisms, since treatment of murine macrophages with anti-CR3 and α-methyl-d-mannoside, a competetive inhibitor of the binding of mannose, decreased phagocytosis of P. brasiliensis (76). In the same way, the mannose-binding lectin complement pathway was demonstrated to play a key role in complement activation by P. brasiliensis (309). A Paracoccidioides-specific ligand for this receptor has not been described until now.
Overall, interactions between host immune cells and Paracoccidioides spp. are mediated by the recognition of Dectin-1 which controls internalization by phagocytes as well as lymphocyte proliferation during P. brasiliensis infection. However, further studies are necessary, since few receptors and no ligands have been studied.
Penicillium spp.
Penicillium species are rarely considered as human pathogens except Talaromyces (Penicillium) marneffei, which can cause opportunistic infections, called penicilliosis, in immunocompromised patients, especially in HIV positive persons, but also in old and new born (310). The infection is most prevalent in South-east Asia and is characterized by symptoms, such as weight loss and fever, skin lesions, generalized lymphadenopathy and hepatomegaly, and respiratory signs such as hemoptysis (310, 311). The main virulence factor of T. marneffei is its temperature-dependent dimorphic growth, owing to which it grows as mycelium at 25°C while at 37°C it grows as yeast (311). In vitro experiments have shown that T. marneffei can be recognized by Dectin-1, DC-SIGN, and MR (Figure 1) (23, 45, 63). Koguchi and colleagues demonstrated that blocking MR with antagonists reduces the osteopontin production from PBMCs upon T. marneffei stimulation and suggested a mannoprotein as the possible ligand (45). Indeed, MR was later found to be involved in adhesion and phagocytosis of the fungus by human monocyte-derived DCs, while DC-SIGN only mediated adhesion (63). IL-12p40 production by bone marrow-derived dendritic cells (BMDCs) upon stimulation with T. marneffei is abrogated in Dectin-1 knockout mice (23). Together, these studies suggest that CLRs modulate the immune response to T. marneffei; however, further studies are needed to dissect the in vivo role of CLRs and their polymorphisms in T. marneffei infections.
Other Fungi Causing Frequent Infections
Trichophyton spp.
Dermatophytosis is one of the most common mycoses worldwide. Compromising keratinized tissues and characterized by establishing chronic inflammatory processes, it is highly resistant to standard antifungal therapies. The main etiological agent in humans is Trichophyton rubrum. It establishes infection after being inoculated in the host tissue, where it survives through the degradation of dead cells, consuming keratin and other host components (312).
Only few studies analyzed the roles of CLRs in Trichophyton spp. infections. Dectin-1 is involved in mediating inflammation induced by trichophytin, a T. mentagrophytes antigen with β-glucans and zymosan as the main components (24). T. rubrum hyphae are recognized by Dectin-1 and Dectin-2 in murine DCs, triggering production of inflammatory cytokines, mainly IL-1β and TNF-α. This inflammatory process is able to promote the clearance of the pathogen in vivo without the involvement of lymphocytes. Even though IL-17 is induced, it is not essential for infection resolution (313). Moreover, trichophytin enhances the Dectin-1 expression in mice, and the blockage of Dectin-1 inhibits the increased IFN-γ production in cervical lymph node cells from mice in vitro (314). Dectin-2 preferentially binds to hyphae of various fungal species, including T. rubrum (11).
Malassezia spp.
The fungal genus Malassezia comprises yeast species that are part of the normal skin microbiota. However, Malassezia spp. can be involved in skin disorders, such as pityriasis versicolor, seborrheic dermatitis, atopic eczema, and folliculitis (315). Malassezia spp. may also cause invasive infections in infants and in immunocompromised individuals. The clinical spectrum ranges from asymptomatic infections to life-threatening sepsis and disseminated diseases (316).
Various Malassezia spp. (M. japonica, M. slooffiae, M. furfur, and M. sympodialis) induce a Dectin-1-dependent NLRP3 inflammasome activation with a subsequent IL-1β secretion in human APCs. This activation is dependent on Dectin-1, since the blocking of Dectin-1 decreased the IL-1β secretion upon M. furfur exposure (25). A Malassezia-specific ligand has not been described until now, but β-1,3-glucan is most likely present on their surface.
Several Malassezia spp. (M. pachydermatis and M. furfur) are recognized by Mincle and Dectin-2 through different ligands. A glyceroglycolipid and mannosyl fatty acids linked to mannitol are two Mincle ligands, and an O-linked mannobiose-rich glycoprotein is a ligand for Dectin-2. Both receptors cooperatively contribute to the TNF and IL-10 production in BMDCs from mice in response to Malassezia spp (36). Other results indicate that Mincle also recognizes Malassezia spp. (M. pachydermatis, M. dermatis, M. japonica, M. nana, M. slooffiae, M. sympodialis, M. furfur, and M. pachydermatis) through α-mannose but not mannan. Mincle may recognize a particular distribution of α-mannosyl residues on Malassezia spp. and use this to discriminate them from other fungi, inducing inflammatory responses (TNFα and IL-10) in murine macrophages (317).
Langerin plays a role in pathogen recognition by facilitating pathogen uptake and processing for antigen presentation (318). Langerin on primary LCs isolated from human epidermis interact strongly with M. furfur via β-glucan structures and the interaction can lead to the phagocytosis of the fungus (81). M. furfur is also recognized through MR and CR3 on THP-1 cells (77).
Fonsecaea spp.
Fonsecaea spp. are found in soil and plants. Although they are considered a worldwide-distributed fungus, they are frequently found in tropical regions (319). Fonsecaea pedrosoi is a frequent causative agent of chromoblastomycosis (or chromomycosis), a chronic fungal disease limited to the skin and subcutaneous tissues. Initial lesions are habitually erythematous papules, which progressively enlarge to morphologies, such as verrucous nodules, cauliflower-like tumors, and psoriasis-like plaques (319).
Dectin-1, Dentin-2, and Mincle have a role in the recognition of this fungus (Table 1). Murine macrophages stimulated by co-culturing with muriform cells (the parasitic form of F. pedrosoi) show an elevated expression of the Dectin-1, and by blocking Dectin-1, the phagocytosis of muriform cells, was impaired, demonstrating that muriform cells are recognized by Dectin-1 in vitro (26). F. pedrosoi spores trigger Dectin-1 and Dectin-2 signaling and induce IL-6 production, but only the Dectin-2 signaling pathway promotes the differentiation of Th17 cells, indicating that the adaptive immune response to F. pedrosoi spores in this murine infection model is determined by Dectin-2 (37). A Fonsecaea-specific ligand has not been described until today.
Mincle acts as a major receptor involved in the innate immune response to F. pedrosoi through the Syk/CARD9 pathway in murine BMDCs (68). However, another study identified Mincle as a suppressor of antifungal defenses by suppressing IL-12. The absence of IL-12 leads to impaired Th1 responses. Dectin-1 binding of F. monophora activates the transcription factor IRF1, which is crucial for the IL12A transcription. However, simultaneous binding of F. monophora to Mincle induces a Mdm2 (E3 ubiquitin ligase)-dependent degradation pathway via Syk-CARD9-mediated PKB signaling, that leads to the loss of nuclear IRF1 activity, therefore, blocking IL12A transcription (320). A Fonsecaea-specific ligand has not been described up to now for this receptor.
Regarding Mincle, it is difficult to ascertain a particular role for this CLR in Fonsecaea infection, since it is not clear if this receptor is mainly involved in the recognition and subsequent clearance of this fungus or acts as a suppressor of antifungal defenses and is exploited for immuno evasive strategies.
Microsporum spp.
Microsporum causes skin infections or dermatophytosis characterized by severe scalp itching and patchy scaly scalp skin which is highly contagious. The pathogenic species include mainly M. cani, M. gypseum, and M. hominis (321). The fungi secrete a number of enzymes and immunomodulators such as keratinolytic subtilase and keratinolytic metalloprotease as well as other cell wall glyco-proteins, endoproteases, and exoproteases (322). M. cani activates the NLRP3 inflammasomes in THP-1 cells. The production of IL-1β and its precursor are decreased in Dectin-1, Syk, and CARD-9 knockdown cells (323). Soluble Dectin-2 can bind the filamentous M. audouinii (11). Further research on CLR ligands, their recognition, and corresponding immune response in Microsporum infection are lacking.
Fusarium spp.
Fusarium species can cause superficial, locally invasive infections in immunocompetent individuals, or disseminated infections in immunocompromised patients. The infection is called fusariosis and is characterized by keratitis, onychomycosis, fungimia with or without organ involvement, and other symptoms depending upon the fungal species, port of entry, and host immune status. In humans, Fusarium solani and F. oxysporum are responsible for most cases of infections by these species (324). Fusarium spp. secrete various mycotoxins as well as certain proteases and collagenases, which modulate the immune response and destroy tissue (324).
Dectin-1 expression is highly elevated in the corneal tissue from patients infected with F. solani when compared to healthy non-infected individuals (325). Human corneal epithelial cells secrete defensive antimicrobial peptides in response to heat-killed F. solani or zymosan and this effect was Dectin-1- and TLR-2-dependent (326). Another more recent study addressed the Dectin-1-dependent CXCL-8 release from the human bronchial epithelial cell line BEAS-2B in response to F. proliferatum and showed that the chemokine release is decreased to various degrees by inhibiting Dectin-1, Syk, MAPKs, PI3K, and NFκB, respectively (27). The expression of SP-D increased in rat corneal cells after F. solani infection but its further role in murine models as well in humans is still to be established (327).
Trichosporon spp.
Trichosporon are ubiquitous dimorphic fungi, which also exist as commenals on the skin and in the gastrointestinal tract in humans. They induce superficial infections such as white piedra characterized by the presence of irregular nodules on the affected hair, as well as invasive infections such as allergic pneumonitis and trichosporonosis (invasive mycoses) especially in immuno-compromised patients and those with hematological malignancies (328, 329). T. asahii, T. asteroids, and T. mucoides are the major causes of trichosporonosis and opportunistic infections (330). Only a little is known about the interaction of these fungi with CLRs. Dectin-1 binds T. asahii via β-glucan recognition (28). Dectin-1-deficient mice with T. asahii induced hypersensitivity pneumonitis show decreased Th-17 cell populations and less monocytes/MDMs compared to wild-type mice (28).
Rare Disease Causing Fungi
Saccharomyces spp.
Classically, Saccharomyces spp. are considered safe, non-pathogenic organisms. Within this genus, Saccharomyces cerevisiae is the most important species (331). However, due to its ubiquity and long association with humans, S. cerevisiae has been implicated as a causative agent of infections in immunocompromised individuals, those with underlying diseases or medical conditions (332). Several cases of life-threatening invasive infections with S. cerevisiae resulting in pneumonia, liver abscess, and sepsis have been reported (333).
Saccharomyces cerevisiae cells cause a subtle upregulation of Dectin-1 from the moment of initial recognition in human DCs (334). Most studies have been performed by evaluating pure soluble and particulate β-glucans such as β-1,6-branched and β-1,3-d-glucan found in the S. cerevisiae cell wall (335), which can be directly recognized by Dectin-1 (29, 336). β-glucan induces Dectin-1 signaling pathways for the activation of TNFα in both human and mouse macrophages. The signaling pathways involve RTKs, ROS production, and NF-κB activation (337, 338). The Dectin-1-dependent response is essential for immunomodulatory effects on DC activation and macrophage phagocytosis. It induces the expression of immuno-regulatory cytokines, such as IL-10, TGF-β1, and IL-2 and can promote both Treg and Th17 responses (339–341). Moreover, the Dectin-1 response was investigated by observing the direct phagocytosis of β-glucan-coated particles by RAW macrophages expressing a GFP-Dectin-1 fusion protein. As expected, the β-1,3-beads induced a higher TNF-α response and a GFP-Dectin-1 recruitment to the phagosome, indicating that Dectin-1 recruitment is specific to β-1,3-glucan (342). In general, binding of particulate β-glucans to Dectin-1 triggers phagocytosis (343, 344). However, phagocytosis of β-glucan-bearing particles by human neutrophils is CR3-dependent, with a very minor role for Dectin-1, if any (78). Like C. albicans, also S. cerevisiae components in form of Zymosan are able to induce anti-inflammatory responses such as IL-10 release in human and mouse DCs and macrophages (345, 346).
Also, Langerin and DC-SIGN interact strongly with S. cerevisiae (64, 81). SP-D but not SP-A binds S. cerevisiae, and β(1→6)-glucan is a ligand for SP-D (48). Moreover, phagocytosis of unopsonized heat-killed yeast by murine macrophages is also mediated by MR (46).
Several studies have concluded that genetically determined low MBL concentrations in patients could be, at least in part, responsible for the enhanced immune reactivity to S. cerevisiae antigens (347–349). The analysis of MBL2 polymorphisms revealed an association between three variants rs930508, rs1800450, and rs5030737, with a reduction in MBL serum levels in Crohn’s disease patients (350). However, these results are in contrast with other reports in which such an association was not found. Therefore, the relationship between enhanced immune reactivity to S. cerevisiae antigens and MBL is still controversial (351, 352).
Despite its low pathogenicity, S. cerevisiae constitutes one of the better studied microorganisms, since it was developed as a model organism for several traits. Zymosan is mostly prepared from S. cerevisiae cell walls and consists of a glucan with repeating glucose units linked by β-1,3-glycosidic linkages, which have served as a model for recognition of microbes by the innate immune system for over 50 years (353). Many studies have been conducted testing and evaluating the zymosan interaction with human receptors and Dectin-1 emerged as the most important receptor for detecting Saccharomyces spp. However, for the other CLRs more studies are required in order to stablish a concrete role for them.
Exserohilum spp.
Exserohilum species are environmental fungi and, although rare, can lead to a number of human diseases such as skin and corneal infection, invasive disease, as well as allergic fungal sinusitis especially during impaired immunity, trauma, and atopy (354). Members of this genus are among the causes of phaeohyphomycosis which is characterized by the presence of dark septate mycelial elements in tissues (355). Not much work has been done to elucidate the mechanism of infection and immune responses against these fungi. A very recent work demonstrates the role of Dectin-1 in the recognition of Exserohilum rostratum (30). Mouse macrophages generate a Dectin-1 dependent TNF-α, IL-1β, MIP-1, and MIP-2 secretion in response to E. rostratum hyphae in vitro and the response is diminished in Dectin-1-deficient macrophages. However, wild-type and Dectin-1-deficient mice show no difference with respect to the type of inflammatory response and fungal control (30).
Cladosporium spp.
The Cladosporium genus consists of ubiquitous fungi that are mainly plant pathogens but few species such as those belonging to Cladosporium cladosporioides and C. herbarum complexes may cause infections in humans (356). The clinical manifestations range from keratitis, opportunistic phaeohyphomycosis including superficial or deep infections such as those of the CNS, to acnes (356–359). Cladosporium conidia are widely present in the air and have also been associated with respiratory allergy (360). The C. cladosporioides cell wall is rich in β-glucans but unavailable for recognition on live spores. A mouse in vivo study demonstrated that C. cladosporioides induces airway hyperresponsiveness and eosinophilia in a Dectin-1-independent manner (361). Furthermore, the heat-induced availability of surface β-glucans is important for a Dectin-1-dependent pulmonary IL-17 response and Dectin-1−/− mouse DCs show a decreased IL-17 response upon stimulation with heat-killed C. cladosporioides (31). The ligands on live Cladosporium cell surfaces and the corresponding CLRs, as well the subsequent immune responses are still to be investigated.
Chrysosporium spp.
The members of this genus are saprophytic soil fungi and many species are keratinolytic (362). Several case reports of superficial infections affecting nails and skin as well as opportunistic infections in immunocompromised patients have been reported (362–365). Superficial infections are mainly caused by species, such as C. keratinophilum, C. tropicum, and C. queenlandicum (362). Invasive infections are rare but have been reported (366–368). A single study demonstrated that DC-SIGN recognizes C. topicum conidia probably by recognition of fungal cell wall mannans in vitro (59).
Sporothrix spp.
These fungi are usually found living as a saprophytes thriving on decaying vegetation or soil. Sporotrichosis, caused by dimorphic Sporothrix spp., is one of the most prevalent forms of subcutaneous mycoses with a worldwide distribution particularly in tropical and subtropical regions (369, 370). While different species of clinical interest have been identified, including S. globosa, S. brasiliensis, S. Mexicana, and S. luriei, the most commonly reported species in human clinical isolates is Sporothrix schenckii (371, 372). Infection results in cutaneous or subcutaneous lesions usually with compromised adjacent lymphatic vessels. Rarely, disseminated disease can also ensue and several publications report infection of the lung, the CNS, bones, and other organs, mostly in immunocompromised individuals (372–375).
In a rat co-infection model of Tenia taeniaeformis and S. schenckii, a high expression of Dectin-1 only occurs in cutaneous lesions of co-infected rats, but is dispensable for the clearance of S. schenckii (376). However, a more recent study shows an increased Dectin-1 expression in peritoneal macrophages from S. schenckii-infected mice. Furthermore, the antibody-mediated blockade of Dectin-1 inhibits the cytokine production in response to different stimuli by peritoneal macrophages. A Dectin-1 blockade additionally results in a decreased phagocytic uptake of S. schenckii yeast cells (32). Martínez-Álvarez et al. proved that Dectin-1 is crucial for the secretion of cytokines by human PBMCs during S. schenckii infection, but dispensable for the recognition of S. brasiliensis. The authors also reported that while MR appears to have only a minor role in the recognition of S. schenckii yeast-like cells, it mediates the production of proinflammatory cytokines by human PBMCs in response to conidia from this fungus as well as yeasts from S. brasiliensis (47). An earlier study on the contribution of MR in the recognition of S. schenckii evidenced the presence of mannose residues in the cell wall of S. schenckii conidia and yeasts. Nevertheless, MR seemed to be involved only in the phagocytosis of opsonized conidia (377). Taken together, Dectin-1 seems to be important for the generation of cytokines while MR mainly plays a role in the phagocytosis of this fungus.
Challenges and Future Directions
C-type lectin receptors recognize carbohydrate ligands in fungal cell walls and years of research have provided a huge body of evidence on their importance in modulating immune responses against fungal infections. However, there are still a number of gaps to be filled and areas that need attention from the scientific community. Almost all of the fungal infecitons are opportunistic, mainly concerning people with a compromised immune system, including HIV-infected individuals. Although they are a major high-risk group, many studies exclude HIV-infected patients and, therefore, this area of research seems to be largely unexplored. More studies focusing on HIV co-infection and the genetic make-up of HIV-infected individuals which affects their susceptibility toward developing specific fungal infections would be fundamental in developing tailored therapies for such cases. Fungi such as Aspergillus and Candida species interact with a number of CLRs upon infection, opening up opportunities to study the interactions between these receptors on a functional level [for example, the formation of heteromers as precdicted in case of Mincle and MCL (378)] as well as on a genetic level, including epistatic effects that the concerned genes have on each other. Another complex aspect that still needs to be studied in detail is how different cell types interact in an in vivo environment in order to control the infection. On the other hand, there are many fungal species such as Paracoccidiodes, Fusarium, etc., with few identified immune receptors, ligands, and/or virulence factors. Discovering these ligands and their corresponding receptors or new virulence factors will not only improve our understanding of fungal interactions with immune cells but also aid in developing vaccines and diagnostic or even therapeutic strategies against these fungi. Moreover, we now also know that CLRs not only recognize carbohydrate ligands but also lipids, proteins, and nucleic acids (3). We need to widen our views and explore this aspect more fully, since the fungal cell walls in addition to their manyfold carbohydrate structures also contain a number of lipids and proteins that may as well serve as potential ligands for CLRs (379). Moreover, some recent studies have demonstrated how differential immune responses are generated by different fungal isolates or strains depending on their individual pahogenic potential and virulence (380–383). Such studies suggest that a strain-specific comparison of immune response might be essential to fully understand the host–pathogen interactions. This knowledge would be helpful in generating data for individual case-specific therapies and, therefore, such studies need to be encouraged. Furthermore, it is often noted that many studies, such as in vivo mouse studies, provide contrasting results that lead to contradictions and questions. Therefore, the scientific studies need to be designed more carefully taking into account the strains of mice and the fungal species being studied, cell type or cell lines being used, and the type of in vitro or in vivo environment provided. Also, population structures and stratification in genetic studies should be addressed. The effects of each of these factors on the outcome of the results should be appropriately discussed in order to have a comprehensive view. In summary, there is a need to further enhance the understanding of how CLRs recognize pathogenic fungi in order to promote approaches to take advantage of this knowledge for future therapeutic interventions. The ability of these receptors to bind a wide variety of pathogens which share the same ligand as fungi, makes them important molecules in the innate immune response. While there are many functional aspects of CLRs yet to be discovered, there is an increasing amount of information published over the past decade that already allows us to benefit from our knowledge on regulatory functions and new translational opportunities.
Conclusion
It is widely excepted that CLRs play a major role in modulating immune responses with respect to fungal infections, being able to recognize the carbohydrate moieties in the fungal cell walls. Fungal infections, though mainly opportunistic, can prove fatal in case of faulty diagnosis or treatment. The fungal recognition by CLRs mainly leads to proinflammatory responses and a subsequent activation of adaptive immunity via Th17 responses. However, negative or anti-inflammatory effects have also been noted and both types of responses are necessary to mount a specific immune response. A considerable body of work has been done with regards to frequent pathogens, such as Candida, Aspergillus, and Cryptococcus, etc., and genetic susceptibilities pertaining to fungal infections have been attributed to various mutations in CLRs. While some fungal infections are frequent, others are emerging into a major health problem with the continous increase in immuno-compromised patients. A thorough knowledge of the molecular mechanisms of fungal infections and the interaction of these fungi with their major receptors, the CLRs, can provide a basis to a better and more specific diagnosis and treatment regime. Also, knowledge about the host-related genetic factors, which can greatly affect the course and outcome of these infections, may advance a timely diagnosis and care for the patient.
Author Contributions
HS conceived the review framework. SG and JC-B wrote the manuscript. EK and HS revised the manuscript. JC-B and SG created the figure. All authors have read and approved the final version of the manuscript.
Conflict of Interest Statement
The authors declare that the research was conducted in the absence of any commercial or financial relationships that could be construed as a potential conflict of interest.
Acknowledgments
We thank Peder Iblher (Kreativdirektion Konzept und Design, Berlin) for the graphical improvement of the figure.
Funding
This work was supported by the Deutsche Forschungsgemeinschaft (DFG) under the framework of the Collaborative Research Center/Transregio 124 “Pathogenic fungi and their human host: Networks of Interaction,” Project A5, to HS and EK, and the International Leibniz Research School for Microbial and Biomolecular Interactions under the framework of the DAAD Graduate School Scholarship Programme to JC-B.
References
1. Plato A, Hardison SE, Brown GD. Pattern recognition receptors in antifungal immunity. Semin Immunopathol (2015) 37(2):97–106. doi:10.1007/s00281-014-0462-4
2. Geijtenbeek TBH, Gringhuis SI. Signalling through C-type lectin receptors: shaping immune responses. Nat Rev Immunol (2009) 9(7):465–79. doi:10.1038/nri2569
3. Zelensky AN, Gready JE. The C-type lectin-like domain superfamily. FEBS J (2005) 272(24):6179–217. doi:10.1111/j.1742-4658.2005.05031.x
4. Drummond RA, Gaffen SL, Hise AG, Brown GD. Innate defense against fungal pathogens. Cold Spring Harb Perspect Med (2015) 5(6). doi:10.1101/cshperspect.a019620
5. Goyal S, Klassert TE, Slevogt H. C-type lectin receptors in tuberculosis: what we know. Med Microbiol Immunol (2016) 205(6):513–35. doi:10.1007/s00430-016-0470-1
6. Drickamer K. C-type lectin-like domains. Curr Opin Struct Biol (1999) 9(5):585–90. doi:10.1016/S0959-440X(99)00009-3
7. Hoving JC, Wilson GJ, Brown GD. Signalling C-type lectin receptors, microbial recognition and immunity. Cell Microbiol (2014) 16(2):185–94. doi:10.1111/cmi.12249
8. Kerrigan AM, Brown GD. Syk-coupled C-type lectin receptors that mediate cellular activation via single tyrosine based activation motifs. Immunol Rev (2010) 234:335–52. doi:10.1111/j.0105-2896.2009.00882.x
9. Drummond RA, Lionakis MS. Mechanistic insights into the role of C-type lectin receptor/CARD9 signaling in human antifungal immunity. Front Cell Infect Microbiol (2016) 6:39. doi:10.3389/fcimb.2016.00039
10. Yamasaki S, Ishikawa E, Sakuma M, Hara H, Ogata K, Saito T. Mincle is an ITAM-coupled activating receptor that senses damaged cells. Nat Immunol (2008) 9(10):1179–88. doi:10.1038/ni.1651
11. Sato K, Yang XL, Yudate T, Chung JS, Wu JM, Luby-Phelps K, et al. Dectin-2 is a pattern recognition receptor for fungi that couples with the Fc receptor gamma chain to induce innate immune responses. J Biol Chem (2006) 281(50):38854–66. doi:10.1074/jbc.M606542200
12. Heyl KA, Klassert TE, Heinrich A, Müller MM, Klaile E, Dienemann H, et al. Dectin-1 is expressed in human lung and mediates the proinflammatory immune response to nontypeable Haemophilus influenzae. MBio (2014) 5:e1492–1414. doi:10.1128/mBio.01492-14
13. Isakov N. ITIMs and ITAMs – the Yin and Yang of antigen and Fc receptor-linked signaling machinery. Immunol Res (1997) 16(1):85–100. doi:10.1007/BF02786325
14. Richard M, Thibault N, Veilleux P, Gareau-Page G, Beaulieu AD. Granulocyte macrophage-colony stimulating factor reduces the affinity of SHP-2 for the ITIM of CLECSF6 in neutrophils: a new mechanism of action for SHP-2. Mol Immunol (2006) 43(10):1716–21. doi:10.1016/j.molimm.2005.10.006
15. Khan NS, Kasperkovitz PV, Timmons AK, Mansour MK, Tam JM, Seward MW, et al. Dectin-1 controls TLR9 trafficking to phagosomes containing β-1,3 glucan. J Immunol (2016) 196:2249–61. doi:10.4049/jimmunol.1401545
16. Sancho D, Reis e Sousa C. Signaling by myeloid C-type lectin receptors in immunity and homeostasis. Annu Rev Immunol (2012) 30:491–529. doi:10.1146/annurev-immunol-031210-101352
17. Gantner BN, Simmons RM, Underhill DM. Dectin-1 mediates macrophage recognition of Candida albicans yeast but not filaments. EMBO J (2005) 24(6):1277–86. doi:10.1038/sj.emboj.7600594
18. Giles SS, Dagenais TRT, Botts MR, Keller NP, Hull CM. Elucidating the pathogenesis of spores from the human fungal pathogen Cryptococcus neoformans. Infect Immun (2009) 77:3491–500. doi:10.1128/IAI.00334-09
19. Rapaka RR, Goetzman ES, Zheng M, Vockley J, McKinley L, Kolls JK, et al. Enhanced defense against Pneumocystis carinii mediated by a novel Dectin-1 receptor Fc fusion protein. J Immunol (2007) 178:3702–12. doi:10.4049/jimmunol.178.6.3702
20. Viriyakosol S, Fierer J, Brown GD, Kirkland TN. Innate immunity to the pathogenic fungus Coccidioides posadasii is dependent on toll-like receptor 2 and Dectin-1. Infect Immun (2005) 73:1553–60. doi:10.1128/IAI.73.3.1553-1560.2005
21. Lin J-S, Huang J-H, Hung L-Y, Wu S-Y, Wu-Hsieh BA. Distinct roles of complement receptor 3, Dectin-1, and sialic acids in murine macrophage interaction with Histoplasma yeast. J Leukoc Biol (2010) 88:95–106. doi:10.1189/jlb.1109717
22. Bonfim CV, Mamoni RL, Lima Blotta MHS. TLR-2, TLR-4 and Dectin-1 expression in human monocytes and neutrophils stimulated by Paracoccidioides brasiliensis. Med Mycol (2009) 47:722–33. doi:10.3109/13693780802641425
23. Nakamura K, Miyazato A, Koguchi Y, Adachi Y, Ohno N, Saijo S, et al. Toll-like receptor 2 (TLR2) and Dectin-1 contribute to the production of IL-12p40 by bone marrow-derived dendritic cells infected with Penicillium marneffei. Microbes Infect (2008) 10:1223–7. doi:10.1016/j.micinf.2008.06.011
24. Nakamura T, Nishibu A, Yoshida N, Yasoshima M, Anzawa K, Watanabe Y, et al. Glycyrrhetinic acid inhibits contact hypersensitivity induced by trichophytin via Dectin-1. Exp Dermatol (2016) 25:299–304. doi:10.1111/exd.12931
25. Kistowska M, Fenini G, Jankovic D, Feldmeyer L, Kerl K, Bosshard P, et al. Malassezia yeasts activate the NLRP3 inflammasome in antigen-presenting cells via Syk-kinase signalling. Exp Dermatol (2014) 23:884–9. doi:10.1111/exd.12552
26. Siqueira IM, de Castro RJA, Leonhardt LCDM, Jerônimo MRS, Soares AZC, Raiol T, et al. Modulation of the immune response by Fonsecaea pedrosoi morphotypes in the course of experimental chromoblastomycosis and their role on inflammatory response chronicity. PLoS Negl Trop Dis (2017) 11:e0005461. doi:10.1371/journal.pntd.0005461
27. Yeh CC, Horng HC, Chou H, Tai HY, Shen HD, Hsieh SL, et al. Dectin-1-mediated pathway contributes to Fusarium proliferatum-induced CXCL-8 release from human respiratory epithelial cells. Int J Mol Sci (2017) 18(3). doi:10.3390/ijms18030624
28. Higashino-Kameda M, Yabe-Wada T, Matsuba S, Takeda K, Anzawa K, Mochizuki T, et al. A critical role of Dectin-1 in hypersensitivity pneumonitis. Inflamm Res (2016) 65:235–44. doi:10.1007/s00011-015-0910-1
29. Graham LM, Tsoni SV, Willment JA, Williams DL, Taylor PR, Gordon S, et al. Soluble Dectin-1 as a tool to detect β-glucans. J Immunol Methods (2006) 314:164–9. doi:10.1016/j.jim.2006.05.013
30. Reedy JL, Negoro PE, Feliu M, Lord AK, Khan NS, Lukason DP, et al. The carbohydrate lectin receptor Dectin-1 mediates the immune response to Exserohilum rostratum. Infect Immun (2017) 85:e903–16. doi:10.1128/IAI.00903-16
31. Mintz-Cole RA, Brandt EB, Bass SA, Gibson AM, Reponen T, Hershey GKK. Surface availability of beta-glucans is critical determinant of host immune response to Cladosporium cladosporioides. J Allergy Clin Immunol (2013) 132(1):159–69. doi:10.1016/j.jaci.2013.01.003
32. Jellmayer JA, Ferreira LS, Manente FA, Goncalves AC, Polesi MC, Batista-Duharte A, et al. Dectin-1 expression by macrophages and related antifungal mechanisms in a murine model of Sporothrix schenckii sensu stricto systemic infection. Microb Pathog (2017) 110:78–84. doi:10.1016/j.micpath.2017.06.025
33. McGreal EP, Rosas M, Brown GD, Zamze S, Wong SYC, Gordon S, et al. The carbohydrate-recognition domain of Dectin-2 is a C-type lectin with specificity for high mannose. Glycobiology (2006) 16:422–30. doi:10.1093/glycob/cwj077
34. Saijo S, Ikeda S, Yamabe K, Kakuta S, Ishigame H, Akitsu A, et al. Dectin-2 recognition of alpha-mannans and induction of Th17 cell differentiation is essential for host defense against Candida albicans. Immunity (2010) 32(5):681–91. doi:10.1016/j.immuni.2010.05.001
35. Wang H, LeBert V, Hung CY, Galles K, Saijo S, Lin X, et al. C-Type lectin receptors differentially induce Th17 cells and vaccine immunity to the endemic mycosis of North America. J Immunol (2014) 192:1107–19. doi:10.4049/jimmunol.1302314
36. Ishikawa T, Itoh F, Yoshida S, Saijo S, Matsuzawa T, Gonoi T, et al. Identification of distinct ligands for the C-type lectin receptors mincle and Dectin-2 in the pathogenic fungus Malassezia. Cell Host Microbe (2013) 13:477–88. doi:10.1016/j.chom.2013.03.008
37. Wüthrich M, Wang H, Li M, Lerksuthirat T, Hardison SE, Brown GD, et al. Fonsecaea pedrosoi-induced Th17-cell differentiation in mice is fostered by Dectin-2 and suppressed by Mincle recognition. Eur J Immunol (2015) 45:2542–52. doi:10.1002/eji.201545591
38. Wang Q, Zhao G, Lin J, Li C, Jiang N, Xu Q, et al. Role of the mannose receptor during Aspergillus fumigatus infection and interaction with Dectin-1 in corneal epithelial cells. Cornea (2016) 35:267–73. doi:10.1097/ICO.0000000000000710
39. Cambi A, Netea MG, Mora-Montes HM, Gow NA, Hato SV, Lowman DW, et al. Dendritic cell interaction with Candida albicans critically depends on N-linked mannan. J Biol Chem (2008) 283(29):20590–9. doi:10.1074/jbc.M709334200
40. Szolnoky G, Bata-Csorgo Z, Kenderessy AS, Kiss M, Pivarcsi A, Novak Z, et al. A mannose-binding receptor is expressed on human keratinocytes and mediates killing of Candida albicans. J Invest Dermatol (2001) 117(2):205–13. doi:10.1046/j.1523-1747.2001.14071.x
41. Neumann AK, Jacobson K. A novel pseudopodial component of the dendritic cell anti-fungal response: the fungipod. PLoS Pathog (2010) 6(2):e1000760. doi:10.1371/journal.ppat.1000760
42. Mansour MK, Schlesinger LS, Levitz SM. Optimal T cell responses to Cryptococcus neoformans mannoprotein are dependent on recognition of conjugated carbohydrates by mannose receptors. J Immunol (2002) 168:2872–9. doi:10.4049/jimmunol.168.6.2872
43. Zhang J, Zhu J, Imrich A, Cushion M, Kinane TB, Koziel H. Pneumocystis activates human alveolar macrophage NF-kappaB signaling through mannose receptors. Infect Immun (2004) 72:3147–60. doi:10.1128/IAI.72.6.3147-3160.2004
44. Nakaira-Takahagi E, Golim MA, Bannwart CF, Puccia R, Peraçoli MTS. Interactions between TLR2, TLR4, and mannose receptors with gp43 from Paracoccidioides brasiliensis induce cytokine production by human monocytes. Med Mycol (2011) 49:1–10. doi:10.3109/13693786.2011.565485
45. Koguchi Y, Kawakami K, Kon S, Segawa T, Maeda M, Uede T, et al. Penicillium marneffei causes osteopontin-mediated production of interleukin-12 by peripheral blood mononuclear cells. Infect Immun (2002) 70(3):1042–8. doi:10.1128/IAI.70.3.1042-1048.2002
46. Giaimis J, Lombard Y, Fonteneau P, Muller CD, Levy R, Makaya-Kumba M, et al. Both mannose and beta-glucan receptors are involved in phagocytosis of unopsonized, heat-killed Saccharomyces cerevisiae by murine macrophages. J Leukoc Biol (1993) 54:564–71. doi:10.1002/jlb.54.6.564
47. Martinez-Alvarez JA, Perez-Garcia LA, Mellado-Mojica E, Lopez MG, Martinez-Duncker I, Lopes-Bezerra LM, et al. Sporothrix schenckii sensu stricto and Sporothrix brasiliensis are differentially recognized by human peripheral blood mononuclear cells. Front Microbiol (2017) 8:843. doi:10.3389/fmicb.2017.00843
48. Allen MJ, Voelker DR, Mason RJ. Interactions of surfactant proteins A and D with Saccharomyces cerevisiae and Aspergillus fumigatus. Infect Immun (2001) 69:2037–44. doi:10.1128/IAI.69.4.2037-2044.2001
49. Schelenz S, Malhotra R, Sim RB, Holmskov U, Bancroft GJ. Binding of host collectins to the pathogenic yeast Cryptococcus neoformans: human surfactant protein D acts as an agglutinin for acapsular yeast cells. Infect Immun (1995) 63:3360–6.
50. van de Wetering JK, Coenjaerts FEJ, Vaandrager AB, van Golde LMG, Batenburg JJ. Aggregation of Cryptococcus neoformans by surfactant protein D is inhibited by its capsular component glucuronoxylomannan. Infect Immun (2004) 72:145–53. doi:10.1128/IAI.72.1.145-153.2004
51. Vuk-Pavlovic Z, Standing JE, Crouch EC, Limper AH. Carbohydrate recognition domain of surfactant protein D mediates interactions with Pneumocystis carinii glycoprotein A. Am J Respir Cell Mol Biol (2001) 24:475–84. doi:10.1165/ajrcmb.24.4.3504
52. McCormack FX, Festa AL, Andrews RP, Linke M, Walzer PD. The carbohydrate recognition domain of surfactant protein A mediates binding to the major surface glycoprotein of Pneumocystis carinii. Biochemistry (1997) 36:8092–9. doi:10.1021/bi970313f
53. Awasthi S, Magee DM, Coalson JJ. Coccidioides posadasii infection alters the expression of pulmonary surfactant proteins (SP)-A and SP-D. Respir Res (2004) 5:28. doi:10.1186/1465-9921-5-28
54. McCormack FX, Gibbons R, Ward SR, Kuzmenko A, Wu H, Deepe GS. Macrophage-independent fungicidal action of the pulmonary collectins. J Biol Chem (2003) 278:36250–6. doi:10.1074/jbc.M303086200
55. Neth O, Jack DL, Dodds AW, Holzel H, Klein NJ, Turner MW. Mannose-binding lectin binds to a range of clinically relevant microorganisms and promotes complement deposition. Infect Immun (2000) 68:688–93. doi:10.1128/IAI.68.2.688-693.2000
56. Lillegard JB, Sim RB, Thorkildson P, Gates MA, Kozel TR. Recognition of Candida albicans by mannan-binding lectin in vitro and in vivo. J Infect Dis (2006) 193(11):1589–97. doi:10.1086/503804
57. Mershon-Shier KL, Vasuthasawat A, Takahashi K, Morrison SL, Beenhouwer DO. In vitro C3 deposition on cryptococcus capsule occurs via multiple complement activation pathways. Mol Immunol (2011) 48:2009–18. doi:10.1016/j.molimm.2011.06.215
58. Laursen AL, Obel N, Holmskov U, Jensenius JC, Aliouat EM, Andersen PL. Activation of the respiratory burst by Pneumocystis carinii. Efficiency of different antibody isotypes, complement, lung surfactant protein D, and mannan-binding lectin. APMIS (2003) 111:405–15. doi:10.1034/j.1600-0463.2003.t01-1-1110205.x
59. Serrano-Gómez D, Leal JA, Corbí AL. DC-SIGN mediates the binding of Aspergillus fumigatus and keratinophylic fungi by human dendritic cells. Immunobiology (2005) 210:175–83. doi:10.1016/j.imbio.2005.05.011
60. Serrano-Gómez D, Domínguez-Soto A, Ancochea J, Jimenez-Heffernan JA, Leal JA, Corbí AL. Dendritic cell-specific intercellular adhesion molecule 3-grabbing nonintegrin mediates binding and internalization of Aspergillus fumigatus conidia by dendritic cells and macrophages. J Immunol (2004) 173:5635–43. doi:10.4049/jimmunol.173.9.5635
61. Cambi A, Gijzen K, de Vries IJM, Torensma R, Joosten B, Adema GJ, et al. The C-type lectin DC-SIGN (CD209) is an antigen-uptake receptor for Candida albicans on dendritic cells. Eur J Immunol (2003) 33:532–8. doi:10.1002/immu.200310029
62. Mansour MK, Latz E, Levitz SM. Cryptococcus neoformans glycoantigens are captured by multiple lectin receptors and presented by dendritic cells. J Immunol (2006) 176:3053–61. doi:10.4049/jimmunol.176.5.3053
63. Ngaosuwankul P, Pongtanalert P, Engering A, Chaiyaroj SC. Differential gene expression profiles of human monocyte-derived antigen presenting cells in response to Penicillium marneffei: roles of DC-SIGN (CD209) in fungal cell uptake. Asian Pac J Allergy Immunol (2008) 26(2–3):151–63.
64. Takahara K, Arita T, Tokieda S, Shibata N, Okawa Y, Tateno H, et al. Difference in fine specificity to polysaccharides of Candida albicans mannoprotein between mouse SIGNR1 and human DC-SIGN. Infect Immun (2012) 80:1699–706. doi:10.1128/IAI.06308-11
65. Zhao G, Xu Q, Lin J, Chen W, Cui T, Hu L, et al. The role of Mincle in innate immune to fungal keratitis. J Infect Dev Ctries (2017) 11:89–97. doi:10.3855/jidc.7570
66. Wells CA, Salvage-Jones JA, Li X, Hitchens K, Butcher S, Murray RZ, et al. The macrophage-inducible C-type lectin, Mincle, is an essential component of the innate immune response to Candida albicans. J Immunol (2008) 180(11):7404–13. doi:10.4049/jimmunol.180.11.7404
67. Kottom TJ, Hebrink DM, Jenson PE, Nandakumar V, Wüthrich M, Wang H, et al. The interaction of Pneumocystis with the C-type lectin receptor mincle exerts a significant role in host defense against infection. J Immunol (2017) 198:3515–25. doi:10.4049/jimmunol.1600744
68. da Glória Sousa M, Reid DM, Schweighoffer E, Tybulewicz V, Ruland JR, Langhorne J, et al. Restoration of pattern recognition receptor costimulation to treat chromoblastomycosis, a chronic fungal infection of the skin. Cell Host Microbe (2011) 9:436–43. doi:10.1016/j.chom.2011.04.005
69. Kerscher B, Willment JA, Brown GD. The Dectin-2 family of C-type lectin-like receptors: an update. Int Immunol (2013) 25(5):271–7. doi:10.1093/intimm/dxt006
70. Zhu LL, Zhao XQ, Jiang C, You Y, Chen XP, Jiang YY, et al. C-type lectin receptors Dectin-3 and Dectin-2 form a heterodimeric pattern-recognition receptor for host defense against fungal infection. Immunity (2013) 39:324–34. doi:10.1016/j.immuni.2013.05.017
71. Hole CR, Leopold Wager CM, Mendiola AS, Wozniak KL, Campuzano A, Lin X, et al. Antifungal activity of plasmacytoid dendritic cells against Cryptococcus neoformans in vitro requires expression of Dectin-3 (CLEC4D) and reactive oxygen species. Infect Immun (2016) 84:2493–504. doi:10.1128/IAI.00103-16
72. Gresnigt MS, Becker KL, Smeekens SP, Jacobs CWM, Joosten LAB, van der Meer JWM, et al. Aspergillus fumigatus-induced IL-22 is not restricted to a specific Th cell subset and is dependent on complement receptor 3. J Immunol (2013) 190:5629–39. doi:10.4049/jimmunol.1202601
73. Forsyth CB, Plow EF, Zhang L. Interaction of the fungal pathogen Candida albicans with integrin CD11b/CD18: recognition by the I domain is modulated by the lectin-like domain and the CD18 subunit. J Immunol (1998)161(11):6198–205.
74. Soloviev DA, Fonzi WA, Sentandreu R, Pluskota E, Forsyth CB, Yadav S, et al. Identification of pH-regulated antigen 1 released from Candida albicans as the major ligand for leukocyte integrin alphaMbeta2. J Immunol (2007) 178(4):2038–46. doi:10.4049/jimmunol.178.4.2038
75. Huang J-H, Lin C-Y, Wu S-Y, Chen W-Y, Chu C-L, Brown GD, et al. CR3 and Dectin-1 collaborate in macrophage cytokine response through association on lipid rafts and activation of Syk-JNK-AP-1 pathway. PLoS Pathog (2015) 11:e1004985. doi:10.1371/journal.ppat.1004985
76. Jiménez Mdel P, Restrepo A, Radzioch D, Cano LE, García LF. Importance of complement 3 and mannose receptors in phagocytosis of Paracoccidioides brasiliensis conidia by Nramp1 congenic macrophages lines. FEMS Immunol Med Microbiol (2006) 47:56–66. doi:10.1111/j.1574-695X.2006.00059.x
77. Suzuki T, Ohno N, Ohshima Y, Yadomae T. Soluble mannan and Î2-glucan inhibit the uptake of Malassezia furfur by human monocytic cell line, THP-1. FEMS Immunol Med Microbiol (1998) 21:223–30. doi:10.1111/j.1574-695X.1998.tb01169.x
78. van Bruggen R, Drewniak A, Jansen M, van Houdt M, Roos D, Chapel H, et al. Complement receptor 3, not Dectin-1, is the major receptor on human neutrophils for β-glucan-bearing particles. Mol Immunol (2009) 47:575–81. doi:10.1016/j.molimm.2009.09.018
79. Gao X, Zhao G, Li C, Lin J, Jiang N, Wang Q, et al. LOX-1 and TLR4 affect each other and regulate the generation of ROS in A. fumigatus keratitis. Int Immunopharmacol (2016) 40:392–9. doi:10.1016/j.intimp.2016.09.027
80. Li C, Zhao G, Che C, Lin J, Li N, Hu L, et al. The role of LOX-1 in innate immunity to Aspergillus fumigatus in corneal epithelial cells. Invest Opthalmol Vis Sci (2015) 56:3593. doi:10.1167/iovs.14-15989
81. de Jong MA, Vriend LE, Theelen B, Taylor ME, Fluitsma D, Boekhout T, et al. C-type lectin Langerin is a beta-glucan receptor on human Langerhans cells that recognizes opportunistic and pathogenic fungi. Mol Immunol (2010) 47:1216–25. doi:10.1016/j.molimm.2009.12.016
82. Stappers MHT, Clark AE, Aimanianda V, Bidula S, Reid DM, Asamaphan P, et al. Recognition of DHN-melanin by a C-type lectin receptor is required for immunity to Aspergillus. Nature (2018) 555(7696):382–6. doi:10.1038/nature25974
83. Havlickova B, Czaika VA, Friedrich M. Epidemiological trends in skin mycoses worldwide. Mycoses (2008) 51(Suppl 4):2–15. doi:10.1111/j.1439-0507.2008.01606.x
84. Brown GD, Denning DW, Gow NA, Levitz SM, Netea MG, White TC. Hidden killers: human fungal infections. Sci Transl Med (2012) 4(165):165rv13. doi:10.1126/scitranslmed.3004404
85. Kim JY. Human fungal pathogens: Why should we learn? J Microbiol (2016) 54:145–8. doi:10.1007/s12275-016-0647-8
86. Gow NAR, Latge JP, Munro CA. The fungal cell wall: structure, biosynthesis, and function. Microbiol Spectr (2017) 5(3). doi:10.1128/microbiolspec.FUNK-0035-2016
87. Warris A. The biology of pulmonary Aspergillus infections. J Infect (2014) 69:S36–41. doi:10.1016/j.jinf.2014.07.011
88. Loures FV, Röhm M, Lee CK, Santos E, Wang JP, Specht CA, et al. Recognition of Aspergillus fumigatus hyphae by human plasmacytoid dendritic cells is mediated by Dectin-2 and results in formation of extracellular traps. PLoS Pathog (2015) 11:e1004643. doi:10.1371/journal.ppat.1004643
89. Gersuk GM, Underhill DM, Zhu L, Marr KA. Dectin-1 and TLRs permit macrophages to distinguish between different Aspergillus fumigatus cellular states. J Immunol (2006) 176:3717–24. doi:10.4049/jimmunol.176.6.3717
90. Sun H, Xu X-Y, Shao H-T, Su X, Wu X-D, Wang Q, et al. Dectin-2 is predominately macrophage restricted and exhibits conspicuous expression during Aspergillus fumigatus invasion in human lung. Cell Immunol (2013) 284:60–7. doi:10.1016/j.cellimm.2013.06.013
91. Madan T, Eggleton P, Kishore U, Strong P, Aggrawal SS, Sarma PU, et al. Binding of pulmonary surfactant proteins A and D to Aspergillus fumigatus conidia enhances phagocytosis and killing by human neutrophils and alveolar macrophages. Infect Immun (1997) 65:3171–9.
92. Steele C, Rapaka RR, Metz A, Pop SM, Williams DL, Gordon S, et al. The beta-glucan receptor Dectin-1 recognizes specific morphologies of Aspergillus fumigatus. PLoS Pathog (2005) 1:e42. doi:10.1371/journal.ppat.0010042
93. Hohl TM, Van Epps HL, Rivera A, Morgan LA, Chen PL, Feldmesser M, et al. Aspergillus fumigatus triggers inflammatory responses by stage-specific β-glucan display. PLoS Pathog (2005) 1:e30. doi:10.1371/journal.ppat.0010030
94. Lamoth F, Rubino I, Bochud P-Y. Immunogenetics of invasive aspergillosis. Med Mycol (2011) 49:S125–36. doi:10.3109/13693786.2010.516408
95. Gravelat FN, Beauvais A, Liu H, Lee MJ, Snarr BD, Chen D, et al. Aspergillus galactosaminogalactan mediates adherence to host constituents and conceals hyphal β-glucan from the immune system. PLoS Pathog (2013) 9:e1003575. doi:10.1371/journal.ppat.1003575
96. Savers A, Rasid O, Parlato M, Brock M, Jouvion G, Ryffel B, et al. Infection-mediated priming of phagocytes protects against lethal secondary Aspergillus fumigatus challenge. PLoS One (2016) 11:e0153829. doi:10.1371/journal.pone.0153829
97. Yang J-X, Liu W, Lu Q-Y, Wan Z, Wang X-H, Li R-Y. Different expression of Dectin-1 and toll-like receptor 2 in the lungs of different immune status mice infected with Aspergillus fumigatus. Chin Med J(Engl) (2009) 122:2017–21.
98. Werner JL, Metz AE, Horn D, Schoeb TR, Hewitt MM, Schwiebert LM, et al. Requisite role for the Dectin-1-glucan receptor in pulmonary defense against Aspergillus fumigatus. J Immunol (2009) 182:4938–46. doi:10.4049/jimmunol.0804250
99. Mezger M, Kneitz S, Wozniok I, Kurzai O, Einsele H, Loeffler J. Proinflammatory response of immature human dendritic cells is mediated by Dectin-1 after exposure to Aspergillus fumigatus germ tubes. J Infect Dis (2008) 197:924–31. doi:10.1086/528694
100. Wang M, Liu Z, Liu C, Wu T, Cai F, Wang Q, et al. PU.1 is involved in the immune response to Aspergillus fumigatus through upregulating Dectin-1 expression. BMC Infect Dis (2016) 16:297. doi:10.1186/s12879-016-1632-x
101. Serezani CH, Kane S, Collins L, Morato-Marques M, Osterholzer JJ, Peters-Golden M. Macrophage Dectin-1 expression is controlled by leukotriene B4 via a GM-CSF/PU.1 axis. J Immunol (2012) 189:906–15. doi:10.4049/jimmunol.1200257
102. Toyotome T, Adachi Y, Watanabe A, Ochiai E, Ohno N, Kamei K. Activator protein 1 is triggered by Aspergillus fumigatus β-glucans surface-exposed during specific growth stages. Microb Pathog (2008) 44:141–50. doi:10.1016/j.micpath.2007.08.015
103. Luther K, Torosantucci A, Brakhage AA, Heesemann J, Ebel F. Phagocytosis of Aspergillus fumigatus conidia by murine macrophages involves recognition by the Dectin-1 beta-glucan receptor and toll-like receptor 2. Cell Microbiol (2007) 9:368–81. doi:10.1111/j.1462-5822.2006.00796.x
104. Camargo JF, Bhimji A, Kumar D, Kaul R, Pavan R, Schuh A, et al. Impaired T cell responsiveness to interleukin-6 in hematological patients with invasive aspergillosis. PLoS One (2015) 10:e0123171. doi:10.1371/journal.pone.0123171
105. Xia D, Sun W-K, Tan M-M, Ding Y, Liu Z-C, Li P, et al. An adenoviral vector encoding full-length Dectin-1 promotes Aspergillus-induced innate immune response in macrophages. Lung (2015) 193:549–57. doi:10.1007/s00408-015-9740-8
106. Sun W-K, Lu X, Li X, Sun Q-Y, Su X, Song Y, et al. Dectin-1 is inducible and plays a crucial role in Aspergillus-induced innate immune responses in human bronchial epithelial cells. Eur J Clin Microbiol Infect Dis (2012) 31:2755–64. doi:10.1007/s10096-012-1624-8
107. Rivera A, Hohl TM, Collins N, Leiner I, Gallegos A, Saijo S, et al. Dectin-1 diversifies Aspergillus fumigatus-specific T cell responses by inhibiting T helper type 1 CD4 T cell differentiation. J Exp Med (2011) 208:369–81. doi:10.1084/jem.20100906
108. Zhong J, Huang W, Deng Q, Wu M, Jiang H, Lin X, et al. Inhibition of TREM-1 and Dectin-1 alleviates the severity of fungal keratitis by modulating innate immune responses. PLoS One (2016) 11:e0150114. doi:10.1371/journal.pone.0150114
109. Xu Q, Zhao G, Lin J, Wang Q, Hu L, Jiang Z. Role of Dectin-1 in the innate immune response of rat corneal epithelial cells to Aspergillus fumigatus. BMC Ophthalmol (2015) 15:126. doi:10.1186/s12886-015-0112-1
110. Leal SM, Cowden S, Hsia Y-C, Ghannoum MA, Momany M, Pearlman E. Distinct roles for Dectin-1 and TLR4 in the pathogenesis of Aspergillus fumigatus keratitis. PLoS Pathog (2010) 6:e1000976. doi:10.1371/journal.ppat.1000976
111. Sun H, Xu X-Y, Tian X-L, Shao H-T, Wu X-D, Wang Q, et al. Activation of NF-κB and respiratory burst following Aspergillus fumigatus stimulation of macrophages. Immunobiology (2014) 219:25–36. doi:10.1016/j.imbio.2013.06.013
112. Allen MJ, Harbeck R, Smith B, Voelker DR, Mason RJ. Binding of rat and human surfactant proteins A and D to Aspergillus fumigatus conidia. Infect Immun (1999) 67:4563–9.
113. Kaur S, Gupta VK, Thiel S, Sarma PU, Madan T. Protective role of mannan-binding lectin in a murine model of invasive pulmonary aspergillosis. Clin Exp Immunol (2007) 148:382–9. doi:10.1111/j.1365-2249.2007.03351.x
114. Geunes-Boyer S, Heitman J, Wright JR, Steinbach WJ. Surfactant protein D binding to Aspergillus fumigatus hyphae is calcineurin-sensitive. Med Mycol (2010) 48:580–8. doi:10.3109/13693780903401682
115. Singh M, Madan T, Waters P, Sonar S, Singh SK, Kamran MF, et al. Therapeutic effects of recombinant forms of full-length and truncated human surfactant protein D in a murine model of invasive pulmonary aspergillosis. Mol Immunol (2009) 46:2363–9. doi:10.1016/j.molimm.2009.03.019
116. Che C-Y, Jia W-Y, Xu Q, Li N, Hu L-T, Jiang N, et al. The roles of surfactant protein D during Aspergillus fumigatus infection in human corneal epithelial cells. Int J Ophthalmol (2012) 5:13–7. doi:10.3980/j.issn.2222-3959.2012.01.03
117. Wu X, Zhao G, Lin J, Jiang N, Li C, Hu L, et al. The production mechanism and immunosuppression effect of pulmonary surfactant protein D via toll like receptor 4 signaling pathway in human corneal epithelial cells during Aspergillus fumigatus infection. Int Immunopharmacol (2015) 29:433–9. doi:10.1016/j.intimp.2015.10.018
118. Sainz J, Lupiáñez CB, Segura-Catena J, Vazquez L, Ríos R, Oyonarte S, et al. Dectin-1 and DC-SIGN polymorphisms associated with invasive pulmonary aspergillosis infection. PLoS One (2012) 7:e32273. doi:10.1371/journal.pone.0032273
119. Cunha C, Di Ianni M, Bozza S, Giovannini G, Zagarella S, Zelante T, et al. Dectin-1 Y238X polymorphism associates with susceptibility to invasive aspergillosis in hematopoietic transplantation through impairment of both recipient- and donor-dependent mechanisms of antifungal immunity. Blood (2010) 116:5394–402. doi:10.1182/blood-2010-04-279307
120. Chai LYA, de Boer MGJ, van der Velden WJFM, Plantinga TS, van Spriel AB, Jacobs C, et al. The Y238X stop codon polymorphism in the human β-glucan receptor Dectin-1 and susceptibility to invasive aspergillosis. J Infect Dis (2011) 203:736–43. doi:10.1093/infdis/jiq102
121. Qu X, Che C, Gao A, Lin J, Wang N, Du X, et al. Association of Dectin-1 and DC-SIGN gene single nucleotide polymorphisms with fungal keratitis in the Northern Han Chinese population. Mol Vis (2015) 21:391–402.
122. Sainz J, Segura-Catena J, Jurado M. [Association between genetic polymorphism in the promotor region of CD209 and propensity to develop invasive pulmonary aspergillosis]. Methods Find Exp Clin Pharmacol (2010) 32(Suppl A):9–13.
123. Madan T. Potential of lung surfactant proteins, SP-A and SP-D, and mannan binding lectin for therapy and genetic predisposition to allergic and invasive aspergillosis. Recent Pat Inflamm Allergy Drug Discov (2007) 1:183–7. doi:10.2174/187221307782418874
124. Madan T, Kaur S, Saxena S, Singh M, Kishore U, Thiel S, et al. Role of collectins in innate immunity against aspergillosis. Med Mycol (2005) 43:155–63. doi:10.1080/13693780500088408
125. Kaur S, Gupta VK, Shah A, Thiel S, Sarma PU, Madan T. Elevated levels of mannan-binding leptin (MBL) and eosinophilia in patients of bronchial asthma with allergic rhinitis and allergic bronchopulmonary aspergillosis associate with a novel intronic polymorphism in MBL. Clin Exp Immunol (2006) 143:414–9. doi:10.1111/j.1365-2249.2006.03007.x
126. Saxena S, Madan T, Shah A, Muralidhar K, Sarma PU. Association of polymorphisms in the collagen region of SP-A2 with increased levels of total IgE antibodies and eosinophilia in patients with allergic bronchopulmonary aspergillosis. J Allergy Clin Immunol (2003) 111:1001–7. doi:10.1067/mai.2003.1395
127. Vaid M, Kaur S, Sambatakou H, Madan T, Denning DW, Sarma PU. Distinct alleles of mannose-binding lectin (MBL) and surfactant proteins A (SP-A) in patients with chronic cavitary pulmonary aspergillosis and allergic bronchopulmonary aspergillosis. Clin Chem Lab Med (2007) 45:183–6. doi:10.1515/CCLM.2007.033
128. Crosdale DJ, Poulton KV, Ollier WE, Thomson W, Denning DW. Mannose-binding lectin gene polymorphisms as a susceptibility factor for chronic necrotizing pulmonary aspergillosis. J Infect Dis (2001) 184(5):653–6. doi:10.1086/322791
129. Gow NA, van de Veerdonk FL, Brown AJ, Netea MG. Candida albicans morphogenesis and host defence: discriminating invasion from colonization. Nat Rev Microbiol (2011) 10(2):112–22. doi:10.1038/nrmicro2711
130. Calderone RA, Fonzi WA. Virulence factors of Candida albicans. Trends Microbiol (2001) 9(7):327–35. doi:10.1016/S0966-842X(01)02094-7
131. Hani U, Shivakumar HG, Vaghela R, Osmani RA, Shrivastava A. Candidiasis: a fungal infection – current challenges and progress in prevention and treatment. Infect Disord Drug Targets (2015) 15(1):42–52. doi:10.2174/1871526515666150320162036
132. Brown GD, Herre J, Williams DL, Willment JA, Marshall AS, Gordon S. Dectin-1 mediates the biological effects of beta-glucans. J Exp Med (2003) 197(9):1119–24. doi:10.1084/jem.20021890
133. Adachi Y, Ishii T, Ikeda Y, Hoshino A, Tamura H, Aketagawa J, et al. Characterization of beta-glucan recognition site on C-type lectin, Dectin 1. Infect Immun (2004) 72(7):4159–71. doi:10.1128/IAI.72.7.4159-4171.2004
134. Kashem SW, Igyarto BZ, Gerami-Nejad M, Kumamoto Y, Mohammed JA, Jarrett E, et al. Candida albicans morphology and dendritic cell subsets determine T helper cell differentiation. Immunity (2015) 42(2):356–66. doi:10.1016/j.immuni.2015.01.008
135. Bain JM, Louw J, Lewis LE, Okai B, Walls CA, Ballou ER, et al. Candida albicans hypha formation and mannan masking of beta-glucan inhibit macrophage phagosome maturation. MBio (2014) 5(6):e01874. doi:10.1128/mBio.01874-14
136. Cheng SC, van de Veerdonk FL, Lenardon M, Stoffels M, Plantinga T, Smeekens S, et al. The Dectin-1/inflammasome pathway is responsible for the induction of protective T-helper 17 responses that discriminate between yeasts and hyphae of Candida albicans. J Leukoc Biol (2011) 90(2):357–66. doi:10.1189/jlb.1210702
137. Gantner BN, Simmons RM, Canavera SJ, Akira S, Underhill DM. Collaborative induction of inflammatory responses by Dectin-1 and toll-like receptor 2. J Exp Med (2003) 197(9):1107–17. doi:10.1084/jem.20021787
138. Rogers NC, Slack EC, Edwards AD, Nolte MA, Schulz O, Schweighoffer E, et al. Syk-dependent cytokine induction by Dectin-1 reveals a novel pattern recognition pathway for C type lectins. Immunity (2005) 22(4):507–17. doi:10.1016/j.immuni.2005.06.005
139. Underhill DM, Rossnagle E, Lowell CA, Simmons RM. Dectin-1 activates Syk tyrosine kinase in a dynamic subset of macrophages for reactive oxygen production. Blood (2005) 106(7):2543–50. doi:10.1182/blood-2005-03-1239
140. Gross O, Gewies A, Finger K, Schafer M, Sparwasser T, Peschel C, et al. Card9 controls a non-TLR signalling pathway for innate anti-fungal immunity. Nature (2006) 442(7103):651–6. doi:10.1038/nature04926
141. Cohen-Kedar S, Baram L, Elad H, Brazowski E, Guzner-Gur H, Dotan I. Human intestinal epithelial cells respond to beta-glucans via Dectin-1 and Syk. Eur J Immunol (2014) 44(12):3729–40. doi:10.1002/eji.201444876
142. Gow NA, Netea MG, Munro CA, Ferwerda G, Bates S, Mora-Montes HM, et al. Immune recognition of Candida albicans beta-glucan by Dectin-1. J Infect Dis (2007) 196(10):1565–71. doi:10.1086/523110
143. Smeekens SP, Gresnigt MS, Becker KL, Cheng SC, Netea SA, Jacobs L, et al. An anti-inflammatory property of Candida albicans beta-glucan: induction of high levels of interleukin-1 receptor antagonist via a Dectin-1/CR3 independent mechanism. Cytokine (2015) 71(2):215–22. doi:10.1016/j.cyto.2014.10.013
144. Da Silva CA, Chalouni C, Williams A, Hartl D, Lee CG, Elias JA. Chitin is a size-dependent regulator of macrophage TNF and IL-10 production. J Immunol (2009) 182(6):3573–82. doi:10.4049/jimmunol.0802113
145. Singh A, Lelis F, Braig S, Schafer I, Hartl D, Rieber N. Differential regulation of myeloid-derived suppressor cells by Candida species. Front Microbiol (2016) 7:1624. doi:10.3389/fmicb.2016.01624
146. de Turris V, Teloni R, Chiani P, Bromuro C, Mariotti S, Pardini M, et al. Candida albicans targets a lipid raft/Dectin-1 platform to enter human monocytes and induce antigen specific T cell responses. PLoS One (2015) 10:e0142531. doi:10.1371/journal.pone.0142531
147. Smith IM, Baker A, Christensen JE, Boekhout T, Frokiaer H, Arneborg N, et al. Kluyveromyces marxianus and Saccharomyces boulardii induce distinct levels of dendritic cell cytokine secretion and significantly different T cell responses in vitro. PLoS One (2016) 11(11):e0167410. doi:10.1371/journal.pone.0167410
148. Daley D, Mani VR, Mohan N, Akkad N, Ochi A, Heindel DW, et al. Dectin 1 activation on macrophages by galectin 9 promotes pancreatic carcinoma and peritumoral immune tolerance. Nat Med (2017) 23(5):556–67. doi:10.1038/nm.4314
149. Strasser D, Neumann K, Bergmann H, Marakalala MJ, Guler R, Rojowska A, et al. Syk kinase-coupled C-type lectin receptors engage protein kinase C-sigma to elicit Card9 adaptor-mediated innate immunity. Immunity (2012) 36(1):32–42. doi:10.1016/j.immuni.2011.11.015
150. Nieto-Patlan A, Campillo-Navarro M, Rodriguez-Cortes O, Munoz-Cruz S, Wong-Baeza I, Estrada-Parra S, et al. Recognition of Candida albicans by Dectin-1 induces mast cell activation. Immunobiology (2015) 220(9):1093–100. doi:10.1016/j.imbio.2015.05.005
151. Pinke KH, Lima HG, Cunha FQ, Lara VS. Mast cells phagocyte Candida albicans and produce nitric oxide by mechanisms involving TLR2 and Dectin-1. Immunobiology (2016) 221(2):220–7. doi:10.1016/j.imbio.2015.09.004
152. Netea MG, Gow NA, Munro CA, Bates S, Collins C, Ferwerda G, et al. Immune sensing of Candida albicans requires cooperative recognition of mannans and glucans by lectin and toll-like receptors. J Clin Invest (2006) 116(6):1642–50. doi:10.1172/JCI27114
153. Ferwerda G, Meyer-Wentrup F, Kullberg BJ, Netea MG, Adema GJ. Dectin-1 synergizes with TLR2 and TLR4 for cytokine production in human primary monocytes and macrophages. Cell Microbiol (2008) 10(10):2058–66. doi:10.1111/j.1462-5822.2008.01188.x
154. del Fresno C, Soulat D, Roth S, Blazek K, Udalova I, Sancho D, et al. Interferon-beta production via Dectin-1-Syk-IRF5 signaling in dendritic cells is crucial for immunity to C. albicans. Immunity (2013) 38(6):1176–86. doi:10.1016/j.immuni.2013.05.010
155. Jia XM, Tang B, Zhu LL, Liu YH, Zhao XQ, Gorjestani S, et al. CARD9 mediates Dectin-1-induced ERK activation by linking Ras-GRF1 to H-Ras for antifungal immunity. J Exp Med (2014) 211(11):2307–21. doi:10.1084/jem.20132349
156. Kennedy AD, Willment JA, Dorward DW, Williams DL, Brown GD, DeLeo FR. Dectin-1 promotes fungicidal activity of human neutrophils. Eur J Immunol (2007) 37(2):467–78. doi:10.1002/eji.200636653
157. Maneu V, Yanez A, Murciano C, Molina A, Gil ML, Gozalbo D. Dectin-1 mediates in vitro phagocytosis of Candida albicans yeast cells by retinal microglia. FEMS Immunol Med Microbiol (2011) 63(1):148–50. doi:10.1111/j.1574-695X.2011.00829.x
158. Mansour MK, Tam JM, Khan NS, Seward M, Davids PJ, Puranam S, et al. Dectin-1 activation controls maturation of beta-1,3-glucan-containing phagosomes. J Biol Chem (2013) 288(22):16043–54. doi:10.1074/jbc.M113.473223
159. Goodridge HS, Simmons RM, Underhill DM. Dectin-1 stimulation by Candida albicans yeast or zymosan triggers NFAT activation in macrophages and dendritic cells. J Immunol (2007) 178(5):3107–15. doi:10.4049/jimmunol.178.5.3107
160. Gringhuis SI, den Dunnen J, Litjens M, van der Vlist M, Wevers B, Bruijns SC, et al. Dectin-1 directs T helper cell differentiation by controlling noncanonical NF-kappaB activation through Raf-1 and Syk. Nat Immunol (2009) 10(2):203–13. doi:10.1038/ni.1692
161. Smeekens SP, van de Veerdonk FL, van der Meer JW, Kullberg BJ, Joosten LA, Netea MG. The Candida Th17 response is dependent on mannan- and beta-glucan-induced prostaglandin E2. Int Immunol (2010) 22(11):889–95. doi:10.1093/intimm/dxq442
162. van de Veerdonk FL, Joosten LA, Devesa I, Mora-Montes HM, Kanneganti TD, Dinarello CA, et al. Bypassing pathogen-induced inflammasome activation for the regulation of interleukin-1beta production by the fungal pathogen Candida albicans. J Infect Dis (2009) 199(7):1087–96. doi:10.1086/597274
163. Hise AG, Tomalka J, Ganesan S, Patel K, Hall BA, Brown GD, et al. An essential role for the NLRP3 inflammasome in host defense against the human fungal pathogen Candida albicans. Cell Host Microbe (2009) 5(5):487–97. doi:10.1016/j.chom.2009.05.002
164. Gringhuis SI, Kaptein TM, Wevers BA, Theelen B, van der Vlist M, Boekhout T, et al. Dectin-1 is an extracellular pathogen sensor for the induction and processing of IL-1 beta via a noncanonical caspase-8 inflammasome. Nat Immunol (2012) 13(3):246–54. doi:10.1038/ni.2222
165. Ganesan S, Rathinam VAK, Bossaller L, Army K, Kaiser WJ, Mocarski ES, et al. Caspase-8 modulates Dectin-1 and complement receptor 3-driven IL-1beta production in response to beta-glucans and the fungal pathogen, Candida albicans. J Immunol (2014) 193(5):2519–30. doi:10.4049/jimmunol.1400276
166. LeibundGut-Landmann S, Gross O, Robinson MJ, Osorio F, Slack EC, Tsoni SV, et al. Syk- and CARD9-dependent coupling of innate immunity to the induction of T helper cells that produce interleukin 17. Nat Immunol (2007) 8(6):630–8. doi:10.1038/ni1460
167. Takahara K, Tokieda S, Nagaoka K, Takeda T, Kimura Y, Inaba K. C-type lectin SIGNR1 enhances cellular oxidative burst response against C. albicans in cooperation with Dectin-1. Eur J Immunol (2011) 41(5):1435–44. doi:10.1002/eji.200940188
168. Li X, Utomo A, Cullere X, Choi MM, Milner DA Jr, Venkatesh D, et al. The beta-glucan receptor Dectin-1 activates the integrin Mac-1 in neutrophils via Vav protein signaling to promote Candida albicans clearance. Cell Host Microbe (2011) 10(6):603–15. doi:10.1016/j.chom.2011.10.009
169. Le HT, Tran VG, Kim W, Kim J, Cho HR, Kwon B. IL-33 priming regulates multiple steps of the neutrophil-mediated anti-Candida albicans response by modulating TLR and Dectin-1 signals. J Immunol (2012) 189(1):287–95. doi:10.4049/jimmunol.1103564
170. Zawrotniak M, Bochenska O, Karkowska-Kuleta J, Seweryn-Ozog K, Aoki W, Ueda M, et al. Aspartic proteases and major cell wall components in Candida albicans trigger the release of neutrophil extracellular traps. Front Cell Infect Microbiol (2017) 7:414. doi:10.3389/fcimb.2017.00414
171. Byrd AS, O’Brien XM, Johnson CM, Lavigne LM, Reichner JS. An extracellular matrix-based mechanism of rapid neutrophil extracellular trap formation in response to Candida albicans. J Immunol (2013) 190(8):4136–48. doi:10.4049/jimmunol.1202671
172. Blanco-Menendez N, Del Fresno C, Fernandes S, Calvo E, Conde-Garrosa R, Kerr WG, et al. SHIP-1 Couples to the Dectin-1 hemITAM and selectively modulates reactive oxygen species production in dendritic cells in response to Candida albicans. J Immunol (2015) 195(9):4466–78. doi:10.4049/jimmunol.1402874
173. Rieber N, Singh A, Oz H, Carevic M, Bouzani M, Amich J, et al. Pathogenic fungi regulate immunity by inducing neutrophilic myeloid-derived suppressor cells. Cell Host Microbe (2015) 17(4):507–14. doi:10.1016/j.chom.2015.02.007
174. Drummond RA, Dambuza IM, Vautier S, Taylor JA, Reid DM, Bain CC, et al. CD4(+) T-cell survival in the GI tract requires Dectin-1 during fungal infection. Mucosal Immunol (2016) 9(2):492–502. doi:10.1038/mi.2015.79
175. Taylor PR, Tsoni SV, Willment JA, Dennehy KM, Rosas M, Findon H, et al. Dectin-1 is required for beta-glucan recognition and control of fungal infection. Nat Immunol (2007) 8(1):31–8. doi:10.1038/ni1408
176. Saijo S, Fujikado N, Furuta T, Chung SH, Kotaki H, Seki K, et al. Dectin-1 is required for host defense against Pneumocystis carinii but not against Candida albicans. Nat Immunol (2007) 8(1):39–46. doi:10.1038/ni1425
177. Marakalala MJ, Vautier S, Potrykus J, Walker LA, Shepardson KM, Hopke A, et al. Differential adaptation of Candida albicans in vivo modulates immune recognition by Dectin-1. PLoS Pathog (2013) 9(4):e1003315. doi:10.1371/journal.ppat.1003315
178. Chen SM, Shen H, Zhang T, Huang X, Liu XQ, Guo SY, et al. Dectin-1 plays an important role in host defense against systemic Candida glabrata infection. Virulence (2017) 8:1643–56. doi:10.1080/21505594.2017.1346756
179. Ferwerda B, Ferwerda G, Plantinga TS, Willment JA, van Spriel AB, Venselaar H, et al. Human Dectin-1 deficiency and mucocutaneous fungal infections. N Engl J Med (2009) 361(18):1760–7. doi:10.1056/NEJMoa0901053
180. Rosentul DC, Plantinga TS, Oosting M, Scott WK, Velez Edwards DR, Smith PB, et al. Genetic variation in the Dectin-1/CARD9 recognition pathway and susceptibility to candidemia. J Infect Dis (2011) 204(7):1138–45. doi:10.1093/infdis/jir458
181. Plantinga TS, van der Velden WJFM, Ferwerda B, van Spriel AB, Adema G, Feuth T, et al. Early stop polymorphism in human DECTIN-1 is associated with increased Candida colonization in hematopoietic stem cell transplant recipients. Clin Infect Dis (2009) 49(5):724–32. doi:10.1086/604714
182. Plantinga TS, Hamza OJM, Willment JA, Ferwerda B, van de Geer NMD, Verweij PE, et al. Genetic variation of innate immune genes in HIV-infected African patients with or without oropharyngeal candidiasis. J Acquir Immune Defic Syndr (2010) 55(1):87–94. doi:10.1097/QAI.0b013e3181e53c64
183. Robinson MJ, Osorio F, Rosas M, Freitas RP, Schweighoffer E, Gross O, et al. Dectin-2 is a Syk-coupled pattern recognition receptor crucial for Th17 responses to fungal infection. J Exp Med (2009) 206(9):2037–51. doi:10.1084/jem.20082818
184. Bi L, Gojestani S, Wu W, Hsu YM, Zhu J, Ariizumi K, et al. CARD9 mediates Dectin-2-induced IkappaBalpha kinase ubiquitination leading to activation of NF-kappaB in response to stimulation by the hyphal form of Candida albicans. J Biol Chem (2010) 285(34):25969–77. doi:10.1074/jbc.M110.131300
185. Ifrim DC, Bain JM, Reid DM, Oosting M, Verschueren I, Gow NAR, et al. Role of Dectin-2 for host defense against systemic infection with Candida glabrata. Infect Immun (2014) 82(3):1064–73. doi:10.1128/IAI.01189-13
186. Ifrim DC, Quintin J, Courjol F, Verschueren I, van Krieken JH, Koentgen F, et al. The role of Dectin-2 for host defense against disseminated candidiasis. J Interferon Cytokine Res (2016) 36(4):267–76. doi:10.1089/jir.2015.0040
187. Gorjestani S, Yu M, Tang B, Zhang DK, Wang DM, Lin X. Phospholipase C gamma 2 (PLC gamma 2) is key component in Dectin-2 signaling pathway, mediating anti-fungal innate immune responses. J Biol Chem (2011) 286(51):43651–9. doi:10.1074/jbc.M111.307389
188. Marodi L, Korchak HM, Johnston RB. Mechanisms of host defense against Candida species. I. Phagocytosis by monocytes and monocyte-derived macrophages. J Immunol (1991) 146(8):2783–9.
189. Yamamoto Y, Klein TW, Friedman H. Involvement of mannose receptor in cytokine interleukin-1beta (IL-1beta), IL-6, and granulocyte-macrophage colony-stimulating factor responses, but not in chemokine macrophage inflammatory protein 1beta (MIP-1beta), MIP-2, and KC responses, caused by attachment of Candida albicans to macrophages. Infect Immun (1997) 65(3):1077–82.
190. Newman SL, Holly A. Candida albicans is phagocytosed, killed, and processed for antigen presentation by human dendritic cells. Infect Immun (2001) 69(11):6813–22. doi:10.1128/IAI.69.11.6813-6822.2001
191. Romani L, Montagnoli C, Bozza S, Perruccio K, Spreca A, Allavena P, et al. The exploitation of distinct recognition receptors in dendritic cells determines the full range of host immune relationships with Candida albicans. Int Immunol (2004) 16(1):149–61. doi:10.1093/intimm/dxh012
192. Claudia M, Bacci A, Silvia B, Gaziano R, Spreca A, Romani L. The interaction of fungi with dendritic cells: implications for Th immunity and vaccination. Curr Mol Med (2002) 2(6):507–24. doi:10.2174/1566524023362203
193. Gazi U, Rosas M, Singh S, Heinsbroek S, Haq I, Johnson S, et al. Fungal recognition enhances mannose receptor shedding through Dectin-1 engagement. J Biol Chem (2011) 286(10):7822–9. doi:10.1074/jbc.M110.185025
194. Shepherd VL, Lane KB, Abdolrasulnia R. Ingestion of Candida albicans down-regulates mannose receptor expression on rat macrophages. Arch Biochem Biophys (1997) 344(2):350–6. doi:10.1006/abbi.1997.0219
195. Heinsbroek SE, Taylor PR, Martinez FO, Martinez-Pomares L, Brown GD, Gordon S. Stage-specific sampling by pattern recognition receptors during Candida albicans phagocytosis. PLoS Pathog (2008) 4(11):e1000218. doi:10.1371/journal.ppat.1000218
196. Lee SJ, Zheng NY, Clavijo M, Nussenzweig MC. Normal host defense during systemic candidiasis in mannose receptor-deficient mice. Infect Immun (2003) 71(1):437–45. doi:10.1128/IAI.71.1.437-445.2003
197. van de Veerdonk FL, Marijnissen RJ, Kullberg BJ, Koenen HJ, Cheng SC, Joosten I, et al. The macrophage mannose receptor induces IL-17 in response to Candida albicans. Cell Host Microbe (2009) 5(4):329–40. doi:10.1016/j.chom.2009.02.006
198. Smeekens SP, van de Veerdonk FL, Joosten LA, Jacobs L, Jansen T, Williams DL, et al. The classical CD14(+)(+) CD16(-) monocytes, but not the patrolling CD14(+) CD16(+) monocytes, promote Th17 responses to Candida albicans. Eur J Immunol (2011) 41(10):2915–24. doi:10.1002/eji.201141418
199. Castro M, Ralston NVC, Morgenthaler TI, Rohrbach MS, Limper AH. Candida albicans stimulates arachidonic-acid liberation from alveolar macrophages through alpha-mannan and beta-glucan cell-wall components. Infect Immun (1994) 62(8):3138–45.
200. Estrada-Mata E, Navarro-Arias MJ, Perez-Garcia LA, Mellado-Mojica E, Lopez MG, Csonka K, et al. Members of the Candida parapsilosis complex and Candida albicans are differentially recognized by human peripheral blood mononuclear cells. Front Microbiol (2016) 6:1527. doi:10.3389/fmicb.2015.01527
201. Donini M, Zenaro E, Tamassia N, Dusi S. NADPH oxidase of human dendritic cells: role in Candida albicans killing and regulation by interferons, Dectin-1 and CD206. Eur J Immunol (2007) 37(5):1194–203. doi:10.1002/eji.200636532
202. Marodi L, Schreiber S, Anderson DC, Macdermott RP, Korchak HM, Johnston RB. Enhancement of macrophage candidacidal activity by interferon-gamma – increased phagocytosis, killing, and calcium signal mediated by a decreased number of mannose receptors. J Clin Invest (1993) 91(6):2596–601. doi:10.1172/JCI116498
203. Pellis V, De Seta F, Crovella S, Bossi F, Bulla R, Guaschino S, et al. Mannose binding lectin and C3 act as recognition molecules for infectious agents in the vagina. Clin Exp Immunol (2005) 139(1):120–6. doi:10.1111/j.1365-2249.2005.02660.x
204. Ip WK, Lau YL. Role of mannose-binding lectin in the innate defense against Candida albicans: enhancement of complement activation, but lack of opsonic function, in phagocytosis by human dendritic cells. J Infect Dis (2004) 190:632–40. doi:10.1086/422397
205. Brouwer N, Dolman KM, van Houdt M, Sta M, Roos D, Kuijpers TW. Mannose-binding lectin (MBL) facilitates opsonophagocytosis of yeasts but not of bacteria despite MBL binding. J Immunol (2008) 180(6):4124–32. doi:10.4049/jimmunol.180.6.4124
206. van Asbeck EC, Hoepelman AI, Scharringa J, Herpers BL, Verhoef J. Mannose binding lectin plays a crucial role in innate immunity against yeast by enhanced complement activation and enhanced uptake of polymorphonuclear cells. BMC Microbiol (2008) 8:229. doi:10.1186/1471-2180-8-229
207. Li D, Dong B, Tong Z, Wang Q, Liu W, Wang Y, et al. MBL-mediated opsonophagocytosis of Candida albicans by human neutrophils is coupled with intracellular Dectin-1-triggered ROS production. PLoS One (2012) 7:e50589. doi:10.1371/journal.pone.0050589
208. Held K, Thiel S, Loos M, Petry F. Increased susceptibility of complement factor B/C2 double knockout mice and mannan-binding lectin knockout mice to systemic infection with Candida albicans. Mol Immunol (2008) 45(15):3934–41. doi:10.1016/j.molimm.2008.06.021
209. Choteau L, Parny M, Francois N, Bertin B, Fumery M, Dubuquoy L, et al. Role of mannose-binding lectin in intestinal homeostasis and fungal elimination. Mucosal Immunol (2016) 9(3):767–76. doi:10.1038/mi.2015.100
210. Wang M, Wang F, Yang J, Zhao D, Wang H, Shao F, et al. Mannan-binding lectin inhibits Candida albicans-induced cellular responses in PMA-activated THP-1 cells through toll-like receptor 2 and toll-like receptor 4. PLoS One (2013) 8:e83517. doi:10.1371/journal.pone.0083517
211. Liu C, He T, Rong Y, Du F, Ma D, Wei Y, et al. Association of mannose-binding lectin polymorphisms with tuberculosis susceptibility among Chinese. Sci Rep (2016) 6:36488. doi:10.1038/srep36488
212. Toivonen L, Vuononvirta J, Mertsola J, Waris M, He Q, Peltola V. Polymorphisms of mannose-binding lectin and toll-like receptors 2, 3, 4, 7 and 8 and the risk of respiratory infections and acute otitis media in children. Pediatr Infect Dis J (2017) 36(5):e114–22. doi:10.1097/INF.0000000000001479
213. Babula O, Lazdane G, Kroica J, Linhares IM, Ledger WJ, Witkin SS. Frequency of interleukin-4 (IL-4) -589 gene polymorphism and vaginal concentrations of IL-4, nitric oxide, and mannose-binding lectin in women with recurrent vulvovaginal candidiasis. Clin Infect Dis (2005) 40(9):1258–62. doi:10.1086/429246
214. Liu F, Liao Q, Liu Z. Mannose-binding lectin and vulvovaginal candidiasis. Int J Gynaecol Obstet (2006) 92(1):43–7. doi:10.1016/j.ijgo.2005.08.024
215. Babula O, Lazdane G, Kroica J, Ledger WJ, Witkin SS. Relation between recurrent vulvovaginal candidiasis, vaginal concentrations of mannose-binding lectin, and a mannose-binding lectin gene polymorphism in Latvian women. Clin Infect Dis (2003) 37(5):733–7. doi:10.1086/377234
216. van Till JW, Modderman PW, de Boer M, Hart MH, Beld MG, Boermeester MA. Mannose-binding lectin deficiency facilitates abdominal Candida infections in patients with secondary peritonitis. Clin Vaccine Immunol (2008) 15(1):65–70. doi:10.1128/CVI.00297-07
217. Osthoff M, Wojtowicz A, Tissot F, Jorgensen C, Thiel S, Zimmerli S, et al. Association of lectin pathway proteins with intra-abdominal Candida infection in high-risk surgical intensive-care unit patients. A prospective cohort study within the fungal infection network of Switzerland. J Infect (2016) 72(3):377–85. doi:10.1016/j.jinf.2015.12.011
218. Giraldo PC, Babula O, Goncalves AK, Linhares IM, Amaral RL, Ledger WJ, et al. Mannose-binding lectin gene polymorphism, vulvovaginal candidiasis, and bacterial vaginosis. Obstet Gynecol (2007) 109(5):1123–8. doi:10.1097/01.AOG.0000260386.17555.a5
219. Donders GG, Babula O, Bellen G, Linhares IM, Witkin SS. Mannose-binding lectin gene polymorphism and resistance to therapy in women with recurrent vulvovaginal candidiasis. BJOG (2008) 115(10):1225–31. doi:10.1111/j.1471-0528.2008.01830.x
220. Nedovic B, Posteraro B, Leoncini E, Ruggeri A, Amore R, Sanguinetti M, et al. Mannose-binding lectin codon 54 gene polymorphism and vulvovaginal candidiasis: a systematic review and meta-analysis. Biomed Res Int (2014) 2014:738298. doi:10.1155/2014/738298
221. Granell M, Urbano-Ispizua A, Suarez B, Rovira M, Fernandez-Aviles F, Martinez C, et al. Mannan-binding lectin pathway deficiencies and invasive fungal infections following allogeneic stem cell transplantation. Exp Hematol (2006) 34(10):1435–41. doi:10.1016/j.exphem.2006.06.005
222. de Mare-Bredemeijer EL, Mancham S, Utomo WK, de Canck I, van Thielen M, de Meester E, et al. Genetic polymorphisms in innate immunity receptors do not predict the risk of bacterial and fungal infections and acute rejection after liver transplantation. Transpl Infect Dis (2013) 15(2):120–33. doi:10.1111/tid.12034
223. Beltrame MH, Boldt AB, Catarino SJ, Mendes HC, Boschmann SE, Goeldner I, et al. MBL-associated serine proteases (MASPs) and infectious diseases. Mol Immunol (2015) 67(1):85–100. doi:10.1016/j.molimm.2015.03.245
224. Klassert TE, Goyal S, Stock M, Driesch D, Hussain A, Berrocal-Almanza LC, et al. AmpliSeq screening of genes encoding the C-type lectin receptors and their signaling components reveals a common variant in MASP1 associated with pulmonary tuberculosis in an Indian population. Front Immunol (2018) 9:242. doi:10.3389/fimmu.2018.00242
225. te Riet J, Reinieren-Beeren I, Figdor CG, Cambi A. AFM force spectroscopy reveals how subtle structural differences affect the interaction strength between Candida albicans and DC-SIGN. J Mol Recognit (2015) 28(11):687–98. doi:10.1002/jmr.2481
226. Netea MG, Gijzen K, Coolen N, Verschueren I, Figdor C, Van der Meer JWM, et al. Human dendritic cells are less potent at killing Candida albicans than both monocytes and macrophages. Microbes Infect (2004) 6(11):985–9. doi:10.1016/j.micinf.2004.05.013
227. Mittal R, Bulgheresi S, Emami C, Prasadarao NV. Enterobacter sakazakii targets DC-SIGN to induce immunosuppressive responses in dendritic cells by modulating MAPKs. J Immunol (2009) 183(10):6588–99. doi:10.4049/jimmunol.0902029
228. Gringhuis SI, den Dunnen J, Litjens M, van Het Hof B, van Kooyk Y, Geijtenbeek TB. C-type lectin DC-SIGN modulates toll-like receptor signaling via Raf-1 kinase-dependent acetylation of transcription factor NF-kappaB. Immunity (2007) 26(5):605–16. doi:10.1016/j.immuni.2007.03.012
229. Takahara K, Tokieda S, Nagaoka K, Inaba K. Efficient capture of Candida albicans and zymosan by SIGNR1 augments TLR2-dependent TNF-alpha production. Int Immunol (2012) 24(2):89–96. doi:10.1093/intimm/dxr103
230. Bugarcic A, Hitchens K, Beckhouse AG, Wells CA, Ashman RB, Blanchard H. Human and mouse macrophage-inducible C-type lectin (Mincle) bind Candida albicans. Glycobiology (2008) 18(9):679–85. doi:10.1093/glycob/cwn046
231. Vijayan D, Radford KJ, Beckhouse AG, Ashman RB, Wells CA. Mincle polarizes human monocyte and neutrophil responses to Candida albicans. Immunol Cell Biol (2012) 90(9):889–95. doi:10.1038/icb.2012.24
232. Szabo I, Guan LM, Rogers TJ. Modulation of macrophage phagocytic-activity by cell-wall components of Candida albicans. Cell Immunol (1995) 164(2):182–8. doi:10.1006/cimm.1995.1160
233. Forsyth CB, Mathews HL. Lymphocyte adhesion to Candida albicans. Infect Immun (2002) 70(2):517–27. doi:10.1128/IAI.70.2.517-527.2002
234. Forsyth CB, Mathews HL. Lymphocytes utilize CD11b/CD18 for adhesion to Candida albicans. Cell Immunol (1996) 170(1):91–100. doi:10.1006/cimm.1996.0138
235. Losse J, Svobodova E, Heyken A, Hube B, Zipfel PF, Jozsi M. Role of pH-regulated antigen 1 of Candida albicans in the fungal recognition and antifungal response of human neutrophils. Mol Immunol (2011) 48(15–16):2135–43. doi:10.1016/j.molimm.2011.07.007
236. Choi W, Yoo YJ, Kim M, Shin D, Jeon HB, Choi W. Identification of proteins highly expressed in the hyphae of Candida albicans by two-dimensional electrophoresis. Yeast (2003) 20(12):1053–60. doi:10.1002/yea.1022
237. Soloviev DA, Jawhara S, Fonzi WA. Regulation of innate immune response to Candida albicans infections by alpha(M)beta(2)-Pra1p interaction. Infect Immun (2011) 79(4):1546–58. doi:10.1128/IAI.00650-10
238. Thornton BP, Vetvicka V, Pitman M, Goldman RC, Ross GD. Analysis of the sugar specificity and molecular location of the beta-glucan-binding lectin site of complement receptor type 3 (CD11b/CD18). J Immunol (1996) 156(3):1235–46.
239. Le Cabec V, Carreno S, Moisand A, Bordier C, Maridonneau-Parini I. Complement receptor 3 (CD11b/CD18) mediates type I and type II phagocytosis during nonopsonic and opsonic phagocytosis, respectively. J Immunol (2002) 169(4):2003–9. doi:10.4049/jimmunol.169.4.2003
240. Lavigne LM, Albina JE, Reichner JS. Beta-glucan is a fungal determinant for adhesion-dependent human neutrophil functions. J Immunol (2006) 177(12):8667–75. doi:10.4049/jimmunol.177.12.8667
241. Tateno H, Ohnishi K, Yabe R, Hayatsu N, Sato T, Takeya M, et al. Dual specificity of Langerin to sulfated and mannosylated glycans via a single C-type carbohydrate recognition domain. J Biol Chem (2010) 285(9):6390–400. doi:10.1074/jbc.M109.041863
242. De Jesus M, Rodriguez AE, Yagita H, Ostroff GR, Mantis NJ. Sampling of Candida albicans and Candida tropicalis by langerin-positive dendritic cells in mouse Peyer’s patches. Immunol Lett (2015) 168(1):64–72. doi:10.1016/j.imlet.2015.09.008
243. Igyarto BZ, Haley K, Ortner D, Bobr A, Gerami-Nejad M, Edelson BT, et al. Skin-resident murine dendritic cell subsets promote distinct and opposing antigen-specific T helper cell responses. Immunity (2011) 35(2):260–72. doi:10.1016/j.immuni.2011.06.005
244. Haley K, Igyarto BZ, Ortner D, Bohr A, Kashem S, Schenten D, et al. Langerhans cells require MyD88-dependent signals for Candida albicans response but not for contact hypersensitivity or migration. J Immunol (2012) 188(9):4334–9. doi:10.4049/jimmunol.1102759
245. Illnait-Zaragozi MT, Martínez-Machín GF, Fernández-Andreu CM, Perurena-Lancha MR, Hagen F, Meis JF. Cryptococcus and cryptococcosis in Cuba. A minireview. Mycoses (2014) 57:707–17. doi:10.1111/myc.12275
246. May RC, Stone NRH, Wiesner DL, Bicanic T, Nielsen K. Cryptococcus: from environmental saprophyte to global pathogen. Nat Rev Microbiol (2015) 14:106–17. doi:10.1038/nrmicro.2015.6
247. Cross CE, Bancroft GJ. Ingestion of acapsular Cryptococcus neoformans occurs via mannose and beta-glucan receptors, resulting in cytokine production and increased phagocytosis of the encapsulated form. Infect Immun (1995) 63:2604–11.
248. Nakamura K, Kinjo T, Saijo S, Miyazato A, Adachi Y, Ohno N, et al. Dectin-1 is not required for the host defense to Cryptococcus neoformans. Microbiol Immunol (2007) 51:1115–9. doi:10.1111/j.1348-0421.2007.tb04007.x
249. Nakamura Y, Sato K, Yamamoto H, Matsumura K, Matsumoto I, Nomura T, et al. Dectin-2 deficiency promotes Th2 response and mucin production in the lungs after pulmonary infection with Cryptococcus neoformans. Infect Immun (2015) 83:671–81. doi:10.1128/IAI.02835-14
250. Dan JM, Kelly RM, Lee CK, Levitz SM. Role of the mannose receptor in a murine model of Cryptococcus neoformans infection. Infect Immun (2008) 76:2362–7. doi:10.1128/IAI.00095-08
251. Syme RM, Spurrell JCL, Amankwah EK, Green FHY, Mody CH. Primary dendritic cells phagocytose Cryptococcus neoformans via mannose receptors and Fcgamma receptor II for presentation to T lymphocytes. Infect Immun (2002) 70:5972–81. doi:10.1128/IAI.70.11.5972-5981.2002
252. Walenkamp AM, Verheul AF, Scharringa J, Hoepelman IM. Pulmonary surfactant protein A binds to Cryptococcus neoformans without promoting phagocytosis. Eur J Clin Invest (1999) 29:83–92. doi:10.1046/j.1365-2362.1999.00429.x
253. Geunes-Boyer S, Beers MF, Perfect JR, Heitman J, Wright JR. Surfactant protein D facilitates Cryptococcus neoformans infection. Infect Immun (2012) 80:2444–53. doi:10.1128/IAI.05613-11
254. Giles SS, Zaas AK, Reidy MF, Perfect JR, Wright JR. Cryptococcus neoformans is resistant to surfactant protein A mediated host defense mechanisms. PLoS One (2007) 2:e1370. doi:10.1371/journal.pone.0001370
255. Holmer SM, Evans KS, Asfaw YG, Saini D, Schell WA, Ledford JG, et al. Impact of surfactant protein D, interleukin-5, and eosinophilia on cryptococcosis. Infect Immun (2014) 82:683–93. doi:10.1128/IAI.00855-13
256. Geunes-Boyer S, Oliver TN, Janbon G, Lodge JK, Heitman J, Perfect JR, et al. Surfactant protein D increases phagocytosis of hypocapsular Cryptococcus neoformans by murine macrophages and enhances fungal survival. Infect Immun (2009) 77:2783–94. doi:10.1128/IAI.00088-09
257. Panepinto JC, Komperda KW, Hacham M, Shin S, Liu X, Williamson PR. Binding of serum mannan binding lectin to a cell integrity-defective Cryptococcus neoformans ccr4 mutant. Infect Immun (2007) 75:4769–79. doi:10.1128/IAI.00536-07
258. Ibrahim AS, Spellberg B, Walsh TJ, Kontoyiannis DP. Pathogenesis of mucormycosis. Clin Infect Dis (2012) 54:S16–22. doi:10.1093/cid/cir865
259. Katragkou A, Walsh TJ, Roilides E. Why is mucormycosis more difficult to cure than more common mycoses? Clin Microbiol Infect (2014) 20:74–81. doi:10.1111/1469-0691.12466
260. Mendoza L, Vilela R, Voelz K, Ibrahim AS, Voigt K, Lee SC. Human fungal pathogens of mucorales and entomophthorales. Cold Spring Harb Perspect Med (2015) 5(4). doi:10.1101/cshperspect.a019562
261. Gigliotti F, Limper AH, Wright T. Pneumocystis. Cold Spring Harb Perspect Med (2014) 4:a019828. doi:10.1101/cshperspect.a019828
262. Vassallo R, Standing JE, Limper AH. Isolated Pneumocystis carinii cell wall glucan provokes lower respiratory tract inflammatory responses. J Immunol (2000) 164:3755–63. doi:10.4049/jimmunol.164.7.3755
263. Hoffman OA, Standing JE, Limper AH. Pneumocystis carinii stimulates tumor necrosis factor-alpha release from alveolar macrophages through a beta-glucan-mediated mechanism. J Immunol (1993) 150:3932–40.
264. Nandakumar V, Hebrink D, Jenson P, Kottom T, Limper AH. Differential macrophage polarization from Pneumocystis in immunocompetent and immunosuppressed hosts: potential adjunctive therapy during pneumonia. Infect Immun (2017) 85:e939–916. doi:10.1128/IAI.00939-16
265. Zhang C, Wang S-H, Liao C-P, Shao S, Lasbury ME, Durant PJ, et al. Downregulation of PU.1 leads to decreased expression of Dectin-1 in alveolar macrophages during Pneumocystis pneumonia. Infect Immun (2010) 78:1058–65. doi:10.1128/IAI.01141-09
266. Steele C, Marrero L, Swain S, Harmsen AG, Zheng M, Brown GD, et al. Alveolar macrophage-mediated killing of Pneumocystis carinii f. sp. muris involves molecular recognition by the Dectin-1 β-glucan receptor. J Exp Med (2003) 198:1677–88. doi:10.1084/jem.20030932
267. Evans SE, Hahn PY, McCann F, Kottom TJ, Pavlovic’ ZV, Limper AH. Pneumocystis cell wall β-glucans stimulate alveolar epithelial cell chemokine generation through nuclear factor-κB-dependent mechanisms. Am J Respir Cell Mol Biol (2005) 32:490–7. doi:10.1165/rcmb.2004-0300OC
268. Hahn PY, Evans SE, Kottom TJ, Standing JE, Pagano RE, Limper AH. Pneumocystis carinii cell wall β-glucan induces release of macrophage inflammatory protein-2 from alveolar epithelial cells via a lactosylceramide-mediated mechanism. J Biol Chem (2003) 278:2043–50. doi:10.1074/jbc.M209715200
269. Fraser IP, Takahashi K, Koziel H, Fardin B, Harmsen A, Ezekowitz RAB. Pneumocystis carinii enhances soluble mannose receptor production by macrophages. Microbes Infect (2000) 2:1305–10. doi:10.1016/S1286-4579(00)01283-1
270. Zhang J, Zhu J, Bu X, Cushion M, Kinane TB, Avraham H, et al. Cdc42 and RhoB activation are required for mannose receptor-mediated phagocytosis by human alveolar macrophages. Mol Biol Cell (2005) 16:824–34. doi:10.1091/mbc.e04-06-0463
271. Stehle SE, Rogers RA, Harmsen AG, Ezekowitz RA. A soluble mannose receptor immunoadhesin enhances phagocytosis of Pneumocystis carinii by human polymorphonuclear leukocytes in vitro. Scand J Immunol (2000) 52:131–7. doi:10.1046/j.1365-3083.2000.00755.x
272. Ezekowitz RAB, Williams DJ, Koziel H, Armstrong MYK, Warner A, Richards FF, et al. Uptake of Pneumocystis carinii mediated by the macrophage mannose receptor. Nature (1991) 351:155–8. doi:10.1038/351155a0
273. Koziel H, Eichbaum Q, Kruskal BA, Pinkston P, Rogers RA, Armstrong MY, et al. Reduced binding and phagocytosis of Pneumocystis carinii by alveolar macrophages from persons infected with HIV-1 correlates with mannose receptor downregulation. J Clin Invest (1998) 102:1332–44. doi:10.1172/JCI560
274. Tachado SD, Zhang J, Zhu J, Patel N, Cushion M, Koziel H. Pneumocystis-mediated IL-8 release by macrophages requires coexpression of mannose receptors and TLR2. J Leukoc Biol (2006) 81:205–11. doi:10.1189/jlb.1005580
275. Zhang J, Tachado SD, Patel N, Zhu J, Imrich A, Manfruelli P, et al. Negative regulatory role of mannose receptors on human alveolar macrophage proinflammatory cytokine release in vitro. J Leukoc Biol (2005) 78:665–74. doi:10.1189/jlb.1204699
276. Linke M, Ashbaugh A, Koch J, Tanaka R, Walzer P. Surfactant protein A limits Pneumocystis murina infection in immunosuppressed C3H/HeN mice and modulates host response during infection. Microbes Infect (2005) 7:748–59. doi:10.1016/j.micinf.2005.01.011
277. Atochina EN, Beck JM, Preston AM, Haczku A, Tomer Y, Scanlon ST, et al. Enhanced lung injury and delayed clearance of Pneumocystis carinii in surfactant protein A-deficient mice: attenuation of cytokine responses and reactive oxygen-nitrogen species. Infect Immun (2004) 72:6002–11. doi:10.1128/IAI.72.10.6002-6011.2004
278. Atochina Elena N, Gow Andrew J, Beck James M, Haczku A, Inch A, Kadire H, et al. Delayed clearance of Pneumocystis carinii infection, increased inflammation, and altered nitric oxide metabolism in lungs of surfactant protein-D knockout mice. J Infect Dis (2004) 189:1528–39. doi:10.1086/383130
279. Linke MJ, Ashbaugh AD, Demland JA, Walzer PD. Pneumocystis murina colonization in immunocompetent surfactant protein A deficient mice following environmental exposure. Respir Res (2009) 10:10. doi:10.1186/1465-9921-10-10
280. Linke M, Ashbaugh A, Koch J, Tanaka R, Walzer P. Efficient resolution of Pneumocystis murina infection in surfactant protein A-deficient mice following withdrawal of corticosteroid-induced immunosuppression. J Med Microbiol (2006) 55:143–7. doi:10.1099/jmm.0.46190-0
281. Linke Michael J, Harris Christopher E, Korfhagen Thomas R, McCormack Francis X, Ashbaugh Alan D, Steele P, et al. Immunosuppressed surfactant protein A-deficient mice have increased susceptibility to Pneumocystis carinii infection. J Infect Dis (2001) 183:943–52. doi:10.1086/319252
282. Zhu S, Kachel DL, Martin WJ, Matalon S. Nitrated SP-A does not enhance adherence of Pneumocystis carinii to alveolar macrophages. Am J Physiol (1998) 275:L1031–9.
283. Williams MD, Wright JR, March KL, Martin WJ. Human surfactant protein A enhances attachment of Pneumocystis carinii to rat alveolar macrophages. Am J Respir Cell Mol Biol (1996) 14:232–8. doi:10.1165/ajrcmb.14.3.8845173
284. Limper AH, Crouch EC, O’Riordan DM, Chang D, Vuk-Pavlovic Z, Standing JE, et al. Surfactant protein-D modulates interaction of Pneumocystis carinii with alveolar macrophages. J Lab Clin Med (1995) 126:416–22.
285. Koziel H, Phelps DS, Fishman JA, Armstrong MYK, Richards FF, Rose RM. Surfactant protein-A reduces binding and phagocytosis of Pneumocystis carinii by human alveolar macrophages in vitro. Am J Respir Cell Mol Biol (1998) 18:834–43. doi:10.1165/ajrcmb.18.6.3059
286. Yong S-J, Vuk-Pavlovic Z, Standing JE, Crouch EC, Limper AH. Surfactant protein D-mediated aggregation of Pneumocystis carinii impairs phagocytosis by alveolar macrophages. Infect Immun (2003) 71:1662–71. doi:10.1128/IAI.71.4.1662-1671.2003
287. Vuk-Pavlovic Z, Mo EK, Icenhour CR, Standing JE, Fisher JH, Limper AH. Surfactant protein D enhances Pneumocystis infection in immune-suppressed mice. Am J Physiol Lung Cell Mol Physiol (2005) 290:L442–9. doi:10.1152/ajplung.00112.2005
288. Linke MJ, Ashbaugh AA, Koch JV, Levin L, Tanaka R, Walzer PD. Effects of surfactant protein-A on the interaction of Pneumocystis murina with its host at different stages of the infection in mice. J Eukaryot Microbiol (2009) 56:58–65. doi:10.1111/j.1550-7408.2008.00363.x
289. O’Riordan DM, Standing JE, Kwon KY, Chang D, Crouch EC, Limper AH. Surfactant protein D interacts with Pneumocystis carinii and mediates organism adherence to alveolar macrophages. J Clin Invest (1995) 95:2699–710. doi:10.1172/JCI117972
290. Zimmerman PE, Voelker DR, McCormack FX, Paulsrud JR, Martin WJ. 120-kD surface glycoprotein of Pneumocystis carinii is a ligand for surfactant protein A. J Clin Invest (1992) 89:143–9. doi:10.1172/JCI115554
291. Yanagisawa K, Ogawa Y, Uchiumi H, Gohda F, Mawatari M, Ishizaki T, et al. Gene polymorphisms of mannose-binding lectin confer susceptibility to Pneumocystis pneumonia in HIV-infected patients. J Infect Chemother (2015) 21:769–75. doi:10.1016/j.jiac.2015.07.006
292. Schildgen V, Mai S, Khalfaoui S, Lüsebrink J, Pieper M, Tillmann RL, et al. Pneumocystis jirovecii can be productively cultured in differentiated CuFi-8 airway cells. MBio (2014) 5:e1186–14. doi:10.1128/mBio.01186-14
293. Skalski JH, Kottom TJ, Limper AH. Pathobiology of Pneumocystis pneumonia: life cycle, cell wall and cell signal transduction. FEMS Yeast Res (2015) 15:fov046. doi:10.1093/femsyr/fov046
294. Powers-Fletcher MV, Kendall BA, Griffin AT, Hanson KE. Filamentous Fungi. Microbiol Spectr (2016) 4(3). doi:10.1128/microbiolspec.DMIH2-0002-2015
295. del Pilar Jiménez AM, Viriyakosol S, Walls L, Datta SK, Kirkland T, Heinsbroek SEM, et al. Susceptibility to Coccidioides species in C57BL/6 mice is associated with expression of a truncated splice variant of Dectin-1 (Clec7a). Genes Immun (2008) 9:338–48. doi:10.1038/gene.2008.23
296. Viriyakosol S, Jimenez MDP, Gurney MA, Ashbaugh ME, Fierer J. Dectin-1 is required for resistance to coccidioidomycosis in mice. MBio (2013) 4:e597–12. doi:10.1128/mBio.00597-12
297. Ampel NM, Dionne SO, Giblin A, Podany AB, Galgiani J. Mannose-binding lectin serum levels are low in persons with clinically active coccidioidomycosis. Mycopathologia (2009) 167:173–80. doi:10.1007/s11046-008-9172-6
298. Viriyakosol S, Jimenez MDP, Saijo S, Fierer J. Neither Dectin-2 nor the mannose receptor is required for resistance to Coccidioides immitis in mice. Infect Immun (2014) 82:1147–56. doi:10.1128/IAI.01355-13
299. Garfoot AL, Rappleye CA. Histoplasma capsulatum surmounts obstacles to intracellular pathogenesis. FEBS J (2016) 283:619–33. doi:10.1111/febs.13389
300. Nanjappa SG, Heninger E, Wüthrich M, Gasper DJ, Klein BS. Tc17 cells mediate vaccine immunity against lethal fungal pneumonia in immune deficient hosts lacking CD4+ T cells. PLoS Pathog (2012) 8:e1002771. doi:10.1371/journal.ppat.1002771
301. Garfoot AL, Shen Q, Wüthrich M, Klein BS, Rappleye CA. The Eng1 β-glucanase enhances Histoplasma virulence by reducing β-glucan exposure. MBio (2016) 7:e1388–1315. doi:10.1128/mBio.01388-15
302. Rappleye CA, Eissenberg LG, Goldman WE. Histoplasma capsulatum alpha-(1,3)-glucan blocks innate immune recognition by the beta-glucan receptor. Proc Natl Acad Sci U S A (2007) 104:1366–70. doi:10.1073/pnas.0609848104
303. Gonzalez A, Hernandez O. New insights into a complex fungal pathogen: the case of Paracoccidioides spp. Yeast (2016) 33:113–28. doi:10.1002/yea.3147
304. Rodrigues DR, Fernandes RK, Balderramas HDA, Penitenti M, Bachiega TF, Calvi SA, et al. Interferon-gamma production by human neutrophils upon stimulation by IL-12, IL-15 and IL-18 and challenge with Paracoccidioides brasiliensis. Cytokine (2014) 69:102–9. doi:10.1016/j.cyto.2014.05.009
305. Balderramas HA, Penitenti M, Rodrigues DR, Bachiega TF, Fernandes RK, Ikoma MRVR, et al. Human neutrophils produce IL-12, IL-10, PGE2 and LTB4 in response to Paracoccidioides brasiliensis. Involvement of TLR2, mannose receptor and Dectin-1. Cytokine (2014) 67:36–43. doi:10.1016/j.cyto.2014.02.004
306. Bachiega TF, Dias-Melicio LA, Fernandes RK, de Almeida Balderramas H, Rodrigues DR, Ximenes VF, et al. Participation of Dectin-1 receptor on NETs release against Paracoccidioides brasiliensis: role on extracellular killing. Immunobiology (2016) 221:228–35. doi:10.1016/j.imbio.2015.09.003
307. Loures FVV, Araújo EF, Feriotti C, Bazan SB, Costa TNA, Brown GD, et al. Dectin-1 induces M1 macrophages and prominent expansion of CD8+IL-17+ cells in pulmonary paracoccidioidomycosis. J Infect Dis (2014) 210:762–73. doi:10.1093/infdis/jiu136
308. Feriotti C, Loures FV, Frank de Araújo E, Costa TAD, Calich VLG. Mannosyl-recognizing receptors induce an M1-like phenotype in macrophages of susceptible mice but an M2-like phenotype in mice resistant to a fungal infection. PLoS One (2013) 8:e54845. doi:10.1371/journal.pone.0054845
309. Toledo RG, Da Silva WD, Calich VLG, Kipnis TL. Mannose-binding lectin complement pathway plays a key role in complement activation by Paracoccidioides brasiliensis. Mol Immunol (2010) 48:26–36. doi:10.1016/j.molimm.2010.09.015
310. Chan JFW, Lau SKP, Yuen KY, Woo PCY. Talaromyces (Penicillium) marneffei infection in non-HIV-infected patients. Emerg Microbes Infect (2016) 5:e19. doi:10.1038/emi.2016.18
311. Vanittanakom N, Cooper CR Jr, Fisher MC, Sirisanthana T. Penicillium marneffei infection and recent advances in the epidemiology and molecular biology aspects. Clin Microbiol Rev (2006) 19(1):95–110. doi:10.1128/CMR.19.1.95-110.2006
312. Zhan P, Liu W. The changing face of dermatophytic infections worldwide. Mycopathologia (2017) 182(1–2):77–86. doi:10.1007/s11046-016-0082-8
313. Yoshikawa FS, Yabe R, Iwakura Y, de Almeida SR, Saijo S. Dectin-1 and Dectin-2 promote control of the fungal pathogen Trichophyton rubrum independently of IL-17 and adaptive immunity in experimental deep dermatophytosis. Innate Immun (2016) 22:316–24. doi:10.1177/1753425916645392
314. Nakamura T, Nishibu A, Yasoshima M, Tanoue C, Yoshida N, Hatta J, et al. Analysis of Trichophyton antigen-induced contact hypersensitivity in mouse. J Dermatol Sci (2012) 66:144–53. doi:10.1016/j.jdermsci.2012.02.008
315. Velegraki A, Cafarchia C, Gaitanis G, Iatta R, Boekhout T, Otranto D. Malassezia infections in humans and animals: pathophysiology, detection, and treatment. PLoS Pathog (2015) 11:e1004523. doi:10.1371/journal.ppat.1004523
316. Tragiannidis A, Bisping G, Koehler G, Groll AH. Minireview: Malassezia infections in immunocompromised patients. Mycoses (2010) 53:187–95. doi:10.1111/j.1439-0507.2009.01814.x
317. Yamasaki S, Matsumoto M, Takeuchi O, Matsuzawa T, Ishikawa E, Sakuma M, et al. C-type lectin Mincle is an activating receptor for pathogenic fungus, Malassezia. Proc Natl Acad Sci U S A (2009) 106:1897–902. doi:10.1073/pnas.0805177106
318. Van Der Vlist M, Geijtenbeek TB. Langerin functions as an antiviral receptor on Langerhans cells. Immunol Cell Biol (2010) 88(4):410–5. doi:10.1038/icb.2010.32
319. Santos ALS, Palmeira VF, Rozental S, Kneipp LF, Nimrichter L, Alviano DS, et al. Biology and pathogenesis of Fonsecaea pedrosoi, the major etiologic agent of chromoblastomycosis. FEMS Microbiol Rev (2007) 31:570–91. doi:10.1111/j.1574-6976.2007.00077.x
320. Wevers BA, Kaptein TM, Zijlstra-Willems EM, Theelen B, Boekhout T, Geijtenbeek TBH, et al. Fungal engagement of the C-type lectin Mincle suppresses Dectin-1-induced antifungal immunity. Cell Host Microbe (2014) 15:494–505. doi:10.1016/j.chom.2014.03.008
321. Skerlev M, Miklic P. The changing face of Microsporum spp. infections. Clin Dermatol (2010) 28(2):146–50. doi:10.1016/j.clindermatol.2009.12.007
322. Zahur M, Afroz A, Rashid U, Khaliq S. Dermatomycoses: challenges and human immune responses. Curr Protein Pept Sci (2014) 15(5):437–44. doi:10.2174/1389203715666140512121349
323. Mao LM, Zhang LP, Li H, Chen W, Wang HB, Wu SX, et al. Pathogenic fungus Microsporum canis activates the NLRP3 inflammasome. Infect Immun (2014) 82(2):882–92. doi:10.1128/IAI.01097-13
324. Nucci M, Anaissie E. Fusarium infections in immunocompromised patients. Clin Microbiol Rev (2007) 20(4):695–704. doi:10.1128/CMR.00014-07
325. Karthikeyan RS, Leal SM Jr, Prajna NV, Dharmalingam K, Geiser DM, Pearlman E, et al. Expression of innate and adaptive immune mediators in human corneal tissue infected with Aspergillus or Fusarium. J Infect Dis (2011) 204(6):942–50. doi:10.1093/infdis/jir426
326. Kolar SS, Baidouri H, McDermott AM. Role of pattern recognition receptors in the modulation of antimicrobial peptide expression in the corneal epithelial innate response to F. solani. Invest Ophthalmol Vis Sci (2017) 58(5):2463–72. doi:10.1167/iovs.16-20658
327. Che CY, Li XJ, Jia WY, Li N, Xu Q, Lin J, et al. Early expression of surfactant proteins D in Fusarium solani infected rat cornea. Int J Ophthalmol (2012) 5(3):297–300. doi:10.3980/j.issn.2222-3959.2012.03.09
328. Colombo AL, Padovan ACB, Chaves GM. Current knowledge of Trichosporon spp. and trichosporonosis. Clin Microbiol Rev (2011) 24(4):682–700. doi:10.1128/CMR.00003-11
329. Almeida JND, Hennequin C. Invasive Trichosporon infection: a systematic review on a re-emerging fungal pathogen. Front Microbiol (2016) 7:1629. doi:10.3389/fmicb.2016.01629
330. Duarte-Oliveira C, Rodrigues F, Goncalves SM, Goldman GH, Carvalho A, Cunha C. The cell biology of the Trichosporon-host interaction. Front Cell Infect Microbiol (2017) 7:118. doi:10.3389/fcimb.2017.00118
331. Pérez-Torrado R, Querol A. Opportunistic strains of Saccharomyces cerevisiae: a potential risk sold in food products. Front Microbiol (2015) 6:1522. doi:10.3389/fmicb.2015.01522
332. Anoop V, Rotaru S, Shwed PS, Tayabali AF, Arvanitakis G. Review of current methods for characterizing virulence and pathogenicity potential of industrial Saccharomyces cerevisiae strains towards humans. FEMS Yeast Res (2015) 15:fov057. doi:10.1093/femsyr/fov057
333. Aucott JN, Fayen J, Grossnicklas H, Morrissey A, Lederman MM, Salata RA. Invasive infection with Saccharomyces cerevisiae: report of three cases and review. Rev Infect Dis (1990) 12:406–11. doi:10.1093/clinids/12.3.406
334. Rizzetto L, Buschow SI, Beltrame L, Figdor CG, Schierer S, Schuler G, et al. The modular nature of dendritic cell responses to commensal and pathogenic fungi. PLoS One (2012) 7:e42430. doi:10.1371/journal.pone.0042430
335. Aimanianda V, Clavaud C, Simenel C, Fontaine T, Delepierre M, Latgé J-P. Cell wall beta-(1,6)-glucan of Saccharomyces cerevisiae: structural characterization and in situ synthesis. J Biol Chem (2009) 284:13401–12. doi:10.1074/jbc.M807667200
336. Huang H, Ostroff GR, Lee CK, Wang JP, Specht CA, Levitz SM. Distinct patterns of dendritic cell cytokine release stimulated by fungal beta-glucans and toll-like receptor agonists. Infect Immun (2009) 77:1774–81. doi:10.1128/IAI.00086-09
337. Roy S, Dickerson R, Khanna S, Collard E, Gnyawali U, Gordillo GM, et al. Particulate β-glucan induces TNF-α production in wound macrophages via a redox-sensitive NF-κβ-dependent pathway. Wound Repair Regen (2011) 19:411–9. doi:10.1111/j.1524-475X.2011.00688.x
338. Yang Z, Marshall JS. Zymosan treatment of mouse mast cells enhances Dectin-1 expression and induces Dectin-1-dependent reactive oxygen species (ROS) generation. Immunobiology (2009) 214:321–30. doi:10.1016/j.imbio.2008.09.002
339. Karumuthil-Melethil S, Gudi R, Johnson BM, Perez N, Vasu C. Fungal β-glucan, a Dectin-1 ligand, promotes protection from type 1 diabetes by inducing regulatory innate immune response. J Immunol (2014) 193:3308–21. doi:10.4049/jimmunol.1400186
340. Qi C, Cai Y, Gunn L, Ding C, Li B, Kloecker G, et al. Differential pathways regulating innate and adaptive antitumor immune responses by particulate and soluble yeast-derived β-glucans. Blood (2011) 117:6825–36. doi:10.1182/blood-2011-02-339812
341. Saegusa S, Totsuka M, Kaminogawa S, Hosoi T. Saccharomyces cerevisiae and Candida albicans stimulate cytokine secretion from human neutrophil-like HL-60 cells differentiated with retinoic acid or dimethylsulfoxide. Biosci Biotechnol Biochem (2009) 73:2600–8. doi:10.1271/bbb.90410
342. Tam JM, Mansour MK, Khan NS, Yoder NC, Vyas JM. Use of fungal derived polysaccharide-conjugated particles to probe Dectin-1 responses in innate immunity. Integr Biol (Camb) (2012) 4:220–7. doi:10.1039/c2ib00089j
343. McCann F, Carmona E, Puri V, Pagano RE, Limper AH. Macrophage internalization of fungal beta-glucans is not necessary for initiation of related inflammatory responses. Infect Immun (2005) 73:6340–9. doi:10.1128/IAI.73.10.6340-6349.2005
344. Goodridge HS, Reyes CN, Becker CA, Katsumoto TR, Ma J, Wolf AJ, et al. Activation of the innate immune receptor Dectin-1 upon formation of a ‘phagocytic synapse’. Nature (2011) 472:471–5. doi:10.1038/nature10071
345. Dillon S, Agrawal S, Banerjee K, Letterio J, Denning TL, Oswald-Richter K, et al. Yeast zymosan, a stimulus for TLR2 and Dectin-1, induces regulatory antigen-presenting cells and immunological tolerance. J Clin Invest (2006) 116(4):916–28. doi:10.1172/JCI27203
346. Elcombe SE, Naqvi S, Van Den Bosch MW, MacKenzie KF, Cianfanelli F, Brown GD, et al. Dectin-1 regulates IL-10 production via a MSK1/2 and CREB dependent pathway and promotes the induction of regulatory macrophage markers. PLoS One (2013) 8(3):e60086. doi:10.1371/journal.pone.0060086
347. Schoepfer AM, Flogerzi B, Seibold-Schmid B, Schaffer T, Kun JFJ, Pittet V, et al. Low mannan-binding lectin serum levels are associated with complicated Crohn’s disease and reactivity to oligomannan (ASCA). Am J Gastroenterol (2009) 104:2508–16. doi:10.1038/ajg.2009.315
348. Seibold F, Boldt ABW, Seibold-Schmid B, Schoepfer AM, Flogerzi B, Müller S, et al. Deficiency for mannan-binding lectin is associated with antibodies to Saccharomyces cerevisiae in patients with Crohn’s disease and their relatives. Gut (2007) 56:152. doi:10.1136/gut.2006.110007
349. Seibold F, Konrad A, Flogerzi B, Seibold-Schmid B, Arni S, Jüliger S, et al. Genetic variants of the mannan-binding lectin are associated with immune reactivity to mannans in Crohn’s disease. Gastroenterology (2004) 127:1076–84. doi:10.1053/j.gastro.2004.07.056
350. Choteau L, Vasseur F, Lepretre F, Figeac M, Gower-Rousseau C, Dubuquoy L, et al. Polymorphisms in the mannose-binding lectin gene are associated with defective mannose-binding lectin functional activity in Crohn’s disease patients. Sci Rep (2016) 6:29636. doi:10.1038/srep29636
351. Joossens S, Pierik M, Rector A, Vermeire S, Ranst MV, Rutgeerts P, et al. Mannan binding lectin (MBL) gene polymorphisms are not associated with anti-Saccharomyces cerevisiae (ASCA) in patients with Crohn’s disease. Gut (2006) 55:746. doi:10.1136/gut.2005.089136
352. Kim YS, Kim Y-H, Ye BD, Park DW, Kim JW, Han DS. Mannose-binding lectin deficiency is not associated with anti-Saccharomyces cerevisiae antibody in Korean Crohn’s disease patients. Clin Chim Acta (2014) 429:206–11. doi:10.1016/j.cca.2013.12.019
353. Underhill DM. Macrophage recognition of zymosan particles. J Endotoxin Res (2003) 9:176–80. doi:10.1179/096805103125001586
354. Katragkou A, Pana ZD, Perlin DS, Kontoyiannis DP, Walsh TJ, Roilides E. Exserohilum infections: review of 48 cases before the 2012 United States outbreak. Med Mycol (2014) 52(4):376–86. doi:10.1093/mmy/myt030
355. Adler A, Yaniv I, Samra Z, Yacobovich J, Fisher S, Avrahami G, et al. Exserohilum: an emerging human pathogen. Eur J Clin Microbiol (2006) 25(4):247–53. doi:10.1007/s10096-006-0093-3
356. Sandoval-Denis M, Gene J, Sutton DA, Wiederhold NP, Cano-Lira JF, Guarro J. New species of Cladosporium associated with human and animal infections. Persoonia (2016) 36:281–98. doi:10.3767/003158516X691951
357. Cheng SC, Lin YY, Kuo CN, Lai LJ. Cladosporium keratitis – a case report and literature review. BMC Ophthalmol (2015) 15:106. doi:10.1186/s12886-015-0092-1
358. Zhou YB, Chen P, Sun TT, Wang XJ, Li DM. Acne-like subcutaneous phaeohyphomycosis caused by Cladosporium cladosporioides: a rare case report and review of published literatures. Mycopathologia (2016) 181(7–8):567–73. doi:10.1007/s11046-016-9995-5
359. Dixon DM, Walsh TJ, Merz WG, Mcginnis MR. Infections due to xylohypha-bantiana (Cladosporium, trichoides). Rev Infect Dis (1989) 11(4):515–25. doi:10.1093/clinids/11.4.515
360. Sandoval-Denis M, Sutton DA, Martin-Vicente A, Cano-Lira JF, Wiederhold N, Guarro J, et al. Cladosporium species recovered from clinical samples in the United States. J Clin Microbiol (2015) 53(9):2990–3000. doi:10.1128/JCM.01482-15
361. Mintz-Cole RA, Gibson AM, Bass SA, Budelsky AL, Reponen T, Hershey GK. Dectin-1 and IL-17A suppress murine asthma induced by Aspergillus versicolor but not Cladosporium cladosporioides due to differences in beta-glucan surface exposure. J Immunol (2012) 189(7):3609–17. doi:10.4049/jimmunol.1200589
362. Anstead GM, Sutton DA, Graybill JR. Adiaspiromycosis causing respiratory failure and a review of human infections due to Emmonsia and Chrysosporium spp. J Clin Microbiol (2012) 50(4):1346–54. doi:10.1128/JCM.00226-11
363. Hayashi S, Naitoh K, Matsubara S, Nakahara Y, Nagasawa Z, Tanabe I, et al. Pulmonary colonization by Chrysosporium zonatum associated with allergic inflammation in an immunocompetent subject. J Clin Microbiol (2002) 40(3):1113–5. doi:10.1128/JCM.40.3.1113-1115.2002
364. Suchonwanit P, Chaiyabutr C, Vachiramon V. Primary cutaneous Chrysosporium infection following ear piercing: a case report. Case Rep Dermatol (2015) 7(2):136–40. doi:10.1159/000436989
365. Siddiqui AS, Zimmerman JL. Pulmonary infection secondary to Chrysosporium zonatum in an immunocompetent man. Ann Am Thorac Soc (2016) 13(5):757–8. doi:10.1513/AnnalsATS.201601-083LE
366. Warwick A, Ferrieri P, Burke B, Blazar BR. Presumptive invasive Chrysosporium infection in a bone-marrow transplant recipient. Bone Marrow Transplant (1991) 8(4):319–22.
367. Levy FE, Larson JT, George E, Maisel RH. Invasive Chrysosporium infection of the nose and paranasal sinuses in an immunocompromised host. Otolaryngol Head Neck Surg (1991) 104(3):384–8. doi:10.1177/019459989110400317
368. Suankratay C, Dhissayakamol O, Uaprasert N, Chindamporn A. Invasive pulmonary infection caused by Chrysosporium articulatum: the first case report. Mycoses (2015) 58(1):1–3. doi:10.1111/myc.12270
369. Kenyon EM, Russell LH, Mcmurray DN. Isolation of Sporothrix schenckii from potting soil. Mycopathologia (1984) 87(1–2):128. doi:10.1007/BF00436641
370. Mehta KIS, Sharma NL, Kanga AK, Mahajan VK, Ranjan N. Isolation of Sporothrix schenckii from the environmental sources of cutaneous sporotrichosis patients in Himachal Pradesh, India: results of a pilot study. Mycoses (2007) 50(6):496–501. doi:10.1111/j.1439-0507.2007.01411.x
371. Barros MBD, Paes RD, Schubach AO. Sporothrix schenckii and sporotrichosis. Clin Microbiol Rev (2011) 24(4):633–54. doi:10.1128/CMR.00007-11
372. Moreira JA, Freitas DF, Lamas CC. The impact of sporotrichosis in HIV-infected patients: a systematic review. Infection (2015) 43(3):267–76. doi:10.1007/s15010-015-0746-1
373. Aung AK, Spelman DW, Thompson PJ. Pulmonary sporotrichosis: an evolving clinical paradigm. Semin Respir Crit Care Med (2015) 36(5):756–66. doi:10.1055/s-0035-1562901
374. Lederer HT, Sullivan E, Crum-Cianflone NF. Sporotrichosis as an unusual case of osteomyelitis: a case report and review of the literature. Med Mycol Case Rep (2016) 11:31–5. doi:10.1016/j.mmcr.2016.04.001
375. Vasquez-del-Mercado E, Arenas R, Padilla-Desgarenes C. Sporotrichosis. Clin Dermatol (2012) 30(4):437–43. doi:10.1016/j.clindermatol.2011.09.017
376. Zhang X, Zhang J, Huang H, Xue R, Hu X, Li M, et al. Taenia taeniaeformis in rat favors protracted skin lesions caused by Sporothrix schenckii infection: Dectin-1 and IL-17 are dispensable for clearance of this fungus. PLoS One (2012) 7(12):e52514. doi:10.1371/journal.pone.0052514
377. Guzman-Beltran S, Perez-Torres A, Coronel-Cruz C, Torres-Guerrero H. Phagocytic receptors on macrophages distinguish between different Sporothrix schenckii morphotypes. Microbes Infect (2012) 14(12):1093–101. doi:10.1016/j.micinf.2012.06.001
378. Kerscher B, Dambuza IM, Christofi M, Reid DM, Yamasaki S, Willment JA, et al. Signalling through MyD88 drives surface expression of the mycobacterial receptors MCL (Clecsf8, Clec4d) and Mincle (Clec4e) following microbial stimulation. Microbes Infect (2016) 18(7–8):505–9. doi:10.1016/j.micinf.2016.03.007
379. Longo LV, Nakayasu ES, Gazos-Lopes F, Vallejo MC, Matsuo AL, Almeida IC, et al. Characterization of cell wall lipids from the pathogenic phase of Paracoccidioides brasiliensis cultivated in the presence or absence of human plasma. PLoS One (2013) 8(5):e63372. doi:10.1371/journal.pone.0063372
380. Rizzetto L, De Filippo C, Cavalieri D. Richness and diversity of mammalian fungal communities shape innate and adaptive immunity in health and disease. Eur J Immunol (2014) 44(11):3166–81. doi:10.1002/eji.201344403
381. Cavalieri D, Di Paola M, Rizzetto L, Tocci N, De Filippo C, Lionetti P, et al. Genomic and phenotypic variation in morphogenetic networks of two Candida albicans isolates subtends their different pathogenic potential. Front Immunol (2017) 8:1997. doi:10.3389/fimmu.2017.01997
382. Schonherr FA, Sparber F, Kirchner FR, Guiducci E, Trautwein-Weidner K, Gladiator A, et al. The intraspecies diversity of C. albicans triggers qualitatively and temporally distinct host responses that determine the balance between commensalism and pathogenicity. Mucosal Immunol (2017) 10(5):1335–50. doi:10.1038/mi.2017.2
Keywords: pattern recognition receptor, C-type lectin receptor, Candida, Aspergillus, pathogenic fungi, single nucleotide polymorphisms
Citation: Goyal S, Castrillón-Betancur JC, Klaile E and Slevogt H (2018) The Interaction of Human Pathogenic Fungi With C-Type Lectin Receptors. Front. Immunol. 9:1261. doi: 10.3389/fimmu.2018.01261
Received: 09 March 2018; Accepted: 18 May 2018;
Published: 04 June 2018
Edited by:
Bernd Lepenies, University of Veterinary Medicine Hannover, GermanyReviewed by:
Gabriel Adrián Rabinovich, Instituto de Biología y Medicina Experimental (IBYME), ArgentinaLisa Rizzetto, Fondazione Edmund Mach, Italy
Copyright: © 2018 Goyal, Castrillón-Betancur, Klaile and Slevogt. This is an open-access article distributed under the terms of the Creative Commons Attribution License (CC BY). The use, distribution or reproduction in other forums is permitted, provided the original author(s) and the copyright owner are credited and that the original publication in this journal is cited, in accordance with accepted academic practice. No use, distribution or reproduction is permitted which does not comply with these terms.
*Correspondence: Hortense Slevogt, hortense.slevogt@med.uni-jena.de
†These authors have contributed equally to this work.