- 1Department of Ophthalmology, Graduate School of Medicine, The University of Tokyo, Tokyo, Japan
- 2Isotope Science Center, The University of Tokyo, Tokyo, Japan
- 3Department of Ophthalmology, Jichi Medical University Saitama Medical Center, Saitama, Japan
Recently developed technologies have revealed that the genomes of many organisms produce transcripts that do not encode proteins. These are called non-coding RNAs. Long non-coding RNAs (lncRNAs) are important regulators of the expression of their target genes at the levels of transcription, translation, and degradation. Multiple studies have demonstrated a role for lncRNAs in various biological responses, including pathogenic infection. Upon pathogenic infection, the expression levels of lncRNAs are dynamically altered, suggesting that lncRNAs are involved in the host immune response or propagation of pathogens. In this review, we focused on host lncRNAs that are involved in pathogenic infection. Some host lncRNAs act as host defense molecules to prevent pathogenic proliferation, while others are utilized by the pathogen to enhance the propagation of pathogens.
Introduction
Recent transcriptome analyses using next-generation sequencing have revealed that the genomes of many species produce a large number of RNAs with low protein-coding potential, known as non-coding RNAs (ncRNAs). These ncRNAs were originally considered junk RNAs with no cellular functions; however, later studies have demonstrated that many of these ncRNAs are functional (Rinn and Chang, 2012).
Infectious diseases are caused by pathogens, including bacteria, viruses, fungi, or other parasites. The invasion of these pathogens often induces an inflammatory (Mogensen, 2009) or immune response of the host cell through the interferon pathway (Haller et al., 2006). In this review, we focus on bacteria and viruses. Although both (though not all bacteria) can proliferate inside the host cells, the mechanisms are different. As for bacteria, they proliferate in distinct compartments like organelles of the host cells. In contrast, viruses replicate themselves and are packaged within the host cells; that is, they can directly interact their host DNAs, RNAs, and proteins. Therefore, viruses can modulate or even hijack their host gene expression processes or metabolic pathways for their proliferation. For example, influenza A virus (IAV) robs host mRNAs of capped 5′ RNA nucleotides for efficient transcription of viral transcripts, a process called cap-snatching (Dias et al., 2009).
Although the protein network involved in protection against pathogen infection has been intensively investigated (de Chassey et al., 2008), RNA-mediated regulation remains largely unknown. Recent studies have examined the miRNA-regulated response against pathogen infection. MiRNAs of both host cells and viruses regulate each other’s gene expression by binding their target transcripts using seed sequences (reviewed in Skalsky and Cullen, 2010; Bruscella et al., 2017). However, little is known about lncRNA-mediated regulatory mechanisms during infection.
In this review, lncRNAs are defined as transcripts longer than 200 nucleotides with no protein-coding potential. Here we reviewed the lncRNAs involved in pathogen infection, especially bacteria and viruses, with a focus on host lncRNAs. These lncRNAs are either upregulated or downregulated during infection and can function in enhancing the host defense program or promoting pathogen invasion or replication within the host cells.
Transcriptional Changes of LncRNAs Upon Pathogenic Infection
Transcriptome analyses revealed that lncRNAs as well as mRNAs show differential expression patterns during pathogen infection. For example, in response to meningitic Escherichia coli strain PCN033 infection of primary human brain microvascular endothelial cells, 382 lncRNAs were significantly upregulated and 513 were significantly downregulated (Yang et al., 2016). In WI-38 cells, antisense RNAs, a type of lncRNA expressed from the opposite strand of coding genes, are induced genome-widely after herpes simplex virus-1 (HSV-1) infection (Wyler et al., 2017). Another study revealed that 145 lncRNAs, including enhancer RNAs, were stabilized after Salmonella enterica serovar typhimurium virulent strain χ3306 infection, and knockdown of lncRNA NEAT1v2 or enhancer RNA eRNA07573 decreased cell survival rates after Salmonella infection, thus raising the possibility that these lncRNAs positively affect cell survival against Salmonella infection (Imamura et al., 2018). Together, these studies demonstrate that lncRNA expression levels are responsive to pathogenic infection.
LncRNAs That Regulate Host Immune Response to Pathogenic Infection (Table 1)
There are several types of lncRNA-mediated host defense responses during infection. In this section, we focus on the lncRNAs involved in host defense by regulating immune-related genes.
In human primary monocytes, stimulation by lipopolysaccharide (LPS), which is a main component of the outer membrane of Gram-negative bacteria, induces lncRNAs, enhancer RNAs, and bidirectional transcription. The IL1β locus is surrounded by enhancer RNA IL1β-eRNA and the bidirectionally transcribed transcript IL1β-RBT46. These lncRNAs localize in the nucleus and their expressions are dependent on NF-κB. Both IL1β-eRNA and IL1β-RBT46 regulate LPS-induced transcription of IL1β and CXCL8 (IIott et al., 2014).
In CD11c positive bone-marrow-derived dendritic cells, the long intervening non-coding RNA (lincRNA)-Cox2 is an important regulator that is induced during LPS stimulation (Guttman et al., 2009). In mouse bone-marrow derived macrophages, the lincRNA-Cox2 binds with heterogeneous nuclear ribonucleoprotein A/B and A2/B1 to induce and repress genes involved in the inflammation response (Carpenter et al., 2013). Recently, functions of lincRNA-Cox2 were identified using lincRNA-Cox2 KO mice, and this lncRNA regulates its neighbor gene Ptgs2 in cis and inflammatory genes in trans (Elling et al., 2018). In HD11 cells, LPS stimulation initiates the transient synthesis of a non-coding RNA which is transcribed from the upstream region of the lysozyme gene, and this leads chromatin to an open conformation and thus activates lysozyme expression (Lefevre et al., 2008).
In HuH7 cells derived from human hepatocarcinoma, both lncISG15 and lncBST2/BISPR were identified upon IFNα2 treatment and were also induced by hepatitis C virus infection. These lncRNAs are induced by interferon-dependent pathway and expressed near interferon-stimulated gene loci. Knockdown of lncBST2/BISPR by siRNA leads to a reduction of BST2 expression, suggesting that this lncRNA has some roles in regulating the expression of its neighboring genes (Barriocanal et al., 2014).
Some lncRNAs regulate gene expression of immune responsive genes by interacting with histone modification enzymes. For example, Nest is an lncRNA gene located adjacent to the interferon (IFN)-γ-encoding gene in both mice and human. In both genomes, NeST RNA is encoded on the DNA strand opposite to that coding for IFN-γ, and the two genes are transcribed by convergently (Vigneau et al., 2001). This lncRNA binds to WDR5, which is a component of the H3 lysine 4 methyltransferase complex, thus increasing expression of the IFN-γ locus (Figure 1A and Gomez et al., 2013). In CD8 positive T cells, tuberculosis infection-induced lncRNA-CD244 recruits enhancer of zeste homolog 2 (EZH2) polycomb protein and enhances H3K27 trimethylation at promoter regions of IFN-γ/TNF-α gene, thus downregulating these gene expression (Figure 1B and Wang et al., 2015). The lncRNA NRAV is suppressed upon IAV, Sendai virus, Muscovy Duck Reovirus, and a herpes simplex virus (HSV) infection to human alveolar epithelial cells. By overexpressing NRAV lncRNAs, the authors found that ISGs such as IFITM3 and MxA are downregulated with a decrease of H3K4me3 at their transcription start sites. In summary, NRAV acts as a negative regulator of ISGs (Ouyang et al., 2014).
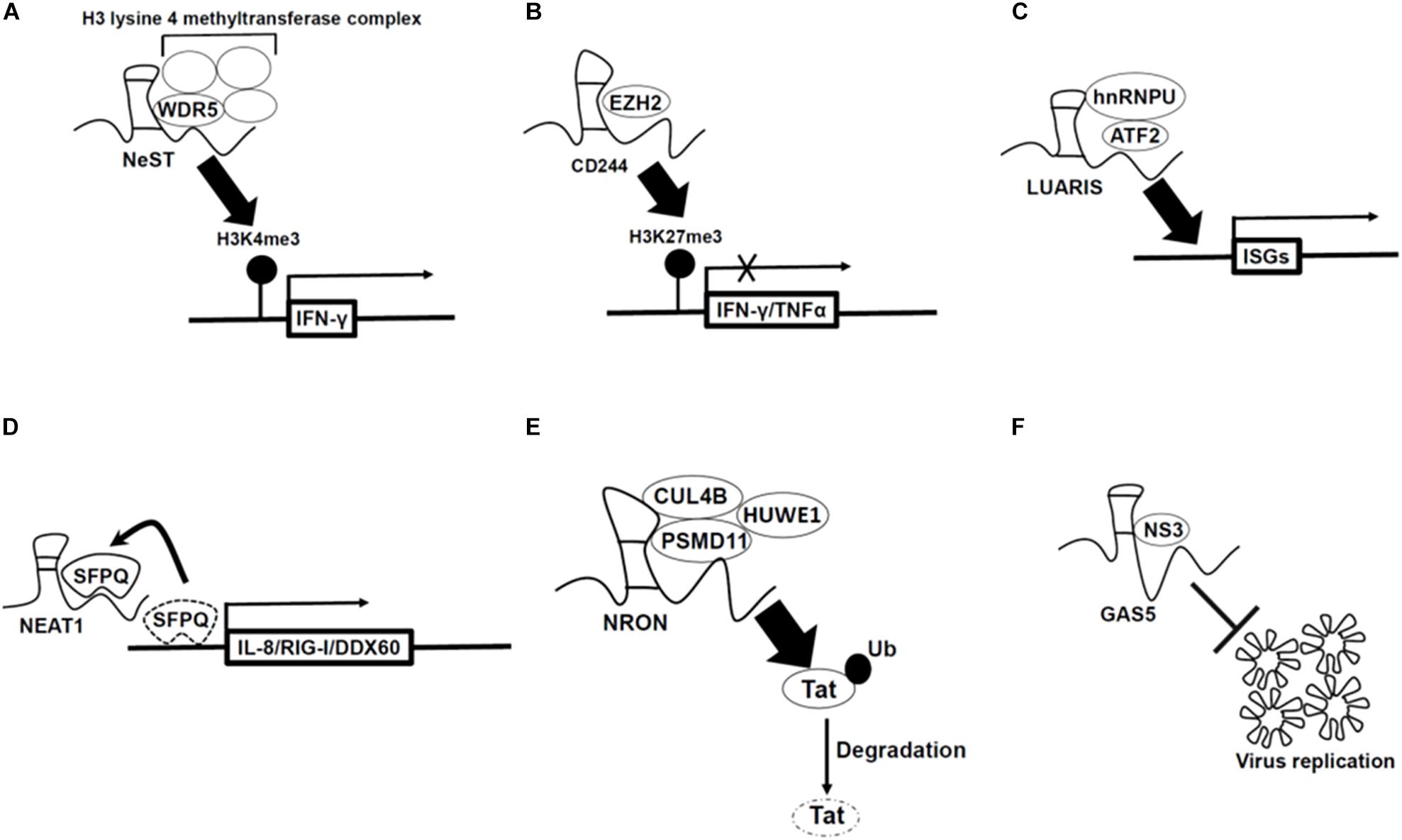
Figure 1. Mechanism by which long non-coding RNAs (lncRNAs) regulate antiviral genes expression (A–D) and viral replication (E,F). (A) LncRNA-Nest promotes IFN-γ gene expression by binding to WD repeat-containing protein 5 (WDR5) which is a component of the H3 lysine 4 methyltransferase complex. (B) LncRNA-CD244 recruits enhancer of zeste homolog 2 (EZH2) polycomb protein which is H3K27 methyltransferase, and thus enhances H3K27 trimethylation at promoter regions of IFN-γ/TNF-α gene and inhibits gene expression. (C) LncRNA-LUARIS recruits transcription factors [heterogeneous nuclear ribonucleoprotein U (hnRNPU), activating transcription factor 2 (ATF2)], and thus binds to promoter region of ISGs and promotes gene expression. (D) NEAT1 sequesters SFPQ proteins which suppress immune-related genes under non-infected condition, and thus increases those gene expression. (E) LncRNA-NRON binds with ubiquitin ligase (CUL4B, PSMD1, and HUWE1) and then ubiquitylates Tat protein (viral transcription activator) to degrade, which inhibits viral replication. (F) LncRNA-GAS5 acts as decoy of hepatitis C virus non-structural protein 3 (NS3), which inhibits viral replication and assembly.
Long non-coding RNA-LUARIS was identified by microarray analysis after treating human hepatocytes with poly(I:C), which mimics viral double-stranded RNAs. LUARIS is an antisense transcript of the protein-coding gene HECW1 and is suppressed by type I interferon signaling. Knockdown of LUARIS by siRNA led to a reduction of ISGs expression. LUARIS directly interacts with heterogeneous nuclear ribonucleoprotein U (hnRNPU) and activating transcription factor 2 (ATF2) to regulate expressions of ISGs (Figure 1C and Nishitsuji et al., 2016).
The lncRNA NEAT1, an architectural non-coding RNA that forms paraspeckles, is also involved in antiviral response. NEAT1 expression is upregulated upon influenza virus and HSV infection in HeLa cells. Splicing factor proline- and glutamine-rich (SFPQ) binds to the promoter region of IL8 in non-infected cells. However, after poly(I:C) treatment which is mimicking of viral infection, NEAT1 is induced and sequesters SFPQ to paraspeckles, thus enabling the expression of antiviral genes including those encoding cytokines such as IL-8 (Figure 1D and Imamura et al., 2014). A similar mechanism was also reported upon infection of Hantaan virus. In human umbilical vein endothelial cells, activation of the RIG-I-IRF7 pathway by Hantaan virus infection induced transcription of the NEAT1 gene. Then, NEAT1 sequesters SFPQ to paraspeckles to induce RIG-I and DDX60 transcription, thus leading to interferon-β production (Ma et al., 2017). NEAT1 also interacts with HEXIM1 which was previously discovered as a transcription inhibitor. In HeLa cells, NEAT1-HEXIM1 complex regulates the immune response through the cGAS-STING pathway upon infection by DNA viruses such as Kaposi’s sarcoma-associated herpesvirus (Morchikh et al., 2017).
LncRNAs That Inhibit Pathogen Proliferation (Table 2)
Another mechanism for lncRNA function in host defense is the prevention of pathogen replication. For example, in Jurkat cells, the lncRNA NRON represses human immunodeficiency virus type 1 (HIV-1) replication by inhibiting the transcription factor nuclear factor of activated T cells (NFAT) which enhances viral replication of HIV-1 (Imam et al., 2015). Recent studies showed that lncRNA NRON degrades Tat protein (viral transcription activator) through ubiquitination by interacting with the ubiquitin ligase CUL4B, PSMD11, and HUWE1 (UREB1) in TZM-bl cells (Figure 1E and Li et al., 2016).
Previously annotated lncRNAs have also been identified as repressors of viral replication. LncRNA GAS5, which was first identified as a growth-arrest specific transcript (Schneider et al., 1988) and related to cancer (Pickard et al., 2013), prevents hepatitis C virus replication in Huh7 cells. In addition, this lncRNA acts as a decoy of the hepatitis C virus NS3 protein which is important for viral replication and assembly (Figure 1F and Qian et al., 2016).
The lncRNA NEAT1 represses HIV-1 replication. HIV-1 mRNAs are spliced and exported to the cytoplasm through a Rev-dependent pathway (Malim et al., 1989). When NEAT1 is downregulated using siRNA, in HeLa cells, the non-spliced form of HIV-1 mRNAs that contain instability elements are found in the cytoplasm and leads to increased HIV-1 replication (Zhang et al., 2013). Similarly, in HeLa cells, NEAT1 and protein components of paraspeckles directly interact with HSV-1 genomes, and modulate both viral gene expression and replication of HSV-1 through the STAT3 transcription factor (Wang Z. et al., 2017).
LncRNAs That Promote Pathogen Proliferation (Table 2)
Some host lncRNAs are used by pathogens to promote pathogen proliferation. For example, lncRNA VIN was identified by microarray analysis in human lung epithelial cells infected with IAV. Downregulation of the lncRNA VIN by siRNA reduced IAV replication, thus indicating that this lncRNA is involved in efficient proliferation of IAV in host cells (Winterling et al., 2014). The lncRNA PAAN, which is upregulated upon IAV infection in HEK293T cells, helps the formation of the viral RNA polymerase complex by interacting with its component PA protein (Wang et al., 2018). The lncRNA ACOD1 is upregulated upon infection by viruses such as vesicular stomatitis virus, Sendai virus, HSV-1, and Vaccinia virus in mouse pertitoneal macrophages. Transcription of the lncRNA ACOD1 is independent of type-1 interferon but is induced by the NF-κB pathway which is activated by viral infection. The lncRNA ACOD1 directly interacts with the metabolic enzyme glutamic-oxaloacetic transaminase to enhance its catalytic activity, thus leading to viral proliferation (Wang P. et al., 2017). The lncRNA EGOT is increased by infection of hepatitis C virus, influenza virus or Semliki Forest virus through RIG-I and PKR activation in HuH7 cells. Downregulation of EGOT resulted in a decrease of hepatitis C virus replication. By performing guilt-by-association analysis, it was predicted that EGOT is negatively correlated with innate immune responsive genes such as TLR3, NF-κB, and IRF3. Therefore, the authors suggested that EGOT regulates the antiviral pathway negatively (Carnero et al., 2016).
Concluding Remarks and Perspectives
Long non-coding RNAs have attracted lots of attention because they are involved in various cellular functions despite their “non-coding” nature. Among them, some lncRNAs are identified as important regulators of host response toward pathogen infection as described in this review. However, considering that the functions of lncRNAs are regulated spatio-temporally, these analyses have dismissed the time-dependent effect or localization-dependent function of lncRNAs. Therefore, it might be possible that there are unknown lncRNAs whose expression levels do not change during infection but function by changing their localization patterns. In addition, NGS analyses using bulk (mixed population) samples disregard the heterogeneity of host cells or the difference of infection stages of the host cells, which results in difficulty in identifying functional lncRNAs whose expression levels differ among each host cell. The recently developed single cell RNA-seq (scRNA-seq) approach for infected cells is more informative (reviewed in Cristinelli and Ciuffi, 2018); however, scRNA-seq also is limited in its ability to obtain sufficient read depths of rarely expressed RNAs, such as lncRNAs or pathogen-derived RNAs.
The development of new technologies to overcome the limitations of read-depths or methods for detecting changes of RNA localization will identify new aspects of lncRNA-mediated responses toward pathogen infection.
Author Contributions
All authors listed have made a substantial, direct and intellectual contribution to the work, and approved it for publication.
Funding
This work was supported by the MEXT Kakenhi (18H02570 to NA).
Conflict of Interest
The authors declare that the research was conducted in the absence of any commercial or financial relationships that could be construed as a potential conflict of interest.
Acknowledgments
We thank Edanz Group (www.edanzediting.com/ac) for editing a draft of this manuscript.
References
Barriocanal, M., Carnero, E., Segura, V., and Fortes, P. (2014). Long non-coding RNA BST2/BISPR is induced by IFN and regulates the expression of the antiviral factor Tetherin. Front. Immunol. 5:655. doi: 10.3389/fimmu.2014.00655
Bruscella, P., Bottini, S., Baudesson, C., Pawlotsky, J.-M., Feray, C., and Trabucchi, M. (2017). Viruses and miRNAs: more friends than foes. Front. Microbiol. 8:824. doi: 10.3389/fmicb.2017.00824
Carnero, E., Barriocanal, M., Prior, C., Pablo Unfried, J., Segura, V., Guruceaga, E., et al. (2016). Long noncoding RNA EGOT negatively affects the antiviral response and favors HCV replication. EMBO Rep. 17, 1013–1028. doi: 10.15252/embr.201541763
Carpenter, S., Aiello, D., Atianand, M. K., Ricci, E. P., Gandhi, P., Hall, L. L., et al. (2013). A long noncoding RNA mediates both activation and repression of immune response genes. Science 341, 789–792. doi: 10.1126/science.1240925
Cristinelli, S., and Ciuffi, A. (2018). The use of single-cell RNA-Seq to understand virus–host interactions. Curr. Opin. Virol. 29, 39–50. doi: 10.1016/j.coviro.2018.03.001
de Chassey, B., Navratil, V., Tafforeau, L., Hiet, M. S., Aublin-Gex, A., Agaugué, S., et al. (2008). Hepatitis C virus infection protein network. Mol. Syst. Biol. 4:230. doi: 10.1038/msb.2008.66
Dias, A., Bouvier, D., Crépin, T., McCarthy, A. A., Hart, D. J., Baudin, F., et al. (2009). The cap-snatching endonuclease of influenza virus polymerase resides in the PA subunit. Nature 458:914. doi: 10.1038/nature07745
Elling, R., Robinson, E. K., Shapleigh, B., Liapis, S. C., Covarrubias, S., Katzman, S., et al. (2018). Genetic models reveal cis and trans immune-regulatory activities for lincRNA-Cox2. Cell Rep. 25, 1511–1524.e6. doi: 10.1016/j.celrep.2018.10.027
Gomez, J. A., Wapinski, O. L., Yang, Y. W., Bureau, J. F., Gopinath, S., Monack, D. M., et al. (2013). The NeST long ncRNA controls microbial susceptibility and epigenetic activation of the interferon-γ locus. Cell 152, 743–754. doi: 10.1016/j.cell.2013.01.015
Guttman, M., Amit, I., Garber, M., French, C., Lin, M. F., Feldser, D., et al. (2009). Chromatin signature reveals over a thousand highly conserved large non-coding RNAs in mammals. Nature 458, 223–227. doi: 10.1038/nature07672
Haller, O., Kochs, G., and Weber, F. (2006). The interferon response circuit: Induction and suppression by pathogenic viruses. Virology 344, 119–130. doi: 10.1016/j.virol.2005.09.024
IIott, N. E., Heward, J. A., Roux, B., Tsitsiou, E., Fenwick, P. S., Lenzi, L., et al. (2014). Long non-coding RNAs and enhancer RNAs regulate the lipopolysaccharide-induced inflammatory response in human monocytes. Nat. Commun. 5:3979. doi: 10.1038/ncomms4979
Imam, H., Bano, A. S., Patel, P., Holla, P., and Jameel, S. (2015). The lncRNA NRON modulates HIV-1 replication in a NFAT-dependent manner and is differentially regulated by early and late viral proteins. Sci. Rep. 5:8639. doi: 10.1038/srep08639
Imamura, K., Imamachi, N., Akizuki, G., Kumakura, M., Kawaguchi, A., Nagata, K., et al. (2014). Long noncoding RNA NEAT1-dependent SFPQ relocation from promoter region to paraspeckle mediates IL8 expression upon immune stimuli. Mol. Cell. 53, 393–406. doi: 10.1016/j.molcel.2014.01.009
Imamura, K., Takaya, A., Ishida, Y., Fukuoka, Y., Taya, T., Nakaki, R., et al. (2018). Diminished nuclear RNA decay upon Salmonella infection upregulates antibacterial noncoding RNAs. EMBO J. 37:e97723. doi: 10.15252/embj.201797723
Lefevre, P., Witham, J., Lacroix, C. E., Cockerill, P. N., and Bonifer, C. (2008). The LPS-induced transcriptional upregulation of the chicken lysozyme locus involves CTCF eviction and noncoding RNA transcription. Mol. Cell. 32, 129–139. doi: 10.1016/j.molcel.2008.07.023
Li, J., Chen, C., Ma, X., Geng, G., Liu, B., Zhang, Y., et al. (2016). Long noncoding RNA NRON contributes to HIV-1 latency by specifically inducing tat protein degradation. Nat. Commun. 7:11730. doi: 10.1038/ncomms11730
Ma, H., Han, P., Ye, W., Chen, H., Zheng, X., Cheng, L., et al. (2017). The long noncoding RNA NEAT1 exerts antihantaviral effects by acting as positive feedback for RIG-I signaling. J. Virol. 91:e02250-16. doi: 10.1128/JVI.02250-16
Malim, M. H., Hauber, J., Le, S.-Y., Maizel, J. V., and Cullen, B. R. (1989). The HIV-1 rev trans-activator acts through a structured target sequence to activate nuclear export of unspliced viral mRNA. Nature 338, 254–257. doi: 10.1038/338254a0
Mogensen, T. H. (2009). Pathogen recognition and inflammatory signaling in innate immune defenses. Clin. Microbiol. Rev. 22, 240–273. doi: 10.1128/CMR.00046-08
Morchikh, M., Cribier, A., Raffel, R., Amraoui, S., Cau, J., Severac, D., et al. (2017). HEXIM1 and NEAT1 long non-coding RNA form a multi-subunit complex that regulates DNA-mediated innate immune response. Mol. Cell 67, 387–399. doi: 10.1016/j.molcel.2017.06.020
Nishitsuji, H., Ujino, S., Yoshio, S., Sugiyama, M., Mizokami, M., Kanto, T., et al. (2016). Long noncoding RNA #32 contributes to antiviral responses by controlling interferon-stimulated gene expression. Proc. Natl. Acad. Sci. U.S.A. 113, 10388–10393. doi: 10.1073/pnas.1525022113
Ouyang, J., Zhu, X., Chen, Y., Wei, H., Chen, Q., Chi, X., et al. (2014). NRAV, a long noncoding RNA, modulates antiviral responses through suppression of interferon-stimulated gene transcription. Cell Host Microbe 16, 616–626. doi: 10.1016/j.chom.2014.10.001
Pickard, M. R., Mourtada-Maarabouni, M., and Williams, G. T. (2013). Long non-coding RNA GAS5 regulates apoptosis in prostate cancer cell lines. Biochim. Biophys. Acta 1832, 1613–1623. doi: 10.1016/j.bbadis.2013.05.005
Qian, X., Xu, C., Zhao, P., and Qi, Z. (2016). Long non-coding RNA GAS5 inhibited hepatitis C virus replication by binding viral NS3 protein. Virology 492, 155–165. doi: 10.1016/j.virol.2016.02.020
Rinn, J. L., and Chang, H. Y. (2012). Genome regulation by long noncoding RNAs. Annu. Rev. Biochem. 81, 145–166. doi: 10.1146/annurev-biochem-051410-092902
Schneider, C., King, R. M., and Philipson, L. (1988). Genes specifically expressed at growth arrest of mammalian cells. Cell 54, 787–793. doi: 10.1016/S0092-8674(88)91065-3
Skalsky, R. L., and Cullen, B. R. (2010). Viruses, microRNAs, and host interactions. Annu. Rev. Microbiol. 64, 123–141. doi: 10.1146/annurev.micro.112408.134243
Vigneau, S., Levillayer, F., Crespeau, H., Cattolico, L., Caudron, B., Bihl, F., et al. (2001). Homology between a 173-kb region from mouse chromosome 10, telomeric to the Ifng locus, and human chromosome 12q15. Genomics 78, 206–213. doi: 10.1006/geno.2001.6656
Wang, J., Wang, Y., Zhou, R., Zhao, J., Zhang, Y., Yi, D., et al. (2018). Host long noncoding RNA lncRNA-PAAN regulates the replication of influenza A virus. Viruses 10:E330. doi: 10.3390/v10060330
Wang, P., Xu, J., Wang, Y., and Cao, X. (2017). An interferon-independent lncRNA promotes viral replication by modulating cellular metabolism. Science 358, 1051–1055. doi: 10.1126/science.aao0409
Wang, Y., Zhong, H., Xie, X., Chen, C. Y., Huang, D., Shen, L., et al. (2015). Long noncoding RNA derived from CD244 signaling epigenetically controls CD8+ T-cell immune responses in tuberculosis infection. Proc. Natl. Acad. Sci. U.S.A. 112, E3883–E3892. doi: 10.1073/pnas.1501662112
Wang, Z., Fan, P., Zhao, Y., Zhang, S., Lu, J., Xie, W., et al. (2017). NEAT1 modulates herpes simplex virus-1 replication by regulating viral gene transcription. Cell. Mol. Life Sci. 74, 1117–1131. doi: 10.1007/s00018-016-2398-4
Winterling, C., Koch, M., Koeppel, M., Garcia-Alcalde, F., Karlas, A., and Meyer, T. F. (2014). Evidence for a crucial role of a host non-coding RNA in influenza A virus replication. RNA Biol. 11, 66–75. doi: 10.4161/rna.27504
Wyler, E., Menegatti, J., Franke, V., Kocks, C., Boltengagen, A., Hennig, T., et al. (2017). Widespread activation of antisense transcription of the host genome during herpes simplex virus 1 infection. Genome Biol. 18:209. doi: 10.1186/s13059-017-1329-5
Yang, R., Huang, F., Fu, J., Dou, B., Xu, B., Miao, L., et al. (2016). Differential transcription profiles of long non-coding RNAs in primary human brain microvascular endothelial cells in response to meningitic Escherichia coli. Sci. Rep. 6:38903. doi: 10.1038/srep38903
Keywords: long non-coding RNA, immune response, infection, bacteria, virus
Citation: Shirahama S, Miki A, Kaburaki T and Akimitsu N (2020) Long Non-coding RNAs Involved in Pathogenic Infection. Front. Genet. 11:454. doi: 10.3389/fgene.2020.00454
Received: 15 April 2019; Accepted: 14 April 2020;
Published: 26 May 2020.
Edited by:
Helder Nakaya, University of São Paulo, BrazilReviewed by:
Fatah Kashanchi, George Mason University, United StatesHaitao Luo, Institute of Computing Technology (CAS), China
Copyright © 2020 Shirahama, Miki, Kaburaki and Akimitsu. This is an open-access article distributed under the terms of the Creative Commons Attribution License (CC BY). The use, distribution or reproduction in other forums is permitted, provided the original author(s) and the copyright owner(s) are credited and that the original publication in this journal is cited, in accordance with accepted academic practice. No use, distribution or reproduction is permitted which does not comply with these terms.
*Correspondence: Nobuyoshi Akimitsu, YWtpbWl0c3VAcmljLnUtdG9reW8uYWMuanA=