- Grupo de Investigación en Reumatología. Instituto de Investigación Biomédica de A Coruña (INIBIC), Complexo Hospitalario Universitario de A Coruña (CHUAC), Sergas, Universidade da Coruña (UDC), A Coruña, Spain
During recent years, the significant influence of mitochondria on osteoarthritis (OA), the most common joint disease, has been consistently demonstrated. Not only mitochondrial dysfunction but also mitochondrial genetic polymorphisms, specifically the mitochondrial DNA haplogroups, have been shown to have an important influence on different OA-related features, including the prevalence, severity, incidence, and progression of the disease. This influence could probably be mediated by the role of mitochondria in the regulation of different processes involved in the pathogenesis of OA, such as energy production, the generation of reactive oxygen and nitrogen species, apoptosis, and inflammation. The regulation of these processes is at least partially controlled by the bi-directional communication between the nucleus and mitochondria, which permits the regulation of adaptation to a wide range of stressors and the maintenance of cellular homeostasis. This bi-directional communication consists of an “anterograde regulation” by which the nucleus regulates mitochondrial biogenesis and activity and a “retrograde regulation” by which both mitochondria and mitochondrial genetic variation exert a regulatory signaling control over the nuclear epigenome, which leads to the modulation of nuclear genes. Throughout this mini review, we will describe the evidence that demonstrates the profound influence of the mitochondrial genetic background in the pathogenesis of OA, as well as its influence on the nuclear DNA methylome of the only cell type present in the articular cartilage, the chondrocyte. This evidence leads to serious consideration of the mitochondrion as an important therapeutic target in OA.
Introduction
Osteoarthritis (OA) is the most common chronic progressive disorder that involves movable joints, occurring in 10-20% of the population over 50 years of age; the incidence of OA is estimated to double within the next 30 years (Blanco et al., 2011). The pathogenesis of OA is characterized by extracellular matrix degradation and cell stress initiated by micro- and macro-injuries that lead to the activation of maladaptive repair responses, including proinflammatory pathways of innate immunity. The disease manifests first as a molecular derangement (abnormal tissue metabolism) followed by anatomical and/or physiological derangements (characterized by cartilage degradation, bone remodeling, osteophyte formation, joint inflammation, and the loss of normal joint function) that can culminate in illness (Kraus et al., 2015). OA is actually considered a disease of the whole joint as an organ, resulting in organ dysfunction or joint failure (Blanco, 2014). In addition, this disease is one of the most common reasons for visits to primary care physicians and is also the leading cause of permanent work incapacitation. OA has no effective treatment today, and joint replacement is the only choice in cases of total joint dysfunction.
The nature of OA is heterogeneous, because a combination of factors such as occupation, age, gender, body mass index, and genetics have a profound influence on its pathogenesis. As a consequence of this, several different phenotypes characterize this disease, including inflammatory, aging-related, metabolic, pain, and post-traumatic phenotypes (Berenbaum, 2013; Sellam and Berenbaum, 2013; Blanco et al., 2018). In agreement with this heterogeneous nature, it has also been proposed that, on the one hand, mitochondria and mitochondrial DNA (mtDNA) have an important impact on the development of OA (Valdes and Goldring, 2017; Blanco et al., 2018; Li et al., 2019) and, on the other hand, that epigenetics is one of the main actors involved in the phenotypic modulation that articular chondrocytes, the only cell type present in articular cartilage, undergo during the OA process (Roach et al., 2005; Reynard and Loughlin, 2012; Blanco and Rego-Pérez, 2014). In this sense, it has also been consistently shown that specific mtDNA polymorphisms, called mtDNA haplogroups, have been associated not only with different OA-related features such as the incidence or progression of the disease (Fernandez-Moreno et al., 2017a; Fernandez-Moreno et al., 2017b; Koo et al., 2019) but also with the differential methylation status of articular cartilage (Cortes-Pereira et al., 2019). In light of the involvement of mitochondrial DNA in cell behavior and metabolism (Wallace et al., 1999; Martínez-Redondo et al., 2010), in this review, we discuss some of the evidence implicating the epigenetic effect of mtDNA variation in the pathogenesis of OA.
Mitochondria in Osteoarthritis
Because articular chondrocytes are highly glycolytic cells that obtain their energy mainly from anaerobic glucose metabolism, the role of mitochondria in the pathogenesis of OA was not studied in depth until early 2000. However, a large number of studies have demonstrated the profound influence of mitochondria in the pathogenesis of OA.
Terkeltaub and co-workers published a full review describing how mitochondrial impairment of chondrocytes is an important mediator of the establishment of OA (Terkeltaub et al., 2002). Specifically, these and other authors described that mitochondrial dysfunction mediates several specific pathogenic pathways implicated in the OA process, including oxidative stress, chondrocyte apoptosis, cartilage matrix calcification, autophagy, impaired anabolic and growth response of chondrocytes, and increased cytokine-induced inflammation (Terkeltaub et al., 2002; Blanco et al., 2004; Lotz and Loeser, 2012; López de Figueroa et al., 2015). In agreement with these findings, our group also demonstrated that, compared with healthy chondrocytes from patients of the same age, osteoarthritic chondrocytes have reduced mitochondrial activity, mainly in complexes II and III, and increased mitochondrial mass (Maneiro et al., 2003). In addition, the apoptotic mitochondrial pathway is implicated in the apoptosis of osteoarthritic chondrocytes (Hwang and Kim, 2015), and the inhibition of mitochondrial complexes III and V increases the mitochondrial-mediated inflammatory response in OA chondrocytes mediated by an overproduction of reactive oxygen species (ROS) (Vaamonde-García et al., 2012). Mitochondrial dysfunction has also been associated with a significant downregulation of superoxide dismutase 2 (SOD2) (Gavriilidis et al., 2013), one of the major mitochondrial antioxidant proteins, whose levels are also diminished in the superficial layers of end-stage OA cartilage (Ruiz-Romero et al., 2009; Scott et al., 2010).
Mitophagy is a form of autophagy, a process that involves the removal of damaged macromolecules and organelles to regulate cell homeostasis (Choi et al., 2013); specifically, mitophagy consists of the elimination of depolarized and damaged mitochondria. Different studies have demonstrated that the activation of this process protects against mitochondrial dysfunction, prevents ROS production and improves chondrocyte survival under pathological conditions (López de Figueroa et al., 2015; Ansari et al., 2018). It has also been demonstrated that mitochondrial biogenesis is deficient in human OA chondrocytes, leading the chondrocyte to adopt procatabolic responses; however, the activation of the AMP-activated protein kinase (AMPK)-NAD-dependent protein deacetylase sirtuin-1 (SIRT1)-peroxisome proliferator-activated receptor γ co-activator 1α (PGC1α) pathway reverses impaired mitochondrial biogenesis, which is mediated by mitochondrial transcriptional factor A (TFAM) (Wang et al., 2015).
mtDNA Haplogroups and Osteoarthritis
Mitochondria are considered unique organelles because they contain their own maternally inherited DNA, the mtDNA, containing 2 rRNAs, 22 tRNAs, and 13 essential mitochondrial protein-coding genes. The mutation rate of this circular molecule is higher than that of the nuclear DNA, mainly due to i) its proximity to the main source of ROS production, ii) the lack of an efficient repair system, and iii) the higher replication rate of mtDNA (Li et al., 2019). As a consequence of this, mtDNA sequences evolved by sequentially accumulating functional mutations along radiating maternal lineages when humans migrated out of Africa and adapted their energy metabolism to different environments, giving rise to mtDNA haplogroups (Torroni et al., 1996; Wallace, 2016). However, these types of mtDNA variants, though providing an important degree of adaptation as people migrated into new environments, might also have contributed to modern human disorders such as hypertension, diabetes, obesity, or neurodegenerative diseases (Ruiz-Pesini et al., 2004; Marom et al., 2017).
In this sense, OA is not an exception. During recent years, different studies have shown clinical associations between specific mtDNA haplogroups and different OA-related features, including the prevalence, progression, and incidence of the disease (Table 1).
mtDNA Haplogroups and the Prevalence of OA
Different studies have shown significant associations between specific mtDNA haplogroups and the prevalence of OA. Specifically, mtDNA variants belonging to the European cluster JT have been associated with a lower risk of knee and hip OA in a cohort of Spanish patients (Rego-Perez et al., 2008; Rego et al., 2010). mtDNA haplogroup T was also associated with a decreased risk of knee OA in a population from the United Kingdom (Soto-Hermida et al., 2014a); however, a later study in a larger population cohort from the same country failed to replicate these findings (Hudson et al., 2013). In addition, Asian mtDNA haplogroups B and G have been described as protective and risk factors respectively for knee OA in a population of southern China (Fang et al., 2014). The authors have proposed that the mechanisms that could explain that association are related to the alteration of both mitochondrial function and OA-related signaling pathways (Fang et al., 2016). A meta-analysis summarizing most of the studies described above concluded that mtDNA cluster JT is associated with a lower risk of OA prevalence in Spanish populations (Shen et al., 2014).
A plausible cause for the lack of replication among different case-control studies, even when nuclear DNA polymorphisms are analyzed, could be the unavailability of knee (or hip) radiographs for most population-based controls. This is not a minor issue, since up to 50% of people without joint symptoms may develop radiographic changes comparable to OA (Hannan et al., 2000). In this sense, our group has always considered the radiological status, because we believe that both mitochondrial dysfunction and mtDNA variation have a greater impact on the evolution of joint structure than on pain.
mtDNA Haplogroups and Radiographic OA Progression and Incidence
The use of well-characterized prospective cohorts of patients permits rigorous studies to analyze the influence of mtDNA haplogroups on the rate of progression and incidence of OA over time. mtDNA variants within mitochondrial cluster JT were associated with lower rates of radiographic knee OA progression in different world populations, including Spain, the USA, and the Netherlands. Specifically, in a Spanish cohort, mtDNA cluster JT was associated with a lower rate of radiographic progression in terms of Kellgren and Lawrence grade (Kellgren and Lawrence, 1957), and even patients with haplogroup H were more prone to requiring total joint replacement (Soto-Hermida et al., 2015). mtDNA haplogroup T was associated with a decreased rate of radiographic knee OA progression, as well as a reduced loss of knee cartilage integrity over time in patients of the Osteoarthritis Initiative (OAI) of the US National Institutes of Health (NIH) (Soto-Hermida et al., 2014b). This association was then replicated in the CHECK cohort (cohort hip and cohort knee), another prospective cohort of OA patients from the Netherlands (Fernandez-Moreno et al., 2017b). Finally, a subsequent meta-analysis including the above-mentioned studies confirmed that mtDNA variants of the JT cluster act as protective factors against the radiographic progression of the disease (Fernandez-Moreno et al., 2017b).
In terms of disease incidence, a meta-analysis including 3217 individuals from the OAI and CHECK cohorts concluded that, compared with the most common Caucasian haplogroup H, subjects with mtDNA haplogroup J show a lower rate of incident knee OA over an eight-year period (Fernandez-Moreno et al., 2017a). This study included the design of a cellular model of transmitochondrial cybrids, consisting of cells with a defined and uniform nuclear background containing mitochondria from different sources, to demonstrate the existence of functional differences between haplogroups H and J; the study concluded that, compared with H cybrids, J cybrids produce less ATP, but this was accompanied with lower amounts of peroxynitrite and mitochondrial superoxide anion together with a lower rate of apoptosis under stress conditions as well as an increased ability to cope with oxidative stress (Fernandez-Moreno et al., 2017a). Another study in Korean populations showed that Asian mtDNA haplogroup B, described as a protective factor against knee OA prevalence in a population from the south of China (Fang et al., 2014), was a risk factor for the incidence of knee OA over an eight-year period (Koo et al., 2019).
mtDNA Haplogroups and Biomarkers in OA
In an effort to detect structural changes in an early stage of the disease, to monitor disease progression, or even to assess therapeutic responses with more sensitivity and reliability, molecular biomarkers have been developed in OA (Garnero et al., 2002; Rousseau and Delmas, 2007; Camacho-Encina et al., 2019). In a set of clinically relevant studies, OA-protective haplogroup J has been significantly associated with lower serum levels of catabolic type II collagen biomarkers and matrix metalloproteinases, in contrast to haplogroup H carriers, which showed significantly higher levels (Rego-Perez et al., 2010; Rego-Perez et al., 2011). Despite not being considered a biomarker of the disease, although higher-than-normal production has been described in OA chondrocytes (Maneiro et al., 2005; Henrotin and Kurz, 2007), the production of nitric oxide (NO) is significantly lower in articular chondrocytes harboring mtDNA haplogroup J than in non-J chondrocytes (Fernandez-Moreno et al., 2011). Based on these findings, haplogroups J and H represent two different OA phenotypes, leading to the consideration of these mtDNA haplogroups as complementary genetic biomarkers of the disease (Fernandez-Moreno et al., 2012).
In terms of imaging biomarkers, the identification and quantification of early bone marrow lesions (BMLs) has great relevance for assessing symptomatic progression and radiographic worsening over time in patients with OA (Roemer et al., 2016). In this sense, a longitudinal study including 255 participants from the OAI cohort that developed incident knee OA at 48 months revealed that patients with mtDNA haplogroup J were less likely to develop large BMLs in the tibiofemoral compartment of the knee than those with mtDNA haplogroup H (Rego-Perez et al., 2018). This association could be due to the differential behavior of mtDNA haplogroups H and J in terms of the metabolic activity and inflammation that takes place in BMLs (Kuttapitiya et al., 2017).
In summary, in Caucasian populations, it seems that haplogroups belonging to mitochondrial cluster JT are protective against OA and have even been associated with increased longevity in some European populations (Dato et al., 2004). However, in energy-deficiency diseases, such as LHON (Leber Hereditary Optic Neuropathy) these haplogroups, specifically haplogroup J, are risk factors (Hudson et al., 2007). This controversy is potentially related to the uncoupling nature of the genetic polymorphisms associated with these haplogroups, by which ATP production is reduced but, conversely, lower ROS generation, oxidative damage, and apoptosis are also expected (Ruiz-Pesini et al., 2004).
The Mitochondrial Genome as an Epigenetic Regulator in Articular Cartilage
It is well known that bi-directional communication exists between the nucleus and mitochondria with the aim of maintaining cellular homeostasis and regulating adaptation to a broad range of stressors (Quirós et al., 2016; Wallace, 2016). This communication implies, on the one hand, that mitochondria are controlled by the nucleus by means of an “anterograde regulation,” a mechanism that regulates mitochondrial activity and biogenesis to provide cellular needs; on the other hand, mitochondria and mtDNA variation maintain partial regulatory signaling control over the nucleus through a “retrograde regulation,” which leads to the modification of cellular metabolism and function by activating the expression of nuclear genes with the aim of protecting against mitochondrial dysfunction (Jazwinski, 2013; Horan and Cooper, 2014; Matilainen et al., 2017).
Based on the above findings, and taking into account the reported associations between common mtDNA variants and different physiological and pathological phenotypes (Gómez-Durán et al., 2010; Martínez-Redondo et al., 2010; Marom et al., 2017), it can be deduced that interactions between mtDNA sequences, nuclear DNA, and the environment have important effects on mammalian biology (Kenney et al., 2014). In this sense, the effect of specific nuclear polymorphisms classically identified as risk factors for different diseases, such as Parkinson´s disease, cancer, and severe cardiopathy, is also modulated by mtDNA variation (Maruszak et al., 2008; Strauss et al., 2013; Blein et al., 2015). In the case of OA, as subjects carrying mtDNA haplogroup J are not fully protected from suffering from the disease, it would be interesting to investigate potential interactions between this haplogroup, as well as the biochemically opposite haplogroup H (Wallace et al., 1999; Martínez-Redondo et al., 2010), and the most robust nuclear polymorphisms described in different GWAS performed in OA (Warner and Valdes, 2017; Zengini et al., 2018; Tachmazidou et al., 2019).
In terms of animal models, interesting work in conplastic mice (mice with a constant nuclear background but different mtDNA genomes) showed profound differences in health longevity between conplastic strains. The level of divergence between the two strains, equivalent to that between human African and Eurasian mtDNAs, showed different behavior in terms of mitochondrial proteostasis, reactive oxygen generation, obesity, and insulin signaling as well as in cell-senescence-related parameters such as telomere shortening and mitochondrial dysfunction (Latorre-Pellicer et al., 2016). Most of the altered processes described in the work of Latorre-Pellicer and co-workers are also involved in many common human diseases. In agreement with this, preliminary analyses of OA-related features using the two strains of these animals revealed significant differences between them in terms of the expression of the autophagy-related protein microtubule-associated protein 1 light chain 3 (LC3) and extracellular matrix-degrading protein metalloproteinase-13 (MMP-13) as well as significant differences in the Mankin score, a scoring system for the histopathological classification of the severity of cartilage lesions in OA (Scotece et al., 2019).
In this context of mitochondrial-nuclear interactions, epigenetics emerges as an important mechanism. Specifically, DNA methylation is the best-characterized epigenetic mechanism in OA. DNA methylation consists of the addition of a methyl group (CH3) by S-adenosyl-methionine (SAM) to a cytosine that lies at 5´ of guanine (CpG site) to give rise to methylated cytosine. When this process occurs in high-density CpG regions of promoters, gene silencing occurs; in contrast, when methylation occurs in gene bodies, it leads to increased gene expression (Hellman and Chess, 2007). In contrast to nuclear DNA, the effects of mtDNA methylation in OA have not been explored so far; however, it is well accepted that mtDNA variation is able to modulate the nuclear methylome. Consistent with this concept, two independent studies using transmitochondrial cybrids showed that global DNA methylation levels are differentially modulated by mtDNA haplogroups J and H (Bellizzi et al., 2012; Atilano et al., 2015); in addition, these two haplogroups also mediate the methylation profile and the expression levels of genes involved in angiogenesis, inflammation, and other signaling pathways (Atilano et al., 2015). Moreover, different haplogroups on a uniform nuclear background of mouse embryonic stem cells were also associated with different methylation profiles and gene expression (Kelly et al., 2013).
In the case of OA, DNA methylation is involved in the phenotypic modulation that articular chondrocytes undergo during the OA process (Roach et al., 2005). After the few first studies, in which the methylation pattern of specific genes involved in OA pathogenesis was explored (Roach et al., 2005; Hashimoto et al., 2013), genome-wide DNA methylation assays were performed. These studies not only showed that knee and hip OA cartilages have different DNA methylation patterns but also identified a subgroup of OA patients with an enrichment of altered genes involved in inflammation and immunity (Fernandez-Tajes et al., 2014; Rushton et al., 2014). The only study that has so far analyzed the effect of mtDNA variation on the methylome of articular cartilage revealed that cartilages harboring haplogroups H and J show a differential methylation pattern, regardless of diagnosis. The study consisted of a genome-wide DNA methylation approach followed by a whole transcriptomic assay and demonstrated that apoptosis is enhanced in haplogroup H cartilage samples, together with an enrichment of overexpressed genes related to cell death; on the contrary, apoptosis appeared more repressed in haplogroup J cartilages. In addition, compared with H cartilages, samples with haplogroup J also showed a significant enrichment of hypomethylated CpGs of genes related to developmental process, including those belonging to the homeobox family of transcription factors, while H cartilages showed an enrichment of genes related to metabolic processes (Cortes-Pereira et al., 2019). These findings reflect that the epigenetic modifications that occur during the pathogenesis of OA and affect different key processes, such as metabolic alterations or apoptosis, vary depending on the mitochondrial genetic background, and this could determine the evolution of the disease (Figure 1).
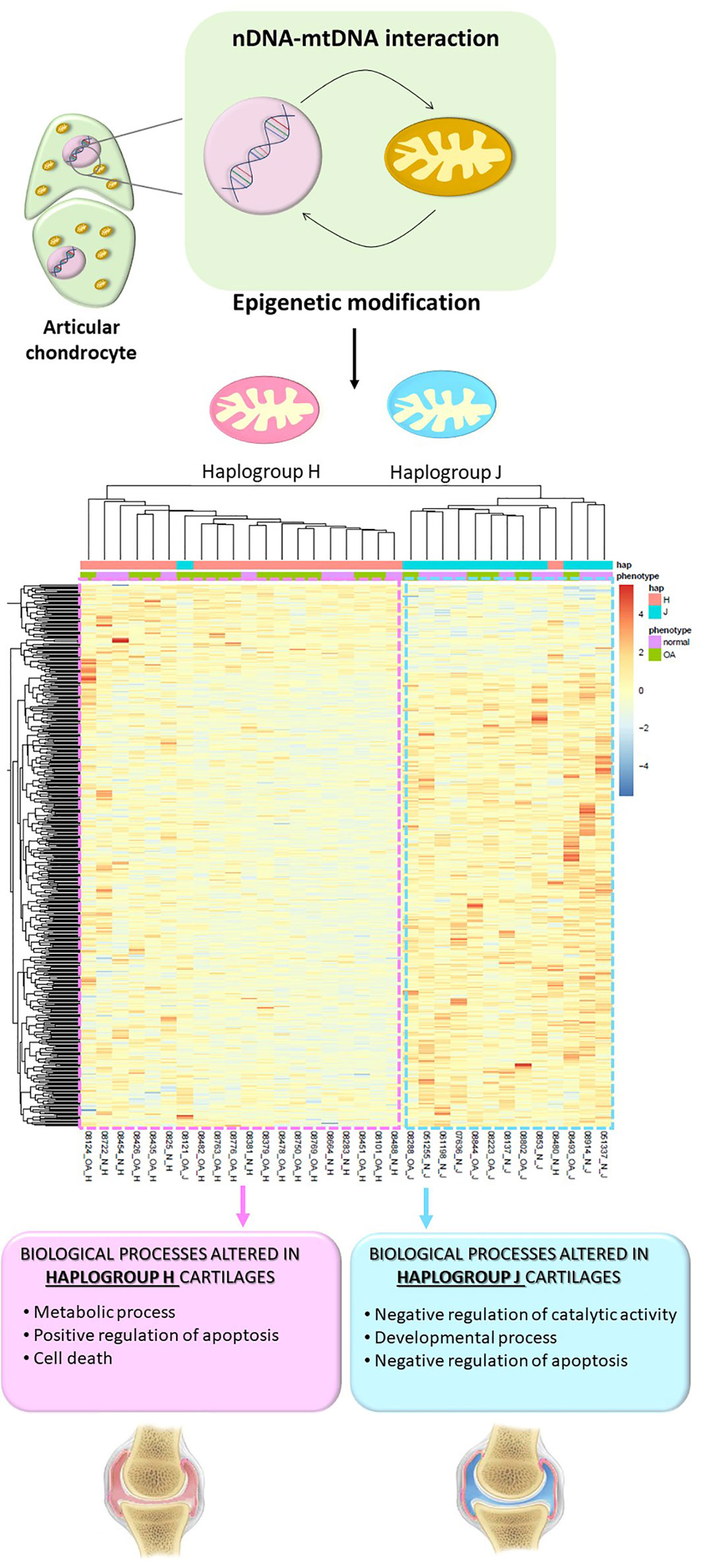
Figure 1 The interactions between the nucleus and mitochondria that take place inside articular chondrocytes give rise to epigenetic modifications that are mediated by mitochondrial DNA haplogroups. As a consequence, different haplogroup-associated methylation patterns condition key processes related to the development of OA. Permission is granted for publication of this figure as a modified version of the figure that appeared on page A17 of the July 2019 issue of Arthritis & Rheumatology (Clinical Connections).
Based on the description above, it is conceivable to consider the mitochondrial genome as an epigenetic regulator of the nuclear genome in articular cartilage. Stimuli such as reactive metabolic intermediates from the mitochondrial metabolism, small RNAs, mitochondria-derived peptides, and/or reactive oxygen species could be considered among the underlying mechanisms by which mitochondrial variation promotes modifications in the nuclear DNA methylome in OA (Schroeder et al., 2013; Horan and Cooper, 2014; Shadel and Horvath, 2015).
Conclusions and Future Directions
The evidence presented in this review supports the hypothesis that mitochondrial genetics not only influences different features of OA disease but also modulates, in terms of mtDNA haplogroups, the nuclear DNA methylome of the only cell type present in articular cartilage, the chondrocyte. Based on this evidence, a broad field of promising research lies ahead.
Efforts must be made in the use of conplastic mice to investigate the influence of the mitochondrial background on specific OA-related features in animal models that develop spontaneous OA as well as in conplastic animals with induced OA. On the other hand, of special interest would be the design of transmitochondrial cybrids using chondrocytes as stable nuclear donors to subsequently test the influence of mtDNA variants in the native cells of articular cartilage. The use of both animal and cellular models, together with the methylation data originating from different genome-wide methylation studies, could contribute to the development of molecular biomarkers aimed at identifying specific OA phenotypes from the design of CpG classifier panels combined with the mitochondrial genetic background.
Given the role of adaptive selection in the origin of mtDNA haplogroups, and recognizing that they could be maladaptive in different environments with new lifestyles (Wallace, 2005), the proposed study of potential interactions between mtDNA variants and different nuclear DNA polymorphisms previously associated with OA susceptibility in various GWAS should be conducted taking into account the specific environment. Because none of the studies described in Table 1 aimed to identify specific or single mitochondrial polymorphisms associated with different OA-related features, the precise identification through next-generation sequencing techniques of these specific mtDNA polymorphisms, characteristic or not of each haplogroup, from both isolated blood and articular cartilage from the same patient, would be a powerful tool for the consideration of mtDNA variation as a potential robust biomarker of OA.
In terms of therapeutic research, the restoration of mitochondrial function in OA chondrocytes would be the ultimate goal. This can be achieved by using different approaches to design different drugs that are capable of: i) suppressing mitochondrial oxidative damage and restoring extracellular matrix homeostasis (Farnaghi et al., 2017), ii) activating the AMPK-SIRT1-PGC1α pathway to induce mitochondrial biogenesis, therefore decreasing the pro-catabolic response of chondrocytes (Wang et al., 2015), iii) activating mitophagy, given its importance in preventing mitochondrial dysfunction (López de Figueroa et al., 2015), or iv) emulating the physiological effects of the OA-protective mtDNA haplogroup J on mitochondrial activity, as well as administering healthy isolated mitochondria into the osteoarthritic joint. On the other hand, given the bi-directional communication between the nucleus and mitochondria, interventions focused on the management of mitochondrial dysfunction by targeting the epigenome, or vice versa (Matilainen et al., 2017), would also be of interest.
Author Contributions
FB and IR-P contributed equally to the design and coordination of the study; both conceived the study and participated in its design. AD-S and PR-L contributed to some of the findings described in the manuscript and helped to draft the final version of the manuscript.
Funding
This work is supported by grants from Fondo de Investigación Sanitaria (CIBERCB06/01/0040-Spain, RETIC-RIER-RD16/0012/0002, PRB2-ISCIII-PT17/0019/0014, PI14/01254, PI16/02124 and PI17/00210) integrated in the National Plan for Scientific Program, Development and Technological Innovation 2013–2016 and funded by the ISCIII-General Subdirection of Assessment and Promotion of Research-European Regional Development Fund (FEDER) “A way of making Europe”. IR-P is supported by Contrato Miguel Servet-II Fondo de Investigación Sanitaria (CPII17/00026).
Conflict of Interest
The authors declare that the research was conducted in the absence of any commercial or financial relationships that could be construed as a potential conflict of interest.
Acknowledgments
Figure 1 is a modification of a figure originally published in the Clinical Connections section of Arthritis & Rheumatology, volume 71 (2019).
References
Ansari, M. Y., Khan, N. M., Ahmad, I., Haqqi, T. M. (2018). Parkin clearance of dysfunctional mitochondria regulates ROS levels and increases survival of human chondrocytes. Osteoarthritis Cartilage 26 (8), 1087–1097. doi: 10.1016/j.joca.2017.07.020
Atilano, S. R., Malik, D., Chwa, M., Cáceres-Del-Carpio, J., Nesburn, A. B., Boyer, D. S., et al. (2015). Mitochondrial DNA variants can mediate methylation status of inflammation, angiogenesis and signaling genes. Hum. Mol. Genet. 24 (16), 4491–4503. doi: 10.1093/hmg/ddv173
Bellizzi, D., D'Aquila, P., Giordano, M., Montesanto, A., Passarino, G. (2012). Global DNA methylation levels are modulated by mitochondrial DNA variants. Epigenomics 4 (1), 17–27. doi: 10.2217/epi.11.109
Berenbaum, F. (2013). Osteoarthritis as an inflammatory disease (osteoarthritis is not osteoarthrosis)! Osteoarthritis Cartilage 21 (1), 16–21. doi: 10.1016/j.joca.2012.11.012
Blanco, F. J., Rego-Pérez, I. (2014). Editorial: is it time for epigenetics in osteoarthritis? Arthritis Rheumatol. 66 (9), 2324–2327. doi: 10.1002/art.38710
Blanco, F. J., Lopez-Armada, M. J., Maneiro, E. (2004). Mitochondrial dysfunction in osteoarthritis. Mitochondrion. Netherlands 4 (5–6), 715–728. doi: 10.1016/j.mito.2004.07.022
Blanco, F. J., Rego, I., Ruiz-Romero, C. (2011). The role of mitochondria in osteoarthritis. Nat. Rev. Rheumatol. 7 (3), 161–169. doi: 10.1038/nrrheum.2010.213
Blanco, F. J., Valdes, A. M., Rego-Perez, I. (2018). Mitochondrial DNA variation and the pathogenesis of osteoarthritis phenotypes. Nature Reviews Rheumatology 14 (6), 327–340. doi: 10.1038/s41584-018-0001-0
Blanco, F. J. (2014). Osteoarthritis: something is moving. Reumatol. Clin. 10 (1), 4–5. doi: 10.1016/j.reuma.2013.12.001
Blein, S., Bardel, C., Danjean, V., McGuffog, L., Healey, S., Barrowdale, D., et al. (2015). An original phylogenetic approach identified mitochondrial haplogroup T1a1 as inversely associated with breast cancer risk in BRCA2 mutation carriers. Breast Cancer Res. 17, 61. doi: 10.1186/s13058-015-0567-2
Camacho-Encina, M., Balboa-Barreiro, V., Rego-Perez, I., Picchi, F., VanDuin, J., Qiu, J., et al. (2019). Discovery of an autoantibody signature for the early diagnosis of knee osteoarthritis: data from the Osteoarthritis Initiative. Ann. Rheum. Dis. 78 (12), 1699–1705. doi: 10.1136/annrheumdis-2019-215325
Choi, A. M., Ryter, S. W., Levine, B. (2013). Autophagy in human health and disease. N. Engl. J. Med. 368 (19), 1845–1846. doi: 10.1056/nejmra1205406
Cortes-Pereira, E., Fernandez-Tajes, J., Fernandez-Moreno, M., Vazquez-Mosquera, M. E., Relano, S., Ramos-Louro, P., et al. (2019). Mitochondrial DNA (mtDNA) haplogroups J and H are differentially associated with the methylation status of articular cartilage: potential role in apoptosis and metabolic and developmental processes. Arthritis Rheumatology (Hoboken NJ). 71 (7), 1191–1200. doi: 10.1002/art.40857
Dato, S., Passarino, G., Rose, G., Altomare, K., Bellizzi, D., Mari, V., et al. (2004). Association of the mitochondrial DNA haplogroup J with longevity is population specific. Eur. J. Hum. Genet. 12 (12), 1080–1082. doi: 10.1038/sj.ejhg.5201278
Fang, H., Liu, X., Shen, L., Li, F., Liu, Y., Chi, H., et al. (2014). Role of mtDNA haplogroups in the prevalence of knee osteoarthritis in a southern Chinese population. Int. J. Mol. Sci. 15 (2), 2646–2659. doi: 10.3390/ijms15022646
Fang, H., Zhang, F., Li, F., Shi, H., Ma, L., Du, M., et al. (2016). Mitochondrial DNA haplogroups modify the risk of osteoarthritis by altering mitochondrial function and intracellular mitochondrial signals. Biochim. Biophys. Acta 1862 (4), 829–836. doi: 10.1016/j.bbadis.2015.12.017
Farnaghi, S., Prasadam, I., Cai, G., Friis, T., Du, Z., Crawford, R., et al. (2017). Protective effects of mitochondria-targeted antioxidants and statins on cholesterol-induced osteoarthritis. FASEB J 31 (1), 356–367. doi: 10.1096/fj.201600600r
Fernandez-Moreno, M., Tamayo, M., Soto-Hermida, A., Mosquera, A., Oreiro, N., Fernandez-Lopez, C., et al. (2011). mtDNA haplogroup J Modulates telomere length and nitric oxide production. Bmc Musculoskeletal Disord. 283. doi: 10.1186/1471-2474-12-283
Fernandez-Moreno, M., Soto-Hermida, A., Oreiro, N., Pertega, S., Fenandez-Lopez, C., Rego-Perez, I., et al. (2012). Mitochondrial haplogroups define two phenotypes of osteoarthritis. Front. Physiol. 129. doi: 10.3389/fphys.2012.00129.
Fernandez-Moreno, M., Soto-Hermida, A., Vazquez-Mosquera, M. E., Cortes-Pereira, E., Relano, S., Hermida-Gomez, T., et al. (2017a). Mitochondrial DNA haplogroups influence the risk of incident knee osteoarthritis in OAI and CHECK cohorts. A meta-analysis and functional study. Ann. Rheum. Dis. 76 (6), 1114–1122. doi: 10.1136/annrheumdis-2016-210131
Fernandez-Moreno, M., Soto-Hermida, A., Vazquez-Mosquera, M. E., Cortes-Pereira, E., Pertega, S., Relano, S., et al. (2017b). A replication study and meta-analysis of mitochondrial DNA variants in the radiographic progression of knee osteoarthritis. Rheumatology 56 (2), 263–270. doi: 10.1093/rheumatology/kew394
Fernandez-Tajes, J., Soto-Hermida, A., Vazquez-Mosquera, M. E., Cortes-Pereira, E., Mosquera, A., Fernandez-Moreno, M., et al. (2014). Genome-wide DNA methylation analysis of articular chondrocytes reveals a cluster of osteoarthritic patients. Ann. Rheum. Dis. 73 (4), 668–677. doi: 10.1136/annrheumdis-2012-202783
Garnero, P., Ayral, X., Rousseau, J. C., Christgau, S., Sandell, L. J., Dougados, M., et al. (2002). Uncoupling of type II collagen synthesis and degradation predicts progression of joint damage in patients with knee osteoarthritis. Arthritis Rheum. 46 (10), 2613–2624. doi: 10.1002/art.10576
Gavriilidis, C., Miwa, S., von Zglinicki, T., Taylor, R. W., Young, D. A. (2013). Mitochondrial dysfunction in osteoarthritis is associated with down-regulation of superoxide dismutase 2. Arthritis Rheum. 65 (2), 378–387. doi: 10.1002/art.37782
Gómez-Durán, A., Pacheu-Grau, D., López-Gallardo, E., Díez-Sánchez, C., Montoya, J., López-Pérez, M. J., et al. (2010). Unmasking the causes of multifactorial disorders: OXPHOS differences between mitochondrial haplogroups. Hum. Mol. Genet. 19 (17), 3343–3353. doi: 10.1093/hmg/ddq246
Hannan, M. T., Felson, D. T., Pincus, T. (2000). Analysis of the discordance between radiographic changes and knee pain in osteoarthritis of the knee. J. Rheumatol. 27 (6), 1513–1517.
Hashimoto, K., Otero, M., Imagawa, K., de Andrés, M. C., Coico, J. M., Roach, H. I., et al. (2013). Regulated transcription of human matrix metalloproteinase 13 (MMP13) and interleukin-1β (IL1B) genes in chondrocytes depends on methylation of specific proximal promoter CpG sites. J. Biol. Chem. 288 (14), 10061–10072. doi: 10.1074/jbc.m112.421156
Hellman, A., Chess, A. (2007). Gene body-specific methylation on the active X chromosome. Science 315 (5815), 1141–1143. doi: 10.1126/science.1136352
Henrotin, Y., Kurz, B. (2007). Antioxidant to treat osteoarthritis: dream or reality? Curr. Drug Targets 8 (2), 347–357. doi: 10.2174/138945007779940151
Horan, M. P., Cooper, D. N. (2014). The emergence of the mitochondrial genome as a partial regulator of nuclear function is providing new insights into the genetic mechanisms underlying age-related complex disease. Hum. Genet. 133 (4), 435–458. doi: 10.1007/s00439-013-1402-4
Hudson, G., Carelli, V., Spruijt, L., Gerards, M., Mowbray, C., Achilli, A., et al. (2007). Clinical expression of Leber hereditary optic neuropathy is affected by the mitochondrial DNA-haplogroup background. Am. J. Hum. Genet. 81 (2), 228–233. doi: 10.1086/519394
Hudson, G., Panoutsopoulou, K., Wilson, I., Southam, L., Rayner, N. W., Arden, N., et al. (2013). No evidence of an association between mitochondrial DNA variants and osteoarthritis in 7393 cases and 5122 controls. Ann. Rheum. Dis. 72 (1), 136–139. doi: 10.1136/annrheumdis-2012-201932
Hwang, H. S., Kim, H. A. (2015). Chondrocyte Apoptosis in the Pathogenesis of Osteoarthritis. Int. J. Mol. Sci. 16 (11), 26035–26054. doi: 10.3390/ijms161125943
Jazwinski, S. M. (2013). The retrograde response: when mitochondrial quality control is not enough. Biochim. Biophys. Acta 1833 (2), 400–409. doi: 10.1016/j.bbamcr.2012.02.010
Kellgren, J. H., Lawrence, J. S. (1957). Radiological assessment of osteo-arthrosis. Ann. Rheum. Dis. 16 (4), 494–502. doi: 10.1136/ard.16.4.494
Kelly, R. D., Rodda, A. E., Dickinson, A., Mahmud, A., Nefzger, C. M., Lee, W., et al. (2013). Mitochondrial DNA haplotypes define gene expression patterns in pluripotent and differentiating embryonic stem cells. Stem Cells 31 (4), 703–716. doi: 10.1002/stem.1313
Kenney, M. C., Chwa, M., Atilano, S. R., Falatoonzadeh, P., Ramirez, C., Malik, D., et al. (2014). Inherited mitochondrial DNA variants can affect complement, inflammation and apoptosis pathways: insights into mitochondrial-nuclear interactions. Hum. Mol. Genet. 23 (13), 3537–3551. doi: 10.1093/hmg/ddu065
Koo, B. S., Song, Y., Lee, S., Sung, Y. K., Shin, K. J., Cho, N. H., et al. (2019). Association of Asian mitochondrial DNA haplogroup B with new development of knee osteoarthritis in Koreans. Int. J. Rheum. Dis 22 (3), 411–416. doi: 10.1111/1756-185x.13453
Kraus, V. B., Blanco, F. J., Englund, M., Karsdal, M. A., Lohmander, L. S. (2015). Call for standardized definitions of osteoarthritis and risk stratification for clinical trials and clinical use. Osteoarthritis Cartilage 23 (8), 1233–1241. doi: 10.1016/j.joca.2015.03.036
Kuttapitiya, A., Assi, L., Laing, K., Hing, C., Mitchell, P., Whitley, G., et al. (2017). Microarray analysis of bone marrow lesions in osteoarthritis demonstrates upregulation of genes implicated in osteochondral turnover, neurogenesis and inflammation. Ann. Rheum. Dis. 76 (10), 1764–1773. doi: 10.1136/annrheumdis-2017-211396
López de Figueroa, P., Lotz, M. K., Blanco, F. J., Caramés, B. (2015). Autophagy activation and protection from mitochondrial dysfunction in human chondrocytes. Arthritis Rheumatol. 67 (4), 966–976. doi: 10.1002/art.39025
Latorre-Pellicer, A., Moreno-Loshuertos, R., Lechuga-Vieco, A. V., Sánchez-Cabo, F., Torroja, C., Acín-Pérez, R., et al. (2016). Mitochondrial and nuclear DNA matching shapes metabolism and healthy ageing. Nature 535 (7613), 561–565. doi: 10.1038/nature18618
Li, P., Ning, Y., Guo, X., Wen, Y., Cheng, B., Ma, M., et al. (2019). Integrating transcriptome-wide study and mRNA expression profiles yields novel insights into the biological mechanism of chondropathies. Arthritis Res. Ther. 21 (1), 194.
Li, H., Slone, J., Fei, L., Huang, T. (2019). Mitochondrial DNA variants and common diseases: a mathematical model for the diversity of age-related mtDNA Mutations. Cells 8 (6).
Lotz, M., Loeser, R. F. (2012). Effects of aging on articular cartilage homeostasis. Bone 51 (2), 241–248. doi: 10.1016/j.bone.2012.03.023
Maneiro, E., Martin, M. A., de Andres, M. C., Lopez-Armada, M. J., Fernandez-Sueiro, J. L., del Hoyo, P., et al. (2003). Mitochondrial respiratory activity is altered in osteoarthritic human articular chondrocytes. Arthritis Rheum. 48 (3), 700–708. doi: 10.1002/art.10837
Maneiro, E., López-Armada, M. J., de Andres, M. C., Caramés, B., Martín, M. A., Bonilla, A., et al. (2005). Effect of nitric oxide on mitochondrial respiratory activity of human articular chondrocytes. Ann. Rheum. Dis. 64 (3), 388–395. doi: 10.1136/ard.2004.022152
Marom, S., Friger, M., Mishmar, D. (2017). MtDNA meta-analysis reveals both phenotype specificity and allele heterogeneity: a model for differential association. Sci. Rep. 7, 43449. doi: 10.1038/srep43449
Martínez-Redondo, D., Marcuello, A., Casajús, J. A., Ara, I., Dahmani, Y., Montoya, J., et al. (2010). Human mitochondrial haplogroup H: the highest VO2max consumer–is it a paradox? Mitochondrion 10 (2), 102–107. doi: 10.1016/j.mito.2009.11.005
Maruszak, A., Canter, J. A., Styczynska, M., Zekanowski, C., Barcikowska, M. (2008). Mitochondrial haplogroup H and Alzheimer's disease-Is there a connection? Neurobiol. Aging 30 (11), 1749–1755. doi: 10.1016/j.neurobiolaging.2008.01.004
Matilainen, O., Quirós, P. M., Auwerx, J. (2017). Mitochondria and Epigenetics - crosstalk in homeostasis and stress. Trends Cell Biol. 27 (6), 453–463. doi: 10.1016/j.tcb.2017.02.004
Quirós, P. M., Mottis, A., Auwerx, J. (2016). Mitonuclear communication in homeostasis and stress. Nat. Rev. Mol. Cell Biol. 17 (4), 213–226. doi: 10.1038/nrm.2016.23
Rego, I., Fernandez-Moreno, M., Fernandez-Lopez, C., Gomez-Reino, J. J., Gonzalez, A., Arenas, J., et al. (2010). Role of European mitochondrial DNA haplogroups in the prevalence of hip osteoarthritis in Galicia, Northern Spain. Ann. Rheum. Dis. 69 (1), 210–213. doi: 10.1136/ard.2008.105254
Rego-Perez, I., Fernandez-Moreno, M., Fernandez-Lopez, C., Arenas, J., Blanco, F. J. (2008). Mitochondrial DNA haplogroups: role in the prevalence and severity of knee osteoarthritis. Arthritis Rheum. 58 (8), 2387–2396. doi: 10.1002/art.23659
Rego-Perez, I., Fernandez-Moreno, M., Deberg, M., Pertega, S., Fenandez-Lopez, C., Oreiro, N., et al. (2010). Mitochondrial DNA haplogroups modulate the serum levels of biomarkers in patients with osteoarthritis. Ann. Rheum. Dis. 69 (5), 910–917. doi: 10.1136/ard.2009.117416
Rego-Perez, I., Fernandez-Moreno, M., Deberg, M., Pertega, S., Fernandez-Lopez, C., Oreiro, N., et al. (2011). Mitochondrial DNA haplogroups and serum levels of proteolytic enzymes in patients with osteoarthritis. Ann. Rheum. Dis. 70 (4), 646–652. doi: 10.1136/ard.2010.133637
Rego-Perez, I., Blanco, F. J., Roemer, F. W., Guermazi, A., Ran, D., Ashbeck, E. L., et al. (2018). Mitochondrial DNA haplogroups associated with MRI-detected structural damage in early knee osteoarthritis. Osteoarthritis Cartilage 26 (11), 1562–1569. doi: 10.1016/j.joca.2018.06.016
Reynard, L. N., Loughlin, J. (2012). Genetics and epigenetics of osteoarthritis. Maturitas. 71 (3), 200–204. doi: 10.1016/j.maturitas.2011.12.001
Roach, H. I., Yamada, N., Cheung, K. S., Tilley, S., Clarke, N. M., Oreffo, R. O., et al. (2005). Association between the abnormal expression of matrix-degrading enzymes by human osteoarthritic chondrocytes and demethylation of specific CpG sites in the promoter regions. Arthritis Rheum. 52 (10), 3110–3124. doi: 10.1002/art.21300
Roemer, F. W., Guermazi, A., Collins, J. E., Losina, E., Nevitt, M. C., Lynch, J. A., et al. (2016). Semi-quantitative MRI biomarkers of knee osteoarthritis progression in the FNIH biomarkers consortium cohort - Methodologic aspects and definition of change. BMC Musculoskelet Disord. 17 (1), 466. doi: 10.1186/s12891-016-1310-6
Rousseau, J. C., Delmas, P. D. (2007). Biological markers in osteoarthritis. Nat Clin. Pract. Rheumatol. 3 (6), 346–356. doi: 10.1038/ncprheum0508
Ruiz-Pesini, E., Mishmar, D., Brandon, M., Procaccio, V., Wallace, D. C. (2004). Effects of purifying and adaptive selection on regional variation in human mtDNA. Science 303 (5655), 223–226. doi: 10.1126/science.1088434
Ruiz-Romero, C., Calamia, V., Mateos, J., Carreira, V., Martinez-Gomariz, M., Fernandez, M., et al. (2009). Mitochondrial dysregulation of osteoarthritic human articular chondrocytes analyzed by proteomics: a decrease in mitochondrial superoxide dismutase points to a redox imbalance. Mol. Cell Proteomics 8 (1), 172–189. doi: 10.1074/mcp.m800292-mcp200
Rushton, M., Reynard, L., Barter, M., Refaie, R., Rankin, K., Young, D., et al. (2014). Characterization of the cartilage DNA methylome in knee and hip osteoarthritis. Arthritis Rheum. 66 (9), 2450–2460. doi: 10.1002/art.38713
Schroeder, E. A., Raimundo, N., Shadel, G. S. (2013). Epigenetic silencing mediates mitochondria stress-induced longevity. Cell Metab. 17 (6), 954–964. doi: 10.1016/j.cmet.2013.04.003
Scotece, M., Rego-Perez, I., Lechuga Vieco, A. V., Filgueira-Fernández, P., Enriquez, J. A., Blanco, F. J. (2019). Mitochondrial background impact on the joint degeneration process during aging and forced exercise: a conplastic mouse model. Ann. Rheum. Dis. 78, p. A956. doi: 10.1136/annrheumdis-2019-eular.3201
Scott, J. L., Gabrielides, C., Davidson, R. K., Swingler, T. E., Clark, I. M., Wallis, G. A., et al. (2010). Superoxide dismutase downregulation in osteoarthritis progression and end-stage disease. Ann. Rheum. Dis. England, 69 (8), 1502–1510. doi: 10.1136/ard.2009.119966
Sellam, J., Berenbaum, F. (2013). Is osteoarthritis a metabolic disease? Joint Bone Spine 80 (6), 568–573. doi: 10.1016/j.jbspin.2013.09.007
Shadel, G. S., Horvath, T. L. (2015). Mitochondrial ROS signaling in organismal homeostasis. Cell 163 (3), 560–569. doi: 10.1016/j.cell.2015.10.001
Shen, J. M., Feng, L., Feng, C. (2014). Role of mtDNA haplogroups in the prevalence of osteoarthritis in different geographic populations: a meta-analysis. PLoS One 9 (10), e108896. doi: 10.1371/journal.pone.0108896
Soto-Hermida, A., Fernández-Moreno, M., Oreiro, N., Fernández-López, C., Rego-Pérez, I., Blanco, F. J. (2014a). mtDNA haplogroups and osteoarthritis in different geographic populations. Mitochondrion. 15, 18–23. doi: 10.1016/j.mito.2014.03.001
Soto-Hermida, A., Fernandez-Moreno, M., Oreiro, N., Fernandez-Lopez, C., Pertega, S., Cortes-Pereira, E., et al. (2014b). Mitochondrial DNA (mtDNA) haplogroups influence the progression of knee osteoarthritis. Data from the Osteoarthritis Initiative (OAI). PloS One 9 (11), e112735–e. doi: 10.1371/journal.pone.0112735
Soto-Hermida, A., Fernandez-Moreno, M., Pertega-Diaz, S., Oreiro, N., Fernandez-Lopez, C., Blanco, F. J., et al. (2015). Mitochondrial DNA haplogroups modulate the radiographic progression of Spanish patients with osteoarthritis. Rheum. Int. 35 (2), 337–344. doi: 10.1007/s00296-014-3104-1
Strauss, K. A., DuBiner, L., Simon, M., Zaragoza, M., Sengupta, P. P., Li, P., et al. (2013). Severity of cardiomyopathy associated with adenine nucleotide translocator-1 deficiency correlates with mtDNA haplogroup. Proc. Natl. Acad. Sci. U.S.A. 110 (9), 3453–3458. doi: 10.1073/pnas.1300690110
Tachmazidou, I., Hatzikotoulas, K., Southam, L., Esparza-Gordillo, J., Haberland, V., Zheng, J., et al. (2019). Identification of new therapeutic targets for osteoarthritis through genome-wide analyses of UK Biobank data. Nat. Genet. 51 (2), 230–236. doi: 10.1038/s41588-018-0327-1
Terkeltaub, R., Johnson, K., Murphy, A., Ghosh, S. (2002). Invited review: the mitochondrion in osteoarthritis. Mitochondrion. 1 (4), 301–319. doi: 10.1016/s1567-7249(01)00037-x
Torroni, A., Huoponen, K., Francalacci, P., Petrozzi, M., Morelli, L., Scozzari, R., et al. (1996). Classification of European mtDNAs from an analysis of three European populations. Genetics 144 (4), 1835–1850.
Vaamonde-García, C., Riveiro-Naveira, R. R., Valcárcel-Ares, M. N., Hermida-Carballo, L., Blanco, F. J., López-Armada, M. J. (2012). Mitochondrial dysfunction increases inflammatory responsiveness to cytokines in normal human chondrocytes. Arthritis Rheum. 64 (9), 2927–2936. doi: 10.1002/art.34508
Valdes, A. M., Goldring, M. B. (2017). Mitochondrial DNA haplogroups and ageing mechanisms in osteoarthritis. Ann. Rheum. Dis. 76 (6), 939–941. doi: 10.1136/annrheumdis-2016-210783
Wallace, D. C., Brown, M. D., Lott, M. T. (1999). Mitochondrial DNA variation in human evolution and disease. Gene 238 (1), 211–230. doi: 10.1016/s0378-1119(99)00295-4
Wallace, D. C. (2005). A mitochondrial paradigm of metabolic and degenerative diseases, aging, and cancer: a dawn for evolutionary medicine. Annu. Rev. Genet. 39, 359–407. doi: 10.1146/annurev.genet.39.110304.095751
Wallace, D. C. (2016). Genetics: mitochondrial DNA in evolution and disease. Nature 535 (7613), 498–500. doi: 10.1038/nature18902
Wang, Y., Zhao, X., Lotz, M., Terkeltaub, R., Liu-Bryan, R. (2015). Mitochondrial biogenesis is impaired in osteoarthritis chondrocytes but reversible via peroxisome proliferator-activated receptor γ coactivator 1. Arthritis Rheum. 67 (8), 2141–2153. doi: 10.1002/art.39182
Warner, S. C., Valdes, A. M. (2017). Genetic association studies in osteoarthritis: is it fairytale? Curr. Opin. Rheumatol. 29 (1), 103–109. doi: 10.1097/bor.0000000000000352
Keywords: mitochondria, genetics, epigenetics, osteoarthritis, methylation
Citation: Rego-Pérez I, Durán-Sotuela A, Ramos-Louro P and Blanco FJ (2020) Mitochondrial Genetics and Epigenetics in Osteoarthritis. Front. Genet. 10:1335. doi: 10.3389/fgene.2019.01335
Received: 25 September 2019; Accepted: 06 December 2019;
Published: 17 January 2020.
Edited by:
Joanna Rorbach, Karolinska Institutet (KI), SwedenReviewed by:
Claudia Calabrese, University of Cambridge, United KingdomIan Trounce, Centre for Eye Research Australia, Australia
Copyright © 2020 Rego-Pérez, Durán-Sotuela, Ramos-Louro and Blanco. This is an open-access article distributed under the terms of the Creative Commons Attribution License (CC BY). The use, distribution or reproduction in other forums is permitted, provided the original author(s) and the copyright owner(s) are credited and that the original publication in this journal is cited, in accordance with accepted academic practice. No use, distribution or reproduction is permitted which does not comply with these terms.
*Correspondence: Ignacio Rego-Pérez, aWduYWNpby5yZWdvLnBlcmV6QHNlcmdhcy5lcw==
†These authors have contributed equally to this work