- 1Sherbrooke Research and Development Centre, Agriculture and Agri-Food Canada, Sherbrooke, QC, Canada
- 2Department of Animal Science, Berry College, Mount Berry, GA, United States
- 3Department of Animal Breeding and Genetics, Federal University of Agriculture, Abeokuta, Abeokuta, Nigeria
- 4School of Life Sciences, University of Kwazulu-Natal, Durban, South Africa
The African continent is home to diverse populations of livestock breeds adapted to harsh environmental conditions with more than 70% under traditional systems of management. Animal productivity is less than optimal in most cases and is faced with numerous challenges including limited access to adequate nutrition and disease management, poor institutional capacities and lack of adequate government policies and funding to develop the livestock sector. Africa is home to about 1.3 billion people and with increasing demand for animal proteins by an ever growing human population, the current state of livestock productivity creates a significant yield gap for animal products. Although a greater section of the population, especially those living in rural areas depend largely on livestock for their livelihoods; the potential of the sector remains underutilized and therefore unable to contribute significantly to economic development and social wellbeing of the people. With current advances in livestock management practices, breeding technologies and health management, and with inclusion of all stakeholders, African livestock populations can be sustainably developed to close the animal protein gap that exists in the continent. In particular, advances in gene technologies, and application of genomic breeding in many Western countries has resulted in tremendous gains in traits like milk production with the potential that, implementation of genomic selection and other improved practices (nutrition, healthcare, etc.) can lead to rapid improvement in traits of economic importance in African livestock populations. The African livestock populations in the context of this review are limited to cattle, goat, pig, poultry, and sheep, which are mainly exploited for meat, milk, and eggs. This review examines the current state of livestock productivity in Africa, the main challenges faced by the sector, the role of various stakeholders and discusses in-depth strategies that can enable the application of genomic technologies for rapid improvement of livestock traits of economic importance.
Introduction
The African continent is home to diverse populations of livestock breeds adapted to their local environments in diverse agro-ecological zones. The diversity of the various cattle, sheep, goat, pig, and chicken breeds since their introduction or domestication has been shaped by a delicate balance between human and natural selection, and environmental adaptation. Livestock are central to the Africa society and economy and serve diverse roles such as: (1) source of food (provides meat and milk in the diet); (2) income generation through sale of meat, milk, and hide; (3) savings and insurance; (4) source of draft power and manure in crop production; (5) a means of transportation; (6) use in festivals and traditional ceremonies (marriage, birth, death, coronation, and initiation ceremonies) and, (7) source of power, pride, prestige, and status. Despite these benefits, livestock productivity is less than optimal, not sustainable and unable to match demand and population growth.
The Food and Agricultural Organization (FAO) estimates the total African population at 1.3 billion in 2017 (FAOSTATS, 2018) with rural and urban populations of 717 million and 505 million, respectively. Furthermore, the urban population has witnessed a steady annual increase of 3.59% since 2010, as compared to 1.74% annual rural population increase, and these increases are accompanied by increased demand for animal products. To meet this demand in the face of low productivity of livestock, African governments have increased imports of cattle meat from 482,111 tons in 2012 to 612,353 tons in 2016 and pig meat from 184,322 tons in 2012 to 252,611 tons in 2016 (Table 1). The populations of cattle, sheep, goat, pig, and chicken in the African continent and the various regions in 2016 are shown in Table 2. Similarly, the statistics on livestock productivity (meat, milk, and eggs) from 2010 are shown in Figure 1. To position the livestock sector to adequately contribute to food supply and economic development of the continent, measures must be taken to ensure sustainability in African livestock production systems which form part of FAO’s strategic objectives1. The livestock sector has the potential to enhance the livelihoods of Africa’s rural poor and genomic selection can play a key role. Given that the human population growth of the continent is higher than its food protein production, the need for targeted action to increase livestock productivity has never been greater, and genomic selection may play a significant role.
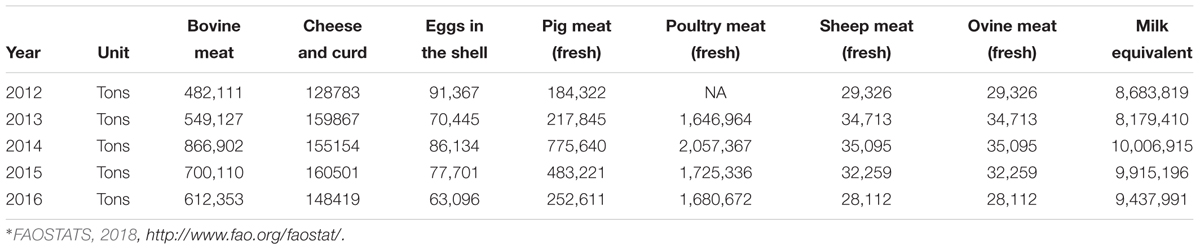
Table 1. Statistics∗ on major meat and livestock products imported by Africa in the period 2012–2016.
African Livestock Productivity in the Era of Genomic Breeding
State of Genomic Breeding Application in Western Countries
Genomic breeding in simple terms refers to the inclusion of deoxyribonucleic acid (DNA) or genomic information to select superior animals and make them parents of the next generation (Meuwissen et al., 2001, 2016). Thus, genomic selection simply is application of the knowledge of genetic variations found in the genome and their relationship with traits or phenotypes (e.g., milk yield, body weight, egg size, etc.) in selection for improved productivity (e.g., litter size, milk yield, etc.). The application of quantitative genetic theories, statistical approaches, artificial insemination and organized breeding practices resulted to rapid gains in livestock traits in the last nine decades (Blasco, 2013; Hill, 2014; Oldenbroek and Waaij, 2014; Weller, 2016). Mostly, the exact mechanisms behind these gains were not known but with the discovery of the DNA structure and developments in DNA sequencing and genotyping techniques, knowledge on the association between DNA variations and livestock traits began to emerge. Thus, with increasing demands for animal products by an ever growing population and changing societal needs, the animal breeding act needed to evolve to incorporate genomic information in order to speed up response and increase productivity.
Genomic breeding started with the application of marker assisted selection considering a few markers at a time and has evolved to the use of thousands of markers and even whole genome data (Hayes and Goddard, 2001; Hayes et al., 2013). Genomic selection entails the estimation of breeding values from markers spanning the entire genome. The estimation of marker effects is carried out within a reference population (a population of individuals with phenotype and marker genotype information). These effects are then applied to select candidates with marker genotype information without phenotypes to estimate genomic breeding value (GEBV). The reliability and accuracy of this approach depends on many factors including the number of individuals genotyped, the density of the markers on the genome, effective population size, the genetic relationship between the reference and predicted populations, the nature of the traits and the applied methods, etc. (Habier et al., 2007, 2011; Bolormaa et al., 2013a,b, 2014; Meuwissen et al., 2016). Genomic selection for milk and beef traits has been successfully implemented in several countries including Australia, Canada, France, Germany, Great Britain, Ireland, the Netherlands, New Zealand, the Scandinavian countries and the United States of America (Silva et al. (2014); Weller et al., 2017). Genomic breeding application in these countries is facilitated by many factors including: (1) large population of animals; (2) specialized farms; (3) comprehensive data on animals; (4) access to genotyping platforms which makes single nucleotide polymorphism (SNP) genotyping more cost effective; (5) available resources (finance, technical knowhow) to accomplish genotyping; (6) existence of breed associations; (7) application of artificial insemination; (8) development of breeds for specific purposes; (9) large scale international breeding companies that sell semen from high performing males for use in breeding for specific traits; (10) creation of farmer organizations, (11) implementation of national evaluation schemes; (12) development of statistical models to handle large data and (13) computing infrastructure to deliver genomics information which helps to facilitate genetic gains in livestock.
In addition, several initiatives have been undertaken to make available data on all sources of genomic variation in livestock genomes to further increase the success of genomic breeding. Such efforts include, but not limited to, the 1,000 bull genome project with aim to re-sequence the whole genomes of 1,000 bulls and has already made available about 84 million SNPs and 2.5 million small insertions/deletions (Hayes and Daetwyler, 2019) and the international consortium for Functional Annotation of Animal Genomes (FAANG2) established to provide the infrastructure to detect and proficiently analyze genome wide functional regulatory elements (DNA methylation, histone modifications, chromatin remodeling, non-coding RNA) in animal genomes (cattle, chicken, goat, pig, and sheep) necessary to understand how variation in gene sequences and functional components determines phenotypic diversity, and how this is translated into complex phenotypes; and thus fill the genotype-to-phenotype gap that is missing in current livestock improvement programs (Andersson et al., 2015; Tuggle et al., 2016). Major gains achieved with the use of genomic information and implementation of genomic selection include higher rate of genetic gain, increased reliability of predicting breeding values, higher intensity of selection, shortened generation interval, selection of animals possible at early age, and rapid genetic improvement in lowly heritable traits (e.g., fertility, lifespan, health, etc.) (reviewed by Hayes et al., 2009; Ibeagha-Awemu and Khatib, 2017; Weller et al., 2017; Mrode et al., 2018).
The application of genomic selection in Western countries and the advances that have been made in breeding (e.g., dairy traits) have been driven by the economic needs of the producers. However, challenges regarding sustainability of livestock production necessitate consideration of the economic, societal and environmental factors. A focus on increased milk production for example and intensive selection for this trait for several decades resulted in a deterioration of many traits like fertility, udder width/circumference and disease resistance (e.g., mastitis, metabolic diseases) traits, etc., and an increase in its ecological footprint (e.g., greenhouse gas emission) (Boichard and Brochard, 2012; Egger-Danner et al., 2015). These factors together with growing demand by consumers for animal safety warrant that successful programs for sustainable animal improvement should create a balance between selection for traits of economic value, animal health, conformation traits, adaptation traits, animal welfare and environmental foot-print.
The successes of genomic selection in Western countries mentioned above were possible through organized and sustained breeding practices supported by government regulations, finance and involvement of private companies. The picture for the majority of African countries is different given that, most livestock are kept for multi-purposes (meat, milk, traction, hides/wool, as a savings account, social status, cultural reasons, etc.), in small herds and flock sizes, under small scale to mid-scale low performing and low input systems, and lack of enabling government policies and financial support. Thus, procedures to increase livestock productivity in Africa in the era of genomic breeding must take into consideration the different production systems, ecological zones and participation of all stakeholders.
African Livestock Production Systems
In majority of African countries, livestock production is managed under small to large scale systems (Table 3). Small scale production systems include pastoral, agro-pastoral and mixed smallholder farming. Large scale systems include ranching, large scale commercial farming, cooperative farming and state owned farms. About 70% of livestock productivity occurs under the small scale systems characterized by small animal population sizes, low inputs and outputs, etc. Devising appropriate policies for such systems with the right government support is of utmost importance in increasing livestock productivity for food production and income generation. The characteristics of the various systems of livestock production are summarized in Table 3. Under predominantly small scale farming systems, it is important to determine whether or not such systems are ready for genomic breeding. Genomic breeding implementation relies on available genetic resources/diversity, and genomic variation and its association with desired traits.
African Livestock Genetic Resources, Diversity and Genomic Variation
Effective management of farm animal genetic resources requires adequate information on population size and structure, geographical distribution, the production environment, and within- and between-breed genetic diversity (Groeneveld et al., 2010). Assessment of diversity levels in breeds is necessary owing to husbandry systems which may affect diversity levels through inbreeding and high gene flow between breeds (Ibeagha-Awemu et al., 2004). Information on biodiversity is necessary for preparation of national action plan for improvement of animal genetic resources (Manirakiza et al., 2017). Meanwhile, a consideration for inclusion of genetic information in breed improvement requires knowledge of genomic variation and relationship with traits of interest.
Cattle
Africa is home to about 150 cattle breeds distributed across the continent, with the exception of the Sahara and the river Congo basin (Mwai et al., 2015), majority of which are uncharacterized (Nyamushamba et al., 2017). Various categories of cattle are present in the continent including zebu or Bos indicus breeds (African humped cattle), taurine or Bos taurus breeds (African humpless cattle), hybrids between humpless and humped cattle (e.g., sanga) and sanga and zebu backcross (e.g., zenga). The highest population of cattle and products from cattle are found in the East African region (Table 2 and Figure 1). Clear genetic divergence was revealed between B. taurus cattle and zebu breeds of West/Central Africa (Ibeagha-Awemu et al., 2004), and between South African indigenous and locally developed cattle breeds (Makina et al., 2014). However, the breed status of African cattle populations are in danger of disappearing rapidly following uncontrolled crossbreeding and breed replacements with exotic breeds (Ibeagha-Awemu et al., 2004; Mwai et al., 2015; Traoré et al., 2017).
Using microsatellite markers, candidate gene and genome wide approaches, genomic variation in some African cattle populations have been assessed and in some cases associated with production traits. Using 28 autosomal markers, Ibeagha-Awemu et al. (2004) revealed that zebu breeds in Cameroon and Nigeria are highly diverse as well as closely related. Whole genome SNP panel indicated close relationships between South African indigenous and locally developed cattle breeds (Makina et al., 2014) as well as pure and crossbred cattle in Burundi (Manirakiza et al., 2017). Genome characterization by sequencing of five indigenous African cattle breeds representatives of the cattle diversity of the continent [namely N’Dama (West African taurine), Ankole (African sanga cattle), Boran (East African zebu), Kenana (East African zebu), and Ogaden (East African zebu)] revealed a high number of SNPs in the breeds as well as breed specific SNPs (Kim et al., 2017). On a genome-wide window scale of 10 Mb, all indigenous African breeds had higher levels of nucleotide diversity compared to commercial European breeds (Angus, Jersey, and Holstein) which have been subjected to intensive artificial selection over generations (Kim et al., 2017). Genome wide characterization with Illumina BovineHD or BovineSNP50 Genotyping BeadChip of cattle breeds from East Africa, North Africa, South African, and West Africa revealed positional candidate positive selection regions which encompass genes and quantitative trait loci (QTL) for milk traits, reproduction and environmental stress (immunity and heat stress), candidate genes associated with biological pathways important for adaptation to marginal environments such as immunity, reproduction, development, and heat tolerance, copy number variations enriched for a number of biological processes, molecular functions and cellular components as well as potential to improve some of the breeds for dairy traits through breeding (Bahbahani et al., 2015, 2017, 2018; Pierce et al., 2018). Moreover, footprints of adaptive selection at the whole genome level (genotyping with 36,320 SNPs) were identified in nine West African cattle populations, including 53 genomic regions and 42 candidate genes enriched in physiological functions such as immune response, nervous system, and skin and hair properties (Gautier et al., 2009). From these data, high levels of genetic diversity is evident within African cattle populations which have been attributed to domestication, long history of migrations, selection and adaptation (Luikart et al., 2001; Groeneveld et al., 2010; Kim et al., 2017). Due to exposure to strong environmental pressures (hot, dry, or humid tropical climate conditions), diverse disease and nutritional challenges and water shortages, African livestock populations display unique adaptive traits (Table 4) which are necessary to support productivity and survivability in the different ecological zones.
Goat
The domestic goat, Capra hircus, is an important livestock species that is well suited to small-holder production systems throughout the entire African continent. Unique to West Africa is a great genetic diversity of goat types; the long-legged and trypano-susceptible types (e.g., Sahel and Red Sokoto goats) found in tsetse free areas and the trypano-tolerant type (West African Dwarf goat) found in the humid zone. According to Missohou et al. (2011), different ecotypes have emerged under varying selection pressures and diversified climate and topography in different countries. The largest goat populations are found in the Eastern and Western African regions (Table 2). Genetic diversity study on African goats is generally limited compared to other continents (Groeneveld et al., 2010). Microsatellite studies revealed a substantial amount of within breed diversity based on mean number of alleles observed (Muema et al., 2009; Missohou et al., 2011; Traore et al., 2012; Murital et al., 2015). Using genome-wide SNP data, Mdladla et al. (2016) reported high level of genetic diversity in South African indigenous goats including three locally developed meat type breeds of Boer, Savanna and Kalahari Red, a feral breed of Tankwa and unimproved non-descript village ecotypes. Some African goats have been characterized for polymorphisms in genes that control economically important traits (milk traits and litter size) (Bemji et al., 2006; Missohou et al., 2006; Caroli et al., 2007; Isa et al., 2017; Bemji et al., 2018), pointing to their potential application for genetic improvement for these traits.
Sheep
Diverse populations of sheep are found in the African continent with about 170 breeds of domestic sheep found in sub-Saharan Africa (Kemp et al., 2007). Present-day African sheep population is about 352 million (FAOSTATS, 2018) out of which ∼62% are found in Northern and Western Africa (Table 2). Investigations from different African countries based on microsatellite markers (Gaouar et al., 2015), mitochondrial DNA (Agaviezor et al., 2012; Brahi et al., 2015) and genome-wide SNP chip (Edea et al., 2017) revealed high within breed than between breed genetic diversity with clear evidence of admixture between breeds of sheep. The latter authors further observed that North African sheep breeds showed higher levels of within-breed diversity but were less differentiated than breeds from Eastern and Southern Africa, confirming previous reports that sheep from South Africa showed low to moderate genetic diversity (Qwabe et al., 2013). The initially domesticated sheep breeds in West Africa have also been genetically mixed with European breeds (Brahi et al., 2015). Using the OvineSNP50 beadchip, Molotsi et al. (2017) reported that the smallholder Dorper sheep was introgressed with Namaqua Afrikaner, South African Mutton Merino and White Dorpers genes. They further reported that the smallholder Dorper population was more genetically diverse than the pure-bred Dorper, South African Mutton Merino and Namaqua Afrikaner. Sheriff and Alemayehu (2018) reported low observed and expected heterozygosity in Ethiopian, Kenyan, South African and Nigerian sheep populations. They opined that the low heterozygosity may be due to the effect of small population sizes, inbreeding and minimal or null immigration of new genetic materials into the close populations. These data suggest close relationships and high levels of genetic admixture between African sheep breeds, especially among populations in the same geographic area.
Chicken
The domestic chicken with an estimated population of more than 1.9 billion in 2016 (FAOSTATS, 2018) is the most common and widespread domestic animal species kept mainly for food (meat and eggs) by resource poor farmers in Africa. Large-scale analyses involving microsatellite loci in domestic chickens, commercial lines and chickens sampled from the European region revealed high mean numbers of alleles and high degree of heterozygosity in Asian and African chickens as well as in Red Jungle fowl (Lyimo et al., 2014). Lower degree of population stratification as well as high within-breed genetic diversity in African chickens are supported by analyses with microsatellite markers (Muchadeyi et al., 2007; Adebambo et al., 2010; Mtileni et al., 2011), mtDNA (Wani et al., 2014; Hassaballah et al., 2015; Eltanany and Hemeda, 2016) and genome-wide SNP chips (Khanyile et al., 2015a,b; Fleming et al., 2016, 2017). Reduced genetic diversity was, however, witnessed with conservation flocks in South Africa which represented a limited sample of the gene pool (Muchadeyi et al., 2007; Mtileni et al., 2016). Increasing expansion of the commercial chicken industry and intermixing of commercial hybrids with local strains in rural backyards are eroding the genetic uniqueness of native breeds and their potential to adapt to local conditions (reviewed by Eltanany et al., 2011). Lawal et al. (2018) reported the use of whole-genome resequencing data of Red Jungle fowl and Indigenous Village Chicken populations from Ethiopia, Saudi Arabia, and Sri Lanka to decipher regions of the genome with functions relating to adaptation to temperature gradient, reproduction and immunity. All these results indicate the presence of genetic variation that can be utilized in genomic breeding.
Pig
The local African Pig is small in size and is likely the same breed in all African countries known under various names (African Union Inter-African Bureau for Animal Resources [AU-IBAR], 2015a), such as: Kolbroek (South Africa), Somo (Mali), Bakosi (Gabon and Cameroon), West African Dwarf pig (Nigeria), Ashanti Dwarf pig (Ghana), Bush pig (Togo), Mukota pig, or Zimbabwe Mukota pig (Zimbabwe). Despite cultural and religious influences in parts of the continent that limit pork production and consumption, pig farming is generally growing across West, East, Central and Southern Africa (African Union Inter-African Bureau for Animal Resources [AU-IBAR], 2015a) with the highest populations in Eastern and Western Africa (Table 2).
Findings based on joint analysis of mitochondrial, microsatellite and Y-chromosome polymorphisms in pigs and wild boars with a worldwide distribution revealed remarkably weak genetic differentiation between pigs and wild boars (Ramirez et al., 2009). This was attributed to a consequence of a sustained gene flow between both populations. More recent findings on pig populations indigenous to southern Africa based on different microsatellite loci (Halimani et al., 2012) similarly revealed lack of substructure in the pig populations, corroborating the general similarity in phenotypes commonly reported (Halimani et al., 2012). Sampled pigs in Ghana represented distinct populations with a moderate amount (12%) of genetic differentiation (Ayizanga et al., 2016). A study on the estimation of genetic parameters for growth performance and carcass traits in Mukota pigs in South Africa reported the presence of sufficient genetic variation that can support genetic improvement for many growth and carcass traits in the breed (Chimonyo and Dzama, 2007). Using the porcine genome wide SNP chip, Mujibi et al. (2018) observed a significant introgression of genes from international commercial breeds into Busia pigs from Busia county in Kenya. The authors also reported that pigs from Homabay county in Kenya are distinct from the international breeds and thus represent a local indigenous gene resource.
Considerations and Strategies for Implementation of Organized Genomic Breeding in Africa
For successful implementation of structured genomic breeding programs for African livestock populations, several factors deserve consideration as well as collective action and cooperation by all stakeholders (farmers, governments, research professionals, research organizations, universities, breed societies, private businesses, and support organizations) working together to achieve a common goal as illustrated in Figure 2.
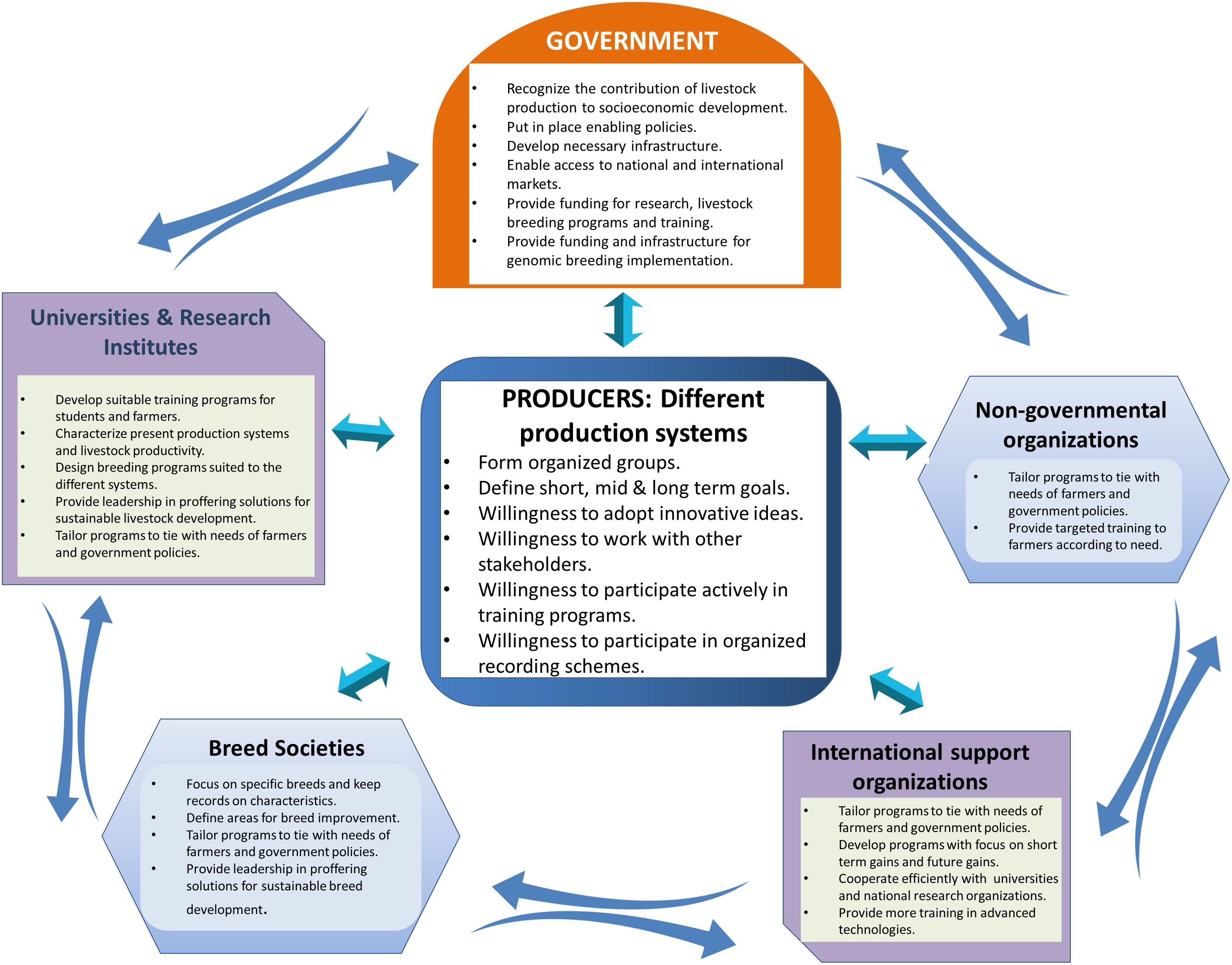
Figure 2. Different stakeholders that should be harnessed for successful livestock breeding programs in Africa.
Availability of Genetic Material for Breeding
As discussed above, the African continent is home to diverse livestock populations which also display rich genomic variations within and between breeds and have acquired special adaptive characteristics that support adaptation to poor quality feed, limited water supply, hot environments and disease (Psifidi et al., 2016; Mrode et al., 2018; Lawal et al., 2018). Some of these characteristics are summarized in Table 4. The indigenous breeds have acquired important characteristics for survival in their environments and in addition to being developed systematically, should be conserved for future survival and exploitation. Therefore, conservation of local breeds (highly utilized and less utilized) must be part of national breeding plans and should not be an exercise undertaken by individual farmers. Systematic breed development strategies including selection within breeds, controlled crossbreeding and upgrading programs and development of new breeds to exploit special adaptive and/or production traits must be done under organized systems with specific goals. This will address the practice of indiscriminate crossbreeding between local breeds and, between imported breeds and local breeds that is eroding the continent’s animal genetic diversity, a much needed resource for present and future exploitation.
Understand Production Systems, Production Potentials of Livestock and Needs of Farmers
There is still a lack of understanding about situating livestock development programs within prevailing low-input production systems, societal preferences and environmental conditions. Most international development programs have been based on ‘top-down approaches,’ considering single commodities and technology focused orientation with little or no participation of farmers nor formation of strong farmer-based institutions (Kaasschieter et al., 1992) and with no regard to prevailing environmental conditions. Majority of livestock are produced under the small holder system composed of pastoralists, agro-pastoralist and small holder farm families which have different breed preferences, inputs and challenges. Therefore, the prevailing production systems and livestock production potential under the different systems must be characterized and their specific needs identified in relation to local preferences and market needs, with the participation of producers. Data on productivity of local breeds under their prevailing conditions of production are largely unavailable. Such data is necessary as it will form the basis for improvement plans for each system. Indigenous African breeds are generally considered as underproductive without giving thought to the low-input and harsh environments in which they are raised. For example, under farmer management, the Butana and Kenana zebu breeds of Sudan produce averagely 538.26 and 598.73 kg of milk per lactation, respectively, while under research station conditions, they produce 1,400–2,100 kg of milk per lactation, respectively (Musa et al., 2005, 2006; Yousif and El- Moula, 2006). This implies that, although Butana and Kenana seem to produce less under low-input systems, they have the ability to produce more given improved conditions of nutrition, health care and production management. Another factor is the bias in judging local breeds based on parameters that have been selected and developed in exotic breeds. An example is the focus on lean specialized pig breeds like Large White at the expense of local relatively fat breeds that are well adapted to local environmental conditions. As such, policies are put in place that disfavor production of local pigs but encourage their replacement with exotic breeds (Chimonyo and Dzama, 2007).
Furthermore, emphasis has been placed on realizing quick gains by adopting ‘shortcut approaches’ like introduction of exotic genes that have been developed over a long period of time in different environments. This exotic stock does experience genotype by environment interaction which dramatically lowers performance in the local environment where they are introduced. African livestock have acquired the characteristics necessary to produce under their prevailing environmental conditions and on minimal resources. Before introducing exotic genes in a controlled manner, firstly, the productivity of local breeds must be assessed under optimal conditions (e.g., adequate feeding, housing, and disease management). The main limiting factors of local breed productivity could just be management and limited feed resources and disease control measures, as exemplified in Butana and Kenana cattle (Musa et al., 2005, 2006; Yousif and El- Moula, 2006). Under optimal management conditions, local breeds could be selected for desired traits in their prevailing environmental conditions. The adoption of most ‘shortcut approaches’ utilizing exotic genes has generally not resulted in substantial gains and sustainable long-term increases in productivity or contributed to poverty alleviation. Most donors or policy makers are only interested in immediate short term gains (visible) with the result of reckless crossbreeding of indigenous cattle resources with exotic breeds or their complete replacement with exotic breeds. These ‘short term gains’ are usually lost when such programs end and usually, the offspring of crossbred animals underperform under the prevailing conditions or lose the adaptive productive ability of the local breeds.
Breeding Goals
Setting a clear breeding goal is a prerequisite for animal improvement planning and implementation of genomic selection. A definition for animal breeding goals planning as a procedure with ethical priorities and weighing of market and non-market values has been suggested (Olesen et al., 2000). The decision to develop an animal for specific products has primarily been for the common interest of the farmer or the society or market demand. For example, increased market demand for milk and its products drove dairy breeding objectives in Western countries toward increased milk yield which unfortunately has resulted to problems of fertility and huge environmental footprints (Gill et al., 2010; Wall et al., 2010; Hayes et al., 2013; Knapp et al., 2014). Hence, sustainable animal breeding goals should consider market economic and non-market value traits, farmer specific needs, social, ecological and environmental needs supported by appropriate government policies, education, more cooperation between stakeholders and, short and long term needs, etc. The breeding goals must be adapted to fit each production system and environment. In recent times, breeding goals considering new phenotypes or non-traditional or production oriented traits and genetic traits of relevance to breeding sustainability have been proposed for cattle, sheep and pigs (Merks et al., 2012; Banga et al., 2014; Miglior et al., 2017; Molotsi et al., 2017). Recently, results of a survey of 160 farmers in southern Mali identified draft power and savings as the most important production objectives while preferred traits included fertility, draft ability and milk yield, in that order (Traoré et al., 2017).
Feed Resources and Animal Health
Optimal animal productivity is supported by adequate nutrition and disease management. Options for quantitative and qualitative improvements of the feed resources according to the needs of the different production systems are required for sustainable livestock systems (Duncan et al., 2013; Thornton and Herrero, 2015). For example, under the pastoral system, communal access to rotational grazing pastures and fodder banks which should be maintained to ensure quality of feed resources will support sustained livestock production. Legislations instituting the development of watersheds, restrictions on indiscriminate burning of grazing land and use of such land for other purposes are of necessity. Other vital aspects include development of improved pastures and fodders, increased grain production, development of agricultural bi-products as feed resources, access to water resources, etc. Although some of the local breeds have adapted to the disease burdens of their environments, disease is still a major limiting factor to livestock productivity in the region (De Garine-Wichatitsky et al., 2013; Okuni, 2013; Vanderburg et al., 2014). Particular attention should be paid to disease control measures like access to drugs, vaccines and veterinarians, and sound management practices developed for each system (Maclachlan and Mayo, 2013; Miguel et al., 2013).
Data Acquisition
Precise phenotypic data is crucial for genetic improvement. In Western countries, systems have been put in place to support high throughput phenotyping (e.g., milk yield, milk component yields, feed intake, etc.) thus enabling the accurate and consistent collection of large amounts of data on animal productivity. The formation of livestock trade databases is worthy of consideration since livestock movement contributes to the spread of animal and zoonotic diseases. In Western countries, this database is important for researchers to describe mobility patterns, optimize disease surveillance and control and predict possible epidemic scenarios (Apolloni et al., 2018). Therefore, it is necessary to sensitize producers on the importance of data collection and record keeping on the productivity of their animals, as well as formation of data storage facilities that can facilitate data storage and sharing within and between countries.
Infrastructure and Environmental Considerations
Besides the common issues with infrastructure for general development of the economy, infrastructural development to promote livestock production within the continent must be considered such as basic equipment for sample storage, data collection and data trace, livestock markets, slaughter facilities, animal housing and pasture development. With advances in genomics and other omics technologies, the livestock sector in several countries has moved to the area of big data research and application (VanderWaal et al., 2017; Morota et al., 2018; White et al., 2018). African livestock infrastructure must be developed to optimize the use of big data. Moreover, farmers need to be sensitized and prepared for adoption of these technologies. Technologies need to be adapted to farmers’ specific needs according to the system of production since there are differences in farmers’ access to farm resources, technological inputs and differences in access to output markets (Birhanu et al., 2017; Feder and Savastano, 2017). For instance, adapted dairy technologies varies widely among smallholders (Staal et al., 2002; Abdulai and Huffman, 2005; Amlaku et al., 2012) and also strongly affected by their social networks (Amlaku et al., 2012). The environmental impact is now a major concern for livestock production the world over due to its impact on greenhouse gas emissions and consequently climate change. The livestock sector in Africa also pose a challenge to the environment and climate change depending on the management and farming system. For instance, the semi-arid region is faced with the problem of overgrazing of rangelands which is caused by population pressure and a decline in traditional management systems (Fratkin, 2001; Ngongoni et al., 2006).
Development of National and Regional Policies and Priorities That Support Effective Production and Utilization of Livestock
The success of sustainable livestock development in any country or region hinges on development of national and or regional policies or guiding principles in the conduct of affairs. The decision by an international support organization or by a farmer group to import specific germplasm for crossbreeding with local breeds must be backed by national polices and priorities. Supply and demand policies favoring local production and supply chains will stimulate local production. Recognizing the important contribution of livestock production to the livelihood of farm families and to the nutrition and economy of the state/country necessitates a political commitment to stimulate, develop and financially sustain livestock development. The African Union has in place a Livestock Development Strategy (LiDeSa) for Africa (2015–2035) which was developed through an inclusive consultation process involving experts and stakeholders at national, regional, and continental levels (African Union Inter-African Bureau for Animal Resources AU-IBAR [AU-IBAR], 2015b). The strategy recognizes the central role played by livestock as a livelihood sustainer for rural Africa and with the support of a grant from the Bill and Melinda Gates foundation, seeks to transform the livestock sector by invigorating its untapped potentials. This is a laudable process that if implemented could truly transform lives. However, country level initiatives must follow suit for a transformed livestock sector to emerge in the continent. Today, the South African government is the only African government that is playing an active role in the conservation of animal genetic resources (Nyamushamba et al., 2017) and in supporting livestock breeding programs (Van Marle-Köster et al., 2017). Moreover, it is also important to take into account farmer’s preferences in the development of breeding polices (Wale and Yalew, 2007). Development of national policies and regional priorities should also focus on mitigation in the livestock sector due to the impact of climate change which varies with location. A program called climate-smart agriculture (CSA) has been implemented recently in the West African region and sub-Saharan Africa in general (Amole and Ayantunde, 2016). CSA is an approach that provides a conceptual basis for assessing the effectiveness of agricultural practice change to support food security under changing climatic conditions (Amole and Ayantunde, 2016).
Creation of Markets and Facilitation of Access to Markets
Appropriate economic incentives are important for livestock genetic improvement. Breeding programs should be market-oriented and the government should provide the right incentives. Several countries have made efforts on the extension of market access as well as to encourage foreign trade. For example, the Ethiopian government has completely strategized to encourage foreign trade for sheep and goat products which has led to the creation of employment opportunities for its citizens (Nwogwugwu et al., 2018) or the emergence of livestock feed market in Ghana (Konlan et al., 2018).
Education and Training, and Information Sharing
Education and training, and information sharing are vital aspects in sustainable livestock improvement breeding. The training curriculum in higher institutions should be adapted to fully address the needs of the various production systems. Formal training of students and informal training of the producers is vital. Greater cooperation between universities, research organizations (international, national, and regional), producer groups, non-governmental organizations and governments will ensure the flow and sharing of information and knowledge (Figure 2). The International Livestock Research Institute (ILRI) in Kenya has and continues to train students, research professionals and farm groups in various aspects of livestock breeding and production and molecular biology/genomics techniques. ILRI’s work in consultation with and tailored to meet the needs of farm families has resulted to initiatives like the Dairy Genetics East Africa project (DGEA), African Dairy Genetic Gains (ADGG), etc3. These programs were tailored to increase farmer productivity and profitability through the use of cross-bred animal types supported by extension and training systems tailored to their needs. The influence of ILRI amidst other successes led to rapid increase in cow milk production between 2011 and 2012 in the East African region (Figure 1). A national milk recording scheme has been instituted and supported by the government of Kenya4. The challenges faced by the program include limited number of breed inspectors, unawareness by many farmers of the importance of livestock registration, delay in issuance of livestock certificates and poor record keeping by farmers. Some of the suggested solutions include: training of more livestock inspectors by breed societies in conjunction with government, create farmer awareness using sensitization campaigns through mass media, exhibitions, shows, field days and direct consultations with interested farm groups, decentralization of services and investment in manpower and infrastructure. National animal production research institutes and universities in the various countries can emulate some of the practices of ILRI given that farmer’s participation in the development of projects tailored to their needs is a vital aspect in the successes of such programs.
Regional and continent wide sharing of information is vital for the sustainability of the livestock sector. The Forum for Agricultural Research in Africa5,6 , a technical arm of the African union, coordinates and advocates for agricultural research-for-development in the continent. Regular meetings of stake holders (professionals, farmers, students, and industry) interested in the act of animal production in forums like the All African Conference on Animal Agriculture, country and regional conferences on animal production all serve vital roles in the flow of information and technology advancements. However, producer focused meetings that provide informal training to farmers are generally lacking.
Application of Modern Genomic Breeding Technologies in African Livestock
Rapid improvement of African livestock productivity can benefit from current modern breeding technologies but many limitations abound. Some breeding programs that have been implemented for genetic improvement of livestock in Africa and the challenges faced are summarized in Table 5.
Livestock in the African continent are highly adapted to the prevailing environmental conditions characterized by heavy disease burden and marginal feed resources, but with marginal productivity because they are still largely unselected. African countries can benefit from genomic selection because it could be done even without pedigree information which is essential to traditional best linear unbiased prediction (BLUP)-EBV and the selection of candidates does not necessarily have to be based on trait records. The potential to generate GEBV using molecular information makes genomic selection a very attractive alternative to improving livestock in developing countries where adequate phenotypes and pedigree records are lacking. Genomic breeding has been reported to be more accurate than traditional BLUP because genomic relationships are more accurate than pedigree relationships (Meuwissen et al., 2016). Moreover, understanding of the fundamental genetic mechanisms influencing traits can be useful for setting up priors for (genetic) variances to increase the accuracy of genomic selection. Several successful approaches have been introduced such as BLUP| GA (BLUP-given genetic architecture; Zhang et al., 2014) or BayesRC (which adapted BayesR methods) incorporating prior biological information in the analysis by defining classes of variants likely to be enriched for causal mutations (MacLeod et al., 2016) or single step GBLUP with prior information (Fragomeni et al., 2017). These methods can be particularly useful for genomic selection in Africa with some prior biological knowledge of traits obtained from studies in the populations and other populations. Using genomic selection, Pitchford et al. (2017) concluded that heterozygosity effects were substantial for reproduction and growth in a tropically adapted composite beef program.
Our high enthusiasm about the potential application of genomic selection in African countries is immediately dampened with the reality that animals are held in small populations and in many small holder units. Furthermore, male animals that drive the genetic gain are often sold to generate income for farm families. These caveats can be overcome by the formation and practice of communal management and breeding systems.
Lack of phenotypes recorded in accurately defined contemporary groups is one of the constraints to the implementation of genomic selection in Africa and many developing countries (Burrow et al., 2017). Acquiring the genomic information for genomic selection is limited because genotyping is still expensive in many developing countries because incomes are very low compared to developed countries. The few studies on genomic selection in developing countries are characterized by small population sizes and validations were undertaken with test day data sets (Neves et al., 2014; Brown et al., 2016; Kariuki et al., 2017; Ducrocq et al., 2018; Mrode et al., 2019).
Traditional animal breeding requires the use of pedigree records to support selection decisions but most small holder farms in Africa do not have these types of records and the measure of relationships between animals are merely speculative. Furthermore, the application of genomic selection will require the use of reference populations which are generally lacking in Africa and many developing countries (Burrow et al., 2017). Mrode et al. (2019) reported the presence of small reference populations of between 500 and 3,000 animals (composed of mostly cows) in dairy and beef cattle in developing countries. The use of small reference populations that combined both bull and cow data, as in the case in Africa, has implications for the accuracy of genomic prediction, which is lower when compared to those obtained in Western countries given the limited information of the response variables when using cow records. It is important to state here that the inclusion of cows in the reference population has resulted to up to fivefold increase in the size of the reference population in some cases and increases of up to 12% in accuracy of selection compared to using bulls alone (Boison et al., 2017; Mrode et al., 2019). Mrode et al. (2018) reported some success by modeling and pooling data on the accuracy of genomic prediction in limited dairy data in East Africa. Brown et al. (2016) specifically reported the practice of genomic selection in a crossbred cattle population using data from the dairy genetic project of East Africa.
The cost of genotyping is a major issue limiting the adoption of genomic selection in Africa and to overcome this problem, the use of low density SNP panels have been suggested and this can be followed with imputation to improve the accuracy of genomic predictions (Meuwissen et al., 2016; Boison et al., 2017). Furthermore, low cost genome wide genotyping solution like genotyping-by-sequencing can generate high numbers of population specific SNPs (De Donato et al., 2013; Ibeagha-Awemu et al., 2016; Gurgul et al., 2018) that can support genomic selection in African livestock populations. Illumina7 and Affymetrix8 commercial SNP panels used for genotyping contains SNPs discovered in breeds and population of animals of Western origin and only very few breeds of African origin were included in the discovery of SNPs. This is the reason for ascertainment bias, which may affect accuracies of genomic selection from the use of commercially available SNP panels to genotype African indigenous livestock. Thus, the development of genotyping solutions specific for African breeds is necessary and the genotyping-by-sequencing approach can play a major role.
Some notable developments in the use of genomic tools include the sequencing of some indigenous cattle in Africa (Kim et al., 2017), developments on the genomic selection for disease resistance (Hanotte et al., 2010; Mwai et al., 2015) and for adaptation to hot arid condition (Kim et al., 2016). Other important efforts that may increase the quality of data includes the project of epidemiology of the Infectious Diseases of East African Livestock and a longitudinal calf cohort study in western Kenya (de Clare Bronsvoort et al., 2013) and strategies for bridging the gap between the developed and developing livestock sector (Van Marle-Koster and Visser, 2018). Recently, Canovas et al. (2017) discussed the application of new genomic technologies including transcriptomics, metagenomics, metabolomics, and epigenomics that are pertinent to speed-up genetic improvement of cattle. As a matter of priority, Burrow et al. (2017) suggested that research to improve grazing livestock should include cross-country genetic/genomic evaluations, use of sequence data in genetic evaluations, multi-breed genomic evaluations, selection index and genotype × environment interactions. Furthermore, numerous studies in Nellore, an indicine beef cattle breed suggests that genomic selection is a realistic alternative to traditional selection strategies (Neves et al., 2014). In small ruminants like sheep and goats, Mrode et al. (2018) observed that innovative genetic selection strategies will be needed to ensure adaptive balance between production and adaptation.
Emerging gene editing technologies like transcription activator-like effector nucleases (TALEN), zinc finger nucleases (ZFN), and clustered regularly interspaced short palindromic repeats (CRISPR)/Cas9 which can achieve any change in the genome, including introduction of alleles of interest into a recipient genome and switching on/off genes of interest can also play vital roles in rapid genomic improvement of African livestock traits. These tools offer an opportunity to intensify the frequency of desired alleles in a population through gene-edited individuals more rapidly than conventional breeding (Bhat et al., 2017). Genome editing in livestock has been reported for the double muscling gene in cattle, sheep, and pigs (Proudfoot et al., 2015; Qian et al., 2015), the polled allele introduction in dairy cattle (Tan et al., 2013; Carlson et al., 2016); gene edits that confer resistance to African Swine fever virus in pigs (Lillico et al., 2013; Whitworth et al., 2016) and the low-density lipoprotein receptor gene in a pig model of atherosclerosis (Carlson et al., 2012). These examples indicate that attempts at gene editing in livestock have targeted traits controlled by few variants with major effects. However, most livestock traits of economic importance are quantitatively controlled by many genes each contributing small effects, suggesting potential pitfalls in the implementation of these technologies for such traits. However, a recent simulation study indicated that editing for fewer casual variants of polygenic traits can double the rate of both short term and long term genetic gains when compared to conventional genomic selection (Jenko et al., 2015).
Challenges and Way Forward
As mentioned above, most countries in Africa lack functional breeding programs due to lack of involvement and engagement of farmers or producers and other stakeholders. Therefore, it is important to have long-term plans for breeding programs, which can meet present and anticipated future market needs (Zonabend et al., 2013). The major constraints to implementation of genomic breeding approaches for African livestock populations and the way forward have been discussed in Section “Considerations and Strategies for Implementation of Organized Genomic Breeding in Africa” and summarized in Table 6 and the major roles of each stakeholder are summarized in Figure 2.
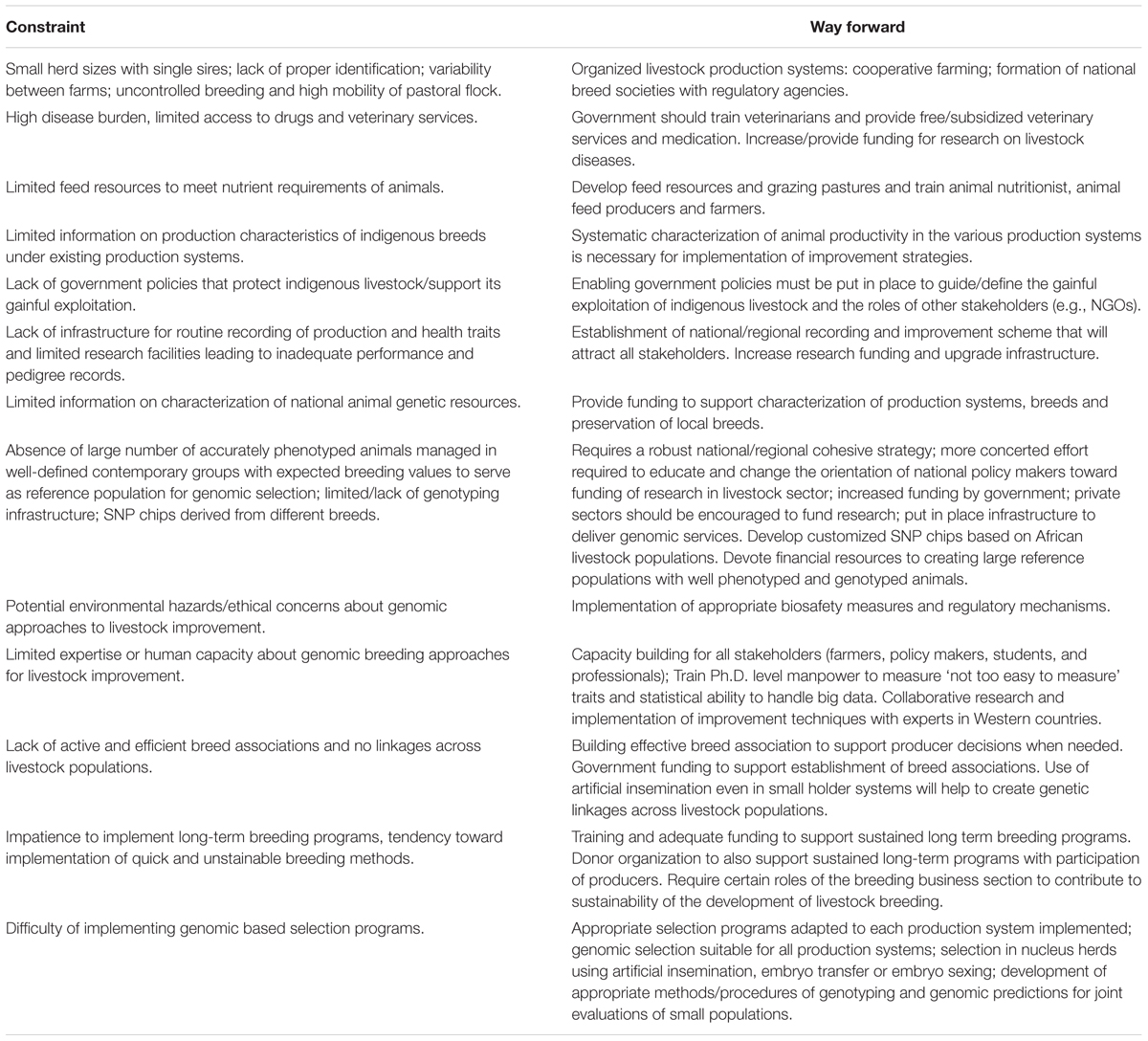
Table 6. Major concerns and possible solutions for development of improved livestock breeding programs in Africa.
For farmers/producers to play central roles in the success of any breeding program, they need support from different organizations such as (i) government (to put in place enabling polices, infrastructure, funding, incentives, and markets for their products), (ii) universities and research institutions (to guide, develop up-to-date curriculum, train and provide necessary information for breeding programs, setup and implementation), (iii) international organizations (funding and technological support), (iv) breed societies (maintain records and production characteristics for specific breeds, provide farmers with breed specific information and maintain purebreds). However, the producers themselves need to be actively involved in different breed associations as well as form farmer associations so they can work together to define their priorities (short, medium and long term goals) for implementation in breeding programs. For example; the South African government through its Technology Innovation Agency- TIA initiated a “Beef Genomics Program” in 2014 and a similar program for Dairy was started in 2016 with the goal of expanding to other species in the future (Burrow et al., 2017). Under this scheme, breed associations were expected to develop their own strategy with respect to use of genomic information. This type of approach can be replicated throughout Africa and most developing countries. Unfortunately, there is currently lack of leading roles by most African governments on issues related to livestock development.
The preferences of smallholder farmers is governed by their contextual household characteristics, institutional, and socioeconomic factors (Wale and Yalew, 2007) so their involvement in designing breeding programs is a must. In fact, community based breeding program (CBBP), which refers to improving livestock genetics with the incorporation of farmer participation in selection and breeding activities, has been successfully implemented for several breeds in different countries (Mueller et al., 2015). The CBBP place the farmer’s views, needs and decisions as the most important values and encourage them to participate through the life-cycle of the program from the interception to implementation. The CBBP also allow optimized use of genetic resources and genomic data to support breeding programs suited to specific regions (Kahi et al., 2005; Muniz et al., 2016).
Data collection and storage pose great challenges for African smallholders and even for commercial producers due to the nature of the farming systems (Table 3). At country levels, national improvement schemes to help farmers register and collect data on herd’s performance is scarce. A national milk recording scheme is operational in South Africa and Kenya. In Kenya, however, the willingness of farmers to register with the milk recording scheme and collect data on the productivity of their animals is low. The infrastructure for sample storage is also important for genetic materials. For example, DNA and biological samples need special procedures and materials for collection. The necessary infrastructure to carry out genetic improvement operations is severely constrained in Africa in general. Moreover, lack of baseline epidemiological data on the dynamics and impact of infectious cattle diseases in east Africa seriously limits animal improvement decisions (de Clare Bronsvoort et al., 2013). It is evident that the basic prerequisites for carrying out sample collection in livestock disease outbreaks is lacking for most African countries. It is worthy of note that the current animal health research focus on specific major infectious diseases, particularly tick-borne and tsetse-borne diseases, does not adequately address animal health issues because livestock in the continent are routinely exposed to a wide variety of pathogens. Therefore, the ability to determine correct pathogen effect is important for disease control and quality of data collection.
Most countries have recognized the importance of livestock breeding policy for direction of priorities and activities to be conducted in livestock breeding (Zonabend et al., 2013). However, questions regarding efficiency of implementation of policies and the frequency with which policies are updated to adapt to frequent changes in livestock breeding situations abound. Governments are not only required to draft policies but also to make sure that they are properly implemented. Governments are also required to create access to markets. However, many market problems exist for African countries such as lack of marketing facilities, inadequate marketing organization and methods, and inadequate government policies and marketing-facilitating services.
There is a chronic lack of skilled animal breeders in the African continent which limits the roles of research institutions and universities in designing breeding programs. Universities with Animal Breeding and Genetics programs need to update their curricula to reflect the current state of knowledge in animal breeding and genetics. Students need to be trained in statistics and on how to handle big data associated with advances in the application of knowledge of biotechnology to identify the best animals and make those the parents of the next generation. Also, lack of funding and promotion of research are limitations of African continent based researchers. Moreover, pressure to realize short-term benefits/outcome from research projects impacts negatively sustained gain that can accrue from effective long-term breeding programs. For certain traits, the breeding program needs a long time to realize gains or the impact is slowly accumulated through the years and it is hard to visualize, therefore the need for appropriate methods for measuring the success of breeding programs are required.
Non-governmental organizations (NGOs) are important stakeholders that contribute consultation services, support grass root livestock development programs and are vital partners in tailoring/implementing sustainable breeding programs. NGOs like Heifer Project International9, Vétérinaires sans Frontìeres Germany10, Send a Cow11, etc., have been supporting livestock development projects in the continent. However, greater cooperation between NGOs, international research organizations, national research organizations, universities and farmers will facilitate livestock development programs and widespread adoption of genomic breeding on the continent of Africa.
Conclusion
The African continent is home to diverse populations of livestock breeds that possess extremely valuable genetic materials but which are not utilized effectively to support economic development or to meet up with increasing demand. Owing to the rich genetic resources and availability of advanced breeding technologies, genomic breeding can be used to speedup livestock development on the continent of Africa. However, the promise and usefulness of genomic tools (especially genomic selection), which have supported livestock gains in many Western countries are yet to be implemented in most of Africa; the major constraints being lack of supportive government policies, funding, nutrition/health challenges, infrastructure and human knowhow. Thus, national governments need to recognize the contribution of livestock production to economic development and the wellbeing of citizens, and put in place enabling policies, necessary infrastructure and funding. Farmers must organize while universities and research institutions should tailor training to the needs of students and farmers. Furthermore, to design effective and sustainable livestock development programs, current production state of breeds and production systems must be adequately characterized through carefully designed investigations for production, reproduction, robustness and fitness traits, and all stakeholders must work together to achieve common goals. The notable success of the community based breeding program could be extended with the inclusion of genomic data as well as by better integration of other stakeholders and clearer government policies. Great opportunities for livestock development exist but all stakeholders must work together to leverage genetic resources for improvement of livestock breeding in Africa.
Author Contributions
EI-A conceptualized the review, followed by equal distribution of the different sections by EI-A, SP, MB, MA, and DD.
Funding
Funding was provided by Agriculture and Agri-Food Canada.
Conflict of Interest Statement
The authors declare that the research was conducted in the absence of any commercial or financial relationships that could be construed as a potential conflict of interest.
Footnotes
- ^ http://www.fao.org/3/MW154en/mw154en.pdf
- ^ www.faang.org
- ^ www.ilri.org
- ^ http://www.nafis.go.ke/livestock/livestock-registration/milk-recording/
- ^ https://faraafrica.org/
- ^ https://dgroups.org/fara-net
- ^ www.illumina.com/
- ^ www.affymetrix.com/
- ^ https://www.heifer.org/
- ^ http://www.vsfg.org/
- ^ https://www.sendacow.org/
References
Abdulai, A., and Huffman, W. E. (2005). The diffusion of new agricultural technologies: the case of crossbred-cow technology in Tanzania. Am. J. Agric. Econ. 87, 645–659.
Achukwi, M. D., Ibeagha-Awemu, E. M., Musongong, G. A., and Erhardt, G. (2009). Doayo (Namchi) Bos taurus cattle with low zebu attributes are trypanotolerant under natural vector challenge. Online J. Vet. Res. 13, 94–105.
Adebambo, A. O., Mobegi, V. A., Mwacharo, J. M., Oladejo, B. M., Adewale, R. A., Ilori, L. O., et al. (2010). Lack of phylogeographic structure in Nigerian village chickens revealed by mitochondrial DNA D-loop sequence analysis. Int. J. Poult. Sci. 9, 503–507.
Adebambo, O. A. (2001). The Muturu: a rare sacred breed of cattle in Nigeria. Anim. Genet. Resour. 31, 27–36.
African Union Inter-African Bureau for Animal Resources [AU-IBAR] (2015a). Local African Pig. Available at: www.au-ibar.org/component/jdownloads/finish/129-kya/2423-local-african-pig (accessed February 17, 2018).
African Union Inter-African Bureau for Animal Resources [AU-IBAR] (2015b). The Livestock Development Strategy for Africa 2015-2035. Nairobi: AU-IBAR.
Agaviezor, B. O., Adefenwa, M. A., Peters, S. O., Yakubu, A., Adebambo, O. A., Ozoje, M. O., et al. (2012). Genetic diversity analysis of the mitochondrial D-loop of Nigerian indigenous sheep. Anim. Genet. Resour. 50, 13–20.
Amlaku, A., Sölkner, J., Puskur, R., and Wurzinger, M. (2012). The impact of social networks on dairy technology adoption: evidence from Northwest Ethiopia. Int. J. Agrisci. 2, 1062–1083.
Amole, T. A., and Ayantunde, A. (2016). Climate-Smart Livestock Interventions in West Africa: a Review. CCAFS Working Paper No. 178. Copenhagen: CGIAR Research Program on Climate Change.
Andersson, L., Archibald, A. L., Bottema, C. D., Brauning, R., Burgess, S. C., Burt, D. W., et al. (2015). Coordinated international action to accelerate genome-to-phenome with FAANG, the Functional Annotation of Animal Genomes project. Genome Biol. Evol. 16:57. doi: 10.1186/s13059-015-0622-4
Apolloni, A., Nicolas, G., Coste, C., El Mamy, A. B., Yahya, B., El Arbi, A. S., et al. (2018). Towards the description of livestock mobility in Sahelian Africa: some results from a survey in Mauritania. PLoS One 13:e0191565. doi: 10.1371/journal.pone.0191565
Asselbergs, M., Jongejan, F., Langa, A., Neves, L., and Afonso, S. (1993). Antibodies to Cowdria ruminantium in Mozambican goats and cattle detected by immunofluorescence using endothelial cell culture antigen. Trop. Anim. Health Prod. 25, 144–150.
Ayizanga, R. A., Kayang, B. B., Adomako, K., Adenyo, C., Inoue-Murayama, M., and Asamoah, L. (2016). Genetic diversity of some Ghanaian pigs based on microsatellite markers. Livest. Res. Rural Dev. 28:24.
Bahbahani, H., Clifford, H., Wragg, D., Mbole-Kariuki, M. N., Van Tassell, C., Sonstegard, T., et al. (2015). Signatures of positive selection in East African Shorthorn Zebu: a genome-wide single nucleotide polymorphism analysis. Sci. Rep. 5:11729. doi: 10.1038/srep11729
Bahbahani, H., Salim, B., Almathen, F., Al Enezi, F., Mwacharo, J. M., and Hanotte, O. (2018). Signatures of positive selection in African Butana and Kenana dairy zebu cattle. PLoS One 13:e0190446. doi: 10.1371/journal.pone.0190446
Bahbahani, H., Tijjani, A., Mukasa, C., Wragg, D., Almathen, F., Nash, O., et al. (2017). Signatures of selection for environmental adaptation and zebu x taurine hybrid fitness in East African Shorthorn Zebu. Front. Genet. 8:68. doi: 10.3389/fgene.2017.00068
Banga, C. B., Neser, F. W. C., and Garrick, D. J. (2014). Breeding objectives for Holstein cattle in South Africa. S. Afr. J. Anim. Sci. 44, 199–214.
Bayemi, P. H., Nsongka, M. V., Leinyuy, I., Webb, E. C., Nchadji, J. M., Cavestany, D., et al. (2015). Effect of pre-partum feed supplementation on post-partum ovarian activity, milk production and calf growth of small holder dairy Cattle in Cameroon. Trop. Anim. Health Prod. 47, 153–158. doi: 10.1007/s11250-014-0700-8
Bemji, M. N., Ibeagha-Awemu, E. M., Osinowo, O. A., and Erhardt, G. (2006). Casein (CSN3) genetic variability of the Nigerian Red Sokoto goat. Niger. J. Genet. 20, 1–6.
Bemji, M. N., Isa, A. M., Ibeagha-Awemu, E. M., and Wheto, M. (2018). Polymorphisms of caprine GnRHR gene and their association with litter size in West African Dwarf goats. Mol. Biol. Rep. 45, 63–69. doi: 10.1007/s11033-017-4141-0
Bett, R. C., Kosgey, I. S., Kahi, A. K., and Peters, K. J. (2011). Definition of breeding objectives and optimum crossbreeding levels for goats in the smallholder production systems. Small Rumin. Res. 96, 16–24.
Bhat, S. A., Malik, A. A., Ahmad, S. M., Shah, R. A., Ganai, N. A., Shafi, S. S., et al. (2017). Advances in genome editing for improved animal breeding: a review. Vet. World 10, 1361–1366. doi: 10.14202/vetworld.2017.1361-1366
Birhanu, M. Y., Girma, A., and Puskur, R. (2017). Determinants of success and intensity of livestock feed technologies use in Ethiopia: evidence from a positive deviance perspective. Technol. Forecast. Soc. Change 115, 15–25.
Blasco, A. (2013). ”Animal breeding methods and sustainability animal breeding sustainability,” in Sustainable Food Production, eds P. Christou, R. Savin, B. A. Costa-Pierce, I. Misztal and C. B. A. Whitelaw (New York, NY: Springer), 41–57.
Boichard, D., and Brochard, M. (2012). New phenotypes for new breeding goals in dairy cattle. Animal 6, 544–550. doi: 10.1017/S1751731112000018
Boison, S. A., Utsunomiya, A. T. H., Santos, D. J. A., Neves, H. H. R., Carvalheiro, R., Mészáros, G., Utsunomiya, Y. T., et al. (2017). Accuracy of genomic predictions in Gyr (Bos indicus) dairy cattle. J. Dairy Sci. 100, 5479–5490. doi: 10.3168/jds.2016-11811
Bolormaa, S., Pryce, J. E., Kemper, K., Savin, K., Hayes, B. J., Barendse, W., et al. (2013a). Accuracy of prediction of genomic breeding values for residual feed intake and carcass and meat quality traits in Bos taurus, Bos indicus, and composite beef cattle. J. Anim. Sci. 91, 3088–3104. doi: 10.2527/jas.2012-5827
Bolormaa, S., Pryce, J. E., Kemper, K. E., Hayes, B. J., Zhang, Y., Tier, B., et al. (2013b). Detection of quantitative trait loci in Bos indicus and Bos taurus cattle using genome-wide association studies. Genet. Sel. Evol. 45:43. doi: 10.1186/1297-9686-45-43
Bolormaa, S., Pryce, J. E., Reverter, A., Zhang, Y., Barendse, W., Kemper, K., et al. (2014). A multi-trait, meta-analysis for detecting pleiotropic polymorphisms for stature, fatness and reproduction in beef cattle. PLoS Genet. 10:e1004198. doi: 10.1371/journal.pgen.1004198
Brahi, O. H., Xiang, H., Chen, X., Farougou, S., and Zhao, X. (2015). Mitogenome revealed multiple postdomestication genetic mixtures of West African sheep. J. Anim. Breed. Genet. 132, 399–405. doi: 10.1111/jbg.12144
Brown, A., Ojango, J., Gibson, J., Coffey, M., Okeyo, M., and Mrode, R. (2016). Genomic selection in a crossbred cattle population using data from the Dairy Genetics East Africa Project. J. Dairy Sci. 99, 7308–7312. doi: 10.3168/jds.2016-11083
Burrow, H. M., Wolcott, M. L., Maiwashe, A., Makgahlela, M. L., Hayes, B. J., Rees, J. G., and Bradfield, M. J. (2017). Can grazing livestock in developing countries benefit from use of genomic selection? Proc. Assoc. Advmt. Anim. Breed. Genet. 22, 353–360.
Canovas, A., Casellas, J., Thomas, M., and Medrano, J. F. (2017). Applying new genomic technologies to accelerate genetic improvement in beef and dairy cattle. Proc. Assoc. Advmt. Anim. Breed. Genet. 22, 377–383.
Carlson, D. F., Lancto, C. A., Zang, B., Kim, E. S., Walton, M., Oldeschulte, D., et al. (2016). Production of hornless dairy cattle from genome-edited cell lines. Nat. Biotechnol. 34, 479–481.
Carlson, D. F., Tan, W., Lillico, S. G., Stverakova, D., Proudfoot, C., Christian, M., et al. (2012). Efficient TALEN-mediated gene knockout in livestock. Proc. Natl. Acad. Sci. U.S.A. 109, 17382–17387. doi: 10.1073/pnas.1211446109
Caroli, A., Chiatti, F., Chessa, S., Rignanese, D., Ibeagha-Awemu, E. M., and Erhardt, G. (2007). Characterization of the casein gene complex in West African goats and description of a new alpha(s1)-casein polymorphism. J. Dairy Sci. 90, 2989–2996.
Catley, A., Lind, J., and Scoones, I., (2016). The futures of pastoralism in the Horn of Africa: pathways of growth and change. Rev. Sci. Tech. 35, 389–403. doi: 10.20506/rst.35.2.2524
Chimonyo, M., and Dzama, K. (2007). Estimation of genetic parameters for growth performance and carcass traits in Mukota pigs. Animal 1, 317–323. doi: 10.1017/S1751731107661849
DAGRIS (2007). Domestic Animal Genetic Resources Information System (DAGRIS). eds S. Kemp, Y. Mamo, B. Asrat, and T. Dessie (Addis Ababa: International Livestock Research Institute).
Dana, N., Van der Waaij, L. H., Dessie, T., and van Arendonk, J. A. (2010). Production objectives and trait preferences of village poultry producers of Ethiopia: implications for designing breeding schemes utilizing indigenous chicken genetic resources. Trop. Anim. Health Prod. 42, 1519–1529. doi: 10.1007/s11250-010-9602-6
Dayo, G. K., Gautier, M., Berthier, D., Poivey, J. P., and Sidibe, I., Bengaly, Z., et al. (2011). Association studies in QTL regions linked to bovine trypanotolerance in a West African crossbred population. Anim. Genet. 43, 123–132. doi: 10.1111/j.1365-2052.2011.02227.x
de Clare Bronsvoort, B. M., Thumbi, S. M., Poole, E. J., Kiara, H., Auguet, O. T., Handel, I. G., et al. (2013). Design and descriptive epidemiology of the Infectious Diseases of East African Livestock (IDEAL) project, a longitudinal calf cohort study in western Kenya. BMC Vet. Res. 9:171. doi: 10.1186/1746-6148-9-171
De Donato, M., Peters, S. O., Mitchell, S. E., Hussain, T., and Imumorin, I. G. (2013). Genotyping-by-sequencing (GBS): a novel, efficient and cost-effective genotyping method for cattle using next-generation sequencing. PLoS One 8:e62137. doi: 10.1371/journal.pone.0062137
De Garine-Wichatitsky, M., Caron, A., Kock, R., Tschopp, R., Munyeme, M., Hofmeyr, M., et al. (2013). A review of bovine tuberculosis at the wildlife–livestock–human interface in sub-Saharan Africa. Epidemiol. Infect. 141, 1342–1356. doi: 10.1017/S0950268813000708
Ducrocq, V., Denis, L., Marimuthu, S., Xavier, R., Michèle, T. B., and Tatiana, Z. (2018). Genomics for ruminants in developing countries: from principles to practice. Front. Genet. 9:251. doi: 10.3389/fgene.2018.00251
Duncan, A. J., Tarawali, S. A., Thorne, P., Valbuena, D., Descheemaeker, K., and Tui, S. H.-K. (2013). Integrated crop-livestock systems- a key to sustainable intensification in Africa. Trop. Grassl. Forrajes 1, 202–206.
Edea, Z., Dessie, T., Dadi, H., Do, K. T., and Kim, K. S. (2017). Genetic diversity and population structure of ethiopian sheep populations revealed by high-density SNP markers. Front. Genet. 8:218. doi: 10.3389/fgene.2017.00218
Egger-Danner, C., Cole, J. B., Pryce, J. E., Gengler, N., Heringstad, B., Bradley, A., et al. (2015). Invited review: overview of new traits and phenotyping strategies in dairy cattle with a focus on functional traits. Animal 9, 191–207. doi: 10.1017/S1751731114002614
Eltanany, M., Philipp, U., Weigend, S., and Distl, O. (2011). Genetic diversity of ten Egyptian chicken strains using 29 microsatellite markers. Anim. Genet. 42, 666–669. doi: 10.1111/j.1365-2052.2011.02185.x
Eltanany, M. A., and Hemeda, S. A. (2016). Deeper insight into maternal genetic assessments and demographic history for Egyptian indigenous chicken populations using mtDNA analysis. J. Adv. Res. 7, 615–623. doi: 10.1016/j.jare.2016.06.005
Etela, I., Bamikole, M. A., Ikhatua, U. J., and Kalio, G. A. (2008). Sweet potato and Green panic as sole fodder for stall-fed lactating White Fulani cows and growing calves. Trop. Anim. Health Prod. 40, 117–124.
FAOSTATS (2018). Food and Agricultural Organization Statistics for Year 2018. Available at: www.fao.org/faostat/ (accessed May 21, 2018).
Faye, D., Osaer, S., Goossens, B., Van Winghem, J., Dorny, P., Lejon, V., et al. (2002). Susceptibility of trypanotolerant West African Dwarf goats and F1 crosses with the susceptible Sahelian breed to experimental Trypanosoma congolense infection and interactions with helminth infections and different levels of diet. Vet. Parasitol. 108, 117–136.
Feder, G., and Savastano, S. (2017). “Modern 2 agricultural technology adoption in sub-Saharan Africa,” in Agriculture and Rural Development in a Globalizing World: Challenges and Opportunities, eds P. Pingali and G. Feder (London: Routledge), 11.
Fleming, D., Koltes, J., Markey, A., Schmidt, C., Ashwell, C., Rothschild, M., et al. (2016). Genomic analysis of Ugandan and Rwandan chicken ecotypes using a 600 k genotyping array. BMC Genomics 17:407. doi: 10.1186/s12864-016-2711-5
Fleming, D. S., Weigend, S., Simianer, H., Weigend, A., Rothschild, M., Schmidt, C., et al. (2017). Genomic comparison of indigenous African and Northern European chickens reveals putative mechanisms of stress tolerance related to environmental selection pressure. G3 7, 1525–1537. doi: 10.1534/g3.117.041228
Fragomeni, B. O., Lourenco, D. A. L., Masuda, Y., Legarra, A., and Misztal, I. (2017). Incorporation of causative quantitative trait nucleotides in single-step GBLUP. Genet. Sel. Evol. 49:59. doi: 10.1186/s12711-017-0335-0
Fratkin, E. (2001). East African pastoralism in transition: Maasai, Boran, and Rendille cases. Afr. Stud. Rev. 44, 1–25.
Gaouar, S. B., Da Silva, A., Ciani, E., Kdidi, S., Aouissat, M., Dhimi, L., et al. (2015). Admixture and local breed marginalization threaten Algerian sheep diversity. PLoS One 10:e0122667. doi: 10.1371/journal.pone.0122667
Gautier, M., Flori, L., Riebler, A., Jaffrezic, F., Laloe, D., Gut, I., et al. (2009). A whole genome Bayesian scan for adaptive genetic divergence in West African cattle. BMC Genomics 10:550. doi: 10.1186/1471-2164-10-550
Geerts, S., Osaer, S., Goossens, B., and Faye, D. (2009). Trypanotolerance in small ruminants of sub-Saharan Africa. Trends Parasitol. 25, 132–138. doi: 10.1016/j.pt.2008.12.004
Gill, M., Smith, P., and Wilkinson, J. (2010). Mitigating climate change: the role of domestic livestock. Animal 4, 323–333. doi: 10.1017/S1751731109004662
Gizaw, S., Arendonk, J. A. M., Valle-Zárate, A., Haile, A., Rischkowsky, B., Dessie, T., et al. (2014a). Breeding programmes for smallholder sheep farming systems: II. Optimization of cooperative village breeding schemes. Trop. Anim. Health Prod. 131, 350–357. doi: 10.1111/jbg.12102
Gizaw, S., Getachew, T., Goshme, S., Valle-Zárate, A., Van Arendonk, J. A. M., Kemp, S., et al. (2014b). Efficiency of selection for body weight in a cooperative village breeding program of Menz sheep under smallholder farming system. Animal 8, 1249–1254. doi: 10.1017/S1751731113002024
Groeneveld, L. F., Lenstra, J. A., Eding, H., Toro, M. A., Scherf, B., Pilling, D., et al. (2010). Genetic diversity in farm animals – a review. Anim. Genet. 41, 6–31. doi: 10.1111/j.1365-2052.2010.02038.x
Gurgul, A., Miksza-Cybulska, A., Szmatola, T., Jasielczuk, I., Piestrzynska-Kajtoch, A., Fornal, A., et al. (2018). Genotyping-by-sequencing performance in selected livestock species. Genomics 111, 186–195. doi: 10.1016/j.ygeno.2018.02.002
Habier, D., Fernando, R. L., and Dekkers, J. C. (2007). The impact of genetic relationship information on genome-assisted breeding values. Genetics 177, 2389–2397.
Habier, D., Fernando, R. L., Kizilkaya, K., and Garrick, D. J. (2011). Extension of the bayesian alphabet for genomic selection. BMC Bioinformatics 12:186. doi: 10.1186/1471-2105-12-186
Halimani, T. E., Muchadeyi, F. C., Chimonyo, M., and Dzama, K. (2012). Some insights into the phenotypic and genetic diversity of indigenous pigs in southern Africa. S. Afr. J. Anim. Sci. 42, 507–510.
Hanotte, O., Dessie, T., and Kemp, S. (2010). Time to tap Africa’s livestock genomes. Science 328, 1640–1641.
Hassaballah, K., Zeuh, V., Lawal, R. A., Hanotte, O., and Sembene, M. (2015). Diversity and origin of indigenous village chickens (Gallus gallus) from Chad, Central Africa. Adv. Biosci. Biotechnol. 6, 592–600.
Hayes, B., and Goddard, M. E. (2001). The distribution of the effects of genes affecting quantitative traits in livestock. Genet. Sel. Evol. 33, 209–229.
Hayes, B. J., Bowman, P. J., Chamberlain, A., and Goddard, M. (2009). Invited review: genomic selection in dairy cattle: progress and challenges. J. Dairy Sci. 92, 433–443. doi: 10.3168/jds.2008-1646
Hayes, B. J., and Daetwyler, H. D. (2019). 1000 bull genomes project to map simple and complex genetic traits in cattle: applications and outcomes. Annu. Rev. Anim. Biosci. 7, 89–102. doi: 10.1146/annurev-animal-020518-115024
Hayes, B. J., Lewin, H. A., and Goddard, M. E. (2013). The future of livestock breeding: genomic selection for efficiency, reduced emissions intensity, and adaptation. Trends Genet. 29, 206–214. doi: 10.1016/j.tig.2012.11.009
Hill, W. G. (2014). Applications of population genetics to animal breeding, from wright, fisher and lush to genomic prediction. Genetics 196, 1–16. doi: 10.1534/genetics.112.147850
Ibeagha-Awemu, E. M., Jann, O. C., Weimann, C., and Erhardt, G. (2004). Genetic diversity, introgression and relationships among West/Central African cattle breeds. Genet. Sel. Evol. 36, 673–690.
Ibeagha-Awemu, E. M., Peters, S. O., Akwanji, K. A., Imumorin, I. G., and Zhao, X. (2016). High density genome wide genotyping-by-sequencing and association identifies common and low frequency SNPs, and novel candidate genes influencing cow milk traits. Sci. Rep. 6:31109. doi: 10.1038/srep31109
Ibeagha-Awemu, E. M., and Khatib, H. (2017). “Epigenetics of livestock breeding,” in Handbook of Epigenetics. The New Molecular and Medical Genetics, 2nd Edn, ed. T. O. Tollefsbol (Cambridge, MA: Academic Press), 441–463.
Ibrahim, H. (1998). Small Ruminant Production Techniques. ILRI Manual 3. Nairobi: International Livestock Research Institute, 207.
Isa, A. M., Bemji, M. N., Wheto, M., Williams, T. J., and Ibeagha-Awemu, E. M. (2017). Mutations in inhibin alpha gene and their association with litter size in Kalahari Red and Nigerian goats. Livest. Sci. 203, 106–109.
Jenko, J., Gorjanc, G., Cleveland, M. A., Varshney, R. K., Whitelaw, C. B. A., Woolliams, J. A., et al. (2015). Potential of promotion of alleles by genome editing to improve quantitative traits in livestock breeding programs. Genet. Sel. Evol. 47:55. doi: 10.1186/s12711-015-0135-3
Kaasschieter, G. A., De Jong, R., Schiere, J. B., and Zwart, D. (1992). Towards a sustainable livestock production in developing countries and the importance of animal health strategy therein. Vet. Q. 14, 66–75.
Kahi, A., Rewe, T., and Kosgey, I. (2005). Sustainable community-based organizations for the genetic improvement of livestock in developing countries. Outlook Agric. 34, 261–270.
Kariuki, C. M., Brascamp, E. W., Komen, H., Kahi, A. K., and Van Arendonk, J. A. M. (2017). Economic evaluation of progeny-testing and genomic selection schemes for small-sized nucleus dairy cattle breeding programs in developing countries. J. Dairy Sci. 100, 2258–2268. doi: 10.3168/jds.2016-11816
Kemp, S., Mamo, Y., Asrat, B., Dessie, T. (eds). (2007). Domestic Animal Genetic Resources Information System. Addis Ababa: International Livestock Research Institute.
Kennedy, G. M. (2016). Diversity, Genetic Background and Hsp70 Gene Functional Polymorphisms for Heat Tolerance in Indigenous Chickens in Kenya. Master thesis, Jomo Kenyatta University of Agriculture and Technology, Juja.
Khanyile, K. S., Dzomba, E. F., and Muchadeyi, F. C. (2015a). Haplo-block structure of Southern African village chicken populations inferred using genome-wide SNP data. Genet. Mol. Res. 14, 12276–12287. doi: 10.4238/2015.October.9.16
Khanyile, K. S., Dzomba, E. F., and Muchadeyi, F. C. (2015b). Population genetic structure, linkage disequilibrium and effective population size of conserved and extensively raised village chicken populations of Southern Africa. Front. Genet. 6:13. doi: 10.3389/fgene.2015.00013
Kim, E.-S., Elbeltagy, A., Aboul-Naga, A., Rischkowsky, B., Sayre, B., Mwacharo, J., et al. (2016). Multiple genomic signatures of selection in goats and sheep indigenous to a hot arid environment. Heredity 116, 255–264. doi: 10.1038/hdy.2015.94
Kim, J., Hanotte, O., Mwai, O. A., Dessie, T., Bashir, S., Diallo, B., et al. (2017). The genome landscape of indigenous African cattle. Genome Biol. 18:34. doi: 10.1186/s13059-017-1153-y
Knapp, J., Laur, G., Vadas, P., Weiss, W., and Tricarico, J. (2014). Invited review: enteric methane in dairy cattle production: quantifying the opportunities and impact of reducing emissions. J. Dairy Sci. 97, 3231–3261. doi: 10.3168/jds.2013-7234
Konlan, S., Ayantunde, A. A., Addah, W., Dei, H., and Karbo, N. (2018). Emerging feed markets for ruminant production in urban and peri-urban areas of Northern Ghana. Trop. Anim. Health Prod. 50, 169–176. doi: 10.1007/s11250-017-1418-1
Kosgey, I. S., Baker, R. L., Udo, H. M. J., and Van Arendonk J. A. M. (2006). Successes and failures of small ruminant breeding programmes in the tropics: a review. Small Rumin. Res. 61, 13–28.
Lawal, R. A., Al-Atiyat, R. M., Aljumaah, R. S., Silva, P., Mwacharo, J. M., and Hanotte, O. (2018). Whole-genome resequencing of Red Junglefowl and Indigenous Village Chicken reveal new insights on the genome dynamics of the species. Front. Genet. 9:264. doi: 10.3389/fgene.2018.00264
Lemecha, H., Mulatu, W., Hussein, I., Rege, E., Tekle, T., Abdicho, S., et al. (2006). Response of four indigenous cattle breeds to natural tsetse and trypanosomosis challenge in the Ghibe valley of Ethiopia. Vet. Parasitol. 141, 165–176.
Lillico, S. G., Proudfoot, C., Carlson, D. F., Stverakova, D., Neil, C., Blain, C., et al. (2013). Live pigs produced from genome edited zygotes. Sci. Rep. 3:2847. doi: 10.1038/srep02847
Luikart, G., Gielly, L., Excoffier, L., Vigne, J. D., Bouvet, J., and Taberlet, P. (2001). Multiple maternal origins and weak phylogeographic structure in domestic goats. Proc. Natl. Acad. Sci. U.S.A. 98, 5927–5932.
Lyimo, C. M., Weigend, A., Msoffe, P. L., Eding, H., Simianer, H., and Weigend, S. (2014). Global diversity and genetic contributions of chicken populations from African, Asian and European regions. Anim. Genet. 45, 836–848. doi: 10.1111/age.12230
Maclachlan, N. J., and Mayo, C. E. (2013). Potential strategies for control of bluetongue, a globally emerging, Culicoides-transmitted viral disease of ruminant livestock and wildlife. Antiviral Res. 99, 79–90. doi: 10.1016/j.antiviral.2013.04.021
MacLeod, I. M., Bowman, P. J., Vander Jagt, C. J., Haile-Mariam, M., Kemper, K. E., Chamberlain, A. J. et al. (2016). Exploiting biological priors and sequence variants enhances QTL discovery and genomic prediction of complex traits. BMC Genomics 17:144. doi: 10.1186/s12864-016-2443-6
Madzimure, J., Chimonyo, M., Zander, K. K., and Dzama, K. (2013). Potential for using indigenous pigs in subsistence-oriented and market-oriented small-scale farming systems of Southern Africa. Trop. Anim. Health Prod. 45, 135–142.
Mahoro, J., Muasya, T. K., Mbuza, F., Mbuthia, J., and Kahi, A. K. (2018). Farmers’ breeding practices and traits of economic importance for indigenous chicken in RWANDA. Trop. Anim. Health Prod. 50, 121–128. doi: 10.1007/s11250-017-1411-8
Maichomo, M. W., Ndungu, J. M., Ngare, P., and Ole-Mapenay, I. M. (2005). The performance of Orma Boran and Maasai Zebu crossbreeds in a trypanosomosis endemic area of Nguruman, south western Kenya. Onderstepoort J. Vet. Res. 72, 87–93.
Majekodunmi, A. O., Dongkum, C., Langs, T., Shaw, A. and Welburn, S. (2016). Improved productivity and sustainable pastoral systems in an era of insecurity - Fulani herds of the southern Jos Plateau, North-Central Nigeria. Trop. Anim. Health Prod. 48, 1719–1728.
Makina, S. O., Muchadeyi, F. C., Van Marle-Koster, E., Macneil, M. D., and Maiwashe, A. (2014). Genetic diversity and population structure among six cattle breeds in South Africa using a whole genome SNP panel. Front. Genet. 5:333. doi: 10.3389/fgene.2014.00333
Mamoudou, A., Njanloga, A., Hayatou, A., Suh, P. F., and Achukwi, M. D. (2016). Animal trypanosomosis in clinically healthy cattle of north Cameroon: epidemiological implications. Parasit. Vectors 9:206. doi: 10.1186/s13071-016-1498-1
Manirakiza, J., Hatungumukama, G., Thevenon, S., Gautier, M., Besbes, B., Flori, L., et al. (2017). Effect of genetic European taurine ancestry on milk yield of Ankole-Holstein crossbred dairy cattle in mixed smallholders system of Burundi highlands. Anim. Genet. 48, 544–550. doi: 10.1111/age.12578
Mattioli, R. C., Pandey, V. S., Murray, M., and Fitzpatrick, J. L. (2000). Immunogenetic influences on tick resistance in African cattle with particular reference to trypanotolerant N’Dama (Bos taurus) and trypanosusceptible Gobra zebu (Bos indicus) cattle. Acta Trop. 75, 263–277.
Mdladla, K., Dzomba, E. F., Huson, H. J., and Muchadeyi, F. C. (2016). Population genomic structure and linkage disequilibrium analysis of South African goat breeds using genome-wide SNP data. Anim. Genet. 47, 471–482. doi: 10.1111/age.12442
Merks, J. W., Mathur, P. K., and Knol, E. F. (2012). New phenotypes for new breeding goals in pigs. Animal 6, 535–543. doi: 10.1017/S1751731111002266
Meuwissen, T., Hayes, B., and Goddard, M. (2016). Genomic selection: a paradigm shift in animal breeding. Anim. Front. 6, 6–14.
Meuwissen, T. H., Hayes, B. J., and Goddard, M. E. (2001). Prediction of total genetic value using genome-wide dense marker maps. Genetics 157, 1819–1829.
Miglior, F., Fleming, A., Malchiodi, F., Brito, L. F., Martin, P., and Baes, C. F. (2017). A 100-Year Review: identification and genetic selection of economically important traits in dairy cattle. J. Dairy Sci. 100, 10251–10271. doi: 10.3168/jds.2017-12968
Miguel, E., Grosbois, V., Caron, A., Boulinier, T., Fritz, H., Cornélis, D., et al. (2013). Contacts and foot and mouth disease transmission from wild to domestic bovines in Africa. Ecosphere 4, 1–32.
Missohou, A., Poutya, M. R., Nenonene, A., Dayo, G. K., Ayssiwede, S. B., Talaki, E., Issa, Y., et al. (2011). Genetic diversity and differentiation in nine West African local goat breeds assessed via microsatellite polymorphism. Small Rumin. Res. 99, 20–24.
Missohou, A., Talaki, E., and Laminou, I. M. (2006). Diversity and genetic relationships among seven West African goat breeds. Asian Australas. J. Anim. Sci. 19, 1245–1251.
Molotsi, A., Dube, B., Oosting, S., Marandure, T., Mapiye, C., Cloete, S., et al. (2017). Genetic traits of relevance to sustainability of smallholder sheep farming systems in South Africa. Sustainability 9:1225.
Morota, G., Ventura, R., Silva, F., Koyama, M., and Fernando, S. (2018). Machine learning and data mining advance predictive big data analysis in precision animal agriculture. J. Anim. Sci. 96, 1540–1550. doi: 10.1093/jas/sky014
Mrode, R., Ojango, J. M. K., Okeyo, A. M., and Nwacharo, J. M., (2019). Genomic selection and use of molecular tools in breeding programs for indigenous crossbred cattle in developing countries: current status and future prospects. Front. Genet. 9:694. doi: 10.3389/fgene.2018.00694
Mrode, R., Tarekegn, G., Mwacharo, J., and Djikeng, A. (2018). Invited review: genomic selection for small ruminants in developed countries: how applicable for the rest of the world? Animal 12, 1333–1340. doi: 10.1017/S1751731117003688
Mtileni, B., Dzama, K., Nephawe, K., and Rhode, C. (2016). Estimates of effective population size and inbreeding in South African indigenous chicken populations: implications for the conservation of unique genetic resources. Trop. Anim. Health Prod. 48, 943–950. doi: 10.1007/s11250-016-1030-9
Mtileni, B. J., Muchadeyi, F. C., Maiwashe, A., Chimonyo, M., Groeneveld, E., Weigend, S., et al. (2011). Diversity and origin of South African chickens. Poult. Sci. 90, 2189–2194. doi: 10.3382/ps.2011-01505
Muchadeyi, F. C., Eding, H., Wollny, C. B., Groeneveld, E., Makuza, S. M., Shamseldin, R., et al. (2007). Absence of population substructuring in Zimbabwe chicken ecotypes inferred using microsatellite analysis. Anim. Genet. 38, 332–339.
Mueller, J., Rischkowsky, B., Haile, A., Philipsson, J., Mwai, O., Besbes, B., et al. (2015). Community-based livestock breeding programmes: essentials and examples. J. Anim. Breed. Genet. 132, 155–168. doi: 10.1111/jbg.12136
Muema, E. K., Wakhungu, J. W., Hannotte, O., and Jianlin, H. (2009). Genetic diversity and relationship of indigenous goats of Sub-Saharan Africa using microsatellite DNA markers. Livest. Res. Rural Dev. 21:28.
Mujibi, F. D., Okoth, E., Cheruiyot, E. K., Onzere, C., Bishop, R. P., Fèvre, E. M., et al. (2018). Genetic diversity, breed composition and admixture of Kenyan domestic pigs. PLoS One 13:e0190080. doi: 10.1371/journal.pone.0190080
Muniz, M. M. M., Caetano, A. R., Mcmanus, C., Cavalcanti, L. C. G., Façanha, D. A. E., Leite, J. H. G. M., et al. (2016). Application of genomic data to assist a community-based breeding program: a preliminary study of coat color genetics in Morada Nova sheep. Livest. Sci. 190, 89–93.
Murital, I., Afolayan, O., Bemji, M. N., Dadi, O., Landi, V., Martínez, A., et al. (2015). Genetic diversity and population structure of Nigerian indigenous goat using DNA microsatellite markers. Arch. Zootec. 64, 93–98.
Musa, L. M.-A., Ahmed, M.-K. A., Peters, K. J., Zumbach, B., and Gubartalla, K. E. A. (2005). The reproductive and milk performance merit of Butana cattle in Sudan. Arch. Anim. Breed. 48, 445–495.
Musa, L. M.-A., Peters, K. J., and Ahmed, M.-K. A. (2006). On farm characterization of Butana and Kenana cattle breed production systems in Sudan. Livest. Res. Rural Dev. 18:117.
Mwai, O., Hanotte, O., Kwon, Y.-J., and Cho, S. (2015). African indigenous cattle: unique genetic resources in a rapidly changing world. Asian Australas. J. Anim. Sci. 28, 911–921. doi: 10.5713/ajas.15.0002R
Neves, H. H., Carvalheiro, R., O’brien, A. M., Utsunomiya, Y. T., Do Carmo, A. S., Schenkel, F. S., et al. (2014). Accuracy of genomic predictions in Bos indicus (Nellore) cattle. Genet. Sel. Evol. 46:17. doi: 10.1186/1297-9686-46-17
Ngongoni, N., Mapiye, C., Mwale, M., and Mupeta, B. (2006). Factors affecting milk production in the smallholder dairy sector of Zimbabwe. Livest. Res. Rural Dev. 18, 1–21.
Njogu, A. R., Dolan, R. B., Wilson, A. J., and Sayer, P. D. (1985). Trypanotolerance in East African Orma Boran cattle. Vet. Rec. 117, 632–636.
Nwogwugwu, C. P., Lee, S.-H., Freedom, E. C., Manjula, P., and Lee, J. H. (2018). Review on challenges, opportunities and genetic improvement of sheep and goat productivity in Ethiopia. J. Anim. Breed. Genomics 2, 1–8.
Nyamushamba, G. B., Mapiye, C., Tada, O., Halimani, T. E., and Muchenje, V. (2017). Conservation of indigenous cattle genetic resources in Southern Africa’s smallholder areas: turning threats into opportunities—A review. Asian Australas. J. Anim. Sci. 30, 603–621. doi: 10.5713/ajas.16.0024
O’Gorman, G. M., Park, S. D., Hill, E. W., Meade, K. G., Coussens, P. M., Agaba, M., et al. (2009). Transcriptional profiling of cattle infected with Trypanosoma congolense highlights gene expression signatures underlying trypanotolerance and trypanosusceptibility. BMC Genomics 10:207. doi: 10.1186/1471-2164-10-207
Okuni, J. B. (2013). Occurrence of paratuberculosis in African countries: a review. J. Vet. Sci 3, 1–8.
Olesen, I., Groen, A. F., and Gjerde, B. (2000). Definition of animal breeding goals for sustainable production systems. J. Anim. Sci. 78, 570–582.
Onteru, S. K., Ampaire, A., and Rothschild, M. F. (2010). Biotechnology developments in the livestock sector in developing countries. Biotechnol. Genet. Eng. Rev. 27, 217–228.
Osei-Amponsah, R., Skinner, B. M., Adjei, D. O., Bauer, J., Larson, G., Affara, N. A., et al. (2017). Origin and phylogenetic status of the local Ashanti Dwarf pig (ADP) of Ghana based on genetic analysis. BMC Genomics 18:193. doi: 10.1186/s12864-017-3536-6
Pierce, M. D., Dzama, K., and Muchadeyi, F. C. (2018). Genetic diversity of seven cattle breeds inferred using copy number variations. Front. Genet. 9:163. doi: 10.3389/fgene.2018.00163
Pitchford, W. S., Popplewell, G. I., and Terle, R. (2017). Use of genomic selection in a tropically adapted composite beef program. Proc. Assoc. Advmt. Anim. Breed. Genet. 22, 179–182.
Proudfoot, C., Carlson, D. F., Huddart, R., Long, C. R., Pryor, J. H., King, T. J., et al. (2015). Genome edited sheep and cattle. Transgenic Res. 24, 147–153. doi: 10.1007/s11248-014-9832-x
Psifidi, A., Fife, M., Howell, J., Matika, O., Van Diemen, P. M., Kuo, R., et al. (2016). The genomic architecture of resistance to Campylobacter jejuni intestinal colonisation in chickens. BMC Genomics 17:293. doi: 10.1186/s12864-016-2612-7
Pullan, N. B., and Grindle, R. J. (1980). Productivity of white Fulani cattle on the Jos plateau, Nigeria. IV. Economic factors. Trop. Anim. Health Prod. 12, 161–170.
Qian, L., Tang, M., Yang, J., Wang, Q., Cai, C., Jiang, S., et al. (2015). Targeted mutations in myostatin by zinc-finger nucleases result in double-muscled phenotype in Meishan pigs. Sci. Rep. 5:14435. doi: 10.1038/srep14435
Qwabe, S. O., Van Marle-Koster, E., and Visser, C. (2013). Genetic diversity and population structure of the endangered Namaqua Afrikaner sheep. Trop. Anim. Health Prod. 45, 511–516. doi: 10.1007/s11250-012-0250-x
Ramirez, O., Ojeda, A., Tomas, A., Gallardo, D., Huang, L. S., Folch, J. M., et al. (2009). Integrating Y-chromosome, mitochondrial, and autosomal data to analyze the origin of pig breeds. Mol. Biol. Evol. 26, 2061–2072. doi: 10.1093/molbev/msp118
Scholtz, M. M., and Theunissen, A. (2010). The use of indigenous cattle in terminal cross-breeding to improve beef cattle production in Sub-Saharan Africa. Anim. Genet. Resour. 46, 33–39.
Sheriff, O., and Alemayehu, K. (2018). Genetic diversity studies using microsatellite markers and their contribution in supporting sustainable sheep breeding programs: a review. Cogent Food Agric. 4:1459062.
Silva, M. V. B., Dos Santos, D. J. A., Boison, S. A., Utsunomiya, A. T. H., Carmo, A. S., Sonstegard, T. S., et al. (2014). The development of genomics applied to dairy breeding. Livest. Sci. 166, 66–75.
Ssewannyana, E., Oluka, J., and Masaba, J. K. (2004). Growth and performance of indigenous and crossbred goats. Uganda J. Agric. Sci. 9, 537–542. doi: 10.2527/jas.2014-8548
Staal, S., Baltenweck, I., Waithaka, M., and Njoroge, L. (2002). Location and uptake: integrated household and GIS analysis of technology adoption and land use, with application to smallholder dairy farms in Kenya. Agric. Econ. 27, 295–315.
Tan, W., Carlson, D. F., Lancto, C. A., Garbe, J. R., Webster, D. A., Hackett, P. B., et al. (2013). Efficient nonmeiotic allele introgression in livestock using custom endonucleases. Proc. Natl. Acad. Sci. U.S.A. 110, 16526–16531. doi: 10.1073/pnas.1310478110
Thornton, P. K., and Herrero, M. (2015). Adapting to climate change in the mixed crop and livestock farming systems in sub-Saharan Africa. Nat. Clim. Change 5, 830–836.
Traore, A., Alvarez, I., Fernandez, I., Perez-Pardal, L., Kabore, A., Ouedraogo-Sanou, G. M., et al. (2012). Ascertaining gene flow patterns in livestock populations of developing countries: a case study in Burkina Faso goat. BMC Genet. 13:35. doi: 10.1186/1471-2156-13-35
Traoré, S. A., Markemann, A., Reiber, C., Piepho, H. P., and Valle Zarate, A. (2017). Production objectives, trait and breed preferences of farmers keeping N’Dama, Fulani Zebu and crossbred cattle and implications for breeding programs. Animal 11, 687–695. doi: 10.1017/S1751731116002196
Tuggle, C. K., Giuffra, E., White, S. N., Clarke, L., Zhou, H., Ross, P. J., et al. (2016). GO-FAANG meeting: a gathering on functional annotation of animal genomes. Anim. Genet. 47, 528–533. doi: 10.1111/age.12466
Van Marle-Koster, E., and Visser, C. (2018). Genetic improvement in South African livestock: can genomics bridge the gap between the developed and developing sectors? Front. Genet. 9:331. doi: 10.3389/fgene.2018.00331
Van Marle-Köster, E., Visser, C., and Van der Westhuizen, R. R. (2017). “Genomic technology for the advancement of livestock production,” in Proceedings of the 50th Congress of the South African Society for Animal Science. 20-22 Septemeber, Port Elizabeth.
Vanderburg, S., Rubach, M. P., Halliday, J. E., Cleaveland, S., Reddy, E. A., and Crump, J. A. (2014). Epidemiology of Coxiella burnetii infection in Africa: a OneHealth systematic review. PLoS Negl. Trop. Dis. 8:e2787. doi: 10.1371/journal.pntd.0002787
VanderWaal, K., Morrison, R. B., Neuhauser, C., Vilalta, C., and Perez, A. M. (2017). translating Big Data into smart Data for veterinary epidemiology. Front. Vet. Sci. 4:110. doi: 10.3389/fvets.2017.00110
Wale, E., and Yalew, A. (2007). Farmers’ variety attribute preferences: implications for breeding priority setting and agricultural extension policy in Ethiopia. Afr. Dev. Rev. 19, 379–396.
Wall, E., Simm, G., and Moran, D. (2010). Developing breeding schemes to assist mitigation of greenhouse gas emissions. Animal 4, 366–376. doi: 10.1017/S175173110999070X
Wani, C. E., Yousif, I. A., Ibrahim, M. E., and Musa, H. H. (2014). Molecular characterization of Sudanese and southern Sudanese chicken breeds using mtDNA D-Loop. Genet. Res. Int. 2014:928420. doi: 10.1155/2014/928420
Weller, J., Ezra, E., and Ron, M. (2017). Invited review: a perspective on the future of genomic selection in dairy cattle. J. Dairy Sci. 100, 8633–8644. doi: 10.3168/jds.2017-12879
White, B., Amrine, D., and Larson, R. (2018). Big data analytics and precision animal agriculture symposium: data to decisions. J. Anim. Sci. 96, 1531–1539. doi: 10.1093/jas/skx065
Whitworth, K. M., Rowland, R. R., Ewen, C. L., Trible, B. R., Kerrigan, M. A., Cino-Ozuna, A. G., et al. (2016). Gene-edited pigs are protected from porcine reproductive and respiratory syndrome virus. Nat. Biotechnol. 34, 20–22.
Yousif, I. A., and El- Moula, A. A. F. (2006). Characterisation of Kenana cattle breed and its production environment. AGRI 38, 47–56.
Zhang, Z., Ober, U., Erbe, M., Zhang, H., Gao, N., He, J., et al. (2014). Improving the accuracy of whole genome prediction for complex traits using the results of genome wide association studies. PLoS One 9:e93017. doi: 10.1371/journal.pone.0093017
Keywords: Africa, breed improvement, genomic breeding, stakeholders, sustainable livestock development
Citation: Ibeagha-Awemu EM, Peters SO, Bemji MN, Adeleke MA and Do DN (2019) Leveraging Available Resources and Stakeholder Involvement for Improved Productivity of African Livestock in the Era of Genomic Breeding. Front. Genet. 10:357. doi: 10.3389/fgene.2019.00357
Received: 28 August 2018; Accepted: 03 April 2019;
Published: 24 April 2019.
Edited by:
Peter Dovc, University of Ljubljana, SloveniaReviewed by:
Mekonnen Haile-Mariam, Department of Economic Development, Jobs, Transport and Resources, AustraliaXiangdong Ding, China Agricultural University, China
Copyright © 2019 Ibeagha-Awemu, Peters, Bemji, Adeleke and Do. This is an open-access article distributed under the terms of the Creative Commons Attribution License (CC BY). The use, distribution or reproduction in other forums is permitted, provided the original author(s) and the copyright owner(s) are credited and that the original publication in this journal is cited, in accordance with accepted academic practice. No use, distribution or reproduction is permitted which does not comply with these terms.
*Correspondence: Eveline M. Ibeagha-Awemu, ZXZlbGluZS5pYmVhZ2hhLWF3ZW11QGNhbmFkYS5jYQ==