- 1Engelhardt Institute of Molecular Biology, Russian Academy of Sciences, Moscow, Russia
- 2Department of Agrobiotechnology, Peoples’ Friendship University of Russia (RUDN University), Moscow, Russia
Secoisolariciresinol diglucoside (SDG), found mainly in flaxseed, is one of the essential lignans. SDG, as well as the beneficial fatty acid composition and high fiber content, has made flaxseed an important source of functional food or nutraceutical ingredients. Various studies have shown that SDG offers several health benefits, including protective effects against cardiovascular diseases, diabetes, cancer, and mental stress. These health benefits have been attributed to the antioxidant properties of SDG. Additionally, SDG metabolites, namely mammalian lignans, enterodiol and enterolactone, have shown promising effects against cancer. Therefore, understanding the biosynthetic pathway of SDG and its molecular mechanisms is a key to enable the production of new flaxseed cultivars rich in nutraceutical content. The present review highlights studies on the different health benefits of SDG, as well as lignan biosynthesis in flaxseed and genes involved in the biosynthetic pathway. Since SDG, the predominant lignan in flaxseed, is a glycosylated lignan, we also focus on studies investigating the genes involved in secoisolariciresinol glycosylation. These genes can be used to produce new cultivars with a novel level of glycosylation or lignan composition to maximize the yields of lignans with a therapeutic or protective potential.
Introduction
Secoisolariciresinol (SECO) diglucoside (SDG) is one of the essential dietary lignans, found in high levels in flaxseed (Frank et al., 2004). Along with α-linolenic acid, lignans, mainly SDG, have made flaxseed derivatives (flax oil and lignan extracts) important sources of functional food or nutraceutical ingredients (Hu et al., 2007; Patel et al., 2012). Although flaxseed possesses beneficial fatty acid composition and high fiber content, the phytoestrogenic, anticarcinogenic, and antiatherogenic effects have been attributed to its lignan content (Muir and Westcott, 2003; Frank et al., 2004; Webb and McCullough, 2005; Carraro et al., 2012). Moreover, ingested SDG is converted into mammalian lignans, enterodiol (END) and enterolactone (ENL), by the gut microflora enzymes (Carraro et al., 2012; Struijs, 2008), and these mammalian lignans have been found to negatively correlate with the incidence of breast cancer (Jenab and Thompson, 1996; Frank et al., 2004; Adolphe et al., 2010).
SDG was isolated from flaxseed by Bakke and Klosterman (1956), and in contrast to free lignans found in most plants, SDG is present in an oligomeric structure, referred to as lignan macromolecule (Bakke and Klosterman, 1956; Muir and Westcott, 2003; Struijs, 2008). Flaxseed is the richest source of SDG; however, it also contains small amounts of other lignans, namely pinoresinol, lariciresinol, and matairesinol (Muir and Westcott, 2003; Struijs, 2008; Adolphe et al., 2010). SDG has two enantiomers (+) and (-), whose distribution varies in different Linum species. A study showed that one of the two enantiomers of SDG was predominant in the seed, except for some species, such as L. elegans and L. flavum, that contained both enantiomers (Muir and Westcott, 2003). The (+) enantiomer was predominant in L. usitatissimum (flaxseed); however, the concentration of SDG varied in different varieties (Muir and Westcott, 2003). Although new technologies, such as in vitro morphogenesis (Lalaleo et al., 2018b), transformed hairy root culture (Gabr et al., 2018) and other biotechnological systems (Lalaleo et al., 2018a), are being used to increase the content of lignans in Linum species, the difference in varieties still affects the health beneficial properties of the lignan fractions (Ezzat et al., 2018). In this review, we highlight studies on the biosynthesis of SDG in flaxseed and its health benefits, as well as the genes involved in this metabolic pathway.
Lignans From Flaxseed
The main lignan in flaxseed is SDG, which is found in a linked macromolecular structure (lignan macromolecule) (Muir and Westcott, 2003; Suzuki and Umezawa, 2007; Struijs, 2008; Touré and Xueming, 2010; Schmidt et al., 2012). In flaxseed, the hull fraction contains higher amounts of lignans than that in the whole seeds (Oomah and Mazza, 1997; Madhusudhan et al., 2000; Wiesenborn et al., 2003; Struijs et al., 2006), suggesting that lignans are mainly biosynthesized in the hull.
Biosynthetic Pathways of Flaxseed Lignans
The biosynthesis of lignans in flaxseed involves the following pathways (Umezawa, 2003; Hano et al., 2006; Struijs, 2008; Ghose et al., 2014) (KEGG PATHWAY: map01061):
• the phenylpropanoid pathway
• stereospecific coupling by dirigent proteins
• biosynthesis of dibenzylbutane lignans
• glycosylation of lignans into SDG
The Phenylpropanoid Pathway
The phenylpropanoid pathway is responsible for the formation of the C6-C3 basic unit of lignans. The first step of this pathway is the deamination of phenylalanine by phenylalanine-ammonia lyase producing cinnamic acid (Struijs, 2008). Cinnamic acid is oxidized to p-coumaric acid, which can further be oxidized to caffeic acid. Then, caffeic acid can be metabolized into ferulic acid by caffeic acid O-methyltransferase. Ferulic acid is converted into its coenzyme A-activated form, feruloyl-CoA by 4-coumarate:CoA ligase. Feruloyl-CoA is further reduced to coniferaldehyde by cinnamoyl-CoA reductase and finally into coniferyl alcohol by cinnamyl alcohol dehydrogenase and sinapyl alcohol dehydrogenase (Boerjan et al., 2003).
Stereospecific Coupling by Dirigent Proteins
The formation of a lignan via stereoselective coupling of two coniferyl alcohols was first studied in Forsythia species in 1990, when two phenylpropanoid monomers, coniferyl alcohols, were oxidized in the presence of the insoluble fraction of Forsythia stem, yielding pinoresinol (Umezawa et al., 1990). Later, a protein purified from the insoluble fraction of Forsythia stem was used to catalyze the dimerization of coniferyl alcohols only in the presence of an oxidase and was called a dirigent protein since it is not involved in the reaction (Davin et al., 1997; Corbin et al., 2018). The discovery of the dirigent protein (Davin et al., 1997; Davin and Lewis, 2000) allowed the understanding of phenolic radical coupling: an oxidase forms two radicals from the alcohols, and the dirigent protein aligns the radicals, followed by the stereoselective radical coupling reaction. Of all the C6-C3 units formed by the phenylpropanoid pathway, only coniferyl alcohol is dimerized in a stereospecific way (Davin et al., 1997).
After the formation of pinoresinol, lignan biosynthesis can proceed by two different pathways. In the first pathway, the furan structures are reduced, and dibenzylbutane lignans, such as SECO, are formed. In the second pathway, the furan structures remain intact, and methylenedioxy bridged furanofuran lignans, such as sesamin, are formed (Suzuki and Umezawa, 2007; Struijs, 2008; Schmidt et al., 2012). In flaxseed, the biosynthesis follows the first pathway.
Biosynthesis of Dibenzylbutane Lignans
Pinoresinol is subsequently converted into lariciresinol and secoisolariciresinol by a bifunctional NADPH-dependent pinoresinol/lariciresinol reductase (PLR) (Ford et al., 2001). PLRs completely convert pinoresinol into lariciresinol first before lariciresinol is converted to SECO (Von Heimendahl et al., 2005; Hemmati et al., 2007). The other lignan found in flaxseed, matairesinol, is formed by secoisolariciresinol dehydrogenase (SDH) (Ford et al., 2001; Xia et al., 2001; Umezawa, 2003; Struijs, 2008). The main lignan in flaxseed is found in a glycosylated form, indicating an additional role of the genes responsible for the glycosylation of SECO. These genes are UDPG: glucosyltransferases (GTs), which glycosylate the C-9 and C-9′ hydroxyl positions of SECO (Ford et al., 2001).
Glycosylation of Seco
In flaxseed, this represents the last step in the biosynthesis of SDG. Glycosylation is catalyzed by GTs, which are highly divergent, polyphyletic, and belong to a multigene family found in all living organisms (Mackenzie et al., 1997; Barvkar et al., 2012).
Several studies have shown the presence of plant GTs (Ross et al., 2001; Wang and Hou, 2009; Caputi et al., 2012). In plants, they belong to family 1 GTs, known as uridine GTs (UGTs) (Caputi et al., 2012; Fofana et al., 2017b). UGTs catalyze the transfer of UDP-activated sugars to specific acceptor molecules (Jones and Vogt, 2001; Ross et al., 2001; Witte et al., 2009). In plants, they are characterized by a unique, well-conserved PSPG (plant secondary product glycosyltransferases) box, formed by a sequence of 44-amino acid residues (Paquette et al., 2003; Gachon et al., 2005; Barvkar et al., 2012) and a catalytic mechanism that inverts the anomeric configuration of the transferred sugar (Wang and Hou, 2009; Barvkar et al., 2012).
In flax, 137 UGT genes have been identified (Barvkar et al., 2012). UGT74S1 gene expression was high in the seed coat. Since SDG accumulates in the seed coat (Hano et al., 2006), it was suggested that UGT74S1 might play a role as SECO GT (Barvkar et al., 2012). Further studies found that two UGT genes, UGT74S1 and UGT94H, exhibited high expression level in the developing seeds, and their expression correlated with that of PLR genes, involved in SECO biosynthesis (Ghose et al., 2014). However, upon reaction of the purified proteins with SECO and UDP-glucose, only UGT74S1 produced both SECO monoglucoside (SMG) and SDG metabolites, showing that UGT74S1 is involved in SECO glycosylation to form flax SDG lignan (Ghose et al., 2014).
The uniqueness of UGT74S1 in controlling SDG formation from SECO was further proven in a study characterizing a mutagenized flax population with mutations in UGT74S1 and altered lignan glycosylation, which showed that the loss-of-function of SECO glycosylation into SDG was attributable solely to UGT74S1 mutation (Fofana et al., 2017b). UGT74S1 may be the key enzyme controlling SECO glycosylation in flax, although two closely related genes, UGT74S4 and UGT74S3, may also contribute to a minor extent, where they may play a role in supplying SMG as a substrate to UGT74S1 for the second glycosylation step (Fofana et al., 2017a). Moreover, several studies have shown that specific amino acids within the PSPG motif might play a role (He et al., 2006; Noguchi et al., 2007; Modolo et al., 2009). Additionally, Ghose et al. (2015) have shown that Trp355 and His352 are critical amino acids within the PSPG motif and are determinant for UGT74S1 glycosylating activity (Ghose et al., 2015).
A summary of the biosynthesis of SDG is shown in Figure 1.
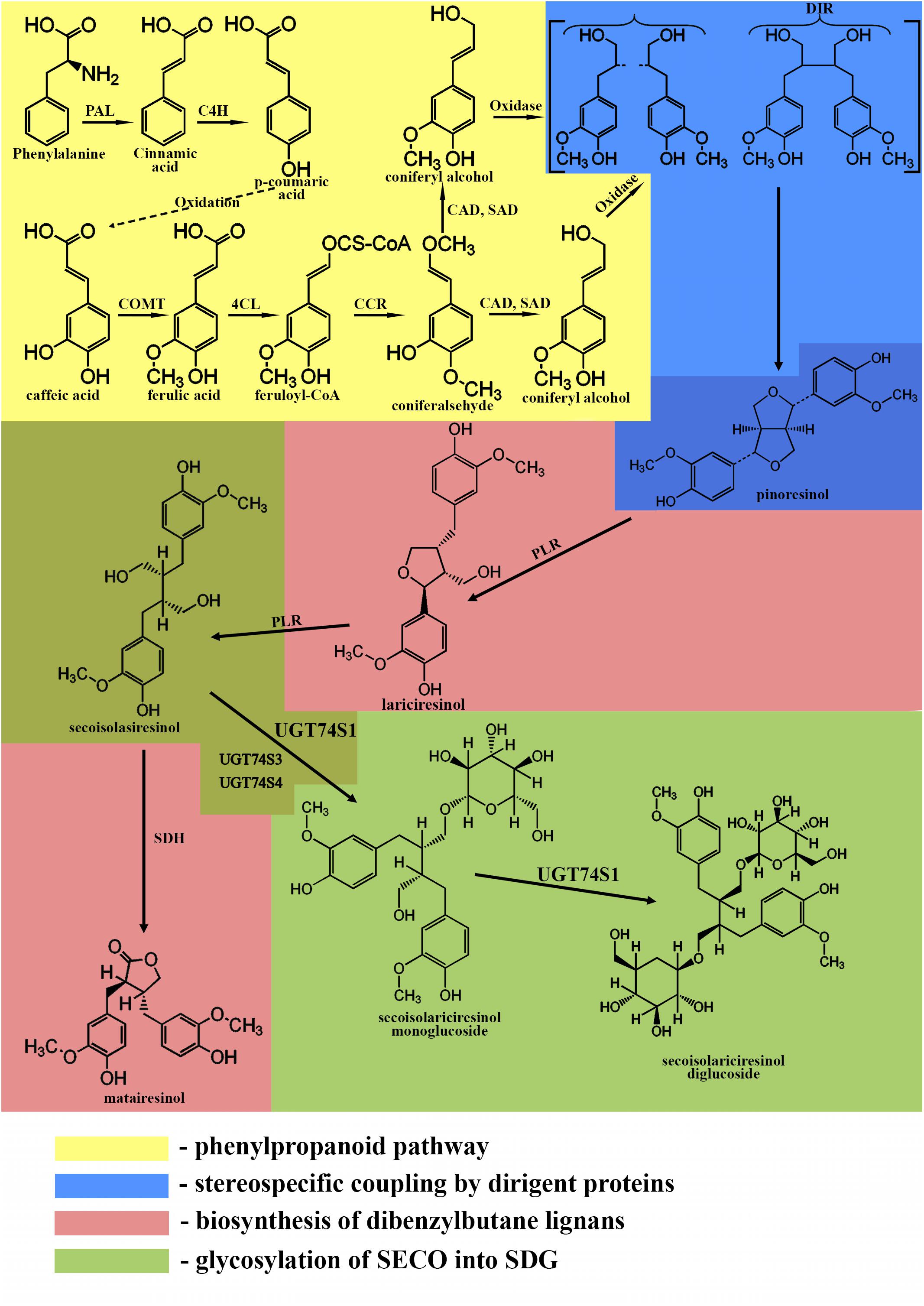
Figure 1. Biosynthetic pathway of SDG. Phenylpropanoid pathway (yellow): PAL, phenylalanine ammonium lyase; C4H, cinnamate 4- hydroxylase; COMT, caffeic acid O-methyltransferase; 4CL, 4-coumarate:CoA ligase; CCR, cinnamoyl-CoA reductase; CAD, cinnamyl alcohol dehydrogenase; SAD, sinapyl alcohol dehydrogenase. Stereospecific coupling (blue); DIR, dirigent proteins. Biosynthesis of dibenzylbutane lignans (pink); PLR, pinoresinol/lariciresinol reductase; SDH, secoisolariciresinol dehydrogenase. Glycosylation of SECO (green); UGT74S1, UGT74S3, UGT74S4, uridine glucosyltransferases (UGTs).
Health Benefits of Sdg
Various studies on the health effects of flaxseed lignans have shown some potential beneficial effects on human and animal health. The conversion of SDG into mammalian lignans, ENL and END, by the intestinal microflora contributes to the bioavailability of SDG; however, other factors, such as diet, antibiotics, and obesity, also affect the circulating lignan levels in the body (Adlercreutz, 2007). Therefore, different levels of lignan bioactivation are observed owing to variations in these factors.
SDG and its mammalian metabolites have been shown to act as nutraceutical agents against some diseases, such as cancer, diabetes, and heart diseases (Touré and Xueming, 2010; Imran et al., 2015). Some of these health effects (Table 1) are discussed in this review.
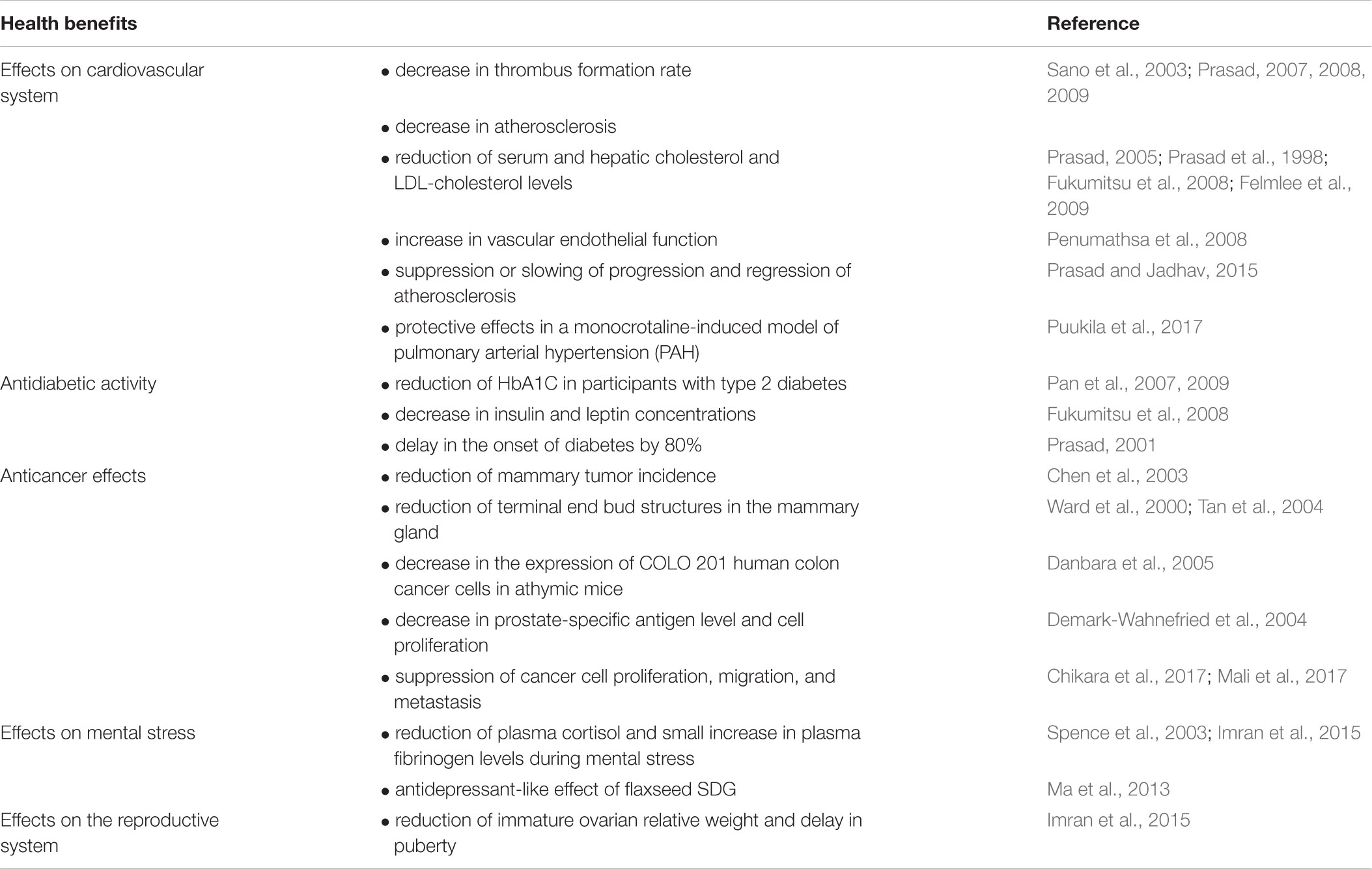
Table 1. Health benefits of secoisolariciresinol diglucoside (SDG) and its metabolites, namely secoisolariciresinol (SECO), enterolactone (ENL), and enterodiol (END).
Antioxidant Activity of SDG
Some beneficial effects of SDG have been attributed to its antioxidant properties. The ability of SDG to scavenge hydroxyl radicals might contribute to its effects in cancer and lupus nephritis (Prasad, 1997; Touré and Xueming, 2010). SDG and its metabolites have been also shown to prevent DNA oxidative damage and lipid peroxidation (Katare et al., 2012). The antioxidant activities of SECO, SDG, END, and ENL were shown to be involved in the hypocholesterolemic and antiatherogenic effects (Prasad, 2000a,b). Moreover, a recent study comparing flaxseed oil and flaxseed lignan showed that SDG could prevent oxidative stress associated with metabolic syndrome (Pilar et al., 2017).
Additionally, SDG scavenged radiation-induced HOCl in physiological solutions, and a synthetic SDG (LGM2605) was suggested as a promising attenuator of oxidative stress-induced inflammatory tissue damage (Mishra et al., 2018).
Effects on the Cardiovascular System
Chronic heart diseases, caused directly or indirectly by oxidative stress, inflammation, obesity, diabetes, dyslipidemia, and hypertension, are the leading causes of death in developed nations (Imran et al., 2015). SDG and its metabolites were reported to exhibit cardiovascular protective effects, where they lowered total cholesterol, LDL-cholesterol (LDL-C), and triglyceride levels and normalized HDL-cholesterol (HDL-C) and glucose metabolism, leading to less cardiovascular complications (Zhang W. et al., 2008; Zanwar et al., 2013, 2014). SDG consumption may protect against the development of chronic diseases, such as cardiovascular diseases (Mathieu et al., 2009; O’Keefe et al., 2009; Adolphe et al., 2010).
A recent study on the potential protective effects of SDG in monocrotaline-induced pulmonary arterial hypertension was conducted using male rats. Pretreatment with SDG decreased right ventricular hypertrophy, reactive oxygen species (ROS) levels, lipid peroxidation, catalase, superoxide dismutase, glutathione peroxidase activities, alanine transaminase (ALT), and aspartate transaminase (AST) plasma levels, compared to those in the monocrotaline group. However, cotreatment with SDG did not attenuate ventricular hypertrophy, ALT or AST levels, but decreased ROS levels and catalase and superoxide dismutase activities, compared to those in the monocrotaline group (Puukila et al., 2017).
Secoisolariciresinol diglucoside, even at very low doses (15 mg/kg), suppressed the development of hypercholesterolemic atherosclerosis by 73%, and this effect was associated with a decrease in serum total cholesterol, LDL-C, and oxidative stress, and an increase in HDL-C levels. Therefore, SDG can prevent or delay the progression of atherosclerosis, and hence prevent coronary artery disease, stroke, and peripheral arterial vascular diseases (Prasad and Jadhav, 2015).
Anticancer Effects
Secoisolariciresinol diglucoside has been shown to prevent some malignancies, such as breast, lung, and colon cancers, owing to its strong antiproliferative, antioxidant, antiestrogenic, and/or antiangiogenic activities. Additionally, the anticancer activity of SDG was suggested to be associated with inhibition of enzymes involved in carcinogenesis (Imran et al., 2015). SDG reduced aberrant crypt multiplicity that may protect against colon cancer (Jenab and Thompson, 1996), and supplementation of SDG in diet resulted in reduction of the volume, area, and number of tumors (Li et al., 1999). In humans, the protective effects of SDG against cancer have been associated with its capability to affect hormone levels and cancer progression (Imran et al., 2015). Furthermore, SDG was considered a chemopreventive agent against malignant mesothelioma owing to its ability to reduce acute asbestos-induced peritoneal inflammation, nitrosative, and oxidative stress (Pietrofesa et al., 2016).
Several studies showed a correlation between SDG metabolites and breast cancer in women, where the serum levels of intestinal microbial END and ENL showed inverse association with breast cancer (Pietinen et al., 2001; Boccardo et al., 2004; Imran et al., 2015). SDG might have the potential to promote early enhancement of mammary gland differentiation, which could protect against breast cancer (Tan et al., 2004; Adolphe et al., 2010). However, García-Mateos et al. (2018) showed that the preventive potential of SDG and its metabolites could be affected by the breast cancer resistance protein (BCRP/ABCG2) (García-Mateos et al., 2018).
Secoisolariciresinol diglucoside was suggested to protect against breast cancer owing to its regulation of the expression level of zinc transporters since the concentration of zinc is higher in breast cancer cells than in the normal breast cells (Zhang L. Y. et al., 2008). Moreover, studies have shown that the anticancer potential of SDG metabolite, ENL, is related to its ability to suppress the proliferation, migration, and metastasis of cancer cells (Chikara et al., 2017; Mali et al., 2017). A research group of Dr. Thompson (University of Toronto) verified the anticancer activity of SDG, where SDG supplementation in mouse diet resulted in a decrease in the tumor load (Shareef and Sarfraz, 2016).
In a clinical trial, the anticancer effects of SDG were studied in 45 premenopausal women with suspicious breast biopsies or who were former breast cancer survivors. SDG lignan (50 mg) was administered daily for a year. Women receiving SDG exhibited less breast precancerous changes, and 80% of them showed a decrease in the levels of Ki-67, a biomarker of cell proliferation (Fabian et al., 2010).
Additionally, the anticancer effects of a purified flaxseed hydrolysate (PFH), a lignan-rich fraction, were studied. PFH of the cultivar Giza9 (rich in SDG) reduced the expression of the metastasis marker, 1-α, metalloproteinases, and vascular endothelial growth factor, one of the most potent stimulators of angiogenesis, whereas it increased caspase-3-dependent apoptosis, suggesting that it exhibited anticancer activity in a human breast cancer cell line T47D. Dietary intake of Giza9 cultivar-derived PFH resulted in a decrease in the tumor volume and an increase in the expression of caspase-3, suggesting that it exerted anticancer activity in tumor-bearing mice (Ezzat et al., 2018).
Antidiabetic Activity
Apart from flaxseed fibers that may affect insulin secretion and plasma glucose homeostasis, studies have shown that SDG-containing nutrients also affect plasma glucose homeostasis (Touré and Xueming, 2010). SDG reduced C-reactive protein concentration, which is related to insulin resistance in type 2 diabetes (Prasad, 2009; Peterson et al., 2010), and decreased the development of diet-induced obesity (Fukumitsu et al., 2008) and glucosuria (Prasad, 2001). Flax lignan complex decreased the metabolic syndrome composite score in males; however, no effects were observed in females (Cornish et al., 2009). The antidiabetic activity of SDG was suggested to be related to its antioxidant effects (Prasad, 2000b; Prasad et al., 2000).
Secoisolariciresinol diglucoside treatment reduced the incidence of diabetes by 75% in streptozotocin-induced model of diabetes and by 72% in the Bio-Breed diabetic rat model of diabetes. These effects were associated with a decrease in oxidative stress, as evidenced by the decrease in serum and pancreatic malondialdehyde (MDA) levels. SDG was also shown to delay the development of diabetes in Zucker fatty rat type 2 diabetes model, which was associated with reduction of serum MDA and glycated hemoglobin (A1C) levels (Prasad and Dhar, 2015). Thus, SDG may have antidiabetic potential, where it can reduce the incidence of type 1 diabetes and delay the development of type 2 diabetes in humans.
Effects on Mental Stress
Administration of SDG inhibited stress-induced behavioral changes, whereas treatment with high doses of SDG reversed chronic stress-induced increase in serum corticosterone and adrenocorticotropic hormone (ACTH) levels. Additionally, the effects of SDG on the behaviors of ovariectomized mice might be related to modulation of the neuroendocrine-immune network and neurotrophin factor expression (Ma et al., 2013). Three flax cultivars with different contents of alpha-linolenic acid and lignans significantly reduced blood pressure during frustrating cognitive task-induced mental stress in postmenopausal women with vascular diseases. The cultivar with the highest lignan and lowest alpha-linolenic acid contents was associated with the minimum increase in peripheral resistance and plasma fibrinogen levels and the maximum reduction of plasma cortisol levels during mental stress (Spence et al., 2003).
Effects on the Reproductive System
The potential effects of SDG on the reproductive system were studied since lignans were reported to have estrogen agonist or antagonist properties. Flaxseed had no effect on rat pregnancy outcome; however, 10% flaxseed-containing diet lowered the birth weight, compared to that of other treatments. Additionally, it exhibited estrogenic effects, including greater uterine and ovarian relative weights, earlier age and lighter body weight at puberty in female offspring, as well as reduced postnatal weight gain and greater sex gland and prostate relative weights in males. However, 5% flaxseed-containing diet reduced immature ovarian relative weight by 29%, delayed puberty by approximately 5 days, and tended to prolong the diestrus, indicating an antiestrogenic effect. Therefore, flaxseed was shown to affect the development of the reproductive system in offspring; thus, caution should be taken when consuming flaxseed during pregnancy and lactation (Tou et al., 1998). Exposure to lignans during lactation reduced the susceptibility to mammary carcinogenesis later in life without adverse effects on selective reproductive indices in dams or offspring (Chen et al., 2003).
Conclusion
This review summarized the health beneficial effects of SDG, the most predominant lignan in flaxseed, where it can protect against several diseases, including cardiovascular diseases, cancer, diabetes, and mental stress, and affect the reproductive system. Additionally, we reviewed the biosynthetic pathways of SDG in plants.
Author Contributions
All authors contributed to the preparation of this review.
Funding
This work was financially supported by the Russian Science Foundation (Grant No. 16-16-00114; the part devoted to biosynthetic pathways) and «RUDN University Program 5-100» (the part devoted to health benefits).
Conflict of Interest Statement
The authors declare that the research was conducted in the absence of any commercial or financial relationships that could be construed as a potential conflict of interest.
References
Adlercreutz, H. (2007). Lignans and human health. Crit. Rev. Clin. Lab. Sci. 44, 483–525. doi: 10.1080/10408360701612942
Adolphe, J. L., Whiting, S. J., Juurlink, B. H. J., Thorpe, L. U., and Alcorn, J. (2010). Health effects with consumption of the flax lignan secoisolariciresinol diglucoside. Br. J. Nutr. 103, 929–938. doi: 10.1017/S0007114509992753
Bakke, J. E., and Klosterman, H. J. (1956). A new diglucoside from flaxseed. Proc. N. D. Acad. Sci. 10, 18–22.
Barvkar, V. T., Pardeshi, V. C., Kale, S. M., Kadoo, N. Y., and Gupta, V. S. (2012). Phylogenomic analysis of UDP glycosyltransferase 1 multigene family in Linum usitatissimum identified genes with varied expression patterns. BMC Genomics 13:175. doi: 10.1186/1471-2164-13-175
Boccardo, F., Lunardi, G., Guglielmini, P., Parodi, M., Murialdo, R., Schettini, G., et al. (2004). Serum enterolactone levels and the risk of breast cancer in women with palpable cysts. Eur. J. Cancer 40, 84–89. doi: 10.1016/S0959-8049(03)00576-8
Boerjan, W., Ralph, J., and Baucher, M. (2003). Lignin biosynthesis. Annu. Rev. Plant Biol. 54, 519–546. doi: 10.1146/annurev.arplant.54.031902.134938
Caputi, L., Malnoy, M., Goremykin, V., Nikiforova, S., and Martens, S. (2012). A genome-wide phylogenetic reconstruction of family 1 UDP- glycosyltransferases revealed the expansion of the family during the adaptation of plants to life on land. Plant J. 69, 1030–1042. doi: 10.1111/j.1365-313X.2011.04853.x
Carraro, J. C. C., de Souza Dantas, M. I., Espeschit, A. C. R., Martino, H. S. D., and Ribeiro, S. M. R. (2012). Flaxseed and human health: reviewing benefits and adverse effects. Food Rev. Int. 28, 203–230. doi: 10.1080/87559129.2011.595025
Chen, J., Tan, K. P., Ward, W. E., and Thompson, L. U. (2003). Exposure to flaxseed or its purified lignan during suckling inhibits chemically induced rat mammary tumorigenesis. Exp. Biol. Med. 228, 951–958. doi: 10.1177/153537020322800811
Chikara, S., Lindsey, K., Borowicz, P., Christofidou-Solomidou, M., and Reindl, K. M. (2017). Enterolactone alters FAK-Src signaling and suppresses migration and invasion of lung cancer cell lines. BMC Complement. Altern. Med. 17:30. doi: 10.1186/s12906-016-1512-3
Corbin, C., Drouet, S., Markulin, L., Auguin, D., Lainé,É, Davin, L. B., et al. (2018). A genome-wide analysis of the flax (Linum usitatissimum L.) dirigent protein family: from gene identification and evolution to differential regulation. Plant Mol. Biol. 97, 73–101. doi: 10.1007/s11103-018-0725-x
Cornish, S. M., Chilibeck, P. D., Paus-Jennsen, L., Biem, H. J., Khozani, T., Senanayake, V., et al. (2009). A randomized controlled trial of the effects of flaxseed lignan complex on metabolic syndrome composite score and bone mineral in older adults. Appl. Physiol. Nutr. Metab. 34, 89–98. doi: 10.1139/H08-142
Danbara, N., Yuri, T., Tsujita-Kyutoku, M., Tsukamoto, R., Uehara, N., and Tsubura, A. (2005). Enterolactone induces apoptosis and inhibits growth of colo 201 human colon cancer cells both in vitro and in vivo. Anticancer Res. 25, 2269–2276.
Davin, L. B., and Lewis, N. G. (2000). Dirigent proteins and dirigent sites explain the mystery of specificity of radical precursor coupling in lignan and lignin biosynthesis. Plant Physiol. 123, 453–461. doi: 10.1104/pp.123.2.453
Davin, L. B., Wang, H. B., Crowell, A. L., Bedgar, D. L., Martin, D. M., Sarkanen, S., et al. (1997). Stereoselective bimolecular phenoxy radical coupling by an auxiliary (dirigent) protein without an active center. Science 275, 362–366. doi: 10.1126/SCIENCE.275.5298.362
Demark-Wahnefried, W., Robertson, C. N., Walther, P. J., Polascik, T. J., Paulson, D. F., and Vollmer, R. T. (2004). Pilot study to explore effects of low-fat, flaxseed-supplemented diet on proliferation of benign prostatic epithelium and prostate-specific antigen. Urology 63, 900–904. doi: 10.1016/j.urology.2003.12.010
Ezzat, S. M., Shouman, S. A., Elkhoely, A., Attia, Y. M., Elsesy, M. S., El Senousy, A. S., et al. (2018). Anticancer potentiality of lignan rich fraction of six Flaxseed cultivars. Sci. Rep. 8:544. doi: 10.1038/s41598-017-18944-0
Fabian, C. J., Kimler, B. F., Zalles, C. M., Klemp, J. R., Petroff, B. K., Khan, Q. J., et al. (2010). Reduction in Ki-67 in benign breast tissue of high-risk women with the lignan secoisolariciresinol diglycoside. Cancer Prev. Res. 3, 1342–1350. doi: 10.1158/1940-6207.CAPR-10-0022
Felmlee, M. A., Woo, G., Simko, E., Krol, E. S., Muir, A. D., and Alcorn, J. (2009). Effects of the flaxseed lignans secoisolariciresinol diglucoside and its aglycone on serum and hepatic lipids in hyperlipidaemic rats. Br. J. Nutr. 102, 361–369. doi: 10.1017/S0007114508207488
Fofana, B., Ghose, K., McCallum, J., You, F. M., and Cloutier, S. (2017a). UGT74S1 is the key player in controlling secoisolariciresinol diglucoside (SDG) formation in flax. BMC Plant Biol. 17:35. doi: 10.1186/s12870-017-0982-x
Fofana, B., Ghose, K., Somalraju, A., McCallum, J., Main, D., Deyholos, M. K., et al. (2017b). Induced mutagenesis in UGT74S1 gene leads to stable new flax lines with altered secoisolariciresinol diglucoside (SDG) profiles. Front. Plant Sci. 8:1638. doi: 10.3389/fpls.2017.01638
Ford, J. D., Huang, K. S., Wang, H. B., Davin, L. B., and Lewis, N. G. (2001). Biosynthetic pathway to the cancer chemopreventive secoisolariciresinol diglucoside - Hydroxymethyl glutaryl ester-linked lignan oligomers in flax (Linum usitatissimum) seed. J. Nat. Prod. 64, 1388–1397. doi: 10.1021/np010367x
Frank, J., Eliasson, C., Leroy-Nivard, D., Budek, A., Lundh, T., Vessby, B., et al. (2004). Dietary secoisolariciresinol diglucoside and its oligomers with 3-hydroxy-3-methyl glutaric acid decrease vitamin E levels in rats. Br. J. Nutr. 92, 169–176. doi: 10.1079/BJN20041154
Fukumitsu, S., Aida, K., Ueno, N., Ozawa, S., Takahashi, Y., and Kobori, M. (2008). Flaxseed lignan attenuates high-fat diet-induced fat accumulation and induces adiponectin expression in mice. Br. J. Nutr. 100, 669–676. doi: 10.1017/S0007114508911570
Gabr, A. M. M., Mabrok, H. B., Abdel-Rahim, E. A., El-Bahr, M. K., and Smetanska, I. (2018). Determination of lignans, phenolic acids and antioxidant capacity in transformed hairy root culture of Linum usitatissimum. Nat. Prod. Res. 32, 1867–1871. doi: 10.1080/14786419.2017.1405405
Gachon, C. M. M., Langlois-Meurinne, M., and Saindrenan, P. (2005). Plant secondary metabolism glycosyltransferases: the emerging functional analysis. Trends Plant Sci. 10, 542–549. doi: 10.1016/j.tplants.2005.09.007
García-Mateos, D., García-Villalba, R., Otero, J. A., Marañón, J. A., Espín, J. C., Álvarez, A. I., et al. (2018). An altered tissue distribution of flaxseed lignans and their metabolites in Abcg2 knockout mice. Food Funct. 9, 636–642. doi: 10.1039/C7FO01549F
Ghose, K., McCallum, J., Sweeney-Nixon, M., and Fofana, B. (2015). Histidine 352 (His352) and tryptophan 355 (Trp355) are essential for flax UGT74S1 glucosylation activity toward secoisolariciresinol. PLoS One 10:e116248. doi: 10.1371/journal.pone.0116248
Ghose, K., Selvaraj, K., McCallum, J., Kirby, C. W., Sweeney-Nixon, M., Cloutier, S. J., et al. (2014). Identification and functional characterization of a flax UDP-glycosyltransferase glucosylating secoisolariciresinol (SECO) into secoisolariciresinol monoglucoside (SMG) and diglucoside (SDG). BMC Plant Biol. 14:82. doi: 10.1186/1471-2229-14-82
Hano, C., Martin, I., Fliniaux, O., Legrand, B., Gutierrez, L., Arroo, R. R. J., et al. (2006). Pinoresinol-lariciresinol reductase gene expression and secoisolariciresinol diglucoside accumulation in developing flax (Linum usitatissimum) seeds. Planta 224, 1291–1301. doi: 10.1007/s00425-006-0308-y
He, X.-Z., Wang, X., and Dixon, R. A. (2006). Mutational analysis of the Medicago glycosyltransferase UGT71G1 reveals residues that control regioselectivity for (iso)flavonoid glycosylation. J. Biol. Chem. 281, 34441–34447. doi: 10.1074/jbc.M605767200
Hemmati, S., Schmidt, T. J., and Fuss, E. (2007). (+)-Pinoresinol/(-)-lariciresinol reductase from Linum perenne Himmelszelt involved in the biosynthesis of justicidin B. FEBS Lett. 581, 603–610. doi: 10.1016/j.febslet.2007.01.018
Hu, C., Yuan, Y. V., and Kitts, D. D. (2007). Antioxidant activities of the flaxseed lignan secoisolariciresinol diglucoside, its aglycone secoisolariciresinol and the mammalian lignans enterodiol and enterolactone in vitro. Food Chem. Toxicol. 45, 2219–2227. doi: 10.1016/j.fct.2007.05.017
Imran, M., Ahmad, N., Anjum, F. M., Khan, M. K., Mushtaq, Z., Nadeem, M., et al. (2015). Potential protective properties of flax lignan secoisolariciresinol diglucoside. Nutr. J. 14:71. doi: 10.1186/s12937-015-0059-3
Jenab, M., and Thompson, L. U. (1996). The influence of flaxseed and lignans on colon carcinogenesis and beta-glucuronidase activity. Carcinogenesis 17, 1343–1348. doi: 10.1093/carcin/17.6.1343
Jones, P., and Vogt, T. (2001). Glycosyltransferases in secondary plant metabolism: tranquilizers and stimulant controllers. Planta 213, 164–174. doi: 10.1007/s004250000492
Katare, C., Saxena, S., Agrawal, S., and Prasad, G. (2012). Flax seed: a potential medicinal food. J. Nutr. Food Sci. 2:120. doi: 10.4172/2155-9600.1000120
Lalaleo, L., Alcazar, R., Palazon, J., Moyano, E., Cusido, R. M., and Bonfill, M. (2018a). Comparing aryltetralin lignan accumulation patterns in four biotechnological systems of Linum album. J. Plant Physiol. 228, 197–207. doi: 10.1016/j.jplph.2018.06.006
Lalaleo, L., Testillano, P., Risueño, M.-C., Cusidó, R. M., Palazon, J., Alcazar, R., et al. (2018b). Effect of in vitro morphogenesis on the production of podophyllotoxin derivatives in callus cultures of Linum album. J. Plant Physiol. 228, 47–58. doi: 10.1016/J.JPLPH.2018.05.007
Li, D., Yee, J. A., Thompson, L. U., and Yan, L. (1999). Dietary supplementation with secoisolariciresinol diglycoside (SDG) reduces experimental metastasis of melanoma cells in mice. Cancer Lett. 142, 91–96. doi: 10.1016/S0304-3835(99)00158-5
Ma, X., Wang, R., Zhao, X., Zhang, C., Sun, J., Li, J., et al. (2013). Antidepressant-like effect of flaxseed secoisolariciresinol diglycoside in ovariectomized mice subjected to unpredictable chronic stress. Metab. Brain Dis. 28, 77–84. doi: 10.1007/s11011-012-9371-1
Mackenzie, P. I., Owens, I. S., Burchell, B., Bock, K. W., Bairoch, A., Belanger, A., et al. (1997). The UDP glycosyltransferase gene superfamily: recommended nomenclature update based on evolutionary divergence. Pharmacogenetics 7, 255–269. doi: 10.1097/00008571-199708000-00001
Madhusudhan, B., Wiesenborn, D., Schwarz, J., Tostenson, K., and Gillespie, J. (2000). A dry mechanical method for concentrating the lignan secoisolariciresinol diglucoside in flaxseed. LWT Food Sci. Technol. 33, 268–275. doi: 10.1006/FSTL.2000.0652
Mali, A. V., Joshi, A. A., Hegde, M. V., and Kadam, S. S. (2017). Enterolactone suppresses proliferation, migration and metastasis of MDA-MB-231 breast cancer cells through inhibition of uPA induced plasmin activation and MMPs-mediated ECM remodeling. Asian Pac. J. Cancer Prev. 18, 905–915. doi: 10.22034/APJCP.2017.18.4.905
Mathieu, P., Poirier, P., Pibarot, P., Lemieux, I., and Despres, J.-P. (2009). Visceral obesity: the link among inflammation, hypertension, and cardiovascular disease. Hypertension 53, 577–584. doi: 10.1161/HYPERTENSIONAHA.108.110320
Mishra, O. P., Popov, A. V., Pietrofesa, R. A., Nakamaru-Ogiso, E., Andrake, M., and Christofidou-Solomidou, M. (2018). Synthetic secoisolariciresinol diglucoside (LGM2605) inhibits myeloperoxidase activity in inflammatory cells. Biochim. Biophys. Acta 1862, 1364–1375. doi: 10.1016/J.BBAGEN.2018.03.003
Modolo, L. V., Escamilla-Treviño, L. L., Dixon, R. A., and Wang, X. (2009). Single amino acid mutations of Medicago glycosyltransferase UGT85H2 enhance activity and impart reversibility. FEBS Lett. 583, 2131–2135. doi: 10.1016/j.febslet.2009.05.046
Noguchi, A., Saito, A., Homma, Y., Nakao, M., Sasaki, N., Nishino, T., et al. (2007). A UDP-glucose:isoflavone 7-O-glucosyltransferase from the roots of soybean (Glycine max) seedlings. Purification, gene cloning, phylogenetics, and an implication for an alternative strategy of enzyme catalysis. J. Biol. Chem. 282, 23581–23590. doi: 10.1074/jbc.M702651200
O’Keefe, J. H., Carter, M. D., and Lavie, C. J. (2009). Primary and secondary prevention of cardiovascular diseases: a practical evidence-based approach. Mayo Clin. Proc. 84, 741–757. doi: 10.1016/S0025-6196(11)60525-9
Oomah, B. D., and Mazza, G. (1997). Effect of dehulling on chemical composition and physical properties of flaxseed. LWT Food Sci. Technol. 30, 135–140. doi: 10.1006/FSTL.1996.0141
Pan, A., Demark-Wahnefried, W., Ye, X., Yu, Z., Li, H., Qi, Q., et al. (2009). Effects of a flaxseed-derived lignan supplement on C-reactive protein, IL-6 and retinol-binding protein 4 in type 2 diabetic patients. Br. J. Nutr. 101, 1145–1149. doi: 10.1017/S0007114508061527
Pan, A., Sun, J., Chen, Y., Ye, X., Li, H., Yu, Z., et al. (2007). Effects of a flaxseed-derived lignan supplement in type 2 diabetic patients: a randomized, double-blind, cross-over trial. PLoS One 2:e1148. doi: 10.1371/journal.pone.0001148
Paquette, S., Møller, B. L., and Bak, S. (2003). On the origin of family 1 plant glycosyltransferases. Phytochemistry 62, 399–413. doi: 10.1016/S0031-9422(02)00558-7
Patel, D., Vaghasiya, J., Pancholi, S. S., and Paul, A. (2012). Therapeutic potential of secoisolariciresinol diglucoside: a plant lignan. Int. J. Pharm. Sci. Drug Res. 4, 15–18.
Penumathsa, S. V., Koneru, S., Zhan, L., John, S., Menon, V. P., Prasad, K., et al. (2008). Secoisolariciresinol diglucoside induces neovascularization-mediated cardioprotection against ischemia-reperfusion injury in hypercholesterolemic myocardium. J. Mol. Cell. Cardiol. 44, 170–179. doi: 10.1016/j.yjmcc.2007.09.014
Peterson, J., Dwyer, J., Adlercreutz, H., Scalbert, A., Jacques, P., and McCullough, M. L. (2010). Dietary lignans: physiology and potential for cardiovascular disease risk reduction. Nutr. Rev. 68, 571–603. doi: 10.1111/j.1753-4887.2010.00319.x
Pietinen, P., Stumpf, K., Männistö, S., Kataja, V., Uusitupa, M., and Adlercreutz, H. (2001). Serum enterolactone and risk of breast cancer: a case-control study in eastern Finland. Cancer Epidemiol. Biomarkers Prev. 10, 339–344.
Pietrofesa, R. A., Velalopoulou, A., Arguiri, E., Menges, C. W., Testa, J. R., Hwang, W.-T., et al. (2016). Flaxseed lignans enriched in secoisolariciresinol diglucoside prevent acute asbestos-induced peritoneal inflammation in mice. Carcinogenesis 37, 177–187. doi: 10.1093/carcin/bgv174
Pilar, B., Güllich, A., Oliveira, P., Ströher, D., Piccoli, J., and Manfredini, V. (2017). Protective role of flaxseed oil and flaxseed lignan secoisolariciresinol diglucoside against oxidative stress in rats with metabolic syndrome. J. Food Sci. 82, 3029–3036. doi: 10.1111/1750-3841.13964
Prasad, K. (1997). Hydroxyl radical-scavenging property of secoisolariciresinol diglucoside (SDG) isolated from flax-seed. Mol. Cell. Biochem. 168, 117–123. doi: 10.1023/A:1006847310741
Prasad, K. (2000a). Antioxidant activity of secoisolariciresinol diglucoside-derived metabolites, secoisolariciresinol, enterodiol, and enterolactone. Int. J. Angiol. 9, 220–225.
Prasad, K. (2000b). Oxidative stress as a mechanism of diabetes in diabetic BB prone rats: effect of secoisolariciresinol diglucoside (SDG). Mol. Cell. Biochem. 209, 89–96. doi: 10.1023/A:1007079802459
Prasad, K. (2001). Secoisolariciresinol diglucoside from flaxseed delays the development of type 2 diabetes in Zucker rat. J. Lab. Clin. Med. 138, 32–39. doi: 10.1067/mlc.2001.115717
Prasad, K. (2005). Hypocholesterolemic and antiatherosclerotic effect of flax lignan complex isolated from flaxseed. Atherosclerosis 179, 269–275. doi: 10.1016/j.atherosclerosis.2004.11.012
Prasad, K. (2007). A study on regression of hypercholesterolemic atherosclerosis in rabbits by flax lignan complex. J. Cardiovasc. Pharmacol. Ther. 12, 304–313. doi: 10.1177/1074248407307853
Prasad, K. (2008). Regression of hypercholesterolemic atherosclerosis in rabbits by secoisolariciresinol diglucoside isolated from flaxseed. Atherosclerosis 197, 34–42. doi: 10.1016/j.atherosclerosis.2007.07.043
Prasad, K. (2009). Flax lignan complex slows down the progression of atherosclerosis in hyperlipidemic rabbits. J. Cardiovasc. Pharmacol. Ther. 14, 38–48. doi: 10.1177/1074248408330541
Prasad, K., and Dhar, A. (2015). Flaxseed and diabetes. Curr. Pharm. Des. 22, 141–144. doi: 10.2174/1381612822666151112151230
Prasad, K., and Jadhav, A. (2015). Prevention and treatment of atherosclerosis with flaxseed -derived compound secoisolariciresinol diglucoside. Curr. Pharm. Des. 22, 214–220. doi: 10.2174/1381612822666151112151130
Prasad, K., Mantha, S., Muir, A., and Westcott, N. (2000). Protective effect of secoisolariciresinol diglucoside against streptozotocin-induced diabetes and its mechanism. Mol. Cell. Biochem. 206, 141–150. doi: 10.1023/A:1007018030524
Prasad, K., Mantha, S. V., Muir, A. D., and Westcott, N. D. (1998). Reduction of hypercholesterolemic atherosclerosis by CDC-flaxseed with very low alpha-linolenic acid. Atherosclerosis 136, 367–375. doi: 10.1016/S0021-9150(97)00239-6
Puukila, S., Fernandes, R. O., Türck, P., Carraro, C. C., Bonetto, J. H. P., de Lima-Seolin, B. G., et al. (2017). Secoisolariciresinol diglucoside attenuates cardiac hypertrophy and oxidative stress in monocrotaline-induced right heart dysfunction. Mol. Cell. Biochem. 432, 33–39. doi: 10.1007/s11010-017-2995-z
Ross, J., Li, Y., Lim, E.-K., and Bowles, D. J. (2001). Higher plant glycosyltransferases. Genome Biol. 2, REVIEWS3004. doi: 10.1186/gb-2001-2-2-reviews3004
Sano, T., Oda, E., Yamashita, T., Shiramasa, H., Ijiri, Y., Yamashita, T., et al. (2003). Antithrombic and anti-atherogenic effects of partially defatted flaxseed meal using a laser-induced thrombosis test in apolipoprotein E and low-density lipoprotein receptor deficient mice. Blood Coagul. Fibrinolysis 14, 707–712. doi: 10.1097/01.mbc.0000061370.39736.ee
Schmidt, T. J., Klaes, M., and Sendker, J. (2012). Lignans in seeds of Linum species. Phytochemistry 82, 89–99. doi: 10.1016/j.phytochem.2012.07.004
Shareef, M., and Sarfraz, M. (2016). Natural cures for breast cancer treatment. Saudi Pharm. J. 24, 233–240. doi: 10.1016/J.JSPS.2016.04.018
Spence, J. D., Thornton, T., Muir, A. D., and Westcott, N. D. (2003). The effect of flax seed cultivars with differing content of alpha-linolenic acid and lignans on responses to mental stress. J. Am. Coll. Nutr. 22, 494–501. doi: 10.1080/07315724.2003.10719327
Struijs, K. (2008). The Lignan Macromolecule from Flaxseed. Structure and Bioconversion of Lignans. Ph.D. thesis, Wageningen University, Wageningen.
Struijs, K., Vincken, J.-P., Verhoef, R., Van Oostveen-Van Casteren, W. H. M., Voragen, A. G. J., and Gruppen, H. (2006). The flavonoid herbacetin diglucoside as a constituent of the lignan macromolecule from flaxseed hulls. Phytochemistry 68, 1227–1235. doi: 10.1016/j.phytochem.2006.10.022
Suzuki, S., and Umezawa, T. (2007). Biosynthesis of lignans and norlignans. J. Wood Sci. 53, 273–284. doi: 10.1007/s10086-007-0892-x
Tan, K. P., Chen, J., Ward, W. E., and Thompson, L. U. (2004). Mammary gland morphogenesis is enhanced by exposure to flaxseed or its major lignan during suckling in rats. Exp. Biol. Med. 229, 147–157. doi: 10.1177/153537020422900203
Tou, J. C., Chen, J., and Thompson, L. U. (1998). Flaxseed and its lignan precursor, secoisolariciresinol diglycoside, affect pregnancy outcome and reproductive development in rats. J. Nutr. 128, 1861–1868. doi: 10.1093/jn/128.11.1861
Touré, A., and Xueming, X. (2010). Flaxseed lignans: source, biosynthesis, metabolism, antioxidant activity, Bio-active components, and health benefits. Compr. Rev. Food Sci. Food Saf. 9, 261–269. doi: 10.1111/j.1541-4337.2009.00105.x
Umezawa, T. (2003). Diversity in lignan biosynthesis. Phytochem. Rev. 2, 371–390. doi: 10.1023/B:PHYT.0000045487.02836.32
Umezawa, T., Yamamoto, E., and Lewis, N. G. (1990). Lignan biosynthesis in forsythia species. J. Chem. Soc. Chem. Commun. 20, 1405–1408. doi: 10.1039/c39900001405
Von Heimendahl, C. B. I., Schäfer, K. M., Eklund, P., Sjöholm, R., Schmidt, T. J., and Fuss, E. (2005). Pinoresinol-lariciresinol reductases with different stereospecificity from Linum album and Linum usitatissimum. Phytochemistry 66, 1254–1263. doi: 10.1016/j.phytochem.2005.04.026
Wang, J., and Hou, B. (2009). Glycosyltransferases: key players involved in the modification of plant secondary metabolites. Front. Biol. China 4, 39–46. doi: 10.1007/s11515-008-0111-1
Ward, W. E., Jiang, F. O., and Thompson, L. U. (2000). Exposure to flaxseed or purified lignan during lactation influences rat mammary gland structures. Nutr. Cancer 37, 187–192. doi: 10.1207/S15327914NC372_11
Webb, A. L., and McCullough, M. L. (2005). Dietary lignans: potential role in cancer prevention. Nutr. Cancer 51, 117–131. doi: 10.1207/s15327914nc5102_1
Wiesenborn, D., Tostenson, K., and Kangas, N. (2003). Continuous abrasive method for mechanically fractionating flaxseed. J. Am. Oil Chem. Soc. 80, 295–300. doi: 10.1007/s11746-003-0692-2
Witte, S., Moco, S., Vervoort, J., Matern, U., and Martens, S. (2009). Recombinant expression and functional characterisation of regiospecific flavonoid glucosyltransferases from Hieracium pilosella L. Planta 229, 1135–1146. doi: 10.1007/s00425-009-0902-x
Xia, Z.-Q., Costa, M. A., Pélissier, H. C., Davin, L. B., and Lewis, N. G. (2001). Secoisolariciresinol dehydrogenase purification, cloning, and functional expression. J. Biol. Chem. 276, 12614–12623. doi: 10.1074/jbc.M008622200
Zanwar, A. A., Hegde, M. V., and Bodhankar, S. L. (2013). “Flax lignan in the prevention of atherosclerotic cardiovascular diseases,” in Polyphenols in Human Health and Disease, eds R. Watson, V. Preedy, and S. Zibadi (Amsterdam: Elsevier), 915–921. doi: 10.1016/B978-0-12-398456-2.00071-2
Zanwar, A. A., Hegde, M. V., Rojatkar, S. R., Sonawane, K. B., Rajamohanan, P. R., and Bodhankar, S. L. (2014). Isolation, characterization and antihyperlipidemic activity of secoisolariciresinol diglucoside in poloxamer-407-induced experimental hyperlipidemia. Pharm. Biol. 52, 1094–1103. doi: 10.3109/13880209.2013.877492
Zhang, L. Y., Wang, X., Sun, D., Liu, X., Hu, X., and Kong, F. (2008). Regulation of zinc transporters by dietary flaxseed lignan in human breast cancer xenografts. Mol. Biol. Rep. 35, 595–600. doi: 10.1007/s11033-007-9129-8
Keywords: lignans, flaxseed, glycosyltransferases, Linum, pinoresinol-lariciresinol reductase, secoisolariciresinol diglucoside, UGT74S1, health benefits
Citation: Kezimana P, Dmitriev AA, Kudryavtseva AV, Romanova EV and Melnikova NV (2018) Secoisolariciresinol Diglucoside of Flaxseed and Its Metabolites: Biosynthesis and Potential for Nutraceuticals. Front. Genet. 9:641. doi: 10.3389/fgene.2018.00641
Received: 31 August 2018; Accepted: 27 November 2018;
Published: 12 December 2018.
Edited by:
Sami Dridi, University of Arkansas, United StatesReviewed by:
Sun-Ok Lee, University of Arkansas, United StatesWimal Pathmasiri, University of North Carolina at Chapel Hill, United States
Copyright © 2018 Kezimana, Dmitriev, Kudryavtseva, Romanova and Melnikova. This is an open-access article distributed under the terms of the Creative Commons Attribution License (CC BY). The use, distribution or reproduction in other forums is permitted, provided the original author(s) and the copyright owner(s) are credited and that the original publication in this journal is cited, in accordance with accepted academic practice. No use, distribution or reproduction is permitted which does not comply with these terms.
*Correspondence: Parfait Kezimana, k1par@mail.ru; kezipar@outlook.com Nataliya V. Melnikova, mnv-4529264@yandex.ru