- 1Science and Technology Branch, Environment Canada and Climate Change, Montreal, QC, Canada
- 2Ouranos, Montréal, QC, Canada
- 3Carbone Boréal, Département des Sciences Fondamentales, Université du Québec à Chicoutimi, Saguenay, QC, Canada
- 4Centre d’Étude de la Forêt, Université du Québec à Montréal, Montréal, QC, Canada
- 5Service Canadien des Forêts, Centre de Foresterie des Laurentides, Sainte-Foy, QC, Canada
- 6Independent Researcher, Lévis, QC, Canada
Increases in mean annual air temperature (MAAT) and mean annual precipitation (MAP) are projected for north eastern North America, which will alter soil hydro-climatic conditions and hence the rate of many soil processes. Among them, chemical weathering of soil minerals is an essential source of base cations (BC) which controls the acid-base status of surface waters and is crucial for forest nutrition. In this modeling study, MAAT and MAP projections from a regional and a global climate models were first used to project changes in soil temperature (MAST) and soil water content (SWC) with the ForStem and ForHym models for 21 eastern Canadian forested catchments. The models predicted an increase in MAST by 2.03–2.05°C and 2.87–3.42°C for the 2041–2070 and 2071–2100 periods, respectively, and a small decrease (<5%) in SWC. In a second step, these projected changes in MAST and SWC were used to estimate changes in BC weathering rates (WR) and soil pH with the geochemical model PROFILE. The simulations indicated that BC WR would increase by 13–15% and 20–22% for the 2041–2070 and 2071–2100 periods, respectively. The increase in BC WR was accompanied by an increase not only in base cation concentrations, but also in soil pH at most sites, suggesting that future temperature increase has the potential to ameliorate the acid-base status and the fertility of soils in eastern Canada through its impact on WR.
Introduction
Recent climatic simulations have shown that mean annual air temperature (MAAT) may rise by up to 4–7°C by the end of the century relative to the 1971–2000 period under a scenario of high greenhouse gas (GHG) emissions (RCP 8.5) in southern Québec, Canada (Ouranos, 2015). This increase will impact soil temperature and moisture, two variables that play a key role in several processes in forest ecosystems, including soil organic matter (SOM) mineralization (Melillo et al., 2002; Davidson and Janssens, 2006), nitrification (Gessler et al., 2004; Groffman et al., 2009; Durán et al., 2014), tree growth and forest productivity (Gessler et al., 2004; Hernandez-Santana et al., 2009) and forest fire intensity and frequency (Rennenberg et al., 2006). Over long periods of time, such changes could also affect the distribution and composition of forests. In addition, soil temperature and moisture have a strong impact on the rate of chemical weathering of soil minerals (White and Blum, 1995), which is the main source of essential nutrients for tree nutrition such as base cations (BC) and other essential elements (e.g., P, Fe, Mn). A 44-year study in Iceland has shown a positive effect of MAAT on chemical and mechanical weathering rates (WRs) in glacial catchments (Gislason et al., 2009). Higher weathering rates driven by increasing temperatures was also suggested to explain increased alkalinity and pH of lake waters for 47 temperate and boreal catchments in the province of Quebec, Canada (Houle et al., 2010). More recently, it was shown that MAAT and precipitation had a positive effect on BC budget and concentrations in lake water across 72 forested catchments of eastern Canada (Augustin et al., 2015b). Results reported by Li et al. (2016) also showed a strong positive correlation between mean annual temperature and the rate of CO2 consumption associated with the weathering of basaltic rocks. Although these studies suggest an increase in chemical weathering rates in response to climate warming, potential reduction of soil moisture resulting from increased evapotranspiration (Milly, 1992) associated with higher temperature may nevertheless counteract the increase in soil processes due to warmer temperatures. Such a mechanism has been reported for microbial SOM mineralization and nitrification rates (Venterea et al., 2003; Groffman et al., 2009; Durán et al., 2014).
A wide range of methodological approaches have been developed to quantify BC WRs (Augustin et al., 2016). These include soil profile mass balance calculations (Brimhall et al., 1991; Egli and Fitze, 2000), watershed input-output budgets (Clayton, 1979; Velbel, 1985), laboratory dissolution experiments (Chou and Wollast, 1984; Oelkers et al., 1994), test-mineral techniques (Ranger et al., 1990), strontium isotope ratio methods (Åberg et al., 1989), as well as geochemical modeling using the MAGIC (Cosby et al., 2001) or PROFILE models (Warfvinge and Sverdrup, 1992). The geochemical model PROFILE is widely used to evaluate chemical and geochemical processes involved in forest soils and watersheds (Koptsik et al., 1999; Duan et al., 2002; Duchesne and Houle, 2006; Sverdrup, 2009; Erlandson Lampa et al., 2020). Previous works have shown that PROFILE is enough powerful to reproduce spatial geographical gradients in WRs for relatively large areas with contrasted mineralogy (Houle et al., 2012b; Augustin et al., 2016), while yielding reliable BC WRs (Jönsson et al., 1995). Since soil temperature and moisture both greatly affect the chemical, physical, and biological processes that are occurring in soils, it is essential to better understand how climate change will affect resulting future soil conditions. However, changes in soil moisture and temperature cannot be directly deduced from projected changes in air temperature or precipitation. For example, an increase in winter air temperature would have a slight impact on soil temperature because of the insulation properties of the snowpack. Although global and regional climate models have a land surface scheme that can diagnose soil temperature and moisture, their use is limited for site-specific studies because their spatial resolution is too coarse to allow taking the characteristics of soils specific to each site into account (presence of organic soils, organic soil depth, mineral soil depth, root zone depth, clay content, soil porosity, vegetation type, etc.). The objectives of this study were thus: (1) to predict climate change impact on soil moisture and temperature at 21 forested catchments of the Quebec portion of the Long-Range Transport of Airborne Pollutants (LRTAP) program; and (2) to simulate future BC WRs, soil pH, and BC availability associated with changes in soil temperature and moisture by using the geochemical model PROFILE.
Materials and Methods
Study Sites and Sampling
The study took place in 21 forested watersheds of the LRTAP-Quebec network. All watersheds are located in the temperate and boreal forests of the province of Quebec, Canada, within a ∼90 000 km2 area defined as a 150 km wide strip, parallel to the St. Lawrence River (Figure 1) and it has been extensively described in previous studies (Houle et al., 2012b; Augustin et al., 2015a, 2016). In this region, the Canadian Shield is composed of bedrocks formed during the Precambrian, which consists mainly of igneous (granite, syenite, anorthosite) and metamorphic (gneiss, granitic gneiss, paragneiss) rocks.
Site boundaries were delimited from topographical maps analysis (1:20,000). Catchments surface areas are indicated in Houle et al. (2012b). The 21 catchments comprised no peat bogs and are protected by a provincial law against forest harvesting and major human activity. Most of them are only accessible by helicopter. The vegetation ranges from forests dominated by sugar maple (Acer saccharum Marsh.) in the southwest, to balsam fir (Abies balsamea L.) or black spruce (Picea mariana Mill.) in the northeast of the study area (Houle et al., 2006; Marty et al., 2015). The soils in the watersheds are mainly orthic humo-ferric or ferro-humic podzols. These types of soils are typically shallow, covered with a humus layer of 7–10 cm underlained by a thin (sometime discontinuous) eluviated Ae horizon and with a thick B-horizon (Houle et al., 2012b). The thickness of the till deposit is not exactly known for each catchment but it typically reaches 2–4 m (including the developed soil profile) at mid-slope. The C-horizon, which gets rapidly heavily compacted with depth, lies on the impermeable rock of the Canadian Shield.
Soil sampling was conducted during the summer and fall of 2001 and 2002. Details about sampling procedure can be found elsewhere (Augustin et al., 2015a; Marty et al., 2015, 2017). Briefly, three soil pits were dug to a depth of about 1 m within each site. The soil profiles locations were evenly distributed on flat surfaces around the lake perimeter, approximately 50 m from the lake shore. Percent volume of large stone was visually estimated in the field. The Munsell Soil Color Chart (Munsell Soil Color Charts, 1994) were used to characterize the soil colors. The organic soil layers (L, F, and H) were sampled with a 177 cm2 sampling frame up to the top of the mineral horizons. The thickness of both organic and mineral horizons was measured and soils were sampled by genetic horizons. Main mineral horizons were also core sampled (diameter, 53 mm; length, 60 mm) to determine bulk density and their thickness measured in the field.
Climate Models and Projections of Soil Moisture and Temperature
Average monthly air temperatures, rainfall and the fraction of precipitation falling as snow were generated for each catchment over the reference period (1971–2000) with the BioSIM model (Régnière and Bolstad, 1994; Régnière and St-Amant, 2007), based on historical observations as described in Houle et al. (2012a). For each catchment, simulations of the same variables were also performed for the reference as well as two other distinct periods (2041–2070, and 2071–2100), using two climate models: (i) the global model ECHAM 5 (member 1 SRES A1B) (300 × 300 km grid) that has a median behavior among a large set of global climate models (Figure 3); and (ii) the Canadian Regional Climate Model (CRCM 4.2.3 AET CGCM3.1v2 member 4 SRES A2) that was developed within the framework of the existing global climate modeling (Scinocca et al., 2016). MAAT and MAP were then computed for the reference and the two other distinct periods. Changes in MAAT and MAP were expressed as the difference between values simulated for the 2041–2070 and 2071–2100 periods on the one hand, and the reference period (1971–2000) on the other hand.
Climate data derived from BioSIM as well as both the regional and global models described above were used as inputs in the forest hydrology model ForHyM and the forest soil temperature model ForSTeM to simulate mean annual soil temperature (MAST) and soil water content (SWC), respectively, for the reference (1971–2000) as well as the 2041–2070 and the 2071–2100 periods (Arp and Yin, 1992; Yin and Arp, 1993; Houle et al., 2002, 2012a). The ForHyM sub-model simulates variations in soil water content and the main hydrological flows among the different compartments of a forest ecosystem. Regarding the ForSTeM sub-model, it simulates soil temperatures at the desired depths (here within the rooting zone, at ∼28 cm depth), while accounting for the latent heat transfers due to freeze-thaw events as well as changes in the thermal properties of the soil. The two models were calibrated for three distinct forest ecosystems (maple, fir and spruce) based on site characteristics (canopy density, soil texture, horizon depths, extent of root zone, etc.) and on long-term and high temporal resolution measurements of soil temperature and humidity at different depths (Houle et al., 2012b). The model performance was very good with low calibration effort, and it has been observed that calibration had a very low impact on future soil temperature and SWC projections (Houle et al., 2012b). Changes in SWC and MAST were computed as the difference between values simulated for the 2041–2070 and 2071–2100 periods on the one hand, and the reference period on the other hand.
Soil Solution Chemistry and Mineral Weathering Rate Estimates
Soil solution pH and BC concentration, as well as BC weathering rate (WR) were simulated for the 62 pedons distributed within the 21 catchments (2–3 pedons per site), using the geochemical model PROFILE (Warfvinge and Sverdrup, 1992; Sverdrup and Warfvinge, 1995, 1999). This model requires several input data, such as climate conditions, soil properties (e.g., mineralogical composition, specific surface area, soil moisture, soil temperature) and vegetation cover characteristics. Data acquisition methods regarding soil properties and site characteristics for the 21 watersheds were described elsewhere (Houle et al., 2012b; Augustin et al., 2015a). Only a brief description is provided here for some parameters. Determination of soil texture distribution (clay, sand and silt) was conducted on air-dried soil samples sieved through a 2 mm-sieve as described in Augustin et al. (2015a). Specific soil surface area was calculated from measured grain size distribution, dry bulk density, and coarse fragments according to Jönsson et al. (1995). Total elemental concentrations in soil was obtained by X-ray fluorescence (XRF) spectrometry, a non-consumptive technique for multi-element determination. These data were then used to quantitatively estimate the mineralogical composition of the soil samples with the stoichiometric model UPPSALA (Sverdrup, 1990; Sandén and Warfvinge, 1992; Houle et al., 2012b; Augustin et al., 2015a). Soil water content and temperature used in PROFILE were simulated with ForHyM and ForSTeM as described above.
Results
Baseline and Projected Climate and Soil Hydro-Climatic Conditions
The 21 sites exhibited substantial ranges in MAAT (−1.1 to 5.1°C) and MAP (960–1,600 mm) (Table 1). MAST and SWC, respectively, ranged from 1.98 to 6.24°C and from 0.159 to 0.269 m3 m–3. For the 21 watersheds, average increases in MAAT of 3.01 ± 0.03°C and 4.38 ± 0.11°C were projected with the global model (ECHAM5) for the 2041–2070 and 2071–2100 periods, respectively, whereas the regional model (CRCM) projected increases by 2.85 ± 0.06°C and 4.92 ± 0.09°C, respectively (Figure 2A). ECHAM 5 also predicted average increases in MAP by 10.7 and 13.8% (125 ± 29 mm and 160 ± 28 mm) for the 2041–2070 and 2071–2100 periods respectively, whereas CRCM predicted increases by 9.0 and 12.8% (104 ± 20 mm and 147 ± 21 mm), respectively (Figure 2B). The projected MAAT and MAP values by the two models are close to the median values of 11 other simulations under high and low GHG emissions scenarios (RCP4.5 and RCP8.5, respectively) (Figure 3). When fed with the results of the climate models, the ForSTeM-ForHyM models projected an increase in MAST by 2.05 ± 0.25°C and 2.03 ± 0.22°C for the 2041–2070 and by 3.42 ± 0.44°C and 2.87 ± 0.30°C for the 2071–2100 periods relative to the reference period (Figure 2C), while only small changes in SWC (<5%) were projected for both models and periods (Figure 2D).
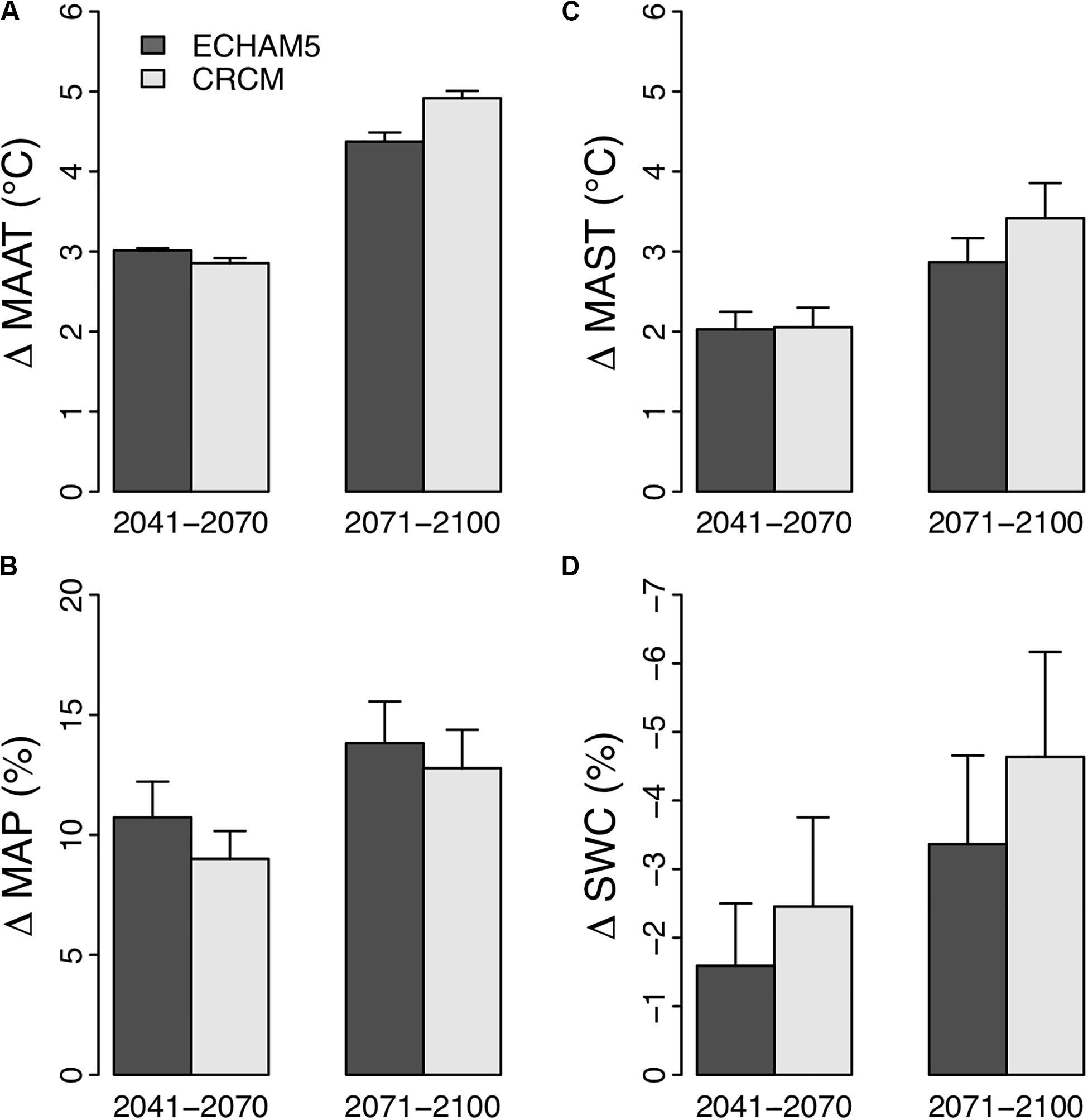
Figure 2. Changes in mean annual air temperature (MAAT) (A), mean annual precipitation (MAP) (B), mean annual soil temperature (MAST) (C) and soil water content (SWC) (D) between the reference period (1971–2000) and the 2041–2070 and 2071–2100 periods. MAAT and MAP values were projected by the ECHAM 5 (global) and CRCM (regional) climate models and were used as inputs in the FORHYM-FORSTEM model for MAST and SWC projections.
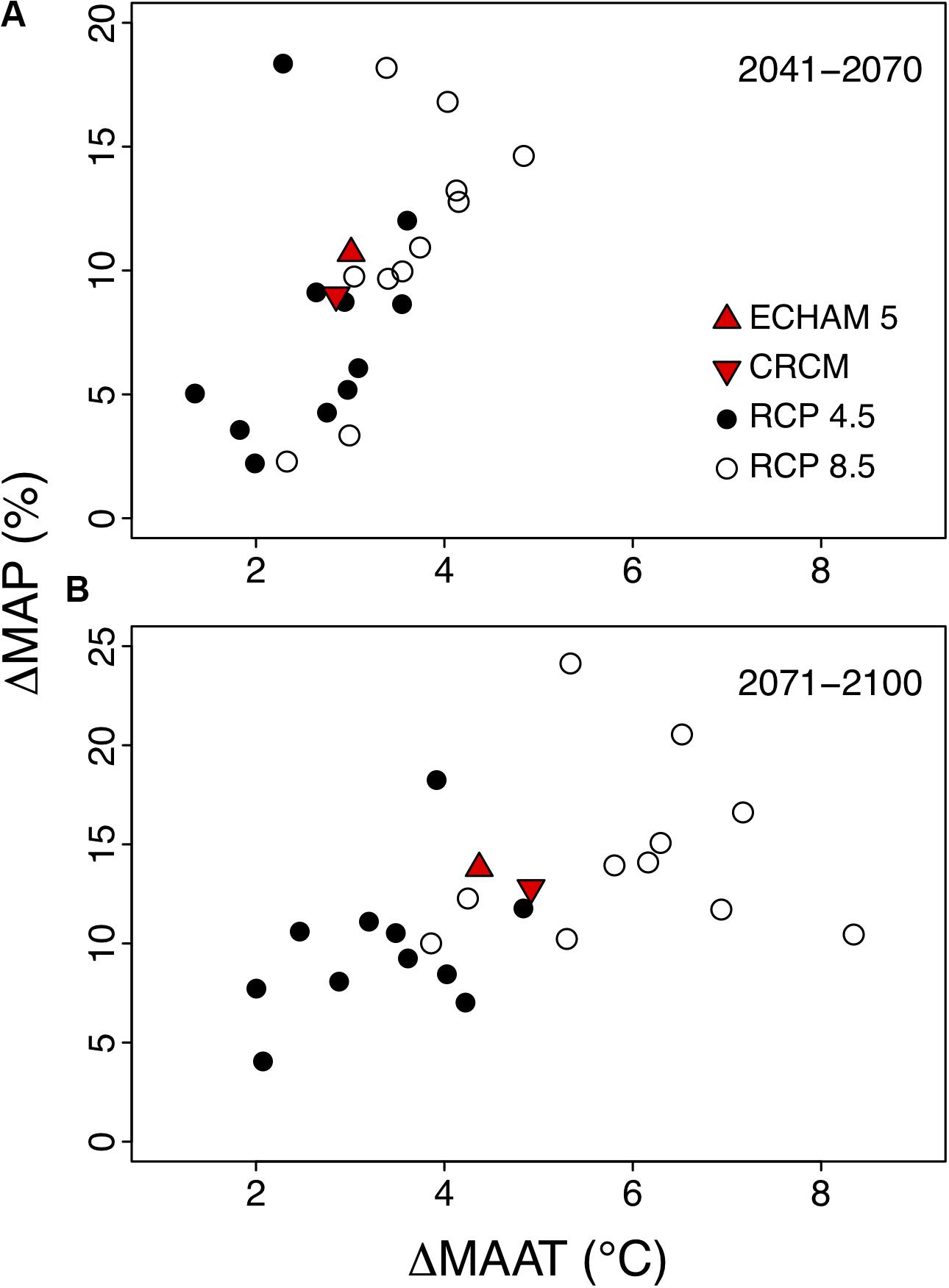
Figure 3. Relative changes in mean annual precipitation (MAP) and mean annual air temperature (MAAT) for the study region as projected by 11 models under two GHG emission scenarios (RCP 4.5 and RCP 8.5) for the periods 2041–2070 (A) and 2071–2100 (B). Projected changes by the climate models used in this study for coupled simulations (ECHAM 5 and CRCM) are also shown on the graphs. The 11 reference models are: ACCESS1.3, BNU-ESM, CMCC-CMS, CanESM2, GFDL-ESM2M, HadGEM2, INM-CM4, IPSL-CM5A-LR, IPSL-CM5B-LR, MPIESM-LR, and NorESM.
Base Cation Weathering Rate Projections
Weathering rates estimates reported here for the reference period (1971–2000) are presented in Table 2. The obtained results are close to those reported by Houle et al. (2012a) but differed slightly because the climate inputs were slightly different for the time period considered, i.e., 1971–2000 here as compared to 1997–2002 for Houle et al. (2012a). Across the study sites, total BC WRs simulated using PROFILE ranged from 0.54 to 4.36 kmolc ha–1 yr–1 and averaged 1.70 ± 1.11 kmolc ha–1 yr–1 for the reference period (Table 2). The simulations showed large ranges in individual base cation weathering rates across the 21 sites, especially for Ca (0.21–3.39 kmolc ha–1 yr–1).

Table 2. Ranges and mean (SD) values for base cations weathering rates (WR; kmolc ha–1 yr–1) during the reference period (1971–2000) as simulated by PROFILE.
Projected increases in the sum of BC WRs averaged 13–15% and 20–22% for the 2041–2070 and 2071–2100 periods, respectively (Figure 4A). Ca and Mg increases ranged from 13 to 16% (2041–2070) and from 20 to 24% (2071–2100) (Figures 4B,C), but were slightly lower for K and Na with values of 11–13% and 17–19% for the 2041–2070 and 2071–2100 periods, respectively (Figures 4D,E).
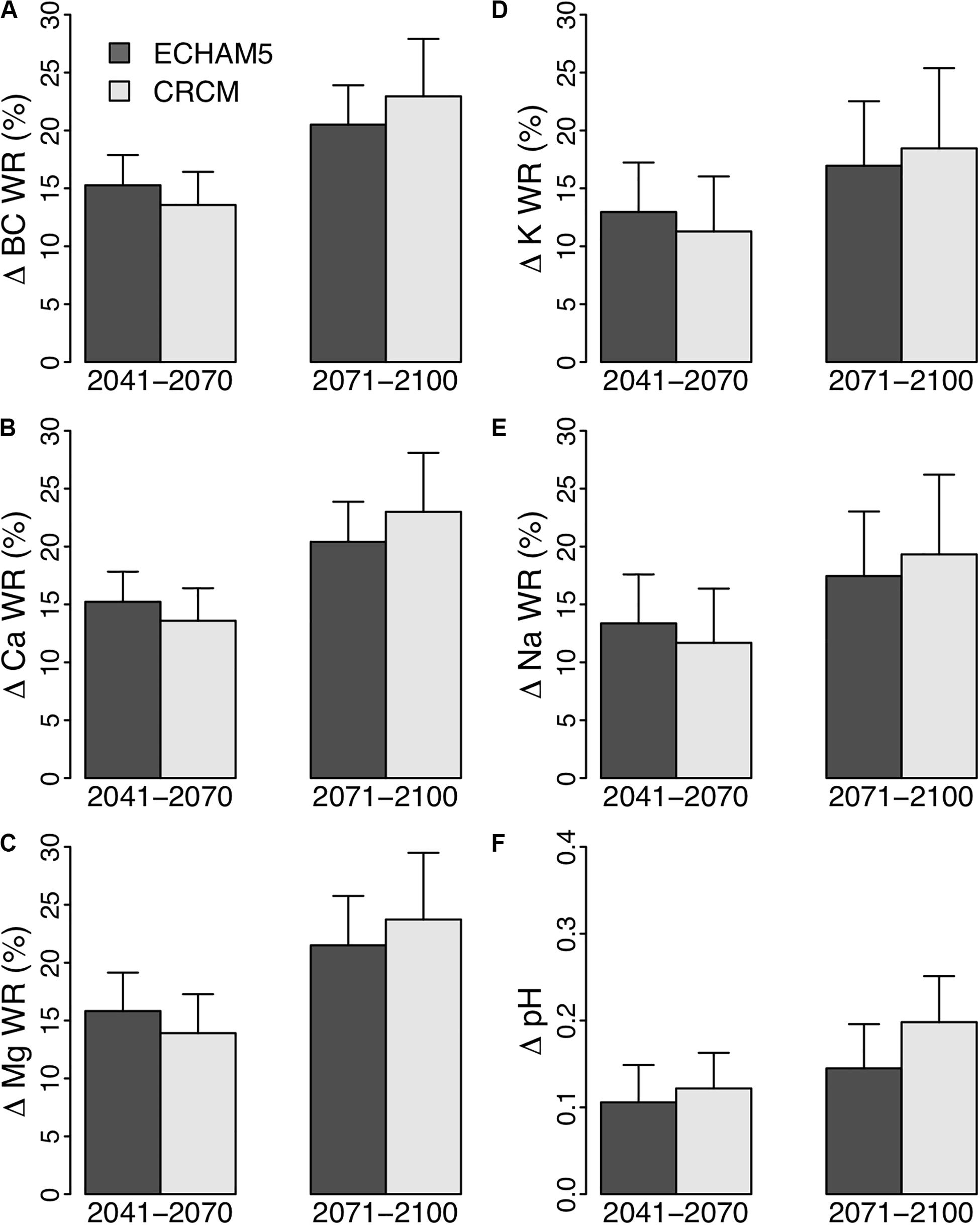
Figure 4. Mean (±SD) relative changes in base cation weathering rates (ΔWR; %) (A–E) and pH (F) for the periods 2041–2070 and 2071–2100 relative to the reference period (1971–2000) with climate projections generated by the ECHAM 5 (global) and CRCM (regional) climate models.
Simulated pH and Base Cation Concentrations in the Soil Solution
There was a large variation in BC concentrations in soils among sites (Table 1). Among BC, Ca2+ had the largest contribution to total BC concentration (range of 41–715 μmolc l–1; average of 190 ± 194 μmolc l–1), followed by Mg2+ (range of 24–151 μmolc l–1; average of 67 ± 41 μmolc l–1), Na+ (range of 15–106 μmolc l–1; average of 48 ± 25 μmolc l–1) and K+ (range of 8–32 μmolc l–1; average of 20 ± 7 μmolc l–1). The sum of BC concentration simulated for each site is strongly correlated to total lake BC concentration (Figure 5) showing that the model is able to reproduce the spatial variability pattern within the study area although simulated values were slightly higher. Model simulations indicate that soil BC concentrations would increase in the future (Figure 6). However, the magnitude of the projected increases varied markedly among sites, cations and climate projections. The increase was more pronounced with CRCM than ECHAM 5 climate projections, and for Ca2+, Mg2+ and K+ than for Na+. On average, Ca2+, Mg2+ and K+ concentrations in the soil would increase by 8–21% and 15–35% respectively in 2041–2070 and 2071–2100 relative to the reference period, and by slightly less for Na+ (6–17% and 13–27%). The increased BC concentration resulted in a slight average increase in soil pH from 5.5 ± 0.6 (reference period) to 5.6 ± 0.6 (2041–2070) and 5.7 ± 0.6 (2071–2100) (Figure 4F).
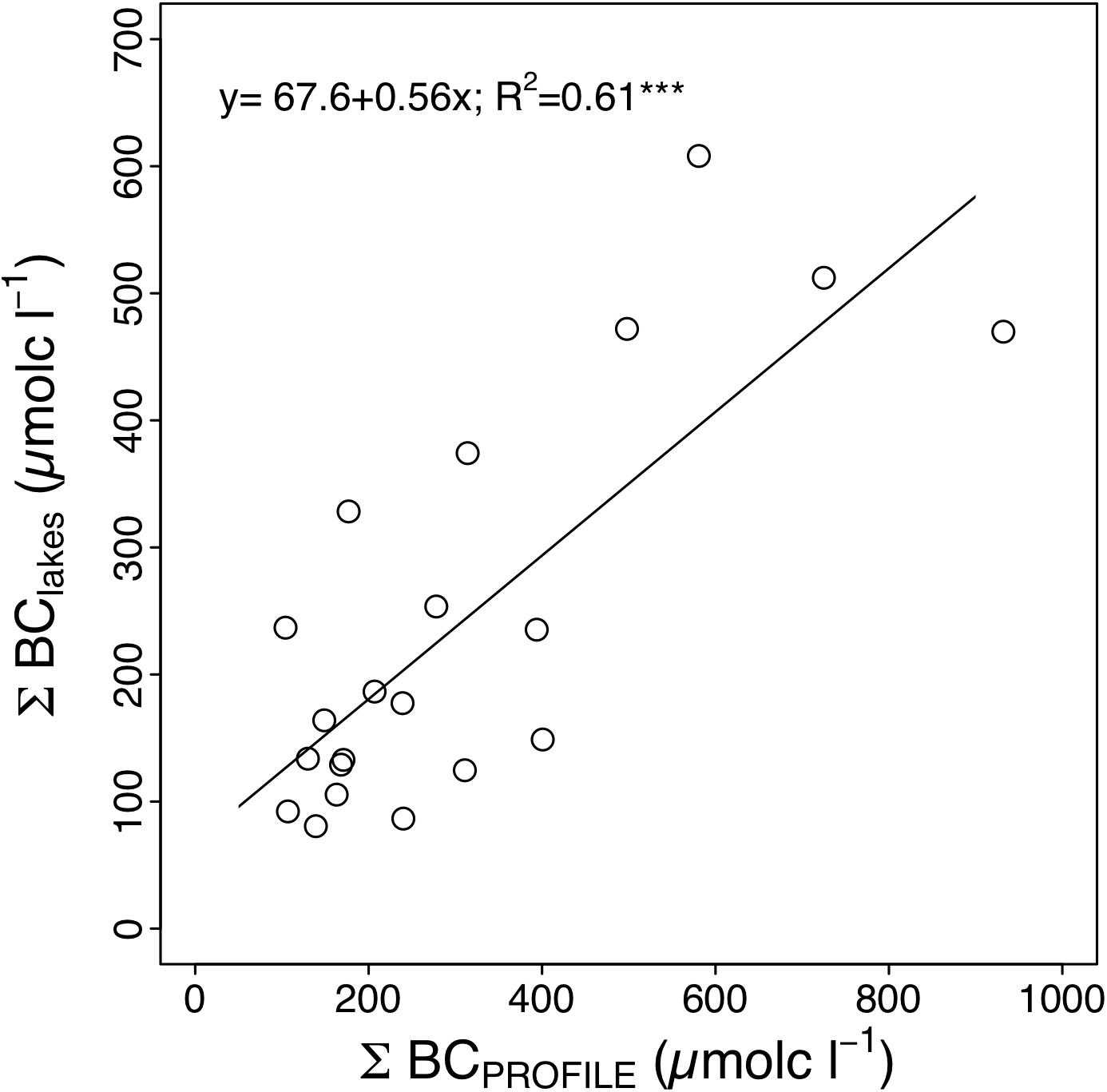
Figure 5. Relationship between projected (Σ BCPROFILE; μmolc l–1) base cation concentrations in the soil solution and measured base cation concentrations in lake water (Σ BClakes; μmolc l–1) at the study for the reference period (1971–2000).
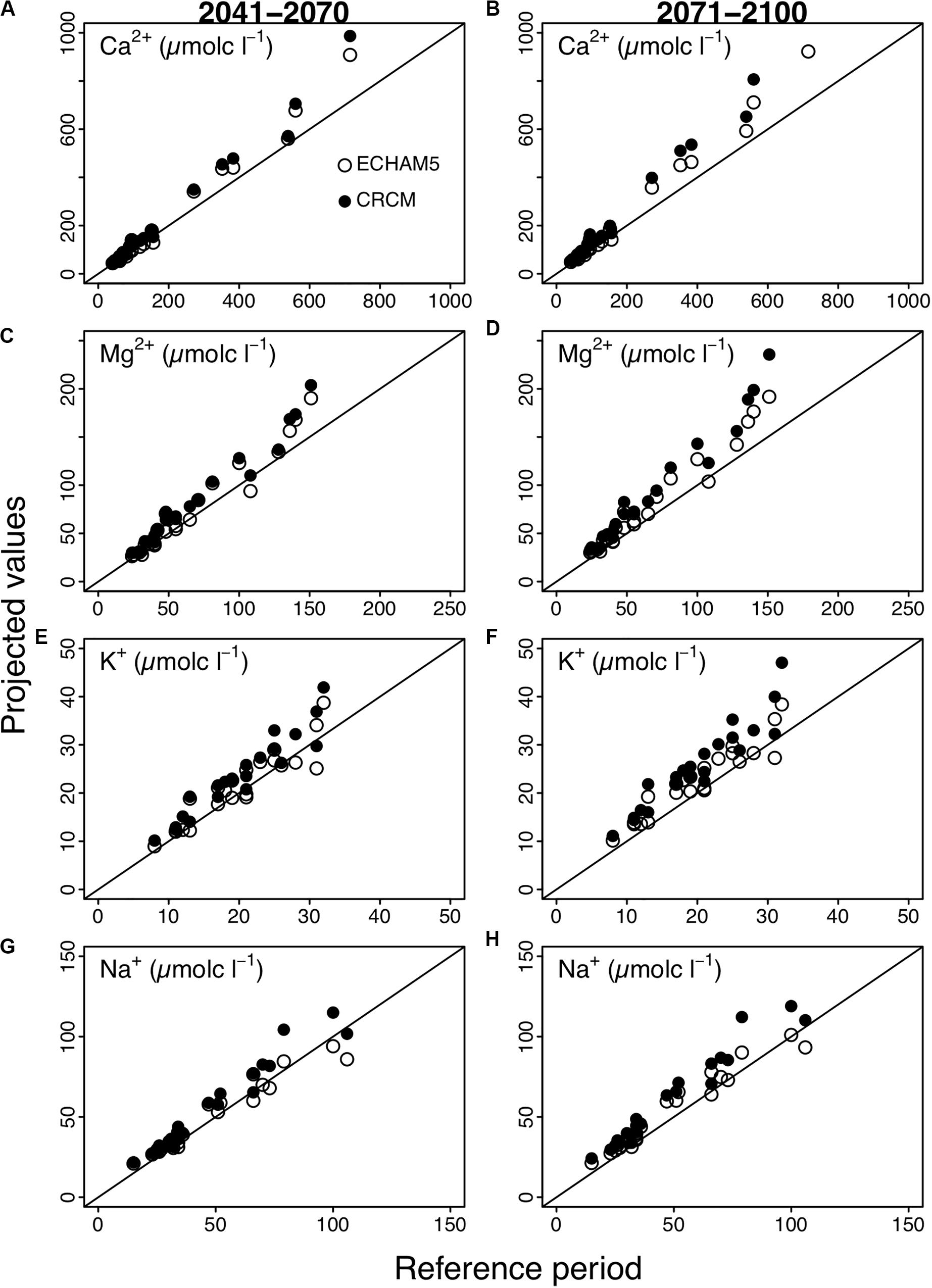
Figure 6. Soil base cation concentrations (μmolc l–1) for the reference period (x-axis, 1971–2000) vs. the 2041–2070 (A,C,E,G) and 2071–2100 (B,D,F,H) periods as projected by the ECHAM 5 (global) and CRCM (regional) climate models. The full line is the 1:1 line.
Discussion
The average increases in mean annual temperature (MAAT) and mean annual precipitation (MAP) projected by the CRCM and ECHAM 5 climate models (+2.9 and 4.8°C, and +9 and 13% for the 2041–2070 and 2071–2100 periods, respectively) are very close to the median values of a large ensemble of climate simulations for both periods (Figure 3). We assessed the impact of these projected changes in MAAT and MAP on soil hydroclimatic variables, namely mean annual soil temperature (MAST) and soil water content (SWC), using forests hydrological models. Based on changes in MAST and SWC, future base cations (BC) weathering rates (WR) as well as soil pH and BC concentrations in soil solutions were simulated with the geochemical model PROFILE. We therefore estimated the potential changes in chemical WR and BC availability that would result from changes in soil hydroclimatic conditions only. We deliberately made not attempts to model the influence of other factors caused by global change that could potentially affect these variables such as changes in atmospheric nitrogen and sulfur depositions, changes in forest growth rates or changes in land use. The latter are too uncertain, especially forest dynamics which could be affected not only by increasing temperatures but also by changes in the rate of natural perturbations such as fires, insect outbreaks and droughts. We discuss the results of the study in relation to previous works and with respect to their general implications and also, limitations.
Projected Changes in Soil Hydro-Climatic Conditions
Our results indicate that climate change will result in an average MAST increase by 2°C in 2041–2070 and 3.5°C by the end of the century. These increases represent on average 69% of the increases in air temperature, mostly due to the insulating impact of the snow cover during winter (Zhang et al., 2005; Sushama et al., 2006; Jungqvist et al., 2014), which corroborates other projections for temperate and boreal soils in Canada and Scandinavian countries (Houle et al., 2012b; Jungqvist et al., 2014). Jungqvist et al. (2014) simulated the effects of climate change on soil temperature in four forested catchments along a climatic gradient in Sweden and found that future changes in winter soil temperature were rather strongly dependent on projected snow cover (Jungqvist et al., 2014), highlighting the complexity of projecting soil winter temperature in snow-dominated area (Mott et al., 2018). Our data also showed higher variability in simulated MAST data compared to MAAT. This is the result of site-specific characteristics such as topography, vegetation, soil characteristics, snow pack depth and duration. Despite a 9–15% projected increase in MAP, a small decrease in average annual soil water content (<5%) was projected for both future periods (Figure 2D) relative to the reference period because of the impact of increased MAAT on evapotranspiration (Houle et al., 2012b).
Future Changes in BC Weathering Rates
The estimates of BC WR simulated with the PROFILE model for the reference period are within the range reported for southern Quebec or similar soil types using other methods (Courchesne et al., 2002; Schaller et al., 2009; Augustin et al., 2016, 2018). The data show substantial BC WR increases (+ 11–25%) for the two future periods relative to the reference period. Our projected range of future BC WR is consistent with other studies including data published by Gislason et al. (2009) who showed positive relationships between MAAT and chemical weathering in 8 catchments in Iceland. Akselsson et al. (2016) projected weathering rate increases of 20–30% in Swedish soils in response to a soil warming of 2–3°C, which is slightly higher than our projected values. Within the conditions of our simulations (i.e., significant soil temperature increases with small decreases in soil water content), 79% of changes in BC WR was explained by changes in MAST (Figure 7) while no significant relationship was obtained between WR and SWC changes (data not shown). According to the slope of the regression line, BC WR is projected to increase by around 7% for each 1°C rise in MAST. A slightly higher value of 9% was obtained by Belyazid and Giuliana (2019) for coniferous forest soils in Sweden using the ForSAFE model.
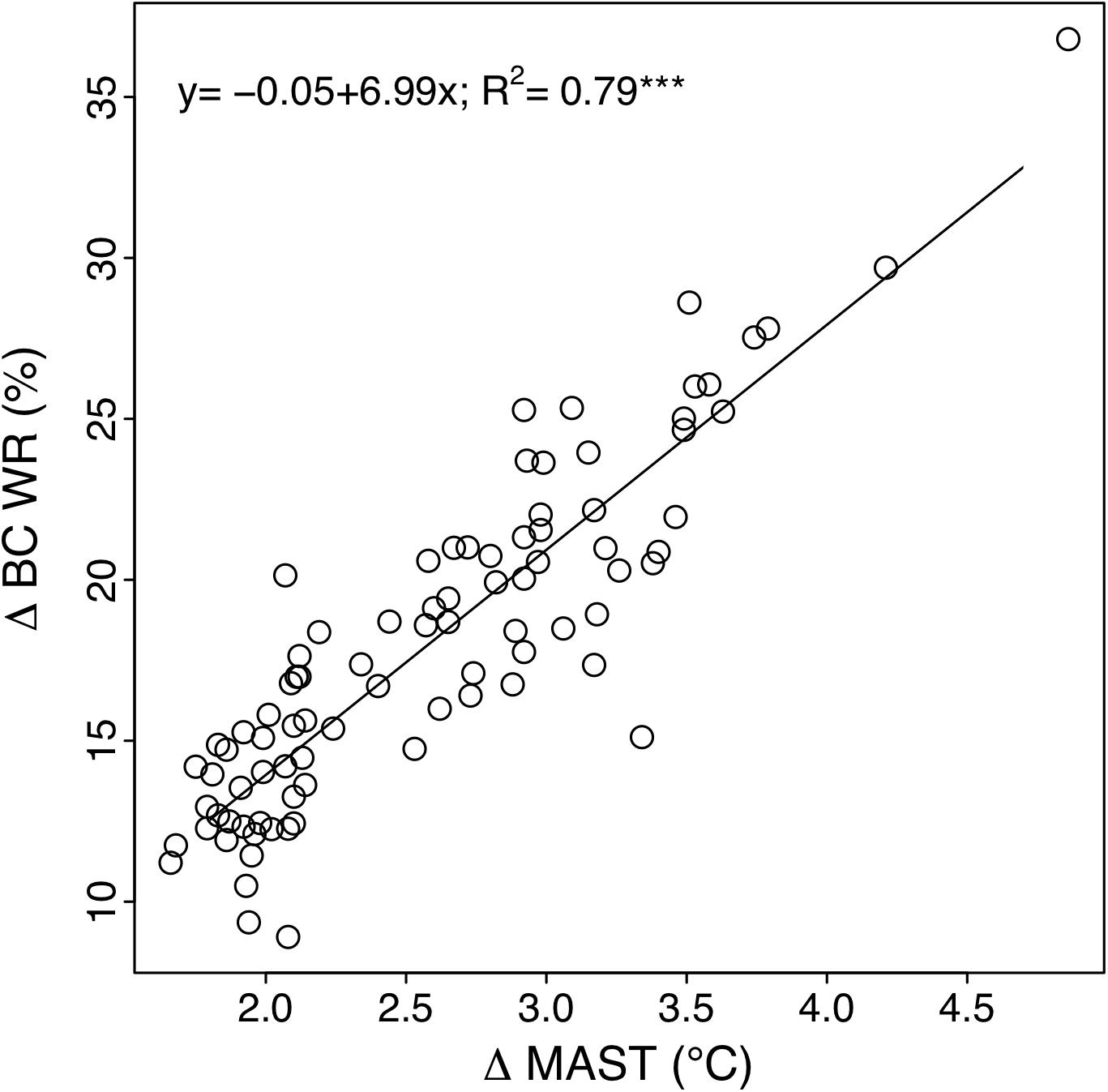
Figure 7. Relationship between changes in base cations weathering rate (ΔBC WR; %) and changes in mean annual soil temperature (Δ MAST; °C). Values are derived from PROFILE projections from the 2041–2070 and 2071–2100 periods. The line is the linear regression (P < 0.005).
However, our results may be conservative as the simulation did not take the length of the growing season into account. In cold regions, warmer air temperature lead to longer growing season (Euskirchen et al., 2006; Öquist and Laudon, 2008), reduced number of frost days and smaller duration of the snowpack (Jungqvist et al., 2014). As a result, the seasonality of biological activity in the soils would be affected (Kaiser et al., 2010, 2011), with more intense activity commencing earlier and continuing later as growing season extends. Such conditions would lengthen the period during which weathering reactions are taking place in addition to the soils being warmer, thereby potentially increasing the annual weathering rate. For forested catchments of eastern Canada, the length of the growing season was positively correlated with BC WR (Augustin et al., 2015b, 2018).
Projected Soil pH and BC Concentrations in the Soil Solution
Because soil solution and surface waters are strongly influenced by the biogeochemical processes that occur in the surrounding soils, including mineral weathering (Hairston and Fussmann, 2002), it is likely that the factors affecting BC mineral weathering in soils influence chemical properties of the soil solution (Ryan and Kahler, 1987). The projected WR increases were accompanied by increases in BC concentration in the soil solution (+6–35%). The capability of the PROFILE model to yield realistic BC concentrations (Figure 5) and to reproduce its spatial variability in the studied region (Houle et al., 2012b; Augustin et al., 2016) supports the validity of our projections. The projected increases by up to 31% in Ca2+ concentrations may have a significant beneficial impact on acid-base status of soils and potentially forest productivity. The region under study has actually been affected by acid rain in the last decades, which has led to reduced Ca availability in soils (Houle et al., 1997) and tree growth (Duchesne et al., 2002). In addition, the projected soil warming will likely increase forest floor respiration rate as well as soil organic matter decomposition (Marty et al., 2019), which will release nutrients (among which base cations) in the soil solution which may stimulate tree growth, at least in the short term (Melillo et al., 2011). Our simulations agree with previous observations that the temporal variation in pH and alkalinity in surface water drained from forested catchments were mainly controlled by temperature and not only by decreasing precipitation acidity (Houle et al., 2010), suggesting that increased BC WR, driven by warming temperature, are already involved in the actual recovery of surface water chemistry (acid-base status). Overall our results suggest that climate warming has the potential to improve the acid-base status of surface waters as well as to maintain forest growth by increasing BC availability for trees (Schaberg and Hawley, 2010; Moore et al., 2012).
Conclusion
This study shows that the projected climate warming in the region (increase in MAAT by 2.85–3.01°C and 4.38–4.92°C for the 2041–2070 and 2071–2100 periods, respectively) would result in a MAST increase of 2.03–2.05°C and 2.87–3.42°C during the same periods. Meanwhile, soil moisture would only decrease slightly (<−5%) due to the increase in MAP by 9–14%. According to model simulations, these future soil conditions would increase BC WR and thereby soil pH and BC concentration in the soil solution, contributing to increase the acid-base status of surface waters and fertility of the soils. In our simulations, acidic ions depositions were kept constant (i.e., at actual levels). It is, however, likely that the observed decreasing trends in S and N depositions in the study area (Houle et al., 2010, 2014, 2015; Marty et al., 2012) will be prolonged in the future, which would further increase the beneficial effect of higher weathering rates on soil conditions. It is, however, important to underline that such an increase in BC WR was likely made possible because SWC was only slightly decreased (<−5%) by the increase in evapo-transpirative demand associated with increased MAST, due to substantial projected increase in MAP for the region under study (Figure 3). It is likely that for any regions where precipitation will stay constant or decrease in the future, decreased SWC may limit the stimulation of higher MAST on BC WR. Our study has nevertheless limitation since the PROFILE model is driven by annual climate inputs. As a result, other climate change impacts on WR driven by seasonality such as changes in growing season length or increasing probability of summer drought (resulting in lower SWC) have not been quantified. Also, other impacts of climate change such as a potential increase of nutrients demands by trees due to a speculated positive impact of higher temperature on growth may eventually result in lower BC availability. The present study represents a first step in trying to project future WR in response to climate change in eastern North-America. More research is needed to model these complex biogeochemical processes and refine these projections.
Data Availability Statement
The datasets generated for this study are available on request to the corresponding author.
Author Contributions
DH designed the study. GD made the simulations. CM made the first data analysis. All authors contributed to data interpretation and writing of the manuscript.
Conflict of Interest
The authors declare that the research was conducted in the absence of any commercial or financial relationships that could be construed as a potential conflict of interest.
References
Åberg, G., Jacks, G., and Hamilton, P. J. (1989). Weathering rates and 87Sr/86Sr ratios: an isotopic approach. J. Hydrol. 109, 65–78. doi: 10.1016/0022-1694(89)90007-3
Akselsson, C., Olsson, J., Belyazid, S., and Capell, R. (2016). Can increased weathering rates due to future warming compensate for base cation losses following whole-tree harvesting in spruce forests? Biogeochemistry 128, 89–105. doi: 10.1007/s10533-016-0196-6
Arp, P. A., and Yin, X. W. (1992). Predicting water fluxes through forests from monthly precipitation and mean monthly air temperature records. Can. J. For. Res. 22, 864–877. doi: 10.1139/x92-116
Augustin, F., Houle, D., and Courchesne, F. (2018). An approach at estimating present day base cation weathering rates: a case study for the Hermine watershed, Canada. Biogeochemistry 140, 127–144. doi: 10.1007/s10533-018-0479-1
Augustin, F., Houle, D., Gagnon, C., and Courchesne, F. (2015a). Long-term mineral weathering in forested catchments of the Canadian shield, Québec. Geoderma 247–248, 12–23. doi: 10.1016/j.geoderma.2015.01.016
Augustin, F., Houle, D., Gagnon, C., and Courchesne, F. (2016). Evaluation of three methods for estimating the weathering rates of base cations in forested catchments. Catena 144, 1–10. doi: 10.1016/j.catena.2016.04.022
Augustin, F., Houle, D., Gagnon, C., Couture, S., and Courchesne, F. (2015b). Partitioning the impact of environmental factors on lake concentrations and catchment budgets for base cations in forested ecosystems. Appl. Geochem. 53, 1–12. doi: 10.1016/j.apgeochem.2014.11.013
Belyazid, S., and Giuliana, Z. (2019). Water limitation can negate the effect of higher temperatures on forest carbon sequestration. Eur. J. For. Res. 138, 287–297. doi: 10.1007/s10342-019-01168-4
Brimhall, G. H., Lewis, C. J., Ford, C., Bratt, J., Taylor, G., and Warin, O. (1991). Quantitative geochemical approach to pedogenesis importance of parent material reduction, volumetric expansion, and eolian influx in laterization. Geoderma 51, 51–91. doi: 10.1016/0016-7061(91)90066-3
Chou, L., and Wollast, R. (1984). Study of the weathering of albite at room temperature and pressure with a fluidized bed reactor. Geochim. Cosmochim. Acta 48, 2205–2218. doi: 10.1016/0016-7037(84)90217-5
Clayton, J. L. (1979). “Nutrient supply to soil by rock weathering,” in Impact of Intensive Harvesting on Forest Nutrient Cycling, ed. A. Leaf (Syracuse, NY: State University of New York), 75–96.
Cosby, B. J., Ferrier, R. C., Jenkins, A., and Wright, R. F. (2001). Modelling the effects of acid deposition: refinements, adjustments and inclusion of nitrogen dynamics in the MAGIC model. Hydrol. Earth Syst. Sci. 5, 499–517. doi: 10.5194/hess-5-499-2001
Courchesne, F., Hallé, J. P., and Turmel, M. C. (2002). Bilans élémentaires holocèneset altération des minéraux dans trois sols forestiers du Québec méridional. Géog. Phys. Quatern. 56, 3–124.
Davidson, E. A., and Janssens, I. A. (2006). Temperature sensitivity of soil carbon decomposition and feedbacks to climate change. Nature 440, 165–173. doi: 10.1038/nature04514
Duan, L., Hao, J., Xie, S., Zhou, Z., and Ye, X. (2002). Determining weathering rates of soils in China. Geoderma 110, 205–225. doi: 10.1016/s0016-7061(02)00231-8
Duchesne, L., and Houle, D. (2006). Base cation cycling in a pristine watershed of the Canadian boreal forest. Biogeochemistry 78, 195–216. doi: 10.1007/s10533-005-4174-7
Duchesne, L., Ouimet, R., and Houle, D. (2002). Basal area growth of sugar maple in relation to acid deposition, stand health, and soil nutrients. J. Environ. Qual. 31, 1676–1683. doi: 10.2134/jeq2002.1676
Durán, J., Morse, J. L., Groffman, P. M., Campbell, J. L., Christenson, L. M., Driscoll, C. T., et al. (2014). Winter climate change affects growing-season soil microbial biomass and activity in northern hardwood forests. Glob. Chang. Biol. 20, 3568–3577. doi: 10.1111/gcb.12624
Egli, M., and Fitze, P. (2000). Formulation of pedologic mass balance based on immobile elements: a revision. Soil Sci. 165, 437–443. doi: 10.1097/00010694-200005000-00008
Erlandson Lampa, M., Sverdrup, H. U., Bishop, K. H., Belyazid, S., Ameli, A., and Köhler, S. J. (2020). Catchment export of base cations: improved mineral dissolution kinetics influence the role of water transit time. Soil 6, 231–244. doi: 10.5194/soil-6-231-2020
Euskirchen, E., McGuire, A. D., Kicklighter, D. W., Zhuang, Q., Clein, J. S., Dargaville, R. J., et al. (2006). Importance of recent shifts in soil thermal dynamics on growing season length, productivity, and carbon sequestration in terrestrial high latitude ecosystems. Glob. Chang. Biol. 12, 731–750. doi: 10.1111/j.1365-2486.2006.01113.x
Gessler, A., Keitel, C., Nahm, M., and Rennenberg, H. (2004). Water shortage affects the water and nitrogen balance in Central European beech forests. Plant Biol. 6, 289–298. doi: 10.1055/s-2004-820878
Gislason, S. R., Oelkers, E. H., Eiriksdottir, E. S., Kardjilov, M. I., Gisladottir, G., Sigfusson, B., et al. (2009). Direct evidence of the feedback between climate and weathering. Earth Planet. Sci. Lett. 277, 213–222. doi: 10.1016/j.epsl.2008.10.018
Groffman, P. M., Hardy, J. P., Fisk, M. C., Fahey, T. J., and Driscoll, C. T. (2009). Climate variation and soil carbon and nitrogen cycling processes in a Northern Hardwood Forest. Ecosystems 12, 927–943. doi: 10.1007/s10021-009-9268-y
Hairston, N. G. Jr., and Fussmann, G. F. (2002). “Lake ecosystems,” in Encyclopedia Life Science, ed. eLS (London: Macmillian Reference Ltd), 1–3. doi: 10.1038/npg.els.0003191
Hernandez-Santana, V., Martinez-Vilalta, J., Martinez-Fernandez, J., and Williams, M. (2009). Evaluating the effect of drier and warmer conditions on water use by Quercus pyrenaica. For. Ecol. Manage. 258, 1719–1730. doi: 10.1016/j.foreco.2009.07.038
Houle, D., Bouffard, A., Duchesne, L., Logan, T., and Harvey, R. (2012a). Projections of future soil temperature and water content for three Southern Quebec forested sites. J. Clim. 25, 7690–7701. doi: 10.1175/jcli-d-11-00440.1
Houle, D., Couture, S., and Gagnon, C. (2010). Relative role of decreasing precipitation sulfate and climate on recent lake recovery. Global Biogeochem. Cycles 24:GB4029. doi: 10.1029/2009GB003757
Houle, D., Duchesne, L., Ouimet, R., Paquin, R., Meng, F. R., and Arp, P. A. (2002). Evaluation of the FORHYM2 model for prediction of hydrologic fluxes and soil temperature at the Lake Clair Watershed (Duchesnay, Quebec). For. Ecol. Manage. 159, 249–260. doi: 10.1016/s0378-1127(01)00438-8
Houle, D., Lamoureux, P., Bélanger, N., Bouchard, M., Gagnon, C., Couture, S., et al. (2012b). Soil weathering rates in 21 catchments of the Canadian Shield. Hydrol. Earth Syst. Sci. 16, 685–697. doi: 10.5194/hess-16-685-2012
Houle, D., Marty, C., and Duchesne, L. (2015). Response of canopy nitrogen uptake to a rapid decrease in bulk nitrate deposition in two eastern Canadian boreal forests. Oecologia 177, 29–37. doi: 10.1007/s00442-014-3118-0
Houle, D., Marty, C., Duchesne, L., and Gagnon, C. (2014). Humus layer is the main locus of secondary SO4 production in boreal forests. Geochim. Cosmochim. Acta 126, 18–29. doi: 10.1016/j.gca.2013.10.038
Houle, D., Ouimet, R., Couture, S., and Gagnon, C. (2006). Base cation reservoirs in soil control the buffering capacity of lakes in forested catchments. Can. J. Fish. Aquat. Sci. 63, 471–474. doi: 10.1139/f06-007
Houle, D., Paquin, R., Camiré, C., Ouimet, R., and Duchesne, L. (1997). Response of the Lake Clair Watershed (Duchesnay, Québec) to changes in precipitation chemistry (1988-1994). Can. J. For. Res. 27, 1813–1821. doi: 10.1139/x97-143
Jönsson, C., Warfvinge, P., and Sverdrup, H. (1995). Uncertainty in predicting weathering rate and environmental stress factors with the PROFILE model. Water Air Soil Pollut. 81, 1–23. doi: 10.1007/bf00477253
Jungqvist, G., Oni, S. K., Teutschbein, C., and Futter, M. N. (2014). Effect of climate change on soil temperature in swedish boreal forests. PLoS One 9:e93957. doi: 10.1371/journal.pone.0093957
Kaiser, C., Fuchslueger, L., Koranda, M., Gorfer, M., Stange, C. F., Kitzler, B., et al. (2011). Plants control the seasonal dynamic of microbial N cycling in a beech forest soil by belowground C allocation. Ecology 92, 1036–1051. doi: 10.1890/10-1011.1
Kaiser, C., Koranda, M., Kitzler, B., Fuchslueger, L., Schnecker, J., Schweiger, P., et al. (2010). Belowground carbon allocation by trees drive seasonal pattern of extracellular enzyme activities by altering microbial community composition in a beech forest soil. New Phytol. 187, 843–858. doi: 10.1111/j.1469-8137.2010.03321.x
Koptsik, G., Teveldal, S., Aamlid, D., and Venn, K. (1999). Calculations of weathering rate and soil solution chemistry for forest soils in the Norwegian–Russian border area with the PROFILE model. Appl. Geochem. 14, 173–185. doi: 10.1016/s0883-2927(98)00048-1
Li, G., Hartmann, J., Derry, L. A., West, A. J., You, C. F., Long, X., et al. (2016). Temperature dependence of basalt weathering. Earth Planet. Sci. Lett. 443, 59–69. doi: 10.1016/j.epsl.2016.03.015
Marty, C., Houle, D., Duchesne, L., and Gagnon, C. (2012). Canopy interaction with precipitation and sulphur deposition in two boreal forests of Quebec, Canada. Environ. Pollut. 162, 354–360. doi: 10.1016/j.envpol.2011.12.007
Marty, C., Houle, D., and Gagnon, C. (2015). Effect of the relative abundance of conifers versus hardwoods on soil δ13C enrichment with soil depth in eastern Canadian forests. Ecosystems 18, 629–642. doi: 10.1007/s10021-015-9852-2
Marty, C., Houle, D., Gagnon, C., and Courchesne, F. (2017). The relationships of soil total nitrogen concentrations, pools and C:N ratios with climate, vegetation types and nitrate deposition in temperate and boreal forests of eastern Canada. Catena 152, 163–172. doi: 10.1016/j.catena.2017.01.014
Marty, C., Piquette, J., Morin, H., Bussières, D., Thiffault, N., Houle, D., et al. (2019). Nine years of in situ soil warming and topography impact the temperature sensitivity and basal respiration rate of the forest floor in a Canadian boreal forest. PLoS One 14:e0226909. doi: 10.1371/journal.pone.0226909
Melillo, J. M., Butler, S., Johnson, J., Mohan, J., Steudler, P., Lux, H., et al. (2011). Soil warming, carbon-nitrogen interactions, and forest carbon budgets. Proc. Natl. Acad. Sci. U.S.A. 108, 9508–9512. doi: 10.1073/pnas.1018189108
Melillo, J. M., Steudler, P. A., Aber, J. D., Newkirk, K., Lux, H., Bowles, F. P., et al. (2002). Soil warming and carbon-cycle feedbacks to the climate system. Science 298, 2173–2176. doi: 10.1126/science.1074153
Milly, P. C. D. (1992). Potential evapotranspiration and soil moisture in general circulation models. J. Clim. 5, 209–226. doi: 10.1175/1520-0442(1992)005<0209:peasmi>2.0.co;2
Moore, J. D., Ouimet, R., and Duchesne, L. (2012). Soil and sugar maple response 15 years after dolomitic lime application. For. Ecol. Manage. 281, 130–139. doi: 10.1016/j.foreco.2012.06.026
Mott, R., Vionnet, V., and Grünewald, T. (2018). The seasonal snow cover dynamics: review on wind-driven coupling processes. Front. Earth Sci. 6:197. doi: 10.3389/feart.2018.00197
Munsell Soil Color Charts (1994). Munsell Color, Macbeth Division of Kollmorgen Instruments Corporation.
Oelkers, E. H., Schott, J., and Devidal, J. L. (1994). The effect of aluminum, pH, and chemical affinity on the rates of aluminosilicate dissolution reactions. Geochim. Cosmochim. Acta 58, 2011–2024. doi: 10.1016/0016-7037(94)90281-x
Öquist, M., and Laudon, H. (2008). Winter soil frost conditions in boreal forests control growing season soil CO2 concentration and its atmospheric exchange. Glob. Chang. Biol. 14, 2839–2847. doi: 10.1111/j.1365-2486.2008.01669.x
Ouranos (2015). Vers L’adaptation. Synthèse des Connaissances sur les Changements Climatiques au Québec. Édition 2015. Montréal: Ouranos, 415.
Ranger, J., Robert, M., Bonnaud, P., and Nys, C. (1990). Les minéraux-test, une approche expérimentale in situ de l’altération biologique et du fonctionnement des écosystèmes forestiers. Effets des types de sols et des essences feuillues et résineuses. Ann. Sci. For. 47, 529–550. doi: 10.1051/forest:19900601
Régnière, J., and Bolstad, P. (1994). Statistical simulation of daily air temperature patterns eastern North America to forecast seasonal events in insect pest management. Environ. Entomol. 23, 1368–1380. doi: 10.1093/ee/23.6.1368
Régnière, J., and St-Amant, R. (2007). Stochastic simulation of daily air temperature and precipitation from monthly normals in North America north of Mexico. Int. J. Biometeorol. 51, 415–430. doi: 10.1007/s00484-006-0078-z
Rennenberg, H., Loreto, F., Polle, A., Brilli, F., Fares, S., Beniwal, R. S., et al. (2006). Physiological responses of forest trees to heat and drought. Plant Biol. 8, 556–571. doi: 10.1055/s-2006-924084
Ryan, D. F., and Kahler, D. M. (1987). Geochemical and mineralogical indications of pH in lakes and soils in central New Hampshire in the early Holocene. Limnol. Oceanogr. 32, 751–757. doi: 10.4319/lo.1987.32.3.0751
Sandén, P., and Warfvinge, P. (1992). Modelling Groundwater Response to Acidification: Report from the Swedish Integrated Groundwater Acidification Project. Coll. Reports Hydrology, no 5. Lund: Lund University, 186.
Schaberg, P. G., Hawley, G. J. (2010). “Disruption of calcium nutrition at Hubbard Brook Experimental Forest (New Hampshire) alters the health and productivity of red spruce and sugar maple trees and provides lessons pertinent to other sites and regions,” in Proceedings of the Conference on the Ecology and Management of High-Elevation Forests in the Central and Southern Appalachian Mountains. 2009 May 14-15; Slatyfork, WV. Gen. Tech. Rep. NRS-P-64, eds Rentch, S. James, Schuler, and M. Thomas (Newtown Square, PA: U.S. Department of Agriculture), 190–200.
Schaller, M., Blum, J. D., Hamburg, S. P., and Vadeboncoeur, M. A. (2009). Spatial variability of long-term chemical weathering rates in the White Mountains, New Hampshire, USA. Geoderma 154, 294–301. doi: 10.1016/j.geoderma.2009.10.017
Scinocca, J. F., Kharin, V. V., Jiao, Y., Qian, M. W., Lazare, M., Solheim, L., et al. (2016). Coordinated global and regional climate modeling. J. Clim. 29, 17–35. doi: 10.1175/JCLI-D-15-0161.1
Sushama, L., Laprise, R., and Allard, M. (2006). Modeled current and future soil thermal regime for northeast Canada. J. Geophys. Res. 111:D18111. doi: 10.1029/2005JD007027
Sverdrup, H. (1990). The Kinetics of Base Cation Release Due to Chemical Weathering. Lund: Lund University Press, 246.
Sverdrup, H. (2009). Chemical weathering of soil minerals and the role of biological processes. Fungal Biol. Rev. 23, 94–100. doi: 10.1016/j.fbr.2009.12.001
Sverdrup, H., and Warfvinge, P. (1995). Critical loads of acidity for Swedish forest ecosystems. Ecol. Bull. 44, 75–89.
Sverdrup, H., and Warfvinge, P. (1999). Calculating field weathering rates using a mechanistic geochemical model PROFILE. Appl. Geochem. 8, 273–283. doi: 10.1016/0883-2927(93)90042-f
Velbel, M. A. (1985). Geochemical mass balances and weathering rates in forested watersheds of the Southern Blue Ridge. Am. J. Sci. 285, 904–930. doi: 10.2475/ajs.285.10.904
Venterea, R. T., Lovett, G. M., Groffman, P. M., and Schwarz, P. A. (2003). Landscape patterns of net nitrification in a northern hardwood-conifer forest. Soil Sci. Soc. Am. J. 67, 527–539. doi: 10.2136/sssaj2003.0527
Warfvinge, P., and Sverdrup, H. (1992). Calculating critical loads of acid deposition with PROFILE—a steady-state soil chemistry model. Water Air Soil Pollut. 63, 119–143. doi: 10.1007/bf00475626
White, A. F., and Blum, A. E. (1995). Effects of climate on chemical weathering in watersheds. Geochim. Cosmochim. Acta 59, 1729–1747. doi: 10.1016/0016-7037(95)00078-e
Yin, X. W., and Arp, P. A. (1993). Predicting forest soil temperatures from monthly air temperature and precipitation records. Can. J. For. Res. 23, 2521–2536. doi: 10.1139/x93-313
Keywords: soil weathering, climate change, forest ecosystems and biogeochemical processes, tree nutrition, surface water acidity
Citation: Houle D, Marty C, Augustin F, Dermont G and Gagnon C (2020) Impact of Climate Change on Soil Hydro-Climatic Conditions and Base Cations Weathering Rates in Forested Watersheds in Eastern Canada. Front. For. Glob. Change 3:535397. doi: 10.3389/ffgc.2020.535397
Received: 15 February 2020; Accepted: 03 September 2020;
Published: 23 September 2020.
Edited by:
Klaus Kaiser, Martin Luther University Halle-Wittenberg, GermanyReviewed by:
Salim Belyazid, Stockholm University, SwedenHui Wang, Chinese Academy of Forestry, China
Pavel Kram, Czech Geological Survey, Czechia
Copyright © 2020 Houle, Marty, Augustin, Dermont and Gagnon. This is an open-access article distributed under the terms of the Creative Commons Attribution License (CC BY). The use, distribution or reproduction in other forums is permitted, provided the original author(s) and the copyright owner(s) are credited and that the original publication in this journal is cited, in accordance with accepted academic practice. No use, distribution or reproduction is permitted which does not comply with these terms.
*Correspondence: Daniel Houle, daniel.houle2@canada.ca