- 1Grupo de Ecología de Invasiones, Instituto de Investigaciones en Biodiversidad y Medioambiente, Consejo Nacional de Investigaciones Científicas y Técnicas – Universidad Nacional del Comahue, San Carlos de Bariloche, Argentina
- 2Department of Biology, Boston University, Boston, MA, United States
- 3Department of Environmental Forest Biology, State University of New York College of Environmental Science and Forestry, Syracuse, NY, United States
Temperate and boreal forests are increasingly suffering from anthropic degradation. Ectomycorrhizal fungi (EMF) are symbionts with most temperate and boreal forest trees, providing their hosts with soil nutrients and water in exchange for plant carbon. This group of fungi is involved in woody plants’ survival and growth and helps plants tolerate harsh environmental conditions. Here, we describe the current understanding of how EMF can benefit temperate and boreal forest restoration projects. We review current evidence on promising restoration plans that actively use EMF in sites contaminated with heavy metals, affected by soil erosion, and degraded due to clearcut logging and wildfire. We discuss the potential role of this group of fungi for restoring sites invaded by non-native plant species. Additionally, we explore limitations, knowledge gaps, and possible undesired outcomes of the use of EMF in forest restoration, and we suggest how to further incorporate this fungal group into forest management. We conclude that considering EMF–host interactions could improve the chances of success of future restoration programs in boreal and temperate forests.
Introduction
Forest ecosystems are undergoing a process of anthropic degradation globally (DeFries et al., 2010). This process is reducing biodiversity and negatively affecting the goods and services they provide (Chazdon, 2008; Aerts and Honnay, 2011). In temperate and boreal forests, most woody plant species are obligate partners with ectomycorrhizal fungi (EMF), which provide nutrients (e.g., nitrogen, N) and water to host trees in exchange for photosynthetically fixed carbon (C) (Smith and Read, 2008). Due to the prevalence of this symbiotic interaction, EMF have a potentially critical role in restoration and management interventions in these ecosystems (Hawkins et al., 2015, Box1). However, their current use in forest restoration and management is limited and their direct manipulation to achieve restoration objectives is even less common (Heneghan et al., 2008).
Several lines of evidence indicate that using mycorrhizal fungi as a tool for restoration can be cost-effective and have long-term results. Anthropic activities can negatively affect the abundance and richness of the EMF community in forest soils due to soil erosion, changes in land use, inorganic toxins, fire, and non-native plant invasions (Pringle et al., 2009; Maltz and Treseder, 2015; Asmelash et al., 2016). Inoculation with specific soil microbes can facilitate the establishment and growth of plant species of interest in degraded ecosystems, while also improving soil quality (Requena et al., 2001; Jeffries et al., 2003; Harris, 2009; Kalucka and Jagodzinski, 2016). For example, inoculation with arbuscular mycorrhizal fungi (AMF) can promote the development of early successional grasses in tallgrass prairies (Smith et al., 1998; Richter and Stutz, 2002) and may help restore focal plant species to degraded soils, such as mining soils (Maltz and Treseder, 2015; Koziol et al., 2018). Additionally, the use of transplanted seedlings with appropriate microbes in their roots, or the use of cover crops associated with mycorrhizal symbionts, can help ensure inoculum availability for later-establishing plant species (Jeffries et al., 2003; Piñeiro et al., 2013). There are some examples in which no effect of mycorrhizal inoculation was detected in field restoration experiments (Teste et al., 2004; White et al., 2008; Sýkorová et al., 2016), yet these constitute a small fraction of the published studies on microbial-assisted restoration.
Box 1. What is forest restoration?
Forest restoration often aims to repair ecosystem functions, re-introduce species of special concern, or return forest ecosystems to specified reference conditions (e.g. a historical state) in terms of specific community structures or other traits. Restoration goals are often pragmatic and set by decision makers and institutions investing in them (Stanturf et al., 2014). Goals are generally influenced by the degree of degradation, regional climate, and interests of local people and stakeholders (Jacobs et al., 2015). It is generally impossible to recreate ecosystem conditions that existed prior to anthropic or natural disturbance because of local extinctions, shifts in climate conditions, or other irreversible changes in conditions. Thus, many restorations focus on restoring specific ecosystem functions, particularly in a context of rapid climate and land use changes (Stanturf et al., 2014).
Ectomycorrhizal fungi interactions with the dominant plant species in forests are better understood and described than those of other soil organisms (Horton and Bruns, 2001; Soudzilovskaia et al., 2019), which makes them particularly promising for use in restoration of specific associated plant species. Moreover, their functional role in ecosystems and their interactions with other plant and soil organisms in forests are becoming clearer (Lilleskov et al., 2002; Phillips et al., 2013; Hewitt et al., 2015; Steidinger et al., 2019), which is particularly important for managers aiming to restore ecosystem functionality (Heneghan et al., 2008). EMF are involved in post fire regeneration, plants’ tolerance and absorption of inorganic contaminants, and recovery after plant invasions (Colinas et al., 1994; Baar et al., 1999; Martínez et al., 2012; Sousa et al., 2014; Dickie et al., 2016; Kalucka and Jagodzinski, 2016).
Here, our objective is to gather current evidence on the effectiveness of actively using EMF in temperate and boreal forests restoration programs. We discuss their application in different degraded ecosystems and the possible limitations in their use. Specifically, we ask: Are plant–EMF interactions considered by active restoration plans? Is there evidence that the use of EMF can enhance restoration outcomes? Which are the main disturbances after which EMF restoration have been applied? What are the potential uses of EMF restoration in the future and what are the possible limitations in their use? To answer these questions, we performed a global review of the literature in November 2019, gathering published works addressing the use of EMF for a forest restoration objective. We used Scopus to perform a first search with the terms ectomycorrhiza∗ AND forest AND restor∗ in the title, abstract and/or keywords. After generating a list of 101 documents, we complemented that list with the same search in Google Scholar and Web of Science to check for possible missing papers in the Scopus search. We obtained a final list that contained a total of 140 documents. We only considered papers that specifically evaluated the role of EMF in restoration programs performed in temperate and boreal forests and used ectomycorrhizal plant hosts, checked for effective EMF colonization of plants’ roots, and considered the use of untreated plants for analyses (e.g., comparing biomass of EMF inoculated vs. non-inoculated plants in the field). We discarded those papers that evaluated only AMF, or addressed the effects of any kind of disturbance on diversity of the EMF community. From the papers obtained, we gathered and summarized information about the study site, the EMF species inoculated and the target host species, if informed.
Promising Applications of EMF in Forest Restoration
Despite its potential as a restoration tool, a relatively low number of studies have directly manipulated the EMF community to meet a restoration goal in temperate or boreal forests (Table 1). A total of 13 studies met the criteria used, all of them performed in the Northern Hemisphere. In most of the studies, mining is addressed as the major source of forest degradation, considering heavy metal contamination in the soil and soil erosion. Clearcut logging, other sources of soil erosion and wildfire are the other disturbances addressed. Although a relatively high number of papers addressed the effects of plant invasions on the EMF community, we found no papers directly manipulating the EMF community (native or non-native) to achieve forest restoration in invasion contexts. Most of the studies used EMF-inoculated plants in the nursery prior to assessing their growth and survival in the field, although we found some cases in which direct manipulation in the field was performed in post-mining sites. Restoration, measured in terms of establishment and growth of inoculated (compared to non-inoculated) target plant hosts was generally reported to be successful, although we found some exceptions (Table 1).
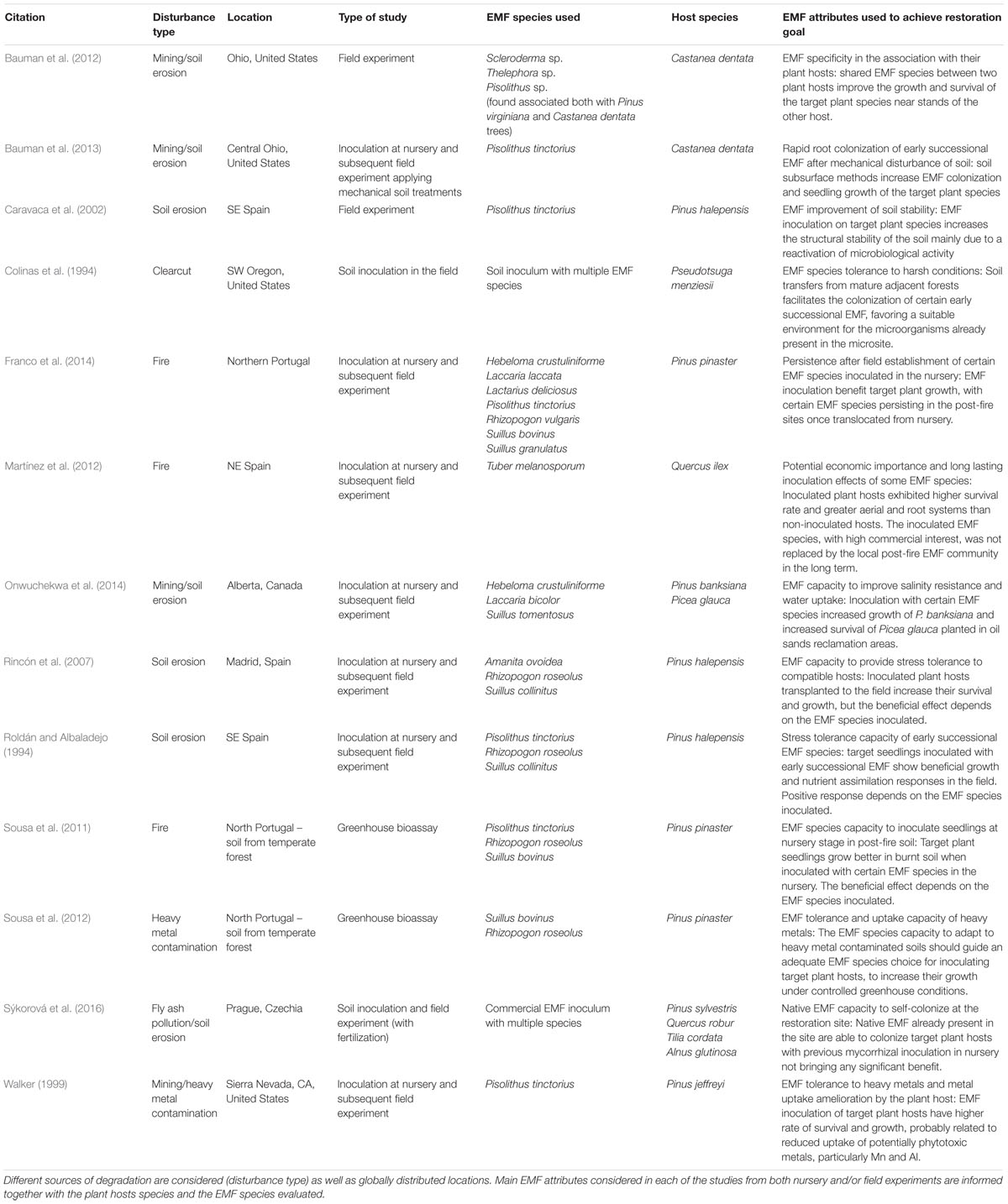
Table 1. Summary of the studies that address the role of ectomycorrhizal fungi in temperate or boreal forest restoration.
Heavy Metal Contamination
One type of restoration in which EMF appears to be particularly effective is in restoring forest soils contaminated with heavy metals (Figure 1A). While organic contaminants can be degraded, metals generally need to be physically removed or immobilized. Certain fungi and bacteria are able to make metals bioavailable (Krumins et al., 2015). From the 13 papers found, five addressed restoration of contaminated areas after mining, but two of them specifically addressed contamination with heavy metals in those sites (Walker, 1999; Sousa et al., 2012, Table 1). All of the studies found better growth and survival rates of EMF-inoculated hosts compared to non-inoculated plants, although there were differences according to the EMF species inoculated (Table 1). This species-level variation in protecting host plants from heavy metal toxicity may be due to uneven distribution of specific macrostructure traits across EMF species. Some EMF species can be negatively affected by high levels of heavy metals, reducing the number of sporocarps (i.e., ‘mushrooms’) produced at increasing levels of soil heavy metal pollution (Rühling and Söderström, 1990). However, common EMF species like Amanita muscaria or Suillus bovinus are able to accumulate high metal contents in their sporocarps (Leyval et al., 1997; Luo et al., 2014, Figure 1A). The protective effect of EMF against metal toxicity is also related to fungal structures in the root acting as a physical barrier to metal uptake in plants (Donnelly and Fletcher, 1994, Figure 1A). EMF tolerance -or capacity to absorb- heavy metals is species-specific and might depend on the amount of extramatrical mycelium produced. EMF with higher production of extramatrical mycelium might offer better protection to the plant host and fewer chances for heavy metals to reach the root. Species of Amanita, Paxillus, Pisolithus, Scleroderma, Suillus, and Rhizopogon accumulate high metal concentrations in the extramatrical mycelium, with decreasing metal concentrations along the Hartig net to the root interior of the plant symbiont (Wilkins, 1991; Khan et al., 2000). The capacity to produce high amounts of extramatrical mycelium, together with the capacity to absorb heavy metals and the tolerance to contaminated soils, will necessarily condition the selection of appropriate EMF species for use in remediation (Khan et al., 2000). Evidence shows that the ability to restrict the entry of toxic metals into the plant’s cell is partly dependent on the bioavailable metal concentration and the EMF species used (Khan et al., 2000; Sousa et al., 2012, Table 1). In recent years, several metal-tolerant isolates of EMF and associated hosts have been identified (Luo et al., 2014). The EMF species Pisolithus albus has been used as inoculant of ectomycorrhizal endemic hosts (Acacia spirorbis and Eucalyptus globulus) in tropical forests of New Caledonia degraded by mining activities, helping plant growth and acting as a protective barrier to toxic metals (Jourand et al., 2014). The restoration success in heavy metal contaminated sites is conditioned by successional interactions within the EMF species, and their ecological traits (e.g., dispersal, host preference, life history) (Kalucka and Jagodzinski, 2016). As both the plant host and the fungi mutually benefit in the ectomycorrhizal symbiosis, the use of plant-EMF pairs for restoration might be more effective than non-mycorrhizal plants or free-living soil microorganisms (Malajczuk et al., 1994; Leyval et al., 1997).
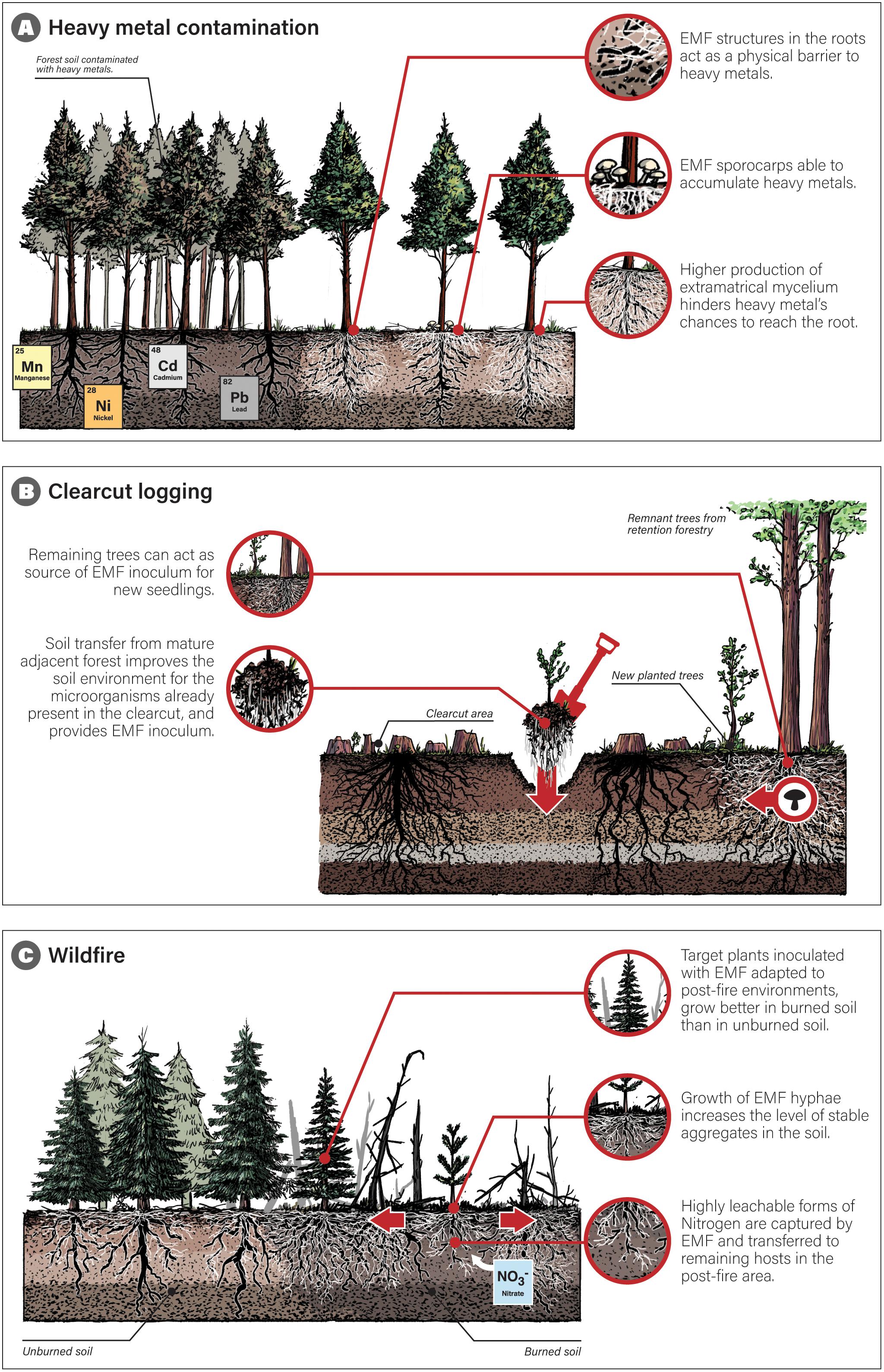
Figure 1. Three different scenarios of temperate or boreal forest degradation and suggested modes of restoration that consider the use of ectomycorrhizal fungi (EMF). In panel (A), specific host trees associated with heavy metal tolerant EMF are planted in forest soil contaminated with heavy metals. In panel (B), retention forestry is presented as an alternative to clearcut logging, in which groups of mature trees are left intact and are able to offer new planted trees access to the existing mycorrhizal network, favoring establishment. Soil translocation from adjacent forest is a complementary option to improve soil conditions in the clearcut area. In panel (C), EMF inoculated plants are planted in the post fire area. EMF traits help increase hosts’ growth, soil aggregates’ stability, and nutrients’ transfer to other hosts nearby.
Soil Erosion
Eroded soils usually include physically and chemically harsh conditions for organismal growth, such as low water-holding capacity, low organic matter, and extreme temperature (Roose et al., 2006). Reforestation programs intending to protect against soil erosion, rarely consider the effect of soil microbiota on increasing soil stability and function, which might partially explain some of reforestation failures in eroded soils. We found seven studies that addressed some form of soil erosion, many of them after mining (Table 1). In combination with other restoration techniques (Löf et al., 2012), EMF inoculation or soil translocation enhances soil restoration and drives plant community development (Wubs et al., 2016). One of the key factors necessary to restore eroded soils is to reactivate microbial activity (Diaz et al., 1994). Many eroded soils have lost the topsoil, in which most microbial activity occurs, so for mycorrhizal plant hosts it is common to find low level of mycorrhizal infectivity (Powell, 1980). To restore this mycorrhizal activity, nursery inoculation of EMF species that tolerate the harsh conditions of eroded soils has proven to be effective (Table 1). Greater EMF activity can increase the level of stable aggregates in the soil due to the growth of fungal hyphae (Caravaca et al., 2002). Mechanical soil treatment (e.g., soil ripping) can encourage the root colonization of new seedlings by EMF, enhancing plant establishment and long-term survival (Bauman et al., 2013). EMF nursery inoculation improved the performance of conifers planted in oil sands reclamation areas and degraded gypsum soil (Rincón et al., 2007; Onwuchekwa et al., 2014). However, these effects are highly dependent on the EMF species inoculated. Even when inoculation with most EMF species tends to be beneficial for plants growth, some species can show no effects or even negative effects (Roldán and Albaladejo, 1994). Due to the absence of viable fungal inoculum in highly eroded soils, further tests are needed to explore the use of soil from adjacent areas as a source of native EMF inoculum. Naturally inoculated seedlings from the field could be transplanted to nursery pots with soil from eroded areas and planted together with new seedlings, which - once inoculated - can be used for reforestation.
Clearcut Logging
Clearcut logging can change the EMF community composition of forests (Jones et al., 2003). The removal of a major plant C source, together with physical and chemical changes in the soil, provoke a change in the composition of the EMF community, favoring those EMF species that are able to disperse into, establish in, or persist under these new conditions (Jones et al., 2003; Sterkenburg et al., 2019). Retention forestry (Figure 1B), a practice that has been implemented in forest management since just a few decades ago, is an alternative to clearcutting, by which a portion of the original stand is left unlogged to maintain the continuity of structural and compositional diversity (Gustafsson et al., 2012). Keeping patches of EMF trees is expected to favor the establishment of new seedlings near those patches, as EMF species abundance and richness depend on the amount of host trees retained (Sterkenburg et al., 2019). In these patches, seedlings can access the existing mycorrhizal network and a larger pool of soil nutrients, water, and potentially C transference from adult plants (Simard and Durall, 2004). The presence of hosts patches nearby can also act as a source of fungal spores, which can increase EMF colonization of new seedlings and hence their nutrient and water uptake (Colinas et al., 1994; Simard, 2009; Van Der Heijden and Horton, 2009, Figure 1B). The study we found addressing the use of EMF to restore clearcut logging sites (Table 1) reported that soil transfers from mature adjacent forests facilitate the formation of ectomycorrhizas by decreasing detrimental rhizosphere bacteria, creating a suitable environment for the organisms already present in the clearcut habitat (Colinas et al., 1994, Figure 1B). Alternatively to soil translocation, nurse plants that tolerate the harsh conditions present in a degraded environment can act as sources of EMF inoculum to facilitate the establishment of target plant species (Bai et al., 2009; Richard et al., 2009; Baohanta et al., 2012; Kennedy et al., 2012).
Wildfire
Wildfire is detrimental to most fungal species (Hart et al., 2005; Cairney and Bastias, 2007). However, several EMF species have been described as fire-adapted depending on the intensity and frequency of fire (Glassman et al., 2016). As it is described for seed banks, ruderal EMF species, can thrive after fire, taking advantage of their spore resistance, available resources and absence of potential competitors (Glassman et al., 2016). These post-fire fungi might be crucial for recruitment of new ectomycorrhizal plant hosts and increasing soil stability (Claridge et al., 2009). Moreover, while most soil N is lost through combustion, ammonium (NH4+) and nitrate (NO3−, which is highly leachable) can increase due to incomplete combustion and pyrolysis of organic matter (Wan et al., 2001). These forms of N can be captured by EMF and transferred to remaining hosts in the post-fire area (Claridge et al., 2009, Figure 1C). As the post-fire EMF community composition can greatly vary according to fire intensity, frequency, and environmental conditions (Dove and Hart, 2017), simple bioassays using seeds from the potential native hosts planted in soil from affected areas can be used to determine the EMF species that would potentially colonize first after fire (Glassman et al., 2016).
Post-fire EMF communities might passively re-establish as long as viable propagules of fire-adapted fungi are present in the soil (Glassman et al., 2016), disperse from adjacent areas (Horton, 2017), or remain present in less affected areas, including deeper soil horizons, as viable mycorrhizal networks (Palfner et al., 2008). However, active restoration might sometimes be needed after wildfire. The three studies we found regarding post-fire restoration actively inoculated EMF (Table 1). Due to inoculation with selected species of EMF in the nursery, the growth of Pinus pinaster in burned soil was promoted compared to unburned soil (Sousa et al., 2011; Franco et al., 2014). Likewise, inoculation with Tuber melanosporum improved the survival rate and the growth of aerial and root systems of Quercus ilex trees in post fire stands (Martínez et al., 2012). Nursery inoculation could provide long-lasting advantage in terms of growth to plant hosts planted in post-fire stands (Martínez et al., 2012; Franco et al., 2014). Similar to plant community restoration, active restoration should prevent and minimize stress upon remnant native EMF communities (e.g., avoid introduction of non-native EMF species or further soil erosion or anthropic disturbance, Claridge et al., 2009).
Potential Use of EMF in Restoring Sites Invaded by Non-Native Plant Species
None of the papers we found used EMF to actively restore invaded or post-invasion plant communities (but see Patterson, 2019; Box 2). Although there is a high potential for active restoration of invaded communities using EMF, there is still a need for experimental evidence that test its effectiveness. Traditionally, restoration approaches have considered aboveground organisms that can be easily monitored over time, with soil biota generally being neglected (Wolfe and Klironomos, 2005). However, belowground microorganisms (such as EMF) are frequently a key mediator of forest invasion by non-native tree species.
Several studies, mostly from the Southern Hemisphere, have shown that many EMF species generally co-invade with non-native invasive woody plants (Nuñez et al., 2009; Dickie et al., 2010; Nuñez and Dickie, 2014). The most studied case is that of plant species in the Pinaceae and their co-invasion with non-native EMF (Dickie et al., 2010). The invasion of pine trees into the Southern Hemisphere is one of the most widespread invasions of non-native species on Earth, transforming native forests, grasslands, and shrublands into conifer-dominated forests (Simberloff et al., 2010; Nuñez et al., 2017). Pine trees obligately associate with diverse EMF communities in their native habitats, but pine invasion into native forests is consistently associated with colonization of the pine roots by a small number of non-native invasive EMF species (Nuñez et al., 2009; Hayward et al., 2015a, b; Policelli et al., 2019). In spite of being highly invasive, Pinaceae species are frequently planted for forestry (Richardson and Rejmánek, 2011). The use of non-invasive EMF as inoculants for new plantations can be an alternative to avoid or hinder the invasion of pine hosts. In regions such as South America and New Zealand, several Pinaceae species invade temperate forests of the native ectomycorrhizal tree species Nothofagus that is associated in turn with native EMF (Policelli et al., 2020). In those regions, EMF species themselves are starting to be considered the subject of possible invasion management (Dickie et al., 2016). Management of the mutualistic interaction between pines and EMF, instead of the species individually, seems to be the next approach to avoid further advance of the invasion.
Box 2. The Albany Pine Bush – An ongoing case study of forest restoration using EMF.
The Albany Pine Bush Preserve (APBP) located in Albany, New York State, United States, contains a fire adapted pine barren system. The community is a savannah-like habitat with Pinus rigida (pitch pine) as the dominant tree species. The community relies on fire which had been suppressed for decades and in its absence, Robinia pseudoacacia (black locust) invaded (Figure B1). R. pseudoacacia is an N-fixing legume that is associated with arbuscular mycorrhizal fungi.
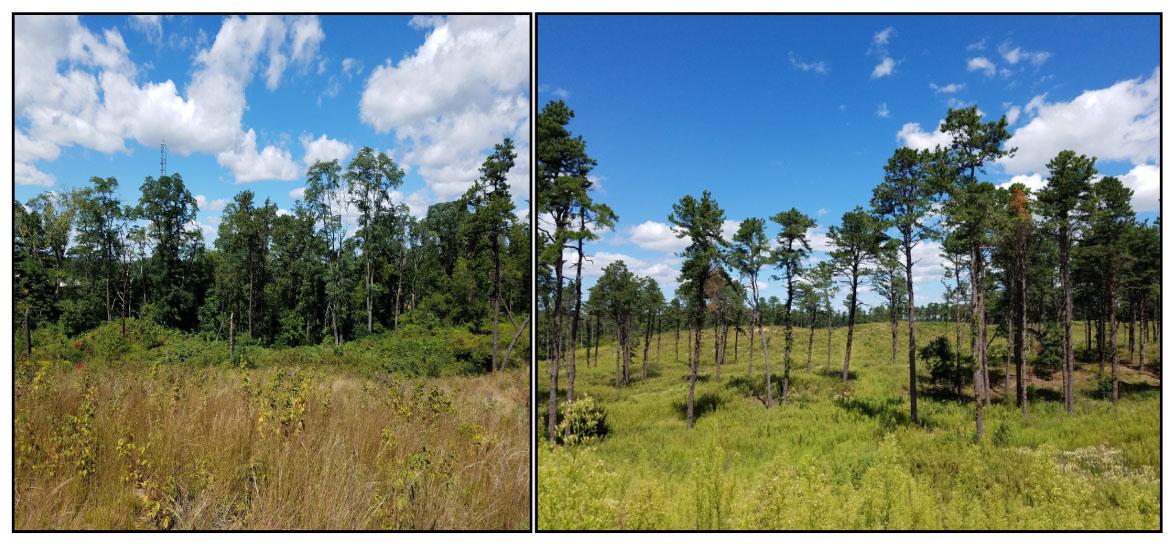
FIGURE B1. Sites at the APBP. Left panel: invaded community before black locust removal. Right panel: target open pine community.
The APBP is actively restoring the pine barrens through prescribed fires (Hayward et al., 2015a). The locust is removed by ripping up the trees and mechanically stirring the soil in an effort to kill off roots that would produce new shoots. After the locust is removed, the managers plant young pitch pine seedlings, which do not always establish.
To increase successful pine establishment, several pine seedlings were inoculated with spore slurries made from locally-collected Suillus brevipes and Rhizopogon pseudoroseolus sporocarps (Hudon, 2019; Patterson, 2019). Inoculation with live spore slurry improved seedling survival after outplanting (F = 39.55, p < 0.001, df 1,8, n = 10; Figure B2).
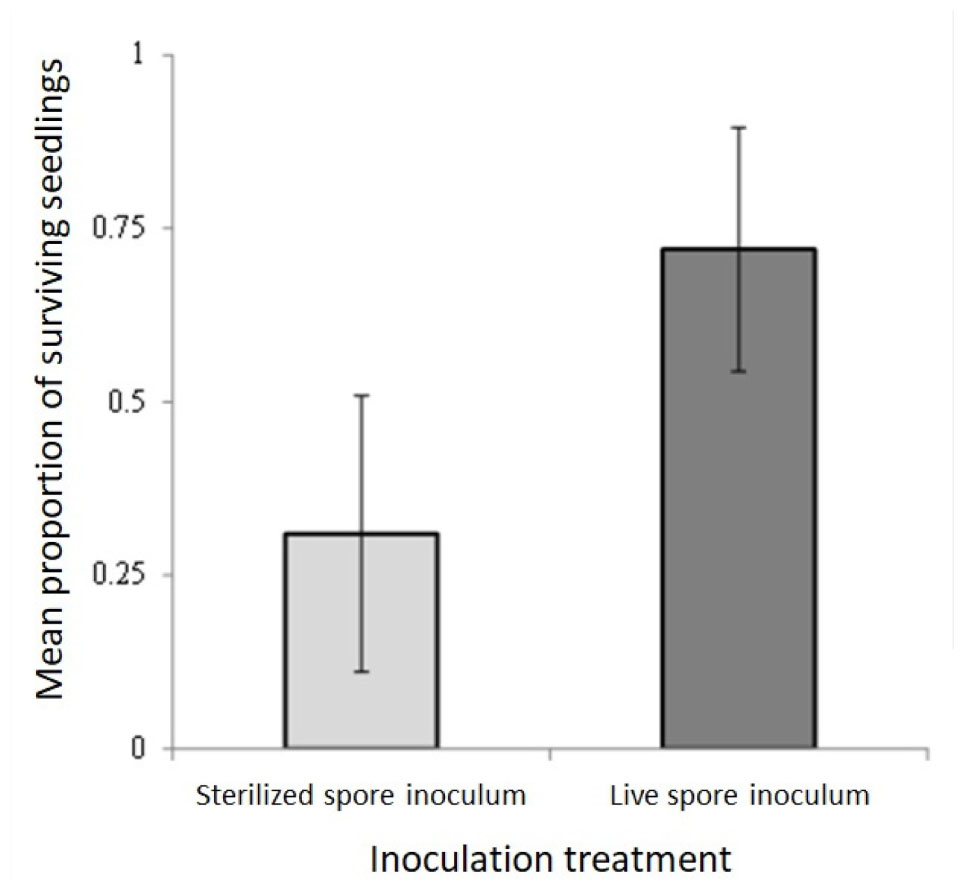
FIGURE B2. Proportion of Pinus rigida seedlings that survived in the field inoculated with sterilized spore inoculum (light grey bar) and live spore inoculum (dark grey bar) of Suillus brevipes and Rhizopogon pseudoroseolus. Error bars show confidence intervals (95%).
After removal of invasive hosts, soil legacies are a major problem for restoring invaded forests. Apart from any change in soil biogeochemistry produced by the invasion, there are also EMF species capable of residing for decades in soil (Dickie et al., 2014). The use of native EMF as source of inocula that can help post-invasion soils recover and improve the establishment of native plants is being increasingly considered (Maltz and Treseder, 2015). Inoculation treatments either with soil from a reference community or with single EMF species - performed both in nurseries or in the field - have worked effectively for re-establishing and increasing the growth of native plants in restoration programs (Dulmer et al., 2014; Henry et al., 2015; Hudon, 2019; Patterson, 2019). Native plants that promote EMF proliferation may also assist in management of invaded forests.
Although EMF have not been directly manipulated for restoration projects in invaded ecosystems, invasion scenarios offer an opportunity to learn potential desired ecological traits of EMF that can be used for restoration in the native habitat (Hoeksema et al., 2020). The same EMF species that aggressively co-invade with Pinaceae trees in the Southern Hemisphere (Hayward et al., 2015a; Policelli et al., 2019) can be used to restore pine forests in the Northern Hemisphere due to their spore resistance and germination rates in the presence of the hosts (Horton, 2017; Box 2). EMF can help to promote seedling survival by improving their resistance to stress, a trait that is likely to benefit reforestation programs over fast growth (Jacobs et al., 2015; Box 2).
Limitations on the Use of EMF in Forest Restoration
Choosing suitable EMF species for restoration is not trivial (Trappe, 1977). Different results - in terms of plant survival and growth - can be obtained depending on the EMF strains and plant hosts used (Onwuchekwa et al., 2014; Sousa et al., 2014). Not all EMF are good candidates for restoration efforts. While some may not survive in the harsh environmental conditions at a degraded site, others do not produce long-lived propagules/spores that can be used as inoculum including many species of Cantharellus, Russula and Tricholoma. EMF should be considered for restoration efforts if they have life history traits that contribute to their success in early successional settings, such as the production of functional spore inoculum (Ashkannejhad and Horton, 2006; Horton, 2017), and include species of Hebeloma, Laccaria, Pisolithus, Rhizopogon, Scleroderma, Suillus and Thelephora (Table 1). While some EMF can be inoculated with spores, many cannot. Even some species that are common in disturbed habitats might require growth of mycelial culture and direct inoculation of fungal plugs onto seedling root tips. The EMF that become established may remain at the site for years, so care must be taken to avoid introducing undesirable species for the habitat. In addition, the selection of EMF species that are not present in reference sites nearby could limit the success of restoration as they may not be adapted to local environmental conditions (Maltz and Treseder, 2015). Multiple inoculation experiments with EMF species that exhibit long distance exploration types such as Scleroderma, or Pisolithus (Agerer, 2001), together with species with the capacity to produce resistant spores (Bruns et al., 2009), and tolerance to harsh environmental condition (e.g., suilloid fungi) might be beneficial for target hosts. The list of EMF species tested for restoration and the empirical knowledge of EMF ecological traits are limited. Moreover, the introduction of novel EMF species or strains can cause unpredictable negative impacts to the ecosystem (e.g., changes in biogeochemical cycles, and facilitation of non-native plants’ invasion) (Pringle et al., 2009). Native EMF should be preferred over non-native EMF species. In a comprehensive review, Azul et al. (2014) proposed a framework on how native EMF diversity and function could be valuable for sustainable habitat restoration in Mediterranean ecosystems. Even after choosing the EMF species to inoculate, growth and survival of plants depends on the way EMF are inoculated, even with the same set of species and in the same site (Walker, 1999, 2003). Nursery inoculation appears to be a cost-effective way to check for effective EMF colonization and initial plant growth before transplanting to the field (Teste et al., 2004).
Nursery and field results do not always correspond. Although inoculation with individual EMF species may be effective at a nursery, it is necessary to experimentally evaluate in the field the growth of plants inoculated with single and multiple EMF species. Some inoculated plants growing well in the nursery can have greater mortality than non-inoculated plants when transplanted to the field (Tosh et al., 1993; Roldán and Albaladejo, 1994; Rincón et al., 2007). Once in the field, EMF colonization of native plant roots might be dependent on the local soil environment. EMF inoculation of target woody plants might be hindered in sites that have suffered from long-term fertilization as farmlands, even when pre-inoculated in a nursery (Menkis et al., 2007). Fertilization of plants in the field tends to hinder colonization by EMF, diminishing growth and establishment of target plants (Sýkorová et al., 2016). If native compatible EMF are already present in the soil, EMF inoculated onto seedlings are often replaced by local fungi that are active in the soils. It should also be stated that mycorrhizal inoculation of hosts in nurseries before planting may not produce any significant benefit to the plant growth (Sýkorová et al., 2016).
Incorrect manipulation of the mycorrhizal component of the soil may have undesired cascading effects on the rest of the belowground and aboveground communities. Comprehensive knowledge of the system involved is required to prevent those undesired outcomes, yet is challenging to obtain. Further, good-intentioned, deliberate introductions of EMF species for restoration purposes increase the chances of invasions (Vellinga et al., 2009). Some EMF invasions can enhance the chances of non-native plants to invade (Policelli et al., 2019), or even be risky for human health if eaten, as the case of the deadly poisoning Amanita phalloides, likely introduced to North America associated with oak and pines (Pringle and Vellinga, 2006; Wolfe et al., 2010; Dickie et al., 2016). Furthermore, it is still unclear if tree hosts inoculated with EMF respond consistently across different ecosystem types, which may have consequences in the restoration outcome.
While EMF are known to be beneficial for plant establishment, a restoration effort involving these fungi is difficult to manage and monitor. One of the biggest issues is that the vegetative body of an EMF is microscopic, interacting with plant roots, belowground and out of site. Most restoration efforts will not have the resources needed to assess successful establishment of the fungi, particularly if there is no dedicated mycologist involved. Restoration efforts using EMF might benefit from selecting specific genotypes for restoration (Figure 2), following similar considerations for plants outlines by Lesica and Allendorf (1999). In that work, the authors proposed guidelines for selecting plant material for restoration that may work adapted for EMF: (1) Use local genotypes when feasible. (2) Initiate new populations with as many genotypes and as much genetic variation as possible (collect fungi from many local microhabitats). (3) Avoid strains that have been maintained in culture for long periods of time. (4) Use species with wide ecological amplitudes when feasible. (5) Use species with low dispersal capabilities to minimize the genetic contamination of resident populations (Lesica and Allendorf, 1999). These guidelines depend on the size and severity of disturbance, while the best choice for all sizes of disturbances is to use locally adapted EMF species (Figure 2). Although it is always wise to use multiple EMF species and genotypes within EMF species, this might be more relevant as the size and severity of the disturbance increases (Figure 2).
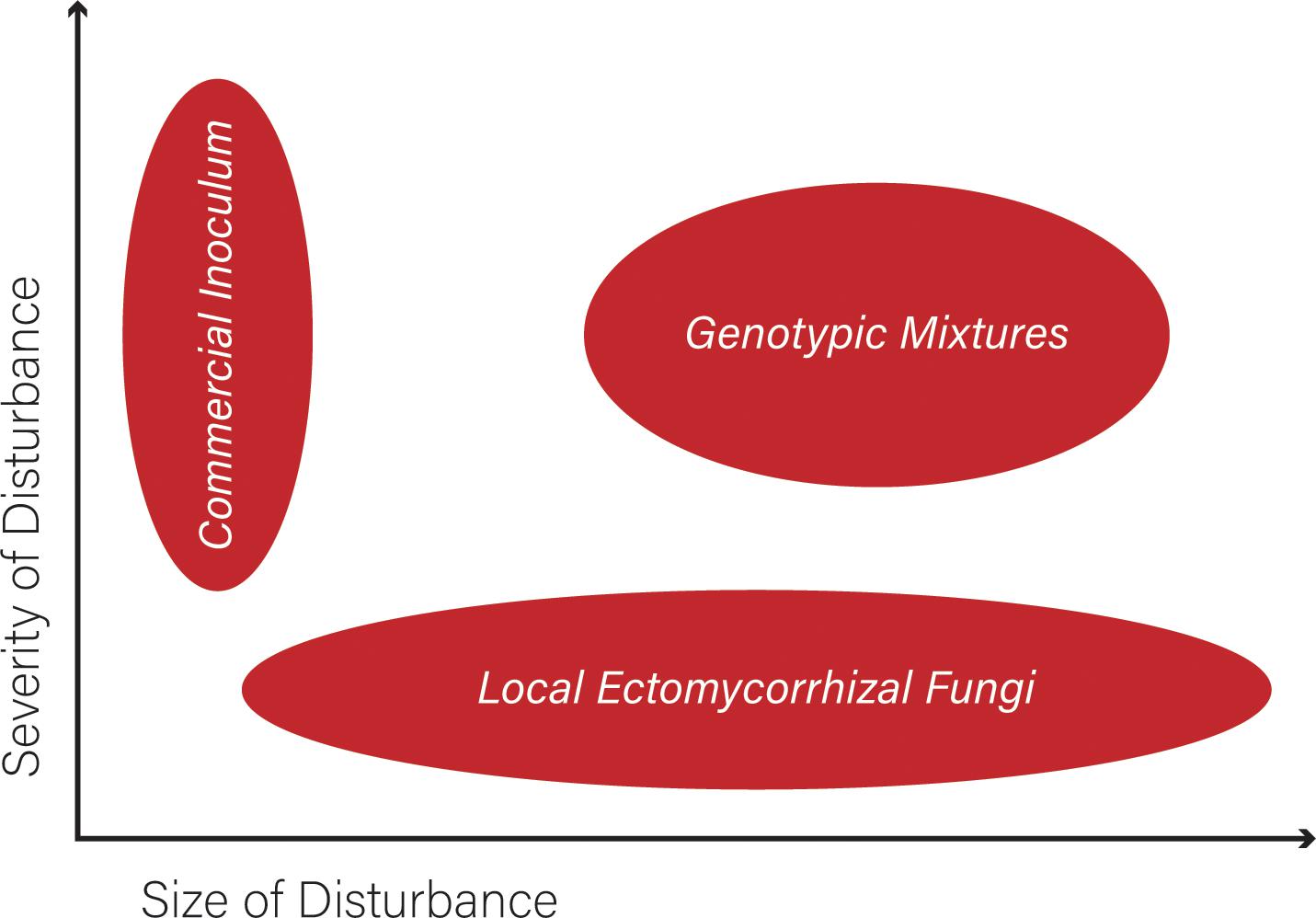
Figure 2. The severity and size of a disturbance will impact the use of ectomycorrhizal fungi in a plant restoration effort. The best choice for all sizes of disturbances is to use locally adapted EMF species. As one moves to more severe disturbances, it may be necessary to use commercial inoculum that is more generally adapted because the site has been too degraded for locally adapted genotypes. As the size and severity of the disturbance increases it is necessary to use multiple EMF species and as much genetic variation as possible within species (e.g., collecting fungi from many local microhabitats) to increase the chances of restoration success. Adapted from Lesica and Allendorf (1999).
When the site is too degraded for locally adapted genotypes, the use of commercial inoculum emerges as possibility, especially in relatively small affected areas (Figure 2). Commercial inocula usually contain sources of N and P, and gels to maintain soil moisture, yet their composition needs to be carefully considered. Plants growing with many commercial inocula will probably grow bigger compared to non-inoculated plants (Tarbell and Koske, 2007) and the use of commercial inoculants to restore mycorrhizal communities in soil has received increasing attention in different parts of the world. However, because of the risk of introducing non-native fungi, or even the use of widely distributed fungi (e.g., Glomus spp.), commercial inoculants have been discouraged (Heneghan et al., 2008; Dickie et al., 2016). In addition, many commercial inoculants are not effective in terms of mycorrhizal colonization of roots; sometimes very few, if any, might be colonized by mycorrhizal fungi. If no roots are colonized, such products risk creating a negative public opinion of mycorrhizal inoculants (Tarbell and Koske, 2007). When using commercial inocula in a restoration plan, the degree of mycorrhizal infection should be monitored. One must also keep in mind that the fungi that work best in controlled settings needed to generate commercial inoculum may not be best adapted for a restoration site. Suillus is commonly included in commercial products and is an excellent choice for restoration involving pine. However, it is specific to pine and will not support establishment of angiosperms such as oak, chestnut or eucalyptus. Indeed, all EMF have varying degrees of specificity, both in their compatibility with host plants (Molina et al., 1992) and tolerance to environmental conditions (so-called ecological specificity; Harley and Smith, 1983).
Using EMF for restoration may not always act synergistically with other restoration actions. Common practices of forest restoration such as reintroducing fire using prescribed burns or thinning, for example, may result in a reduction of EMF species richness, which can in turn translate into poor tree survival and slow stand recovery (Smith et al., 2005; Bastias et al., 2006) together with a reduction in fine root biomass (Hart et al., 2005). Several EMF genera are known to be resistant to disturbance and are expected to be the first taxa to colonize recruiting hosts (Baar et al., 1999; Stendell et al., 1999; Glassman et al., 2016). Nevertheless, target plant species survival and growth can also be negatively affected due to inadequate site preparation techniques. To help counteract this, mechanical methods such as scarification, mounding and subsoiling are used to prepare the soil (Löf et al., 2012). These methods have multiple impacts in soil physical, chemical, and biological properties depending on their intensity and frequency (Löf et al., 2012). In areas with soil compaction due to anthropic degradation (e.g., post mining soils, areas of clearcut logging), soil mechanical treatments enhancing EMF colonization could aid in the establishment and growth of target plants if sources of compatible EMF are nearby (Bauman et al., 2012; Pec et al., 2019). However, intense mechanical treatments such as deep ripping may extensively disrupt existing mycelium networks. Relatively simple greenhouse experiments could address the interactive effects of these mechanical methods and the use of EMF to determine the necessity and time to apply both restoration techniques in the same site (Bauman et al., 2013). Whether the target of restoration is re-establishing certain plant species or the entire plant community, restoration programs should consider the ecological processes and interactions both above and belowground upon which the persistence of that target community is contingent.
Recommendations for Future Research
A limited number of EMF species have been tested for temperate and boreal forests restoration purposes (Table 1). Future experimental tests should gather evidence from other species and their ease of use as inoculants in terms of plant hosts’ growth and survival. Inoculation of Pinus halepensis hosts with P. tinctorius improved the performance of the seedlings and was involved in soil aggregate stabilization in a reforestation program in a semi-arid system in Spain (Caravaca et al., 2002). This EMF has also proven effective in restoration plans using other hosts, such as Quercus suber (Sebastiana et al., 2013). However, Pisolithus is also characterized as a poor competitor, commonly displaced by other ECM fungi shortly after field planting (Bauman et al., 2013). Other more specific EMF species, such as suilloid fungi for pines, seem to be effective (Table 1), in part due to their tolerance to harsh environments. Generalists EMF might also be effective in restoration, as they are shared by different ectomycorrhizal tree host species and can aid in the establishment and growth of a focal species near other tree species already present in the site (Bauman et al., 2012). EMF genera that are effective in nursery inoculation include Laccaria, Hebeloma, Thelephora, Rhizopogon, Suillus, Cenococcum, Scleroderma, Pisolithus (Trappe, 1977, Table 1) and should be further evaluated in field restoration plans. Other EMF genera need to be explored in terms of their inoculation capacity and effectiveness in the field (e.g., native symbionts with native plants in Southern Hemisphere native forests). Exploring other EMF genera will also expand the set of plants hosts evaluated for restoration, which seems to be limited to a set of species mostly in the Pinaceae and Fagaceae families (Table 1).
In addition to promoting above- and below-ground biodiversity, inoculation with EMF to ensure host establishment can be economically profitable. Quercus ilex individuals inoculated with Tuber melanosporum had a higher survival rate and growth that those non-inoculated in a post-fire restoration program in NE Spain (Martínez et al., 2012). Tuber melanosporum is widely known for its gastronomic use and commercial value. Other less expensive - but also edible - EMF (e.g., some Suillus species) have also been used as inoculants and provide the extra economic benefit of an edible species in this restoration context (Box 2). Commercial edible EMF, as with other non-timber forest products, can help local communities become involved and support restoration programs in the medium and long term.
Incorporating EMF in restoration efforts can help increase the rate of restoration success, and restoration goals could be reached in a shorter amount of time after disturbance (Figure 3). Restoration success is hard to define and may vary based on what the restoration goals are. Most of the studies addressing the role of EMF in restoration examined root tips for EMF colonization and measured height or survival of seedlings over a period of time. This may not relate back to the whole picture of a habitat reaching a pre-disturbance state, in an attempt to answer an ecosystem’s change over time (Box 1). It is difficult to maintain long term studies, particularly if there is a need to quantify the success of habitat restoration. Martínez et al. (2012) measured EMF presence 10 years after planting inoculated seedlings but this amount of time was not typical for other studies listed in Table 1. Most completed sampling after 5 years and six were completed after a year or less (Colinas et al., 1994; Roldán and Albaladejo, 1994; Sousa et al., 2011, 2012; Onwuchekwa et al., 2014; Patterson, 2019). If the goal is to get a functional and sustainable plant community of choice on the site, it can take longer. Explicitly identifying the target outcome (i.e., re-introduction of certain plant species, reduction of contamination or erosion in the soil, restoration of certain ecosystem functions) will improve the predictions of the time needed for restoration after disturbance. Additionally, the advance in molecular tools is an advantage in EMF research that aims to link EMF community composition to their role in ecosystem functioning (Horton and Bruns, 2001; Koide et al., 2014; Bonfante, 2018). Future research that focuses on determining the functional role of different EMF species for restoration purposes and understanding the context dependency of these roles in terms of the rest of the soil community will aid restoration efforts (Aerts and Honnay, 2011). Newly available molecular tools to quantify microbial diversity, combined with detailed measurements of forest functioning are likely to increase our insights in how to apply belowground biodiversity for restoration purposes.
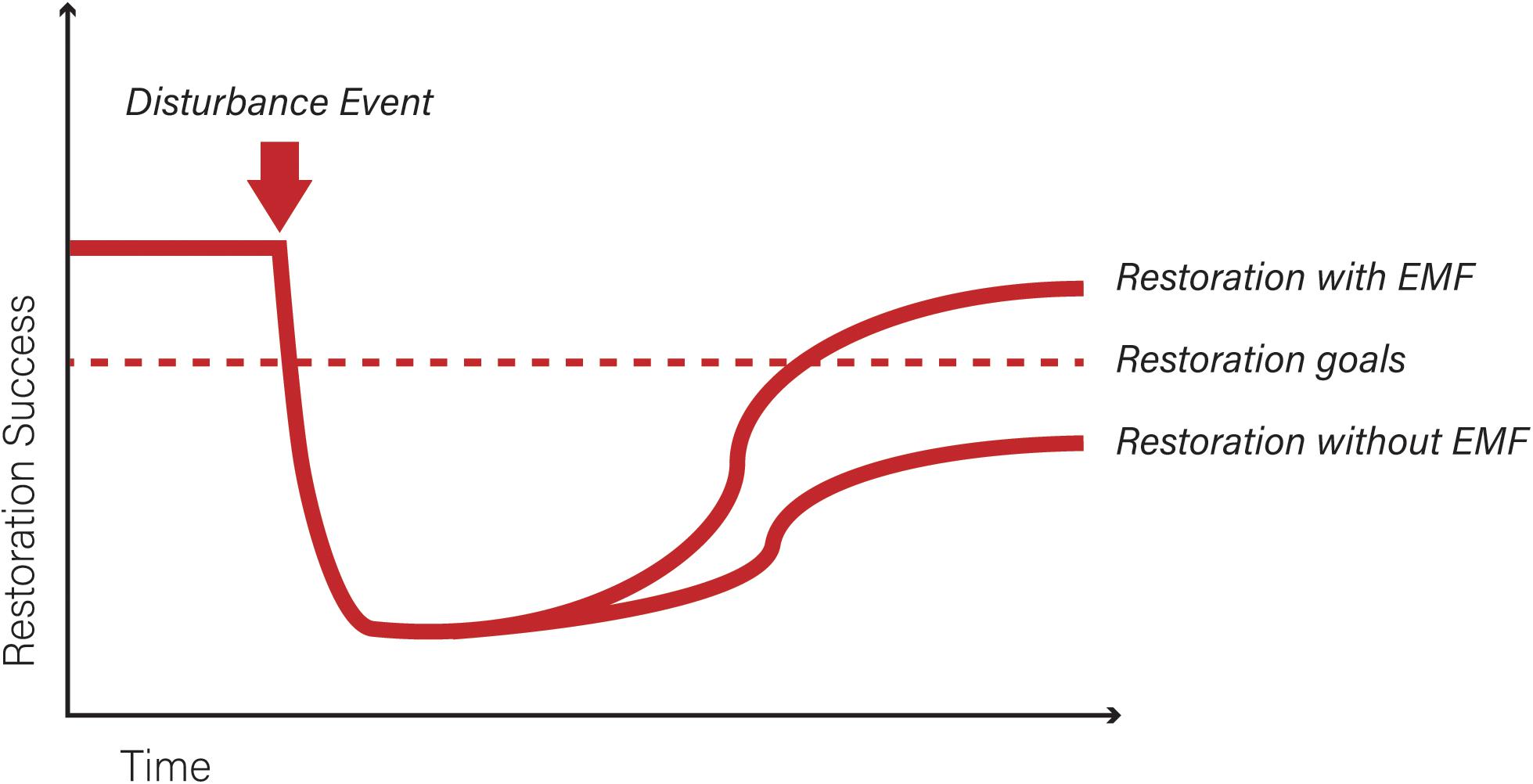
Figure 3. Conceptual model showing restoration success over time. The arrow indicates a disturbance event. The dotted line representstargeted restoration goals at the time of implementation. After a natural or anthropic disturbance event disrupts the original community, a restoration plan is implemented, establishing restoration goals (e.g., restoring specific ecosystem functions, reintroducing a particular species of interest). It is often impossible to recreate the pre-disturbance conditions and there is often a time lag between the initiation of restoration activities and the habitat response to that restoration plan (due to species or communities time to reoccupy suitable restored areas, and time to achieve measurable restoration effects). Although the shapes of the curves and the length of disturbance and success of restoration might be variable and difficult to measure, incorporating suitable EMF in restoration efforts can help increase the rate of restoration success due to increased survival and growth of specific plant hosts, and the improvement of soil properties (e.g., increase soil stability, decrease heavy metal toxicity). Restoration goals could be reached in a shorter amount of time after disturbance compared to a situation in which EMF are not considered and restoration fails to achieve the initial goals. Other trajectories not shown in the figure are possible: if the EMF species are not properly chosen, for example, long-lasting effects might not be attainable and instead of reaching a plateau when restoration goals are achieved, the curve would start decreasing. Monitoring over time is necessary to avoid possible undesired outcomes.
All the studies we found using EMF for temperate and boreal forests restoration were performed in a limited number of locations (Table 1). Experimental evidence of restoration successes or failures should be published from other regions and ecosystems. Particularly in many countries of the Southern Hemisphere where economic limitations may hinder the possibilities of successful restoration programs, EMF offer an economical, sustainable, and long lasting alternative for temperate forest restoration. Moreover, environmental gradients should be further explored to address restoration success variability and suitability of EMF-host pairs. Identifying organisms best adapted to specific environmental and biotic conditions and assessing the potential for managing these organisms are the first steps to introduce the idea of a living soil in restoration, as part of an expanding view of forest ecosystems (Stanturf et al., 2014; Jacobs et al., 2015).
Conclusion
In this article, we address the conceptual incentive and the empirical evidence evaluating EMF as a potential tool for restoration of forest ecosystems. Although there is no “magic bullet” for effective restoration, there is opportunity to use soil organisms as “tools” to achieve restoration success. Restoration projects are not always successful and results are highly context dependent. The relatively low number of studies actively using EMF to restore temperate and boreal forests hinders our capacity to better describe the context dependency of the effectiveness of this restoration technique. At the same time, it emphasizes the necessity of more experimental studies in different climatic zones and disturbance contexts. However, there is evidence that restoration of the native microbiome improves the establishment of plants that are often missing from restored communities (Koziol et al., 2018). An increasing understanding of EMF diversity and functional traits might enhance the ability to better choose and experimentally test which EMF species would work in each restoration scenario. In nursery inoculation and field experiments, native EMF should always be prioritized to avoid possible undesired outcomes (e.g., EMF invasions). Restoration programs considering plant–EMF interactions have been mostly applied in areas affected by soil erosion, heavy metal contamination, wildfire, and clearcut logging. Some of these disturbances are explored to a much lesser extent than others and future experimental approaches should explore other regions and environmental gradients affected by these and other possible disturbances. We outlined some possible undesired outcomes and limitations of the use of EMF in forest restoration, highlighting the idea that not acting does not entail a solution. The use of EMF in forest restoration can be complementary to the increasing application of adaptive management, in which learning and resource management act simultaneously in a context of uncertainty (Jacobs et al., 2015). We suggest a more holistic view of forest management that considers a belowground perspective of forest restoration. Particularly for boreal and temperate forest restoration, we offer evidence that EMF should be considered as a management tool to improve the chances of success of future restoration programs.
Author Contributions
NP and JB conceived the study. NP collected the data and led the writing of the manuscript. NP, TH, AH, TP, and JB participated in data interpretation and revised the manuscript. All authors contributed to the article and approved the submitted version.
Funding
NP was funded by FONCyT grants number PICT 2014-0662 and PICT 2016-1412 of the Agencia Nacional de Promoción Científica y Tecnológica of Argentina and by a Ph.D. scholarship from Consejo Nacional de Investigaciones Científicas y Técnicas of Argentina. JB and NP acknowledges DOE BER awards DE-SC0020403 and AN_360581.
Conflict of Interest
The authors declare that the research was conducted in the absence of any commercial or financial relationships that could be construed as a potential conflict of interest.
References
Aerts, R., and Honnay, O. (2011). Forest restoration, biodiversity and ecosystem functioning. BMC Ecol. 11:29. doi: 10.1186/1472-6785-11-29
Agerer, R. (2001). Exploration types of ectomycorrhizae: a proposal to classify ectomycorrhizal mycelial systems according to their patterns of differentiation and putative ecological importance. Mycorrhiza 11, 107–114. doi: 10.1007/s005720100108
Ashkannejhad, S., and Horton, T. R. (2006). Ectomycorrhizal ecology under primary succession on coastal sand dunes: interactions involving Pinus contorta, suilloid fungi and deer. New Phytol. 169, 345–354. doi: 10.1111/j.1469-8137.2005.01593.x
Asmelash, F., Bekele, T., and Birhane, E. (2016). The potential role of arbuscular mycorrhizal fungi in the restoration of degraded lands. Front. Microbiol. 7:1095. doi: 10.3389/fmicb.2016.01095
Azul, A. M., Nunes, J., Ferreira, I., Coelho, A. S., Veríssimo, P., Trovão, J., et al. (2014). Valuing native ectomycorrhizal fungi as a Mediterranean forestry component for sustainable and innovative solutions. Botany 92, 161–171. doi: 10.1139/cjb-2013-0170
Baar, J., Horton, T. R., Kretzer, A. M., and Bruns, T. D. (1999). Mycorrhizal colonization of Pinus muricata from resistant propagules after a stand-replacing wildfire. New Phytol. 143, 409–418. doi: 10.1046/j.1469-8137.1999.00452.x
Bai, S. L., Li, G. L., Liu, Y., Dumroese, R. K., and Lv, R. H. (2009). Ostryopsis davidiana seedlings inoculated with ectomycorrhizal fungi facilitate formation of mycorrhizae on Pinus tabulaeformis seedlings. Mycorrhiza 19, 425–434. doi: 10.1007/s00572-009-0245-2
Baohanta, R., Thioulouse, J., Ramanankierana, H., Prin, Y., Rasolomampianina, R., Baudoin, E., et al. (2012). Restoring native forest ecosystems after exotic tree plantation in Madagascar: combination of the local ectotrophic species Leptolena bojeriana and Uapaca bojeri mitigates the negative influence of the exotic species Eucalyptus camaldulensis and Pinus pat. Biol. Invasions 14, 2407–2421. doi: 10.1007/s10530-012-0238-5
Bastias, B. A., Xu, Z., and Cairney, J. W. G. (2006). Influence of long-term repeated prescribed burning on mycelial communities of ectomycorrhizal fungi. New Phytol. 172, 149–158. doi: 10.1111/j.1469-8137.2006.01793.x
Bauman, J. M., Keiffer, C. H., and Hiremath, S. (2012). Facilitation of American chestnut (Castanea dentata) seedling establishment by Pinus virginiana in mine restoration. Int. J. Ecol 12:2573. doi: 10.1155/2012/2573
Bauman, J. M., Keiffer, C. H., Hiremath, S., and Mccarthy, B. C. (2013). Soil preparation methods promoting ectomycorrhizal colonization and American chestnut Castanea dentata establishment in coal mine restoration. J. Appl. Ecol. 50, 721–729. doi: 10.1111/1365-2664.12070
Bonfante, P. (2018). The future has roots in the past: the ideas and scientists that shaped mycorrhizal research. New Phytol. 220, 982–995. doi: 10.1111/nph.15397
Bruns, T. D., Peay, K. G., Boynton, P. J., Grubisha, L. C., Hynson, N. A., Nguyen, N. H., et al. (2009). Inoculum potential of Rhizopogon spores increases with time over the first 4 yr of a 99-yr spore burial experiment. New Phytol. 181, 463–470. doi: 10.1111/j.1469-8137.2008.02652.x
Cairney, J. W., and Bastias, B. A. (2007). Influences of fire on forest soil fungal communities. Canad. J. Forest Res. 37, 207–215. doi: 10.1139/x06-190
Caravaca, F., Garcia, C., Hernández, M. T., and Roldán, A. (2002). Aggregate stability changes after organic amendment and mycorrhizal inoculation in the afforestation of a semiarid site with Pinus halepensis. Appl. Soil Ecol. 19, 199–208. doi: 10.1016/S0929-1393(01)00189-5
Chazdon, R. L. (2008). Beyond deforestation: restoring forests and ecosystem services on degraded lands. Science 320, 1458–1460. doi: 10.1126/science.1155365
Claridge, A. W., Trappe, J. M., and Hansen, K. (2009). Do fungi have a role as soil stabilizers and remediators after forest fire? For. Ecol. Manag. 257, 1063–1069. doi: 10.1016/j.foreco.2008.11.011
Colinas, C., Molina, R., Trappe, J., and Perry, D. (1994). Ectomycorrhizas and rhizosphere microorganisms of seedlings of Pseudotsuga menziesii (Mirb.) Franco planted on a degraded site and inoculated with forest soils pretreated with selective biocides. New Phytol. 127, 529–537. doi: 10.1111/j.1469-8137.1994.tb03971.x
DeFries, R. S., Rudel, T., Uriarte, M., and Hansen, M. (2010). Deforestation driven by urban population growth and agricultural trade in the twenty-first century. Nat. Geosci. 3, 178–181. doi: 10.1038/ngeo756
Diaz, E., Roldan, A., Lax, A., and Albaladejo, J. (1994). Formation of stable aggregates in degraded soil by amendment with urban refuse and peat. Geoderma 63, 277–288. doi: 10.1016/0016-7061(94)90069-8
Dickie, I. A., Bolstridge, N., Cooper, J. A., and Peltzer, D. A. (2010). Co-invasion by Pinus and its mychorrhizal fungi. New Phytol. 10, 475–484. doi: 10.1111/j.1469-8137.2010.03277.x
Dickie, I. A., Nuñez, M. A., Pringle, A., Lebel, T., Tourtellot, S. G., and Johnston, P. R. (2016). Towards management of invasive ectomycorrhizal fungi. Biol. Invasions 18, 3383–3395. doi: 10.1007/s10530-016-1243-x
Dickie, I. A., St John, M. G., Yeates, G. W., Morse, C. W., Bonner, K. I., Orwin, K., et al. (2014). Belowground legacies of Pinus contorta invasion and removal result in multiple mechanisms of invasional meltdown. AoB Plants 6, 1–15. doi: 10.1093/aobpla/plu056
Donnelly, P. K., and Fletcher, J. S. (1994). Potential use of mycorrhizal fungi as bioremediation agents. ACS Symp. Ser. 563, 93–99. doi: 10.1021/bk-1994-0563.ch008
Dove, N. C., and Hart, S. C. (2017). Fire reduces fungal species richness and in situ mycorrhizal colonization: a meta-analysis. Fire Ecol. 13, 37–65. doi: 10.4996/fireecology.130237746
Dulmer, K. M., LeDuc, S. D., and Horton, T. R. (2014). Ectomycorrhizal inoculum potential of northeastern US forest soils for American chestnut restoration: results from field and laboratory bioassays. Mycorrhiza 24, 65–74. doi: 10.1007/s00572-013-0514-y
Franco, A. R., Sousa, N. R., Ramos, M. A., Oliveira, R. S., and Castro, P. M. (2014). Diversity and Persistence of ectomycorrhizal fungi and their effect on nursery-inoculated Pinus pinaster in a Post-fire plantation in Northern Portugal. Microb. Ecol. 68, 761–772. doi: 10.1007/s00248-014-0447-9
Glassman, S. I., Levine, C. R., DiRocco, A. M., Battles, J. J., and Bruns, T. D. (2016). Ectomycorrhizal fungal spore bank recovery after a severe forest fire: some like it hot. ISME J. 10, 1228–1239. doi: 10.1038/ismej.2015.182
Gustafsson, L., Baker, S. C., Bauhus, J., Beese, W. J., Brodie, A., Kouki, J., et al. (2012). retention forestry to maintain multifunctional forests: a world perspective. Bioscience 62, 633–645. doi: 10.1525/bio.2012.62.7.6
Harris, J. (2009). Soil microbial communities and restoration ecology: Facilitators or followers? Science 325, 573–574. doi: 10.1126/science.1172975
Hart, S. C., DeLuca, T. H., Newman, G. S., MacKenzie, M. D., and Boyle, S. I. (2005). Post-fire vegetative dynamics as drivers of microbial community structure and function in forest soils. For. Ecol. Manage. 220, 166–184. doi: 10.1016/j.foreco.2005.08.012
Hawkins, B. J., Jones, M. D., and Kranabetter, J. M. (2015). Ectomycorrhizae and tree seedling nitrogen nutrition in forest restoration. New For. 46, 747–771. doi: 10.1007/s11056-015-9488-2
Hawver, C., Batcher, M. S., Kostrzewski, C. D., and Gifford, N. A. (2010). Fire Management Plan for the Albany Pine Bush Preserve (Appendix C: Management Plan and Final Environmental Impact Statement for the Albany Pine Bush Preserve, September 2010). Albany, NY: Department of Environmental Conservation of the Albany Pine Bush Preserve.
Hayward, J., Horton, T. R., and Nuñez, M. A. (2015a). Ectomycorrhizal fungal communities coinvading with Pinaceae host plants in Argentina: Gringos bajo el bosque. New Phytol. 208, 497–506. doi: 10.1111/nph.13453
Hayward, J., Horton, T. R., Pauchard, A., and Nuñez, M. A. (2015b). A single ectomycorrhizal fungal species can enable a Pinus invasion. Ecology 96, 1438–1444. doi: 10.1890/14-1100.1
Heneghan, L., Miller, S. P., Baer, S., Callaham, M. A., Montgomery, J., Pavao-Zuckerman, M., et al. (2008). Integrating soil ecological knowledge into restoration management. Restor. Ecol. 16, 608–617. doi: 10.1111/j.1526-100X.2008.00477.x
Henry, C., Raivoarisoa, J.-F., Razafimamonjy, A., Ramanankierana, H., Andrianaivomahefa, P., Selosse, M.-A., et al. (2015). Asteropeia mcphersonii, a potential mycorrhizal facilitator for ecological restoration in Madagascar wet tropical rainforests. For. Ecol. Manage. 358, 202–211. doi: 10.1016/j.foreco.2015.09.017
Hewitt, R. E., Bennett, A. P., Breen, A. L., Hollingsworth, T. N., Taylor, D. L., Chapin, F. S., et al. (2015). Getting to the root of the matter: landscape implications of plant-fungal interactions for tree migration in Alaska. Lands. Ecol. 31:306. doi: 10.1007/s10980-015-0306-1
Hoeksema, J., Averill, C., Bhatnagar, J. M., Buscardo, E., Brzostek, E., Chen, K. H., et al. (2020). Ectomycorrhizal invasions as natural experiments for connecting plant and fungal traits to their ecosystem consequences. Front. Fore. Glob. Change 20:84. doi: 10.3389/ffgc.2020.00084
Horton, T. R. (2017). “Spore dispersal in ectomycorrhizal fungi at fine and regional scales,” in Biogeography of Mycorrhizal Symbiosis, ed. L. Tedersoo (Cham: Springer International Publishing), 61–78. doi: 10.1007/978-3-319-56363-3_3
Horton, T. R., and Bruns, T. D. (2001). The molecular revolution in ectomycorrhizal ecology: Peeking into the black-box. Mol. Ecol. 10, 1855–1871. doi: 10.1046/j.0962-1083.2001.01333.x
Hudon, A. T. (2019). Fungi of the Albany Pine Bush Preserve, Albany, New York and Assemblage Structure of Ectomycorrhizal Fungi on Quercus Ilicifolia (scrub oak) Roots in Fire Adapted Pine Barrens. Available from Dissertations & Theses at SUNY College of Environmental Science and Forestry. New York, NY: ProQuest Dissertations Publishing.
Jacobs, D. F., Oliet, J. A., Aronson, J., Bolte, A., Bullock, J. M., Donoso, P. J., et al. (2015). Restoring forests: what constitutes success in the twenty-first century? New For. 46, 601–614. doi: 10.1007/s11056-015-9513-5
Jeffries, P., Gianinazzi, S., and Perotto, S. (2003). The contribution of arbuscular mycorrhizal fungi in sustainable maintenance of plant health and soil fertility. Biol. Fertil Soils 37, 1–16. doi: 10.1007/s00374-002-0546-5
Jones, M. D., Durall, D. M., and Cairney, J. W. G. (2003). Ectomycorrhizal fungal communities in young forest stands regenerating after clearcut logging. New Phytol. 157, 399–422. doi: 10.1046/j.1469-8137.2003.00698.x
Jourand, P., Hannibal, L., Majorel, C., Mengant, S., Ducousso, M., and Lebrun, M. (2014). Ectomycorrhizal Pisolithus albus inoculation of Acacia spirorbis and Eucalyptus globulus grown in ultramafic topsoil enhances plant growth and mineral nutrition while limits metal uptake. J. Plant Physiol. 171, 164–172. doi: 10.1016/j.jplph.2013.10.011
Kalucka, I. L., and Jagodzinski, A. M. (2016). Successional traits of ectomycorrhizal fungi in forest reclamation after surface mining and agricultural disturbances: a review. Dendrobiology 76, 91–104. doi: 10.12657/denbio.076.009
Kennedy, P. G., Smith, D. P., Horton, T. R., and Molina, R. J. (2012). Arbutus menziesii (Ericaceae) facilitates regeneration dynamics in mixed evergreen forests by promoting mycorrhizal fungal diversity and host connectivity. Am. J. Bot. 99, 1691–1701. doi: 10.3732/ajb.1200277
Khan, A. G., Kuek, C., Chaudhry, T. M., Khoo, C. S., and Hayes, W. J. (2000). Role of plants, mycorrhizae and phytochelators in heavy metal contaminated land remediation. Chemosphere 41, 197–207. doi: 10.1016/S0045-6535(99)00412-9
Koide, R. T., Fernandez, C., and Malcolm, G. (2014). Determining place and process: functional traits of ectomycorrhizal fungi that affect both community structure and ecosystem function. New Phytol. 201, 433–439. doi: 10.1111/nph.12538
Koziol, L., Schultz, P. A., House, G. L., Bauer, J. T., Middleton, E. L., and Bever, J. D. (2018). The plant microbiome and native plant restoration: the example of native mycorrhizal fungi. Bioscience 68, 996–1006. doi: 10.1093/biosci/biy125
Krumins, J. A., Goodey, N. M., and Gallagher, F. (2015). Plant–soil interactions in metal contaminated soils. Soil Biol. Biochem. 80, 224–231. doi: 10.1016/j.soilbio.2014.10.011
Lesica, P., and Allendorf, F. W. (1999). Ecological genetics and the restoration of plant communities: mix or match? Restor. Ecol. 7, 42–50. doi: 10.1046/j.1526-100X.1999.07105.x
Leyval, C., Turnau, K., and Haselwandter, K. (1997). Effect of heavy metal pollution on mycorrhizal colonization and function: physiological, ecological and applied aspects. Mycorrhiza 7, 139–153. doi: 10.1007/s005720050174
Lilleskov, E. A., Fahey, T. J., Horton, T. R., and Lovett, G. M. (2002). Belowground ectomycorrhizal fungal community change over a nitrogen deposition gradient in alaska. Ecology 83, 104–115. doi: 10.1890/0012-96582002083[0104:BEFCCO]2.0.CO;2
Löf, M., Dey, D. C., Navarro, R. M., and Jacobs, D. F. (2012). Mechanical site preparation for forest restoration. New Forests 43, 825–848. doi: 10.1007/s11056-012-9332-x
Luo, Z., Bin, Wu, C., Zhang, C., Li, H., Lipka, U., et al. (2014). The role of ectomycorrhizas in heavy metal stress tolerance of host plants. Environ. Exp. Bot. 108, 47–62. doi: 10.1016/j.envexpbot.2013.10.018
Malajczuk, N., Reddell, P., and Brundrett, M. C. (1994). “Role of ectomycorrhizal fungi in minesite reclamation,” in A Reappraisal of Mycorrhizae in Plant Health, eds F. Pfleger and B. Linderman (Washingdon, DC: The American Phytopathological Society), 83–100.
Maltz, M. R., and Treseder, K. K. (2015). Sources of inocula influence mycorrhizal colonization of plants in restoration projects: a meta-analysis. Restor. Ecol. 15:12231. doi: 10.1111/rec.12231
Martínez, A. J. M., Fischer, C., Bonet, J. A., Olivera, A., Oliach, D., et al. (2012). Economically profitable post fire restoration with black truffle (Tuber melanosporum) producing plantations. New For. 43, 615–630. doi: 10.1007/s11056-012-9316-x
Menkis, A., Vasiliauskas, R., Taylor, A. F. S., Stenlid, J., and Finlay, R. (2007). Afforestation of abandoned farmland with conifer seedlings inoculated with three ectomycorrhizal fungi - Impact on plant performance and ectomycorrhizal community. Mycorrhiza 17, 337–348. doi: 10.1007/s00572-007-0110-0
Molina, R., Massicotte, H., and Trappe, J. M. (1992). Specificity Phenomena in Mycorrhizal Symbioses: Community-Ecological Consequences and Practical Implications. Mycorrhizal Functioning: An Integrative Plant-Fungal Process. New York, NY: Chapman and Hall, 357–423.
Nuñez, M. A., Chiuffo, M. C., Torres, A., Paul, T., Dimarco, R. D., Raal, P., et al. (2017). Ecology and management of invasive Pinaceae around the world: progress and challenges. Biol. Invasions 19, 3099–3120. doi: 10.1007/s10530-017-1483-4
Nuñez, M. A., and Dickie, I. A. (2014). Invasive belowground mutualists of woody plants. Biol. Invasions 16, 645–661. doi: 10.1007/s10530-013-0612-y
Nuñez, M. A., Horton, T. R., and Simberloff, D. (2009). Lack of belowground mutualisms hinders Pinaceae invasions. Ecology 90, 2352–2359. doi: 10.1890/08-2139.1
Onwuchekwa, N. E., Zwiazek, J. J., Quoreshi, A., and Khasa, D. P. (2014). Growth of mycorrhizal jack pine (Pinus banksiana) and white spruce (Picea glauca) seedlings planted in oil sands reclaimed areas. Mycorrhiza 24, 431–441. doi: 10.1007/s00572-014-0555-x
Palfner, G., Canseco, M. I., and Casanova-Katny, A. (2008). Post-fire seedlings of Nothofagus alpina in Southern Chile show strong dominance of a single ectomycorrhizal fungus and a vertical shift in root architecture. Plant Soil 313, 237–250. doi: 10.1007/s11104-008-9697-y
Patterson, T. (2019). Legacy of Robinia Pseudoacacia Invasion and use of Ectomycorrhizal Fungi to Restore Pinus Rigida in the Albany Pine Bush Preserve, NY. Available from Dissertations & Theses at SUNY College of Environmental Science and Forestry. New York, NY: ProQuest Dissertations Publishing.
Pec, G. J., Scott, N. M., Hupperts, S. F., Hankin, S. L., Landhäusser, S. M., and Karst, J. (2019). Restoration of belowground fungal communities in reclaimed landscapes of the Canadian boreal forest. Restor. Ecol. 27, 1369–1380. doi: 10.1111/rec.12990
Phillips, R. P., Brzostek, E., and Midgley, M. G. (2013). The mycorrhizal-associated nutrient economy: a new framework for predicting carbon-nutrient couplings in temperate forests. New Phytol. 199, 41–51. doi: 10.1111/nph.12221
Piñeiro, J., Maestre, F. T., Bartolomé, L., and Valdecantos, A. (2013). Ecotechnology as a tool for restoring degraded drylands: a meta-analysis of field experiments. Ecol. Eng. 61, 133–144. doi: 10.1016/j.ecoleng.2013.09.066
Policelli, N., Bruns, T. D., Vilgalys, R., and Nuñez, M. A. (2019). Suilloid fungi as global drivers of pine invasions. New Phytol. 222, 714–725. doi: 10.1111/nph.15660
Policelli, N., Horton, T. R., Garcia, R. A., Naour, M., Pauchard, A., and Nuñez, M. A. (2020). Native and non-native trees can find proper mycorrhizal partners in each other’s dominated areas. Plant Soil. 20:4609. doi: 10.1007/s11104-020-04609-x
Powell, C. L. (1980). Mycorrhizal infectivity of eroded soils. Soil Biol. Biochem. 12, 247–250. doi: 10.1016/0038-0717(80)90069-3
Pringle, A., Bever, J. D., Gardes, M., Parrent, J. L., Rillig, M. C., and Klironomos, J. N. (2009). Mycorrhizal symbioses and plant invasions. Annu. Rev. Ecol. Evol. Syst. 40, 699–715. doi: 10.1146/annurev.ecolsys.39.110707.173454
Pringle, A., and Vellinga, E. C. (2006). Last chance to know? Using literature to explore the biogeography and invasion biology of the death cap mushroom Amanita phalloides (Vaill. ex Fr.:Fr.) link. Biol. Invasions 8, 1131–1144. doi: 10.1007/s10530-005-3804-2
Requena, N., Perez-Solis, E., Azcon-Aguilar, C., Jeffries, P., and Barea, J.-M. (2001). Management of indigenous plant-microbe symbioses aids restoration of desertified ecosystems. Appl. Environ. Microbiol. 67, 495–498. doi: 10.1128/AEM.67.2.495-498.2001
Richard, F., Selosse, M. A., and Gardes, M. (2009). Facilitated establishment of Quercus ilex in shrub-dominated communities within a Mediterranean ecosystem: do mycorrhizal partners matter? FEMS Microbiol. Ecol. 68, 14–24. doi: 10.1111/j.1574-6941.2009.00646.x
Richardson, D. M., and Rejmánek, M. (2011). Trees and shrubs as invasive alien species - a global review. Divers. Distrib. 17, 788–809. doi: 10.1111/j.1472-4642.2011.00782.x
Richter, B. S., and Stutz, J. C. (2002). Mycorrhizal inoculation of big sacaton: Implications for grassland restoration of abandoned agricultural fields. Restor. Ecol. 10, 607–616. doi: 10.1046/j.1526-100X.2002.01041.x
Rincón, A., De Felipe, M. R., and Fernández-Pascual, M. (2007). Inoculation of Pinus halepensis Mill. with selected ectomycorrhizal fungi improves seedling establishment 2 years after planting in a degraded gypsum soil. Mycorrhiza 18, 23–32. doi: 10.1007/s00572-007-0149-y
Roldán, A., and Albaladejo, J. (1994). Effect of mycorrhizal inoculation and soil restoration on the growth of Pinus halepensis seedlings in a semiarid soil. Biol. Fertil. Soils 18, 143–149. doi: 10.1007/BF00336461
Roose, E. J., Lal, R., Feller, C., and Barthes, B. (Eds). (2006). Soil Erosion and Carbon Dynamics. Boca Ratón, FL: CRC Press.
Rühling, Å, and Söderström, B. (1990). Changes in fruitbody production of mycorrhizal and litter decomposing macromycetes in heavy metal polluted coniferous forests in North Sweden. Water Air Soil Pollut. 49, 375–387. doi: 10.1007/BF00507077
Sebastiana, M., Pereira, V. T., Alcântara, A., Pais, M. S., and Silva, A. B. (2013). Ectomycorrhizal inoculation with Pisolithus tinctorius increases the performance of Quercus suber L. (cork oak) nursery and field seedlings. New For. 44, 937–949. doi: 10.1007/s11056-013-9386-4
Simard, S. W. (2009). The foundational role of mycorrhizal networks in self-organization of interior Douglas-fir forests. For. Ecol. Manage. 258, 95–107. doi: 10.1016/j.foreco.2009.05.001
Simard, S. W., and Durall, D. M. (2004). Mycorrhizal networks: A review of their extent, function, and importance. Can. J. Bot. 82, 1140–1165. doi: 10.1139/B04-116
Simberloff, D., Nuñez, M. A., Ledgard, N. J., Pauchard, A., Richardson, D. M., Sarasola, M., et al. (2010). Spread and impact of introduced conifers in South America: lessons from other southern hemisphere regions. Austral Ecol. 35, 489–504. doi: 10.1111/j.1442-9993.2009.02058.x
Smith, J. E., McKay, D., Brenner, G., McIver, J., and Spatafora, J. W. (2005). Early impacts of forest restoration treatments on the ectomycorrhizal fungal community and fine root biomass in a mixed conifer forest. J. Appl. Ecol. 42, 526–535. doi: 10.1111/j.1365-2664.2005.01047.x
Smith, M. R., Charvat, I., and Jacobson, R. L. (1998). Arbuscular mycorrhizae promote establishment of prairie species in a tallgrass prairie restoration. Can. J. Bot. 76, 1947–1954. doi: 10.1139/b98-205
Smith, S. E., and Read, D. J. (2008). Mycorrhizal Symbiosis, 3rd editio Edn. Cambridge, MA: Academic Press. doi: 10.1016/B978-0-12-652840-4.X5000-1
Soudzilovskaia, N. A., Van Bodegom, P. M., Terrer, C., Zelfde, M., van’t, McCallum, I., et al. (2019). Global mycorrhizal plant distribution linked to terrestrial carbon stocks. Nat. Commun. 10:5077. doi: 10.1038/s41467-019-13019-2
Sousa, N. R., Franco, A. R., Ramos, M. A., Oliveira, R. S., and Castro, P. M. (2011). Reforestation of burned stands: the effect of ectomycorrhizal fungi on Pinus pinaster establishment. Soil Biol. Biochem. 43, 2115–2120. doi: 10.1016/j.soilbio.2011.06.013
Sousa, N. R., Ramos, M. A., Marques, A. P. G. C., and Castro, P. M. L. (2012). The effect of ectomycorrhizal fungi forming symbiosis with Pinus pinaster seedlings exposed to cadmium. Sci. Total Environ. 414, 63–67. doi: 10.1016/j.scitotenv.2011.10.053
Sousa, N. R., Ramos, M. A., Marques, A. P. G. C., and Castro, P. M. L. (2014). A genotype dependent-response to cadmium contamination in soil is displayed by Pinus pinaster in symbiosis with different mycorrhizal fungi. Appl. Soil Ecol. 76, 7–13. doi: 10.1016/j.apsoil.2013.12.005
Stanturf, J. A., Palik, B. J., and Dumroese, R. K. (2014). Contemporary forest restoration: a review emphasizing function. For. Ecol. Manage. 331, 292–323. doi: 10.1016/j.foreco.2014.07.029
Steidinger, B. S., Crowther, T. W., Liang, J., Van Nuland, M. E., Werner, G. D. A., Reich, P. B., et al. (2019). Climatic controls of decomposition drive the global biogeography of forest-tree symbioses. Nature 569, 404–408. doi: 10.1038/s41586-019-1128-0
Stendell, E. R., Horton, T. R., and Bruns, T. D. (1999). Early effects of prescribed fire on the structure of the ectomycorrhizal fungus community in a Sierra Nevada ponderosa pine forest. Mycol. Res. 103, 1353–1359. doi: 10.1017/S0953756299008618
Sterkenburg, E., Clemmensen, K. E., Lindahl, B. D., and Dahlberg, A. (2019). The significance of retention trees for survival of ectomycorrhizal fungi in clear−cut Scots pine forests. J. Appl. Ecol. 56, 1367–1378. doi: 10.1111/1365-2664.13363
Sýkorová, Z., Rydlová, J., Slavíková, R., Ness, T., Kohout, P., and Püschel, D. (2016). Forest reclamation of fly ash deposit: a field study on appraisal of mycorrhizal inoculation. Restor. Ecol. 24, 184–193. doi: 10.1111/rec.12301
Tarbell, T. J., and Koske, R. E. (2007). Evaluation of commercial arbuscular mycorrhizal inocula in a sand/peat medium. Mycorrhiza 18, 51–56. doi: 10.1007/s00572-007-0152-3
Teste, F. P., Schmidt, M. G., Berch, S. M., Bulmer, C., and Egger, K. N. (2004). Effects of ectomycorrhizal inoculants on survival and growth of interior Douglas-fir seedlings on reforestation sites and partially rehabilitated landings Effects of ectomycorrhizal inoculants on survival and growth of interior Douglas-fir seedlings on re. Canad. J. Forest Res. 34, 2074–2088. doi: 10.1139/x04-083
Tosh, J. E., Senior, E., Smith, J. E., and Watson−Craik, I. A. (1993). The role of ectomycorrhizal inoculations in landfill site restoration programmes. Lett. Appl. Microbiol. 16, 187–191. doi: 10.1111/j.1472-765X.1993.tb01393.x
Trappe, J. M. (1977). Selection of fungi for ectomycorrhizal inoculation in nurseries. Annu. Rev. Phytopathol. 15, 203–222. doi: 10.1146/annurev.py.15.090177.001223
Van Der Heijden, M. G. A., and Horton, T. R. (2009). Socialism in soil? the importance of mycorrhizal fungal networks for facilitation in natural ecosystems. J. Ecol. 97, 1139–1150. doi: 10.1111/j.1365-2745.2009.01570.x
Vellinga, E. C., Wolfe, B. E., and Pringle, A. (2009). Global patterns of ectomycorrhizal introductions. New Phytol. 181, 960–973. doi: 10.1111/j.1469-8137.2008.02728.x
Walker, R. F. (1999). Reforestation of an eastern sierra nevada surface mine with containerized jeffrey pine: seedling growth and nutritional responses to controlled release fertilization and ectomycorrhizal inoculation. J. Sustain. For. 9, 127–147. doi: 10.1300/J091v09n03_06
Walker, R. F. (2003). Comparison of organic and chemical soil amendments used in the reforestation of a Harsh Sierra Nevada site. Restor. Ecol. 11, 466–474. doi: 10.1046/j.1526-100X.2003.rec0216.x
Wan, S., Hui, D., and Luo, Y. (2001). Fire effects on nitrogen pools and dynamics in terrestrial ecosystems: a meta−analysis. Ecol. Appl. 11, 1349–1365. doi: 10.1890/1051-07612001011[1349:FEONPA]2.0.CO;2
White, J. A., Tallaksen, J., and Charvat, I. (2008). The effects of arbuscular mycorrhizal fungal inoculation at a roadside prairie restoration site. Mycologia 100, 6–11. doi: 10.3852/mycologia.100.1.6
Wilkins, D. A. (1991). The influence of sheating (ecto-)mycorrhizas of trees on the uptake and toxicity of metals. Agric. Ecosyst. Environ. 35, 245–260. doi: 10.1016/0167-8809(91)90053-Z
Wolfe, B. E., and Klironomos, J. N. (2005). Breaking new ground: Soil communities and exotic plant invasion. Bioscience 55, 477–487. doi: 10.1641/0006-35682005055[0477:bngsca]2.0.co;2
Wolfe, B. E., Richard, F., Cross, H. B., and Pringle, A. (2010). Distribution and abundance of the introduced ectomycorrhizal fungus Amanita phalloides in North America. New Phytol. 185, 803–816. doi: 10.1111/j.1469-8137.2009.03097.x
Keywords: belowground biota, clearcutting, soil erosion, plant-soil feedbacks, post-fire restoration, mining reclamation, soil management
Citation: Policelli N, Horton TR, Hudon AT, Patterson TR and Bhatnagar JM (2020) Back to Roots: The Role of Ectomycorrhizal Fungi in Boreal and Temperate Forest Restoration. Front. For. Glob. Change 3:97. doi: 10.3389/ffgc.2020.00097
Received: 18 January 2020; Accepted: 16 July 2020;
Published: 06 August 2020.
Edited by:
Peyton Smith, Texas A&M University College Station, United StatesReviewed by:
Jörg Luster, Swiss Federal Institute for Forest, Snow and Landscape Research (WSL), SwitzerlandZachary E. Kayler, University of Idaho, United States
Copyright © 2020 Policelli, Horton, Hudon, Patterson and Bhatnagar. This is an open-access article distributed under the terms of the Creative Commons Attribution License (CC BY). The use, distribution or reproduction in other forums is permitted, provided the original author(s) and the copyright owner(s) are credited and that the original publication in this journal is cited, in accordance with accepted academic practice. No use, distribution or reproduction is permitted which does not comply with these terms.
*Correspondence: Nahuel Policelli, npol@bu.edu; npolicelli@comahue-conicet.gob.ar