- Chair of Soil Science, Center of Life and Food Sciences Weihenstephan, Technical University München, Freising, Germany
Phosphorus (P) nutrition of forest trees depends on soil P supply, which is poor on sites with sandy, quartz-rich soils and shallow calcareous soils. Soil P is distributed heterogeneously at different spatial scales. I tested the hypothesis that in P-limited forest ecosystems soil P enrichment in hotspots results in improved tree P nutrition and growth compared to soils where the same P amount is distributed evenly. In two field P fertilization experiments, Norway spruce (Picea abies) and European beech (Fagus sylvatica) seedlings were grown in soils with experimental homogeneous or heterogeneous P enrichment. The soils were amended with P to equal amounts of total P, but different patterns of P enrichment and/or as different P forms (orthophosphate [oPO4]; inositol hexaphosphate [IHP]). One experiment (Achenpass) was conducted with P-poor calcareous soil material of a Rendzic Leptosol (low-P soil), the other (Mitterfels) with Bw horizon material of a Cambisol that had formed on silicate bedrock and was characterized by moderate P concentrations (moderate-P soil). Half of the spruce seedlings additionally received experimentally elevated N deposition. At the low-P calcareous site, shoot and foliage biomass as well as foliar P, N, and S amounts of Norway spruce seedlings were considerably (+70–80%) and significantly larger on soils with spatially heterogeneous compared to homogeneous P distribution. Elevated N deposition reduced soil P heterogeneity effects on spruce by improving seedling growth on soils with homogeneous P distribution. No soil P form diversity effects were observed for spruce seedlings on calcareous soil. At the silicate site with moderate P supply, all seedlings showed excellent P nutrition. Here, only marginal, insignificant positive effects of heterogeneous soil P distribution were observed for the growth of spruce and beech seedlings without elevated N deposition, but foliar P concentrations of spruce seedlings increased significantly. Elevated N deposition resulted in a positive effect of heterogeneous vs. homogeneous soil P distribution on spruce growth (+39%; eta squared: 0.163; p = 0.135) and P nutrition. Our results showed that soil P concentration heterogeneity is beneficial for spruce growth and P nutrition at sites with P-poor calcareous soils, and at silicate sites with ongoing N eutrophication.
Introduction
For most forest ecosystems, the majority of its total phosphorus (P) stock is bound in the soil. Soil P is present as different inorganic (phosphate bound to Al, Fe, or Ca minerals; Prietzel et al., 2016) and organic P species (mostly mono- and diesters; Condron et al., 2005). Soils are the central P cycling hub of forest ecosystems, sustaining plant P supply (Walker and Syers, 1976; Wardle et al., 2004), and P acquisition traits of plants and soil microorganisms in forest ecosystems are largely determined by ecosystem P availability (Lang et al., 2016, 2017). Phosphorus nutrition of all major tree species in forests of Central Europe such as Norway spruce (Picea abies) and European beech (Fagus sylvatica) strongly depends on soil P supply which is particularly poor on sites with sandy, quartz-rich soils (Ilg et al., 2009; Achat et al., 2016; Lang et al., 2016, 2017; Augusto et al., 2017) or shallow initial calcareous soils (Prietzel et al., 2015, 2016). Insufficient P nutrition is a critical factor for growth and vitality of the key tree species in these forests. During recent decades, tree P nutrition in many European forest ecosystems has deteriorated markedly, which often has been attributed to effects of elevated atmospheric N deposition (Mohren et al., 1986; Prietzel and Stetter, 2010; Jonard et al., 2015).
Due to its low diffusivity, P in forest soils often is distributed in a heterogeneous pattern at different spatial scales (Werner et al., 2017a,b). In soil micro-regions with increased P loading mean P binding strength to soil minerals is decreased, and soil solution P concentration is increased. It can be assumed that P in hotspots can be acquired more easily by tree roots and associated mycorrhizal fungal communities than P in soil regions with lower P concentration, because plant roots and mycorrhizal fungi can acquire more soil P with little carbon investment due to localized root proliferation and increased P uptake kinetics (Caldwell et al., 1992). Enrichment of P in distinct soil hotspots thus should result in improved tree P nutrition and growth compared to soils where the same total P stock is distributed evenly. This has been shown for other plants than forest trees before. Facelli and Facelli (2002) in a mesocosm experiment showed increased growth and biomass of clover (Trifolium subterraneum), when P was distributed heterogeneously rather than homogeneously in the soil. However, experimental evidence for beneficial effects of heterogeneous soil P distribution on plant P nutrition still are scarce, and soil P heterogeneity effects on plant growth differ among soils and plant species with different P acquisition traits (Casper and Cahill, 1996, 1998). At present, no information is available concerning effects of soil P heterogeneity on forest tree growth and vitality.
To decrease this knowledge deficit, I conducted two field experiments using model soil ecosystems with defined spatial heterogeneity patterns of chemical soil properties, particularly P concentration and/or P speciation. I wanted to investigate whether two key European forest tree species (Norway spruce—Picea abies L. [Karst.]; European beech—Fagus sylvatica) seedlings grow faster and show better P nutrition on soils with heterogeneous compared to homogeneous P distribution. The experiment was conducted at two forest sites. One site has P-poor dolostone bedrock, the other site has silicate bedrock with moderate P content. In different experimental variants, the investigated P heterogeneity effects included P concentration heterogeneity as well as P species diversity.
The basic hypothesis was that in P-limited forest ecosystems soil P enrichment in hotspots results in improved tree P nutrition and growth compared to soils where the same P amount is distributed evenly.
Specifically, I tested the following hypotheses:
(1) Soil P heterogeneity is beneficial for P nutrition and growth of P. abies and F. sylvatica.
(2) Inorganic soil P (orthophosphate) supports P nutrition and growth of P. abies and F. sylvatica better than organic soil P (in our experiment added as inositol hexaphosphate [IHP], which is a predominant organic P form in forest soils; Turner et al., 2002, 2007).
(3) Combination of increased spatial P heterogeneity with increased P species diversity supports P nutrition and growth of P. abies and F. sylvatica better than mere presence of spatial heterogeneity.
(4) Elevated nitrogen deposition changes growth and nutrition responses of P. abies to different soil P heterogeneity patterns.
Materials and Methods
Study Sites
The field experiments were conducted at the sites Achenpass (low-P calcareous site) and Mitterfels (moderate-P silicate site). The Achenpass experiment was established on a small (20 m × 20 m) plateau 47°35′43″N; 11°37′54″E) at 1,130 m elevation on the southern flank of the Guggenauer Köpfl (Mangfall Mts., Germany). The site has been described in detail earlier (Biermayer and Rehfuess, 1985; Prietzel and Ammer, 2008). The climate is cool and humid (MAT 5.5°C; MAP 2110 mm), with a precipitation maximum in July and August. The natural forest community is mixed mountain forest, consisting of Norway spruce, European beech, and silver fir (Abies alba), with some admixed Sycamore maple (Acer pseudoplatanus) and whitebeam (Sorbus aria). Parent material is P-poor (0.15 mg P g−1) Triassic dolostone of the Hauptdolomit formation. Dominating soils are shallow Rendzic Leptosols with low P contents (Prietzel et al., 2016), in the following termed “low-P soil.”
The Mitterfels experiment (location 48°58′35″N; 12°52′49″E) was established in 1,020 m elevation in a mature European beech stand in close vicinity to the Forest Climate Station Mitterfels of the Bavarian State Institute of Forestry (Bayerische Landesanstalt für Wald und Forstwirtschaft; LWF). The site and the soil both have been described repeatedly (Lang et al., 2016, 2017; Werner et al., 2017b). Similar to Achenpass, the climate at Mitterfels is cool and humid (MAT 4.9°C; MAP 1229 mm), with a precipitation maximum in July and August. Natural forest type is a mixed mountain forest, consisting of Norway spruce, European beech, and silver fir. The current forest is a mature pure European beech forest. Parent material is Paleozoic gneiss with moderate P content (1.38 mg P g−1). Dominating soils are Dystric Cambisols with silt-loam texture, in the following text termed “moderate-P soil.” The P speciation and important properties of a soil profile close to the experimental plot were presented in detail in Prietzel et al. (2016) and in Werner et al. (2017b).
Experiment Construction and Execution
Low-P Calcareous Site Achenpass
The Achenpass experiment was constructed in autumn 2015. At the plateau described above, we removed the entire soil down to the solid bedrock on a 25 m2 square plot. The removed soil material was separated by horizon (Ah horizon: pHCaCl2 6.6 / 83 mg OC g−1/ 9 mg carbonate-C g−1/ 0.57 mg P g−1; BwC horizon: pHCaCl2 7.9 / 18 mg OC g−1/103 mg g−1 carbonate-C g−1/<0.1 mg P g−1). We leveled out the ground surface by application of dolomite grit (mean grain diameter 0.5–0.8 cm; 0.1 mg P g−1). On the grit cover (thickness 5–15 cm, depending on original bedrock surface topography), we set up a square timber framework construction (height 40 cm), forming four 2 m × 2 m squares separated by walking stages. The four squares formed the four replicated blocks of our experiment (blocks 1–4; Figure 1A). Adding additional timber boards, we divided each square into four 1 m × 1 m square sections, forming the four different experimental variants A–D described below. The experimental variants A–D were arranged randomly in the different blocks (Figure 1A). In each 1 m × 1 m square, a PVC framework (height of PVC plates 20 cm; length 1 m) was inserted, resulting in division of each square into 36 square or rectangular compartments (Figures 1B,C). Then we filled each compartment with a homogenized 1:1 mixture of dolomite grit with Bw material (“BwC mixture”), which for the experimental variants A–D had been enriched with IHP and/or apatite in different ratios (Table 1; Figures 2A–D). Details concerning this procedure and other experimental design details are presented in the Supporting Information.
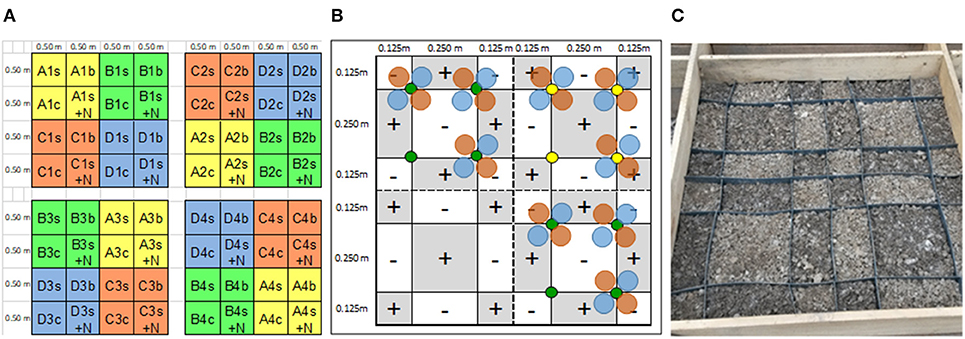
Figure 1. Schematic view showing the layout of the P heterogeneity experiments at sites Achenpass and Mitterfels. (A) Distribution of the four treatment variants (A–D; capital letters; each covering four replicated 1 m2 squares); the four replicated blocks of each treatment variant (1–4), and the four 1 m2 subvariants (tree species: s, spruce; b, beech; +N: experimentally elevated N deposition; C: control) in each treatment variant. (B) Spatial arrangement of artificial soil heterogeneity in each 1 m2 treatment variant. Circles indicate planting positions of spruce (green) and beech (yellow) seedling. “+” and “–” compartments are filled with identical artificial soil material for the “homogeneous” experimental variants, and with different soil materials for the “heterogeneous” variants, separated by PVC walls. (C) Photograph of one 1 m2 tree species variant plot of the Achenpass experiment before being homogeneously covered with Ah horizon material.
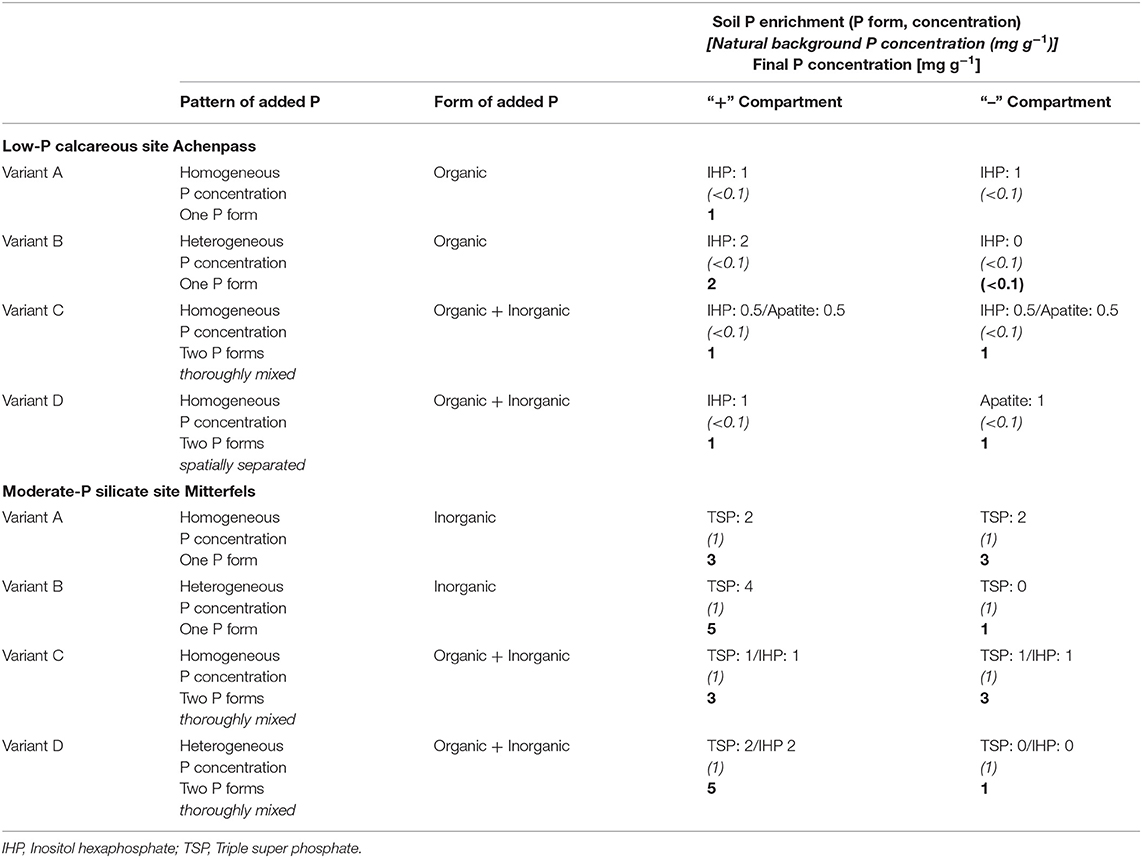
Table 1. Soil P enrichment patterns of “+” and “–” Compartments in different variants of the P heterogeneity experiments Achenpass and Mitterfels.
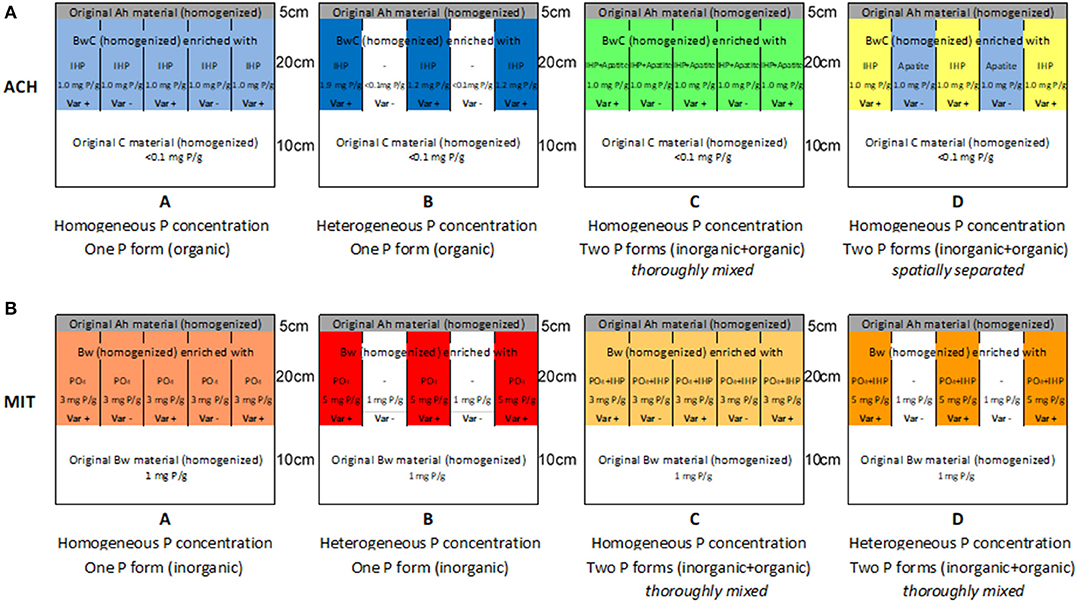
Figure 2. Spatial P concentration heterogeneity patterns and added P forms (vertical profile of 1 m2 soil squares) in different experimental variants of the experiments Achenpass (A) and Mitterfels (B).
After all compartments had been filled with the respective BwC mixtures to the top (Figure 1C), we divided each 1 m × 1 m square section representing an experimental variant in each block into 4 squares (size 0.5 m × 0.5 m; area 0.25 m2) by inserting a cross made of two wooden boards in each 1 m2 square. Then each square was covered with 5 cm original BwC mixture without P addition. On top we homogeneously applied a 5 cm topsoil cover of original Ah horizon material to improve water and nutrient (N, S, K) supply to the seedlings and to warrant seedling infection and colonization with appropriate, site-typical mycorrhizal fungi and soil fauna which is important for soil P cycling (Irshad et al., 2012). The construction was completed in October 2015. In May 2016, 128 seedlings of Norway spruce (Picea abies; height 10–15 cm) were excavated in the surrounding forest. Four seedlings each were planted in the upper left and lower right 0.25 m2 squares of all 16 1m2 squares representing the variants A–D in each experimental block. The squares are indicated by lower case “s” (spruce) and “s+N” (spruce with experimental N deposition) letters in Figure 1A. During planting, we paid utmost attention (i) to use only the uppermost Ah horizon cover as rooting medium, and (ii) to place the plants exactly on the position designated by the green circles in Figure 1B. This assured an equal probability of the developing tree roots to penetrate any of the four different BwC horizon compartments below each plant. In the same way, we planted four Fagus sylvatica seedlings (age: 1 yr) in the upper right 0.5 m × 0.5 m squares of the 16 1m2 squares representing the variants A–D in each block, indicated by lower case “b” (beech) letters in Figure 1A. Planting positions are marked by yellow circles in Figure 1B. The lower left 0.25 m2 square of each 1 m2 square, indicated by a lower case “c” letter in Figure 1A, remained unplanted and served as control variant.
Growth and vitality of all trees were monitored by visual inspection and measurement of shoot heights and shoot basal diameters at the beginning and the end of each growing season, starting in May 2016. Whereas, beech seedlings showed few mortalities, initial losses were considerable (25% of planted seedlings) for spruce. Losses were replaced once in September 2016. At each growth inventory date, the spruce seedlings of subvariants “s+N” received a treatment with dilute NH4NO3 to simulate elevated atmospheric N deposition. At each date, 1.43 g NH4NO3 were dissolved in 100 mL H2O, and the solution was distributed homogenously by spraying on the soil surface (0.25 m2) of each “s+N” plot, resulting in an extra input of 40 kg N ha−1yr−1 in addition to the ambient atmospheric deposition of about 10 kg N ha−1yr−1. Five NH4NO3 applications resulted in a total additional N input of 100 kg ha−1 until termination of the Achenpass experiment in July 2018.
Moderate-P Silicate Site Mitterfels
The Mitterfels experiment (Table 1; Figures 2B, 3C,D) had a similar design as Achenpass (Figures 3A,B) and was constructed in summer 2014. Here, we removed the uppermost 50 cm soil—without reaching the massive bedrock—on a 25 m2 square plot. The removed soil material was separated by horizon (moder-type O layer: pHCaCl2 3.7/400 g OC g−1; Ah horizon: pHCaCl2 3.8/50 g OC g−1/2.0 g P kg−1; Bw horizon: pHCaCl2 4.4/25 mg OC g−1/1.4 mg P g−1). After leveling the bottom, we set up a timber framework construction identical to that at Achenpass. As in the Achenpass experiment, the square sections were assigned randomly to four different experimental variants A–D, which are described below. Each compartment in the 1 m2 squares was filled with Bw material, which for the experimental variants A–D had been enriched with triple superphosphate (TSP; monocalcium phosphate [Ca(H2PO4)H2O]) and/or inositol hexaphosphate (IHP) in different ratios (Table 1; Figure 2B). Due to its high solubility in water, amended TSP dissolves rapidly, liberating orthophosphate anions, which—similar to dissolved IHP—are instantaneously adsorbed to the abundant Al and Fe oxyhydroxides and clay minerals in the Cambisol Mitterfels (Werner et al., 2017b). Orthophosphate and/or IHP have been selected as P amendments because dominating P forms in the original Cambisol at Mitterfels are orthophosphate and IHP bound to Al/Fe oxyhydroxides and clay minerals (Prietzel et al., 2016).
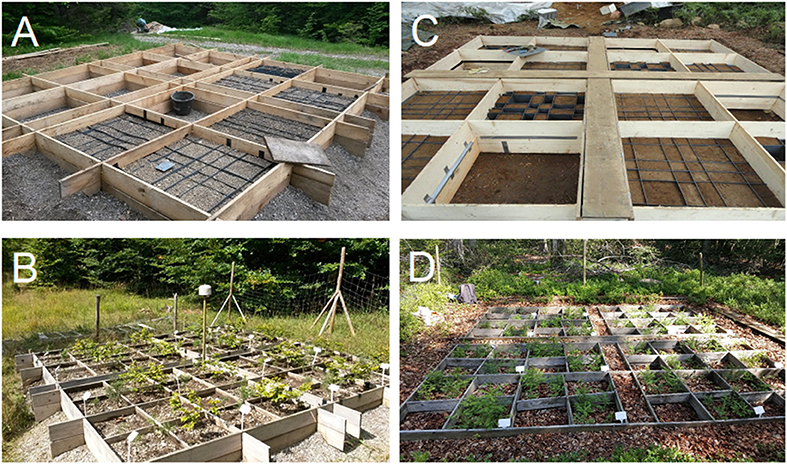
Figure 3. Experiments Achenpass (A,B) and Mitterfels (C,D). (A,C) Construction stage. (B,D) Experiment conduction stage.
The mean P concentration of 3 mg g−1 after experimental P amendment at Mitterfels is at the upper level of forest soil P concentrations in Europe (Prietzel and Stetter, 2010; Lang et al., 2016, 2017; Prietzel et al., 2016), reaching or even exceeding P concentrations in P-rich, basalt-derived soils.
After all compartments had been filled with the respective enriched Bw materials to the top (Figure 1C), each 1 m × 1 m square section representing an experimental variant in each block was divided into 4 squares as described before for Achenpass. Then each square was covered with 5 cm original Bw material without P addition. On top, we homogeneously applied a 5 cm topsoil of original Ah horizon material and a 2 cm cover of original O layer material for the same reasons as mentioned for Achenpass. The construction was completed in August 2014.
In June 2015, 128 Norway spruce seedlings (height 5–10 cm), which had been excavated in the Dragonerfilz forest close to Peiting, Germany, and 64 European beech seedlings (age: 1 year; collected in the nearby forest) were planted in the different square compartments in the same arrangement as described above for the low-P site Achenpass.
As for Achenpass, seedling growth and vitality was recorded at the beginning and at the end of each growing season, starting in June 2015. Whereas, spruce seedlings showed few mortalities, initial losses were tremendous (75% of planted seedlings) for beech seedlings. Losses were replaced twice in June and September 2016. As described for the low-P site Achenpass above, at each growth inventory date, the spruce seedlings of subvariants “s+N” were treated with dilute NH4NO3, resulting in an extra input of 40 kg N ha−1yr−1 in addition to atmospheric deposition of about 20 kg N ha−1yr−1. Seven NH4NO3 applications resulted in an additional N input of 140 kg ha−1 until termination of the Mitterfels experiment in August 2019.
Post-experiment Seedling Processing
Seedling Sampling
After termination of the experiments, we harvested the three largest of the four seedlings in each of the 48 0.25 m2 squares characterized by a given combination of tree species (spruce, beech, spruce with elevated N deposition), fertilizer type (inorganic P, organic P, inorganic + organic P), and spatial pattern of fertilizer application (homogeneous, heterogeneous). This was performed by cutting the seedling shoots at the soil surface and separating foliage from non-foliage parts of each shoot. After 14-d drying at 30°C, we determined foliage and shoot dry masses of each seedling. Then we pooled the respective shoot and root material of the three trees on each 0.25 m2 square, forming a three-seedling-composite sample for each 0.25 m2 square. From each three-seedling-composite sample, we finely ground a subsample for foliar nutrient concentration analysis.
Root Sampling
At Achenpass, we additionally sampled root material from each seedling. This was done by removing the cover topsoil above the compartment hatching and then sampling soil cores at the four positions close to the PVC hatch cross underneath each seedling indicated by large circles in the Figure 1B. The cores were sampled with a small auger (chamber length: 20 cm, diameter: 5 cm). The P-enriched BwC material of the two cores taken from the “+”compartments close to each seedling was pooled; the same was done for the BwC material in the two cores taken from the “–” compartments. After drying the soil samples for 14 d at 30°C, they were sieved. All visible roots were collected with a tweezer and weighed. As for shoot biomass, we pooled the root biomass of the three seedlings in a given 0.25 m2 square to a three-seedling-collective.
Foliar Nutrient Analysis
Concentrations of important elements (N, P, K, Ca, Mg) in fine-ground foliage were analyzed using a CN analyzer (N; HekaTech EA, HekaTech Comp., Wegberg, Germany) or by ICP-OES (other elements; SpectroGenesis) after pressure-digestion with hot concentrated HNO3. Foliar nutrient amounts for each three-seedling-composite sample were calculated by multiplying the foliage mass of each three-seedling-composite sample with the nutrient concentration of its respective foliage sample. The values were divided by 3 to obtain the mean foliar nutrient element amounts of one seedling in each 0.25 m2 square.
Data Processing and Statistics
Based on the 48 foliar nutrient concentration as well as foliage, shoot, and root mass data obtained in each experiment, four replicate samples for each tree species/fertilizer type/spatial fertilizer pattern combination (one for each block 1–4) were available for statistical analysis. All variables were tested for normal distribution, applying a Kolmogorov-Smirnov test. Then we performed ANOVA on shoot mass, foliage mass, foliar nutrient concentrations, and foliar nutrient amounts of each separate tree species variant (spruce, spruce with N deposition, beech). Data were tested separately for each site and tree species, because not only site properties but also ages, provenience, and initial biomasses of the planted seedling varied between sites. Therefore, two one-way ANOVA tests were performed. We tested effects of fertilization regime (homogeneous vs. heterogeneous soil P enrichment) and fertilizer type (single P form vs. mixed P forms) on treatment mean values for statistical significance (p < 0.05). Because the exclusive use of statistical significance for testing experimental treatment effects recently has been criticized (Amrhein et al., 2019), we identified and rated treatment effects by combining p and size effect (eta squared) values. Treatment effects were termed strong and significant, if they met the following criteria: Eta squared > 0.14 and p < 0.05, and moderate for eta squared > 0.06.
Results
Low-P Calcareous Site Achenpass
At the experimental plots of the low-P calcareous site, European beech generally developed better and showed fewer mortalities than Norway spruce. At the end of the experiment, 63 of 64 initial beech seedlings were alive (98% survival); the height of seedlings without browsing damage on average has increased by 50% between September 2016 and August 2018. For Norway spruce, survival rates were 88%, and Norway spruce with elevated N deposition showed a survival rate of 86%. Relative height increases during the experiment on average were +39% for Norway spruce, and +45% for spruce with experimental N amendment.
Norway Spruce
Both shoot and foliage biomass of Norway spruce seedlings grown on soil with heterogeneous P amendment was considerably (shoot biomass: +72%; foliage biomass: +84%; Figure 4) and significantly (Table 2; Supplementary Table 1) larger than for spruces grown on soil with homogeneous P application. In contrast, root biomass was markedly smaller, and shoot/root ratios of spruce seedlings were increased by 50% on plots with heterogeneous compared to homogeneous soil P distribution. However, due to large root mass variance within experimental variants, the effects were statistically weak and insignificant. The form of applied P (IHP vs. IHP + apatite) had no effect on shoot or foliage biomass. However, due to markedly smaller root masses of spruce seedlings grown on soil with combined IHP + apatite amendment compared to exclusive IHP application, shoot/root ratios of spruce seedlings at plots fertilized with IHP + apatite were three times as large as those on plots fertilized with IHP only (Table 2).
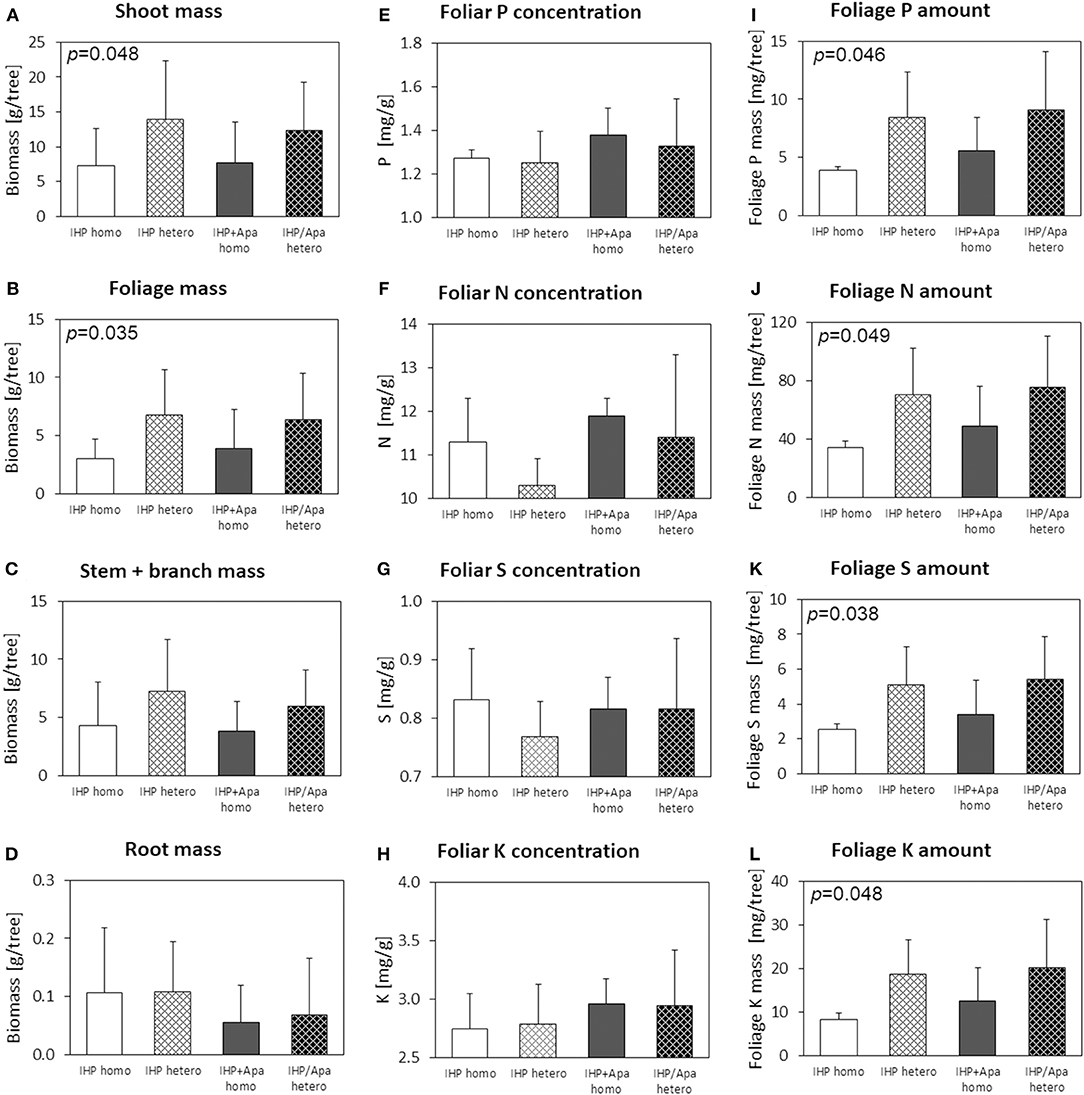
Figure 4. Shoot (A), foliage (B), stem+branch (C), and root (D) biomass, foliar concentrations of N (E), P (F), S (G), and K (H), and nutrient (P, N, S, K) amounts in foliage (I–L) of Picea abies at the low-P calcareous site. Numbers in the upper left panel corner show p < 0.1 for differences between mean values of homogeneous vs. heterogeneous P application calculated by one-way ANOVA.
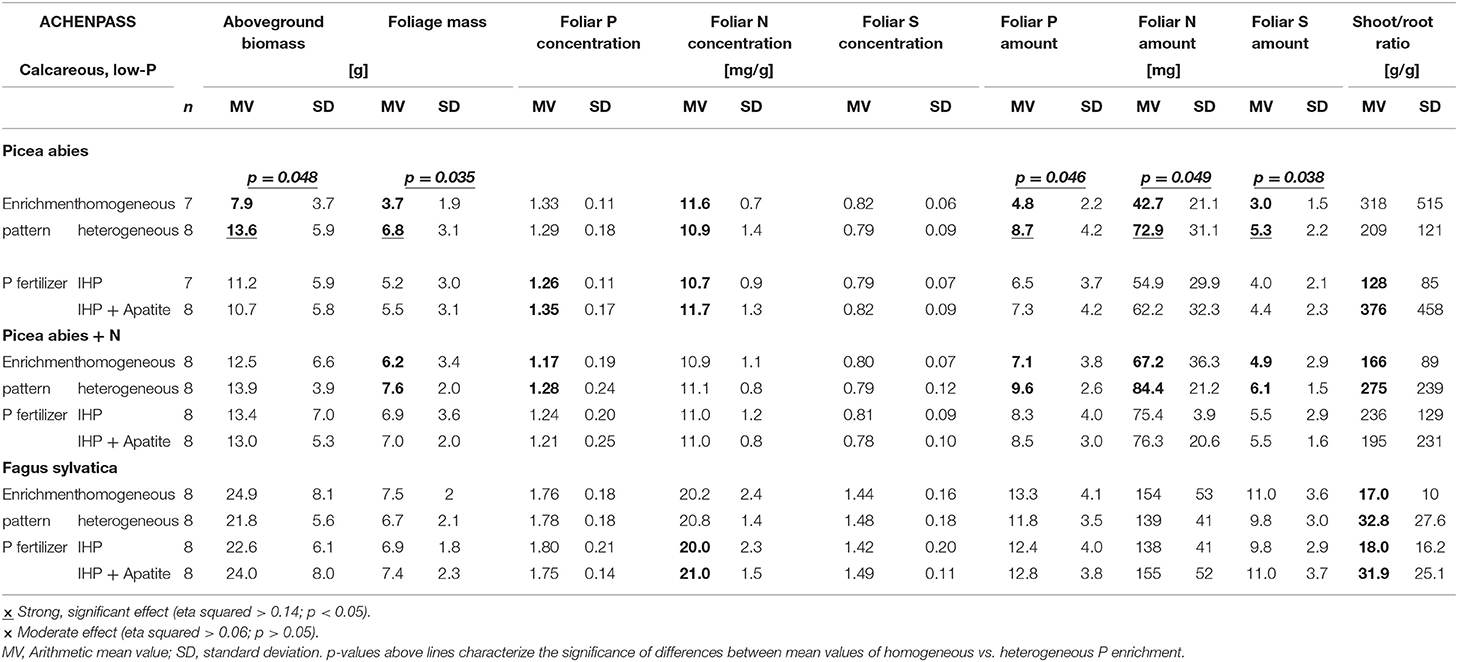
Table 2. Effects of spatial P enrichment pattern and P fertilizer type on growth and nutrition of Picea abies and Fagus sylvatica seedlings in the field experiment at the low-P calcareous site Achenpass (Calcaric Leptosol).
Generally, Norway spruce seedlings at calcareous low-P site were characterized by insufficient N, S, and K nutrition according to the threshold values of Göttlein (2015), whereas tree seedling supply with Ca and Mg nutrition was good. The P nutritional status was latently insufficient (foliar P < 1.3 mg g−1; Göttlein, 2015) for spruces on soils with IHP amendment, and sufficient for those with combined amendment of IHP and apatite. Foliar concentrations of P, N, S, and K were smaller for spruces on soils with heterogeneous compared to homogeneous P amendment, and (except K) for spruces grown on soils with IHP amendment compared to combined IHP + apatite application (Figure 4; Table 2; Supplementary Table 1). P nutrition was sufficient according to Göttlein (2015) for spruces with IHP + apatite treatment, but insufficient for those with exclusive IHP treatment. However, due to larger foliage masses of spruces on soil with heterogeneous compared to homogeneous P amendment, total foliar P amounts (as well as N, S and K amounts) were markedly and significantly larger for spruces with heterogeneous soil P distribution (Table 2; Supplementary Table 1; Figure 4). Additionally, foliar P, N, and K amounts by trend were larger for spruces grown with combined IHP + apatite compared to pure IHP amendment. However, P fertilizer type effects generally were small. For all experimental variants, foliar N/P ratios of 8.4–8.7 indicated balanced N/P nutrition.
Norway Spruce Subject to Experimentally Elevated N Deposition
For the spruce seedlings at the calcareous low-P site subject to artificially elevated N deposition, similar to the spruces grown under ambient N deposition shoot and foliage biomasses of seedlings with heterogeneous soil P amendment were larger than for seedlings grown on soil with homogeneous P application (Figure 5; Table 2; Supplementary Table 2). However, effects generally were smaller than those observed for spruces without experimental N amendment. No effects of P fertilization regime on root biomass were observed for spruce seedlings subject to experimental N deposition, most likely due to the large variation among seedlings representing a fertilization regime.
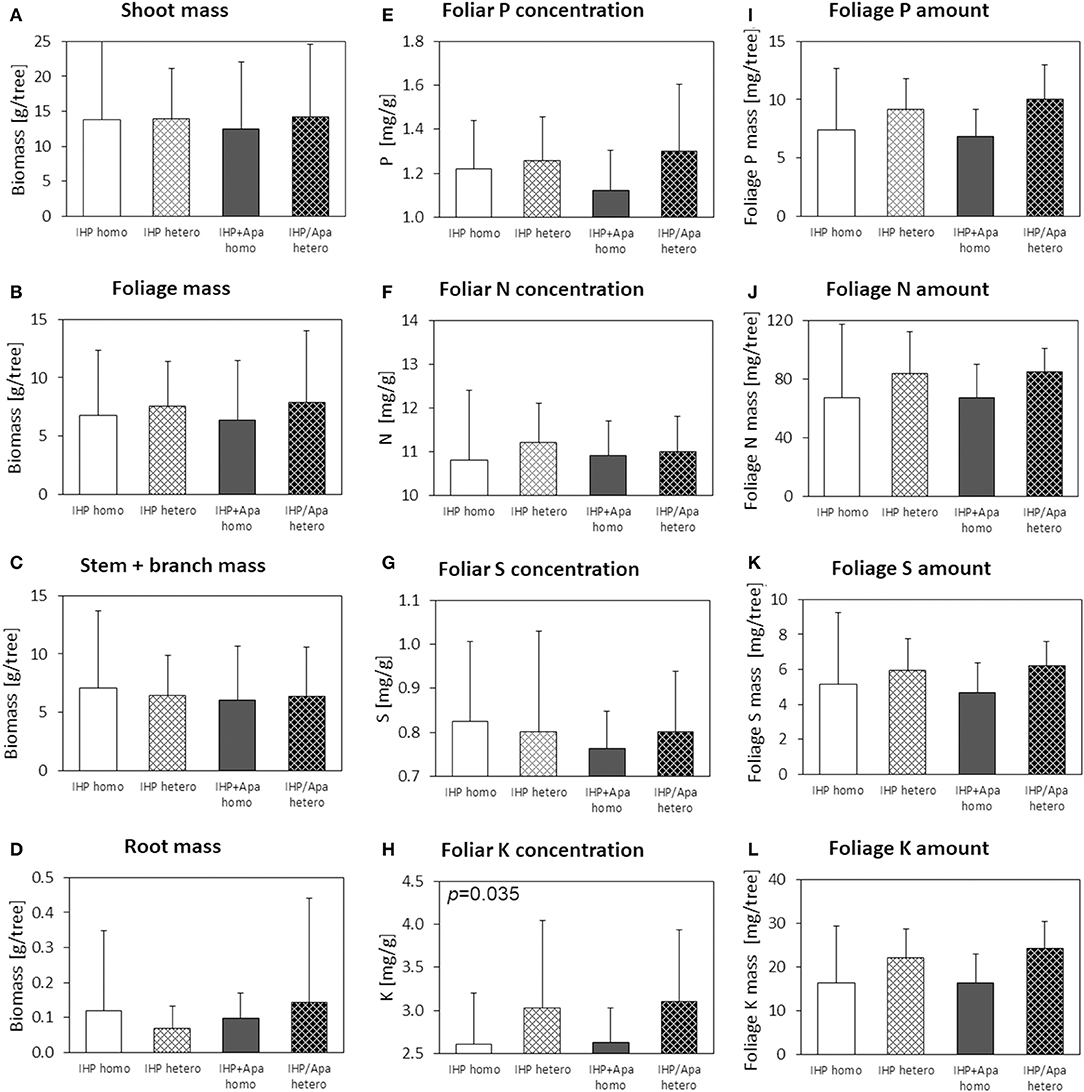
Figure 5. Shoot (A), foliage (B), stem+branch (C), and root (D) biomass, foliar concentrations of N (E), P (F), S (G), and K (H), and nutrient (P, N, S, K) amounts in foliage (I–L) of Picea abies with elevated N deposition at the low-P calcareous site. Numbers in the upper left panel corner show p < 0.1 for differences between mean values of homogeneous vs. heterogeneous P application calculated by one-way ANOVA.
Experimentally elevated N deposition did not increase foliar N concentrations of Norway spruce seedlings at the low-P calcareous site (Table 2; Supplementary Table 2), which as the spruces without experimental N deposition were characterized by insufficient N, S, and K nutrition, but good Ca and Mg nutrition according to Göttlein (2015). The P nutrition status was also insufficient for all spruce seedlings, irrespective of the experimental variant. However, under conditions of elevated N deposition, seedlings on soils with heterogeneous P amendment showed markedly larger foliar P concentrations than seedlings on soils with homogeneous P distribution, whereas N concentrations remained unaffected (Figure 5; Table 2; Supplementary Table 2). Foliar N/P ratios of 8.7–9.3 in the different variants were slightly larger than for the spruce seedlings without experimental N addition, but N/P nutrition was also balanced.
Larger foliage masses of spruce seedlings on soils with heterogeneous compared to homogeneous P amendment together with increased foliar P concentrations resulted in considerably (+35%) and consistently (p: 0.151; eta squared: 0.141; Supplementary Table 2) increased amounts of P, N, S, and K in foliage of spruces with heterogeneous compared to homogeneous soil P amendment (Table 2; Figure 5). The form of applied P (IHP vs. IHP + apatite) had no consistent effect on foliar P, N, and K concentrations. In contrast to the spruce seedlings without experimental N deposition, no consistent effect of the applied P form on foliar P, N, and K amounts as well as on spruce root mass was detected.
European Beech
Beech seedlings by trend showed larger shoot, foliage, and root biomasses for variants where P had been applied homogeneously rather than heterogeneously to the soil (Figure 6). However, these effects were modest and inconsistent (Table 2; Supplementary Table 3). Fertilizer P form also had no consistent effect on shoot or foliage biomass, but root biomass was consistently larger for the pure IHP compared to IHP + apatite variants. Moreover, beech root mass was larger for variants with homogeneous compared to heterogeneous soil P distribution.
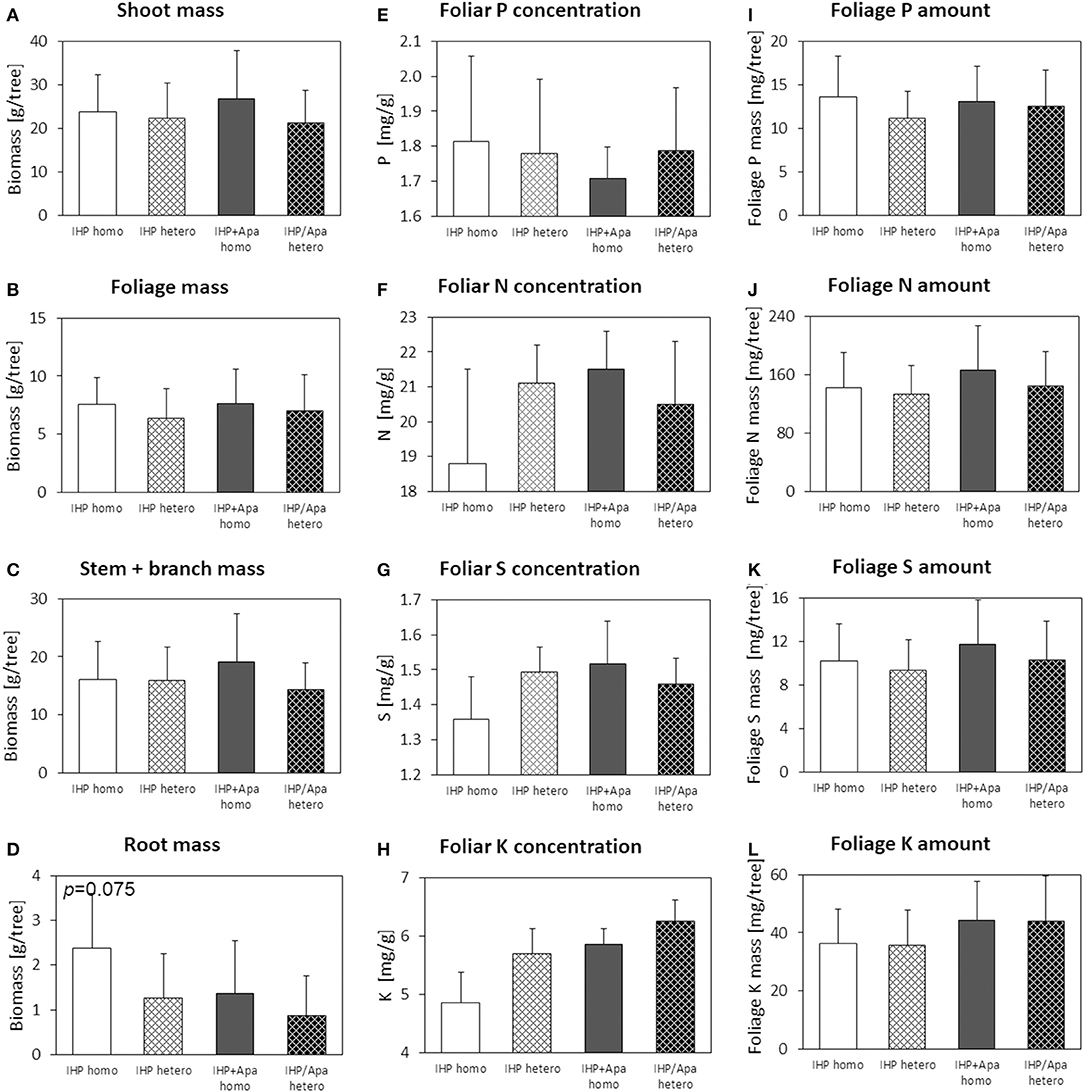
Figure 6. Shoot (A), foliage (B), stem+branch (C), and root (D) biomass, foliar concentrations of N (E), P (F), S (G) and K (H), and nutrient (P, N, S, K) amounts in foliage (I–L) of Fagus sylvatica at the low-P calcareous site. Numbers in the upper left panel corner show p < 0.1 for differences between mean values of homogeneous vs. heterogeneous P application calculated by one-way ANOVA.
The beech seedlings at the low-P calcareous site in contrast to spruce seedlings showed good, well-balanced (foliar N/P ratio: 11.1–12.0) P and N nutrition, but latent S and K deficiency. In accordance with the good P nutritional status of the beech seedlings, neither consistent spatial soil distribution nor P form effects on foliar P and N concentrations or foliar P amounts were observed (Table 2; Supplementary Table 3; Figure 6). Foliar K concentrations in beech seedlings were larger for heterogeneous compared to homogeneous soil P application, and larger when the same P amount was added to the soil as IHP + apatite compared to pure IHP.
Moderate-P Silicate Site Mitterfels
At the moderate-P silicate site Mitterfels, Norway spruce seedlings showed lower mortality rates than beech seedlings during the experiment (13% vs. 22% for beech). This was mainly caused by the fact that the beech seedlings have been strongly browsed by snails and other animals. Additionally, spruce seedlings also developed markedly better than beech seedlings: Relative height increases of seedlings without browsing damage between September 2016 and August 2019 were +206, +200, and +115% for spruce, spruce with elevated N deposition, and beech, respectively. All seedlings at the moderate-P silicate site, irrespective of tree species and absence or presence of experimental N amendment showed acute N and S deficiency, as well as latent Mg deficiency (spruce only), whereas the P nutritional status was sufficient for all experimental variants. Foliar N/P ratios in all variants were consistently <7.5 for beech and <5.2 for spruce, and thus indicated imbalanced N/P nutrition with marked N limitation.
Norway Spruce
Differences of shoot or foliage biomasses of Norway spruce seedlings with heterogeneous compared to homogeneous soil P amendment as well as TSP compared to TSP+IHP amendment were marginal and negligible compared to the biomass variation within a P amendment type (Figure 7; Table 2; Supplementary Table 4). However, P concentrations and amounts in Norway spruce seedling foliage were consistently larger for variants with heterogeneous compared to homogeneous P amendment. Foliar N, S, and Mg concentrations behaved the opposite way; i.e., they were decreased in variants with heterogeneous compared to homogeneous soil P amendment. Foliar N and S amounts were not consistently affected by the applied P form.
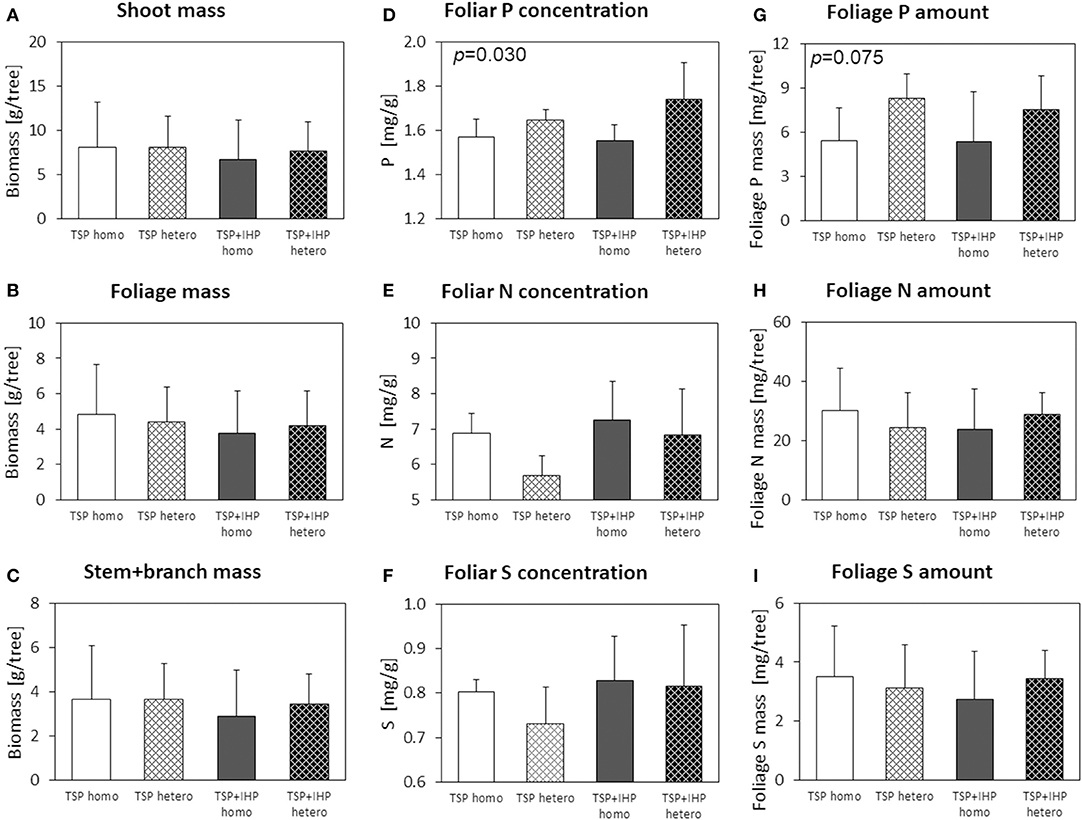
Figure 7. Shoot (A), foliage (B), and stem+branch (C) biomass, foliar concentrations of N (D), P (E), and K (F), and nutrient (P, N, K) amounts in foliage (G–I) of Picea abies at the moderate-P silicate site. Numbers in the upper left panel corner show p < 0.1 for differences between mean values of homogeneous vs. heterogeneous P application calculated by one-way ANOVA.
Norway Spruce Subject to Experimentally Elevated N Deposition
Artificially elevated N deposition on average resulted in modest foliar N concentration increase of Norway spruce seedlings (Table 3; Supplementary Table 5), but the N deficiency was far from being removed. In contrast to spruce seedlings without experimental N deposition, spruce seedlings subject to experimental N deposition showed a marked effect of the spatial soil P enrichment pattern on shoot, foliage, and stem + branch biomasses (Table 3; Supplementary Table 5), which were increased by 37, 41, and 14%, respectively, for heterogeneous compared to homogeneous soil P enrichment (Figure 8). Additionally, foliage biomass and by trend shoot biomass were consistently larger if the P was applied as mixture of TSP and IHP instead of pure TSP.
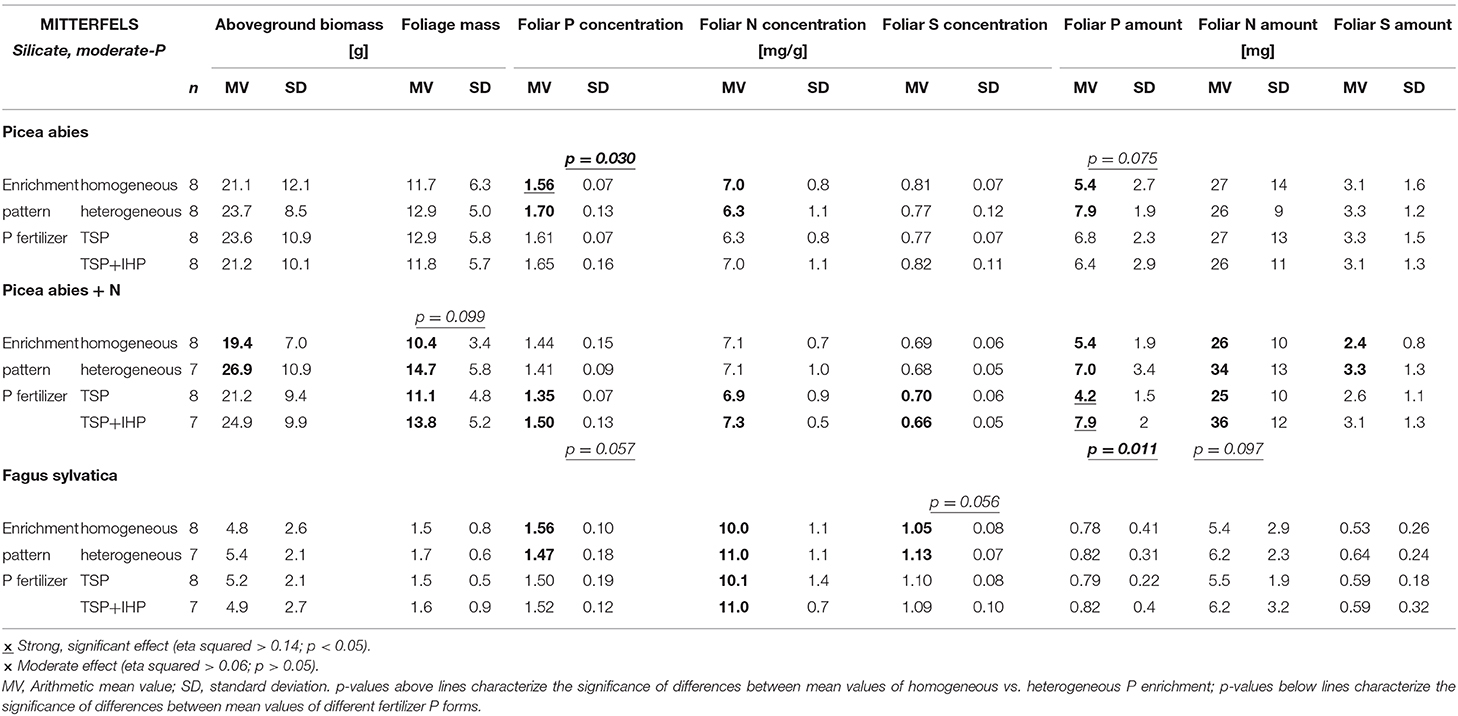
Table 3. Effects of spatial P enrichment pattern and P fertilizer type on growth and nutrition of Picea abies and Fagus sylvatica seedlings in the field experiment at the moderate-P silicate site Mitterfels (Dystric Cambisol).
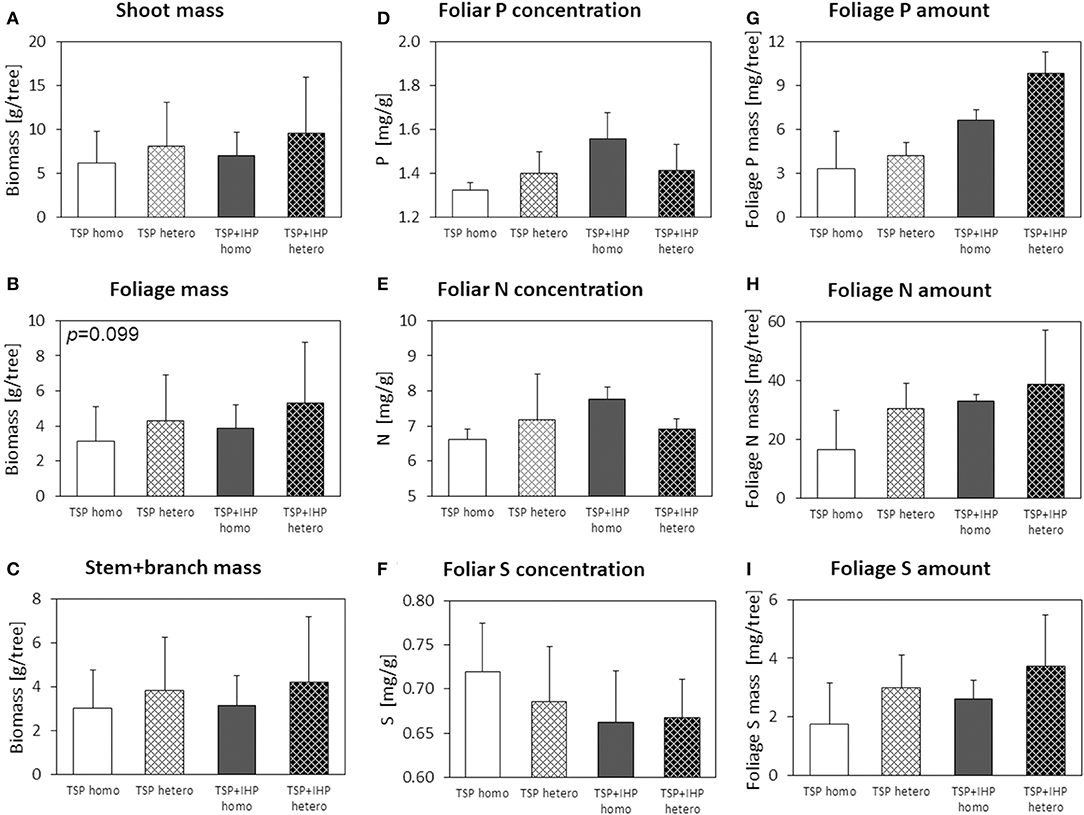
Figure 8. Shoot (A), foliage (B), and stem+branch (C) biomass, foliar concentrations of N (D), P (E), and K (F), and nutrient (P, N, K) amounts in foliage (G–I) of Picea abies with elevated N deposition at the moderate-P silicate site. Numbers in the upper left panel corner show p < 0.1 for differences between mean values of homogeneous vs. heterogeneous P application calculated by one-way ANOVA.
Foliar P concentrations of Norway spruce seedlings subject to experimentally elevated N deposition were not affected consistently by the spatial soil P enrichment pattern. However, foliar P concentrations were consistently larger and foliar S and Mg concentrations were consistently smaller when P was applied as mixture of TSP and IHP instead of pure IHP. Total P, N, S, and Mg amounts in foliage biomass were largest when the P had been applied to the soil in spatial heterogeneous pattern and in two different forms, and smallest when the P had been applied homogeneously as one single P form (TSP).
European Beech
Beech seedlings at the moderate-P silicate site by trend showed larger shoot, foliage, and stem + branch biomasses for variants with heterogeneous compared to homogeneous soil P amendment (Figure 9). However, the effects were modest and inconsistent (Table 3; Supplementary Table 6). Fertilizer P form also had no consistent effect on shoot or foliage biomass. Foliar P concentrations of beech seedlings were smaller for heterogeneous compared to homogenous soil P amendment, whereas the opposite was the case for foliar N, S, and Mg concentrations. Spatial or species P heterogeneity patterns did neither consistently affect the amounts of P nor those of N, S, or Mg in beech foliage at the silicate moderate-P site.
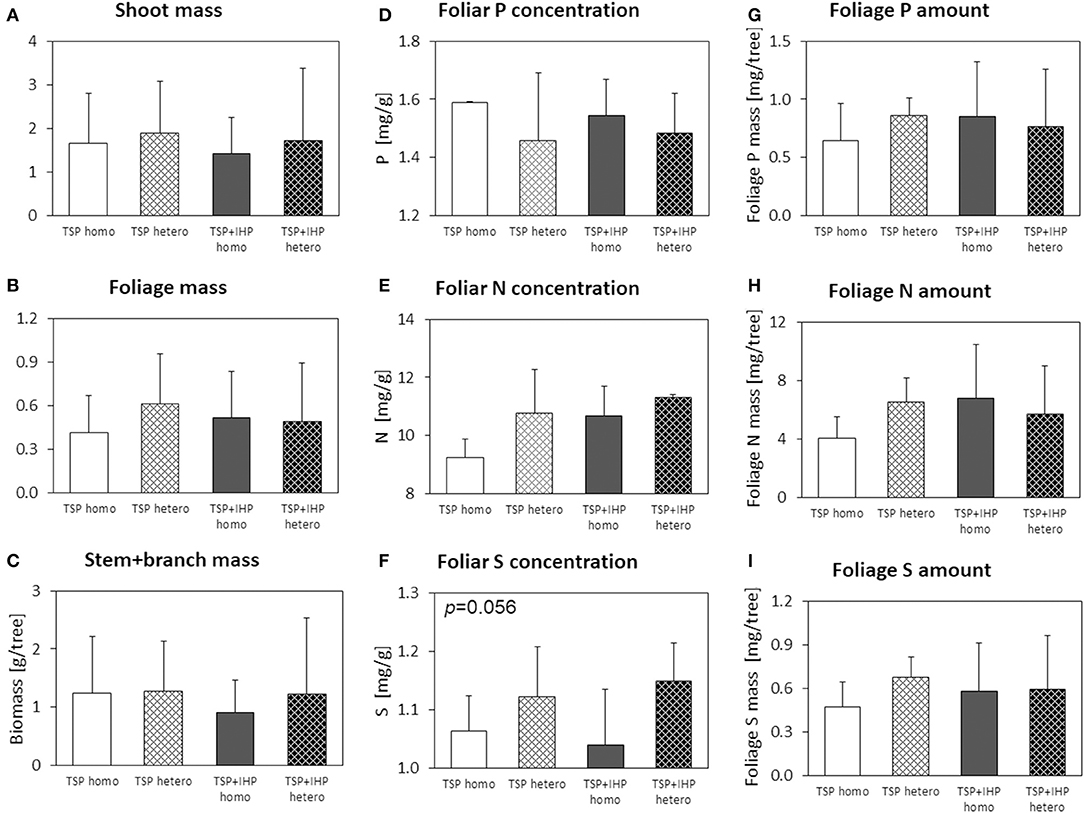
Figure 9. Shoot (A), foliage (B), and stem+branch (C) biomass, foliar concentrations of N (D), P (E), and K (F), and nutrient (P, N, K) amounts in foliage (G–I) of Fagus sylvatica at the moderate-P silicate site. Numbers in the upper left panel corner show p < 0.1 for differences between mean values of homogeneous vs. heterogeneous P application calculated by one-way ANOVA.
Discussion
Spatial Soil P Concentration Heterogeneity Improves P Nutrition and Growth of Norway Spruce in Shallow Initial Calcareous Soils
Our study showed no consistent effects of soil P concentration heterogeneity or P species diversity on beech seedling growth. In contrast, study results clearly indicated that accumulation of a given P amount in distinct spatial soil domains (heterogeneous P soil distribution; presence of P hotspots) improves P nutrition and accelerates shoot growth of Norway spruce seedlings in initial calcareous soils with poor P supply. At the same time, smaller spruce root biomass in variants with heterogeneous compared to homogeneous soil P concentrations suggest that less photosynthesis carbon was invested for root development. Facelli and Facelli (2002) reported positive soil P concentration heterogeneity effects on the growth of the legume species Trifolium subterraneum in a mesocosm experiment, but the effect so far has not been shown for forest trees. Soil P concentration patchiness exists at different scales, ranging from the microscale (Hinsinger et al., 2005; Werner et al., 2017a) over the profile scale (Werner et al., 2017b) up to the stand or landscape scale (Litaor et al., 2005; Liptzin et al., 2013). It can be assumed that similar to grasses and herbs (e.g., Facelli and Facelli, 2002), also trees of different age as well as different tree species may respond differently regarding their P nutrition status and growth to soil P concentration patchiness at different scales, depending on their respective rooted soil volume. Small-scale topsoil patchiness likely is most important for seedlings with small root systems, whereas with increasing tree age and rooted soil volume, larger-scaled soil P heterogeneity patterns (1–10 m) might become more relevant. Possible processes involved in the positive P hotspot effect on tree P nutrition and growth may include (i) easier mobilization of hotspot P by desorption, complexation, dissolution, (ii) enhanced enzymatic P mobilization from a larger substrate pool of organic P (Hinsinger, 2001; Hinsinger et al., 2005), (iii) increased root P uptake kinetics (Caldwell et al., 1992), and (iv) improved competitiveness of plants compared to soil microorganisms for P (Ehlers et al., 2010; Richardson and Simpson, 2011). In the artificial shallow calcareous soil in our study as well as in real-world initial calcareous soils (Celi et al., 2000; Prietzel et al., 2016), P is mostly present as Ca-bound IHP in addition to apatite-P, because IHP rapidly forms sparingly-soluble Ca-IHP precipitates at pH values >6 (Crea et al., 2006). P mobilization from Ca-IHP and apatite is achieved through cleavage of the Ca-PO4 bond; in the case of IHP additionally by enzymatic cleavage of the inositol-PO4 bond. In calcareous soils, Ca-PO4 bond cleavage is predominantly performed through combined attack of protons and chelators from/of (poly)carboxylic acids (Hinsinger, 2001; Hinsinger et al., 2005) which are produced by plant roots, mycorrhizal fungi, and free soil microorganisms. In the case of apatite dissolution, mobilized orthophosphate can directly be taken up by plant roots. In contrast, according to current knowledge plant uptake of P derived from IHP requires prior IHP cycling through bacteria and nematodes, associated with its conversion into orthophosphate, because plants have a poor capacity to use IHP as P source (Irshad et al., 2012). In P-rich soil hotspots all processes mentioned above liberate more P from a given soil volume at a given organism expense of P-mobilizing organic acids. Moreover, soil microorganisms are particularly competitive in acquisition of nutrients as N or P at low soil concentrations (Ehlers et al., 2010; Richardson and Simpson, 2011), resulting in relatively increased microbial P immobilization (“microbial nutrient trapping”; Kuzyakov and Xu, 2013) and decreased P availability to trees. Tree root accumulation in P-rich soil compartments, as proven for roots of herbs, grasses, and crops in earlier studies (cf. references in Felderer et al., 2013) could not be proven in our study due to the large variation of soil volume-related root biomass.
In contrast to spruce, heterogeneous soil P distribution in our study showed only small and inconsistent effects on beech seedling growth and P nutrition. This is likely due to the fact that in contrast to spruce, the P nutritional status of our beech seedlings without exception was good at the moderate-P silicate site Mitterfels and excellent at the low-P site Achenpass according to the threshold values of Göttlein (2015), making a selective exploitation of P hotspots unnecessary (Noy-Meir, 1981; Facelli and Facelli, 2002). The excellent P supply of beech strongly contrasts with the poor P supply (also according to Göttlein, 2015) of spruce seedlings growing under identical soil and site conditions. Large-scale tree inventories revealed P deficiency not only for Norway spruce (Prietzel et al., 2015), but also for European beech growing on shallow calcareous soils in the Alps (Ewald, 2000). The excellent P nutrition status of our beech seedlings in contrast to the spruce seedlings at the low-P site can be explained by the fact that the spruce seedlings had been taken from the nearby forest and showed poor P nutrition at the beginning of the experiment, whereas the beech seedlings were purchased as one-year-old plants from a nursery. In contrast to the spruce seedlings, the beech seedlings at the low-P site Achenpass thus had a good initial P nutritional status and had probably accumulated a substantial P amount during their nursery time, which was sufficient to maintain an excellent P seedling nutrition status during the entire experiment.
No consistent soil P form diversity effects on seedling growth were present, and site- or tree species-specific effects of soil P form diversity also were absent. Yet, combined soil amendment with two different P forms in five of six experimental variants by trend resulted in larger foliage P amounts compared to variants where the same total P amount had been applied as one single P form. This indicates that in addition to spatial P heterogeneity also P form diversity in soils may be beneficial for the P nutrition of forest trees.
Phosphorus Concentration Heterogeneity and P Form Diversity in Acidic Soils Subject to Elevated N Deposition Improves P Nutrition and Growth of Norway Spruce
The Norway spruce variant without additional N input in our experiment at the moderate-P silicate site Mitterfels represents temperate conifer forest ecosystems with acidic soils formed from silicate parent material with good P supply, but N limitation. Such ecosystems are widespread in Scandinavia (Tamm, 1991), and also had been widespread in Central Europe before the onset of large-scale forest ecosystem N eutrophication by long-term elevated atmospheric N deposition (Mohren et al., 1986; Prietzel et al., 2006; Prietzel and Stetter, 2010). At such sites, according to the results of our study, soil P heterogeneity is not relevant for forest tree growth and P nutrition. According to several studies, removal of the initial N limitation in these forest ecosystems by elevated N deposition often has resulted in deterioration of the P nutritional status of conifer and broadleaf forests (Mohren et al., 1986; Ilg et al., 2009; Prietzel and Stetter, 2010; Jonard et al., 2012, 2015). Our experimental variant “Norway spruce subject to elevated atmospheric N deposition” at the moderate-P silicate site Mitterfels represents these forest ecosystems. In that variant we showed that spatial soil P concentration heterogeneity and P form diversity improved P nutrition and growth of Norway spruce seedlings even at sites with large mean soil P concentrations (in our study 3 mg P g−1). It can be assumed that this is also the case for older, larger spruce trees, and also for other tree species in Central European forests subject to elevated N deposition—yet, as explained in section Spatial Soil P Concentration Heterogeneity Improves P Nutrition and growth of Norway Spruce in Shallow Initial Calcareous Soils, at larger spatial scales of soil P heterogeneity, which are related to the age- and species-dependent rooted soil volume. Obviously, soil P concentration heterogeneity and P form diversity plays an important, at the moment underestimated role for tree P acquisition and P cycling in forest ecosystems, and particularly in P-limited forest ecosystems with high structural and/or tree species diversity. Future experiments with defined soil P heterogeneity, including those investigating larger scales of P heterogeneity and/or different P enrichment patch sizes in three dimensions (Liu et al., 2017) will increase our understanding of soil P heterogeneity effects on forest ecosystems.
Conclusion
Soil P concentration heterogeneity obviously is beneficial for growth and P nutrition of Norway spruce on P-poor shallow, calcareous soils. Moreover, it obviously is also beneficial for Norway spruce growth at sites with silicate soils characterized by moderate soil P supply and elevated atmospheric N deposition. In contrast to spatial soil P heterogeneity, soil P form diversity has minor effects on spruce seedling growth or P nutrition.
Data Availability Statement
The raw data supporting the conclusions of this article will be made available by the author, without undue reservation, on request to any qualified researcher.
Author Contributions
JP did all the work needed for production of this manuscript, assisted by the people listed in the Acknowledgments section.
Funding
The study was funded by the German Research Foundation (grant Pr 534/6-2) as part of the Priority program SPP 1685 Ecosystem Nutrition: Forest Strategies for limited Phosphorus Resources.
Conflict of Interest
The author declares that the research was conducted in the absence of any commercial or financial relationships that could be construed as a potential conflict of interest.
Acknowledgments
I gratefully acknowledge the invaluable help of Katharina and Maximilian Prietzel during construction of the experiments. Jakob Prietzel, Agnes Eckstein, and Jasmin Seven helped in soil and tree sampling in the Achenpass experiment. Sigrid Hiesch, Monika Weber, and Christine Pfab assisted during soil and plant sample preparation and analysis.
Supplementary Material
The Supplementary Material for this article can be found online at: https://www.frontiersin.org/articles/10.3389/ffgc.2020.00059/full#supplementary-material
References
Achat, D. L., Pousse, N., Nicolas, M., Brédoire, F., and Augusto, L. (2016). Soil properties controlling inorganic phosphorus availability. General results from a national forest network and a global compilation of the literature. Biogeochemistry 127, 255–272. doi: 10.1007/s10533-015-0178-0
Amrhein, V., Greenland, S., and McShane, B. (2019). Retire statistical significance. Nature 567, 305–307. doi: 10.1038/d41586-019-00857-9
Augusto, L., Achat, D. L., Jonard, M., Vidal, D., and Ringeval, B. (2017). Soil parent material - a major driver of plant nutrient limitations in terrestrial ecosystems. Glob. Change Biol. 23, 3808–3824. doi: 10.1111/gcb.13691
Biermayer, G., and Rehfuess, K. E. (1985). Holozäne terrae fuscae aus carbonatgesteinen in den nördlichen kalkalpen. J. Plant Nutr. Soil Sci. 148, 405–416. doi: 10.1002/jpln.19851480405
Caldwell, M. M., Dudley, L. M., and Lilieholm, B. (1992). Soil solution phosphate, root uptake kinetics and nutrient acquisition: implications for a patchy soil environment. Oecologia 89, 305–309. doi: 10.1007/BF00317406
Casper, B. B., and Cahill, J. F. Jr. (1996). Limited effects of soil nutrient heterogeneity on populations of Abutilon theophrasti (Malvaceae). Am. J. Bot. 83, 333–341. doi: 10.1002/j.1537-2197.1996.tb12714.x
Casper, B. B., and Cahill, J. F. Jr. (1998). Population-level responses to nutrient heterogeneity and density by Abutilon theophrasti (Malvaceae): an experimental neighborhood approach. Am. J. Bot. 85, 1680–1687. doi: 10.2307/2446501
Celi, L., Lamacchia, S., and Barberis, S. (2000). Interaction of inositol phosphate with calcite. Nutr. Cycling Agroecosyst. 57, 271–277. doi: 10.1023/A:1009805501082
Condron, L. M., Turner, B. L., and Cade-Menun, B. J. (2005). “Chemistry and dynamics of soil organic phosphorus,” in Phosphorus: Agriculture and the Environment eds J. Thomas Sims and A. N. Sharpley (Madison, WI: American Society of Agronomy), 87–121.
Crea, P., de Robertis, A., de Stefano, C., and Sammartano, S. (2006). Speciation of phytate ion in aqueous solution. Sequestration of magnesium and calcium by phytate at different temperatures and ionic strengths, in NaClaq. Biophys. Chem. 124, 18–26. doi: 10.1016/j.bpc.2006.05.027
Ehlers, K., Bakken, L. R., Frostegard, A., Frossard, E., and Bünemann, E. K. (2010). Phosphorus limitation in a ferralsol: impact on microbial activity and cell internal P pools. Soil Biol. Biochem. 42, 558–566. doi: 10.1016/j.soilbio.2009.11.025
Ewald, J. (2000). Ist phosphormangel für die geringe vitalität von Buchen (Fagus sylvatica L.) in den Bayerischen Alpen verantwortlich? Forstwiss. Cbl. 119, 276–296. doi: 10.1007/BF02769143
Facelli, E., and Facelli, J. M. (2002). Soil phosphorus heterogeneity and mycorrhizal symbiosis regulate plant intra-specific competition and size distribution. Oecologia 133, 54–61. doi: 10.1007/s00442-002-1022-5
Felderer, B., Boldt-Burisch, K. M., Schneider, B. U., Hüttl, R. F. J., and Schulin, R. (2013). Root growth of Lotus corniculatus interacts with P distribution in young sandy soil. Biogeosci 10, 1737–1749. doi: 10.5194/bg-10-1737-2013
Göttlein, A. (2015). Grenzwertbereiche für die ernährungskundliche Einwertung der Hauptbaumarten Fichte, Kiefer, Eiche, Buche. Allg. Forst Jagdztg. 186, 110–116.
Hinsinger, P. (2001). Bioavailability of soil inorganic P in the rhizosphere as affected by root-induced chemical changes: a review. Plant Soil 237, 173–195. doi: 10.1023/A:1013351617532
Hinsinger, P., Gobran, G., Gregory, P., and Wenzel, W. (2005). Rhizosphere geometry and heterogeneity arising from root mediated physical and chemical processes. New Phytol. 168, 293–303. doi: 10.1111/j.1469-8137.2005.01512.x
Ilg, K., Wellbrock, N., and Lux, W. (2009). Phosphorus supply and cycling at long-term monitoring sites in Germany. Eur. J. For. Res. 128, 483–492. doi: 10.1007/s10342-009-0297-z
Irshad, U., Brauman, A., Villenave, C., and Plassard, C. (2012). Phosphorus acquisition from phytate depends on efficient bacterial grazing, irrespective of the mycorrhizal status of Pinus pinaster. Plant Soil 358, 148–161. doi: 10.1007/s11104-012-1161-3
Jonard, M., Fürst, A., Verstraeten, A., Thimonier, A., Timmermann, V., Potocic, N., et al. (2015). Tree mineral nutrition is deteriorating in Europe. Glob. Change Biol. 21, 418–430. doi: 10.1111/gcb.12657
Jonard, M., Legout, A., Nicolas, M., Dambrine, E., Nys, C., Ulrich, E., et al. (2012). Deterioration of Norway spruce vitality despite a sharp decline in acid deposition: a long-term integrated perspective. Glob. Change Biol. 18, 711–725. doi: 10.1111/j.1365-2486.2011.02550.x
Kuzyakov, Y., and Xu, X. (2013). Competition between roots and microorganisms for nitrogen: mechanisms and ecological relevance. New Phytol. 198, 656–669. doi: 10.1111/nph.12235
Lang, F., Bauhus, J., Frossard, E., George, E., Kaiser, K., Kaupenjohann, M., et al. (2016). Phosphorus in forest ecosystems: new insights from an ecosystem nutrition perspective. J. Plant Nutr. Soil Sci. 179, 129–135. doi: 10.1002/jpln.201500541
Lang, F., Krüger, J., Amelung, W., Willbold, S., Frossard, E., Bünemann, E. K., et al. (2017). Soil phosphorus supply controls P nutrition strategies of beech forest ecosystems in Central Europe. Biogeochem 136, 5–29. doi: 10.1007/s10533-017-0375-0
Liptzin, D., Sanford, R. L. Jr., and Seastedt, T. R. (2013). Spatial patterns of total and available N and P at alpine treeline. Plant Soil 365, 127–140. doi: 10.1007/s11104-012-1379-0
Litaor, M. I., Seastedt, T. R., Walker, M. D., Carbone, M., and Townsend, A. (2005). The biogeochemistry of phosphorus across an alpine topographic/snow gradient. Geoderma 124, 49–61. doi: 10.1016/j.geoderma.2004.04.001
Liu, Y., de Boeck, H., Wellens, M., and Nijs, I. (2017). A simple method to vary soil heterogeneity in three dimensions in experimental mesocosms. Ecol. Res. 32, 287–295. doi: 10.1007/s11284-017-1435-6
Mohren, G. M. J., van den Burg, J., and Burger, F. W. (1986). Phosphorus deficiency induced by nitrogen input in Douglas fir in the Netherlands. Plant Soil 95, 191–200. doi: 10.1007/BF02375071
Noy-Meir, I. (1981). “Spatial effects in modelling of arid ecosystems,” in Arid-land Ecosystems: Structure, Functioning and Management, eds D. W. Goodall, R. A. Perry, and K. M. W. Howes (Cambridge: Cambridge University Press, 411–432.
Prietzel, J., and Ammer, C. (2008). Montane Bergmischwälder der Bayerischen Kalkalpen: Reduktion der Schalenwilddichte steigert nicht nur den Verjüngungserfolg, sondern auch die Bodenfruchtbarkeit. Allg. Forst Jagdztg. 179, 104–112.
Prietzel, J., Christophel, D., Traub, C., Kolb, E., and Schubert, A. (2015). Regional and site-related patterns of soil nitrogen, phosphorus, and potassium stocks and Norway spruce nutrition in mountain forests of the bavarian alps. Plant Soil 386, 151–169. doi: 10.1007/s11104-014-2248-9
Prietzel, J., Klysubun, W., and Werner, F. (2016). Speciation of phosphorus in temperate zone forest soils as assessed by combined wet-chemical fractionation and XANES spectroscopy. J. Plant Nutr. Soil Sci. 179, 168–185. doi: 10.1002/jpln.201500472
Prietzel, J., and Stetter, U. (2010). Long-term effects of atmospheric nitrogen deposition and fertilization on phosphorus nutrition in two German Scots pine (Pinus sylvestris) ecosystems. For. Ecol. Manage. 259, 1141–1150. doi: 10.1016/j.foreco.2009.12.030
Prietzel, J., Stetter, U., Klemmt, H. J., and Rehfuess, K. E. (2006). Recent carbon and nitrogen accumulation and acidification in soils of two Scots pine ecosystems in Southern Germany. Plant Soil 289, 153–170. doi: 10.1007/s11104-006-9120-5
Richardson, A. E., and Simpson, R. J. (2011). Soil microorganisms mediating phosphorus availability. Plant Physiol. 156, 989–996. doi: 10.1104/pp.111.175448
Tamm, C. O. (1991). Nitrogen in Terrestrial Ecosystems, Questions of Productivity, Vegetational Changes, and Ecosystem Stability. Ecological Studies. Berlin: Springer-Verlag.
Turner, B. L., Condron, L. M., Richardson, S. J., Peltzer, D. A., and Allison, V. J. (2007). Soil organic phosphorus transformations during pedogenesis. Ecosystems 10, 1166–1181. doi: 10.1007/s10021-007-9086-z
Turner, B. L., Paphazy, M. J., Haygarth, P. M., and Mckelvie, I. D. (2002). Inositol phosphates in the environment. Philos. Trans. R. Soc. B. 357, 449–469. doi: 10.1098/rstb.2001.0837
Walker, T. W., and Syers, J. K. (1976). The fate of phosphorus during pedogenesis. Geoderma 15, 1–19. doi: 10.1016/0016-7061(76)90066-5
Wardle, D. A., Walker, L. R., and Bardgett, R. D. (2004). Ecosystem properties and forest decline in contrasting long-term chronosequences. Science 305, 509–513. doi: 10.1126/science.1098778
Werner, F., de la Haye, T. R., Spielvogel, S, and Prietzel, J. (2017b). Small-scale spatial distribution of phosphorus fractions in soils from silicate parent material with different degree of podzolization. Geoderma 302, 52–65. doi: 10.1016/j.geoderma.2017.04.026
Keywords: soil P heterogeneity, soil P forms, field experiment, elevated N deposition, orthophosphate, apatite, inositol hexaphosphate
Citation: Prietzel J (2020) Soil Phosphorus Heterogeneity Improves Growth and P Nutrition of Norway Spruce Seedlings. Front. For. Glob. Change 3:59. doi: 10.3389/ffgc.2020.00059
Received: 10 February 2020; Accepted: 23 April 2020;
Published: 21 May 2020.
Edited by:
Sebastian Loeppmann, Christian-Albrechts-Universität zu Kiel, GermanyReviewed by:
Rodica Pena, University of Göttingen, GermanyInger Kappel Schmidt, University of Copenhagen, Denmark
Copyright © 2020 Prietzel. This is an open-access article distributed under the terms of the Creative Commons Attribution License (CC BY). The use, distribution or reproduction in other forums is permitted, provided the original author(s) and the copyright owner(s) are credited and that the original publication in this journal is cited, in accordance with accepted academic practice. No use, distribution or reproduction is permitted which does not comply with these terms.
*Correspondence: Jörg Prietzel, prietzel@wzw.tum.de