- 1U.S. Geological Survey, Western Ecological Research Center, Arcata, CA, United States
- 2Department of Forestry and Wildland Resources, Humboldt State University, Arcata, CA, United States
- 3Department of Geography, Environment, and Spatial Analysis, Humboldt State University, Arcata, CA, United States
Drought, coupled with rising temperatures, is an emerging threat to many forest types across the globe. At least to a degree, we expect management actions that reduce competition (e.g., thinning, prescribed fire, or both) to improve growth of residual trees during drought. The influences of management actions and drought on individual tree growth may be measured with high precision using tree-rings. Here, we summarize tree-ring-based assessments of the effectiveness of thinning and prescribed fire as drought adaptation tools, with special consideration for how these findings might apply to dry coniferous forests in the southwestern United States. The existing literature suggests that thinning treatments generally improve individual tree growth responses to drought, though the literature specific to southwestern coniferous forests is sparse. Assessments from studies beyond the southwestern United States indicate treatment effectiveness varies by thinning intensity, timing of the drought relative to treatments, and individualistic species responses. Several large-scale studies appear to conflict on specifics of how site aridity influences sensitivity to drought following thinning. Prescribed fire effects in the absence of thinning has received much less attention in terms of subsequent drought response. There are limitations for using tree-ring data to estimate drought responses (e.g., difficulties scaling up observations to stand- and landscape-levels). However, tree-rings describe an important dimension of drought effects for individual trees, and when coupled with additional information, such as stable isotopes, aid our understanding of key physiological mechanisms that underlie forest drought response.
Introduction
Periodic droughts are occurring against a backdrop of increasing temperatures, so that drought effects are exacerbated by greater evaporative demand. These “hotter droughts” have been linked to vegetation stress and complete forest diebacks (Allen et al., 2015). In response, forest managers are developing strategies to adapt to these changing conditions (Millar and Stephenson, 2015). The need to develop forest management tools is particularly urgent in the dry coniferous forests of the southwestern United States (here defined as dry forest types in Arizona, California, New Mexico, Nevada, and Utah). In this region, a history of fire exclusion has resulted in many forest types experiencing changes in forest structure, increased accumulation of forest fuels, and increased fire activity with changing climatic conditions (Hurteau et al., 2014). Simultaneously, this region has experienced drought-induced forest diebacks (Allen et al., 2015).
Managers have two primary tools for large-scale forest restoration: mechanical thinning and prescribed fire (with both treatments sometimes applied sequentially to the same stand). Mechanical thinning removes competing vegetation to promote growth of residual trees, and has a long history of use that includes conservation applications (e.g., Kolb et al., 2007). Prescribed fire, where fire is intentionally ignited or let burn under conditions that encourage moderate fire effects, is used to remove surface fuels and continuity of live fuels (shrubs, small trees and low branches) (Ryan et al., 2013). It is less clear if prescribed fire leads to negative or positive growth responses in residual trees. Fire often injures surviving trees, and prescribed fire is typically designed to only remove surface and ladder fuels (i.e., small trees), so that large reductions in stand basal area are uncommon (Schwilk et al., 2009). Managers have much greater control over treatment outcomes with thinning operations relative to prescribed fire, but both types of treatments can be designed to be more or less “aggressive,” with varying numbers of surviving trees.
Tree-ring measurements have been used to provide evidence for the effectiveness of thinning and prescribed fire treatments in terms of drought adaptation. These observations have intuitive appeal for these applications, as tree-rings can be used to measure response to environmental stress and construct indices of drought response. Here, we briefly review the use of tree-ring evidence to support or refute the efficacy of thinning and prescribed fire as a drought adaptation strategy, with a focus on how these results may apply to drought-sensitive, coniferous forest types in the southwestern United States.
Tree-Ring Indices of Drought Response
Drought stress and competition lower photosynthetic capacity, and as a consequence trees may reduce carbon allocation to non-critical functions such as stem growth (Waring, 1987). Therefore, tree-ring records of growth may be an early indicator of stress on individual trees. Drought response as measured by tree-rings can be decomposed into the following elements (Lloret et al., 2011): drought resistance, quantified as growth insensitivity to stress (growth during drought/pre-drought growth); growth recovery, considered as the growth rate following drought relative to growth during drought (post-drought growth/growth during drought); and drought resilience, measured as the magnitude of return to pre-drought growth (post-drought growth/pre-drought growth) (Figure 1). These indices have been used to measure drought response and effects of management in a wide variety of forest types (Sohn et al., 2016). Problematically, indices of drought resistance, recovery, and resilience appear to be correlated (Gazol et al., 2017a, b, 2018). These indices are also sensitive to the measurements of growth that are used (e.g., radial versus basal area increment), and suffer from potential biases arising from length and growth conditions of the pre-drought reference period, lack of standardization in defining drought severity and duration, variation in post-drought conditions, and differential species responses (Schwarz et al., 2019). These shortcomings complicate comparisons of drought and thinning effects across studies.
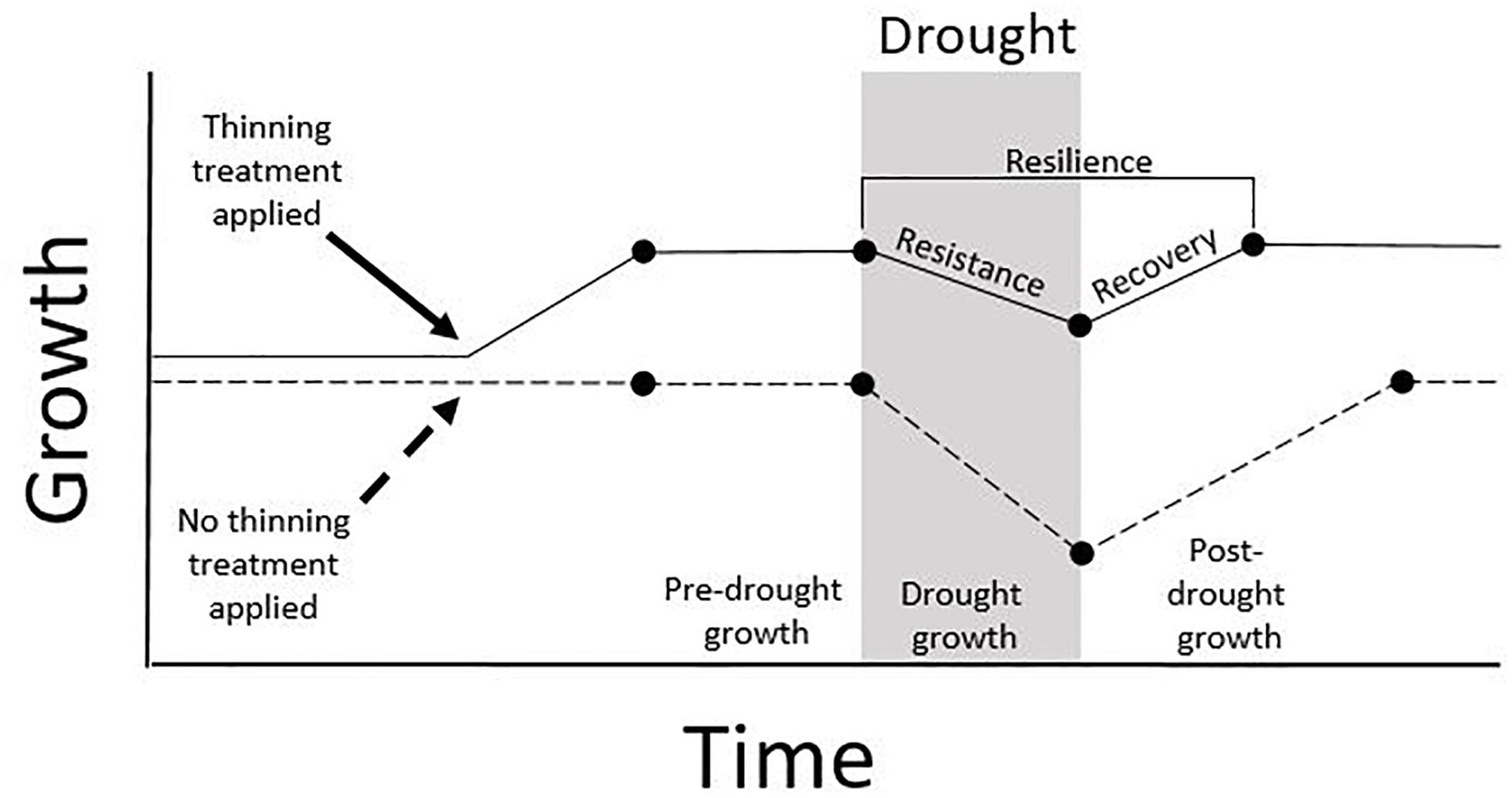
Figure 1. Hypothetical growth responses of individual trees to drought (shaded area) following thinning treatment. The solid line depicts a tree that responds to treatment with higher growth, relatively little loss in growth during drought (high resistance), and a relatively rapid return to pre-drought growth rate (high resilience). The dashed line depicts a tree in an untreated stand that subsequently experiences greater loss of growth during drought (low resistance) and requires longer to recover the pre-drought growth rate (low resilience).
What Is the Evidence?
There are surprisingly few published tree-ring-based studies of management effects on drought resistance and resilience in coniferous forests of the southwestern United States, a region known for its vulnerability to drought-induced tree mortality (Adams et al., 2009; Williams et al., 2013; Table 1). Field studies from outside of this region using tree-ring evidence support the use of thinning treatments to promote resistance and resilience to drought (Figure 1) for conifers (Kohler et al., 2010; Sohn et al., 2016), with the magnitude of response positively related to thinning intensity (Laurent et al., 2003), and negatively related to stand age (D’Amato et al., 2013). Time-since-thinning is also expected to influence outcomes, with treatment effectiveness becoming increasingly muted over time as vegetation regrows (Sohn et al., 2016). However, it is unclear how site aridity influences thinning responses to drought, a critical consideration for forests in the southwestern United States.
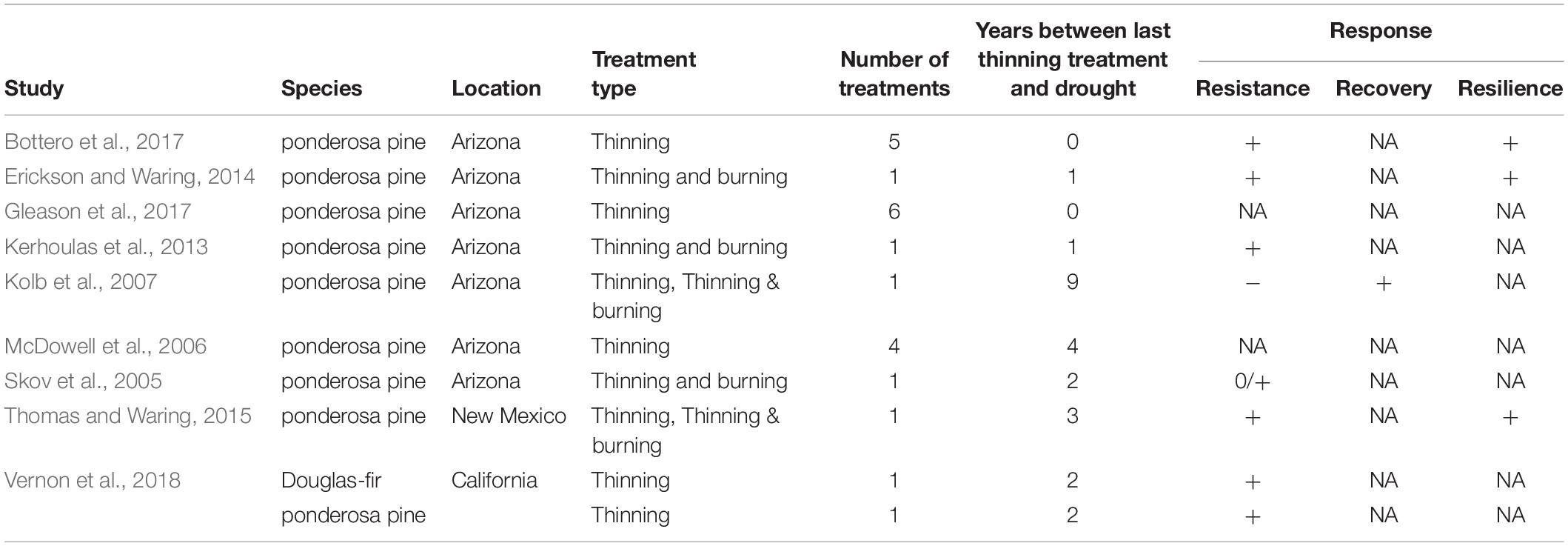
Table 1. Studies using tree-ring evidence to assess thinning and prescribed burning effectiveness in moderating drought response in dry coniferous forests of the southwestern United States.
Large-scale analyses using tree-ring drought response indices (Figure 1) provide somewhat conflicting results concerning the effects of site aridity. Across North America (including the southwestern United States) and Europe, Gazol et al. (2017a) found trees in arid sites had less resistance but greater recovery relative to mesic sites, although they did not consider thinning effects. They speculated that the faster recovery in arid sites may be attributable to species differences, with arid regions containing species better able to recover from drought. Gazol et al. (2017b) and Gazol et al. (2018) found the same drought response pattern with site aridity in intensive studies of sites across Spain. In contrast, a meta-analysis of drought effects and thinning including data from the southwestern United States found radial growth drought resistance (Figure 1) increased with site aridity, at least for unthinned and heavily thinned stands (Sohn et al., 2016). After drought Sohn et al. (2016) found this relationship with site aridity and drought response indices reversed, with aridity generally associated with decreased drought recovery and resilience. The authors hypothesized that trees adapted to drier environments invest more resources in non-structural carbohydrates, allowing them to maintain growth during initial drought stress, but then following drought it may be difficult to replace these resources and recover growth. Another large-scale study of growth response to drought and stand density in North America (using data from similar southwestern United States sites) found drought and competition reduced growth across all observed forest types, but that at arid sites growth was highly sensitive to drought stress and less sensitive to stand density (Gleason et al., 2017). The authors did not employ the drought response indices outlined in Figure 1, instead determining drought response from the relationship between drought severity and stand-level basal area increment based on tree-rings. The findings of Gleason et al. (2017) suggest that thinning can be used as a drought adaptation tool in dry coniferous forests of the United States Southwest, but that these treatments might be less effective relative to mesic forest types.
Specific to forests in the southwestern United States, the few existing reports have generally shown improved growth responses following thinning and drought (Table 1), but with differences among specific drought indices (Figure 1). Building on results from Feeney et al. (1998), Kolb et al. (2007) showed that thinning with and without prescribed fire resulted in lower resistance to severe drought for large (> 40 cm DBH, diameter at breast height, 1.37 m) ponderosa pine (Pinus ponderosa Douglas ex C. Lawson), although growth recovery appeared to be enhanced for trees in treated stands. Skov et al. (2005) found that small (≤ 39 cm DBH) ponderosa pine in northern Arizona had greater drought resistance with increasing thinning intensity across two years of drought, although larger individuals (≤ 55 cm DBH) did not show this response. Erickson and Waring (2014) and Thomas and Waring (2015) found that thinning increased drought resistance and resilience following severe drought for ponderosa pine in New Mexico and Arizona. Bottero et al. (2017) considered stand-level basal area increment based on tree-rings from ponderosa pine in northern Arizona (among other sites), finding that drought resistance and resilience were negatively related to stand density both in early- and late-stage drought. Kerhoulas et al. (2013) found that highly aggressive fuel treatments enhanced radial growth rates in large residual ponderosa pine in northern Arizona, potentially improving resistance to short-duration droughts. However, the resulting changes in tree architecture (i.e., greater leaf-to-sapwood area ratios) may commit residual trees to high transpirational demands, perhaps making them more vulnerable to droughts over the long term (McDowell et al., 2006). In a mixed-conifer forest in northern California, thinning improved drought resistance, but tree size, competition and species identity influenced this response (Vernon et al., 2018). Analyses of prescribed fire effects without mechanical thinning on drought response are far less common. Using repeated stem diameter measurements (not tree-ring data) in a Sierra Nevada mixed conifer forest, Collins et al. (2014) found that prescribed fire did not create growth conditions that reduced vulnerability for most common species.
The large-scale studies outlined above, as well as other studies (e.g., Zang et al., 2014), indicate that species are expected to respond individualistically to drought. For example, within the dry coniferous forests in northern California, Vernon et al. (2018) found that drought-tolerant ponderosa pine responded more positively to thinning treatments during drought relative to less drought-tolerant Douglas-fir [Pseudotsuga menziesii (Mirbel) Franco]. Responses of angiosperms to thinning and drought are less well studied than conifers (Sohn et al., 2016), a pattern that also holds in the southwestern United States. Gazol et al. (2017a, 2018) suggest gymnosperms and angiosperms may have different drought resistance and recovery responses that may be partially determined by species-specific physiological drought responses and adaptive capacities. As such, drought-related mortality risk tends to be associated with lower drought resistance in angiosperms, while in gymnosperms this risk is often associated with lower drought recovery (DeSoto et al., 2020). Further tree-ring-based assessments of hardwood responses to drought and how management may influence these responses are needed. Note that the studies using drought resistance, recovery, and resilience metrics (Figure 1) are subject to important biases outlined by Schwarz et al. (2019), making it challenging to generalize across studies. Also note that many of these studies set in the southwestern United States occurred at similar sites in northern Arizona and focused on ponderosa pine (Table 1).
Limitations of Tree-Ring Evidence
There are several limitations to the use of tree-rings to assess forest adaptation strategies. Tree-rings integrate a wide variety of signals; outside of extreme environments (e.g., high altitude, high latitude, or ecotonal forests) it is difficult to assign causes of growth variability without additional information (Speer, 2010). Typical sampling strategies can be problematic, with tree-ring samples often limited to large living or recently dead trees (i.e., the “fading record” problem) (Swetnam et al., 1999), complicating assessments of long-term growth patterns (Bowman et al., 2013). Tree-rings represent samples from individual trees and growth variability is affected by tree species, age, and size so that samples restricted to large trees cannot be reliably scaled up to stand- and landscape-level growth patterns (Clark et al., 2016). In recognition of this problem, some studies have sampled across a range of tree sizes (Bottero et al., 2017; Gleason et al., 2017).
Tree growth is only one possible measure of drought response. Other important metrics include tree mortality, reproduction, and vulnerability to further disturbances (e.g., wildfires, pests, and diseases). Several large-scale studies in the southwestern United States have demonstrated that competition is directly related to mortality during drought (Bradford and Bell, 2017; Young et al., 2017), so that thinning treatments may lower drought-related mortality (Restaino et al., 2019).
Beyond Growth: Complementary Evidence for Understanding Forest Drought Response
Tree-ring data can be combined with other data sources to provide a better understanding of the patterns and mechanisms underlying forest drought responses. We briefly describe some of these complementary data sources and how they may enhance our understanding of drought responses in dry coniferous forests of the southwestern United States.
Stable Isotopes – Evidence of Physiological Mechanisms Underlying Forest Responses
To better understand forest drought responses, tree-ring stable isotopes can provide insights on possible physiological mechanisms that may vary in response to management with annual and even sub-annual resolution. Examples of such mechanisms include water stress, intrinsic water use efficiency (iWUE, carbon assimilated per water transpired), and source water use. Despite this potential, tree-ring stable isotopes seem relatively underutilized, likely due to the fact that analyses of stable isotope ratios (e.g., δ13C and δ18O) and discrimination rates (Δ13C) generally require skilled expertise in sample preparation methods and data interpretation and costs can be high at commercial isotope laboratories.
Increasing resources for growth (e.g., water, light, and nutrients) by reducing competition seems an obvious result of thinning (and perhaps of prescribed fire), but these treatments can also potentially decrease water availability via increased evapotranspirational losses. Thus, tree-ring based analyses of stable isotopes at inter- and intra-annual timescales provide insights regarding the mechanisms of tree response to stand manipulations and drought. Across a range of environments many tree-ring δ13C analyses suggest that iWUE is a relatively homeostatic trait largely unresponsive to changes in competition (e.g., Fernández-de-Uña et al., 2016), while other studies indicate that the magnitude of change in rates of carbon assimilation and stomatal conductance of water vapor can differ in response to reductions in competition via thinning (McDowell et al., 2003; Giuggiola et al., 2016; Rezaie et al., 2018). Specific to arid southwestern United States coniferous forests, McDowell et al. (2006) found that following thinning treatments, increases in stomatal conductance of water vapor (largely driven by increased water availability) were higher than increases in carbon assimilation rates (largely driven by light and nitrogen availability), such that iWUE can decrease in response to management actions. Although iWUE responses to thinning are variable, the majority of tree-ring stable isotope analyses demonstrate that reductions in competition can increase tree water availability, stomatal conductance, and carbon assimilation, potentially decreasing forest vulnerability to drought, particularly via increased resilience (Figure 1; Sohn et al., 2013). In arid southwestern coniferous forests, tree-ring stable isotopes indicate that heavy thinning treatments can reduce tree water stress (McDowell et al., 2006; Sohn et al., 2014); this finding corroborates growth-based findings that management has the potential to decrease drought vulnerability in these forest types.
Forest Pests and Inducible Defenses
As noted above, drought response can be measured in terms of tree mortality. As in other forest types, mortality in southwestern United States forests may go beyond direct effects of drought stress (e.g., loss of hydraulic function and carbon starvation) (Adams et al., 2017), to include secondary attacks by forest pests. In the southwestern United States, important forest pests include bark beetles (Dendroctonus spp., Ips spp., and Scolytus spp.). Dense stands may be more susceptible to bark beetle attacks and subsequent tree mortality in association with drought (Negrón et al., 2009; Fettig et al., 2019). While drought stress may presage bark beetle attack in southwestern forests, severe drought may lead to bark beetle outbreaks where otherwise vigorous trees (as measured by stem growth rate) are killed (Stephenson et al., 2019). Under these circumstances forest management may be less effective.
In southwestern United States pines, tree-ring-based measurements of resin duct size and area can supplement annual growth data or be used alone to predict successful bark beetle attack and subsequent mortality (Kane and Kolb, 2010). In coniferous forests outside of the United States Southwest, thinning and prescribed fire may stimulate trees to increase resin flow and develop resin duct defenses against bark beetles (Hood et al., 2015, 2016). The increase in resin defenses may be related to improved growth in residual trees or in response to wounding (Hood and Sala, 2015). Thus far, the role of forest management on inducible defenses is not well defined for southwestern United States forests.
Linking Tree-Ring Data With Remotely Sensed Information
Linking tree-ring information with remotely sensed imagery provides a potential method to scale up observations. Gazol et al. (2018) found that tree-ring drought indices (Figure 1) and the Normalized Difference Vegetation Index (NDVI) showed similar responses to drought across several species in Spain. A similar comparison found trends in both records of declining forest productivity (NDVI) and growth (tree-rings) in interior Alaska associated with drought-induced climate changes between 1982 and 2008 (Beck et al., 2011). Expanding such analyses to directly consider the role of management in drought response in southwestern United States forests would help generalize findings based on tree-ring information alone to broader spatial scales.
Discussion
There are surprisingly few tree-ring-based studies of drought response following management treatments in dry coniferous forests of the southwestern United States, a heavily managed region particularly vulnerable to widespread drought-induced forest mortality. Currently published studies in the southwestern United States have focused on a forest type at a particular location (ponderosa pine forests in northern Arizona). These results can be thought of as a model system that anticipate responses in similar southwestern forests and allow analyses across aridity gradients (Sohn et al., 2016; Gleason et al., 2017). The evidence to date suggests we should expect other water limited coniferous forests in the southwestern United States to be highly sensitive to drought, and that growth responses to drought can be improved by stand management. How these responses vary by treatment type (mechanical thinning, prescribed fire, or both), treatment intensity, taxa (particularly for Douglas-fir and common Abies and Quercus spp.), and across elevation and topoclimate (e.g., aspect) remain key unanswered questions in the United States Southwest.
Tree-rings provide one well-studied way to critically evaluate the effectiveness of management treatments, in spite of some well-known limitations. Cross-study comparisons using tree-ring drought response metrics (Figure 1) can be improved with standardization (Schwarz et al., 2019) or using alternate response indices (e.g., Gleason et al., 2017). Tree-ring data can be especially powerful when coupled with additional observations, such as stable isotopes, providing key insights as to how management can shape drought response. The appraisal of management activities is crucially important to develop and refine strategies to respond to rapidly changing environments.
Author Contributions
All authors developed the study and assembled available literature. PM, LK, and ZW wrote the manuscript with contributions from RS.
Funding
This project was supported by the U.S. Geological Survey’s Ecosystems Mission Area, the National Park Service, and the National Science Foundation (Grant # BCS-1853903).
Conflict of Interest
The authors declare that the research was conducted in the absence of any commercial or financial relationships that could be construed as a potential conflict of interest.
Acknowledgments
We thank Jeff Kane, Tom Kolb and two reviewers who provided helpful comments on the manuscript. Any use of trade, firm, or product names is for descriptive purposes only and does not imply endorsement by the United States Government.
References
Adams, H. D., Guardioloa-Claramonte, M., Barron-Gafford, G. A., Villegas, J. C., Breshears, D. D., Zou, C. B., et al. (2009). Temperature sensitivity of drought-induced tree mortality portends increased regional die-off under global-change-type drought. Proc. Natl. Acad. Sci. U.S.A. 106, 7063–7066. doi: 10.1073/pnas.0901438106
Adams, H. D., Zeppel, M. J., Anderegg, W. R., Hartmann, H., Landhäusser, S. M., Tissue, D. T., et al. (2017). A multi-species synthesis of physiological mechanisms in drought-induced tree mortality. Na. Ecol. Evol. 1, 1285–1291.
Allen, C. D., Breshears, D. D., and McDowell, N. G. (2015). On underestimation of global vulnerability to tree mortality and forest die-off from hotter drought in the Anthropocene. Ecosphere 6:art129. doi: 10.1890/es15-00203.1
Beck, P. S. A., Juday, G. P., Alix, C., Barber, V. A., Winslow, S. E., Sousa, E. E., et al. (2011). Changes in forest productivity across Alaska consistent with biome shift. Ecol. Lett. 14, 373–379. doi: 10.1111/j.1461-0248.2011.01598.x
Bottero, A., D’Amato, A. W., Palik, B. J., Bradford, J. B., Fraver, S., Battaglia, M. A., et al. (2017). Density-dependent vulnerability of forest ecosystems to drought. J. Appl. Ecol. 54, 1605–1614. doi: 10.1111/1365-2664.12847
Bowman, D. M. J. S., Brienen, R. J. W., Gloor, E., Phillips, O. L., and Prior, L. D. (2013). Detecting trends in tree growth: not so simple. Trends Plant Sci. 18, 11–17. doi: 10.1016/j.tplants.2012.08.005
Bradford, J. B., and Bell, D. M. (2017). A window of opportunity for climate-change adaptation: easing tree mortality by reducing forest basal area. Front. Ecol. Environ. 15, 11–17. doi: 10.1002/fee.1445
Clark, J. S., Iverson, L., Woodall, C. W., Allen, C. D., Bell, D. M., Bragg, D. C., et al. (2016). The impacts of increasing drought on forest dynamics, structure, and biodiversity in the United States. Global Change Biol. 22, 2329–2352. doi: 10.1111/gcb.13160
Collins, B. M., Das, A. J., Battles, J. J., Fry, D. L., Krasnow, K. D., and Stephens, S. L. (2014). Beyond reducing fire hazard: fuel treatment impacts on overstory tree survival. Ecol. Appl. 24, 1879–1886. doi: 10.1890/14-0971.1
D’Amato, A. W., Bradford, J. B., Fraver, S., and Palik, B. J. (2013). Effects of thinning on drought vulnerability and climate response in north temperate forest ecosystems. Ecol. Appl. 23, 1735–1742. doi: 10.1890/13-0677.1
DeSoto, L., Cailleret, M., Sterck, F., Jansen, S., Kramer, K., Robert, E., et al. (2020). Low growth resilience to drought is related to future mortality risk in trees. Nat. Commun. 11:545.
Erickson, C. C., and Waring, K. M. (2014). Old Pinus ponderosa growth responses to restoration treatments, climate and drought in a southwestern US landscape. Appl. Vegetation Sci. 17, 97–108. doi: 10.1111/avsc.12056
Feeney, S. R., Kolb, T. E., Covington, W. W., and Wagner, M. R. (1998). Influence of thinning and burning restoration treatments on presettlement ponderosa pines at the Gus Pearson Natural Area. Can. J. For. Res. 28, 1295–1306. doi: 10.1139/x98-103
Fernández-de-Uña, L., McDowell, N. G., Cañellas, I., and Gea-Izquierdo, G. (2016). Disentangling the effect of competition, CO2 and climate on intrinsic water-use efficiency and tree growth. J. Ecol. 104, 678–690. doi: 10.1111/1365-2745.12544
Fettig, C. J., Mortenson, L. A., Bulaon, B. M., and Foulk, P. B. (2019). Tree mortality following drought in the central and southern Sierra Nevada. Calif. U.S. For. Ecol. Manag. 432, 164–178. doi: 10.1016/j.foreco.2018.09.006
Gazol, A., Camarero, J. J., Vicente-Serrano, S. M., Sánchez-Salguero, R., Gutiérrez, E., de Luis, M., et al. (2018). Forest resilience to drought varies across biomes. Global Change Biol. 24, 2143–2158. doi: 10.1111/gcb.14082
Gazol, A., Camarero, J. J., Anderegg, W. R. L., and Vicente-Serrano, S. M. (2017a). Impacts of droughts on the growth resilience of Northern Hemisphere forests: forest growth resilience to drought. Global Ecol. Biogeogr. 26, 166–176. doi: 10.1111/geb.12526
Gazol, A., Ribas, M., Gutiérrez, E., and Camarero, J. J. (2017b). Aleppo pine forests from across Spain show drought-induced growth decline and partial recovery. Agric. For. Meteorol. 232, 186–194. doi: 10.1016/j.agrformet.2016.08.014
Giuggiola, A., Ogée, J., Rigling, A., Gessler, A., Bugmann, H., and Treydte, K. (2016). Improvement of water and light availability after thinning at a xeric site: which matters more? A dual isotope approach. New Phytol. 210, 108–121. doi: 10.1111/nph.13748
Gleason, K. E., Bradford, J. B., Bottero, A., D’Amato, A. W., Fraver, S., Palik, B. J., et al. (2017). Competition amplifies drought stress in forests across broad climatic and compositional gradients. Ecosphere 8:e01849. doi: 10.1002/ecs2.1849
Hood, S., and Sala, A. (2015). Ponderosa pine resin defenses and growth: metrics matter. Tree Physiol. 35, 1223–1235.
Hood, S., Sala, A., Heyerdahl, E. K., and Boutin, M. (2015). Low-severity fire increases tree defense against bark beetle attacks. Ecology 96, 1846–1855. doi: 10.1890/14-0487.1
Hood, S. M., Baker, S., and Sala, A. (2016). Fortifying the forest: thinning and burning increase resistance to a bark beetle outbreak and promote forest resilience. Ecol. Appl. 26, 1984–2000. doi: 10.1002/eap.1363
Hurteau, M. D., Bradford, J. B., Fulé, P. Z., Taylor, A. H., and Martin, K. L. (2014). Climate change, fire management, and ecological services in the southwestern US. For. Ecol. Manag. 327, 280–289. doi: 10.1016/j.foreco.2013.08.007
Kane, J. M., and Kolb, T. E. (2010). Importance of resin ducts in reducing ponderosa pine mortality from bark beetle attack. Oecologia 164, 601–609. doi: 10.1007/s00442-010-1683-4
Kerhoulas, L. P., Kolb, T. E., Hurteau, M. D., and Koch, G. W. (2013). Managing climate change adaptation in forests: a case study from the U.S. Southwest. J. Appl. Ecol. 50, 1311–1320.
Kohler, M., Sohn, J., Nägele, G., and Bauhus, J. (2010). Can drought tolerance of Norway spruce (Picea abies (L.) Karst.) be increased through thinning? Eur. J. For. Res. 129, 1109–1118. doi: 10.1007/s10342-010-0397-9
Kolb, T. E., Agee, J. K., Fulé, P. Z., McDowell, N. G., Pearson, K., Sala, A., et al. (2007). Perpetuating old ponderosa pine. For. Ecol. Manag. 249, 141–157. doi: 10.1016/j.foreco.2007.06.002
Laurent, M., Antoine, N., and Joël, G. (2003). Effects of different thinning intensities on drought response in Norway spruce (Picea abies (L.) Karst.). For. Ecol. Manag. 183, 47–60. doi: 10.1016/s0378-1127(03)00098-7
Lloret, F., Keeling, E. G., and Sala, A. (2011). Components of tree resilience: effects of successive low-growth episodes in old ponderosa pine forests. Oikos 120, 1909–1920. doi: 10.1111/j.1600-0706.2011.19372.x
McDowell, N. G., Adams, H. D., Bailey, J. D., Hess, M., and Kolb, T. E. (2006). Homeostatic maintenance of ponderosa pine gas exchange in response to stand density changes. Ecol. Appl. 16, 1164–1182. doi: 10.1890/1051-0761(2006)016
McDowell, N. G., Brooks, J. R., Fitzgerald, S. A., and Bond, B. J. (2003). Carbon isotope discrimination and growth response of old Pinus ponderosa trees to stand density reductions. Plant Cell Environ. 26, 631–644. doi: 10.1046/j.1365-3040.2003.00999.x
Millar, C. I., and Stephenson, N. L. (2015). Temperate forest health in an era of emerging megadisturbance. Science 349, 823–826. doi: 10.1126/science.aaa9933
Negrón, J. F., McMillin, J. D., Anhold, J. A., and Coulson, D. (2009). Bark beetle-caused mortality in a drought-affected ponderosa pine landscape in Arizona. USA. For. Ecol. Manag. 257, 1353–1362. doi: 10.1016/j.foreco.2008.12.002
Restaino, C., Young, D. J., Estes, B., Gross, S., Wuenschel, A., Meyer, M., et al. (2019). Forest structure and climate mediate drought-induced tree mortality in forests of the Sierra Nevada. USA. Ecol. Appl. 29:e01902. doi: 10.1002/eap.1902
Rezaie, N., D’Andrea, E., Bräuning, A., Matteucci, G., Bombi, P., and Lauteri, M. (2018). Do atmospheric CO2 concentration increase, climate and forest management affect iWUE of common beech? Evidences from carbon isotope analyses in tree rings. Tree Physiol. 38, 1110–1126. doi: 10.1093/treephys/tpy025
Ryan, K. C., Knapp, E. E., and Varner, J. M. (2013). Prescribed fire in North American forests and woodlands: history, current practice, and challenges. Front. Ecol. Environ. 11:e15–e24. doi: 10.1890/120329
Schwarz, J. A., Skiadaresis, G., Kohler, M., Kunz, J., Schnabel, F., Vitali, V., et al. (2019). Quantifying growth responses of trees to drought - a critique of the Lloret-indicators and recommendations for future studies. EcoEvoRxiv [Preprint]. doi: 10.32942/osf.io/5ke4f
Schwilk, D. W., Keeley, J. E., Knapp, E. E., McIver, J., Bailey, J. D., Fettig, C. J., et al. (2009). The national Fire and Fire Surrogate study: effects of fuel reduction methods on forest vegetation structure and fuels. Ecol. Appl. 19, 285–304. doi: 10.1890/07-1747.1
Skov, K. R., Kolb, T. E., and Wallin, K. F. (2005). Difference in radial growth response to restoration thinning and burning treatments between young and old ponderosa pine in Arizona. Western J. Appl. For. 20, 36–43. doi: 10.1093/wjaf/20.1.36
Sohn, J. A., Brooks, J. R., Bauhus, J., Kohler, M., Kolb, T. E., and McDowell, N. G. (2014). Unthinned slow-growing ponderosa pine (Pinus ponderosa) trees contain muted isotopic signals in tree rings as compared to thinned trees. Trees 28, 1035–1051. doi: 10.1007/s00468-014-1016-z
Sohn, J. A., Gebhardt, T., Ammer, C., Bauhus, J., Häberle, K.-H., Matyssek, R., et al. (2013). Mitigation of drought by thinning: short-term and long-term effects on growth and physiological performance of Norway spruce (Picea abies). For. Ecol. Manag. 308, 188–197. doi: 10.1016/j.foreco.2013.07.048
Sohn, J. A., Saha, S., and Bauhus, J. (2016). Potential of forest thinning to mitigate drought stress: a meta-analysis. For. Ecol. Manag. 380, 261–273. doi: 10.1016/j.foreco.2016.07.046
Stephenson, N. L., Das, A. J., Ampersee, N. J., Bulaon, B. M., and Yee, J. L. (2019). Which trees die during drought? The key role of insect host-tree selection. J. Ecol. 107, 2383–2401. doi: 10.1111/1365-2745.13176
Swetnam, T. W., Allen, C. D., and Betancourt, J. L. (1999). Applied historical ecology: using the past to manage for the future. Ecol. Appl. 9, 1189–1206. doi: 10.1890/1051-0761(1999)009
Thomas, Z., and Waring, K. M. (2015). Enhancing resiliency and restoring ecological attributes in second-growth ponderosa pine stands in northern New Mexico, USA. For. Sci. 61, 93–104. doi: 10.5849/forsci.13-085
Vernon, M. J., Sherriff, R. L., van Mantgem, P., and Kane, J. M. (2018). Thinning, tree-growth, and resistance to multi-year drought in a mixed-conifer forest of northern California. For. Ecol. Manag. 422, 190–198. doi: 10.1016/j.foreco.2018.03.043
Waring, R. H. (1987). Characteristics of trees predisposed to die. Bioscience 37, 569–574. doi: 10.2307/1310667
Williams, A. P., Allen, C. D., Macalady, A. K., Griffin, D., Woodhouse, C. A., Meko, D. M., et al. (2013). Temperature as a potent driver of regional forest drought stress and tree mortality. Nat. Clim. Change 3, 292–297. doi: 10.1038/nclimate1693
Young, D. J. N., Stevens, J. T., Earles, J. M., Moore, J., Ellis, A., Jirka, A. L., et al. (2017). Long-term climate and competition explain forest mortality patterns under extreme drought. Ecol. Lett. 20, 78–86. doi: 10.1111/ele.12711
Keywords: dendrochronology, ecosystems, fire effects, fuel treatments, thinning
Citation: van Mantgem PJ, Kerhoulas LP, Sherriff RL and Wenderott ZJ (2020) Tree-Ring Evidence of Forest Management Moderating Drought Responses: Implications for Dry, Coniferous Forests in the Southwestern United States. Front. For. Glob. Change 3:41. doi:10.3389/ffgc.2020.00041
Received: 21 December 2019; Accepted: 20 March 2020;
Published: 15 April 2020.
Edited by:
Julia Annick Schwarz, University of Freiburg, GermanyReviewed by:
Peter Fule, Northern Arizona University, United StatesJesús Julio Camarero, Spanish National Research Council, Spain
Copyright © 2020 van Mantgem, Kerhoulas, Sherriff and Wenderott. This is an open-access article distributed under the terms of the Creative Commons Attribution License (CC BY). The use, distribution or reproduction in other forums is permitted, provided the original author(s) and the copyright owner(s) are credited and that the original publication in this journal is cited, in accordance with accepted academic practice. No use, distribution or reproduction is permitted which does not comply with these terms.
*Correspondence: Phillip J. van Mantgem, cHZhbm1hbnRnZW1AdXNncy5nb3Y=