- 1Department of Plant Pathology, The Ohio State University, Columbus, OH, United States
- 2Center for Invasive Species Prevention, Bethesda, MD, United States
- 3Department of Biological Sciences, Wright State University, Dayton, OH, United States
- 4D. B. Warnell School of Forestry and Natural Resources, University of Georgia, Athens, GA, United States
- 5Department of Entomology, North Carolina State University (Emeritus) and Forest Restoration Alliance, Raleigh, NC, United States
- 6Department of Environmental and Forest Biology, College of Environmental Science and Forestry, State University of New York, Syracuse, NY, United States
- 7Department of Plant and Microbial Biology, University of Minnesota, Minneapolis, MN, United States
- 8Rubenstein School of Environment and Natural Resources, University of Vermont, Burlington, VT, United States
- 9USDA Forest Service, Northern Research Station, Burlington, VT, United States
Maintenance and restoration of forest ecosystems will be key to achieving necessary carbon sequestration goals, protecting biodiversity, and supporting healthy economies and societies. Forest ecosystems are increasingly threatened by non-native forest insects and pathogens. A portion of these pests are able to overcome prevention and containment efforts and become established in naïve ecosystems. Once established these pests pose a long-term large-scale threat to forest ecosystems, which current policy and response frameworks are poorly equipped to address. We propose the creation of a federal Center for Forest Pest Control and Prevention to implement end-to-end responses to forest pest invasions using an ecologically-informed framework that fully integrates host tree resistance development and deployment.
Introduction
Forests provide ecosystem services necessary for human welfare and survival, such as oxygen production, moderation of extreme weather, biodiversity maintenance, flood control, air and water purification, as well as timber and fiber used in construction and other industries. Our own health is directly intertwined with trees; for instance, intact tree canopies have been associated with reduced incidence of cardiovascular diseases in urban populations (Donovan et al., 2013), and evidence is mounting for the beneficial effects of “forest bathing,” a practice developed in Japan and known as shinrin-yoku, in which people simply spend time in forest environments (Ideno et al., 2017).
Forests are also essential for carbon sequestration. A recent study suggested that 4.4 billion hectares of tree cover could exist under the current climate (Bastin et al., 2019). Excluding existing trees, agricultural and urban areas, an extra 0.9 billion hectares of tree cover could be accommodated worldwide, and could store up to 205 additional Gt of carbon. Therefore, global tree restoration should be a key goal in our fight against climate change (Bastin et al., 2019).
If forest restoration and expansion is our goal, we must then recognize that modern global connectivity threatens forests worldwide through the unintentional dissemination of hundreds of invasive, non-native, tree-killing pathogens and insect pests (PIPs) that devastate large swaths of forests, in some cases leading to functional extinction of important tree species (Gandhi and Herms, 2010; e.g., Figure 1). Such tree-killing PIPs are often, although not always, intimately associated with their host and destroy high fitness-value, difficult to replace tissues, such as the xylem, phloem or cambium of the main stem or root system; examples include pathogens causing wilts and cankers, and wood boring insects (Showalter et al., 2018). In the US alone, Appalachian forests lost once-dominant chestnut trees to chestnut blight (Anagnostakis, 1987). Cathedral-like canopies of elm trees over streets and boulevards across eastern North America vanished due to Dutch elm disease (Santini and Faccoli, 2015), only to be replaced by ash trees, which are now dying by the billions due to emerald ash borer (Herms and McCullough, 2014). Hundreds of thousands of redbays in the Southeast are being killed by laurel wilt, which is caused by a fungal pathogen vectored by a wood boring insect (Harrington et al., 2008). Millions of tanoak and true oak trees on the west coast have been killed by sudden oak death (Rizzo and Garbelotto, 2003), while millions of whitebark pines in fragile high elevation ecosystems of the Rockies and Cascades of the Western US have succumbed to white pine blister rust (Geils et al., 2010). The iconic and culturally significant koa and ‘ohi‘a trees in Hawaiian forests now face two new devastating diseases, koa wilt (Anderson et al., 2002) and rapid ‘ohi‘a death (Keith et al., 2015).
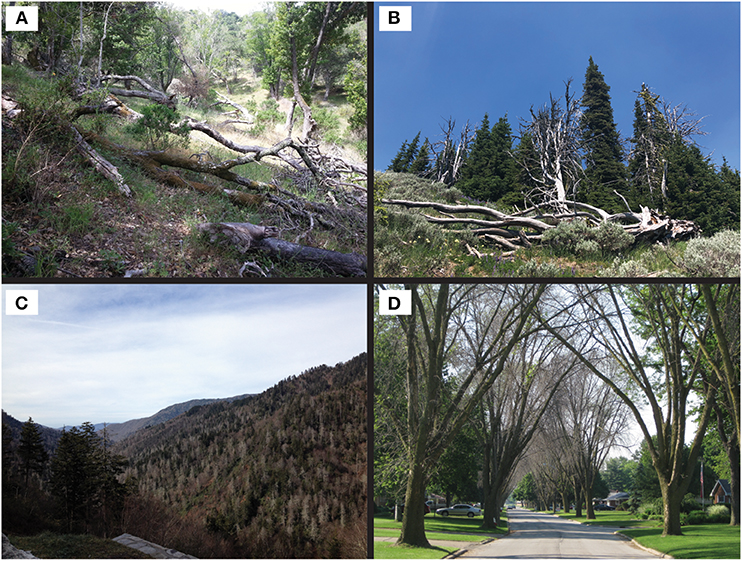
Figure 1. Single tree and ecosystem level damage stemming from large scale tree mortality events due to non-native invasive forest pathogens and pests: (A) sudden oak death in California; (B) white pine blister rust in Oregon; (C) hemlock wooly adelgid in Tennessee; and (D) emerald ash borer in Ohio. Sources: (A,B) Anna O. Conrad; (C) Dylan Parry; (D) Daniel A. Herms (with permission).
This veritable reaper's list is long indeed and plays out similarly across the globe, whether it be pine wilt nematode first in Asia and now Europe (Hirata et al., 2017), red turpentine beetle in China (Sun et al., 2013), or ash dieback in Europe (Mitchell et al., 2014). In addition to lost cultural significance and aesthetic value of native trees, their decline and death have staggering economic impacts. Conservative estimates of the annual costs of bark and woodboring insect invasions in the US alone reach $1.7 billion in local government expenditures and $830 million in lost residential property value (Aukema et al., 2011). Lastly, a recent study found that significant shifts in carbon dynamics are being driven by invasive pests (Fei et al., 2019). Over 40% of the total live biomass in US forests is at risk from currently established pest species. The authors estimated that the carbon released by trees killed by invasive insects and diseases in the US as they decay is equivalent to the carbon output of 4.4 million additional cars on our roadways, or nearly 20% of that released annually by wildfire in the US (https://www.sciencedaily.com/releases/2019/08/190813170729.htm).
Current Approaches Are Not Sufficient: Policy Options and Implications
Important measures have been taken to prevent PIP introductions, and while vital, these efforts are insufficient. For perspective, the number of containers (20-foot equivalent units) entering the U.S. annually through 63 ports increased from just over 11 million in 2000 to well over 22 million in 2017 (Source: U.S. Department of Transportation, Maritime Administration). Approximately 75% of containers used in maritime trade include wood packaging, a well-known source of invaders (Meissner et al., 2009). The opportunity for biological invasions is constant and the threat overwhelming, even at our most regulated ports. Critical advancements have been made in trade policies aimed at preventing invasions, e.g., through efforts by the International Plant Protection Convention (IPPC) by way of its International Standards for Phytosanitary Measures, such as ISPM 07 “Phytosanitary certification system,” ISPM 15 “Regulation of wood packaging material in international trade,” and ISPM 36 “Integrated measures for plants for planting” (https://www.ippc.int/en/core-activities/standards-setting/ispms/#publications).
However, despite these significant preventative efforts, “biological spills” keep occurring at an alarming rate and show no signs of abating (Seebens et al., 2017; Meurisse et al., 2019; Thakur et al., 2019). While prevention, early detection, and eradication are our critical frontline defenses, once breached, established, non-native, tree-killing forest PIPs often cause widespread mortality of native trees. Efforts to reduce the magnitude and extent of tree mortality from PIPs requires vast human and monetary capital. Despite such expenditures, these invasions often run their course. Thus, if the primary objective following successful PIP invasion is to protect and restore an invaded forest ecosystem to its former state, we have largely failed. This is likely due to existing mandates of the primary agencies tasked with managing PIP invasions in the US, which are disproportionately focused on prevention, early detection/rapid response, and management/mitigation (USDA-APHIS), or are stretched to the breaking point by competing urgent issues, such as wildfire (US Forest Service). A steady loss of research capacity is also contributing to this state of affairs (Gandhi et al., 2019). The lack of a single authority with a clear mandate prioritizing long-term response to established PIPs is a major impediment to effective research efforts and management, for example with respect to restoration by way of resistant trees. In the meantime, our forest ecosystems change fundamentally with no hope of reversing course, and the local communities continue to bear the costs of tree dieback and mortality.
Actionable Recommendations: A New Way Forward—Centers for Forest Pest Control and Prevention
The UN General Assembly has declared 2020 the International Year of Plant Health (IYPH). Thus, we have a special opportunity to raise awareness of the need for much better cooperation, both within and between countries, to manage invasive forest PIPs and herewith propose a new structural approach.
In many ways, biological invasions are like natural disasters (Ricciardi et al., 2011). Many societies devote significant resources to preparedness for dealing with events that are unpredictable, yet explainable in hindsight, known as “black swan events.” In most cases, such preparedness is coordinated at the highest levels of government. For example, in the US, the Federal Emergency Management Agency (FEMA) of the Department of Homeland Security, and the Centers for Disease Control and Prevention (CDC), housed in the US Department of Health and Human Services, are always ready for top level coordinated responses in cases of events like earthquakes and hurricanes, or human disease invasions. No such structure exists for forest PIP invasion events, despite the fact that, combined with all other biological invasions worldwide, they cost more every year than all other natural disasters combined (Ricciardi et al., 2011).
Like all natural disasters, the management of forest pest invasions demands national and “international coordination of early-warning systems, immediate access to critical information, specialized training of personnel, and rapid-response strategies” (Ricciardi et al., 2011). To correct the poor response coordination with forest PIP invasions, we strongly advocate for a radical change in approach, based on a highly proactive, highly integrated framework of action: The USDA Centers for Forest Pest Control and Prevention (CFPCPs) (Figure 2). This concept has been endorsed by a wide range of leading forest health professionals both nationally and internationally (https://trag.osu.edu/). A similar proposal for a National Center for Invasive Species Management (NCISM) was made by invasion ecologists to unite numerous federal agencies and address not only forest pest invasions, but other terrestrial and aquatic plant and animal invasions (Lodge et al., 2006). The NCISM was never established, and it could be argued that a center dealing with all invasive species would have been rather unwieldy. Similarly, a proposal for a global surveillance system for food crops has recently emerged (Carvajal-Yepes et al., 2019).
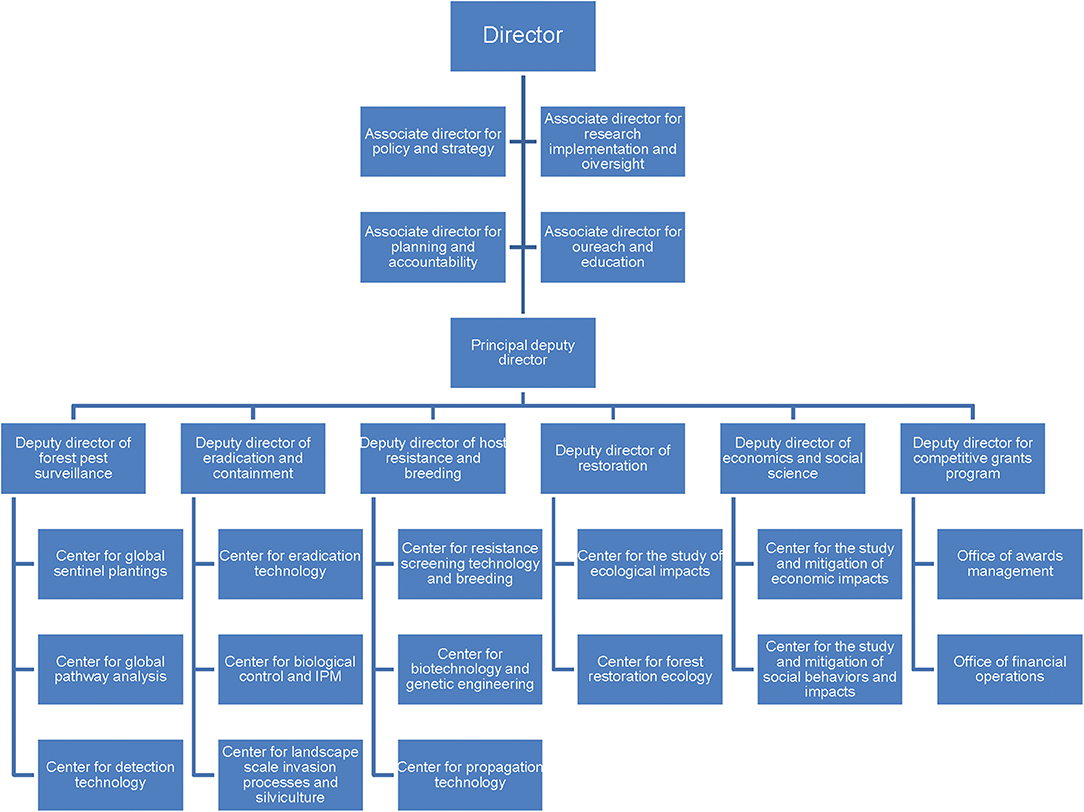
Figure 2. Proposed, hypothetical partial structure of the Centers for Forest Pest Control and Prevention. The structure would be similar to the CDCs in the US Department of Health and Human Services and would be housed in the US Department of Agriculture.
The mission of the CFPCPs would be to coordinate closely with federal agencies and other stakeholders (Figure 3) to develop and—most importantly—ensure application by the agencies of pro-active, long-term strategies addressing each host/PIP combination. Research conducted at the CFPCPs would include PIP and host biology and ecology, pathway vulnerabilities and safeguarding measures, understanding landscape patterns of invasion, detection and control technologies, host resistance mechanisms, breeding, etc. (Figure 2). The goal would be to develop strategies to address each host/PIP combination “end-to-end.” The CFPCPs should be supported by sufficient and reliable base funding along with protection from federal bureaucratic hurdles, so that they can make consistent and critical progress in pursuing these goals. All these efforts individually and collectively would require expert staff (as is the case for FEMA and the CDC) being able to consistently dedicate time to this endeavor. Importantly, a Deputy Director (Figure 2) would be responsible for ensuring that research findings are integrated into agency programs. Strategic committees served by forest health scientists and stakeholders (e.g., forest managers and landowners, conservation organizations) would provide input and direction to research plans.
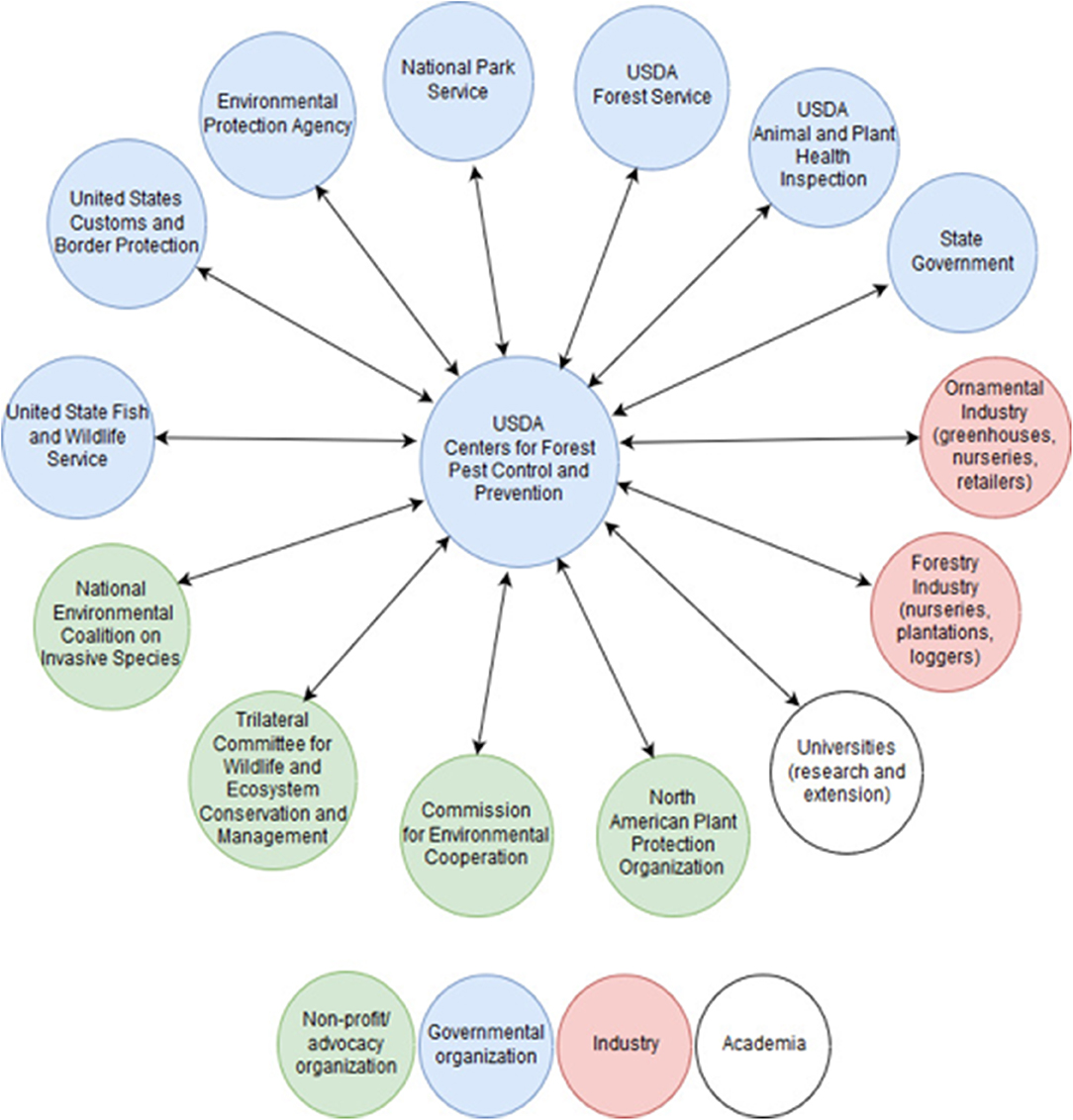
Figure 3. Hypothetical linkages of the CFPCPs with US federal and state agencies, NGOs, academic institutions and experts, and industrial stakeholders, providing a more centralized, coordinated, and effective response to invasive alien forest pathogens and insect pests (PIPs). CFPCPs activities would be geared toward “end-to-end” management of invasive PIPs, from prevention of introduction to ecosystem restoration. For clarity, potential international linkages are not shown.
The Centers would provide the necessary infrastructure to enable engagement of experts, local communities and stakeholders in executing these long-term strategies as well as manage a competitive research grant program. Because the broad geographic extent of American forests encompasses widely divergent ecosystems, we suggest that the Centers be endowed with access to or direct management of regional experimental tree improvement farms and laboratories. These may or may not correspond to USFS Research Stations. The Centers and the farms and associated facilities should be staffed by knowledgeable career experts, including forest pathologists, entomologists, geneticists, breeders, ecologists, economists, and social scientists ready to cope with any new invasive PIP that will undoubtedly continue to arrive and establish beachheads in our forests. A key linkage for the CFPCPs would be with academia: in the last decade, many forest health specialists have been hired at land-grant universities (e.g., in the southern and northeastern regions) and they are training the next generation of scientists in their area of specialization.
The following are two examples of end-to-end practices the Centers might entertain:
1. Coordinate establishment of sentinel plantings in geographic regions from which forest PIPs are likely to be introduced, e.g., east Asia and western Europe (Eschen et al., 2019). Planted in the gardens would be a sample of important North American tree species. These would be closely monitored to detect evidence of damage caused by insects, pathogens, etc. The Centers would ensure that such evidence of PIP vulnerability is immediately evaluated, and the risk is conveyed to APHIS, USFS, US Customs and Border Protection, etc. to inform those agencies' programs.
2. Establish regional experimental tree improvement farms and laboratories modeled on some of the best existing examples of sustained programs for host resistance selection and breeding, e.g., the USFS Dorena Genetic Resource Center in Cottage Grove, Oregon and the Resistance Screening Center in Asheville, North Carolina.
A Missing Key Component: Host Resistance Development and Deployment
A damaging forest PIP invasion can be seen as arising from the intersection of three necessary factors, known as the disease/pest triangle: a virulent pathogen or aggressive insect, a conducive environment, and a susceptible host (Figure 4). In principle, any of these three factors can be targeted for action. However, control and management of forest invasion events are particularly difficult. These high impact events are often discovered only after they are already well-established and past the point where the causal agents can be eradicated or contained (e.g., Cunniffe et al., 2016). While biological control is an important tool and can be effective against some forest pests (Kenis et al., 2017), success against woodboring insects has been poor (Tobin et al., 2014) and we are aware of only one successful biological control program directed against any tree-killing pathogen, involving chestnut blight in Europe (fungal hypovirus) (Heiniger and Rigling, 1994). [Notably, for reasons still not quite clear, this approach has not worked in North America, despite numerous attempts]. This is particularly true when the host trees are highly susceptible (Kenis et al., 2017), a universal situation in high impact events where naïve hosts are exposed to aggressive pathogens or pests with which they have not coevolved. For example, North American green ash trees growing in China commonly succumb to emerald ash borer, i.e., where both the pest and its associated parasitoids are native. Similarly, European birch tree species planted in North America are devastated by bronze birch borer, despite the presence of native parasitoids of this pest. Unfortunately, underlying host resistance is not always reflected in current policy or practice in biological control strategies, even though it is critical for its success (e.g., https://www.federalregister.gov/documents/2018/09/19/2018-20296/removal-of-emerald-ash-borer-domestic-quarantine-regulations). Other control measures, like the application of pesticides, are either not feasible in forests for logistical and economic reasons, or may have unacceptable environmental and human health costs. Hence, successful targeting of the damaging PIP is often not possible, while the environment cannot be easily manipulated to render it less conducive to a given invasive, non-native PIP without adding compounded disturbances to ecosystems.
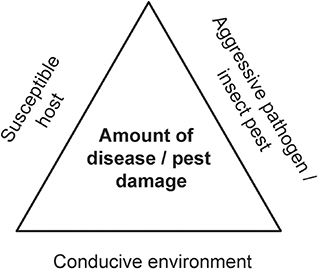
Figure 4. The basic disease/pest triangle visualizes the relationships among the three core elements necessary for development of a plant disease or initiation of an attack by a plant pest. No disease or insect attack occurs in the absence of any one or more of the three elements. This framework is particularly useful to understand forest invasions by non-native pathogens and pests, for which no bottom up forces (such as host resistance) or top-down drivers (such as predators/parasitoids) are available to successfully limit the damage.
What can be done? We posit that the most logical intervention point in the disease/pest triangle is the host, through the development and deployment of genetic resistance using modern techniques that allow for much faster breeding cycles (Harfouche et al., 2012). This largely neglected management approach would find a natural fit within the CFPCPs.
We observe that native tree hosts in the native range of a PIP are generally resistant because PIP and trees have coevolved over millennia. Also, with a few exceptions, a small proportion of trees usually express natural resistance and suffer less severe damage even in areas where invasive PIPs have been introduced. This natural resistance, both in the native and invaded environments, provides a foundation that can be exploited to inform management policy, including the restoration of our forest ecosystems. Genetic resistance is of fundamental importance to plant health in general, a fact well-recognized by crop breeders all over the world for at least a century. Until recently, a similar approach has been lacking or rare for forest trees, in large part due to the long time spans necessary to achieve practical results using traditional breeding. A few successful instances of resistance selection do exist, e.g., jarrah (Eucalyptus marginata) resistant to Phytophthora cinnamomi in Australia (Stukely et al., 2007); elms resistant to Dutch elm disease in Europe and North America (Santini and Faccoli, 2015); incipient work on European ash (Fraxinus excelsior) resistance to Hymenoscyphus fraxineus, the causal agent of ash dieback (Villari et al., 2018); and early work on some North American species (Sniezko and Koch, 2017). However, we are nowhere close to the systematic use of host resistance for conservation and restoration purposes.
Fortunately, modern scientific techniques are now making tree breeding possible at speeds that were unthinkable just a couple of decades ago (Harfouche et al., 2012). In some cases, once the ecological/environmental, economic, and human/cultural costs of giving up the fight are appreciated, the solution is largely a matter of structural organization and policy, and availability and proper allocation of resources (Buggs, 2020).
We argue that developing and deploying tree resistance based on natural genetic variability provides the best foundation for forest restoration after non-native forest PIPs become established. First, resistant trees identified in the forest (e.g., Conrad et al., 2019) could be protected from other anthropogenic disturbances, such as tree removal for slow-the-spread activities, salvage-logging, or fire, so that they can contribute to the process of directed natural selection in favor of resistant offspring. This would not be very different from the strategy of leaving seed trees in some silvicultural operations. Second, whenever we experience a new invasion event, a modern tree breeding program, increasingly capable of enhancing tree defenses and providing trees for planting that are capable of withstanding these invasive PIPs (Sniezko and Koch, 2017), can be rapidly initiated, for instance by applying increasingly user-friendly, non-destructive phenotyping technology (e.g., Conrad and Bonello, 2016). Given the very large potential number of cases, a prioritization of effort is necessary. One such strategy has been codified by Showalter et al. (2018). Once a case has been prioritized, with modern tools a tree improvement cycle can be achieved in as little as 5 years for some species, compared with traditional cycles that can take 26+ years (Harfouche et al., 2012). Resistant stock can then be interplanted into forests undergoing invasion, a strategy called “applied nucleation,” by which discrete areas are restored with resistant stock to serve as “islands of resistance” for future regeneration (Corbin and Holl, 2012). This approach may not be feasible in all areas, depending on hazard rating (Sniezko and Koch, 2017; Sniezko et al., 2019). Finally, even marginally more resistant trees would significantly improve the efficacy of biological control, because they would help counteract the defense-free space that PIPs find in the invaded ecosystems (Showalter et al., 2018).
Conclusions
Unfortunately, general misconceptions by regulatory agencies and often piecemeal emergency responses, exacerbated by a lack of expertise in science and technology among legislators, hamper progress. Lack of foresight, funding limitations, and insufficient infrastructure have relegated host resistance development to almost an afterthought, to be entertained only after everything else has failed. A proactive and sustained approach is necessary for a successful program of tree resistance research and deployment aimed at forest restoration, a concept that was reiterated in a recent report from the National Academies of Sciences, Engineering, and Medicine (National Academies of Sciences, 2019). Such important strategy would be best encoded in the mandate of new CFPCPs, for example through a recently proposed framework of action (Showalter et al., 2018).
Although we propose an initial structure for the CFPCPs in Figure 2, the final organizational chart of the CFPCPs should be defined by an ad hoc committee/task force established by Congress, which would include USDA officials, independent/academic scientists in the disciplines listed above, and representing a diversity of affected geographic regions and PIP problems, as well as industrial stakeholders. Whatever the final structure of the Centers, implementation of the CFPCPs would require strong political will, supported by well-informed legislators, stakeholders and honest brokers. Society cannot wait any longer if we are to avoid unrecoverable damage to our forest ecosystems. Our forests must be preserved, protected, and restored for the present and future of our shared humanity.
Author Contributions
PB: ideation. PB, FC, KG, FH, DS, and CV: conceptualization. PB, FC, KG, FH, DS, and CV: writing original draft. PB, FC, DC, AC, CF, KG, FH, DP, DS, CV, and KW: writing—review and editing.
Conflict of Interest
The authors declare that the research was conducted in the absence of any commercial or financial relationships that could be construed as a potential conflict of interest.
Acknowledgments
The authors appreciate the input of the two reviewers.
References
Anagnostakis, S. L. (1987). Chestnut blight: the classical problem of an introduced pathogen. Mycologia 79, 23–37. doi: 10.1080/00275514.1987.12025367
Anderson, R. C., Gardner, D. E., Daehler, C. C., and Meinzer, F. C. (2002). Dieback of acacia koa in Hawaii: ecological and pathological characteristics of affected stands. For. Ecol. Manage. 162, 273–286. doi: 10.1016/S0378-1127(01)00522-9
Aukema, J. E., Leung, B., Kovacs, K., Chivers, C., Britton, K. O., Englin, J., et al. (2011). Economic impacts of non-native forest insects in the continental United States. PLoS ONE 6:e24587. doi: 10.1371/journal.pone.0024587
Bastin, J. F., Finegold, Y., Garcia, C., Mollicone, D., Rezende, M., Routh, D., et al. (2019). The global tree restoration potential. Science 365, 76–79. doi: 10.1126/science.aax0848
Buggs, R. J. A. (2020). Changing perceptions of tree resistance research. Plants People Planet 2, 2–4. doi: 10.1002/ppp3.10089
Carvajal-Yepes, M., Cardwell, K., Nelson, A., Garrett, K. A., Giovani, B., Saunders, D. G. O., et al. (2019). A global surveillance system for crop diseases. Science 364, 1237–1239. doi: 10.1126/science.aaw1572
Conrad, A. O., and Bonello, P. (2016). Application of infrared and raman spectroscopy for the identification of disease resistant trees. Front. Plant Sci. 6:1152. doi: 10.3389/fpls.2015.01152
Conrad, A. O., McPherson, B. A., Lopez-Nicora, H. D., D'amico, K. M., Wood, D. L., and Bonello, P. (2019). Disease incidence and spatial distribution of host resistance in a coast live oak/sudden oak death pathosystem. For. Ecol. Manage. 433, 618–624. doi: 10.1016/j.foreco.2018.11.035
Corbin, J. D., and Holl, K. D. (2012). Applied nucleation as a forest restoration strategy. For. Ecol. Manage. 265, 37–46. doi: 10.1016/j.foreco.2011.10.013
Cunniffe, N. J., Cobb, R. C., Meentemeyer, R. K., Rizzo, D. M., and Gilligan, C. A. (2016). Modeling when, where, and how to manage a forest epidemic, motivated by sudden oak death in California. Proc. Natl. Acad. Sci. U.S.A. 113, 5640–5645. doi: 10.1073/pnas.1602153113
Donovan, G. H., Butry, D. T., Michael, Y. L., Prestemon, J. P., Liebhold, A. M., Gatziolis, D., et al. (2013). The relationship between trees and human health—-evidence from the spread of the emerald ash borer. Am. J. Prevent. Med. 44, 139–145. doi: 10.1016/j.amepre.2012.09.066
Eschen, R., O'hanlon, R., Santini, A., Vannini, A., Roques, A., Kirichenko, N., et al. (2019). Safeguarding global plant health: the rise of sentinels. J. Pest Sci. 92, 29–36. doi: 10.1007/s10340-018-1041-6
Fei, S., Morin, R. S., Oswalt, C. M., and Liebhold, A. M. (2019). Biomass losses resulting from insect and disease invasions in US forests. Proc. Natl. Acad. Sci. U.S.A. 116, 17371–17376. doi: 10.1073/pnas.1820601116
Gandhi, K. J. K., Campbell, F., and Abrams, J. (2019). Current status of forest health policy in the United States. Insects 10:106. doi: 10.3390/insects10040106
Gandhi, K. J. K., and Herms, D. A. (2010). Direct and indirect effects of alien insect herbivores on ecological processes and interactions in forests of eastern North America. Biol. Invas. 12, 389–405. doi: 10.1007/s10530-009-9627-9
Geils, B. W., Hummer, K. E., and Hunt, R. S. (2010). White pines, ribes, and blister rust: a review and synthesis. For. Pathol. 40, 147–185. doi: 10.1111/j.1439-0329.2010.00654.x
Harfouche, A., Meilan, R., Kirst, M., Morgante, M., Boerjan, W., Sabatti, M., et al. (2012). Accelerating the domestication of forest trees in a changing world. Trends Plant Sci. 17, 64–72. doi: 10.1016/j.tplants.2011.11.005
Harrington, T. C., Fraedrich, S. W., and Aghayeva, D. N. (2008). Raffaelea lauricola, a new ambrosia beetle symbiont and pathogen on the lauraceae. Mycotaxon 104, 399–404.
Heiniger, U., and Rigling, D. (1994). Biological control of chestnut blight in Europe. Ann. Rev. Phytopathol. 32, 581–599. doi: 10.1146/annurev.py.32.090194.003053
Herms, D. A., and McCullough, D. G. (2014). Emerald ash borer invasion of North America: history, biology, ecology, impacts, and management. Ann. Rev. Entomol. 59, 13–30. doi: 10.1146/annurev-ento-011613-162051
Hirata, A., Nakamura, K., Nakao, K., Kominami, Y., Tanaka, N., Ohashi, H., et al. (2017). Potential distribution of pine wilt disease under future climate change scenarios. PLoS ONE 12:e0182837. doi: 10.1371/journal.pone.0182837
Ideno, Y., Hayashi, K., Abe, Y., Ueda, K., Iso, H., Noda, M., et al. (2017). Blood pressure-lowering effect of shinrin-yoku (forest bathing): a systematic review and meta-analysis. BMC Comple. Alter. Med. 17:12. doi: 10.1186/s12906-017-1912-z
Keith, L. M., Hughes, R. F., Sugiyama, L. S., Heller, W. P., Bushe, B. C., and Friday, J. B. (2015). First report of ceratocystis wilt on ‘ohi‘a (metrosideros polymorpha). Plant Dis. 99:1276. doi: 10.1094/PDIS-12-14-1293-PDN
Kenis, M., Hurley, B. P., Hajek, A. E., and Cock, M. J. W. (2017). Classical biological control of insect pests of trees: facts and figures. Biol. Invas. 19, 3401–3417. doi: 10.1007/s10530-017-1414-4
Lodge, D. M., Williams, S., Macisaac, H. J., Hayes, K. R., Leung, B., Reichard, S., et al. (2006). Biological invasions: recommendations for U.S. Pol. Manage. Ecol. Appl. 16, 2035–2054. doi: 10.1890/1051-0761(2006)016[2035:BIRFUP]2.0.CO;2
Meissner, H., Lemay, A., Bertone, C., Schwartzburg, K., Ferguson, L., and Newton, L. (2009). Evaluation of Pathways for Exotic Plant Pest Movement into and within the Greater Caribbean Region. Raleigh, NC: Caribbean Invasive Species Working Group (CISWG) and Plant Epidemiology and Risk Analysis Laboratory (PERAL), Center for Plant Health Science and Technology (CPHST), United States Department of Agriculture (USDA), 267.
Meurisse, N., Rassati, D., Hurley, B. P., Brockerhoff, E. G., and Haack, R. A. (2019). Common pathways by which non-native forest insects move internationally and domestically. J. Pest Sci. 92, 13–27. doi: 10.1007/s10340-018-0990-0
Mitchell, R. J., Beaton, J. K., Bellamy, P. E., Broome, A., Chetcuti, J., Eaton, S., et al. (2014). Ash dieback in the UK: a review of the ecological and conservation implications and potential management options. Biol. Conserv. 175, 95–109. doi: 10.1016/j.biocon.2014.04.019
National Academies of Sciences, Engineering, and Medicine. (2019). Forest Health and Biotechnology: Possibilities and Considerations. Washington, DC.
Ricciardi, A., Palmer, M. E., and Yan, N. D. (2011). Should biological invasions be managed as natural disasters? Bioscience 61, 312–317. doi: 10.1525/bio.2011.61.4.11
Rizzo, D. M., and Garbelotto, M. (2003). Sudden oak death: endangering California and oregon forest ecosystems. Front. Ecol. Environ. 1, 197–204. doi: 10.1890/1540-9295(2003)001[0197:SODECA]2.0.CO;2
Santini, A., and Faccoli, M. (2015). Dutch elm disease and elm bark beetles: a century of association. Ifor. Biogeosci. For. 8, 126–134. doi: 10.3832/ifor1231-008
Seebens, H., Blackburn, T. M., Dyer, E. E., Genovesi, P., Hulme, P. E., Jeschke, J. M., et al. (2017). No saturation in the accumulation of alien species worldwide. Nat. Commun. 8:14435. doi: 10.1038/ncomms14435
Showalter, D. N., Raffa, K. F., Sniezko, R. A., Herms, D. A., Liebhold, A. M., Smith, J. A., et al. (2018). Strategic development of tree resistance against forest pathogen and insect invasions in defense-free space. Front. Ecol. Evol. 6:124. doi: 10.3389/fevo.2018.00124
Sniezko, R., Johnson, J., and Savin, D. (2019). Assessing the durability, stability, and usability of genetic resistance to a non-native fungal pathogen in two pine species. Plants People Planet 2, 57–68. doi: 10.1002/ppp3.49
Sniezko, R. A., and Koch, J. (2017). Breeding trees resistant to insects and diseases: putting theory into application. Biol. Invas. 19, 3377–3400. doi: 10.1007/s10530-017-1482-5
Stukely, M. J. C., Crane, C. E., McComb, J. A., and Bennett, I. J. (2007). Field survival and growth of clonal, micropropagated Eucalyptus marginata selected for resistance to Phytophthora cinnamomi. For. Ecol. Manage. 238, 330–334. doi: 10.1016/j.foreco.2006.10.028
Sun, J., Lu, M., Gillette, N. E., and Wingfield, M. J. (2013). Red turpentine beetle: innocuous native becomes invasive tree killer in China. Ann. Rev. Entomol. 58, 293–311. doi: 10.1146/annurev-ento-120811-153624
Thakur, M. P., Van Der Putten, W. H., Cobben, M. M. P., Van Kleunen, M., and Geisen, S. (2019). Microbial invasions in terrestrial ecosystems. Nat. Rev. Microbiol. 17, 621–631. doi: 10.1038/s41579-019-0236-z
Tobin, P. C., Kean, J. M., Suckling, D. M., McCullough, D. G., Herms, D. A., and Stringer, L. D. (2014). Determinants of successful arthropod eradication programs. Biol. Invas. 16, 401–414. doi: 10.1007/s10530-013-0529-5
Keywords: tree pathogens, insect pests, invasions, forest ecosystems, tree resistance
Citation: Bonello P, Campbell FT, Cipollini D, Conrad AO, Farinas C, Gandhi KJK, Hain FP, Parry D, Showalter DN, Villari C and Wallin KF (2020) Invasive Tree Pests Devastate Ecosystems—A Proposed New Response Framework. Front. For. Glob. Change 3:2. doi: 10.3389/ffgc.2020.00002
Received: 05 November 2019; Accepted: 09 January 2020;
Published: 29 January 2020.
Edited by:
Treena Burgess, Murdoch University, AustraliaReviewed by:
Trudy Paap, University of Pretoria, South AfricaJeff Garnas, University of New Hampshire, United States
Alberto Santini, Italian National Research Council, Italy
Copyright © 2020 Bonello, Campbell, Cipollini, Conrad, Farinas, Gandhi, Hain, Parry, Showalter, Villari and Wallin. This is an open-access article distributed under the terms of the Creative Commons Attribution License (CC BY). The use, distribution or reproduction in other forums is permitted, provided the original author(s) and the copyright owner(s) are credited and that the original publication in this journal is cited, in accordance with accepted academic practice. No use, distribution or reproduction is permitted which does not comply with these terms.
*Correspondence: Pierluigi Bonello, Ym9uZWxsby4yJiN4MDAwNDA7b3N1LmVkdQ==