- 1The Earth Institute, Columbia University, New York, NY, United States
- 2The Nature Conservancy, Arlington, VA, United States
- 3Ecology, Evolution, and Environmental Biology, Columbia University, New York, NY, United States
- 4Nature Conservation Foundation, Mysore, India
- 5Department of Biosciences, University of Exeter, Exeter, United Kingdom
The mammalian and avian assemblages of intact tropical forests are among the most diverse vertebrate communities on Earth and influence the structure, composition, and functioning of these forests in myriad ways. Over recent centuries, however, increasing human domination of the tropics has led to widespread defaunation, or the decline, local-, or global extinction of tropical animal species. Defaunation is one of the defining features of the Anthropocene and is best documented for vertebrate species, especially mammals and birds. Defaunation is driven by several direct (e.g., hunting) and indirect (e.g., habitat alteration) anthropogenic threats, but how these threats differ in the nature and magnitude of their impacts on tropical mammal and bird species remains unclear. Using a meta-analysis of 82 studies on 254 mammal and 1,640 bird species from across the tropics, we assess the effects of three major regional-scale drivers of tropical defaunation, namely hunting, forest degradation and forest conversion, on measures of abundance for tropical mammal and bird species belonging to different dietary guilds and IUCN conservation status groups. Mammal species across dietary guilds either declined or did not change, on average, in response to the three drivers, with hunting having the most consistent negative impacts on abundances of carnivores, frugivores, herbivores/granivores, large-bodied species, and species of high conservation importance. By contrast, bird species declined most strongly in response to forest conversion, with responses varying widely across different dietary and conservation importance groups, and not consistently related to body size. Our results reveal that hunting, forest degradation and conversion are associated with distinct types of defaunation of mammal and bird species, and are therefore likely to have distinct implications for animal-mediated interactions and processes, ecosystem functions, and conservation of tropical forests. Addressing major gaps in our empirical understanding of defaunation effects—e.g., hunting impacts on smaller-bodied mammals and birds, and responses of species in southeast Asian forests—is key to better understanding, predicting, and mitigating the impacts of this pervasive global threat.
Introduction
Tropical forests are among the most biodiverse global biomes and harbor c. 50–70% of all terrestrial vertebrate diversity (Whitmore, 1990; Pimm and Raven, 2000). The rapidly expanding footprint of anthropogenic disturbance is a leading driver of species population declines, resulting in widespread losses of tropical biodiversity (Gibson et al., 2011; Lewis et al., 2015; Watson et al., 2018). Such anthropogenic biodiversity loss, termed defaunation when focusing on animal species, is pervasive among all biomes, terrestrial, freshwater, or marine, and is one of the defining features of this epoch, commonly referred to as the Anthropocene (Dirzo et al., 2014; Young et al., 2016).
There are numerous drivers of Anthropocene defaunation, operating across a variety of scales, ranging from global (e.g., climate change, environmental pollution) to local or regional (e.g., direct harvest) scales (Young et al., 2016). Major regional-scale defaunation drivers include direct (e.g., hunting), and indirect (e.g., habitat degradation), threats (Young et al., 2016). Direct harvest for commercial and subsistence hunting is prevalent in most tropical forest regions (Fa et al., 2002; Peres and Palacios, 2007), including within protected reserves (Harrison, 2011; Laurance et al., 2012). In some regions such as Southeast Asia, hunting is considered the predominant threat to vertebrates including birds and mammals (Harrison et al., 2016). Indirect drivers of faunal change in tropical forests include forest degradation (e.g., fragmentation and unsustainable logging) (Potapov et al., 2008; Malhi et al., 2014), and conversion of forests to plantations, cropland and pastures (Gibson et al., 2011; Young et al., 2016), which affect virtually all tropical landscapes.
Because hunting, forest degradation, and forest conversion are very different types of disturbances, the nature and magnitude of their impacts on different mammal and bird species would be expected to vary. For example, species' responses to hunting depend strongly on the traits preferred by hunters, such as large body size, or herbivorous diet (Fa et al., 2002; Fa and Brown, 2009; Benítez-López et al., 2017). By contrast, forest degradation and conversion drive changes in animal communities by altering resource availability for different dietary guilds (Gray et al., 2007), or by constraining dispersal across degraded or modified landscapes (Şekercioḡlu et al., 2002). Understanding variation in the nature of trait-mediated animal community change is useful not only for assessing conservation threats posed by different defaunation drivers, but can also improve our ability to predict knock-on impacts on forest vegetation dynamics and ecosystem functions such as carbon sequestration (Osuri et al., 2016; Berzaghi et al., 2018). However, even as previous studies have examined faunal responses to individual drivers such as hunting (Benítez-López et al., 2017) or selective logging (Burivalova et al., 2014), or responses of particular species groups such as frugivores to multiple drivers (McConkey et al., 2012), variation in the responses of tropical forest faunal communities to different defaunation drivers has not been systematically assessed.
In this paper, we present a pan-tropical meta-analysis of mammal and bird species responses to three major regional-scale drivers of tropical defaunation, namely hunting, forest degradation and forest conversion (Young et al., 2016). We ask how abundance or relative abundance metrics of different dietary guilds (e.g., carnivores, frugivores) of mammal and bird species vary in response to these drivers, and whether the strength of these responses are influenced by body size.
Materials and Methods
Datasets
Data on the responses of bird and non-volant mammal species to hunting, forest degradation and forest conversion were extracted from published literature and databases. For hunting, a list of potential data sources was derived from a recent pan-tropical meta-analysis of the effects of hunting and distance from human settlements on abundances of mammal and bird species (Benítez-López et al., 2017). We examined all the publications included in the above meta-analysis and retained those studies that were in English, available online, and which explicitly compared and reported metrics of abundance or relative abundance for at least one mammal or bird species from hunted forests and forests experiencing little to no hunting.
For forest degradation and conversion, we extracted data from the PREDICTS database (2016 release) of species responses to anthropogenic habitat disturbance (Hudson et al., 2017). Studies from tropical forests reporting metrics of abundance or relative abundance for at least one bird or mammal species from relatively undisturbed habitats (Predominant_land_use = “Primary vegetation”) and at least one disturbed (Predominant_land_use = “Secondary vegetation”) or converted (Predominant_land_use = “Plantation forest” OR “Pasture” OR “Cropland”) habitat were retained for analysis. The “Secondary vegetation” class included selectively logged forests, forest fragments, and secondary forests growing on shifting or abandoned agriculture.
We extracted the geographic coordinates of each study site and retained only those studies situated within the tropical forest biome as defined by Olson et al. (2001). Next, we reviewed study area and design descriptions of each study to characterize sole or primary disturbances as one of hunting, forest degradation or forest conversion. Studies assessing single disturbance types [e.g., undisturbed vs. hunted forests—Endo et al. (2010)], and studies assessing multiple disturbances separately [undisturbed vs. degraded vs. converted forest—Barlow et al. (2007)], were retained, whereas studies looking at combined impacts of multiple disturbances [e.g., undisturbed vs. hunted + logged forest—Marshall et al. (2006)] were excluded from subsequent analyses.
Our final dataset comprised 117 comparisons (e.g., species abundances in hunted vs. non-hunted forests) in total (from 82 publications), including 60 comparisons based on hunting, 27 on forest degradation, and 30 based on forest conversion, with 37% of the publications reporting more than one type of comparison (Figure 1; Table S1). The dataset spanned 254 mammal and 1,640 bird species belonging to 17 and 28 taxonomic orders, respectively (Table 1). We detected a bias among studies on hunting, which mostly reported responses of species targeted by hunters (e.g., Lwanga, 2006), while responses of non-target species were less frequently reported (e.g., Carrillo et al., 2000), resulting in a skew toward larger-bodied species in hunting studies relative to studies on forest degradation and conversion in our dataset (Figures 1B,C). We explore the implications of this bias in our Discussion.
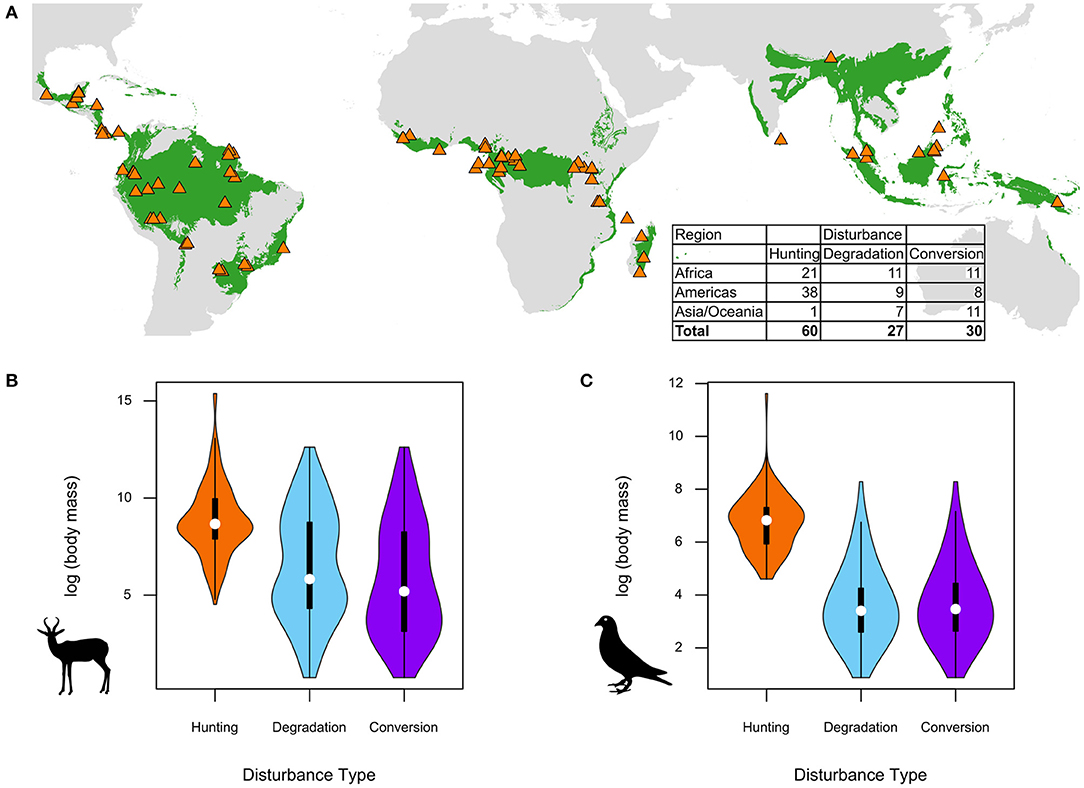
Figure 1. (A) Locations of 82 studies from different parts of the tropical forest biome (green) included in the meta-analysis. The distribution of body sizes of (B) mammal and (C) bird species included in the meta-analysis across different defaunation drivers.
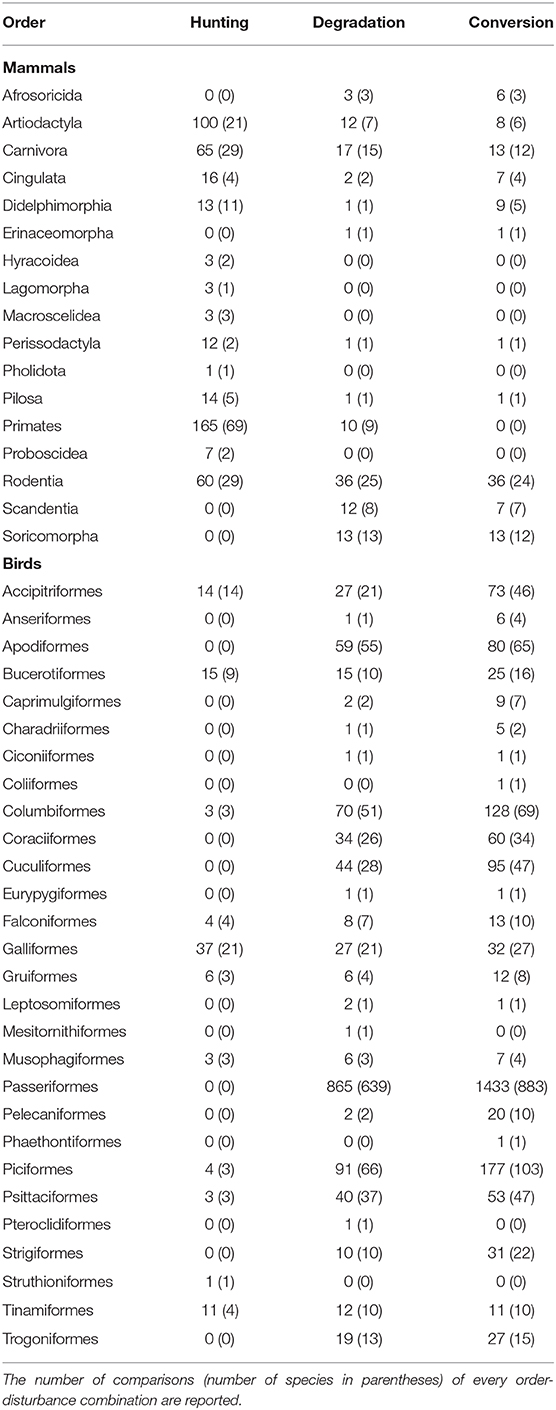
Table 1. The representation of different mammal and bird orders across different disturbance types included in the meta-analysis.
The above dataset was transformed into a species response table that comprised, for every species in each comparison, information on disturbance type, average abundance or relative abundance in undisturbed (control) and disturbed (treatment) habitats. Average body mass (g) and broad dietary guilds of the species were extracted from the EltonTraits 1.0 database (Wilman et al., 2014). For dietary guild, species were assigned one of the following diet classes based on which of those classes represented 40% or more of their known dietary affinity—Carnivore, Frugivore, Herbivore (including Granivore), Insectivore, Nectarivore, and Generalist (including Omnivore). The aggregation and handling of species with ≥40% affinity to more than one class is described in Table S2, and body size characteristics of species in different diet classes are described in Table S3. Information on species' conservation threat status were obtained from the International Union for Conservation of Nature's (IUCN) Red List of Threatened Species (https://www.iucnredlist.org/).
Analyses
The log response ratio (LRR) between observed abundances, densities, relative abundances or encounter rates of species in disturbed and undisturbed treatments was used as a response variable [LRR = ln(disturbed/undisturbed)]. The processing of data to avoid artifacts in the LRR due to log transformation of zeroes is described in the Supplementary Methods section of the Supplementary Material. Analyses were run separately for non-volant mammals and birds using linear mixed-effects models (lme4 package: Bates et al., 2014) in the R statistical and programming environment (version 3.4.4) (R Core Team, 2017). The three-way interaction between disturbance type (hunting, degradation, and conversion), feeding guild (carnivore, frugivore, herbivore, insectivore, generalist, and nectarivore), and species body mass (log transformed) was used as the independent predictor. A unique identifier for study was used as a random factor. Pseudo-replication due to multiple occurrences of the same species across studies was accounted for by including species identity as a second random term.
Phylogenetic similarity between species could be a source of bias when responses of multiple species are aggregated at different treatment levels. This bias stems from the possibility of closely related species, which have similar evolutionary histories, responding in a more consistent manner than those that are more distantly related. To check if phylogenetic similarity influences parameter estimates and differences detected in our analyses, we compared results from the mixed model described above with a separate model, where the species random term was nested within order. Models with and without the nested species-order random term were compared using model BICs and the amount of variation attributed to the random term (following Benítez-López et al., 2017). The premise of this comparison is that if phylogenetic similarity is an important determinant of observed differences in the response variable, it would be reflected in relatively lower BICs (i.e., better model fit) of the model with the nested species-order random term, as well as greater variance associated with the random term. Our analyses showed that the nested random term model did not account for more variation and was a poorer fit to the data relative to the non-nested model (based on model BICs) for both mammals and birds. Hence, we concluded that phylogenetic similarity did not influence the analyses, and we report and interpret results from mixed models with the non-nested random term structure (i.e., where random terms were study identifier and species identity).
A linear mixed-effects model was also used to assess the consistency of effects across species when grouped by IUCN threat status. To ensure adequate sample sizes (numbers of species) in each IUCN threat category, we reclassified threat status into three levels—Critically endangered and Endangered (CR-EN), Vulnerable and Near Threatened (VU-NT), and Least Concern (LC). Log response ratios were modeled as a function of the two-way interaction between disturbance type and our reclassified IUCN threat category. Again, study identity and species identity were used as separate random factors.
We interpreted our results based on modeled effect sizes, their associated 95% confidence intervals (CIs) and biological relevance, rather than relying on p-values. Our inferences were categorized into three classes—(1) where modeled effects were large and CIs do not span zero (directional and consistent effects); (2) where effects were ≥10% change, but CIs span zero (directional, but inconsistent effects); and (3) where effects were <10% change with CIs spanning zero (no effects). The basis for the second interpretation class is that for effects having 95% CIs that span zero, there is as much support for twice the estimated effect (counternull) as there is for no effect (Rosenthal and Rubin, 1994; Stephens et al., 2007), which can be biologically meaningful when average effect sizes are large (e.g., ≥10% in our definition).
Results
Mammal and bird species' abundances varied in response to hunting, forest degradation, and conversion. Mammal species abundances were consistently lower (−49%; LRR mean = −0.67; LRR 95% CI = −0.97 to −0.38) in hunted forests than in forests with little to no hunting (Figure 2A). Forest degradation and conversion also reduced mammal species' abundances by 16% and 27%, respectively, on average, but with 95% CIs overlapping zero, their overall effects on mammals were less consistent, relative to hunting (Figure 2A). For birds, by contrast, hunting and forest degradation were associated with increasing but highly variable overall species' abundance responses of 32% and 16%, respectively, while bird species declined consistently by over 53% in response to forest conversion (LRR mean = −0.76; LRR 95% CI = −1.27 to −0.25; Figure 2B). The full set of model estimated parameters and 95% CIs is provided in Table S4.
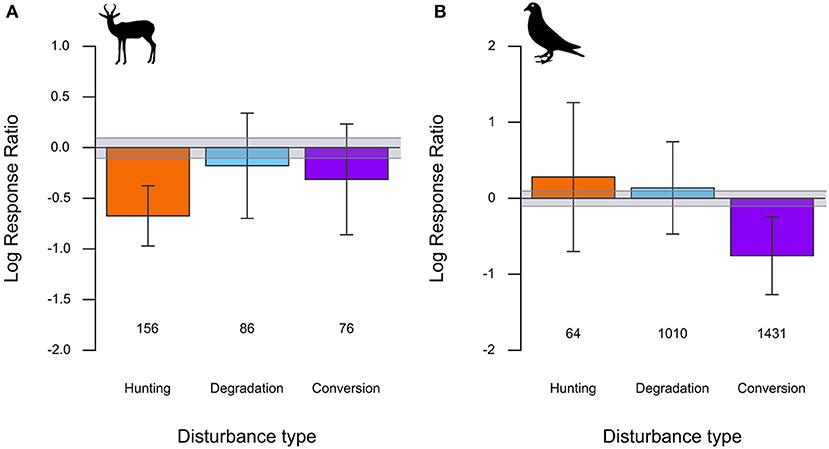
Figure 2. Estimated average effect sizes and 95% CIs from linear mixed-effects models of (A) mammal and (B) bird species Log Response Ratios (LRRs) in comparisons of hunted, degraded, and converted forests to relatively undisturbed forests. Numbers below each bar represent the number of species of that particular category included in the analysis. The gray band depicts a ≤10% difference in species' abundance between disturbed and undisturbed habitats.
Mammal species responses to hunting were negative on average across all major dietary groups, with declines being relatively more consistent among carnivores, frugivores, and herbivores than insectivore and diet-generalist species (Figure 3A). The responses of different mammalian diet groups to forest degradation and conversion were more variable, ranging from negative but inconsistent responses of carnivores and frugivores to positive but inconsistent responses of insectivores (Figure 3A). Among birds, forest conversion was associated with consistent declines of frugivores and insectivores and increases of herbivores/granivores and nectarivores, and inconsistent declines of insectivores, while guild-wise responses to hunting and forest degradation were weaker and more variable (Figure 3B). The full set of model estimated parameters and 95% CIs is provided in Table S4.
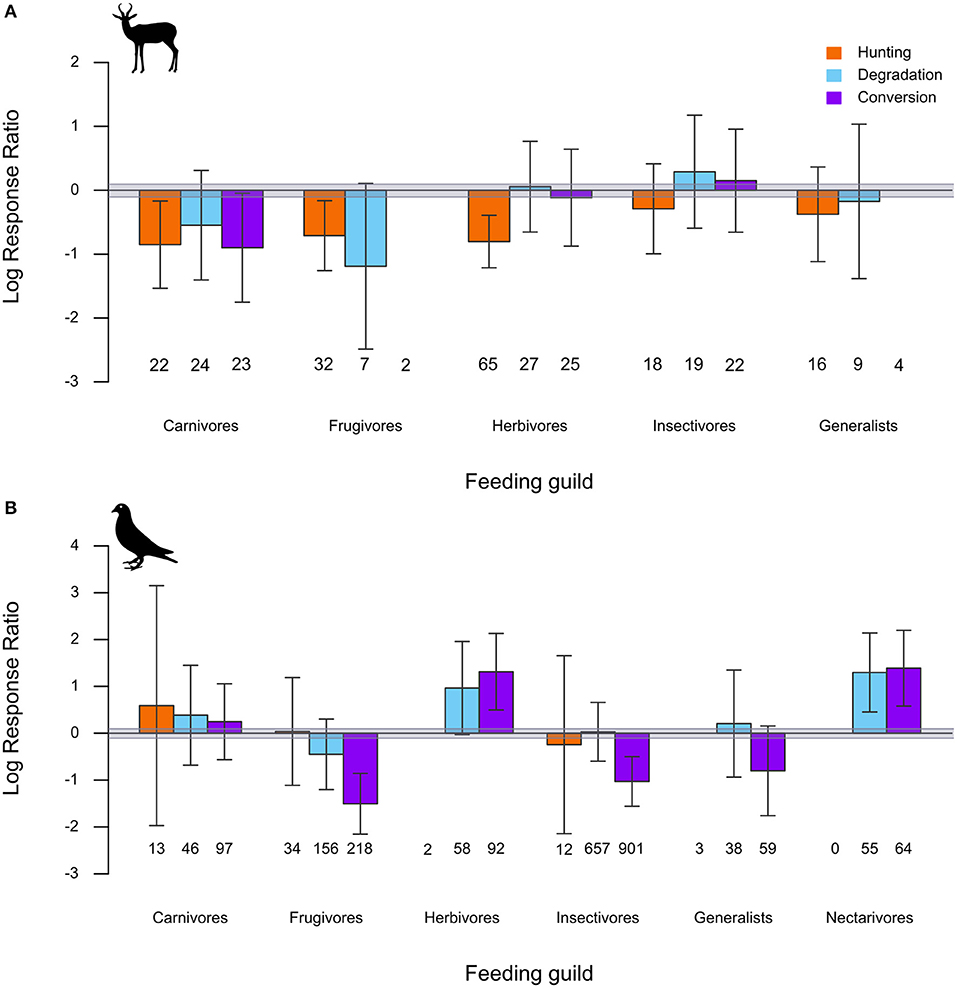
Figure 3. Estimated average effect sizes and 95% CIs from linear mixed-effects models of (A) mammal and (B) bird species guild-wise LRRs in comparisons of hunted, degraded, and converted forests to relatively undisturbed forests. The “Herbivores” category also includes granivores. Numbers below each bar represent the number of species of that particular category included in the analysis. Categories comprising fewer than five species in the dataset (e.g., Frugivore mammals in Forest conversion comparisons; Nectarivore mammals) are not depicted. The gray band depicts a ≤10% difference in species' abundance between disturbed and undisturbed habitats.
The effect of hunting on mammals was consistently more negative for larger-bodied than smaller-bodied species (LRR vs. Log body mass slope = −0.34; Slope 95% CI = −0.46 to −0.21), particularly among carnivores (Slope = −0.4; Slope 95% CI = −0.81 to 0) and frugivores, (Slope = −0.64; Slope 95% CI = −1.29 to 0.01) and less consistently for herbivores, insectivores, and generalists/omnivores (Figure 4A). By contrast, mammal species' responses to forest degradation and conversion were not consistently related to body size overall, with responses among guilds ranging from negative but inconsistent LRR-body size relationships (Insectivore-Degradation) to positive but inconsistent relationships (Frugivore-Degradation) (Figure 4A). Among birds, body size was either unrelated, or weakly negatively related, to species' responses across disturbance types and across most dietary guilds, with the exception of herbivore/granivore and carnivore species which showed a negative but inconsistent LRR-body size relationship under the hunting category (Figure 4B). The full set of model estimated slope parameters and 95% CIs is provided in Table S5.
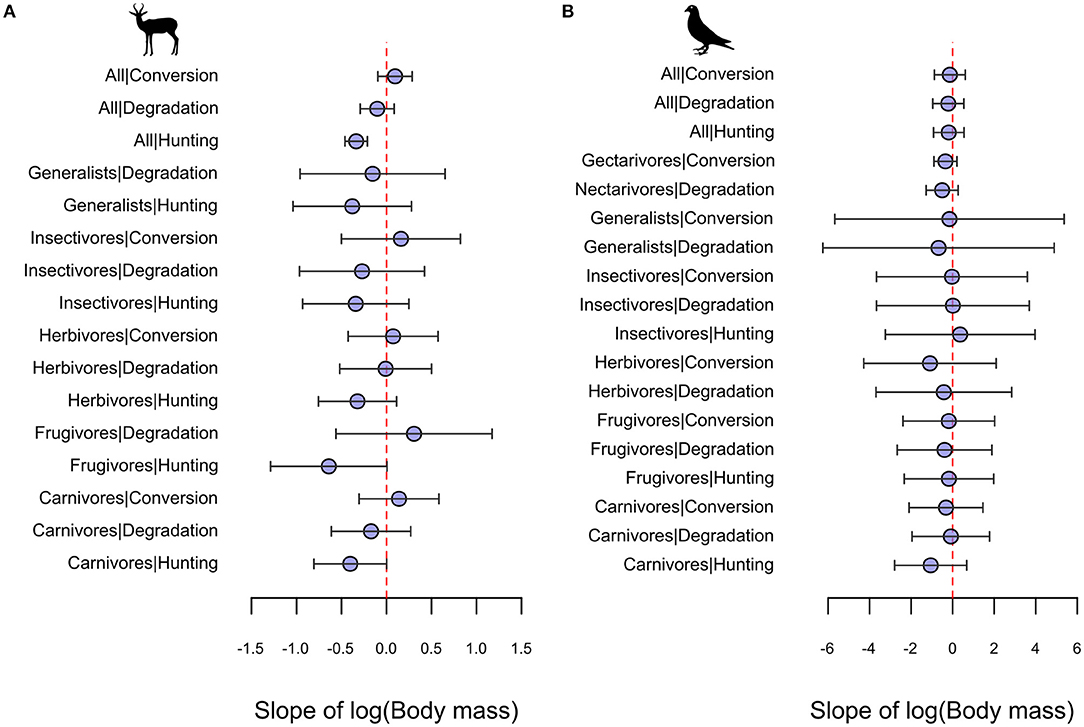
Figure 4. Estimated slopes and 95% CIs of LRR-body size relationships from linear mixed-effects models for (A) mammal and (B) bird species across different diet guild|disturbance type combinations. Categories comprising fewer than five species in the dataset (e.g., Frugivore mammals in Forest conversion comparisons; Nectarivore mammals) are not depicted.
Declines of mammal species in hunted forests were consistent across conservation threat status categories. Forest conversion was associated with consistent declines of VU and NT species, but inconsistent declines in LC species (Figure 5A). Mammal species responses to forest degradation did not show a consistent relationship with any conservation threat category (Figure 5A). By contrast, forest degradation and conversion were consistently associated with large declines of threatened bird species (CR, EN, VU, NT). LC species experienced smaller consistent declines due to forest conversion, but positive if inconsistent effects of hunting and forest degradation (Figure 5B). The full set of model estimated parameters and 95% CIs is provided in Table S6.
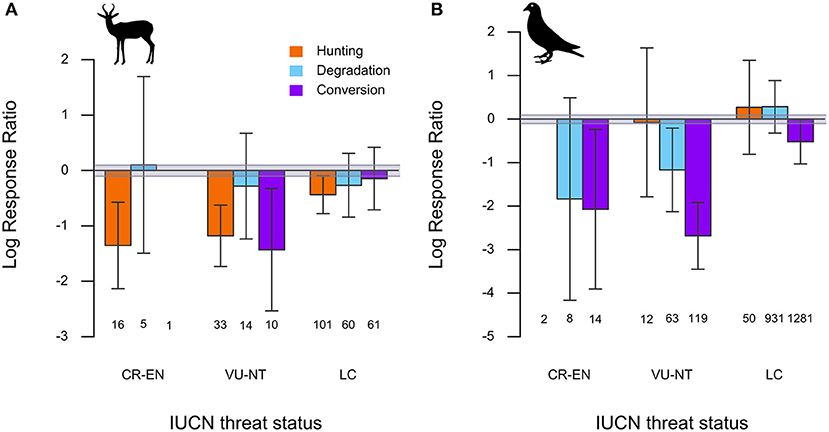
Figure 5. Estimated average effect sizes and 95% CIs from linear mixed-effects models of (A) mammal and (B) bird species LRRs of different IUCN threat status groups in comparisons of hunted, degraded, and converted forests to relatively undisturbed forests. Groups include Critically Endangered and Endangered (CR-EN), Vulnerable and Near Threatened (VU-NT) and Least Concern (LC). Numbers below each bar represent the number of species of that particular category included in the analysis. Categories comprising fewer than five species in the dataset (CR-EN mammals in Forest conversion comparisons) are not depicted. The gray band depicts a ≤10% difference in species' abundance between disturbed and undisturbed habitats.
Discussion
Our findings suggest that hunting, forest degradation and conversion have marked but varied defaunation impacts on mammal and bird communities of relatively intact tropical forests. For mammal abundances, all three disturbance types exerted a negative effect, while the response in birds was more mixed. Hunting had stronger negative impacts than forest degradation or conversion on mammal abundances across all species, with declines evident across the spectrum of high to low conservation priority species in hunted forests. However, given that species groups targeted by hunters (e.g., primates) were overrepresented in our hunting dataset, actual impacts of hunting at the level of mammal communities across targeted and non-targeted groups (e.g., small insectivores) may be weaker, and potentially differ less overall from the impacts of forest degradation or conversion. By contrast, forest conversion was most strongly associated with declines in bird species' abundances (high and low conservation priority species), while hunting—despite underrepresentation of non-targeted species (e.g., passerines)—and forest degradation were associated with weak increases in bird species' abundance, driven by the positive responses of low conservation priority (according to the IUCN) species in hunted and degraded forests. These findings suggest that while interventions aimed at reducing hunting pressure may be important for conserving mammals, incentive schemes for reducing forest degradation and promoting wildlife friendly farming might be equally if not more important for averting declines of bird species in the tropics.
The effects of hunting, forest degradation, and conversion on birds and mammals differed with respect to species' body sizes and dietary guilds. Mammal species across all dietary guilds showed strong (in carnivores, frugivores, and herbivores) to weak (insectivores and generalists) reductions in abundance in hunted forests, while responses to forest degradation and conversion were more variable, ranging from strong declines (carnivores in converted habitats) to weak declines (carnivores in degraded forests) and increases (insectivores in degraded and converted forests). Hunting also had stronger negative impacts on large-bodied than smaller-bodied mammal species, possibly reflecting hunters' preference for larger wildlife (Fa et al., 2002; Young et al., 2016), while the impacts of forest degradation and conversion on mammals were less consistently related to body size. Among birds, while hunting impacts on species' abundance ranged from weakly positive (carnivores) to weakly negative (insectivores) and unknown (herbivores/granivores, generalists and nectarivores, due to a lack of data) across dietary guilds, responses to forest degradation and conversion were stronger in both directions across different guilds. For example, frugivores (conversion and degradation) and insectivores (conversion) showed marked declines in converted habitats, possibly due to reductions in food resources in degraded and non-forest habitats relative to intact forests (Gray et al., 2007; Naniwadekar et al., 2015), or due to the inability of certain species (e.g., understorey insectivores) to disperse across non-forest habitats (Şekercioḡlu et al., 2002). Likewise, increases in the abundances of herbivore/granivore and nectarivore species in degraded and converted habitats relative to intact forests are possibly related to greater food availability in modified habitats [e.g., flowering trees in coffee plantations—Faria et al. (2006)], and a greater ability of birds in these guilds to disperse across open or disturbed habitats (Lees and Peres, 2009; Şekercioḡlu, 2012). Collectively, notwithstanding gaps in current literature and understanding of hunting impacts on smaller-bodied species, our results suggest that defaunation drivers can vary in their impacts on, and result in communities comprising distinct combinations of, dietary guild, size class and conservation status groups of tropical forest mammals and birds.
A central question of both academic and applied relevance pertaining to the Anthropocene defaunation is of how the consequent losses of animal-mediated interactions and processes modify the structure, composition and functioning of the tropical forest ecosystem (Muller-Landau, 2007; Wright et al., 2007; Osuri et al., 2016). Our findings on the contrasting responses of different diet and body-size species groups to hunting, forest degradation and conversion suggest that such knock-on effects of defaunation on ecosystem processes, functions, and services might also vary across defaunation drivers. For example, our results suggest that while abundances of frugivores are reduced by both hunting (mammals) and forest modification (mammals and birds), mammalian herbivores and granivores decline more strongly in hunted than in modified forests (Figure 3). Seed dispersal by frugivores, and seed, seedling, and sapling predation by granivores and herbivores, are known to influence the regeneration of tropical trees (Wright, 2003; Paine et al., 2016; Terborgh et al., 2018). Thus, variation in the response of these guilds to different defaunation drivers could drive distinct trajectories of tree community composition, and consequently, result in distinct outcomes in terms of key ecosystem functions such as carbon storage (Culot et al., 2017). Given the long time periods—typically decades to centuries—that shifts in animal-mediated interactions and processes might take to manifest at the ecosystem level, vegetation-, and other types of models have emerged as an important tool for understanding and attempting to predict longer term ecosystem-level consequences of defaunation (Berzaghi et al., 2018; Schmitz et al., 2018). Our results suggest that incorporating disturbance-specific defaunation parameters and scenarios could improve the ability of such models to distinguish the ecosystem-level impacts of different drivers of faunal loss.
While our meta-analysis uncovered broad patterns of variation in defaunation impacts across different anthropogenic drivers, empirical studies are needed in order to overcome existing biases in the literature and thereby to quantify the differences in faunal responses to different drivers more accurately. For example, most studies on hunting do not report community-wide responses but rather focus on particular target and non-target species, while studies on forest degradation and conversion frequently report community-wide responses. For this reason, differences between disturbance types on mammals should be interpreted cautiously, particularly for carnivore, frugivore, and herbivore guilds, which show size-dependent responses to disturbance. For birds, on the other hand, it is worth noting that forest conversion and degradation show stronger and more consistent effects than hunting in spite of the potential bias. Such biases could be overcome by future studies that assess community-wide faunal responses using consistent methodologies across disturbance types. A number of biases also exist in the geographic coverage of studies of faunal responses to different defaunation drivers—most notably, empirical studies on the effects of hunting are highly underrepresented in Southeast Asia, even as hunting is recognized as a major threat to mammals and birds in this region (Harrison et al., 2016).
Collectively, our findings highlight that the major regional-scale anthropogenic threats to intact tropical forests are associated with distinct types of defaunation, in terms of their impacts on different functional and conservation status groups of mammal and bird species, and consequently, in terms of their potential impacts on forest ecosystem functions and services. Other distinct combinations of species and ecosystem responses could potentially arise from interactions between disturbance types, such as logging and hunting, which frequently co-occur in the tropics (Wilkie et al., 2000; Peres, 2001; Laurance and Useche, 2009), and from interactions of regional drivers with global drivers such as climate change (Young et al., 2016). Incorporating this heterogeneity in species' and community responses to different drivers into defaunation theory and models, and efforts to address key gaps in species and geographic coverage, are important for better understanding, predicting and mitigating the pervasive declines of mammals and bird species, and their cascading impacts on the tropical forest ecosystem.
Data Availability Statement
Data were accessed from published articles and publicly available datasets (see References, Table S1, and Supplementary References). Derived datasets and R scripts used for analysis and graphics are available on request from the authors.
Author Contributions
AO, UM, RN, and VV designed the study, performed literature searches and collated the data, with inputs from SN. AO and VV performed the analysis with inputs from all authors. All authors contributed to writing the manuscript.
Funding
AO was supported by a fellowship from the NatureNet Science Fellows and Earth Institute Fellows programs during the study.
Conflict of Interest
The authors declare that the research was conducted in the absence of any commercial or financial relationships that could be construed as a potential conflict of interest.
Acknowledgments
We thank the editors of this special issue for inviting our contribution. We are grateful to the authors of the various publications and datasets from which data were extracted for our analysis.
Supplementary Material
The Supplementary Material for this article can be found online at: https://www.frontiersin.org/articles/10.3389/ffgc.2019.00087/full#supplementary-material
References
Barlow, J., Mestre, L. A. M., Gardner, T. A., and Peres, C. A. (2007). The value of primary, secondary and plantation forests for Amazonian birds. Biol. Conserv. 136, 212–231. doi: 10.1016/j.biocon.2006.11.021
Bates, D., Mächler, M., Bolker, B., and Walker, S. (2014). Fitting linear mixed-effects models using lme4. J. Stat. Softw. 1406:5823. doi: 10.18637/jss.v067.i01
Benítez-López, A., Alkemade, R., Schipper, A. M., Ingram, D. J., Verweij, P. A., Eikelboom, J. A. J., et al. (2017). The impact of hunting on tropical mammal and bird populations. Science 356, 180–183. doi: 10.1126/science.aaj1891
Berzaghi, F., Verbeeck, H., Nielsen, M. R., Doughty, C. E., Bretagnolle, F., Marchetti, M., et al. (2018). Assessing the role of megafauna in tropical forest ecosystems and biogeochemical cycles – the potential of vegetation models. Ecography 41, 1934–1954. doi: 10.1111/ecog.03309
Burivalova, Z., Sekercioglu, Çagan, H., and Koh, P. L. (2014). Thresholds of logging intensity to maintain tropical forest biodiversity. Curr. Biol. 24, 1893–1898. doi: 10.1016/j.cub.2014.06.065
Carrillo, E., Wong, G., and Cuarón, A. D. (2000). Monitoring mammal populations in costa rican protected areas under different hunting restrictions. Conserv. Biol. 14, 1580–1591. doi: 10.1111/j.1523-1739.2000.99103.x
Culot, L., Bello, C., Batista, J. L. F., do Couto, H. T. Z., and Galetti, M. (2017). Synergistic effects of seed disperser and predator loss on recruitment success and long-term consequences for carbon stocks in tropical rainforests. Sci. Rep. 7:7662. doi: 10.1038/s41598-017-08222-4
Dirzo, R., Young, H. S., Galetti, M., Ceballos, G., Isaac, N. J. B., and Collen, B. (2014). Defaunation in the anthropocene. Science 345, 401–406. doi: 10.1126/science.1251817
Endo, W., Peres, C. A., Salas, E., Mori, S., Sanchez-Vega, J.-L., Shepard, G. H., et al. (2010). Game vertebrate densities in hunted and nonhunted forest sites in manu national park, Peru. Biotropica 42, 251–261. doi: 10.1111/j.1744-7429.2009.00546.x
Fa, J. E., and Brown, D. (2009). Impacts of hunting on mammals in African tropical moist forests: a review and synthesis. Mamm. Rev. 39, 231–264. doi: 10.1111/j.1365-2907.2009.00149.x
Fa, J. E., Peres, C. A., and Meeuwig, J. (2002). Bushmeat exploitation in tropical forests: an intercontinental comparison. Conserv. Biol. 16, 232–237. doi: 10.1046/j.1523-1739.2002.00275.x
Faria, D., Laps, R. R., Baumgarten, J., and Cetra, M. (2006). Bat and bird assemblages from forests and shade cacao plantations in two contrasting landscapes in the Atlantic forest of southern Bahia, Brazil. Biodiver. Conserv. 15, 587–612. doi: 10.1007/s10531-005-2089-1
Gibson, L., Lee, T. M., Koh, L. P., Brook, B. W., Gardner, T. A., Barlow, J., et al. (2011). Primary forests are irreplaceable for sustaining tropical biodiversity. Nature 478, 378–381. doi: 10.1038/nature10425
Gray, M. A., Baldauf, S. L., Mayhew, P. J., and Hill, J. K. (2007). The response of avian feeding guilds to tropical forest disturbance. Conserv. Biol. 21, 133–141. doi: 10.1111/j.1523-1739.2006.00557.x
Harrison, R. D. (2011). Emptying the forest: hunting and the extirpation of wildlife from tropical nature reserves. Bioscience 61, 919–924. doi: 10.1525/bio.2011.61.11.11
Harrison, R. D., Sreekar, R., Brodie, J. F., Brook, S., Luskin, M., O'Kelly, H., et al. (2016). Impacts of hunting on tropical forests in Southeast Asia. Conserv. Biol. 30, 972–981. doi: 10.1111/cobi.12785
Hudson, L. N., Newbold, T., Contu, S., Hill, S. L. L., Lysenko, I., De Palma, A., et al. (2017). The database of the PREDICTS (projecting responses of ecological diversity in changing terrestrial systems) project. Ecol. Evol. 7, 145–188. doi: 10.1002/ece3.2579
Laurance, W. F., Carolina Useche, D., Rendeiro, J., Kalka, M., Bradshaw, C. J. A., Sloan, S. P., et al. (2012). Averting biodiversity collapse in tropical forest protected areas. Nature 489:290. doi: 10.1038/nature11318
Laurance, W. F., and Useche, D. C. (2009). Environmental synergisms and extinctions of tropical species. Conserv. Biol. 23, 1427–1437. doi: 10.1111/j.1523-1739.2009.01336.x
Lees, A. C., and Peres, C. A. (2009). Gap-crossing movements predict species occupancy in amazonian forest fragments. Oikos 118, 280–290. doi: 10.1111/j.1600-0706.2008.16842.x
Lewis, S. L., Edwards, D. P., and Galbraith, D. (2015). Increasing human dominance of tropical forests. Science 349, 827–832. doi: 10.1126/science.aaa9932
Lwanga, J. S. (2006). The influence of forest variation and possible effects of poaching on duiker abundance at ngogo, kibale national park, Uganda. Afr. J. Ecol. 44, 209–218. doi: 10.1111/j.1365-2028.2006.00629.x
Malhi, Y., Gardner, T. A., Goldsmith, G. R., Silman, M. R., and Zelazowski, P. (2014). Tropical forests in the anthropocene. Annu. Rev. Environ. Resour. 39, 125–159. doi: 10.1146/annurev-environ-030713-155141
Marshall, A. J., Engström, L. M. N., Pamungkas, B., Palapa, J., Meijaard, E., et al. (2006). The blowgun is mightier than the chainsaw in determining population density of bornean orangutans (Pongo pygmaeus morio) in the forests of East Kalimantan. Biol. Conserv. 129, 566–578. doi: 10.1016/j.biocon.2005.11.025
McConkey, K. R., Prasad, S., Corlett, R. T., Campos-Arceiz, A., Brodie, J. F., Rogers, H., et al. (2012). Seed dispersal in changing landscapes. Biol. Conserv. 146, 1–13. doi: 10.1016/j.biocon.2011.09.018
Muller-Landau, H. C. (2007). Predicting the long-term effects of hunting on plant species composition and diversity in tropical forests. Biotropica 39, 372–384. doi: 10.1111/j.1744-7429.2007.00290.x
Naniwadekar, R., Shukla, U., Isvaran, K., and Datta, A. (2015). Reduced hornbill abundance associated with low seed arrival and altered recruitment in a hunted and logged tropical forest. PLoS ONE 10:e0120062. doi: 10.1371/journal.pone.0120062
Olson, D. M., Dinerstein, E., Wikramanayake, E. D., Burgess, N. D., Powell, G. V. N., Underwood, E. C., et al. (2001). Terrestrial ecoregions of the world: a new map of life on earth: a new global map of terrestrial ecoregions provides an innovative tool for conserving biodiversity. Bioscience 51, 933–938. doi: 10.1641/0006-3568(2001)051[0933:TEOTWA]2.0.CO;2
Osuri, A. M., Ratnam, J., Varma, V., Alvarez-Loayza, P., Hurtado Astaiza, J., Bradford, M., et al. (2016). Contrasting effects of defaunation on aboveground carbon storage across the global tropics. Nat. Commun. 7:11351. doi: 10.1038/ncomms11351
Paine, C. E. T., Beck, H., and Terborgh, J. (2016). How mammalian predation contributes to tropical tree community structure. Ecology 97, 3326–3336. doi: 10.1002/ecy.1586
Peres, C. A. (2001). Synergistic effects of subsistence hunting and habitat fragmentation on amazonian forest vertebrates. Conserv. Biol. 15, 1490–1505. doi: 10.1046/j.1523-1739.2001.01089.x
Peres, C. A., and Palacios, E. (2007). Basin-wide effects of game harvest on vertebrate population densities in amazonian forests: implications for animal-mediated seed dispersal. Biotropica 39, 304–315. doi: 10.1111/j.1744-7429.2007.00272.x
Potapov, P., Yaroshenko, A., Turubanova, S., Dubinin, M., Laestadius, L., Thies, C., et al. (2008). Mapping the world's intact forest landscapes by remote sensing. Ecol. Soc. 13:51. doi: 10.5751/ES-02670-130251
R Core Team (2017). R: A Language and Environment for Statistical Computing. Vienna: R Foundation for Statistical Computing. Available online at: https://www.R-project.org/ (accessed August 10, 2019).
Rosenthal, R., and Rubin, D. B. (1994). The counternull value of an effect size: a new statistic. Psychol. Sci. 5, 329–334. doi: 10.1111/j.1467-9280.1994.tb00281.x
Schmitz, O. J., Wilmers, C. C., Leroux, S. J., Doughty, C. E., Atwood, T. B., Galetti, M., et al. (2018). Animals and the zoogeochemistry of the carbon cycle. Science 362:eaar3213. doi: 10.1126/science.aar3213
Şekercioḡlu, Ç.H. (2012). Bird functional diversity and ecosystem services in tropical forests, agroforests and agricultural areas. J. Ornithol. 153, 153–161. doi: 10.1007/s10336-012-0869-4
Şekercioḡlu, Ç. H., Ehrlich, P. R., Daily, G. C., Aygen, D., Goehring, D., and Sandí, R. F. (2002). Disappearance of insectivorous birds from tropical forest fragments. Proc. Nat. Acad. Sci. U.S.A. 99, 263–267. doi: 10.1073/pnas.012616199
Stephens, P. A., Buskirk, S. W., and del Rio, C. M. (2007). Inference in ecology and evolution. Trends Ecol. Evol. 22, 192–197. doi: 10.1016/j.tree.2006.12.003
Terborgh, J., Davenport, L. C., Ong, L., and Campos-Arceiz, A. (2018). Foraging impacts of Asian megafauna on tropical rain forest structure and biodiversity. Biotropica 50, 84–89. doi: 10.1111/btp.12488
Watson, J. E. M., Evans, T., Venter, O., Williams, B., Tulloch, A., Stewart, C., et al. (2018). The exceptional value of intact forest ecosystems. Nat. Ecol. Evol. 2, 599–610. doi: 10.1038/s41559-018-0490-x
Wilkie, D., Shaw, E., Rotberg, F., Morelli, G., and Auzel, P. (2000). Roads, development, and conservation in the Congo Basin. Conserv. Biol. 14, 1614–1622. doi: 10.1111/j.1523-1739.2000.99102.x
Wilman, H., Belmaker, J., Simpson, J., de la Rosa, C., Rivadeneira, M. M., and Jetz, W. (2014). Eltontraits 1.0: species-level foraging attributes of the world's birds and mammals. Ecology 95, 2027–2027. doi: 10.1890/13-1917.1
Wright, S. J. (2003). The myriad consequences of hunting for vertebrates and plants in tropical forests. Perspect. Plant Ecol. Evol. Syst. 6, 73–86. doi: 10.1078/1433-8319-00043
Wright, S. J., Stoner, K. E., Beckman, N., Corlett, R. T., Dirzo, R., Muller-Landau, H. C., et al. (2007). The plight of large animals in tropical forests and the consequences for plant regeneration. Biotropica 39, 289–291. doi: 10.1111/j.1744-7429.2007.00293.x
Keywords: anthropocene, avifauna, habitat change, hunting, mammal, meta-analysis, species traits, tropical forest
Citation: Osuri AM, Mendiratta U, Naniwadekar R, Varma V and Naeem S (2020) Hunting and Forest Modification Have Distinct Defaunation Impacts on Tropical Mammals and Birds. Front. For. Glob. Change 2:87. doi: 10.3389/ffgc.2019.00087
Received: 06 January 2019; Accepted: 06 December 2019;
Published: 10 January 2020.
Edited by:
Yadvinder Malhi, University of Oxford, United KingdomReviewed by:
Marion Pfeifer, Newcastle University, United KingdomKim McConkey, National Institute of Advanced Studies, India
Copyright © 2020 Osuri, Mendiratta, Naniwadekar, Varma and Naeem. This is an open-access article distributed under the terms of the Creative Commons Attribution License (CC BY). The use, distribution or reproduction in other forums is permitted, provided the original author(s) and the copyright owner(s) are credited and that the original publication in this journal is cited, in accordance with accepted academic practice. No use, distribution or reproduction is permitted which does not comply with these terms.
*Correspondence: Anand M. Osuri, moanand@gmail.com