- 1School of Forestry and Environmental Studies, Yale University, New Haven, CT, United States
- 2CSIR – Center for Cellular and Molecular Biology, Hyderabad, India
- 3Smithsonian Tropical Research Institute, Balboa, Panama
Growing awareness of the role of enemies in plant community dynamics has led to ecologists exploring how plant-enemy interactions change in human-modified systems. Proximity to forest edges was found to weaken the role of two groups of plant enemies—insect herbivores and fungal pathogens—in increasing plant diversity during the seed-to-seedling transition. However, it is less clear whether edge effects similarly compromise the diversifying effects of fungi and insects on established seedlings. We examined this question in a human-modified wet tropical forest in the Western Ghats of southern India. Over an annual cycle of recruitment, in 730 seedling plots (1 × 1 m each) arrayed at distances 0–100 m from the forest edge across 15 locations in a 30 km2 landscape, we suppressed the activity of fungal pathogens and insect herbivores by applying fungicide and insecticide to soil, seeds, and seedlings in a subset of plots. Suppressing fungi and insects reduced diversity mainly for seedling recruits and not for seedlings that had already established. However, pesticide effects were only apparent at 90–100 m from forest edges. Specifically, in the interior sites, fungi and insect activity increased recruit diversity, which helped maintain local seedling diversity even though diversity of established seedlings declined with annual mortality. By comparison, canopy openness affected neither the diversity of survivors from the initial seedling cohort nor total seedling diversity after an annual cycle of recruitment. Our results indicate that insects and fungi promote diversity more prominently during early seedling establishment rather than through impacts on post-establishment seedling survival. Thus, edge effects can weaken the diversifying effects of plant-insect and plant-fungal interactions during recruitment and thereby modify the seedling template available for the future tree community in human-modified forests.
Introduction
Top-down regulation by enemies plays a key role in mediating coexistence among plant species and maintaining plant diversity (Janzen, 1970; Connell, 1971; Terborgh, 2012). The diversifying effects occur because individuals of a plant species would be more likely to be infected or killed when situated near individuals of their own species vs. other species, due to distance- and/or density-dependent depredation by enemies (Janzen, 1970; Connell, 1971; Klironomos, 2002; Freckleton and Lewis, 2006; Bever et al., 2015; Teste et al., 2017). Among the plethora of plant enemies found in nature, the role of fungal pathogens and insect herbivores in maintaining diversity is particularly evident during the seed-to-seedling transition and early seedling establishment (Packer and Clay, 2000; Bell et al., 2006; Bagchi et al., 2010, 2014; Mangan et al., 2010; Gripenberg et al., 2014; Liang et al., 2016; Bennett et al., 2017). However, the extent to which fungi and insects also regulate diversity among established seedlings and mediate temporal changes in diversity is unclear. In closed canopy forests where seedlings often remain in the understory for many years (Connell and Green, 2000), weaker regulation of seedling abundances by fungi and insects can alter the community structure of seedlings available to become adults, potentially modifying future species composition of the forest (Gilbert, 2002; Bever et al., 2015).
While seedling recruitment is a crucial bottleneck in plant population dynamics, established seedlings also experience diversifying processes (Comita et al., 2010a; Green et al., 2014). Standing seedling crops suffer depredation from fungal pathogens and insect herbivores (Benitez-Malvido et al., 1999; Gilbert and Webb, 2007), and a diversifying effect of these enemies would increase the diversity of survivors compared to the starting cohort. On the other hand, enemies might only play a substantial role during the seed-to-seedling transition (Bagchi et al., 2014; Krishnadas et al., 2018), ensuring that successive crops of recruits enhance local diversity of established seedlings. Against this backdrop, we recently demonstrated that the diversifying effect of fungi and insects during seed-to-seedling transition can weaken in human-modified forests (Krishnadas et al., 2018). If edge effects similarly weaken diversifying effects of fungal pathogens and insect herbivores on established seedlings, it would compound diversity loss by affecting both recruitment and post-establishment seedling survival.
Temporal changes in seedling diversity can also occur in response to spatial variation in light availability (Nicotra et al., 1999; Gravel et al., 2010). In human-modified forests, higher light availability closer to edges can provide more conducive environments for seedling recruitment and survival than the shaded understory (Matlack, 1993; Murcia, 1995). More open canopies may thus support seedlings of many more species and increase diversity. Local diversity can increase with greater canopy openness because increased light availability especially favors the recruitment and survival of shade-intolerant species, otherwise rare in shaded conditions (Hubbell et al., 2001; Montgomery and Chazdon, 2002; Valladares et al., 2016). Alternatively, higher light may decrease recruitment and survival of shade-tolerant species and hence decrease local seedling diversity (Comita et al., 2010b). It is also possible that all species recruit better in conditions of higher light availability, but many shade-intolerant species do not survive as well as they would under shaded conditions, resulting in survivors having lower diversity than recruits.
Previously, in a human-modified forest in southern India, we found that sites at 90–100 m had a higher diversity of seedling recruits than sites within 60 m of edges, despite similar seed diversity at all edge-distances (Krishnadas et al., 2018). At 90–100 m, diversity during the seed-to-seedling transition declined when we applied pesticides to suppress insects and fungi, but pesticides did not alter recruit diversity within 60 m of edges (Krishnadas et al., 2018). We also found that sites > 60 meters from edges had higher diversity of established seedlings than sites closer to edges (Krishnadas et al., 2019). However, we have not examined whether established seedlings also experience diversifying effects due to fungi and insects. Here, we examine whether insects and fungi regulate temporal changes in seedling diversity through effects on seedling survival, recruitment, or both. Specifically, we ask:
1. How does proximity to edges affect diversity of seedlings over an annual cycle of recruitment?
Given our previous findings, we expected that sites farther from edges would maintain higher diversity of seedlings through another recruitment season. We expected that higher diversity farther from edges would accrue from both higher diversity among established seedlings that survive and due to higher recruit diversity farther from edges. Furthermore, we expected that sites farther from edges would have a greater increase in diversity between survivors and the initial seedling cohort, and between total final diversity and the initial seedling cohort.
2. Are edge effects on seedling diversity due to variation in light or natural enemy activity?
We expected that sites with higher light would have higher diversity of established seedlings that survived through the study. However, based on our previous findings, we did not expect a correlation between light and changes in total seedling diversity, i.e., established seedlings and recruits. Furthermore, we expected that suppressing insects and fungi would decrease the diversity of established seedlings that survived through the year, but only farther from forest edges. We expected that total seedling diversity (established + recruits) would be lower in plots treated with fungicide, insecticide or both pesticides and we expected this response to manifest farther from but not near forest edges.
3. Are changes in seedling diversity due to natural enemy effects on established seedlings, recruits alone, or both?
Studies from other tropical forests have found that the impacts of pathogens and insect herbivores are not restricted to the seed-to-seedling transition, but also influence growth and survival at later stages (Gilbert et al., 2001; Gilbert and Webb, 2007; Benítez-Malvido et al., 2018). Hence, we expected that fungi and insects would regulate diversity among established seedlings as well as recruits, but the effects may be stronger during early recruitment due to potentially greater susceptibility of younger seedlings to fungi and insects (Gilbert, 2002).
Methods
Study Site and Sampling Design
This study was conducted in a 3,600 ha human-modified landscape patchwork of tea plantations, abandoned coffee plantations, roads, and grassland located within Kadamane village (12°56'N and 75°39'E) limits in the Western Ghats Biodiversity Hotspot, Karnataka, south India. The forest is classified as tropical wet evergreen (Pascal, 1986). Details of the study site and sampling design can be found in Krishnadas et al. (2018, 2019), where the reader can also find a map of the study area. Here, we provide a brief overview.
To select sites for the experiment, we first generated 45 random points on a digitized map of the study area using QGIS version 2.17, from which we finalized 15 locations based on field surveys of accessibility, ongoing disturbance, and previous history of human use. We chose the least used sites with intact canopies and minimal ongoing disturbances, especially closer to edges. The criteria for generating random points and final selection of locations are detailed in Krishnadas et al. (2018). At each location, we sampled sites at three distances from the edge−0–5 m (E0), 20–30 m (E1), and 50–60 m (E2), with three replicates (hereafter stations) per site, totaling 45 stations across 15 locations. At five of these locations, we also included three sampling replications at 90–100 m from the edge for a total of 15 additional stations. Replicates at 90–100 m at the other 10 locations was not possible due to logistical challenges of reaching some sites and the fact that sites > 90 m from the edge were often situated in topographically steep or rocky areas with markedly different structure and composition of tree communities. However, previous analysis (using linear mixed-effects models and Tukey's post-hoc comparison of means) showed that diversity of both established seedlings and new recruits remain qualitatively similar whether analyses are conducted with data from all 15 locations or with only data from the five locations with stations at 90–100 m (Krishnadas et al., 2018).
To assess both the extant seedling pool and recruitment of new seedlings, we established five permanently marked 1 × 1 m seedling plots. We randomly assigned three of five seedling plots per station to be sprayed with either fungicide (Amistar® + Ridomil®), insecticide (Actara®), or both pesticides to evaluate the role of fungal pathogens and insect herbivores on seedling communities. Amistar® (active drug: azoxystrobin) is a broad-spectrum systemic fungicide and acts against multiple plant pathogenic fungi and Ridomil® (mancozeb and metalxyl) targets both fungi and oomycetes, with low activity against non-target fungi such as mycorrhizae. The insecticide Actara® (thiamethoxam) provides broad-spectrum systemic and contact protection. We prepared solutions of each pesticide in recommended doses (Amistar: 0.01 g, Ridomil: 0.5 g, Actara: 0.021 g in 100 ml water per 1 m2 plot) and used a hand-mister to apply preparations to the soil and seedlings. We sprayed one plot with 100 ml of water and left one plot untreated. Treatments were applied from September 2015 until November 2016, every 10 days during the dry season and every 5–7 days during the rains. Preliminary analysis showed that diversity did not vary between untreated and “water” plots, so the mean diversity of these two plots were used as “Control” for each station (linear mixed-effects models, Table S1).
To quantify the initial diversity of seedlings prior to starting the experiment, we censused and tagged all existing seedlings before we began spraying pesticides (September 2015). We conducted one census in late March 2016 and a final census after the post-monsoon recruitment peak (November 2016). In both censuses we recorded survival among established seedlings and tagged and identified to species all new seedlings. Less than 2% of seedlings were unidentified. For this analysis, seedlings tagged in the initial census in September 2015 and alive in the final census in November 2016 were considered “survivors.” New seedlings tagged in the final census or tagged in the March 2016 census and alive in the final census were considered “recruits.”
Canopy Openness
We characterized canopy openness as a proxy for understory light availability using hemispherical photos taken 0.25 m above ground at the center of each 1 m2 seedling plot, with a Nikon Coolpix 950 digital camera (Melville, NY, USA) fitted with a Nikon FC-E8 fish-eye lens. Photos were taken in the early mornings from mid-June through mid-July when skies are uniformly overcast with the onset of the rainy season. We analyzed images using Gap Light Analyzer version 2.0 (Frazer et al., 1999). Median canopy openness was significantly higher at E0 (0–5 m) than all other distances from the edge (Kruskal-Wallis test, Chi-squared = 33.04, df = 3, P < 0.001; Krishnadas et al., 2018).
Seedling Diversity Estimates
To enable comparison with our previous analyses (Krishnadas et al., 2018), we used the Inverse Simpson index of effective species number to quantify diversity. We assessed seedling diversity over the 14 months of the study using four metrics: (1) the diversity of established seedlings (i.e., those tagged in the initial census) that survived through the experimental period (hereafter “survivor diversity”), (2) difference between final survivor diversity and initial diversity (hereafter “survivor change”), (3) the total diversity of seedlings that were alive at the end of the experiment, including both survivors from the initial census and new seedling recruits (hereafter “total diversity”), and (4) difference between final total diversity and initial diversity (hereafter “total change”).
Statistical Analysis
To assess how proximity to edges correlated with temporal changes in seedling diversity, we used linear mixed effects models (LMMs) to first model how the four metrics of diversity change varied with distance to forest edge as a four-level categorical variable—E0 (0–5 m), E1 (20–30 m), E2 (50–60 m), and E3 (90–100 m). E0 (0–5 m) was the base level. For these models, we only included data from control plots. Next, data from control plots was used in an LMM to model changes in each diversity metric as a function of percent canopy openness to understand how light availability affected temporal changes in seedling diversity. High seedling diversity may just result from higher initial diversity at a site. Therefore, we examined how initial seedling diversity correlated with diversity of survivors and total diversity at the end of the experiment.
Finally, to understand the role of fungi and insects in mediating changes in diversity, we modeled plot-level diversity as an interaction between distance to edge (four-level factor) and pesticide treatment (three levels: fungicide, insecticide and both) using LMM. For these models, control plots at E0 was set as the base level. In all models, stations were included as random intercepts. We checked the distributions of residuals for all models to ensure that assumptions of normality were met. To assess pairwise differences among the estimated means of different levels of factors in the respective models, we used Tukey's post-hoc tests of Honest Significant Difference (HSD), with P-value corrections for multiple comparisons. Significance of results was assessed at alpha = 0.05, unless otherwise stated.
In all figures, letters show the outcome based on all possible pairs of comparisons, but we also provide the results of contrasts set up to compare 90–100 m from the edge with other edge-distance categories. Our rationale in doing so was that the interior-most sites likely represent the most undisturbed sites for the processes being examined. All analysis was conducted using software R version 3.2.1. GLMMs were implemented using package “nlme” (Pinheiro et al., 2015). We used the function “emmeans” to assess all possible pairwise comparisons and the “contrast” function to contrast 90–100 m against all other edge-distances. Both functions are in package “emmeans” (Russell, 2018). All figures were generated using package “ggplot2” (Wickham, 2016).
Results
Distance to Edge and Seedling Diversity Change
Survivor diversity tended to be higher at 90–100 m from edges than up to 60 m from edges, but not all differences were significant after correcting for multiple pairwise comparisons (Figure 1A; Table S2). Pairwise contrasts showed that survivor diversity of sites at 90–100 m from edges differed significantly from sites at 0–5 m (t = −2.3, P = 0.05) and 20–30 m from edges (t = −2.9, P = 0.01), but not 50–60 m (t = 0.45, P = 0.65). At all edge-distance categories, mean change in diversity between initial seedling pool and survivors was negative, i.e., mean diversity decreased through the study period for established seedlings, with no statistically significant differences among edge-categories (Figure 1B; Table S2). Total diversity of seedlings alive at the final census (i.e., survivors and new recruits) was greater at sites at 90–100 m from edges compared to all other edge-distances (Figure 1C; Table S2). Compared to 90–100 m, total diversity decreased at 0–5 m (t = −4.8, P < 0.001), 20–30 m (t = −5.4, P < 0.001), and 50–60 m (t = −4.4, P < 0.001). In addition, sites at 90–100 m also had greater increase in total diversity from the initial diversity than all other edge-distances (Figure 1D; Table S3). Pairwise comparisons show that change in total diversity at 90–100 m was higher than at 0–5 m (t = −2.3, P = 0.02), 20–30 m (t = −2.2, P = 0.03), and 50–60 m (t = −2.3, P = 0.02). In addition, sites with higher initial diversity of seedlings had higher values of both survivor diversity and total diversity.
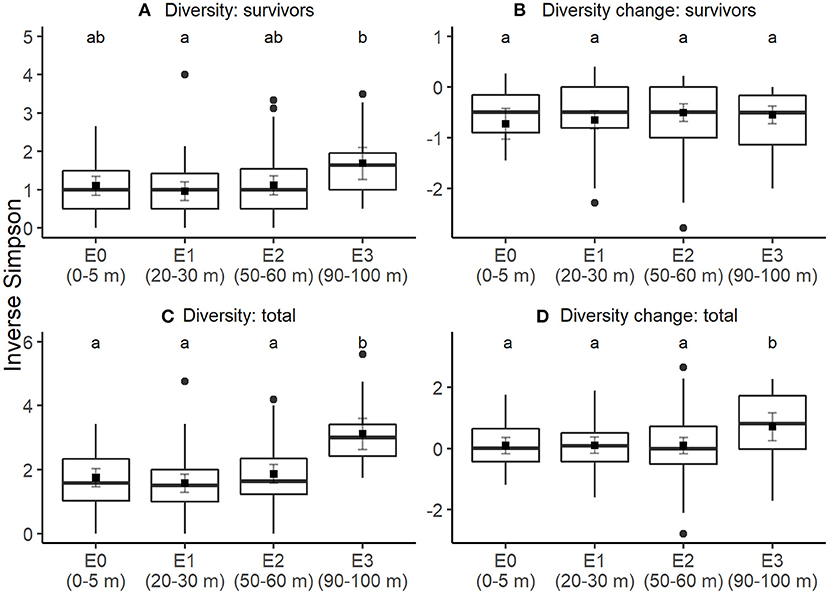
Figure 1. Edge effects on seedling diversity. Through an annual recruitment cycle, at increasing distances from the forest edge, (A) diversity of established seedlings alive at the end of the experiment, (B) change in diversity between initial seedling cohort and final survivors, (C) total seedling diversity including both recruits and survivors, and (D) change in diversity between initial seedling cohort and final seedling cohort including recruits. Boxes correspond to median (central line), first and third quartile of the data and whiskers correspond to 1.5 times the inter-quartile range. Square points show the estimated mean response and gray lines are the associated 95% confidence intervals, assessed using linear mixed effects models with station as a random intercept. Letters denote significance (at alpha = 0.05) of pairwise comparisons assessed using Tukey's post-hoc tests, with P-value correction for multiple comparisons.
Light and Seedling Diversity
Survivor diversity, change in survivor diversity (from the initial pool), and final total diversity did not vary with canopy openness (Figures 2A–C; Table S3). However, sites with more open canopies had larger increases in total diversity from their initial diversities (Figure 2D; Table S3).
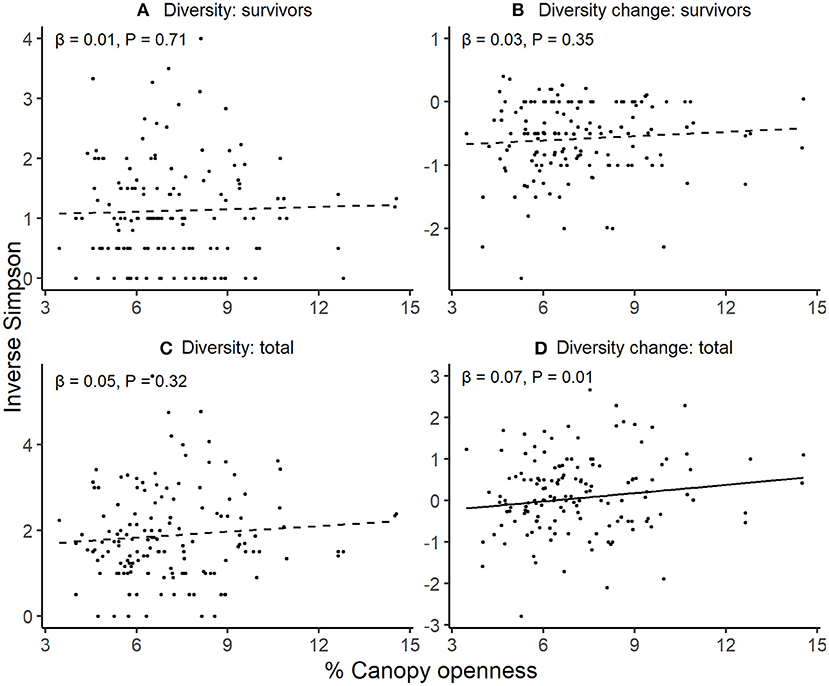
Figure 2. Variation in seedling diversity with canopy openness. Through an annual recruitment cycle, in relation to increasing canopy openness across sites, (A) diversity of established seedlings alive at the end of the experiment, (B) change in diversity between initial seedling cohort and final survivors, (C) total seedling diversity including both recruits and survivors, and (D) change in diversity between initial seedling cohort and final seedling cohort including recruits. For each response, patterns were assessed using linear mixed effects models with station as a random intercept. Lines denote predicted responses from models; solid lines are significant and dashed lines are non-significant relationships estimated at alpha = 0.05.
Effect of Fungi and Insects on Seedling Diversity
Pesticides did not explain differences in survivor diversity among edge-distances (Figures 3A–D; Table S4). Final total diversity of seedlings (i.e., survivors + recruits) varied with pesticide treatment only at 90–100 m from edges (Figures 3E–H; Table S5), where diversity declined in plots treated with fungicide (t = −2.4, P = 0.04) and insecticide (t = −2.7, P = 0.01) and marginally so in plots with both pesticides (t = −2.2, P = 0.07). The change in diversity between survivors and the initial seedling pool did not significantly differ between control plots and treatments of fungicide, insecticide and both pesticides at any edge-distance category (Table S6), but tended to be smaller in fungicide plots at 50–60 m from edges compared to control plots at that edge-distance (Figures 4A–D). Similarly, no edge-distance category showed significant differences between control plots and any pesticide treatment for change in total diversity from the initial diversity. However, at 90–100 m from edges, increases in diversity through recruitment was consistently smaller in plots with insecticide, fungicide and both pesticides compared to control plots (Figures 4E–H; Table S7).
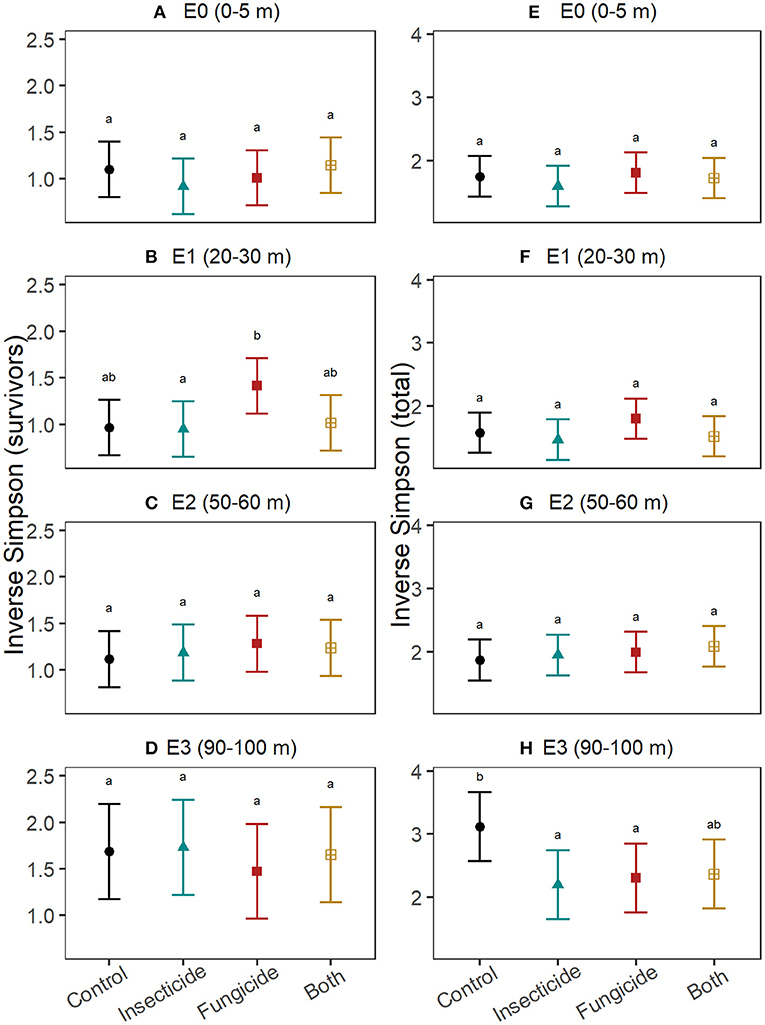
Figure 3. Effects of insects and fungi on seedling diversity. Through an annual recruitment cycle, at increasing distances from the forest edge, the effect of suppressing fungi and insects on (A–D) diversity of established seedlings alive at the end of the experiment and (E–H) total seedling diversity (i.e., both survivors and new recruits). For each response, patterns were assessed using linear mixed effects models with stations included as random intercepts. Points show the estimated mean and error bars are the associated 95% confidence intervals. Letters denote significance (at alpha = 0.05) of pairwise comparisons within each edge-category assessed using Tukey's post-hoc tests with P-value correction for multiple comparisons.
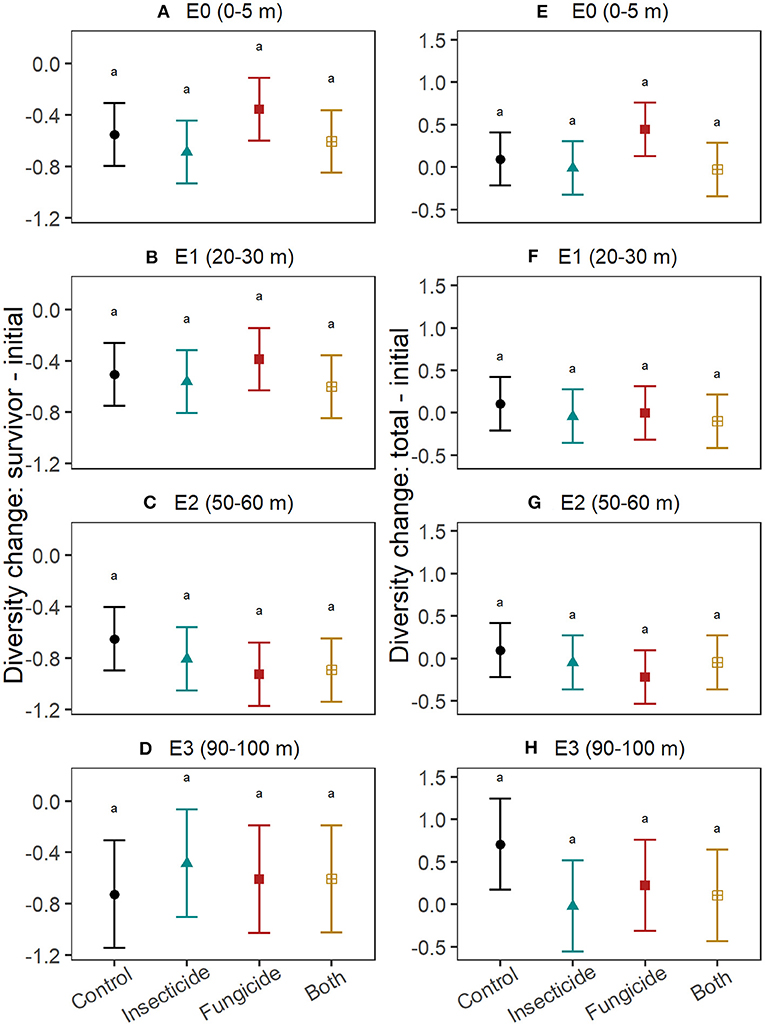
Figure 4. Effects of insects and fungi on change in seedling diversity. Graphs show the effect of suppressing fungi and insects on changes in diversity between seedlings alive in the initial census and those that survived until the end of the study (A–D) and on changes in diversity between seedlings alive in the initial census and all seedlings alive in the final census (survivors + recruits; E–H), at increasing distances from the forest edge. For each response, patterns were assessed using linear mixed effects models with stations included as a random intercept. Points show the estimated mean response and error bars are the associated 95% confidence intervals. Letters denote significance (at alpha = 0.05) of pairwise comparisons assessed using Tukey's post-hoc tests, with P-value correction for multiple comparisons. Differences between control and pesticide treatments within each edge-distance for change in total diversity at E3 (90–100 m) were significant at alpha = 0.1.
Discussion
In a human-modified forest in southern India, we found that edge effects influenced temporal changes in seedling diversity over an annual cycle of recruitment. Sites > 60 m from the edge had higher diversity of established seedlings and higher total diversity when including seedling recruits compared to sites closer to forest edges. These patterns were not explained by light availability, because higher light correlated positively with total diversity, and sites closest to edges had higher light. By comparison, suppressing fungi and insects decreased total seedling diversity at 90–100 m from edges but not sites within 60 m of edges, suggesting that the role of fungi and insects in diversifying seedlings weakens near forest edges. However, change in the diversity of established seedlings did not differ between control plots and when suppressing insect and fungal activity. Overall, these results indicate that edge effects on plant-insect and plant-fungal interactions mainly occur during early seedling establishment and not through short-term impacts on post-establishment seeding survival.
Edge Effects on Temporal Changes in Seedling Diversity
Sites at 90–100 m from forest edges had higher total diversity of seedlings that survived to the end of the experimental period. This was mainly due to the higher initial diversity of seedlings compared to other edge-distances (Krishnadas et al., 2019), because here we found negligible changes in diversity between the initial seedling cohort and those that survived. In fact, diversity of survivors tended to be slightly lower than the initial pool, suggesting that mortality can reduce seedling diversity. Notably though, both survivor diversity and total diversity was higher at sites with higher initial diversity of seedlings (Figure S1). Thus, it appears that edge effects do not affect diversity through survival of established seedlings, at least over the relatively short time periods (14 months) examined in our study. These patterns are consistent with results from an Amazonian wet forest, where Sizer and Tanner (1999) found that edge creation mainly affected recruitment and not so much survival of seedlings.
Compared to sites within 60 m of edges, sites at 90–100 m from edges saw an increase in seedling diversity from the initial diversity of established seedlings, which led to sites at 90–100 m having higher total diversity of seedlings, i.e., including individuals that recruited during the study. Together with our previous findings of higher recruit diversity at 90–100 m from edges relative to all other edge-distances, these results suggest that edge effects on seedling diversity mainly occur through altered recruitment dynamics (Krishnadas et al., 2018). Because processes that regulate seedling recruitment contribute to maintaining tree diversity (Connell and Green, 2000; Green et al., 2014), persistent erosion of plant-enemy interactions during seedling recruitment can compromise future diversity of tree communities in human-modified forests (Muscarella et al., 2013; Larson et al., 2016; Krishnadas et al., 2018). Our study suggests that conserving tree diversity may require retaining forest patches large enough to prevent edge effects on key biotic interactions that maintain diversity.
Canopy Openness and Seedling Diversity
Edge effects on seedling recruitment and survival are often thought to accrue from increased light availability near edges (Sizer and Tanner, 1999; Benitez-Malvido and Martinez-Ramos, 2003b; Tabarelli et al., 2012; Santo-Silva et al., 2013). Consistent with patterns seen in other tropical forests (Sizer and Tanner, 1999), we found that median canopy openness and light availability was greater at 0–5 from edges than at all other edge-distances (Krishnadas et al., 2018). In closed-canopy forests, higher light availability under more open canopies can increase seedling diversity by promoting the recruitment of shade intolerant species, as observed in canopy gaps (Augspurger, 1984; Hubbell et al., 1999). Nonetheless, we found no correlation between canopy openness and diversity of seedlings that survived from the initial pool or the change in diversity between survivors and initial pool.
Increase in the diversity of the final seedling pool compared to the initial pool after the annual cycle of recruitment increased with greater canopy openness, but canopy openness did not correlate with net total diversity (survivors + recruits) or recruit diversity (Krishnadas et al., 2018). Initial diversity of established seedlings was also uncorrelated with canopy openness (linear mixed-effects model, t = −0.65, P = 0.51, Figure S2), suggesting that canopy openness played only a weak role in regulating spatial variation in diversity in this landscape. Thus, the effect of canopy openness on seedling diversity was likely overridden by other factors regulating seedling recruitment, e.g., regulation by insects and fungi. How the relative importance of light and enemies plays out over multiple cycles of recruitment remains to be understood, but our findings highlight why seedling communities vary in their response to edge effects, which have generally been discussed with respect to light availability (Sizer and Tanner, 1999; Benitez-Malvido and Martinez-Ramos, 2003b; Tabarelli et al., 2012; Santo-Silva et al., 2013).
In our study landscape, light availability—estimated using Gap Light Analyzer using the same photos as for canopy openness—was highly correlated with canopy openness (r = 0.81), but not correlated with seedling diversity. This suggests that sustained edge effects on seedling diversity may not occur primarily because of increased light under more open canopies. Of course, canopy openness can be associated with changes in abiotic factors other than light that can affect seedling dynamics, e.g., soil moisture and temperature (Arroyo-Rodríguez et al., 2017b), but we did not measure other abiotic variables in this study. Moreover, we chose sites with relatively intact canopies, avoiding tree fall gaps, historically logged or currently disturbed areas, and the limited variation in canopy openness/light may explain its weak correlation with seedling diversity.
Edge Effects on Insect and Fungal Regulation of Seedling Diversity
While canopy openness did not seem to explain edge effects on seedling diversity, we found that sites at different distances from the forest edge varied in how their seedling communities responded to suppression of fungi and insects. Notably, suppressing insects and fungi affected total seedling diversity at the end of the experimental period and not the diversity of survivors from the initial seedling cohort. While total seedling diversity did not vary with fungicide and insecticide application up to 60 m of forest edges, at 90–100 m from edges, total diversity declined in plots treated with fungicide and insecticide treatment. In relation to our previous findings of significant declines in recruit diversity with fungicide and insecticide application at 90–100 m from edges, our results here suggest that insects and fungi played a stronger diversifying role during early seedling dynamics compared to after seedlings have established (Krishnadas and Comita, 2018; Krishnadas et al., 2018).
At 90–100 m from edges, the change in total diversity with suppression of fungi and insects also resulted in smaller increases in diversity from the initial diversity of established seedlings, albeit the result being marginally insignificant. Overall, our results corroborate previous studies where recruitment dynamics were found to play a key role in maintaining or enhancing local seedling diversity (Harms et al., 2000; Green et al., 2014), which in turn could influence long-term diversity through subsequent life-stages (Wright, 2002). Lower diversity of established seedlings near forest edges, observed here and elsewhere (Benitez-Malvido and Martinez-Ramos, 2003a; Santo-Silva et al., 2013; Krishnadas et al., 2019), may accrue with persistent declines in the ability of fungi and insects to enhance local diversity through annual cycles of recruitment. The lack of fungicide effects on established seedlings may have occurred if fungicides affected mycorrhiza, given their role in growth and survival of established seedlings, but previous studies found limited negative effects of these fungicides on mycorrhizae and other non-target organisms (Gripenberg et al., 2014).
It is possible though that the duration of our study was insufficient to pick up the effects of insects and fungal enemies on established seedlings, which may occur over longer time scales. For example, insect herbivores can alter survival and growth of older seedlings and saplings (Forrister et al., 2019), but these effects may take years to manifest, particularly in terms of aggregate community responses such as diversity or composition. Similarly, fungi infect established seedlings and saplings and impact their growth and survival (Gilbert and Webb, 2007), and foliar fungal infection and associated plant performance can be altered by fragmentation (Benitez-Malvido et al., 1999; Benítez-Malvido et al., 2018). However, the aggregate effects of altered fungal activity on temporal changes in seedling diversity may only manifest over longer time-frames, indicating the need for long-term studies that monitor plant-enemy interactions in human-modified forests (Gilbert, 2002; García-Guzmán and Heil, 2014).
Conclusion
Multiple processes simultaneously affect seedling recruitment and diversity, and the relative importance of processes can change with human modification of forests (Arroyo-Rodríguez et al., 2017a; Comita et al., 2018). Recently, we found that the diversifying effects of fungi and insects during seedling recruitment weakens near forest edges in human-modified forests (Krishnadas et al., 2018), which could be due to weaker regulation of seedling abundances by enemies near forest edges (Krishnadas and Comita, 2018). Here, we find that edge effects on annual changes in seedling diversity mainly ensue from this alteration of early seedling recruitment, and less through the effects of fungi and insects of established seedlings. If these changes to recruitment accumulate and persist, it could compromise long-term maintenance of diversity in human-modified forests, offering an explanation for observed losses of seedling diversity and composition near forest edges (Benitez-Malvido and Martinez-Ramos, 2003a; Krishnadas et al., 2019). Such declines in diversity can potentially affect compositional stability of tree communities, i.e., temporal variation in relative abundances of species, with implications for ecosystem functioning (Tilman et al., 2014). Because seedlings present a crucial population bottleneck and shape community structure of future forests (Green et al., 2014), understanding seedling dynamics will help design ecologically informed strategies for management and restoration of human-modified forests (Larson et al., 2016). While maintaining dispersal and connectivity are known to be important management goals (Damschen and Brudvig, 2012), our findings indicate that conserving tree diversity also require having areas large enough to avoid edge effects on key biotic interactions that maintain diversity.
Data Availability Statement
The datasets generated for this study are available on request to the corresponding author.
Author Contributions
MK conceived the idea, collected data, performed the analysis, and wrote the first draft of the manuscript. LC contributed substantially toward refining the idea and provided feedback on analysis and draft versions.
Funding
Funding for this study came from the Arnold Arboretum at Harvard University (Ashton Award, 2016), the Garden Club of America (Award in Tropical Botany 2015), Yale Institute for Biosphere Studies (YIBS Dissertation Research Grant 2016), and the Yale Tropical Resources Institute.
Conflict of Interest
The authors declare that the research was conducted in the absence of any commercial or financial relationships that could be construed as a potential conflict of interest.
Acknowledgments
We thank Kadamane Estate Company for allowing research on their property. Netraprasad Sharma, Suresh Roy, and Kanjimalai were instrumental during data collection, for which we are very grateful. Thanks to Arun Kumar, Kavya Agarwal, Meghana R, and Sachin Sridhara for offering their time for this project.
Supplementary Material
The Supplementary Material for this article can be found online at: https://www.frontiersin.org/articles/10.3389/ffgc.2019.00076/full#supplementary-material
References
Arroyo-Rodríguez, V., Melo, F. P. L., Martínez-Ramos, M., Bongers, F., Chazdon, R. L., Meave, J. A., et al. (2017a). Multiple successional pathways in human-modified tropical landscapes: new insights from forest succession, forest fragmentation and landscape ecology research. Biol. Rev. Camb. Philos. Soc. 92, 326–340. doi: 10.1111/brv.12231
Arroyo-Rodríguez, V., Saldaña-Vázquez, R. A., Fahrig, L., and Santos, B. A. (2017b). Does forest fragmentation cause an increase in forest temperature? Ecol. Res. 32, 81–88. doi: 10.1007/s11284-016-1411-6
Augspurger, C. K. (1984). Seedling survival of tropical tree species: interactions of dispersal distance, light-gaps, and pathogens. Ecology 65, 1705–1712. doi: 10.2307/1937766
Bagchi, R., Gallery, R. E., Gripenberg, S., Gurr, S. J., Narayan, L., Addis, C. E., et al. (2014). Pathogens and insect herbivores drive rainforest plant diversity and composition. Nature 506, 85–88. doi: 10.1038/nature12911
Bagchi, R., Swinfield, T., Gallery, R. E., Lewis, O. T., Gripenberg, S., Narayan, L., et al. (2010). Testing the Janzen-Connell mechanism: pathogens cause overcompensating density dependence in a tropical tree. Ecol. Lett. 13, 1262–1269. doi: 10.1111/j.1461-0248.2010.01520.x
Bell, T., Freckleton, R. P., and Lewis, O. T. (2006). Plant pathogens drive density-dependent seedling mortality in a tropical tree. Ecol. Lett. 9, 569–574. doi: 10.1111/j.1461-0248.2006.00905.x
Benitez-Malvido, J., Garcia-Guzmán, G., and Kossmann-Ferraz, I. D. (1999). Leaf-fungal incidence and herbivory on tree seedlings in tropical rainforest fragments: an experimental study. Biol. Conserv. 91, 143–150. doi: 10.1016/S0006-3207(99)00090-7
Benítez-Malvido, J., Lázaro, A., and Ferraz, I. D. K. (2018). Effect of distance to edge and edge interaction on seedling regeneration and biotic damage in tropical rainforest fragments: a long-term experiment. J. Ecol. 106, 2204–2217. doi: 10.1111/1365-2745.13003
Benitez-Malvido, J., and Martinez-Ramos, M. (2003a). Impact of forest fragmentation on understory plant species richness in Amazonia. Conserv. Biol. 17, 389–400. doi: 10.1046/j.1523-1739.2003.01120.x
Benitez-Malvido, J., and Martinez-Ramos, M. (2003b). Influence of edge exposure on tree seedling species recruitment in tropical rain forest fragments. Biotropica 35, 530–541. doi: 10.1111/j.1744-7429.2003.tb00609.x
Bennett, J. A., Maherali, H., Reinhart, K. O., Lekberg, Y., Hart, M. M., and Klironomos, J. (2017). Plant-soil feedbacks and mycorrhizal type influence temperate forest population dynamics. Science 355, 181–184. doi: 10.1126/science.aai8212
Bever, J. D., Mangan, S. A., and Alexander, H. M. (2015). Maintenance of plant species diversity by pathogens. Annu. Rev. Ecol. Evol. Syst. 46, 305–325. doi: 10.1146/annurev-ecolsys-112414-054306
Comita, L. S., Muller-Landau, H. C., Aguilar, S., and Hubbell, S. P. (2010a). Asymmetric density dependence shapes species abundances in a tropical tree community. Science 329, 330–332. doi: 10.1126/science.1190772
Comita, L. S., Thompson, J., Uriarte, M., Jonckheere, I., Canham, C. D., and Zimmerman, J. K. (2010b). Interactive effects of land use history and natural disturbance on seedling dynamics in a subtropical forest. Ecol. Appl. 20, 1270–1284. doi: 10.1890/09-1350.1
Comita, L. S., Uriarte, M., Forero-Montaña, J., Kress, W. J., Swenson, N. G., Thompson, J., et al. (2018). Changes in phylogenetic community structure of the seedling layer following hurricane disturbance in a human-impacted tropical forest. Forests 9, 1–17. doi: 10.3390/f9090556
Connell, J. H. (1971). “On the role of natural enemies in preventing competitive exclusion in some marine animals and in rain forest trees,” in Dynamics of Populations, Centre for Agricultural Publishing and Documentation, eds P. J. Den Boer and G. R. Gradwell (Wageningen), 298–312.
Connell, J. H., and Green, P. T. (2000). Seedling dynamics over thirty-two years in a tropical rainforest tree. Ecology 81, 568–584. doi: 10.1890/0012-9658(2000)081[0568:SDOTTY]2.0.CO;2
Damschen, E. I., and Brudvig, L. A. (2012). Landscape connectivity strengthens local-regional richness relationships in successional plant communities. Ecology 93, 704–710. doi: 10.1890/11-1797.1
Forrister, D. L., Endara, M. J., Younkin, G. C., Coley, P. D., and Kursar, T. A. (2019). Herbivores as drivers of negative density dependence in tropical forest saplings. Science 363, 1213–1216. doi: 10.1126/science.aau9460
Frazer, G. W., Canham, C. D., and Lertzman, K. P. (1999). Gap Light Analyzer (GLA), Version 2.0: Imaging Software to Extract Canopy Structure and Gap Light Transmission Indices from True-Colour Fisheye Photographs, Users Manual and Program Documentation. New York, NY: Simon Fraser University, Burnaby, British Columbia, and the Institute of Ecosystem Studies, Millbrook.
Freckleton, R. P., and Lewis, O. T. (2006). Pathogens, density dependence and the coexistence of tropical trees. Proc. R. Soc. Biol. Sci. 273, 2909–2916. doi: 10.1098/rspb.2006.3660
García-Guzmán, G., and Heil, M. (2014). Life histories of hosts and pathogens predict patterns in tropical fungal plant diseases. New Phytol. 201, 1106–1120. doi: 10.1111/nph.12562
Gilbert, G. S. (2002). Evolutionary ecology of plant dieseases in natural ecosystems. Annu. Rev. Phytopathol 40, 13–43. doi: 10.1146/annurev.phyto.40.021202.110417
Gilbert, G. S., Harms, K. E., Hamill, D. N., and Hubbell, S. P. (2001). Effects of seedling size, El Niño drought, seedling density, and distance to nearest conspecific adult on 6-year survival of Ocotea whitei seedlings in Panamá. Oecologia 127, 509–516. doi: 10.1007/s004420000616
Gilbert, G. S., and Webb, C. O. (2007). Phylogenetic signal in plant pathogen-host range. Proc. Natl. Acad. Sci. U.S.A. 104, 4979–4983. doi: 10.1073/pnas.0607968104
Gravel, D., Canham, C. D., Beaudet, M., and Messier, C. (2010). Shade tolerance, canopy gaps and mechanisms of coexistence of forest trees. Oikos 119, 475–484. doi: 10.1111/j.1600-0706.2009.17441.x
Green, P. T., Harms, K. E., and Connell, J. H. (2014). Nonrandom, diversifying processes are disproportionately strong in the smallest size classes of a tropical forest. Proc. Natl. Acad. Sci. U.S.A. 111, 18649–18654. doi: 10.1073/pnas.1321892112
Gripenberg, S., Bagchi, R., Gallery, R. E., Freckleton, R. P., Narayan, L., and Lewis, O. T. (2014). Testing for enemy-mediated density-dependence in the mortality of seedlings: field experiments with five Neotropical tree species. Oikos 123, 185–193. doi: 10.1111/j.1600-0706.2013.00835.x
Harms, K. E., Wright, S. J., Calderón, O., Hernández, A., and Herre, E. A. (2000). Pervasive density-dependent recruitment enhances seedling diversity in a tropical forest. Nature 404, 493–495. doi: 10.1038/35006630
Hubbell, S. P., Ahumada, J. A., Condit, R., and Foster, R. B. (2001). Local neighborhood effects on long-term survival of individual trees in a neotropical forest. Ecol. Res. 16, 859–875. doi: 10.1046/j.1440-1703.2001.00445.x
Hubbell, S. P., Foster, R. B., Brien, S. T. O., Harms, K. E., and Condit, R. (1999). Light-gap disturbances, recruitment limitation, and tree diversity in a neotropical forest. Science 283, 554–557. doi: 10.1126/science.283.5401.554
Janzen, D. H. (1970). Herbivores and the number of tree species in tropical forests. Am. Nat. 104, 501–528. doi: 10.1086/282687
Klironomos, J. N. (2002). Feedback with soil biota contributes to plants rarity and invasiveness in communities. Nature 417, 67–69. doi: 10.1038/417067a
Krishnadas, M., Bagchi, R., Sridhara, S., and Comita, L. S. (2018). Weaker plant-enemy interactions decrease tree seedling diversity with edge-effects in a fragmented tropical forest. Nat. Commun. 9:4523. doi: 10.1038/s41467-018-06997-2
Krishnadas, M., and Comita, L. S. (2018). Influence of soil pathogens on early regeneration success of tropical trees varies between forest edge and interior. Oecologia 186, 259–268. doi: 10.1007/s00442-017-4006-1
Krishnadas, M., Kumar, A. N., and Comita, L. S. (2019). Edge effects reduce α-diversity but not β-diversity during community assembly in a human-modified tropical forest. Ecol. Appl. doi: 10.1002/eap.1996. [Epub ahead of print].
Larson, J. E., Funk, J. L., and Whitney, K. (2016). Regeneration: an overlooked aspect of trait-based plant community assembly models. J. Ecol. 104, 1284–1298. doi: 10.1111/1365-2745.12613
Liang, M., Liu, X., Gilbert, G. S., Zheng, Y., Luo, S., Huang, F., et al. (2016). Adult trees cause density-dependent mortality in conspecific seedlings by regulating the frequency of pathogenic soil fungi. Ecol. Lett. 19, 1448–1456. doi: 10.1111/ele.12694
Mangan, S. A., Herre, E. A., and Bever, J. D. (2010). Specificity between neotropical tree seedlings and their fungal mutualists leads to plant-soil feedback. Ecology 91, 2594–2603. doi: 10.1890/09-0396.1
Matlack, G. R. (1993). Microenvironment variation within and among forest edge sites in the eastern United States. Biol. Conserv. 66, 185–194. doi: 10.1016/0006-3207(93)90004-K
Montgomery, R. A., and Chazdon, R. L. (2002). Light gradient partitioning by tropical tree seedlings in the absence of canopy gaps. Oecologia 131, 165–174. doi: 10.1007/s00442-002-0872-1
Murcia, C. (1995). Edge effects in fragmented forests: implications for conservation. Trends Ecol. Evol. 10, 58–62. doi: 10.1016/S0169-5347(00)88977-6
Muscarella, R., Uriarte, M. M., Forero-Montaña, J., Comita, L. S., Swenson, N. G., Thompson, J., et al. (2013). Life-history trade-offs during the seed-to-seedling transition in a subtropical wet forest community. J. Ecol. 101, 171–182. doi: 10.1111/1365-2745.12027
Nicotra, A. B., Chazdon, R. L., and Iriarte, S. V. B. (1999). Spatial heterogeneity of light and woody seedling regeneration in tropical wet forests. Ecology 80, 1908–1926. doi: 10.1890/0012-9658(1999)080[1908:SHOLAW]2.0.CO;2
Packer, A., and Clay, K. (2000). Soil pathogens and spatial patterns of seedling mortality in a temperate tree. Nature 404, 278–281. doi: 10.1038/35005072
Pascal, J. P. (1986). Explanatory Booklet on the Forest Map of South India. Sheets: Belgaum-Dharwar-Panaji, Shimoga, Mercara-Mysore. Institute Français Pondichery.
Pinheiro, J., Bates, D., DebRoy, S., Sarkar, D., and R Development Core Team, R. (2015). nlme: Linear and Nonlinear Mixed Effects Models. R Package version 3.1-137. Available online at: https://cran.r-project.org/package=nlme (accessed September 15, 2019).
Russell, L. (2018). emmeans: Estimated Marginal Means, aka Least-Squares Means. R Package Version 1.2.4. Available online at: https://CRAN.R-project.org/package=emmeans (accessed September 15, 2019).
Santo-Silva, E. E., Almeida, W. R., Melo, F. P. L., Zickel, C. S., and Tabarelli, M. (2013). The nature of seedling assemblages in a fragmented tropical landscape: implications for forest regeneration. Biotropica 45, 386–394. doi: 10.1111/btp.12013
Sizer, N., and Tanner, E. V. J. (1999). Responses of woody plant seedlings to edge formation in a lowland tropical rainforest, Amazonia. Biol. Conserv. 91, 135–142. doi: 10.1016/S0006-3207(99)00076-2
Tabarelli, M., Peres, C. A., and Melo, F. P. L. (2012). The ‘few winners and many losers' paradigm revisited: Emerging prospects for tropical forest biodiversity. Biol. Conserv. 155, 136–140. doi: 10.1016/j.biocon.2012.06.020
Terborgh, J. (2012). Enemies maintain hyperdiverse tropical forests. Am. Nat. 179, 303–314. doi: 10.1086/664183
Teste, F. P., Kardol, P., Turner, B. L., Wardle, D. A., Zemunik, G., Renton, M., et al. (2017). Plant-soil feedback and the maintenance of diversity in Mediterranean-climate shrublands. Science 355, 173–176. doi: 10.1126/science.aai8291
Tilman, D., Isbell, F., and Cowles, J. M. (2014). Biodiversity and ecosystem functioning. Annu. Rev. Ecol. Evol. Syst. 45, 471–493. doi: 10.1146/annurev-ecolsys-120213-091917
Valladares, F., Laanisto, L., Niinemets, Ü., and Zavala, M. A. (2016). Shedding light on shade: ecological perspectives of understorey plant life. Plant Ecol. Divers. 9, 237–251. doi: 10.1080/17550874.2016.1210262
Keywords: diversity, edge effects, fragmentation, fungal pathogens, insects, plant-enemy interaction, seedling recruitment, tropical forest
Citation: Krishnadas M and Comita LS (2019) Edge Effects on Seedling Diversity Are Mediated by Impacts of Fungi and Insects on Seedling Recruitment but Not Survival. Front. For. Glob. Change 2:76. doi: 10.3389/ffgc.2019.00076
Received: 06 September 2019; Accepted: 01 November 2019;
Published: 19 November 2019.
Edited by:
Treena Burgess, Murdoch University, AustraliaReviewed by:
Denita Hadziabdic, The University of Tennessee, Knoxville, United StatesPeter Matthew Scott, The New Zealand Institute for Plant and Food Research Ltd, New Zealand
Copyright © 2019 Krishnadas and Comita. This is an open-access article distributed under the terms of the Creative Commons Attribution License (CC BY). The use, distribution or reproduction in other forums is permitted, provided the original author(s) and the copyright owner(s) are credited and that the original publication in this journal is cited, in accordance with accepted academic practice. No use, distribution or reproduction is permitted which does not comply with these terms.
*Correspondence: Meghna Krishnadas, c3Jpc2h0aS5tZWdobmEmI3gwMDA0MDtnbWFpbC5jb20=; bWVnaG5hJiN4MDAwNDA7Y2NtYi5yZXMuaW4=