- 1Plant Nutrition Laboratory, Research Faculty of Agriculture, Hokkaido University, Sapporo, Japan
- 2Forest Ecology and Silviculture Laboratory, Graduate School of Agriculture, Hokkaido University, Sapporo, Japan
- 3Field Science Center for Northern Biosphere, Hokkaido University, Sapporo, Japan
- 4State Key Laboratory of Urban and Regional Ecology, Research Center for Eco-Environmental Science, Chinese Academy of Sciences, Beijing, China
- 5University of Chinese Academy of Sciences, College of Resources and Environment, Beijing, China
The effect of elevated ozone (eO3) and soil salinization with alkaline salts in northeastern (NE) China is a serious concern affecting the success of the national replanting project. As planted areas exceed 4 million hectares in China, we must consider future afforestation efforts after thinning and harvesting. Here, we investigated eO3 and salt stress on Dahurian larch (Larix gmelinii var. japonica; DL) and Japanese larch (L. kaempferi; JL) seedlings. The seedlings were exposed to eO3 (~70 nmol mol−1) and ambient ozone (aO3) (~25~40 nmol mol−1) for one growing season in an open top chamber (OTC) system with simulated salinity in NE China (alkaline salt, NaHCO3:NaCl = 9:1, 20mM Na+). The seedlings under salt-free treatment received tap water. Although the effects of eO3 on DL were not significant, eO3 significantly increased total dry mass and total leaf area index of JL. There was no significant reduction in total dry mass under salt stress in both species. The relationship between needle Na and other mineral contents indicated that both species maintained K contents even with excess Na contents in needles. DL showed relatively lower reduction of other mineral contents, indicating higher salt tolerance of needle element homeostasis than JL. Contrary to our hypothesis, there were no interaction effects of eO3 and salt stress on both species. These results indicated that DL seedlings may be more suitable than JL seedlings as a future afforestation species under eO3 levels of <70 nmol mol−1 at saline soil condition.
Introduction
Ground-level ozone (O3) is the most harmful air pollutant to forest ecosystems on both local and global scales (Paoletti et al., 2007, 2010; Matyssek et al., 2012; Sicard and Dalstein-Richier, 2015; Sicard et al., 2016). Ozone suppresses plant growth, accelerates leaf senescence, changes carbon allocation to roots, and induces nutritional imbalances in leaves (Matyssek et al., 2012; Agathokleous et al., 2015; Shi et al., 2017). Effects of elevated O3 (eO3) on forest ecosystems are a concern in northeast (NE) Asia (Koike et al., 2013; Akimoto et al., 2015). In China, the annual daily maximum O3 concentration in some regions often reaches 60 nmol mol−1 (Gaudel et al., 2018). Recent studies have predicted that the annual mean change in surface O3 concentrations in Asia will rise to ~8 nmol mol−1 by 2050 (Lee et al., 2015; Turnock et al., 2018). The current eO3 level is high enough to suppress tree growth and degrade ecosystem health (Chen et al., 2015; Feng et al., 2015). Thus, the conservation of forests in China is an issue of increasing interest in regions that face multiple environmental stresses, such as eO3, nitrogen deposition, and drought (Reilly et al., 2007; Fang et al., 2014; Hu et al., 2015; Yuan et al., 2016).
Several regions of China have begun reforestation because of the intensive thinning and harvesting that has persisted since the Grain for Green Project began in 1999 (Zhang et al., 2000; Feng et al., 2005; Xu, 2011). However, in NE China, where forestry practices have been active, not all afforestation is well established cause to the soil salinization that occurs with low precipitation and high temperature (e.g., Feng et al., 2005; Sakai, 2012). Saline soil occupies ~99,000 km2 of China's land area (Zhang et al., 2010; Xu, 2011; Li et al., 2014). The frequency of extreme high temperatures in summer has been increasing in China over 60-fold since the 1950s (Sun et al., 2014). The relative drought conditions may increase the potential for salinization stresses (Polle and Chen, 2015). Especially in NE China, soil salinization often occurs with low precipitation and high temperature (Ma and Fu, 2006; Zhou et al., 2011; Sakai, 2012). Excess salinity causes physiological and metabolic imbalance in plants via ionic stresses (Marschner, 2012; Polle and Chen, 2015; Wungrampha et al., 2018). The effects of salinity on plants have been investigated with sodium chloride (NaCl), which are known to cause ionic imbalance and inhibit mineral ion uptake of potassium (K) and calcium (Ca) (Gerosa et al., 2014; Guidi et al., 2016; Plesa et al., 2018). Since arid and semi-arid lands contain chlorides and carbonates of several minerals, including Na, Ca, and magnesium (Mg), the severe effects of alkaline salt accumulation on plants and trees have received increased attention (Yang et al., 2008; Liu and Shi, 2010; Wang et al., 2013). For example, in the Songnen plain of Heilongjiang Province, 3.2 million ha are influenced by alkaline salt accumulation, and continues to expand by 20,000 ha per year (Wang et al., 2009). Thus, for forest conservation in NE China, we should investigate the combined stresses of eO3 and salts on forest growth and health.
The combined effects of eO3 and salt have largely been described in Mediterranean areas. For example, metabolic responses in pomegranate (Punica granatum L.) have indicated eco-physiological adaptation up to 50 mM NaCl stress, but did not mitigate O3-induced oxidative stresses (Calzone et al., 2019). Holm oaks (Quercus ilex L.) treated under 150 mM NaCl treatments did not show significant photosynthetic depression by eO3 (Guidi et al., 2016). In addition, previous studies have reported that salt stresses mitigated negative effects of eO3 via stomatal closure (Gerosa et al., 2014), and its effects were exacerbated under salt stresses (Calzone et al., 2019), or no interaction between eO3 and salt stresses (Guidi et al., 2016). Thus, the views of the combined effects of eO3 and salt stresses are still inconsistent.
Larch (Larix sp.) has a higher tolerance to harsh conditions and is widely planted throughout northern hemisphere (Gower and Richards, 1990; Zhang et al., 2000; Ryu et al., 2009; Mao et al., 2010). For examples, Dahurian larch (Larix gmelinii) has a higher expectation as an afforestation species in NE China (Zhang et al., 2000; Shi et al., 2010). Japanese larch (L. kaempferi, JL) has been planted in the Korean peninsula and northern Japan (Lee et al., 2004; Ryu et al., 2009). The current larch forests occupy over 40 % of the total carbon growing stock in Eurasian boreal forests, including NE China (Alexeyev et al., 2000; Wang et al., 2001). Based on the Chinese policy of replantation since 1999, increased afforestation has raised concerns regarding low species diversity in forests and poor ecological functions, such as material cycling and habitat provision (Xu and Wilkes, 2004; Zhang and Zhang, 2007). Thus, the effects of eO3 and salt should be investigated on Larix sp. (Zhang et al., 2000; Chazdon, 2008; Ryu et al., 2009) as well as exciting afforestation species, such as Populus sp.
The purpose of this study was to evaluate the effects of eO3 and alkaline salt on Dahurian larch (DL) and Japanese larch (JL) seedlings. Koike et al. (2012) reported the O3 suppressed photosynthesis rate of only DL but not of JL. While many studies have reported that O3-induce inhibition can be determined by absorbed O3 amount (Matyssek et al., 2004; Hu et al., 2015), there were no significant differences in stomatal conductance between DL and JL seedlings (Koike et al., 2012). These results indicate that O3 sensitivity differences between these two larch species can be caused by non-stomatal factors, which may be evaluated through PSII photochemical systems (Gielen et al., 2006; Guidi et al., 2016) and the leaf nutritional status (e.g., Barnes et al., 1995; Shang et al., 2018).
We expected that the inhibitions of eO3 and salt could be evaluated via nutrient imbalance status. It was reported that the nutrient concentrations in plants, such as N and P, were increased by eO3, which may be adaptive strategy against eO3 (Cao et al., 2016; Shang et al., 2018). Besides, Larch may have specific salt tolerance to the K homeostasis (Renault, 2005; Plesa et al., 2018). It was reported that NaCl treatment did not inhibit K absorption in larch saplings; rather, the saplings took up more K even with NaCl treatment compared to the control condition (Renault, 2005; Plesa et al., 2018). K homeostasis is one of the salt tolerances and it has been considered as secondary importance in many published literatures (Tester and Davenport, 2003; Marschner, 2012). There are several other mechanisms to manage excess Na contents such as the exclusion of Na and osmotic regulation by compatible solutes (Flowers, 2004; Munns et al., 2006). However, it has reported that the practical outcomes of these approaches were not effective on improving salt tolerance of plants under filed conditions (Shabala and Cuin, 2007; Wang et al., 2013; Anschütz et al., 2014).
Increasing K contents could induce the homeostasis of photosynthesis and metabolic activity, and the osmotic adjustment (Volkov et al., 2003; Shabala and Cuin, 2007). In fact, an optimal K status can be index for stress resistance when plants are exposed to abiotic stresses (Marschner, 2012; Wang et al., 2014). It was reported that a K fertilization compensated the O3 effects on potted Norway spruce (Picea abies) seedlings although there were genetic-specific responses (Keller and Matyssek, 1990; Barnes et al., 1995), suggesting that the species with relatively higher K content may show insights for the O3 tolerance. Furthermore, several studies have investigated the mineral homeostasis under eO3 (Alcántara et al., 2006; Agathokleous et al., 2018). These studies suggest that the homeostatic adjustment in leaf element content can mitigate physiological inhibitions of eO3 although they focused on the homeostasis of other mineral element such as iron and P.
Here, we firstly expected that (i) O3 would suppress growth of DL but not of JL as Koike et al. (2012) reported. However, we hypothesized (ii) DL is more tolerant relative to JL since it is more adapted to salty soils due to its ecological distribution (Abaimov, 2010; Mao et al., 2010; Mamet et al., 2019), where soil salinization often occurs (Shi et al., 2010; Zhou et al., 2011), and thus salt treatment would not inhibit K absorption in DL. This comparison of salt responses between the two larches may provide novel insights on the eco-physiology to understand how larch acquires the adaptive capacity to salt stress. Further, it has reported that the reduction of K content in salt-tolerant wheat was lower than in salt-sensitive wheat at combined effect of eO3 and salt treatment (Zheng et al., 2014). Hence, given that DL can maintain higher K contents than JL under eO3 and salt treatment, (iii) DL may show higher tolerance to the synergic effect than JL. Based on our results, we assessed the possibility of future candidate afforestation species in northeast Asian regions.
Materials and Methods
Study Site and Materials
Experiments were conducted at the experimental nursery of the Sapporo Experimental Forest, Hokkaido University in northern Japan (43° 04' N, 141° 20' E 15 m a. s. l.). Two-year-old Dahurian larch (Larix gmelinii var. japonica; DL) and Japanese larch (Larix kaempferi; JL) seedlings were cultivated from seeds in the nursery of the Hokkaido Forestry Research Organization (HRO), Forestry Research Institute in Bibai city near Sapporo. The seedlings were transplanted into 1-L pots (Height: 114 mm, top diameter: 135 mm, bottom diameter: 95 mm) in early May 2017 before buds opened. The initial stem diameter means ± standard deviations were 1.13 ± 0.23 mm for DL and 1.32 ± 0.31 mm for JL, and the height was 8.83 ± 1.35 cm for DL and 8.55 ± 1.73 cm for JL, respectively. Soils underneath the seedlings were removed just before transplantation, with well-weathered volcanic ash soil (Kanuma and Akadama soil, 1:1, v/v) used as cultivation soil in pots. To measure the original nutrient status of the soil, ~10 g of soil and 25 ml ultrapure water were mixed and shaken for 1 h. The samples were poured through filter paper (110 mm mesh, ADVANTEC, Tokyo, Japan) and the filtrate was centrifuged at 2,000 G for 20 min. The supernatant fluid was mixed with 61% nitric acid and adjusted for the concentration of 2% nitric acid. Mineral elements contents of K, Ca, Mg, and Na were measured by ICP-MS (ELAN, DRC-e; Perkin Elmer, Waltham, MA, USA). The Na adsorption rate of this soil medium was also measured. The three levels of NaCl dissolved in demineralized water were loaded on the pot soil, which contained approximately 1,200 g of soil without seedlings, seven times during 3 weeks (110, 190, and 240 mmol Na in total). There were no leaks from the pot after each loading. After completing Na loading, the soil sample was obtained from the well-mixed and dried soil. Na content of the sample was measured by the same method described above. Na adsorption rate was calculated as the difference between total loaded Na and the water extracted Na amount. Results of the original nutrient status and the Na adsorption rate were summarized in Supplemental Data. Until treatments started, irrigation was manually carried out in the morning once every 3 or 4 days to avoid soil drying. Balanced liquid fertilizer (200 ml, 1/2,000, v/v, HYPONEX, Japan; 36.2 mg N L−1, 142 mg P L−1, 47.1 mg K L−1) was applied once every week to ensure nutrient balance (four times in total).
Experimental Design
Four treatments were totally established in this study: ambient O3 and tap water irrigation as the control (aO3+CW), elevated O3 and control water irrigation (eO3+CW), ambient O3 and salt water irrigation (aO3+SW), and elevated O3 and salt water irrigation (eO3+SW), respectively. All treatments were conducted from June 27 to September 25, 2017 before the winter season. The mean temperature was 19.6°C and precipitation was 127.38 mm during the experimental period. The open top chamber (OTC; 1.2 × 1.2 × 1.2 m) system was adopted to control the O3 concentration levels in each treatment. Seedlings were grown in OTC surrounded by a polyvinyl chloride film (Noh-bi Co. Ltd., Sapporo, Japan), such that approximately 88% sunlight could be transmitted without UVB and UVC radiation. The O3 concentration monitored is described in Table 1. More detailed descriptions of the methodology of the O3 exposure system in OTCs were provided in Sugai et al. (2019). Plants in the salt treatment were watered with 200 ml of saline water. Control plants were maintained by watering with 200 ml of tap water. The applications were performed at 3- or 4-day intervals for a total of 20 times. Mixed salt composed of alkaline salt (NaHCO3) and neutral salt (NaCl) was used to imitate the realistic extant salt-alkaline soil condition of NE China (Ge and Li, 1990; Sakai, 2012). The two salts were mixed NaHCO3:NaCl = 9:1 to make 20 mM Na+ saline water. To prevent salt leaching, rainfall was excluded by plastic shelters over the top of the OTC only during rainy days. During the final experimental period when the seedlings were harvested, 10 g of fresh soil samples were taken from the surface and bottom soils in the pots of all treatments to measure soil pH. The depth of soil sampling was set at approximately 2 cm from both the surface and bottom. The soil was shaken for 1 h, with 25 ml ion-exchanged water as soon as possible after sampling. The soil pH was measured by a portable pH meter (M-12, Horiba, Japan). All treatments were replicated four times with 16 OTCs, with a split-plot completely randomized design with four seedlings in each OTC (totally 128 seedlings).
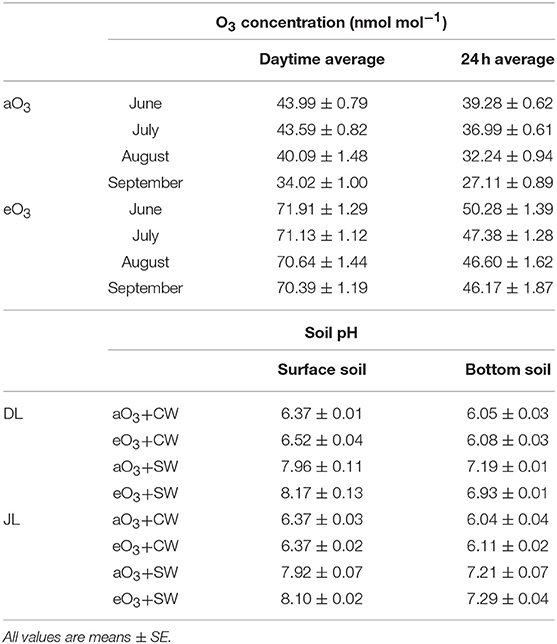
Table 1. Summary of the mean concentration at ambient (aO3) and elevated ozone (eO3) for daytime (06:00–18:00) and over 24 h in the OTC from June to September 2017, and pH in the surface and bottom soil (~2 cm layer) of Dahurian larch (DL) and Japanese larch (JL) seedlings under all treatments: ambient O3 and tap water irrigation (aO3+CW) as the control, elevated O3 and control water irrigation (eO3+CW), ambient O3 and salt water irrigation (aO3+SW), and elevated O3 and salt water irrigation (eO3+SW) in September 2017.
Growth, Leaf Mass Area, and Total Leaf Area Index
Sixty four seedlings for each species were harvested and washed with tap water. After washing, needles, branches, stems, and roots were separated. Each sample was oven dried at 70°C until a constant weight was achieved, and then weighed.
Mature needle leaves were sampled at the same shoot position near the crown in each seedling at the end of September 2017. Part of the needle samples was used to measure the leaf mass area (LMA) and the other part was used to measure the contents of several nutrient elements (see below). The projected area of fresh needles was measured by an image scanner (LIDE 200, Canon, Japan). After the projection measurement, the needle dry mass was measured by the same method as above. LMA was calculated as dry weight divided by the needle area projected.
As a species difference between two studied larches, the lower needle proportion of DL than JL has been reported (Harayama et al., 2013; Dong-Gyu et al., 2015). To evaluate these differences under eO3 and salt stress, we calculated needle dry mass divided by total dry mass as the needle ratio. While larch species have both short and long needles (Gower and Richards, 1990), the ratio of mature short needles dry mass is relatively lower than the mature long needles at a seedling stage (Powell, 1988). Based on this characteristic, we estimated the leaf area at the whole plant level as a total leaf area index (TLA) by LMA of mature needle leaves times needle dry mass per a seedling.
Maximal Photochemical Efficiency of Photosystem II
The maximal photochemical efficiency of photosystem II (Fv/Fm) was measured with a portable fluorometer (PAM-2000, Walz, Effeltrich, Germany) in September 2017. The measurement was conducted after an overnight dark adaptation to evaluate the chronic photoinhibition (Krause et al., 1995; Kitao et al., 2003). Fv/Fm was calculated with the following parameters and formula: F0 for the steady state yields, Fm for the maximum yields measured under irradiance of approximately 5,000 μmol m−2 s−1 photon flux density for 1 s, and Fv/Fm = (Fm-F0)/Fm as defined by Schreiber et al. (1986).
Nutrition and Chlorophyll Contents in Needles
Approximately 15 mg powder samples obtained from mature needles sampled at the end of September 2017 were weighed and used to measure nitrogen (N) content with a NC analyzer (Elementar, Vario EL III, Japan). Approximately 50 mg of powder samples were weighed and digested with 2 ml of 61% nitric acid (Kanto Chemical, Tokyo, Japan) at 110°C for ~2 h. A 0.5 ml solution of hydrogen peroxide was added and the sample was heated continuously at 110°C for 20 min. After cooling to room temperature, 10 ml of 2% nitric acid were added. The concentration of K, Ca, Mg, and Na in these samples was measured by ICP-MS (ELan, DRC-e; PerkinElmer, Waltham, MA, U.S.A.). Element contents were calculated as the dry weight unit and converted as the leaf area unit by LMA.
Samples were stored at −75°C after sampling and weighing. Needles were immersed in 2 ml dimethyl sulfoxide and incubated at 65°C in the dark to extract chlorophyll (Chl). Then, the extracts were measured with a spectrophotometer (Gen spec III, Hitachi, Tokyo, Japan) with two waves; 665 nm (A665) and 648 nm (A648). Chl-a and Chl-b contents were calculated according to Barnes et al. (1992) and Shinano et al. (1996). The total Chl (i.e., Chl a+b) and Chl a/b were also calculated. We also obtained ratio of total Chl/needle N content (Chl/N) as an index of allocation in foliar nitrogen (Kitaoka and Koike, 2004).
Statistical Analyses
Statistical analyses were conducted using R (R Core Team, 2017). All the data of response variables were set as the four mean values per treatment, which were obtained from four seedlings per species in each chamber (n = 4). The effects of eO3 and salt stress were determined by analysis of variance in each species (Two-way ANOVA). The relationships between needle Na and mineral element contents at all treatments in both species were analyzed using a generalized linear mix model (GLMM, n = 32). The random effect was set as the experimental unit. When species differences and their interactions were significant, correlation analysis was performed in each species (n = 16). Relationships between TLA and content of each element in needles were analyzed by the same GLMM. In each model, explanatory variables (= fixed effects) were set with each mineral element, species, and its interaction, whereas the response variables were the mean values at all treatments in both species. In all the linear analyses, the coefficient of determination (R2), P-value, and the Akaike's information criterion were calculated (AIC, Akaike, 1974). AIC is one of the most popular criterion for linear model identification and the optimal model is selected when AIC is minimized.
Results
Response to Elevated O3 and Salt Stress
No significant effects of elevated O3 (eO3) were observed on the total dry mass of DL. We did not observe significant inhibition of O3 on Fv/Fm between both larch species. However, O3 significantly increased total dry mass and TLA of only JL (P < 0.05, Figure 1). The mean value of total dry mass and TLA at eO3 were ~23 and 34% higher than at the ambient O3 condition, respectively. No significant effects of O3 were observed in other biometric (i.e., needle ratio and LMA, Figure 1) and biochemical parameters (i.e., element contents and chlorophyll in needles, Table 2).
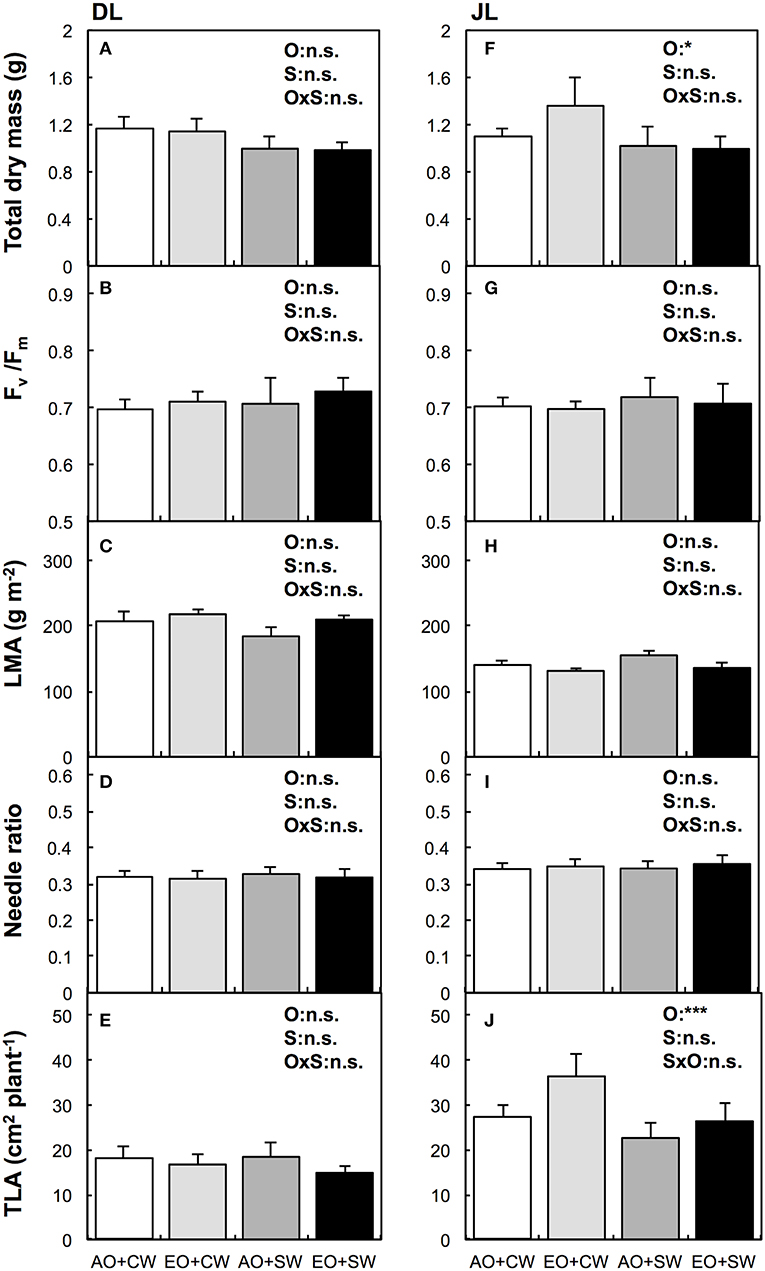
Figure 1. Biometric responses to salt treatment and elevated ozone (O3) in Dahurian larch [DL, Larix gmelinii, (A–E)] and Japanese larch [JL, Larix kaempferi, (F–J)]. White bar means ambient ozone (O3) and control tap water (aO3+CW), light gray bar means elevated O3 and control tap water (eO3+CW), dark gray bar means ambient O3 and salt water (aO3+SW), and black bar means elevated O3 and salt water (eO3+SW). The explanatory variables were set as elevated O3 (O), salt treatment (S), and its interaction (OxS). Results of Two-way ANOVA (n = 4) with statistical significant difference are shown as: ***P < 0.001, *P < 0.05, and n.s. P ≥ 0.05. Fv/Fm, the maximal photochemical efficiency of photosystem II; LMA, leaf mass area; Needle ratio, needle dry mass to total dry mass; TLA, total leaf area index.
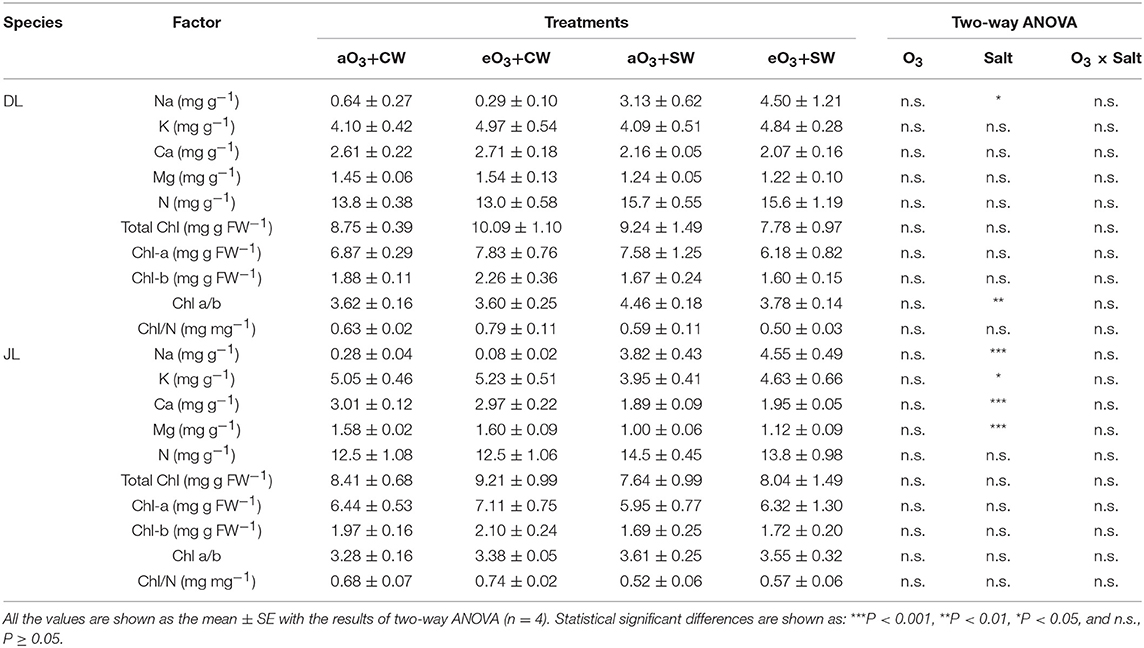
Table 2. Summary of the biochemical needle traits; mineral element contents, total chlorophyll (Chl) contents, Chl a, Chl b, the ratio of Chl a/b, and the ratio of total Chl/ needle N contents in Dahurian larch (DL) and Japanese larch (JL) seedlings at all the treatments.
While salt stress had also no significant effects on all biometric and physiological parameters of the two species, several element contents in needles were significantly changed (Table 2). Needle Na contents were significantly increased under salt stresses in both larches. In the dry mass unit, needle Na content of DL with salt treatment was approximately eight times greater than in the salt-free condition (P < 0.05) whereas Na of JL with salt treatment was at least 13 times greater (P < 0.001). Salt stress increased Chl ratio only in DL, which showed an ~14% increase in average values (P < 0.01). In contrast, salt stress significantly reduced K, Ca, and Mg contents of JL. In the dry mass unit, the K, Ca, and Mg contents of DL decreased ~17% under salt treatment (P < 0.05), 36% (P < 0.001), and 34% (P < 0.001), respectively. In the needle area unit, however, the increment of N content was observed in only JL (P < 0.05).
Relationship Between Needle Na and Other Elements
The overall relationship between Na and K contents was not significant in the larch seedlings (Figure 2). However, a significant relationship between Na and K contents was observed in JL (R2 = 0.2, P < 0.05, AIC = 48.6) but not in DL (R2 = 0.02, P = 0.33, AIC = 47.1). A significant relationship between Ca and Mg were observed in both larch species, as was a significant relationship between Na and Mg content (DL; R2 = 0.35, P < 0.01, AIC = −10.22, JL; R2 = 0.23, P < 0.05, AIC = −4.63). On the contrary, there were species differences in the relationship between Na and Ca content (P < 0.05, Figure 3). The coefficient value of Na on Ca content was ~1.8 times higher in JL than DL (Table 3). All the relationships between Na and N contents in both and each larch species were not significant (Figure 2, Table 3).
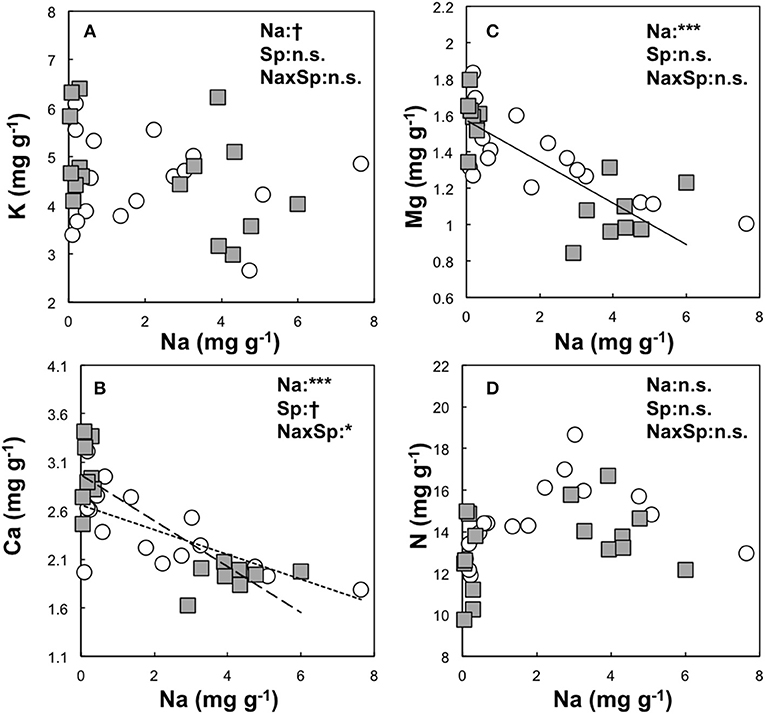
Figure 2. Relationship between the needle Na content and other element contents (K and Na: A, Ca and Na: B, Mg and Na: C, N and Na: D) of mass based unit (mg g−1) in Dahurian larch (DL, white circle) and Japanese larch (JL, gray square) were analyzed by GLMM with statistical significant difference are shown as: ***P < 0.001, *P < 0.05, and n.s. P ≥ 0.05. The explanatory variables were set as needle Na content (Na), species (Sp), and its interaction (NaxSp). The relationships between needle Na content and other element contents are shown as solid lines for both species, a fine dotted line for DL, and a heavy dotted line for JL.
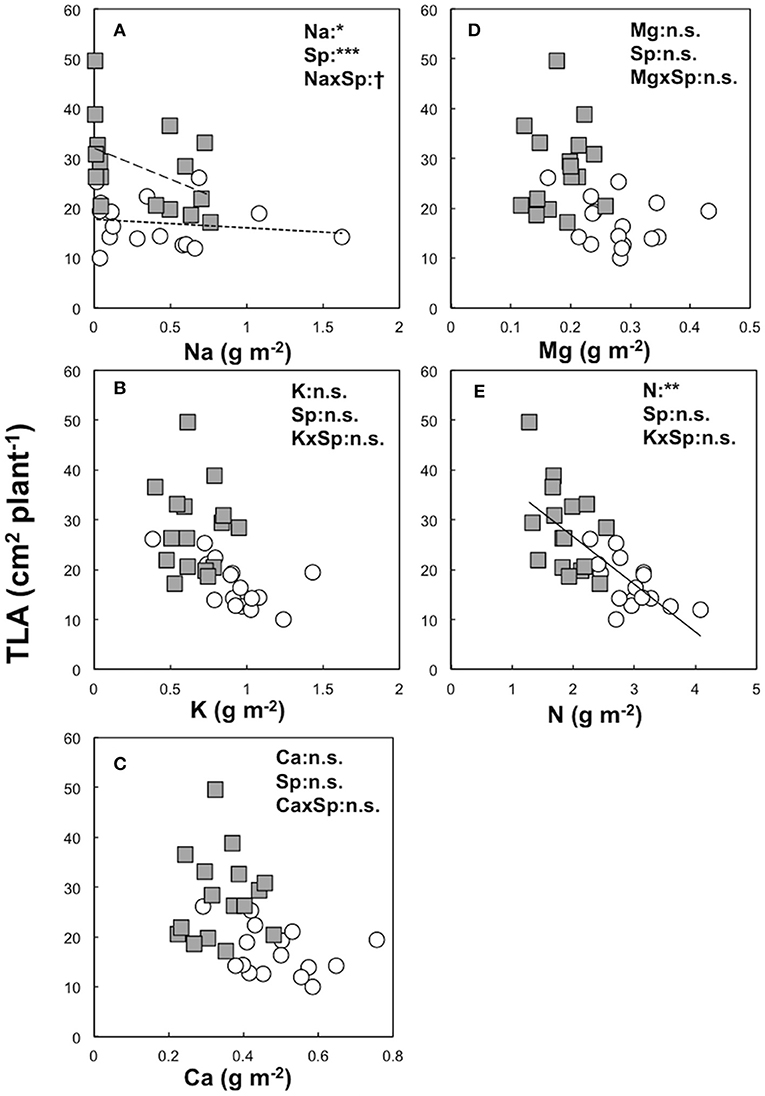
Figure 3. Relationship between the total leaf area index (TLA, cm2 plant−1) and needle element contents (TLA and Na: A, TLA and K: B, TLA and Ca: C, TLA and Mg: D, TLA and N: E) of leaf area-based unit (g m−2) in Dahurian larch (DL, white circle) and Japanese larch (JL, gray square) were analyzed by GLMM with statistical significant difference are shown as: ***P < 0.001, **P < 0.01, *P < 0.05, and n.s. P ≥ 0.05. The explanatory variables were set as each needle element content, species (Sp), and its interaction. The relationships between TLA and each element content are shown as solid lines for both species, a fine dotted line for DL, and a heavy dotted line for JL.
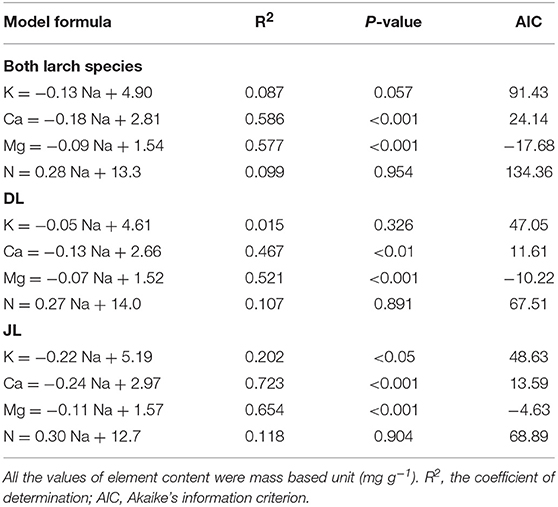
Table 3. Results of the generalized liner mixed models (GLMM) to describe the relationship between needle Na and other element contents in Dahurian larch (DL) and Japanese larch (JL).
Relationship Between TLA and Needle Mineral Elements
Table 3 shows the summary of model formulas to explain TLA by each element in needles. The best model to explain TLA of both larches determined by AIC was constructed with N (R2 = 0.54, P < 0.001, AIC = 211). This significant relationship was also observed within each species (DL; R2 = 0.35, P < 0.01, AIC = 94, JL; R2 = 0.23, P < 0.05, AIC = 116) whereas the highest R2 in model of DL was performed by K (R2 = 0.36, P < 0.01, AIC = 94) although AIC was the same value with N (Figure 3). The second best model of both larches was constructed with K (R2 = 0.28, P < 0.01, AIC = 225) while the third models contained almost equal R2 and AIC values (Ca; R2 = 0.20, P < 0.01, AIC = 229, Mg; R2 = 0.20, P < 0.01, AIC = 229). However, no significant relationships between TLA and K, Ca, and Mg content were observed in JL (Table 4). Although needle Na content was significantly correlated with TLA in both larch species (P < 0.05), the result of GLMM showed a species difference in TLA (P < 0.001). When the effects of Na contents on TLA were analyzed in each species, the significant relationship was observed only in JL (R2 = 0.20, P < 0.05, AIC = 116) but not in DL (R2 = 0.02, P = 0.28, AIC = 101).
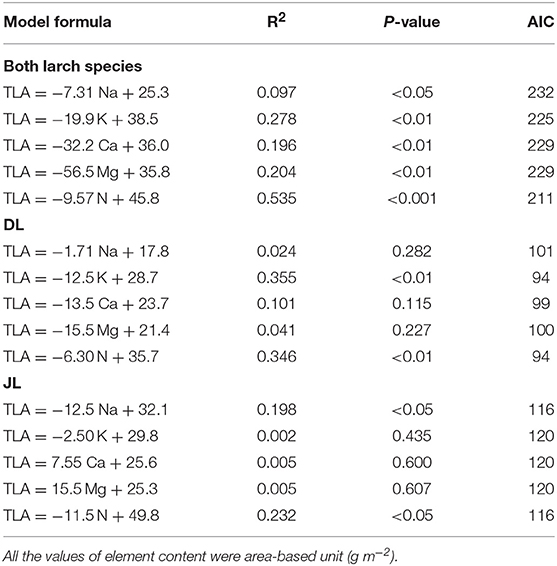
Table 4. Results of the generalized liner mixed models (GLMM) to describe the relationship between total leaf area (TLA, cm2) and needle mineral element contents in Dahurian larch (DL) and Japanese larch (JL).
Discussion
We evaluated initial growth of Dahurian larch (DL) and Japanese larch (JL) seedlings treated with the simulated eO3 and alkaline salt stress. In contrast to our expectations, eO3 did not suppress the total dry mass of both larch species. Moreover, eO3 did not induce significant nutritional imbalance in needles even with salt stress (Table 2). Elevated O3 significantly increased the dry mass of JL while we did not observe any significant inhibitions of biometric and physiological parameters, except for TLA. In fact, the similar results indicated that JL can show hormetic responses of photosynthesis rates and dry mass to mild O3 stress (Dong-Gyu et al., 2015; Sugai et al., 2018). Since leaf quantity as well as photosynthetic capacity are related to plant growth (Poorter, 1989), the dry mass increment might be related to the TLA response (Figure 1).
The best factor explaining variables of TLA was needle N contents. The results of negative correlation between TLA and N contents suggests that DL originally may not produce TLA regardless of any growth conditions as relatively higher N contents per needle area, while JL may be able to adjust producing TLA as relatively lower N contents. This result suggests that the N use efficiency in forming needles may vary between the two larches, and DL may have adaptive abilities for harsh conditions, such as low temperature and limited soil nutrient availability more than JL (Gower and Richards, 1990). Koike et al. (2012) reported that the photosynthetic N use efficiency of DL was higher (over 20%) compared to JL. Instead of these higher abilities in a needle scale, DL may be inferior to adjust TLA along with abiotic environments (Abaimov, 2010; Polezhaeva et al., 2010; Mamet et al., 2019).
In a previous study, 1-month-old saplings of European larch (Larix decidua Mill) showed a 16% lower dry mass at 150 mM NaCl compared to controls (Plesa et al., 2018). While salt treatment showed no significant suppression in any initial growth, we observed significant biochemical responses in needles (i.e., several element contents, Table 2). As expected, DL showed a relatively lower degree of needle Na content under salt stress compared to JL. The increased degree based on mean values was up to 3.5 times, indicating that the capacity to accumulate Na into needles may be higher in JL. Furthermore, DL showed no change in K contents under salt stress as compared with JL. High Na concentration can have negative effects on physiological functions due to the inhibition of enzyme activities and K uptakes (Marschner, 2012; Polle and Chen, 2015). K has an outstanding role in plant-water relations, contributing to the osmotic potential of cells and tissues (Marschner, 2012). Reductions of K contents under salt stress in JL, therefore, supported this competitive absorption with Na. However, the salt effect on needle K content was not significant in DL. Previous studies have also reported stable values of K of other Larix species (e.g., Larix laricina Du Roi; Renault, 2005, L. decidua Mill; Plesa et al., 2018). The K homeostasis can be accomplished by cation selectivity in uptake systems from the cytoplasm into vacuoles. Plesa et al. (2018) have pointed out that needle K content of L. decidua increased under salt treatment. Since we did not observe any increments of K contents across all treatments, future investigations should compare the specific mechanism differences of K homeostasis within the genus Larix.
We observed homeostasis of other mineral contents. Regression analysis showed negative correlations between needle Na and Ca, and between Na and Mg contents in both larches and each larch seedlings (Figure 2), while significant salt effects were only observed in JL (Table 2). In particular, we observed significant species differences regarding the relationship between needle Na and Ca contents, suggesting that the Na sensitivity to needle Ca contents was higher in JL than DL. Ca plays an important role in the cell membrane, cell wall stabilization, signaling functions, and the mitigation of salinity (Köster et al., 2019). These results supported our hypothesis that salt tolerance would be greater in DL, and that mineral homeostasis was related to other mineral elements apart from K. DL may obtain the adaptive capacity to salt stress as the original distribution of the species where the primary salinization is likely to occur cause to drought condition (Polezhaeva et al., 2010; Mamet et al., 2019). In contrast, JL is naturally distributed in central Japan. Species differences in adaptive traits, including salt tolerance, may be induced by the geographical isolation from other continental Larix species in the interglacial period (Polezhaeva et al., 2010)
While we hypothesized that the increment of N content via the synergy with K+ uptake would be either a species specific responses or related to osmotic regulations, we did not observe significant changes in needle N content, except for the area-based value of JL (Table 2). However, we observed higher Chl a/b under salt stress in DL, but not in JL. In tamarack saplings (L. laricina Du Roi), 60 mM NaCl reduced the total Chl and the Chl a/b (Renault, 2005). However, in L. decidua, 150 mM NaCl reduced the total Chl, while the Chl a/b did not change (Plesa et al., 2018). A high value of Chl a/b under salt treatment was previously reported in herbaceous plants, including sunflower (Helianthus annuus L., Liu and Shi, 2010) and wheat (Triticum aestivum, Yang et al., 2008), while a lower Chl a/b was reported in poplar (Populus alba x P. berolinensis, Wang et al., 2013). Given the preferential reduction in Chl-b than Chl-a under salt stresses, it may act to protect the reaction center core more than the light harvesting capacity related to Chl-b. Future studies should continue to investigate N metabolism, with the consideration that this process may be related closely to Chl metabolic regulation (e.g., Yang et al., 2008).
Irrespective of our hypothesis, we observed no clear combined effect of eO3 and salt stress on physiological parameters in larch seedlings (Table 2). Therefore, even if we employ these larch species to manage soil salinization under eO3, their initial growth may not be suppressed by eO3 and salinity stress. Non-significant changes of needle element contents indicated that the element variables were maintained even under the combined effect. These results suggested that eO3 may not inhibit the element homeostasis of larch species, especially in DL. This may be attributed to non-significant changes of the total dry mass in DL treated with single O3 and the combined effect. It was reported that the stable P and iron (Fe) contents in leaves implied homeostatic readjustment, contributing to preventing physiological inhibition of eO3 (Agathokleous et al., 2018). It is possible that the combined effect, and even single O3 effect, were not detected due to the physiological status of seedlings, which were shown in Fv/Fm (Figure 1). In all treatments, Fv/Fm showed almost the same value from 0.70 to 0.72, which were significantly lower than the standard normal value of health condition ranged between 0.81 and 0.83 (Schreiber et al., 1986).
We conclude that the effects of eO3 and alkaline salt stress did not suppress the total dry mass of both larch species. Although we did not find any specific factors explaining species difference in O3 sensitivities between species, TLA may be involved. Stress adaptive capacity to salt was higher in DL than JL, suggesting that salt treatment did not reduce needle K content in DL, and DL also maintained Ca and Mg contents in needles. Specifically, DL may show the greater salt tolerance to the mineral homeostatic capacity even under eO3. Further study should evaluate the relationship between the stomatal responses and K homeostasis under eO3 and salt stress, which may reveal how larch achieves the optimal nutrient balance for these stress resistances. Our results suggest that L. gmelinii seedlings may be future candidates for afforestation, even under eO3 condition at saline soils.
Data Availability
All datasets generated for this study are included in the manuscript/Supplementary Files.
Author Contributions
TS and WY were the same contribution to the planning and working. TS primarily worked on drafting and editing the manuscript with figures and tables. WY performed cultivation and measurements at the field. FS had responsible for managing the experimental fields. All other co-authors (TW, LQ, and TK) equally contributed to funding, manuscript writing, and revision.
Funding
This work Ecological impacts of ozone pollution: monitoring, assessment, and mitigation was supported by the National Key Research and Development Program of China (2017YFE0127700), JST-SICORP-No. 10 and JSPS KAKENHI Grant no. 18J2031908.
Conflict of Interest Statement
The authors declare that the research was conducted in the absence of any commercial or financial relationships that could be construed as a potential conflict of interest.
Acknowledgments
We thank Dr. K. Kita of the Forestry Research Institute for providing planting stocks of three larch species seedlings. We gratefully acknowledge late Mr. K. Ichikawa and the staff of Hokkaido University Forests for planning and maintaining the free air O3 fumigation system.
Supplementary Material
The Supplementary Material for this article can be found online at: https://www.frontiersin.org/articles/10.3389/ffgc.2019.00053/full#supplementary-material
References
Abaimov, A. P. (2010). “Geographical distribution and genetics of Siberian larch species,” in Permafrost Ecosystems, eds A. Osawa, O. A. Zyryanova, Y. Matsuura, T, Kajimoto, and R. W. Wein (Dordrecht: Springer, 41–58.
Agathokleous, E., Kitao, M., Qingnan, C., Saitanis, C. J., Paoletti, E., Manning, W. J., et al. (2018). Effects of ozone (O3) and ethylenediurea (EDU) on the ecological stoichiometry of a willow grown in a free-air exposure system. Environ. Pollut. 238, 663–676. doi: 10.1016/j.envpol.2018.03.061
Agathokleous, E., Saitanis, C. J., and Koike, T. (2015). Tropospheric O3, the nightmare of wild plants: a review study. J. Agric. Meteor. 71, 142–152. doi: 10.2480/agrmet.D-14-00008
Akaike, H. (1974). “A new look at the statistical model identification,” in Selected Papers of Hirotugu Akaike. Springer Series in Statistics (Perspectives in Statistics), eds E. Parzen, K. Tanabe, and G. Kitagawa (New York, NY: Springer, 215–222.
Akimoto, H., Mori, Y., Sasaki, K., Nakanishi, H., Ohizumi, T., and Itano, Y. (2015). Analysis of monitoring data of ground-level ozone in Japan for long-term trend during 1990–2010: causes of temporal and spatial variation. Atmos. Environ. 102, 302–310. doi: 10.1016/j.atmosenv.2014.12.001
Alcántara, E., de la Guardia, M. D., Montilla, I., and Romera, F. J. (2006). Interaction between ozone exposure and iron nutrition in two tobacco cultivars. J. Plant. Nutr. 29, 2245–2255. doi: 10.1080/01904160600975228
Alexeyev, V. A., Birdsey, R. A., Stakanov, V. D., and Korotkov, I. A. (2000). “Carbon storage in the Asian boreal forests of Russia,” in Fire, Climate Change, and Carbon Cycling in the Boreal Forest, eds S. E. Kasischke and J. B. Stocks (Springer), 239–257.
Anschütz, U., Becker, D., and Shabala, S. (2014). Going beyond nutrition: regulation of potassium homeostasis as a common denominator of plant adaptive responses o environment. J. Plant. Physiol. 171, 670–687. doi: 10.1016/j.jplph.2014.01.009
Barnes, J. D., Balaguer, L., Manrique, E., Elvira, S., and Davison, A. W. (1992). A reappraisal of the use of DMSO for the extraction and determination of chlorophylls a and b in lichens and higher plants. Environ. Exp. Bot. 32, 85–100. doi: 10.1016/0098-8472(92)90034-Y
Barnes, J. D., Pfirrmann, T., Steiner, K., Lütz, C., Busch, U., Küchenhoff, H., et al. (1995). Effects of elevated CO2, elevated O3 and potassium deficiency on Norway spruce [Picea abies (L) Karst.]: seasonal changes in photosynthesis and non-structural carbohydrate content. Plant Cell Environ. 18, 1345–1457. doi: 10.1111/j.1365-3040.1995.tb00195.x
Calzone, A., Podda, A., Lorenzini, G., Maserti, B. E., Carrari, E., Deleanu, E., et al. (2019). Cross-talk between physiological and biochemical adjustments by Punica granatum cv. Dente di cavallo mitigates the effects of salinity and ozone stress. Sci. Total. Environ. 656, 589–597. doi: 10.1016/j.scitotenv.2018.11.402
Cao, J., Shang, H., Chen, Z., Tian, Y., and Yu, H. (2016). Effects of elevated ozone on stoichiometry and nutrient pools of Phoebe bournei (Hemsl.) Yang and Phoebe zhennan S. Lee et FN Wei seedlings in subtropical China. Forests 7:78. doi: 10.3390/f7040078
Chazdon, R. L. (2008). Beyond deforestation: restoring forests and ecosystem services on degraded lands. Science 320, 1458–1460. doi: 10.1126/science.1155365
Chen, W., Tang, H. Z., and Zhao, H. M. (2015). Diurnal, weekly and monthly spatial variations of air pollutants and air quality of Beijing. Atmos. Environ. 119, 21–34. doi: 10.1016/j.atmosenv.2015.08.040
Dong-Gyu, K., Shi, C., Watanabe, M., Kita, K., Satoh, F., and Koike, T. (2015). Growth of Japanese and hybrid larch seedlings grown under free-air O3fumigation—an initial assessment of the effects of adequate and excessive nitrogen. J. Agr. Met. 71, 239–244. doi: 10.2480/agrmet.D-14-00029
Fang, J. Y., Guo, Z. D., Hu, H. F., Kato, T., Muraoka, H., and Son, Y. (2014). Forest biomass carbon sinks in East Asia, with special reference to the relative contributions of forest expansion and forest growth. Glob. Change Biol. 20, 2019–2030. doi: 10.1111/gcb.12512
Feng, Z., Yang, Y., Zhang, Y., Zhang, P., and Li, Y. (2005). Grain-for-green policy and its impacts on grain supply in West China. Land Use Policy 22, 301–312. doi: 10.1016/j.landusepol.2004.05.004
Feng, Z. Z., Paoletti, E., Bytnerowicz, A., and Harmens, H. (2015). Ozone and plants. Environ. Pollut. 202, 215–216. doi: 10.1016/j.envpol.2015.02.004
Flowers, T. J. (2004). Improving crop salt tolerance. J. Exp. Bot. 55, 307–319. doi: 10.1093/jxb/erh003
Gaudel, A., Cooper, O. R., Ancellet, G., Barret, B., Boynard, A., Burrows, J. P., et al. (2018). Tropospheric Ozone Assessment Report: Present-day distribution and trends of tropospheric ozone relevant to climate and global atmospheric chemistry model evaluation. Sci. Anthropocene 6, 1–58. doi: 10.1525/elementa.291
Ge, Y., and Li, J. D. (1990). A preliminary study on the effects of halophytes on salt accumulation and desalination in the soil of Songnen Plain, Northeast China. Acta. Pratacult. Sinica 1, 70–76.
Gerosa, G., Marzuoli, R., Finco, A., Monga, R., Fusaro, I., and Faoro, F. (2014). Contrasting effects of water salinity and ozone concentration on two cultivars of durum wheat (Triticum durum Desf.) in Mediterranean conditions. Environ. Pollut. 193, 13–21. doi: 10.1016/j.envpol.2014.05.027
Gielen, B., Löw, M., Deckmyn, G., Metzger, U., Franck, F., Heerdt, C., et al. (2006). Chronic ozone exposure affects leaf senescence of adult beech trees: a chlorophyll fluorescence approach. J. Exp. Bot. 58, 785–795. doi: 10.1093/jxb/erl222
Gower, S. T., and Richards, J. H. (1990). Larches: deciduous conifers in an evergreen world. BioScience 40, 818–826. doi: 10.2307/1311484
Guidi, L., Remorini, D., Cotrozzi, L., Giordani, T., Lorenzini, G., Massai, R., et al. (2016). The harsh life of an urban tree: the effect of a single pulse of ozone in salt-stressed Quercus ilex saplings. Tree Physiol. 37, 246–260. doi: 10.1093/treephys/tpw103
Harayama, H., Ohno, Y., Uemura, A., Kitaoka, S., Utsugi, H., and Kita, K. (2013). Seasonal variations in photosynthetic properties in saplings of three Larix spp. Boreal. For. Soc. 61, 57–58. doi: 10.24494/jfsh.61.0_57
Hu, E., Gao, F., Xin, Y., Jia, H., Li, K., Hu, J., et al. (2015). Concentration-and flux-based ozone dose–response relationships for five poplar clones grown in North China. Environ. Pollut. 207, 21–30. doi: 10.1016/j.envpol.2015.08.034
Keller, T., and Matyssek, R. (1990). Limited compensation of ozone stress by potassium in Norway spruce. Environ. Pollut. 67, 1–14. doi: 10.1016/0269-7491(90)90168-C
Kitao, M., Utsugi, H., Kuramoto, S., Tabuchi, R., Fujimoto, K., and Lihpai, S. (2003). Light-dependent photosynthetic characteristics indicated by chlorophyll fluorescence in five mangrove species native to Pohnpei Island, Micronesia. Physiol. Plant. 117, 376–382. doi: 10.1034/j.1399-3054.2003.00042.x
Kitaoka, S., and Koike, T. (2004). Invasion of broad-leaf tree species into a larch plantation: seasonal light environment, photosynthesis and nitrogen allocation. Physiol. Plant. 121, 604–611. doi: 10.1111/j.1399-3054.2004.00359.x
Koike, T., Mao, Q., Inada, N., Kawaguchi, K., Hoshika, Y., Kita, K., et al. (2012). Growth and photosynthetic responses of cuttings of a hybrid larch (Larix gmelinii var. japonica x L. kaempferi) to elevated ozone and/or carbon dioxide. Asian. J. Atmos. Environ. 6, 104–110. doi: 10.5572/ajae.2012.6.2.104
Koike, T., Watanabe, M., Hoshika, Y., Kitao, M., Matsumura, H., Funada, R., et al. (2013). Effects of ozone on forest ecosystems in East and Southeast Asia. Dev. Environ. Sci. 13, 371–390. doi: 10.1016/B978-0-08-098349-3.00017-7
Köster, P., Wallrad, L., Edel, K. H., Faisal, M., Alatar, A. A., and Kudla, J. (2019). The battle of two ions: signalling against Na+ stress. Plant Bio. 21, 39–48. doi: 10.1111/plb.12704
Krause, G. H., Virgo, A., and Winter, K. (1995). High susceptibility to photoinhibition of young leaves of tropical forest trees. Planta 197, 583–591. doi: 10.1007/BF00191564
Lee, D. K., Kang, H. S., and Park, Y. D. (2004). Natural restoration of deforested woodlots in South Korea. For. Ecol. Manag. 201, 23–32. doi: 10.1016/j.foreco.2004.06.019
Lee, J. B., Cha, J. S., Hong, S. C., Choi, J. Y., Myoung, J. S., Park, R. J., et al. (2015). Projections of summertime ozone concentration over East Asia under multiple IPCC SRES emission scenarios. Atmos. Environ. 106, 335–346. doi: 10.1016/j.atmosenv.2015.02.019
Li, J., Pu, L., Han, M., Zhu, M., Zhang, R., and Xiang, Y. (2014). Soil salinization research in China: advances and prospects. J. Geographic. Sci. 24, 943–960. doi: 10.1007/s11442-014-1130-2
Liu, J., and Shi, D. C. (2010). Photosynthesis, chlorophyll fluorescence, inorganic ion and organic acid accumulations of sunflower in responses to salt and salt-alkaline mixed stress. Photosynthetica 48, 127–134. doi: 10.1007/s11099-010-0017-4
Ma, Z., and Fu, C. (2006). Some evidence of drying trend over northern China from 1951 to 2004. Chin. Sci. Bull. 51, 2913–2925. doi: 10.1007/s11434-006-2159-0
Mamet, S. D., Brown, C. D., Trant, A. J., and Laroque, C. P. (2019). Shifting global Larix distributions: northern expansion and southern retraction as species respond to changing climate. J. Biogeogr. 46, 30–44. doi: 10.1111/jbi.13465
Mao, Q., Watanabe, M., and Koike, T. (2010). Growth characteristics of two promising tree species for afforestation, birch and larch in the northeastern part of Asia. Eurasian J. For. Res. 13, 69–76. Available online at: http://hdl.handle.net/2115/44672
Marschner, H. (2012). “Adaptation of plants to adverse chemical soil conditions,” in Marschner's Mineral Nutrition of Higher Plants, 3rd Edn, ed P. Marschner (San Diego, CA: Academic Press, 455–472.
Matyssek, R., Wieser, G., Calfapietra, C., De Vries, W., Dizengremel, P., Ernst, D., et al. (2012). Forests under climate change and air pollution: gaps in understanding and future directions for research. Environ. Pollut. 160, 57–65. doi: 10.1016/j.envpol.2011.07.007
Matyssek, R., Wieser, G., Nunn, A. J., Kozovits, A. R., Reiter, I. M., Heerdt, C., et al. (2004). Comparison between AOT40 and ozone uptake in forest trees of different species, age and site conditions. Atmos. Environ. 38, 2271–2281. doi: 10.1016/j.atmosenv.2003.09.078
Munns, R., James, R. A., and Läuchli, A. (2006). Approaches toincreasing the salt tolerance of wheat and other cereals. J. Exp. Bot. 57, 1025–1043. doi: 10.1093/jxb/erj100
Paoletti, E., Bytnerowicz, A., Andersen, C., Augustaitis, A., Ferretti, M., Grulke, N., et al. (2007). Impacts of air pollution and climate change on forest ecosystems—emerging research needs. ScientificWorldJournal 7, 1–8. doi: 10.1100/tsw.2007.52
Paoletti, E., Schaub, M., Matyssek, R., Wieser, G., Augustaitis, A., Bastrup-Birk, A. M., et al. (2010). Advances of air pollution science: from forest decline to multiple-stress effects on forest ecosystem services. Environ. Pollut. 158, 1986–1989. doi: 10.1016/j.envpol.2009.11.023
Plesa, I., González-Orenga, S., Al Hassan, M., Sestras, A., Vicente, O., Prohens, J., et al. (2018). Effects of drought and salinity on European Larch (Larix decidua Mill.) seedlings. Forests 9:320. doi: 10.3390/f9060320
Polezhaeva, M. A., Lascoux, M., and Semerikov, V. L. (2010). Cytoplasmic DNA variation and biogeography of Larix Mill. in Northeast Asia. Mol. Eco. 19, 1239–1252. doi: 10.1111/j.1365-294X.2010.04552.x
Polle, A., and Chen, S. (2015). On the salty side of life: molecular, physiological and anatomical adaptation and acclimation of trees to extreme habitats. Plant. Cell Environ. 38, 1794–1816. doi: 10.1111/pce.12440
Poorter, H. (1989). Plant growth analysis: towards a synthesis of the classical and the functional approach. Physiol. Plant. 75, 237–244. doi: 10.1111/j.1399-3054.1989.tb06175.x
Powell, G. R. (1988). Shoot elongation, leaf demography and bud formation in relation to branch position on Larix laricina saplings. Trees 2, 150–164. doi: 10.1007/BF00196020
R Core Team (2017). R: A Statistical Computing. Available online at: https://www.R-project.org (accessed August 10, 2019).
Reilly, J., Paltsev, S., Felzer, B., Wang, X., Kicklighter, D., Melillo, J., et al. (2007). Global economic effects of changes in crops, pasture, and forests due to changing climate, carbon dioxide, and ozone. Energy Policy 35, 5370–5383. doi: 10.1016/j.enpol.2006.01.040
Renault, S. (2005). Tamarack response to salinity: effects of sodium chloride on growth and ion, pigment, and soluble carbohydrate levels. Can. J. For. Res. 35, 2806–2812. doi: 10.1139/x05-194
Ryu, K., Watanabe, M., Shibata, H., Takagi, K., Nomura, M., and Koike, T. (2009). Ecophysiological responses of the larch species in northern Japan to environmental changes as a base of afforestation. Landsc. Ecol. Eng. 5, 99–106. doi: 10.1007/s11355-009-0063-x
Sakai, Y. (2012). Approach for salt-affected soil amelioration by desulfurization of by-products in China and its future perspectives. Bull. Soc. Sea Water Sci. Jpn. 66, 66–73. doi: 10.11457/swsj.66.66
Schreiber, U., Schliwa, U., and Bilger, W. (1986). Continuous recording of photochemical and non-photochemical chlorophyll fluorescence quenching with a new type of modulation fluorometer. Photosyn. Res. 10, 51–62. doi: 10.1007/BF00024185
Shabala, S., and Cuin, T. A. (2007). Potassium transport and plant salt tolerance. Physiol. Plant. 133, 651–669. doi: 10.1111/j.1399-3054.2007.01008.x
Shang, B., Feng, Z., Li, P., and Calatayud, V. (2018). Elevated ozone affects C, N and P ecological stoichiometry and nutrient resorption of two poplar clones. Environ. Pollut. 234, 136–144. doi: 10.1016/j.envpol.2017.11.056
Shi, C., Watanabe, T., and Koike, T. (2017). Leaf stoichiometry of deciduous tree species in different soils exposed to free-air O3 enrichment over two growing seasons. Environ. Exp. Bot. 138, 148–163. doi: 10.1016/j.envexpbot.2017.03.012
Shi, F., Sasa, K., and Koike, T. (2010). “Characteristics of larch forests in Daxingan mountains, Northeast China,” Permafrost Ecosystems, eds. A. Osawa, O. A. Zyryanova, Y. Matsuura, T, Kajimoto, and R. W. Wein (Dordrecht: Springer, 367–383.
Shinano, T., Lei, T. T., Kawamukai, T., Inoue, M. T., Koike, T., and Tadano, T. (1996). Dimethyl sulfoxide method for the extraction of chlorophylls a and b from the leaves of wheat, field bean, dwarf bamboo, and oak. Photosynthetica 32, 409–415.
Sicard, P., Augustaitis, A., Belyazid, S., Calfapietra, C., de Marco, A., Fenn, M., et al. (2016). Global topics and novel approaches in the study of air pollution, climate change and forest ecosystems. Environ. Pollut. 213, 977–987. doi: 10.1016/j.envpol.2016.01.075
Sicard, P., and Dalstein-Richier, L. (2015). Health and vitality assessment of two common pine species in the context of climate change in southern Europe. Environ. Res. 137, 235–245. doi: 10.1016/j.envres.2014.12.025
Sugai, T., Kam, D. G., Agathokleous, E., Watanabe, M., Kita, K., and Koike, T. (2018). Growth and photosynthetic response of two larches exposed to O3 mixing ratios ranging from preindustrial to near future. Photosynthetica 56, 901–910. doi: 10.1007/s11099-017-0747-7
Sugai, T., Watanabe, T., Kita, K., and Koike, T. (2019). Nitrogen loading increases the ozone sensitivity of larch seedlings with higher sensitivity to nitrogen loading. Sci. Total. Environ. 663, 587–595. doi: 10.1016/j.scitotenv.2019.01.292
Sun, Y., Zhang, X., Zwiers, F. W., Song, L., Wan, H., and Hu, T. (2014). Rapid increase in the risk of extreme summer heat in Eastern China. Nat. Clim. Change 4, 1082–1085. doi: 10.1038/nclimate2410
Tester, M., and Davenport, R. (2003). Na1tolerance and Na1transport in higher plants. Ann. Bot. 91, 503–527. doi: 10.1093/aob/mcg058
Turnock, S. T., Wild, O., Dentener, F. J., Davila, Y., Emmons, L. K., Flemming, J., et al. (2018). The impact of future emission policies on tropospheric ozone using a parameterized approach. Atmos. Chem. Phys. 18, 8953–8978. doi: 10.5194/acp-18-8953-2018
Volkov, V., Wang, B., Dominy, P. J., Fricke, W., and Amtmann, A. (2003). Thellungiella halophila, a salt-tolerant relative of Arabidopsis thaliana, possesses effective mechanisms to discriminate between potassium and sodium. Plant. Cell Environ. 27, 1–14. doi: 10.1046/j.0016-8025.2003.01116.x
Wang, C., Gower, S. T., Wang, Y., Zhao, H., Yan, P., and Bond-Lamberty, B. P. (2001). The influence of fire on carbon distribution and net primary production of boreal Larix gmelinii forests in north-eastern China. Glob. Change Biol. 7, 719–730. doi: 10.1046/j.1354-1013.2001.00441.x
Wang, H. M., Wang, W. J., Wang, H. Z., Wang, Y., Xu, H. N., and Zu, Y. G. (2013). Effect of inland salt-alkaline stress on C4 enzymes, pigments, antioxidant enzymes, and photosynthesis in leaf, bark, and branch chlorenchyma of poplars. Photosynthetica 51, 115–126. doi: 10.1007/s11099-013-0008-3
Wang, L., Seki, K., Miyazaki, T., and Ishihama, Y. (2009). The causes of soil alkalinization in the Songnen Plain of Northeast China. Paddy Water Environ. 7, 259–270. doi: 10.1007/s10333-009-0166-x
Wang, M., Zheng, Q., Shen, Q., and Guo, S. (2014). The critical role of potassium in plant stress response. Int. J. Mol. Sci. 14, 7370–7390. doi: 10.3390/ijms14047370
Wungrampha, S., Joshi, R., Singla-Pareek, S. L., and Pareek, A. (2018). Photosynthesis and salinity: are these mutually exclusive? Photosynthetica 56, 366–381. doi: 10.1007/s11099-017-0763-7
Xu, J. (2011). China's new forests aren't as green as they seem. Nat. News 477, 371–371. doi: 10.1038/477371a
Xu, J., and Wilkes, A. (2004). Biodiversity impact analysis in northwest Yunnan, southwest China. Biodivers. Conserv. 13, 959–983. doi: 10.1023/B:BIOC.0000014464.80847.02
Yang, C. W., Wang, P., Li, C. Y., Shi, D. C., and Wang, D. L. (2008). Comparison of effects of salt and alkali stresses on the growth and photosynthesis of wheat. Photosynthetica 46, 107–114. doi: 10.1007/s11099-008-0018-8
Yuan, X., Calatayud, V., Gao, F., Fares, S., Paoletti, E., Tian, Y., et al. (2016). Interaction of drought and ozone exposure on isoprene emission from extensively cultivated poplar. Plant. Cell Environ. 39, 2276–2287. doi: 10.1111/pce.12798
Zhang, J. T., and Zhang, F. (2007). Diversity and composition of plant functional groups in mountain forests of the Lishan Nature Reserve, North China. Bot. Stud. 48, 339–348. Available online at: http://www.airitilibrary.com/Publication/alDetailedMesh?docid=1817406X-200707-48-3-339-348-a
Zhang, P., Shao, G., Zhao, G., Le Master, D. C., Parker, G. R., Dunning, J. B., et al. (2000). China's forest policy for the 21st century. Science 288, 2135–2136. doi: 10.1126/science.288.5474.2135
Zhang, S. W., Yang, J. C., Li, Y., Zhang, Y. Z., and Chang, L. P. (2010). Changes of saline-alkali land in Northeast China and its causes since the mid-1950s. J. Nat. Res. 125, 435–442. doi: 10.11849/zrzyxb.2010.03.009
Zheng, Y., Cheng, D., and Simmons, M. (2014). Ozone pollution effects on gas exchange, growth and biomass yield of salinity-treated winter wheat cultivars. Sci. Total Environ. 499, 18–26. doi: 10.1016/j.scitotenv.2014.08.049
Keywords: combined effect, salt stress, larch, interspecific differences, homeostasis
Citation: Sugai T, Yannan W, Watanabe T, Satoh F, Qu L and Koike T (2019) Salt Stress Reduced the Seedling Growth of Two Larch Species Under Elevated Ozone. Front. For. Glob. Change 2:53. doi: 10.3389/ffgc.2019.00053
Received: 25 June 2019; Accepted: 27 August 2019;
Published: 18 September 2019.
Edited by:
Elena Paoletti, Italian National Research Council (CNR), ItalyReviewed by:
Lina Fusaro, Sapienza University of Rome, ItalyAntonella Calzone, University of Pisa, Italy
Copyright © 2019 Sugai, Yannan, Watanabe, Satoh, Qu and Koike. This is an open-access article distributed under the terms of the Creative Commons Attribution License (CC BY). The use, distribution or reproduction in other forums is permitted, provided the original author(s) and the copyright owner(s) are credited and that the original publication in this journal is cited, in accordance with accepted academic practice. No use, distribution or reproduction is permitted which does not comply with these terms.
*Correspondence: Toshihiro Watanabe, nabe@chem.agr.hokudai.ac.jp; Laiye Qu, lyqu@rcees.ac.cn
†These authors have contributed equally to this work
‡Present address: Wang Yannan, Jiangxi Academy of Forestry, Nanchang, China