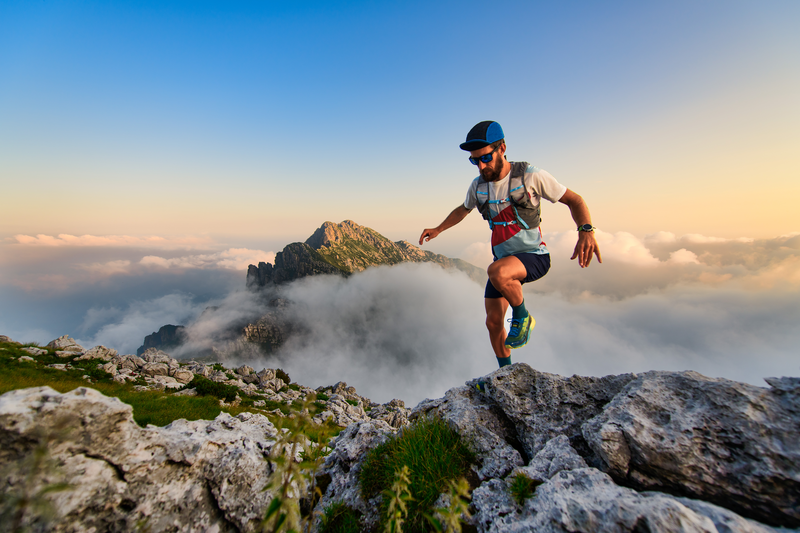
94% of researchers rate our articles as excellent or good
Learn more about the work of our research integrity team to safeguard the quality of each article we publish.
Find out more
ORIGINAL RESEARCH article
Front. Ecol. Evol. , 19 October 2020
Sec. Biogeography and Macroecology
Volume 8 - 2020 | https://doi.org/10.3389/fevo.2020.556856
This article is part of the Research Topic Adaptation of Invasive Species to Islands and the Puerto Rican Honey Bee View all 14 articles
The honey bee (Apis mellifera) is an important pollinator as well as an important test model for pesticide effects on other insect pollinators. Honey bees have been experiencing high mortality in both the United States and worldwide. Pesticide exposure has been identified as one of the many stressors causing this mortality. Effects of various pesticides have been measured for multiple responses such as learning, memory performance, feeding activity, and thermoregulation. These studies were conducted at many different temperatures (11–35°C); however, few studies compared toxicity of the same pesticide to bees at different temperatures. It is possible that the same pesticide might show different toxicity to honey bees at different temperatures. To reveal such potential interactions, we administered low doses of two neonicotinoid insecticides (imidacloprid and thiamethoxam) at three different temperature scenarios (35°C, 24°C, and a varying temperature) and determined the effects on honey bee survivorship. We discovered that honey bees are much more sensitive to the neonicotinoid pesticides imidacloprid and thiamethoxam at a constant 24°C or at a varying temperature (night at 13°C and day at 24°C) compared to bees at 35°C. These results suggest that honey bee colonies during winter time will be more sensitive to pesticides. Doses of neonicotinoids that are safe to colonies during summer might kill them during the winter time.
Pollinators are responsible for the transfer of pollen between flowers, helping fruit and seed production in approximately 88% of flowering plants (Ollerton et al., 2011). Whereas a wide variety of animal taxa can work as pollinators (Ollerton, 2017), honey bees (Apis mellifera) are highly efficient in pollen transfer and are used for the majority of pollination services in both cultivated and wild plants (Willmer et al., 2017).
Pesticides are widely used in agriculture, but they can also be devastating to the health of many non-target organisms (Desneux et al., 2007; Schäfer et al., 2012). Recently, great attention has been paid to the effects of neonicotinoid pesticides on honey bees and their potential role in harming the health of honey bee colonies all over the world (Matsumoto, 2013). These systemic insecticides are strong agonists of the nicotinic acetylcholine receptors (nAChR), mainly circulated in the insect central nervous system, and can interrupt processes related to cholinergic neurotransmission, such as olfaction, learning, and memory (Jones et al., 2006; Williamson and Wright, 2013). Currently, 30% of the insecticides used worldwide are neonicotinoids (Simon-Delso et al., 2015), and honey bees are exposed to neonicotinoids both in the field and inside the hive (Lambert et al., 2013). The sublethal effects of neonicotinoids on honey bees have been extensively studied at many different physiological levels (Aliouane et al., 2009; Henry et al., 2012; Catae et al., 2014; Oliveira et al., 2014; Alburaki et al., 2015), but whether this toxicity interacts with low temperature has not yet been explored.
Honey bees are distributed in a wide geographic range, with greatly differing climatic conditions (Ruttner, 1988). This wide range includes many areas with cold and challenging winters. Successful overwintering of honey bee colonies is crucial to meet the pollination requirements of early spring blossoming crops like as cherries, apples, and almonds (Doeke et al., 2015).
Honey bees survive cold winters through the development of a special type of bees called “winter bees” (Maurizio, 1950; Münch and Amdam, 2010). Winter bees have vital endocrine and metabolic changes that differ from summer adult worker honey bees, which may increase their lifespan by 6–8 times (Huang and Wang, 2015). Numerous studies have found that winter bees have low levels of juvenile hormone as compared to summer bees and high levels of vitellogenin and total proteins in the hemolymph (Fluri et al., 1982; Huang and Robinson, 1995; Hartfelder and Engels, 1998); reduced protein synthesis, transportation through the midgut, and catabolism (Crailsheim, 1990; Haszonits and Crailsheim, 1990); and lesser activity of monooxygenase enzymes that are essential for detoxification of pesticides (Smirle and Winston, 1987). Yet, it remains ambiguous how the physiological variations between summer and winter workers change pesticide sensitivity. One study showed that winter bees are less sensitive to a fungicide (imidazole) and an insecticide (pyrethroid) (Meled et al., 1998), but another one showed higher sensitivity in winter bees (in the spring, after winter was over) to thiamethoxam and clothianidin compared to summer workers (Baines et al., 2017). It is possible that near the end of the winter, these bees might be more similar to summer foragers after their fat and vitellogenin levels are depleted due to brood rearing.
Neonicotinoids have been widely studied for direct effects on honey bees (Gill et al., 2012) as well as changes in behaviors, such as learning (Decourtye et al., 2003; El Hassani et al., 2008; Aliouane et al., 2009), memory performance (Alix et al., 2009; Thompson, 2010), and feeding activity (Blacquière et al., 2012). Effects of thiamethoxam and clothianidin have been shown to affect survival at 29°C (Wood et al., 2020) and thermoregulation at 22 and 33°C (Tosi et al., 2016); however, almost no studies compared the toxicity of the same pesticide to bees at different temperatures. Brood-nest temperature is usually regulated at close to 35°C (Li et al., 2016) but many pesticide toxicities are conducted at room temperature (25°C) (e.g., Burley et al., 2008; Lushchak et al., 2018; and recommended as a standard method by Medrzycki et al., 2013). It is possible that the same pesticide might show different toxicity to honey bees at different temperatures but this is not studies for neonicotinoid pesticides.
Honey bees can be exposed to pesticides via either nectar/honey (energy source), or via pollen (protein source). Honey samples have been shown to contain high enough levels of neonicotinoids to affect honey bees (Mitchell et al., 2017), with the average total concentration of the five measured neonicotinoids at 1.8 ng/g in contaminated samples and a maximum of 56 ng/g. Pollen samples are even worse: almost 60% of samples contained at least one pesticide, with imidacloprid as high as 206 ppb (Mullin et al., 2010). Because an individual honey bee needs to consume about 11 mg of sugar (using honey) per day (Huang et al., 1998) and bees will also consume pollen before becoming winter bees (Maurizio, 1950), bees during winter will also be exposed to pesticides through both of these pathways.
In this study, we tested the effects of sublethal doses of the neonicotinoids thiamethoxam and imidacloprid, two commonly used insecticides at different temperatures, representing different conditions experienced by bees: constant 24 (room temperature), constant 35°C (brood-nest temperature), and 13°C/24°C (representing common night and day time temperatures). The objective of this study was to evaluate whether two neonicotinoid pesticides show different toxicity to honey bees at different temperatures. To meet this objective, we performed multiple cage trials. Firstly, we examined the survival of honey bees exposed to imidacloprid and thiamethoxam at 24°C compared to 35°C, using bees from the brood-nest (Trial 1) and using older workers from outside the cluster (Trial 2). Next, we compared the survival effects of exposure to these two pesticides for older workers at 35°C compared to a constant low temperature (23) and a normal winter temperature variation (ranging from 13°C to 24°C, Trial 3).
Three cage trials were conducted in November 2019 at the Honey Bee Biology Lab, Michigan State University, United States (with colonies at 42°40′45.1″N 84°28′38.8″W). In Trial 1, bees from the brood-nest were used. In Trials 2 and 3, older workers near the top of the winter cluster were sampled with an insect vacuum and then brought into the lab. All bees were anaesthetized with carbon dioxide and divided into cages. The wooden cages (14 × 12 × 9 cm) had mesh in the bottom, a piece transparent plastic sheet with holes in the front, two holes in the top with space for inserting feeding vials, and a piece of cloth was fixed at the center of each cage for possible clustering, if needed. After collection of bees, prior to the start of each trial, the bees were given a 24 h acclimatization period, during which they received untreated 50% sucrose solution. Bees that died during the acclimatization period were removed and excluded from analysis.
In Trial 1 six cages with 40 young bees each were monitored, and Trial 2 six cages containing 25 to 35 older bees, were monitored (Supplementary Table S1). In each trial, three cages were kept in an incubator set at 35°C (brood-nest temperature) and three cages were kept in an incubator set at 24°C (room temperature). Both incubators were maintained at 50% relative humidity. At the end of both Trials 1 and 2 (end of 4 days), the bees that were kept at 35°C were transferred to the 24°C incubator, and all bees were monitored for another day.
In Trial 3, 25 to 40 bees per cage were housed at three temperatures (Supplementary Table S1), with 9 cages (3 treatments × 3 colonies) for each of the following three different temperatures: 35°C, 13 to 24°C, and room temperature (23°C, insider a drawer in complete darkness). The 13–24°C treatment was a changing temperature setting with the incubator programmed to have the temperature at 24°C during the day (7:00 am to 6:00 pm) and 13°C at night (6:30 pm to 6:30 am) to simulate the natural temperature variation during late fall or early winter. Each temperature had three cages of bees (two with pesticides and one control).
Bees were provided with 0.4 ng/μl (0.25 ppm) imidacloprid, or 0.2 ng/μl (0.125 ppm) thiamethoxam (dissolved in acetone then added to syrup) or no pesticide (control). Control bees received the same amount of acetone (12.5 μl in 20 ml of sugar syrup). In Trial 2, bees received a half dose of the pesticides for the first day, i.e., 0.2 ng/μl (0.125 ppm) imidacloprid or 0.1 ng/μl (0.0625 ppm) thiamethoxam. After the first day we adjusted to the same dose as Trials 1 and 3 for the remainder of the experiment.
Data in three trials were analyzed using R 3.5.2 using Kaplan–Meier survival analysis and Log Rank Test test. For Trial 3, three-way ANOVA was also used to analyze the mortality differences among the three temperatures, main treatment (two pesticides and one control) and the three colonies. Mortality data were first transformed [arcsine (square root of mortality)] to make the data fit a normal distribution. Standard errors are reported after the mean.
Digital recordings of temperatures are presented in Table 1 during each of the three trials.
There was a significant negative effect of both neonicotinoid insecticides on the survival of caged honey bees compared to the control when kept at 24°C (Log Rank Test; X2 = 63.5, df = 2, P < 0.001, Figure 1A, Trial 1). This negative effect on survival was not seen when bees were kept at 35°C (Log Rank Test; X2 = 3.4, df = 2, P = 0.2, Figure 1B).
Figure 1. Results of Trials 1 and 2 showing Kaplan–Meier survival curves of bees fed with imidacloprid or thiamethoxam or sugar only (control) at 23/24 at 24°C (left, A,C) or 35°C (right, B,D). Different letters at each line indicate significant differences with Log Rank tests at P < 0.05.
Similar effects negative effects on survival were seen in older workers (Trial 2). When the survival data were analyzed over the 4 days, there was a significant negative effect of both neonicotinoid insecticides on the survival of caged honey bees at 23°C; the survival of bees between the two pesticides was also significantly different, with imidacloprid having a greater negative effect on survival (Log Rank Test; X2 = 24.3, df = 2, P < 0.001, Figure 1C). In contrast, there was no significant difference in bee survival between the bees fed with either pesticide and the control when bees were kept at 35°C (Log Rank Test; X2 = 3.8, df = 2, P = 0.1, Figure 1D). We explored a lower dose (half compared to Trial 1) in Trial 2 for the first day, but no mortality was observed so we adjusted to the same doses as Trial 1 on the second day and observed bees for another 4 days.
At the end of the 4-day survival trials (Trials 1 and 2), we transferred the caged bees (and their food) that were at 35°C to 24°C to determine if pesticides lost their effectiveness at the higher temperature, explaining the differential mortality in bees. In both trials, mortality of bees of a single day after temperature change (from 35 to 24°C, due to the transfer) was significantly higher in the pesticide-treated bees (paired t-test, P < 0.05), but not for the control bees (Table 2).
Table 2. Mortality (%) of bees before and after being transferred from 35°C to 24°C to determine whether pesticides were still active.
In Trial 3, survival of bees under low temperature (23°C) was similar to bees under varying temperature (13°C–24°C), showing a strong negative effect of pesticides on survival (Log Rank Test; X2 = 34.8, df = 2, P < 0.001 for 13–24°C; Log Rank Test; X2 = 136, df = 2, P < 0.001 for 23°C). Bee survival at brood-nest temperature (35°C) was much higher with only one pesticide (imidacloprid) showing a slight, but significant effect (Log Rank Test; X2 = 10.3, df = 2, P = 0.006 for 23°C, Figure 2).
Figure 2. Results of Trial 3 showing Kaplan–Meier survival curves of bees fed with imidacloprid or thiamethoxam or sugar only (control) at 13–24°C (left, A), 23°C (middle, B), or 35°C (right, C). Different letters at each line indicate significant differences with Log Rank tests at P < 0.05.
The total mortality over the 5 days were transformed and analyzed by a three-way ANOVA. There were no significant differences among the three colonies [F (2, 18) = 0.61, P > 0.5] in mortality so further analysis did not include colony in the model. There were significant differences among the different temperatures [F (2, 18) = 121.1, P < 0.01], treatment (pesticides or control) [F (2, 18) = 44.6, P < 0.01], and also significant interactions between the temperature and treatment [F (4, 18) = 11.0, P < 0.01]. Figure 3 shows the changes of mortality among the three pesticide treatments at three different temperatures.
Figure 3. Mean mortality (+SE) of bees exposed to the two pesticides or sugar only (control) at a varying (13°C at night and 24°C during day), constant low (23°C), or brood-nest (35°C) temperature. Control bees showed the highest, intermediate, and lowest mortality, respectively, at 13–24°C, 23°C, and 35°C (all pairwise comparisons significant, t-test, P < 0.05). The two pesticides showed similar high mortality at the two lower temperatures (different from the control, P < 0.01); but at 35°C, there was no significantly difference in mortality among the three treatments (P > 0.05). Statistics done on transformed data but figure here presented un-transformed data. * Denotes significant difference (P < 0.05) between the control and the two pesticides with Fisher’s Protected Least Significant Difference test.
To our understanding, no studies have been carried out on the toxicity of neonicotinoids to winter bees at different temperatures with the same doses. In our study, the major findings are that the imidacloprid (0.25 ppm) and thiamethoxam (0.125 ppm) are more harmful to Apis mellifera at a lower temperature (25°C, normally considered as “room temperature” in many pesticide tests) compared to those at the brood-nest temperature (35°C). We clearly show that temperature should be considered when evaluating risk of pesticides to honey bees.
Honey bee declines have multiple causes and may include parasites, pathogens, pesticides, nutrition/habitat loss/climate change, and transportation (Goulson et al., 2015). Recent attention has focused on how neonicotinoids affect honey bees. Baines et al. (2017) revealed significant negative effects of environmental concentrations of thiamethoxam and clothianidin on winter adult worker bees’ survival under laboratory conditions. More recently, another study reported chronic exposure of thiamethoxam (0.0049, 0.0195, 0.0973 ppm) and clothianidin (0.0042, 0.0167, 0.0832 ppm) significantly reduced survival of winter workers as compared to the control at all tested doses under laboratory conditions (Wood et al., 2020). However, these studies used one single temperature (29°C) for testing. Honey bees are ectotherms when brood is absent, and their body temperature and many of their biological functions are dependent on the environmental temperature. Bees in the center of broodless winter cluster experience a broad range of temperatures (12–33.5°C), with bees at the periphery of the cluster reaching 6°C (Fahrenholz et al., 1989). Numerous studies have documented that the response to pesticides is temperature dependent (Lushchak et al., 2018). Honey bees show negative temperature coefficients in DDT (Ladas, 1972) and cabamates (Georghiou and Atkins, 1964). Our data show similar negative temperature coefficients for both imidacloprid and thiamethoxam. However, we did not directly compare the same breed of honey bees in summer and in winter. It is not clear if they will show similar sensitivity but we postulate that winter bees should be less sensitive because of their higher levels of vitellogenin which reduces oxidative stress (Seehuus et al., 2006), and winter bees already have consumed much pollen which also increases their stress resistance (Huang, 2012). We failed to find a difference though between Trial 1 (brood-nest bees, more likely to be winter bees) and Trials 2 and 3, with old bees outside the cluster (more likely have already foraged in the fall and not real winter bees). Future studies should have better control on these aspects, e.g., with offspring from the same queens, some tested during summer, and some tested as marked real winter bees (e.g., those emerged in November in Michigan, United States).
We found that only a slight decrease (about 11°C) in temperature significantly increased toxicity of both pesticides to bees in all three trials. However, raising bees in a variable temperature environment (day 24°C, night 13°C) did not increase mortality significantly compared to a static cold temperature (23°C). We originally hypothesized that the variable temperature setting should be even more stressful to honey bees, because with presumably higher metabolic demands at 13°C at night, bees might become even more sensitive to pesticides. Instead, we did observe a significant increase of control mortality at the variable temperature (63% + 0.04) compared to 19.2% + 0.06 at 23°C, and 6.7% + 0.03 at 35°C, but no significant difference between the low and variable temperatures, for both pesticides (Figure 2). It is possible that even at 25°C, the mortality was already too high (92–93%) so no further increase was possible. But the survival curves of the three different temperatures (Figure 2) showed similar mortality for 23°C and 13–24°C at days 2–4. These results suggest that a variable temperature at day and night does not significantly change honey bee sensitivity to pesticides compared to a constant low temperature, but a reduction from the brood-nest temperature does make honey bees more sensitive to pesticides. This is rather intriguing because honey bee will forage fine at lower temperatures; in fact, A. mellifera has a preferred foraging temperature of 20°C (Tan et al., 2012) or 23°C (Verma and Dulta, 1986).
One possibility of our observed low toxicity at 35°C for both pesticides is that the pesticides were degraded right away at this high temperature. However, this is not the case because when we transferred bees at 35°C to 24°C on day 5, the caged bees again showed high mortality (Table 2), suggesting that the pesticides were still present in sugar syrup. The low mortality of pesticide-fed bees at 35°C, therefore, must be due to higher tolerance of bees at that temperature.
The one day that the bees received a smaller pesticide dose (day 1, Trial 2) suggests that the differential sensitivity of bees at different temperatures may be dose-dependent. During the day that the bees received a lower dose, we did not see mortality as we did in Trial 1. The dose chosen for the trial was calculated based on many studies (Decourtye et al., 2004; Yang et al., 2008; Eiri and Nieh, 2012; Henry et al., 2012; Schneider et al., 2012; Teeters et al., 2012; Derecka et al., 2013; Sandrock et al., 2014; Tan et al., 2014; and Williamson et al., 2014). Because so little is known about neonicotinoid exposures to winter bees in a diversity of contexts, it will be important to evaluate these findings over a broad range of doses and exposure scenarios. Further study is needed to identify the effects of lower doses at a variety of temperatures, as well as other realistic exposure scenarios including mixtures of pesticides.
We conclude that bees are more sensitive to neonicotinoids at lower temperatures, but a varying temperature does not exacerbate this sensitivity. Honey bee colonies are maintained at 35°C when there is brood, but during the broodless winter period honey bee colonies no longer regulate their temperature to 35°C (Jones et al., 2005). A dose that does not kill honey bees might suddenly cause colony loss during the wintering period. The higher resistance of bees at the brood-nest temperature to neonicotinoid pesticide is intriguing, but perhaps not surprising: workers might be more adapted at this temperature compared to lower temperatures because it is more commonly experienced. For example, it is possible that their detoxifying enzymes show the highest activity at this optimal temperature. Further experiments are needed to study the mechanisms of this temperature-dependent pesticide sensitivity in honey bees.
The raw data supporting the conclusions of this article will be made available by the authors, without undue reservation.
ZYH conceived and designed the experiments, collected the bees, and analyzed the data. MSS conducted the experiments. MOM contributed the materials. All authors contributed to the writing and approved the final version of the manuscript.
Funding was provided by the Higher Education Commission (HEC) of Pakistan (providing support for MSS’s 6-month visit to MSU) and by Michigan Beekeepers Association and the Agricultural Experimental Station at MSU.
The authors declare that the research was conducted in the absence of any commercial or financial relationships that could be construed as a potential conflict of interest.
We thank Ana Heck for maintaining the honey bee colonies and two reviewers for their helpful comments.
The Supplementary Material for this article can be found online at: https://www.frontiersin.org/articles/10.3389/fevo.2020.556856/full#supplementary-material
Alburaki, M., Boutin, S., Mercier, P. L., Loublier, Y., Chagnon, M., and Derome, N. (2015). Neonicotinoid-coated Zea mays seeds indirectly affect honeybee performance and pathogen susceptibility in field trials. PLoS One 10:e0125790. doi: 10.1371/journal.pone.0125790
Aliouane, Y., Adessalam, K., El Hassani, A. K., Gary, V., Armengaud, C., Lambin, M., et al. (2009). Subchronic exposure of honeybees to sublethal doses of pesticides: effect on behavior. Environ. Toxicol. Chem. 28, 113–122. doi: 10.1897/08-110.1
Alix, A., Chauzat, M. P., Duchard, S., Lewis, G., Maus, C., Miles, M. J., et al. (2009). Environmental risk assessment scheme for plant protection products. Chapter 10: Honeybees proposed scheme. Julius-Ku..hn Arch. 423, 27–33.
Baines, D., Wilton, E., Pawluk, A., deGorter, M., and Chomistek, N. (2017). Neonicotinoids act like endocrine disrupting chemicals in newly-emerged bees and winter bees. Sci. Rep. 7:10979.
Blacquière, T., Smagghe, G., van Gestel, C. A. M., and Mommaerts, V. (2012). Neonicotinoids in bees: a review on concentrations, side-effects and risk assessment. Ecotoxicology 21, 973–992. doi: 10.1007/s10646-012-0863-x
Burley, L. M., Fell, R. D., and Saacke, R. G. (2008). Survival of honey bee (Hymenoptera: Apidae) spermatozoa incubated at room temperature from drones exposed to miticides. J. Econ. Entomol. 101, 1081–1087. doi: 10.1603/0022-0493(2008)101[1081:sohbha]2.0.co;2
Catae, A. F., Roat, T. C., De Oliveira, R. A., Nocelli, R. C. F., and Malaspina, O. (2014). Cytotoxic effects of thiamethoxam in the midgut and malpighian tubules of Africanized Apis mellifera (Hymenoptera: Apidae). Microsc. Res. Tech. 77, 274–281. doi: 10.1002/jemt.22339
Crailsheim, K. (1990). The protein balance of the honey bee worker. Apidologie 21, 417–429. doi: 10.1051/apido:19900504
Decourtye, A., Devillers, J., Cluzeau, S., Charreton, M., and Pham-Delègue, M. H. (2004). Effects of imidacloprid and deltamethrin on associative learning in honeybees under semi-field and laboratory conditions. Ecotoxicol. Environ. Saf. 57, 410–419. doi: 10.1016/j.ecoenv.2003.08.001
Decourtye, A., Lacassie, E., and Pham-Delegue, M. H. (2003). Learning performances of honeybees (Apis mellifera L.) are differentially affected by imidacloprid according to the season. Pest Manag Sci. 59, 269–278. doi: 10.1002/ps.631
Derecka, K., Blythe, M. J., Malla, S., Genereux, D. P., Guffanti, A., and Pavan, P. (2013). Transient exposure to low levels of insecticide affects metabolic networks of honeybee larvae. PLoS One 8:e68191. doi: 10.1371/journal.pone.0068191
Desneux, N., Decourtye, A., and Delpuech, J. M. M. (2007). The sublethal effects of pesticides on beneficial arthropods. Annu. Rev. Entomol. 52, 81–106. doi: 10.1146/annurev.ento.52.110405.091440
Doeke, M. A., Frazier, M., and Grozinger, C. M. (2015). Overwintering honey bees: biology and management. Curr. Opin. Insect Sci. 10, 185–193. doi: 10.1016/j.cois.2015.05.014
Eiri, D. M., and Nieh, J. C. (2012). A nicotinic acetylcholine receptor agonist affects honey bee sucrose responsiveness and decreases waggle dancing. J. Exp. Biol. 215(Pt. 12), 2022–2029. doi: 10.1242/jeb.068718
El Hassani, A. K., Dacher, M., Garry, V., Lambin, M., Gauthier, M., and Armengaud, C. (2008). Effects of sublethal doses of acetamiprid and thiamethoxam on the behavior of the honeybee (Apis mellifera). Arch. Environ. Contam. Toxicol. 54, 653–661. doi: 10.1007/s00244-007-9071-8
Fahrenholz, L., Lamprecht, I., and Schricker, B. (1989). Thermal investigations of a honey bee colony: thermoregulation of the hive during summer and winter and heat production of members of different bee castes. J. Comp. Physiol. B 159, 551–560. doi: 10.1007/bf00694379
Fluri, P., Lüscher, M., Wille, H., and Gerig, L. (1982). Changes in weight of the pharyngeal gland and haemolymph titres of juvenile hormone, protein and vitellogenin in worker honey bees. J. Insect Physiol. 28, 61–68. doi: 10.1016/0022-1910(82)90023-3
Georghiou, G. P., and Atkins, E. L. Jr (1964). Temperature coefficient of toxicity of certain n-methylcarbamates against honeybees, and the effect of the synergist piperonyl butoxide. J. Apicult. Res. 3, 31–35. doi: 10.1080/00218839.1964.11100079
Gill, R. J., Ramos-Rodriguez, O., and Raine, N. E. (2012). Combined pesticide exposure severely affects individual- and colony-level traits in bees. Nature 491, 105–108. doi: 10.1038/nature11585
Goulson, D., Nicholls, E., Botías, C., and Rotheray, E. L. (2015). Bee declines driven by combined stress from parasites, pesticides, and lack of flowers. Science. 347:1255957. doi: 10.1126/science.1255957
Graves, J. B., and Mackensen, O. (1965). Topical application and insecticide resistance studies on the honey bee. J. Econ. Entomol. 58, 990–993. doi: 10.1093/jee/58.5.990
Hartfelder, K., and Engels, W. (1998). Social insect polymorphism: Hormonal regulation of plasticity in development and reproduction in the honeybee. Curr. Top. Dev. Biol. 40, 45–77. doi: 10.1016/s0070-2153(08)60364-6
Haszonits, O., and Crailsheim, K. (1990). Uptake of L-leucine into isolated enterocytes of the honeybee (Apis mellifera L.) depending on season. J. Insect Physiol. 36, 835–842. doi: 10.1016/0022-1910(90)90170-k
Henry, M., Rollin, O., Aptel, J., Tchamitchian, S., Beguin, M., Requier, F., et al. (2012). A common pesticide decreases foraging success and survival in honey bees. Science 336, 348–350. doi: 10.1126/science.1215039
Huang, Z. Y. (2012). Pollen nutrition affects honey bee stress resistance. Terrest. Arth. Rev. 5, 175–189. doi: 10.1163/187498312x639568
Huang, Z.-Y., Plettner, E., and Robinson, G. E. (1998). Effect of social environment and mandibular gland removal on division of labor in worker honey bees. J. Comp. Physiol. B 183, 143–152.
Huang, Z. Y., and Robinson, G. E. (1995). Seasonal changes in juvenile hormone titers and rates of biosynthesis in honey bees. J. Comp. Physiol. 165, 18–28. doi: 10.1007/bf00264682
Huang, Z. Y., and Wang, Y. (2015). “Social physiology of honey bees: differentiation in behaviors, castes, and longevity,” in The Hive and the Honey Bee, ed. J. Graham (Hamilton, IL: Dadant and Sons Press), 183–200.
Jones, A. K., Raymond-Delpech, V., Thany, S. H., Gauthier, M., and Sattelle, D. B. (2006). The nicotinic acetylcholine receptor gene family of the honey bee, Apis mellifera. Genome Res. 16, 1422–1430. doi: 10.1101/gr.4549206
Jones, J. C., Helliwell, P., Beekman, M., Maleszka, R., and Oldroyd, B. P. (2005). The effects of rearing temperature on developmental stability and learning and memory in the honey bee, Apis mellifera. J. Comp. Physiol. A Neuroethol. Sens. Neural Behav. Physiol. 191, 1121–1129. doi: 10.1007/s00359-005-0035-z
Ladas, A. (1972). The influence of some internal and external factors upon the insecticide resistance of honeybee. Apidologie 3, 55–78.
Lambert, O., Piroux, M., Puyo, S., Thorin, C., L’Hostis, M., Wiest, L., et al. (2013). Widespread occurrence of chemical residues in beehive matrices from apiaries located in different landscapes of western France. PLoS One 8:e67007. doi: 10.1371/journal.pone.0067007
Li, Z. Y., Huang, Z. Y., Sharma, D. B., Xue, Y., Wang, Z., and Ren, B. (2016). Drone and worker brood microclimates are regulated deferentially in honey bees, Apis mellifera. PLoS One 11:e0148740. doi: 10.1371/journal.pone.0148740
Lushchak, V. I., Matviishyn, T. M., Husak, V. V., Storey, J. M., and Storey, K. B. (2018). Pesticide toxicity: a mechanistic approach. EXCLI J. 17, 1101–1136. doi: 10.17179/excli2018-1710
Matsumoto, T. (2013). Reduction in homing flights in the honey bee Apis Mellifera after a sublethal dose of neonicotinoid insectcides. Bull. Insectol. 66, 1–9.
Maurizio, A. (1950). The influence of pollen feeding and brood rearing on the length of life and physiological conditions of the honeybee. Bee World. 1950, 9–12. doi: 10.1080/0005772X.1950.11094617
Medrzycki, P., Giffard, H., Aupinel, P., Belzunces, L. P., Chauzat, M. P., Claßen, C., et al. (2013). Standard methods for toxicology research in Apis mellifera. J. Apicult. Res. 52, 1–60. doi: 10.3896/ibra.1.52.4.23
Meled, M., Thrasyvoulou, A., and Belzunces, L. P. (1998). Seasonal variations in susceptibility of Apis mellifera to the synergistic action of prochloraz and deltamethrin. Environ. Toxicol. Chem. 17, 2517–2520. doi: 10.1897/1551-5028(1998)017<2517:svisoa>2.3.co;2
Mitchell, E. A., Mulhauser, B., Mulot, M., Mutabazi, A., Glauser, G., and Aebi, A. (2017). A worldwide survey of neonicotinoids in honey. Science 358, 109–111. doi: 10.1126/science.aan3684
Mullin, C. A., Frazier, M., Frazier, J. L., Ashcraft, S., Simonds, R., vanEngelsdorp, D., et al. (2010). High levels of miticides and agrochemicals in north american apiaries: implications for honey bee health. PLoS One 5:e9754. doi: 10.1371/journal.pone.0009754
Münch, D., and Amdam, G. V. (2010). The curious case of aging plasticity in honey bees. FEBS Lett. 584, 2496–2503. doi: 10.1016/j.febslet.2010.04.007
Oliveira, R. A., Roat, T. C., Carvalho, S. M., and Malaspina, O. (2014). Side-effects of thiamethoxam on the brain and midgut of the Africanized honeybee Apis mellifera (Hymenpptera: Apidae). Environ. Toxicol. 29, 1122–1133. doi: 10.1002/tox.21842
Ollerton, J. (2017). Pollinator diversity: distribution, ecological function, and conservation. Annu. Rev. Ecol. Evol. Syst. 48, 353–376. doi: 10.1146/annurev-ecolsys-110316-022919
Ollerton, J., Winfree, R., and Tarrant, S. (2011). How many flowering plants are pollinated by animals? Oikos 120, 321–326. doi: 10.1111/j.1600-0706.2010.18644.x
Sandrock, C., Tanadini, L. G., Pettis, J. S., Biesmeijer, J. C., Potts, S. G., and Neumann, P. (2014). Sublethal neonicotinoid insecticide exposure reduces solitary bee reproductive success. Agric. Forest Entomol. 16, 119–128. doi: 10.1111/afe.12041
Schäfer, R. B., von, der Ohe, P. C., Rasmussen, J., Kefford, B. J., Beketov, M. A., et al. (2012). Thresholds for the effects of pesticides on invertebrate communities and leaf breakdown in stream ecosystems. Environ. Sci. Technol. 46, 5134–5142. doi: 10.1021/es2039882
Schneider, C. W., Tautz, J., Grünewald, B., and Fuchs, S. (2012). RFID tracking of sublethal effects of two neonicotinoid insecticides on the foraging behavior of Apis mellifera. PLoS One 7:e30023. doi: 10.1371/journal.pone.0030023
Seehuus, S. C., Norberg, K., Gimsa, U., Krekling, T., and Amdam, G. V. (2006). Reproductive protein protects functionally sterile honey bee workers from oxidative stress. Proc. Natl. Acad. Sci. U.S.A. 103, 962–967. doi: 10.1073/pnas.0502681103
Simon-Delso, N., Amaral-Rogers, V., Belzunces, L. P., Bonmatin, J. M., Chagnon, M., Downs, C., et al. (2015). Systemic insecticides (neonicotinoids and fipronil): trends, uses, mode of action and metabolites. Environ. Sci. Pollut. Res. 22, 5–34. doi: 10.1007/s11356-014-3470-y
Smirle, M. J., and Winston, M. L. (1987). Inter colony variation in pesticide detoxification by the honey bee (Hymenoptera: Apidae). J. Econ. Entomol. 80, 5–8. doi: 10.1093/jee/80.1.5
Tan, K., Chen, W., Dong, S., Liu, X., Wang, Y., and Nieh, J. C. (2014). Imidacloprid alters foraging and decreases bee avoidance of predators. PLoS One 9:e102725. doi: 10.1371/journal.pone.0102725
Tan, K., Yang, S., Wang, Z. W., Radloff, S. E., and Oldroyd, B. P. (2012). Differences in foraging and brood-nest temperature in the honey bees Apis cerana and A. mellifera. Apidologie 43, 618–623. doi: 10.1007/s13592-012-0136-y
Teeters, B. S., Johnson, R. M., Ellis, M. D., and Siegfried, B. D. (2012). Using video-tracking to assess sublethal effects of pesticides on honey Bees (Apis mellifera L.). Environ. Toxicol. Chem. 31, 1349–1354. doi: 10.1002/etc.1830
Thompson, H. M. (2010). Risk assessment for honey bees and pesticides: recent developments and ‘new issues’. Pest Manag. Sci. 66, 1157–1162. doi: 10.1002/ps.1994
Tosi, S., Démares, F. J., Nicolson, S. W., Medrzycki, P., Pirk, C. W., and Human, H. (2016). Effects of a neonicotinoid pesticide on thermoregulation of African honey bees (Apis mellifera scutellata). J. Insect Physiol. 93, 56–63. doi: 10.1016/j.jinsphys.2016.08.010
Verma, L. R., and Dulta, P. C. (1986). Foraging behaviour of Apis cerana indica and Apis mellifera in pollinating apple flowers. J. Apicult. Res. 25, 197–201.
Williamson, S. M., Willis, S. J., and Wright, G. A. (2014). Exposure to neonicotinoids influences the motor function of adult worker honeybees. Ecotoxicology 23, 1409–1418. doi: 10.1007/s10646-014-1283-x
Williamson, S. M., and Wright, G. A. (2013). Exposure to multiple cholinergic pesticides impairs olfactory learning and memory in honeybees. J. Exp. Biol. 216, 1799–1807. doi: 10.1242/jeb.083931
Willmer, P. G., Cunnold, H., and Ballantyne, G. (2017). Insights from measuring pollen deposition: quantifying the pre-eminence of bees as flower visitors and effective pollinators. Arthropod Plant Interact 11, 411–425.
Wood, S. C., Kozii, I. V., Medici, de Mattos, I., de Carvalho Macedo, Silva, R., et al. (2020). Chronic high-neonicotinoid exposure decreases overwinter survival of Apis mellifera L. Insects 11:30. doi: 10.3390/insects11010030
Keywords: overwintering bees, temperature, neonicotinoids, pesticide toxicity, Apis mellifera
Citation: Saleem MS, Huang ZY and Milbrath MO (2020) Neonicotinoid Pesticides Are More Toxic to Honey Bees at Lower Temperatures: Implications for Overwintering Bees. Front. Ecol. Evol. 8:556856. doi: 10.3389/fevo.2020.556856
Received: 29 April 2020; Accepted: 02 September 2020;
Published: 19 October 2020.
Edited by:
Tugrul Giray, University of Puerto Rico, Puerto RicoReviewed by:
Bert Rivera-Marchand, Inter American University of Puerto Rico, Puerto RicoCopyright © 2020 Saleem, Huang and Milbrath. This is an open-access article distributed under the terms of the Creative Commons Attribution License (CC BY). The use, distribution or reproduction in other forums is permitted, provided the original author(s) and the copyright owner(s) are credited and that the original publication in this journal is cited, in accordance with accepted academic practice. No use, distribution or reproduction is permitted which does not comply with these terms.
*Correspondence: Zachary Y. Huang, YmVlc0Btc3UuZWR1; YmVlcy5tc3VAZ21haWwuY29t
Disclaimer: All claims expressed in this article are solely those of the authors and do not necessarily represent those of their affiliated organizations, or those of the publisher, the editors and the reviewers. Any product that may be evaluated in this article or claim that may be made by its manufacturer is not guaranteed or endorsed by the publisher.
Research integrity at Frontiers
Learn more about the work of our research integrity team to safeguard the quality of each article we publish.