- Centre for Sustainable Aquatic Research (CSAR), Swansea University, Swansea, United Kingdom
A wide trophic niche and high trophic plasticity are often invoked to explain the successful establishment of many aquatic invaders, but there is little information regarding the diet of most invasive fish in European waters. We combined stomach content and stable isotope analysis (SIA) of 13C and 15N to examine the trophic niche of the highly invasive topmouth gudgeon (Pseudorasbora parva) in three contrasting ponds and a reservoir in South Wales. Marked differences in diet and trophic position were found among neighboring systems only a few kilometers apart. The most diverse diet was found in ponds with the fewest number of inter-specific competitors, and resulted in topmouth gudgeon having the highest condition factor, the widest variation in δ13C and δ15N values, and the highest carbon content, typical of generalist feeders. In contrast, topmouth gudgeon that coexisted with other cyprinids, were much more likely to have an empty stomach and relied almost exclusively on plant seeds, resulting in fish having a poor condition factor and low trophic position. Comparisons with other trophic studies indicate that variation in isotope values among neighboring sites can exceed variation across continents, making it difficult to predict the diet or trophic impacts of the invasive topmouth gudgeon. Given the importance of obtaining reliable data on trophic position for risk assessment, our study shows that the use of SIA could be used to prioritize control and eradication measures that take into account trophic plasticity.
Introduction
The topmouth gudgeon (TMG, Pseudorasbora parva) is a highly invasive fish native of Asia that has become one of the worst aquatic invaders in Europe due its potential impact on native biodiversity (Pinder et al., 2005; Britton et al., 2007). TMG possesses many ecological traits that make it a successful invader, including short generation time, high fecundity, and substantial phenotypic plasticity (Gozlan et al., 2010). The species can also grow rapidly (Ye et al., 2006; Adrović and Skenderović, 2007; Kapusta et al., 2008) and mature fast (Pinder et al., 2005), especially when living at high density (Britton et al., 2007, 2008).
During the first few years of the invasion, TMG populations appear to be composed mainly of young-of-the-year, up to 50 mm in total length, although the species can reach up to 120 mm (Ye et al., 2006; Adrović and Skenderović, 2007; Kapusta et al., 2008). The species displays substantial variation in the timing of spawning, depending on water temperature (Yan and Chen, 2009). Its diet can be wide and, although previous studies had described it as mostly omnivorous (Weber, 1984; Xie et al., 2001), it can also adopt a strict planktivorous diet in some cases (Priyadarshana et al., 2001; Asaeda et al., 2007). Depending on prey availability, TMG can feed on chironomid larvae (Wolfram-Wais et al., 1999), but also on the eggs and larvae of other fish, which may pose a threat to native species (Stein and Herl, 1986; Xie et al., 2000). The preferred prey size appears to be size dependent, as observed in a comparative pond study where the smaller size class (20–25 mm) fed exclusively on cladoceran zooplankton, the bigger size classes (35–60 mm) fed mainly on chironomid larvae, and the intermediate size classes fed on a mixture of the two preys (Declerck et al., 2002). The diet of topmouth gudgeon can also change seasonally, shifting from chironomid larvae in spring and summer to ostracods in winter (Xie et al., 2000). Where introduced, topmouth gudgeon has been found to compete for food with native species in Great Britain (Britton et al., 2010), Belgium (Declerck et al., 2002), and Poland (Witkowski, 2002) which can lead to depressed growth rates, reduced reproduction outputs and shifts in the trophic position of native fish (Britton et al., 2010). When other invasive species are present, the topmouth gudgeon’s plasticity in food selection allows it to shift its trophic niche and co-exist with other invaders (Jackson and Britton, 2014).
TMG can negatively impact native fish through competition for food and space, resulting in depressed growth rates (and also in production), and also a shift in trophic levels (Britton et al., 2010). Another relevant threat posed by TMG is its ability to carry non-native pathogens such as the intracellular parasite Sphaerothecum destruens that can become lethal for salmonids (Gozlan et al., 2005; Andreou et al., 2011). For these reasons, it is feared that the spread of TMG could result not just in a significant loss of native river biodiversity, but also on substantial economic losses caused by damage to sport fisheries and salmon farming alike (Gozlan et al., 2005).
TMG was first recorded in Great Britain in 1996, in a pond in Southern England (Domaniewski and Wheeler, 1996). It is thought that the first introduction may have occurred a decade earlier, in the mid 1980’s, when TMG originating from Germany were delivered to an ornamental aquaculture facility in Hampshire, and subsequently became established in Lake Tadburn (Gozlan et al., 2002). Within few years, TMG had spread rapidly and has now been reported in 32 locations across England and Wales, most of which are lentic systems, and 10 of which are connected to major catchments (Britton et al., 2007, 2010). Recent eDNA data show that more sites are infested than previously thought (Robinson et al., 2019), probably because traditional sampling techniques do not detect the species at low densities (Britton et al., 2011). Due to its speed of colonization, and the potential for major ecological and economic impacts, TMG has been classified as highly impactive by the UK Technical Advisory Group on the Water Framework Directive (Panov et al., 2009) and is the subject of costly eradication programs (Robinson et al., 2019).
Stable isotope analysis (SIA) can be used to study food webs (Vander Zanden et al., 1999), based on the fact that the ratios of heavy to light isotopes in animal tissues are often affected by diet (DeNiro and Epstein, 1978, 1981). Carbon and nitrogen isotopes (δ13C and δ15N, respectively) are the ones most commonly used in freshwater biology, as they are related to the sources of primary productivity (δ13C) and to trophic level (δ15N). As trophic markers, SIA can be used at different ecological levels, to study variation among individuals, species, and also among communities (Whitledge and Rabeni, 1997; Newsome et al., 2009). They can be used to examine trophic position (Roth et al., 2006; Cherel et al., 2008), animal migrations (Cherel et al., 2007; McClellan et al., 2010), impacts of invasive species (Vander Zanden et al., 1999; Nilsson et al., 2012), trophic subsidies (Izquierdo-Gomez et al., 2015), and the contribution of allochthonous vs. autochthonous food resources in aquatic ecosystems (Solomon et al., 2011; Venarsky et al., 2014).
Here we employed SIA of nitrogen and carbon, complemented with analysis of stomach contents, to investigate variation in the trophic ecology of topmouth gudgeon in four contrasting freshwater habitats in South Wales. Our aims were twofold: (1) to describe the trophic niche of this highly invasive species to gain a better understanding of the potential for interference competition with native fish, and (2) to assess the value of SIA for monitoring diet plasticity of a rapidly expanding invasive species in different colonized habitats.
Materials and Methods
Origin of Samples
We analyzed 118 topmouth gudgeon originating from four contrasting water bodies in South Wales (Figure 1 and Table 1) that differed greatly in size, use, and fish community composition: two small decorative ponds with few or no fish species other than eel (Turbine Pond, TUR, n = 27; Dyfatty pond, DYF, n = 29), a eutrophic pond used for recreational fishing regularly stocked with eight species of coarse fish (Sylen Lake, SYL, n = 30), and a larger, cooler reservoir used for water supply and stocked with salmonids (Upper Lliedi Reservoir, LLI, n = 32). Sylen Lake, Turbine pond, and Dyfatty pond were fished between November 2012 and February 2013 while the Upper Lliedi Reservoir (LLI) was fished in June 2018 as no fish could be caught during the winter of 2017/2108. Specimens were kept frozen until analysis. We obtained biometry data (total length, weight, condition factor) and stable isotope data (δ13C, δ15N, C:N) for the four ponds, and stomach contents for three of them (no stomach data was available for LLI).
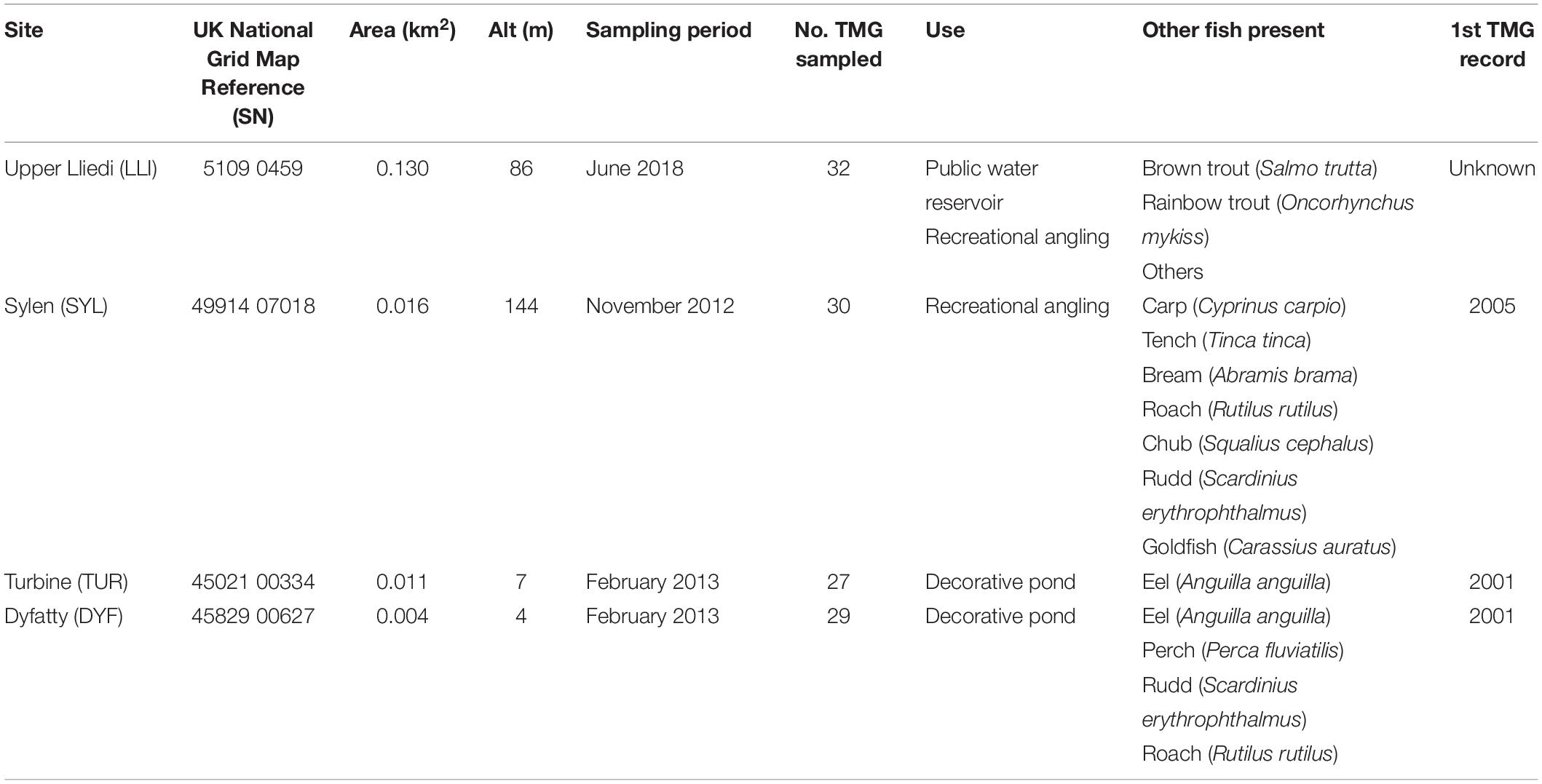
Table 1. Characteristics of the four study sites examined for trophic position of topmouth gudgeon (Pseudorasbora parva) in South Wales.
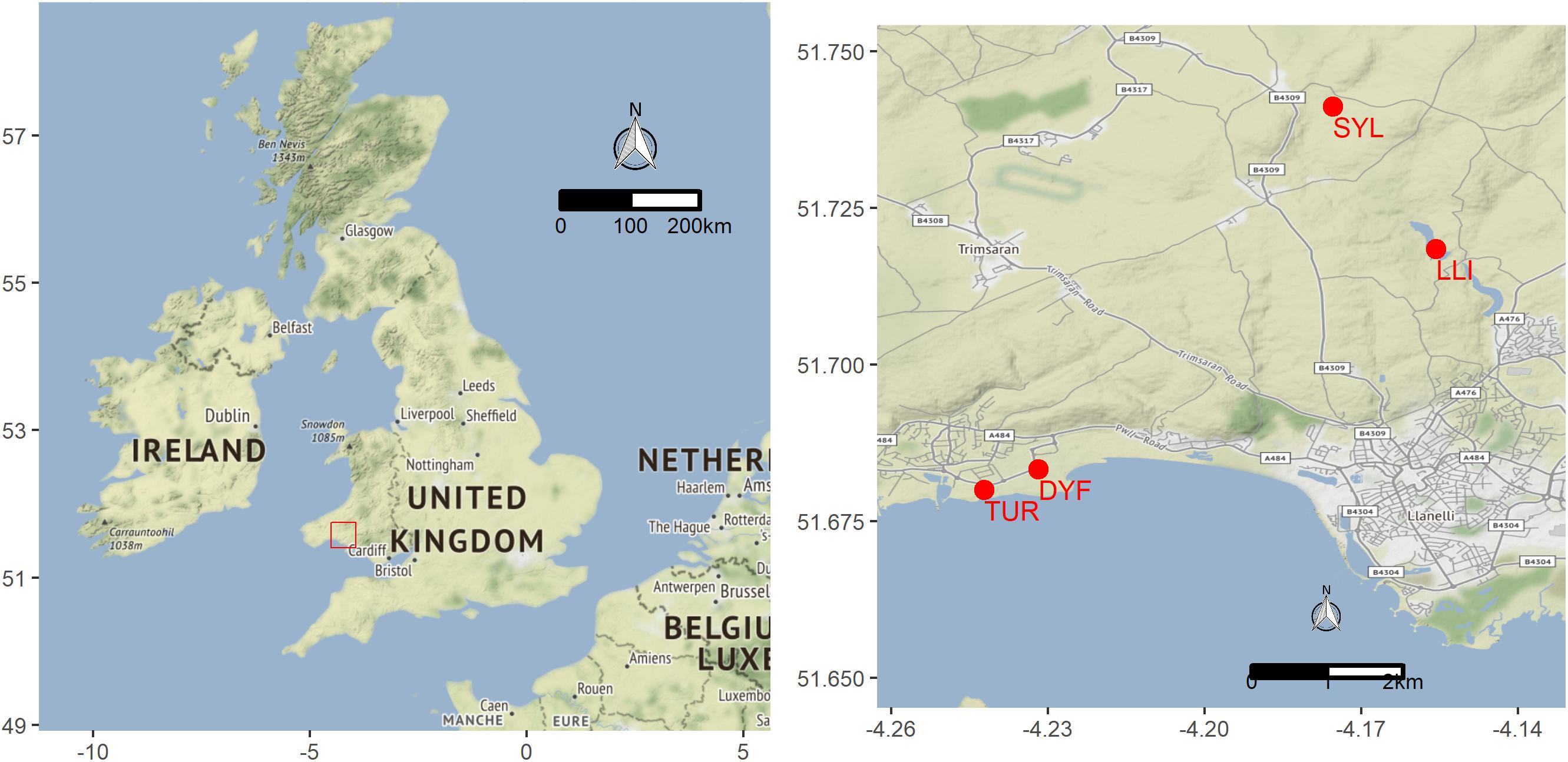
Figure 1. Location of study sites sampled for topmouth gudgeon in Wales (UK): Upper Lliedi Reservoir – LLI, Sylen pond – SYL, Turbine pond – TUR, and Dyfatty pond – DYF.
Biometry Data
We took paired measurements of total length and weight of TMG from one site (LLI) before (fresh) and after they had been frozen to derive a regression equation to estimate fresh body size from frozen specimens collected from the other three populations. The regression equations were:
We calculated Fulton’s condition factor (K) from total length (TL) and weight (WT) measurements [K = (WT ∗ 100) / TL3] to derive an index of body condition (Bolger and Connolly, 1989).
Stomach Content Analysis
For stomach content analysis, frozen samples were slowly thawed and then transferred to 30, 50, and 70% ethanol solutions. We analyzed stomach contents of fish in three ponds (TUR, DYF, SYL) with a stereo microscope (Nikon SMZ1270) at 12.7× (0.63–8×) magnification. The vast majority of prey consisted of zooplankton (67%) that we classified at family level. Insect parts (1%), seeds (24.1%) and plant material were also present, but these could not be identified. We counted every food item and measured the size (longest axis) of a subsample of food items to obtain an average size for each prey category.
Stable Isotope Analyses
We obtained 1–2 mg of white muscle from the caudal part of the fish, above the lateral line, and freeze dried the samples for 24 h before analysis. Analyses of %C, %N, δ13C, and δ15N were conducted by Isotope Ratio Mass Spectrometry (EA-IRMS) at Iso-Analytical Ltd (Marshfield Bank, Crewe CW2 8UY, United Kingdom) using a Europa Scientific 20–20 IRMS Elemental Analyzer, samples being combusted at 1,000°C in the presence of oxygen using IA-R068 as reference material (soy protein, δ13CV–PDB = -25.22‰, δ15NAIR = 0.99‰). The following standards were run as quality controls: IA-R068, IA-R038 (L-alanine, δ13CV–PDB = -24.99‰, δ15NAIR = -0.65‰), IA-R069 (tuna protein, δ13CV–PDB = -18.88‰, δ15NAIR = 11.60‰) and a mixture of IAEA-C7 (oxalic acid, δ13CV–PDB = -14.48‰) and IA-R046 (ammonium sulfate, δ15NAIR = 22.04‰) was run as quality control samples during analysis and were calibrated against inter-laboratory standards distributed by the International Atomic Energy Agency, Vienna. Repeatability of isotope values in samples analyzed in duplicate (20%) was high (Cohen’s weighted kappa coefficient: δ13C = 0.99; δ15N = 0.98; C:N = 0.94) indicating that SIA results were precise. Duplicates were then averaged before numerical analysis. The carbon to nitrogen ratio (C:N) ranged between 3.13 and 3.81, but was significantly below 3.5 (U95CI = 3.35), so lipid correction normalization was not deemed necessary (Post et al., 2007). In total we analyzed 118 specimens for SIA (DYF, n = 29; LLI, n = 32; SYL, n = 30; TUR, n = 27).
Statistical Analysis
We used R 3.3 (R Core Team, 2017) for analyses. We transformed % carbon and % nitrogen content with the arcsine transformation to make the data suitable for linear model analysis. We analyzed nitrogen and carbon content %, δ13C, δ15N and C:N ratio as function of fish size, condition factor and pond (location), and used the dredge and anova functions in the MuMIn R package for model selection. We calculated the Shannon diversity index (H) to estimate the taxonomical diversity of the food items present in fish stomachs. Quadratic weights were applied to give greatest emphasis to large differences between scores. GLM binary logistic regression was used to model the occurrence of an empty stomach (yes or no) as a function of fish size, condition factor and pond type. We performed an analysis of similarities (ANOSIM) with the vegan R package (Oksanen et al., 2013) to test for differences in diet composition among locations, merging together the less frequent food classes (unidentified, insect parts and Cyclops). Differences in the Shannon index of diversity (H) were assessed by bootstrapping (1,000 permutations) to obtain means and 95 confidence intervals (Gardener, 2014), we then used the Hutcheson t-test to compare the diversity among sites (Hutcheson, 1970). Trophic breadth for each pond was calculated using Levin’s measure of niche breadth (Krebs, 1999):
where, B = Levin’s measure of niche breadth.
Nj = Number of individuals using preyj.
Y = ΣNj = Total number of individuals sampled.
Ethics Statement
This study was reviewed and approved by Swansea University, College of Science Ethics Committee, Permit Student Project 210519/1544.
Results
Variation in Body Size and Condition Factor
The body size (total length) of topmouth gudgeon varied from 33 to 100 mm, and the calculated wet weight from 0.44 to 5.02 g. There were no significant differences between ponds in the length or weight after Tukey’s HSD correction (Figures 2A,B) but there was a significant difference in condition factor [F(3,114) = 5.05, P = 0.003; Figure 2C] with fish in Turbine Pond having a better condition than those in Dyfatty pond (P = 0.006) or the Upper Lliedi Reservoir (P = 0.003).
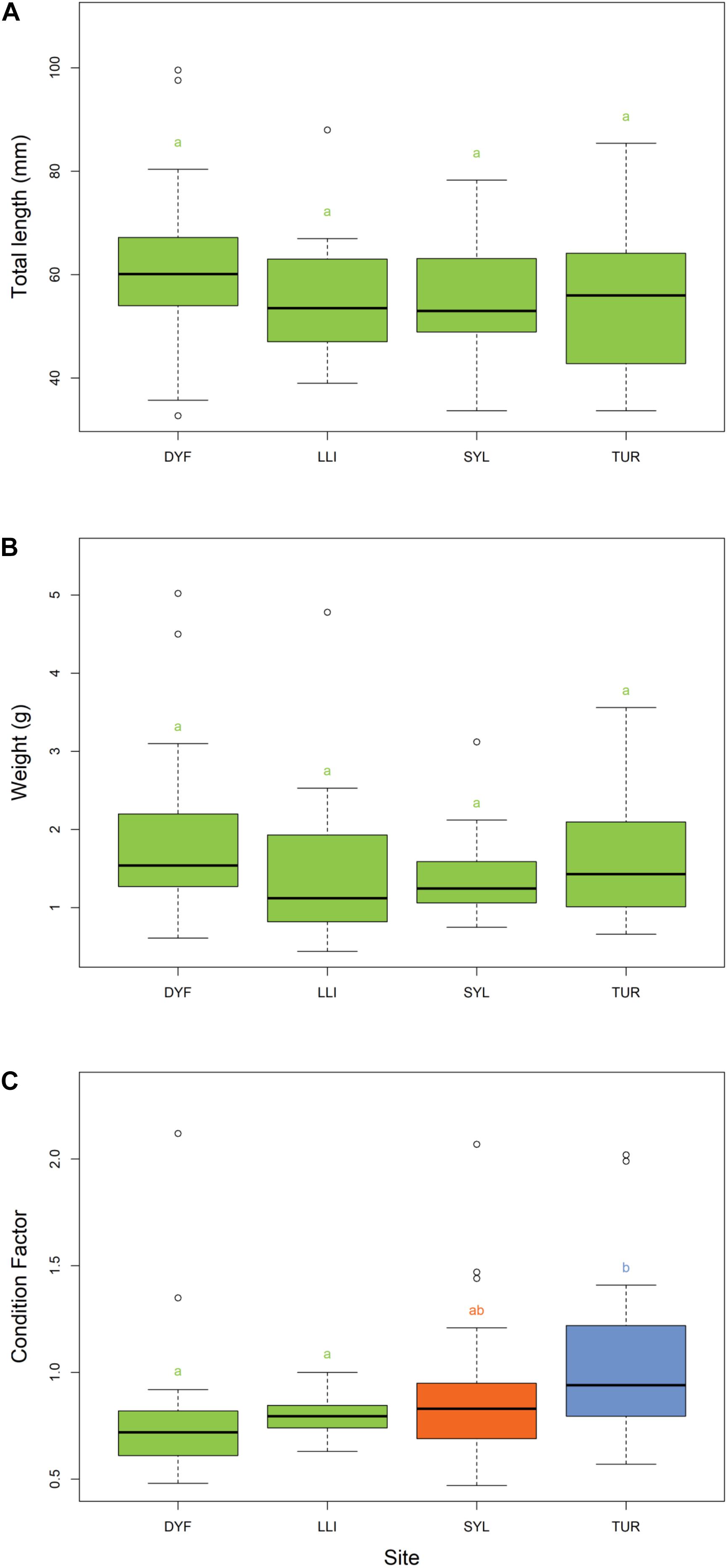
Figure 2. Variation in (A) total length, (B) weight, and (C) Fulton’s body condition factor of topmouth gudgeon across study sites. Sites that have different colors and letter subscripts are statistically different from each other following Tukey’s HSD post-hoc pairwise tests.
Diet Analysis
The proportion of fish with an empty stomach differed significantly among sites (GLM binary logistic regression: deviance = 22.09, P < 0.001), but was unrelated to body size (deviance = 2.57, P = 0.417), or condition factor (deviance = 0.14, P = 0.70). The pond with the greatest incidence of empty stomachs was DYF (21/25 or 84%), followed by SYL (19/29 or 65.5%), while TUR had the lowest incidence (6/27 or 22.2%). Tukey multiple comparisons at 95% family wise confidence level show that the incidence of empty stomachs was significantly lower at TUR than at DYF (P < 0.001) or SYL (P < 0.005), while no significant difference was observed between DYF and SYL (P = 0.237).
The majority of stomach contents (756 food items or 67% of the total) was composed of zooplankton, including Daphniidae (268 specimens or 23.8%), Bosminidae (136 specimens or 12%), Chydoridae (348 specimens or 30.9%), and Cyclopidae (4 specimens or 0.3%). The remaining was composed of plant seeds (272 items or 24.1%), unidentified insect parts (12 items or 1%), and unidentified organic and plant material (88 items or 7.8%; Figure 3). Mean prey size varied considerably, being largest in insect parts (2.14 mm), followed by Cyclopidae (1.51 mm), Daphniidae (1.28 mm), Bosminidae (0.67 mm), plant seeds (0.62 mm), and Chydoridae (0.48 mm).
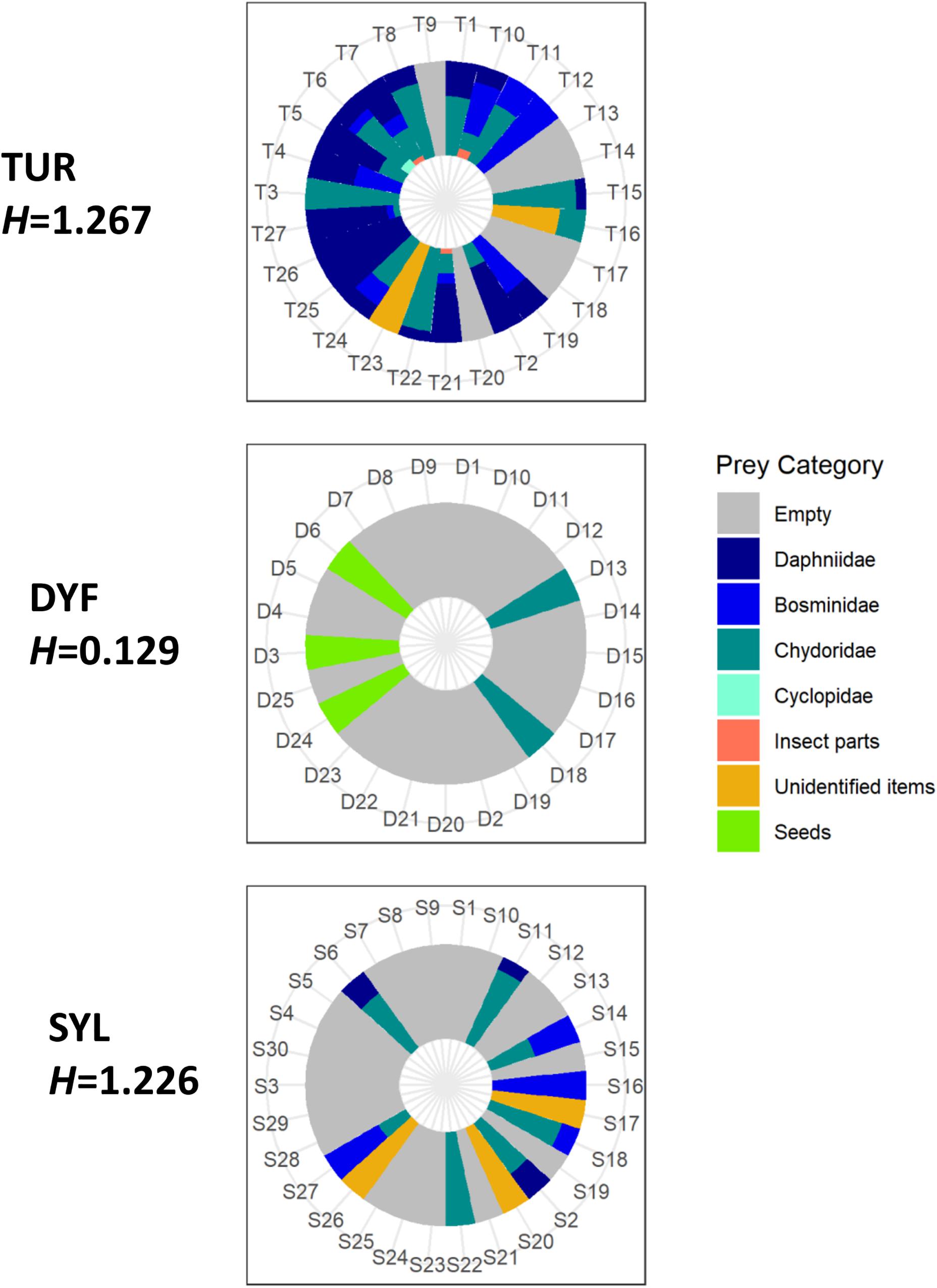
Figure 3. Individual variation in the diet of topmouth gudgeon (% items) across study sites showing Shannon diversity index (H). Each numbered sector represents one fish.
Analysis of similarities (ANOSIM) indicated that the diet of TMG differed markedly among sites (R = 0.28, P = 0.005), and this was reflected in different prey diversity estimates. Thus, the diet of fish at DYF had a much lower prey diversity (mean bootstrapping estimate H = 0.148, 95CI = 0.089–0.218) than those at TUR (H = 1.270, 95CI = 1.223–1.317) or SYL (H = 1.244, 95CI = 1.173–1.318). Pairwise Hutcheson t-test comparisons indicated that there were statistical differences between TUR and DYF (P < 0.001) and between SYL an DYF (P < 0.001), but not between TUR and SYL (P = 0.392).
Differences in diet were reflected in measures of diet breadth (Table 2). Thus, while fish at TUR and SYL had, similarly, wide diets (bootstrapping estimates B, TUR = 3.286, 95CI = 1.666–5.005; SYL = 3.270, 95CI = 1.611–4.998), the diet breadth at DYF was much narrower (B = 1.522, CI = 1.058–2.000), consistent with greater diet specialization, but also with lack of prey choice.
Stable Isotope Analysis
The carbon content in the muscle of topmouth gudgeon ranged between 41 and 60% among individuals, and differed significantly among ponds (Figure 4A, F(3,113) = 11.54, P < 0.001), being lowest in the LLI reservoir and highest in the eutrophic SYL pond (post-hoc pairwise HSD, SYL-LLI, P < 0.001; TUR-LLI, P < 0.001). Carbon content did not depend on body length (t = -0.385, P = 0.701), condition factor (t = -0.035, P = 0.972) or the presence of food items in the stomach (t = -0.69, P = 0.490). The nitrogen content varied from 12 to 18% among individuals, and as with %C, it also varied significantly among ponds (Figure 4B, F(3,113) = 6.77, P < 0.001), but did not depend on body length (t = 0.51, P = 0.610), condition factor (t = 0.31, P = 0.754), or presence of food items in the stomach (t = -1.51, P = 0.133). Nitrogen content was lowest in the reservoir and highest in the eutrophic angling pond (post-hoc pairwise HSD, SYL-LLI P < 0.001; SYL- DYF P = 0.004).
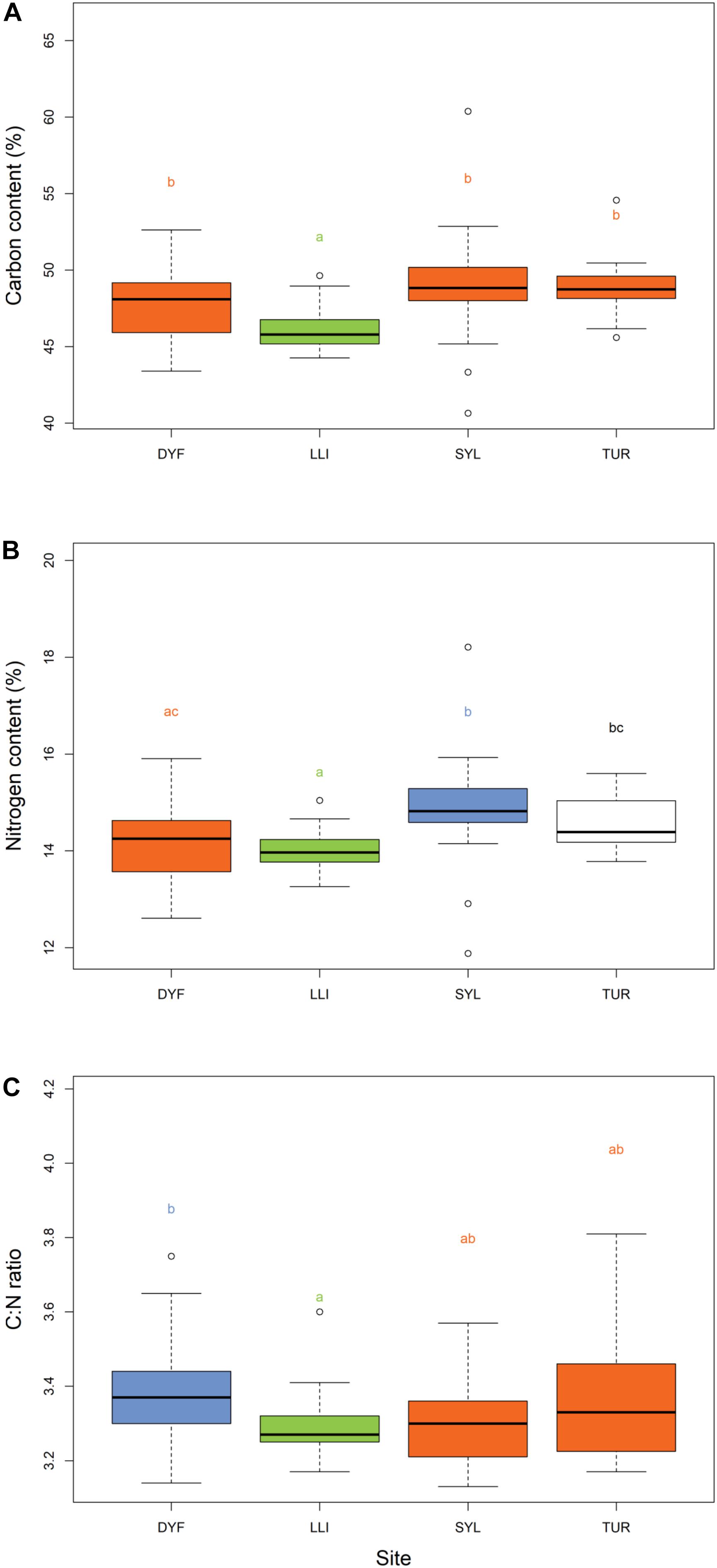
Figure 4. Variation in (A) % carbon content, (B) % nitrogen content, and (C) C:N ratios of topmouth gudgeon across study sites. Sites that have different colors and letter subscripts are statistically different from each other following Tukey’s HSD post-hoc pairwise tests.
C:N ratios (a measure of lipid reserves) varied between 3.13 and 3.81 among individuals, but were unaffected by body length (t = -1.22, P = 0.224), condition factor (t = 0.2, P = 0.835), or presence of food items in the stomach (t = -0.96, P = 0.335). They varied significantly among sites (Figure 4C, F(3,113) = 4.15, P = 0.007), being highest in one of the small ornamental ponds, and lowest in the reservoir (DYF-LLI, P = 0.016).
δ13C varied markedly among individuals (range = –30.95‰ to –16.43‰) and differed significantly among all sites (Figure 5; F(3,113) = 24.77, P < 0.001), being highest in the small ornamental ponds and lowest in the eutrophic angling pond (pairwise comparisons, LLI-DYF, P < 0.001; TUR-DYF, P < 0.001; SYL-LLI, P < 0.001; TUR-SYL, P < 0.001). δ13C was unaffected by body length (t = -0.09, P = 0.927) or condition factor (t = -1.14, P = 0.909), but increased (i.e., it was more enriched) among fish that had food in their stomach (t = 2.90, P = 0.004).
δ15N varied from 5.17 to 15.10‰ among individuals, and also varied significantly among all sites (Figure 5; F(3,113) = 574.27, P < 0.001), all ponds being significantly different from each other (P < 0.001), being highest in the reservoir and lowest in the small ornamental ponds. As for δ13C, δ15N was unaffected by body length (t = -0.567, P = 0.572) or condition factor (t = -0.156, P = 0.876), but increased (i.e., it became more enriched) among fish that had food in their stomach (t = -3.23, P = 0.001).
Examination of the isotopic δ13C vs. δ15N plot (Figure 5) indicate a substantial trophic discrimination among study sites, particularly with respect to δ15N, with the large, deep reservoir (LLI) being nitrogen enriched and the small ornamental pond (DYF) being nitrogen depleted compared to the other sites.
Discussion
Our study sheds light on the topmouth gudgeon’s trophic plasticity and shows that its diet varies markedly among sites, even between neighboring ponds only a few kilometers away from each other. We acknowledge that differences in the timing of sampling must have introduced some unwanted variation in our study, but marked differences in stomach contents, δ13C, δ15N, and condition factor were also found between sites DYF and TUR which were sampled at the same time in February. Thus, season alone cannot explain our results. Isotopic turnover in TMG is not known, but data on other cyprinids indicate that isotopic equilibrium may take several months in muscle tissue. Half-life muscle turnover rate for δ15N was 84 days for barbel Barbus barbus (Busst and Britton, 2018) and 68 days for grass carp Ctenopharyngodon idellus (Xia et al., 2013); equilibrium in barbel was not reached even after 125 days, with 95% turnover taken 371 days (Busst and Britton, 2018). This indicates that SIA signals, unlike stomach contents, are relatively stable over time. Furthermore, as muscle isotopic turnover is mostly influenced by body growth (Busst and Britton, 2018), we would not expect substantial changes in isotopic signatures during the colder parts of the year, when three of our four ponds were sampled. Isotopic signatures of δ13C and δ15N showed little seasonal variation in the muscle of whitefish in Lake Geneva during autumn and winter, and only reflected the food consumed during spring and summer (Perga and Gerdeaux, 2005).
Trophic plasticity, i.e., the ability to switch diets, is expected to facilitate establishment success among invasive species (Schröder and Garcia de Leaniz, 2011; Pettitt-Wade et al., 2015), and may have allowed the topmouth gudgeon to colonize different environments, exploit novel food resources, and outcompete many native fish species (Záhorská et al., 2009, 2010). However, trophic plasticity also carries costs (Shea and Chesson, 2002). Trophic shifts are common in response to changes in habitat and food availability (Werner and Hall, 1977), but can also represent a strategy to avoid potential trophic competition (Jackson et al., 2012). In our study, topmouth gudgeon with a mean size of 57 mm were feeding on relatively small food items, most of which were within the 0.7–1.3 mm size range, and hence less than 2% of their body size. The consumption of small zooplankton, shown at all our sites, has been reported previously (Arnold, 1990; Rosecchi et al., 1993), along with a diet composed of benthic crustaceans (Rosecchi et al., 1993; Xie et al., 2000), chironomids (Declerck et al., 2002), phytoplankton and algae (Muchacheva, 1950). However, the reliance on plant seeds found at one of our sites was unexpected, and may have been the consequence of strong inter-specific competition.
Results from stomach content analysis were largely confirmed by results from SIA and seemed to reflect the combined effects of food availability and competition. However, both techniques are needed to properly characterize individual variation in diet, as found for other lentic fish, for example the pumpkinseed sunfish (Locke et al., 2013). Thus, the widest variation in δ13C and δ15N was observed at one of the small recreational ponds (TUR), which is consistent with a generalist diet largely unaffected by inter-specific competition as only one other fish was recorded (European eel, Anguilla anguilla). In contrast, at the other recreational pond (DYF) topmouth gudgeon coexisted with four other species (eel, perch Perca fluviatilis, rudd Scardinius erythrophthalmus, and roach Rutilus rutilus), displayed a very high occurrence of individuals with an empty stomach (84%) and relied almost exclusively on plant seeds. This may explain why they attained a much lower condition factor, and displayed a lower trophic level, suggesting that plant seeds are nutritionally poor and not a preferred food resource. Britton et al. (2010) have shown that roach occupies a similar trophic position as topmouth gudgeon and this can result in competition for food, while rudd occupies a higher trophic level and may prey on topmouth gudgeon. Therefore, the presence of competitors and predators may have forced the topmouth gudgeon to adopt a more specialized feeding behavior in this pond. In a similar way, the narrow trophic position of TMG at the reservoir could be explained by high predatory pressure, since this reservoir is managed by an angling association which regularly stocks it with trout for recreational fishing.
We found that the body condition factor (a measure of growth performance – Froese, 2006) and the C:N ratio (a proxy for stored lipids – Jardine et al., 2008) was highest in the pond with the most diverse diet, widest diet breadth, and fewest competitors (Turbin pond where only eel was reported). This was also reflected in high carbon and nitrogen contents, as well as in high δ13C values. In aquatic food webs, high δ13C values tend to correlate with better feeding conditions and less competition (Hinz et al., 2017), which is consistent with our results. In contrast, TMG sampled in the much larger reservoir (LLI) where predatory salmonids abound, had the lowest C:N ratios, indicative of low lipid reserves, as well as low carbon and nitrogen contents, which are also consistent with poor feeding conditions (Vander Zanden et al., 1999).
Comparisons of SIA values from our study and other studies (Table 3) indicate that TMG in Wales generally have higher δ13C and δ15N values (i.e., more enriched) than those reported in another study in Great Britain (Britton et al., 2010), indicating a higher trophic level (δ15N) and a different primary source of carbon (δ13C). Our δ13C values are relatively high and closer to the ones reported in China – where topmouth gudgeon is a native species (Mao et al., 2012, 2016; Gao et al., 2017) than to values observed in Belgium where the species is invasive (Tran et al., 2015). High δ13C values are more typical of benthic food pathways, compared to pelagic food pathways which tend to be more 13C depleted (Pinnegar and Polunin, 1999). Variation in δ15N was also greater in our study than those reported in the literature, and one of our sites (DYF) had the lowest δ15N value reported for the species; this coincides with a diet dominated by plant seeds. In general, the variability among sites is so high that for example δ15N values obtained from fish at one of our sites (LLI) are more similar to those found in fish from Lake Taihu in China (Mao et al., 2012) than to those at neighboring sites in Wales. This indicates that it is difficult to predict the diet or trophic position of TMG even within small geographical areas. Substantial spatial variation in stable isotope signatures have also been reported for other lentic fish (Locke et al., 2013).
Conclusion
Our study indicates that when the topmouth gudgeon colonizes a new area it may be difficult to predict its trophic impacts from local information, since differences in diet are largely independent of geographical proximity. Populations living in neighboring water bodies can have diets more dissimilar than those from populations thousands of kilometers away. Given the importance diet information has on models that attempt to predict the risks posed by invasive fish, such as FISK (Copp et al., 2009; Mastitsky et al., 2010; Copp, 2013; Simonovic et al., 2013), uncertainty on the expected diet of the topmouth gudgeon could result in incorrect risk assessments. To improve the precision of risk assessments, information on trophic level seems to be important for predicting the diet, and therefore the likely feeding impacts, of topmouth gudgeon on native communities. As trophic impacts will likely vary widely from site to site, our study shows that SIA could be used, perhaps in combination with eDNA (Robinson et al., 2019), for species detection to prioritize control and eradication measures that consider variation in diet.
Data Availability Statement
The datasets generated by this study are available on Figshare: 10.6084/m9.figshare.1197447.
Ethics Statement
The animal study was reviewed and approved by Swansea University College of Science Ethics Committee, Permit Student 210519/1544.
Author Contributions
CG and SC designed the study and secured the samples and funding. MR collected the data and carried out the analyses with advice from CG. MR and CG wrote the manuscript with contributions from SC. All authors contributed to the article and approved the submitted version.
Funding
Funding was provided by the EC Horizon 2020 Aquainvad-ED project (Marie Skłodowska-Curie ITN-2014-ETN-642197) to SC.
Conflict of Interest
The authors declare that the research was conducted in the absence of any commercial or financial relationships that could be construed as a potential conflict of interest.
Acknowledgments
We are grateful to Ida Tavner and Emma Keenan (Natural Resources Wales) for providing information on the study sites and TMG samples, to Peter Jones (Swansea University) for help trapping topmouth gudgeon, and to Catherine Bradford and Therry Northam (Dwr Cymru Welsh Water) for permission to sample the Lliedi reservoir. This manuscript has been released as a pre-print at bioRxiv (Rolla et al., 2019; https://www.biorxiv.org/content/10.1101/617688v1).
References
Adrović, A., and Skenderović, I. (2007). Allochthonous ichthyofauna of certain water bodies in North-Eastern Bosnia. Acta Agric. Serb. 12, 37–46.
Andreou, D., Gozlan, R., Stone, D., Martin, P., Bateman, K., and Feist, S. (2011). Sphaerothecum destruens pathology in cyprinids. Dis. Aquat. Org. 95, 145–151. doi: 10.3354/dao02356
Arnold, A. (1990). Eingebürgete Fischarten: Zur Biologie und Verbreitung Allochtoner Wilffische in Europa. Wittenberg: Neue Brehm-Bücherei.
Asaeda, T., Manatunge, J., and Fujino, T. (2007). The effects of predation risk and current velocity stress on growth, condition and swimming energetics of Japanese minnow (Pseudorasbora parva). Ecol. Res. 22, 32–40. doi: 10.1007/s11284-006-0186-6
Bolger, T., and Connolly, P. (1989). The selection of suitable indices for the measurement and analysis of fish condition. J. Fish Biol. 34, 171–182. doi: 10.1111/j.1095-8649.1989.tb03300.x
Britton, J., Davies, G., and Brazier, M. (2008). Contrasting life history traits of invasive topmouth gudgeon (Pseudorasbora parva) in adjacent ponds in England. J. Appl. Ichthyol. 24, 694–698. doi: 10.1111/j.1439-0426.2008.01163.x
Britton, J., Davies, G., Brazier, M., and Pinder, A. (2007). A case study on the population ecology of a topmouth gudgeon (Pseudorasbora parva) population in the UK and the implications for native fish communities. Aquat. Conserv.Mar. Freshwat. Ecosyst. 17, 749–759. doi: 10.1002/aqc.809
Britton, J. R., Davies, G. D., and Harrod, C. (2010). Trophic interactions and consequent impacts of the invasive fish Pseudorasbora parva in a native aquatic foodweb: a field investigation in the UK. Biol. Invasions 12, 1533–1542. doi: 10.1007/s10530-009-9566-5
Britton, J. R., Pegg, J., and Gozlan, R. E. (2011). Quantifying imperfect detection in an invasive pest fish and the implications for conservation management. Biol. Cons. 144, 2177–2181. doi: 10.1016/j.biocon.2011.05.008
Busst, G. M., and Britton, J. R. (2018). Tissue-specific turnover rates of the nitrogen stable isotope as functions of time and growth in a cyprinid fish. Hydrobiologia 805, 49–60. doi: 10.1007/s10750-017-3276-2
Cherel, Y., Ducatez, S., Fontaine, C., Richard, P., and Guinet, C. (2008). Stable isotopes reveal the trophic position and mesopelagic fish diet of female southern elephant seals breeding on the Kerguelen Islands. Mar. Ecol. Prog. Ser. 370, 239–247. doi: 10.3354/meps07673
Cherel, Y., Hobson, K. A., Guinet, C., and Vanpe, C. (2007). Stable isotopes document seasonal changes in trophic niches and winter foraging individual specialization in diving predators from the Southern Ocean. J. Anim. Ecol. 76, 826–836. doi: 10.1111/j.1365-2656.2007.01238.x
Copp, G. H. (2013). The fish invasiveness screening kit (FISK) for non-native freshwater fishes: a summary of current applications. Risk Anal. 33, 1394–1396. doi: 10.1111/risa.12095
Copp, G. H., Vilizzi, L., Mumford, J., Fenwick, G. V., Godard, M. J., and Gozlan, R. E. (2009). Calibration of FISK, an invasiveness screening tool for nonnative freshwater fishes. Risk Anal. 29, 457–467. doi: 10.1111/j.1539-6924.2008.01159.x
Declerck, S., Louette, G., De Bie, T., and De Meester, L. (2002). Patterns of diet overlap between populations of non-indigenous and native fishes in shallow ponds. J. Fish Biol. 61, 1182–1197. doi: 10.1111/j.1095-8649.2002.tb02464.x
DeNiro, M. J., and Epstein, S. (1978). Influence of diet on the distribution of carbon isotopes in animals. Geochim. Cosmochim. Acta 42, 495–506. doi: 10.1016/0016-7037(78)90199-0
DeNiro, M. J., and Epstein, S. (1981). Influence of diet on the distribution of nitrogen isotopes in animals. Geochim. Cosmochim. Acta 45, 341–351. doi: 10.1016/0016-7037(81)90244-1
Froese, R. (2006). Cube law, condition factor and weight–length relationships: history, meta-analysis and recommendations. J. Appl. Ichthyol. 22, 241–253. doi: 10.1111/j.1439-0426.2006.00805.x
Gao, J., Zhong, P., Ning, J., Liu, Z., and Jeppesen, E. (2017). Herbivory of omnivorous fish shapes the food web structure of a Chinese tropical eutrophic lake: Evidence from stable isotope and fish gut content analyses. Water 9:69. doi: 10.3390/w9010069
Gardener, M. (2014). Community Ecology: Analytical Methods using R and Excel. Exeter: Pelagic Publishing Ltd.
Gozlan, R., Pinder, A., and Shelley, J. (2002). Occurrence of the Asiatic cyprinid Pseudorasbora parva in England. J. Fish Biol. 61, 298–300. doi: 10.1111/j.1095-8649.2002.tb01755.x
Gozlan, R. E., Andreou, D., Asaeda, T., Beyer, K., Bouhadad, R., Burnard, D., et al. (2010). Pan-continental invasion of Pseudorasbora parva: towards a better understanding of freshwater fish invasions. Fish Fish. 11, 315–340. doi: 10.1111/j.1467-2979.2010.00361.x
Gozlan, R. E., St-Hilaire, S., Feist, S. W., Martin, P., and Kent, M. L. (2005). Biodiversity: disease threat to European fish. Nature 435:1046. doi: 10.1038/4351046a
Hinz, H., Moranta, J., Balestrini, S., Sciberras, M., Pantin, J. R., Monnington, J., et al. (2017). Stable isotopes reveal the effect of trawl fisheries on the diet of commercially exploited species. Sci. Rep. 7:6334.
Hutcheson, K. (1970). A test for comparing diversities based on the Shannon formula. J. Theor. Biol. 29, 151–154. doi: 10.1016/0022-5193(70)90124-4
Izquierdo-Gomez, D., Sanchez-Jerez, P., Bayle-Sempere, J. T., Loader, N., and Garcia de Leaniz, C. (2015). Effects of coastal fish farms on body size and isotope composition of wild penaeid prawn. Fish. Res. 172, 50–56. doi: 10.1016/j.fishres.2015.06.017
Jackson, M. C., and Britton, J. R. (2014). Divergence in the trophic niche of sympatric freshwater invaders. Biol. Invasions 16, 1095–1103. doi: 10.1007/s10530-013-0563-3
Jackson, M. C., Donohue, I., Jackson, A. L., Britton, J. R., Harper, D. M., and Grey, J. (2012). Population-level metrics of trophic structure based on stable isotopes and their application to invasion ecology. PLoS One 7:2. doi: 10.1371/journal.pone.0031757
Jardine, T. D., Kidd, K. A., Polhemus, J. T., and Cunjak, R. A. (2008). An elemental and stable isotope assessment of water strider feeding ecology and lipid dynamics: synthesis of laboratory and field studies. Freshwat. Biol. 53, 2192–2205.
Kapusta, A., Bogacka-Kapusta, E., and Czarnecki, B. (2008). The significance of stone moroko, Pseudorasbora parva (Temminck and Schlegel), in the small-sized fish assemblages in the littoral zone of the heated Lake Licheńskie. Arch. Polish Fish. 16, 49–62.
Locke, S. A., Bulte, G., Forbes, M. R., and Marcogliese, D. J. (2013). Estimating diet in individual pumpkinseed sunfish Lepomis gibbosus using stomach contents, stable isotopes and parasites. J. Fish Biol. 82, 522–537. doi: 10.1111/j.1095-8649.2012.03497.x
Mao, Z., Gu, X., Zeng, Q., Zhou, L., and Sun, M. (2012). Food web structure of a shallow eutrophic lake (Lake Taihu, China) assessed by stable isotope analysis. Hydrobiologia 683, 173–183. doi: 10.1007/s10750-011-0954-3
Mao, Z. G., Gu, X. H., Zeng, Q. F., and Chen, H. H. (2016). Carbon sources and trophic structure in a macrophyte-dominated polyculture pond assessed by stable-isotope analysis. Freshwat. Biol. 61, 1862–1873. doi: 10.1111/fwb.12821
Mastitsky, S. E., Karatayev, A. Y., Burlakova, L. E., and Adamovich, B. V. (2010). Non-native fishes of Belarus: diversity, distribution, and risk classification using the Fish Invasiveness Screening Kit (FISK). Aquat. Invasions 5, 103–114. doi: 10.3391/ai.2010.5.1.12
McClellan, C. M., Braun-McNeill, J., Avens, L., Wallace, B. P., and Read, A. J. (2010). Stable isotopes confirm a foraging dichotomy in juvenile loggerhead sea turtles. J. Exp. Mar. Biol. Ecol. 387, 44–51. doi: 10.1016/j.jembe.2010.02.020
Muchacheva, V. A. (1950). K Biologii Amurskogo Cebacka Pseudorasbora Parva (Schlegel): Trudy Amurskoj Ichtiologiceskoj Ekspedicji, 1945 – 1949. Moscow: Moscow Society of Naturalists, 365–374.
Newsome, S. D., Tinker, M. T., Monson, D. H., Oftedal, O. T., Ralls, K., Staedler, M. M., et al. (2009). Using stable isotopes to investigate individual diet specialization in California sea otters (Enhydra lutris nereis). Ecology 90, 961–974. doi: 10.1890/07-1812.1
Nilsson, E., Solomon, C., Wilson, K., Willis, T., Larget, B., and Vander Zanden, M. (2012). Effects of an invasive crayfish on trophic relationships in north-temperate lake food webs. Freshwat. Biol. 57, 10–23. doi: 10.1111/j.1365-2427.2011.02688.x
Oksanen, J., Blanchet, F. G., Kindt, R., Legendre, P., Minchin, P. R., O’Hara, R., et al. (2013). Package ‘vegan’. Community Ecology Package, Version 2.9. 295.
Panov, V. E., Alexandrov, B., Arbačiauskas, K., Binimelis, R., Copp, G. H., Grabowski, M., et al. (2009). Assessing the risks of aquatic species invasions via European inland waterways: from concepts to environmental indicators. Integr. Environ. Assess. Manag. 5, 110–126.
Perga, M., and Gerdeaux, D. (2005). ‘Are fish what they eat’all year round? Oecologia 144, 598–606. doi: 10.1007/s00442-005-0069-5
Pettitt-Wade, H., Wellband, K. W., Heath, D. D., and Fisk, A. T. (2015). Niche plasticity in invasive fishes in the Great Lakes. Biol. Invasions 17, 2565–2580. doi: 10.1007/s10530-015-0894-3
Pinder, A., Gozlan, R., and Britton, J. (2005). Dispersal of the invasive topmouth gudgeon, Pseudorasbora parva in the UK: a vector for an emergent infectious disease. Fish. Manag. Ecol. 12, 411–414. doi: 10.1111/j.1365-2400.2005.00466.x
Pinnegar, J., and Polunin, N. (1999). Differential fractionation of δ13C and δ15N among fish tissues: implications for the study of trophic interactions. Funct. Ecol. 13, 225–231. doi: 10.1046/j.1365-2435.1999.00301.x
Post, D. M., Layman, C. A., Arrington, D. A., Takimoto, G., Quattrochi, J., and Montana, C. G. (2007). Getting to the fat of the matter: models, methods and assumptions for dealing with lipids in stable isotope analyses. Oecologia 152, 179–189. doi: 10.1007/s00442-006-0630-x
Priyadarshana, T., Asaeda, T., and Manatunge, J. (2001). Foraging behaviour of planktivorous fish in artificial vegetation: the effects on swimming and feeding. Hydrobiologia 442, 231–239.
R Core Team (2017). R: A Language and Environment for Statistical Computing. Version 3.3. Vienna: R Foundation for Statistical Computing.
Robinson, C. V., Garcia de Leaniz, C., Rolla, M., and Consuegra, S. (2019). Monitoring the eradication of the highly invasive topmouth gudgeon (Pseudorasbora parva) using a novel eDNA assay. Environ. DNA 1, 74–85. doi: 10.1002/edn3.12
Rolla, M., Consuegra, S., and Garcia de Leaniz, C. (2019). Trophic plasticity of the highly invasive topmouth gudgeon (Pseudorasbora parva) inferred from stable isotope analysis. bioRxiv [Preprint]. doi: 10.1101/617688
Rosecchi, E., Crivelli, A. J., and Catsadorakis, G. (1993). The establishment and impact of Pseudorasbora parva, an exotic fish species introduced into Lake Mikri Prespa (north-western Greece). Aquat. Conserv. Mar. Freshwat. Ecosyst. 3, 223–231. doi: 10.1002/aqc.3270030306
Roth, B. M., Hein, C. L., and Vander Zanden, M. J. (2006). Using bioenergetics and stable isotopes to assess the trophic role of rusty crayfish (Orconectes rusticus) in lake littoral zones. Can. J. Fish. Aquat. Sci. 63, 335–344. doi: 10.1139/f05-217
Schröder, V., and Garcia de Leaniz, C. (2011). Discrimination between farmed and free-living invasive salmonids in Chilean Patagonia using stable isotope analysis. Biol. Invasions 13, 203–213. doi: 10.1007/s10530-010-9802-z
Shea, K., and Chesson, P. (2002). Community ecology theory as a framework for biological invasions. Trends Ecol. Evol. 17, 170–176. doi: 10.1016/s0169-5347(02)02495-3
Simonovic, P., Tošić, A., Vassilev, M., Apostolou, A., Mrdak, D., Ristovska, M., et al. (2013). Risk assessment of non-native fishes in the Balkans region using FISK, the invasiveness screening tool for non-native freshwater fishes. Mediterr. Mar. Sci. 14, 369–376.
Solomon, C. T., Carpenter, S. R., Clayton, M. K., Cole, J. J., Coloso, J. J., Pace, M. L., et al. (2011). Terrestrial, benthic, and pelagic resource use in lakes: results from a three-isotope Bayesian mixing model. Ecology 92, 1115–1125. doi: 10.1890/10-1185.1
Stein, H., and Herl, O. (1986). Pseudorasbora parva – eine neue Art der mitteleuropaeischen Fischfauna. Z. fuer die Binnenfischerei 36, 1–2.
Tran, T. N. Q., Jackson, M. C., Sheath, D., Verreycken, H., and Britton, J. R. (2015). Patterns of trophic niche divergence between invasive and native fishes in wild communities are predictable from mesocosm studies. J. Anim. Ecol. 84, 1071–1080. doi: 10.1111/1365-2656.12360
Vander Zanden, M. J., Casselman, J. M., and Rasmussen, J. B. (1999). Stable isotope evidence for the food web consequences of species invasions in lakes. Nature 401:464. doi: 10.1038/46762
Venarsky, M. P., Huntsman, B. M., Huryn, A. D., Benstead, J. P., and Kuhajda, B. R. (2014). Quantitative food web analysis supports the energy-limitation hypothesis in cave stream ecosystems. Oecologia 176, 859–869. doi: 10.1007/s00442-014-3042-3
Weber, E. (1984). Die ausbreitung der pseudokeilfleckbarben im donauraum. Österreichs Fischerei 37, 63–65.
Werner, E. E., and Hall, D. J. (1977). Competition and habitat shift in two sunfishes (Centrarchidae). Ecology 58, 869–876. doi: 10.2307/1936222
Whitledge, G. W., and Rabeni, C. F. (1997). Energy sources and ecological role of crayfishes in an Ozark stream: insights from stable isotopes and gut analysis. Can. J. Fish. Aquat. Sci. 54, 2555–2563. doi: 10.1139/f97-173
Witkowski, A. (2002). Introduction of fishes into Poland: benefaction or plague? Nat. Cons. 59, 41–52.
Wolfram-Wais, A., Wolfram, G., Auer, B., Mikschi, E., and Hain, A. (1999). “Feeding habits of two introduced fish species (Lepomis gibbosus, Pseudorasbora parva) in Neusiedler See (Austria), with special reference to chironomid larvae (Diptera: Chironomidae),” in Shallow Lakes’ 98, eds N. Walz and B. Nixdorf (Dordrecht: Springer), 123–129. doi: 10.1007/978-94-017-2986-4_12
Xia, B., Gao, Q.-F., Li, H., Dong, S.-L., and Wang, F. (2013). Turnover and fractionation of nitrogen stable isotope in tissues of grass carp Ctenopharyngodon idellus. Aquat. Environ. Interact. 3, 177–186. doi: 10.3354/aei00061
Xie, S., Cui, Y., Zhang, T., and Li, Z. (2000). Seasonal patterns in feeding ecology of three small fishes in the Biandantang Lake, China. J. Fish Biol. 57, 867–880. doi: 10.1111/j.1095-8649.2000.tb02198.x
Xie, S., Zhu, X., Cui, Y., Wootton, R. J., Lei, W., and Yang, Y. (2001). Compensatory growth in the gibel carp following feed deprivation: temporal patterns in growth, nutrient deposition, feed intake and body composition. J. Fish Biol. 58, 999–1009. doi: 10.1111/j.1095-8649.2001.tb00550.x
Yan, Y., and Chen, Y. (2009). Variations in reproductive strategies between one invasive population and two native populations of Pseudorasbora parva. Curr. Zool. 55, 56–60. doi: 10.1093/czoolo/55.1.56
Yasuno, N., Chiba, Y., Shindo, K., Fujimoto, Y., Shimada, T., Shikano, S., et al. (2012). Size-dependent ontogenetic diet shifts to piscivory documented from stable isotope analyses in an introduced population of largemouth bass. Environ. Biol. Fishes 93, 255–266. doi: 10.1007/s10641-011-9911-2
Ye, S., Li, Z., Lek-Ang, S., Feng, G., Lek, S., and Cao, W. (2006). Community structure of small fishes in a shallow macrophytic lake (Niushan Lake) along the middle reach of the Yangtze River, China. Aquat. Living Resour. 19, 349–359. doi: 10.1051/alr:2007005
Záhorská, E., Kováč, V., Falka, I., Beyer, K., Katina, S., Copp, G., et al. (2009). Morphological variability of the Asiatic cyprinid, topmouth gudgeon Pseudorasbora parva, in its introduced European range. J. Fish Biol. 74, 167–185. doi: 10.1111/j.1095-8649.2008.02121.x
Keywords: aquatic invasive species, diet breadth, generalist feeder, foraging, stomach content analysis, isotopes
Citation: Rolla M, Consuegra S and Garcia de Leaniz C (2020) Trophic Plasticity of the Highly Invasive Topmouth Gudgeon (Pseudorasbora parva) Inferred From Stable Isotope Analysis. Front. Ecol. Evol. 8:212. doi: 10.3389/fevo.2020.00212
Received: 12 March 2020; Accepted: 08 June 2020;
Published: 30 June 2020.
Edited by:
Nildeniz Top Karakuş, Muğla University, TurkeyReviewed by:
Hugo Verreycken, Research Institute for Nature and Forest (INBO), BelgiumNurbanu Partal, Çanakkale Onsekiz Mart University, Turkey
Copyright © 2020 Rolla, Consuegra and Garcia de Leaniz. This is an open-access article distributed under the terms of the Creative Commons Attribution License (CC BY). The use, distribution or reproduction in other forums is permitted, provided the original author(s) and the copyright owner(s) are credited and that the original publication in this journal is cited, in accordance with accepted academic practice. No use, distribution or reproduction is permitted which does not comply with these terms.
*Correspondence: Carlos Garcia de Leaniz, c.garciadeleaniz@swansea.ac.uk