- 1Institute of Zoology, University of Regensburg, Regensburg, Germany
- 2Department of Entomology, University of California, Riverside, Riverside, CA, United States
The cuticle of virtually any insect is covered by a thin layer of cuticular hydrocarbons (CHCs) typically consisting of a complex mixture of n-alkanes, methyl-branched alkanes, and unsaturated hydrocarbons. Apart from their putative primary function as a desiccation barrier, CHCs are used by insects for communication. In many species of parasitoid wasps, males use CHCs to recognize females, and thus the composition of CHC profiles is typically species- and sex-specific. Sometimes, the biological activity of CHCs is synergized by more polar lipids. In most species, however, the contribution of polar lipids and the role of individual CHCs or CHC classes is unclear. Here we report a CHC-based contact sex pheromone in the pteromalid wasp Urolepis rufipes. Males of U. rufipes were arrested on and showed courtship behavior (wing fanning) and copulation attempts toward cadavers of females, whereas male cadavers and solvent-washed cadavers of either sex elicited no responses. Whole body extracts from females and the non-polar CHC fraction thereof elicited arrestment, courtship, and copulation attempts by males, whereas the fractions containing polar lipids were behaviorally inactive. Analyses of male- and female-derived CHC profiles revealed that they consisted exclusively of n-alkanes and methyl-branched alkanes. Removal of n-alkanes from female-derived CHCs with molecular sieves rendered the remaining methyl-branched fraction inactive. Synthetic n-alkanes in female-specific ratios also did not elicit any responses by males. Two 7-methylalkanes (7-MeC31 and 7-MeC33) were the only two components with a higher relative abundance in females compared to males. However, supplementation of male-derived CHCs with synthetic racemic 7-MeC31 and 7-MeC33 to levels found in females was not sufficient to render male-derived CHCs attractive to males. Enantiopure synthetic 7-MeC31 and 7-MeC33 might have caused different effects but were not available. We conclude that female-derived CHCs function as a contact sex pheromone in U. rufipes. Our data suggest that methyl-branched and n-alkanes act synergistically and that the sex-specific relative composition of the whole profile, rather than the abundances of single components, underlies the elicitation of male courtship behavior.
Introduction
The epicuticle of insects is covered by a thin layer of lipids. This lipid layer is typically composed of a complex mixture of straight-chain and methyl-branched alkanes with 1–4 methyl groups, as well as unsaturated hydrocarbons with one or more double bonds (cuticular hydrocarbons, CHCs) (Stanley-Samuelson and Nelson, 1993; Blomquist and Bagneres, 2010). Apart from CHCs, more polar lipid classes such as wax esters, fatty alcohols, aldehydes, and triacylglycerides have been found in insect-derived cuticular extracts (Buckner, 1993; Kühbandner et al., 2012). Because of their hydrophobic properties, these lipids are thought to serve primarily as a desiccation barrier (Gibbs, 1998; Gibbs and Rajpurohit, 2010), but the complexity of cuticular lipids also allows for the encoding of chemical information. Thus, many insect species also use cuticular lipids for contact communicative purposes (Howard and Blomquist, 2005; Blomquist and Bagneres, 2010; Menzel et al., 2019). One important function that cuticular lipids fulfill in insects across numerous taxa is the recognition of potential mates (Chung and Carroll, 2015). Thus, the composition of cuticular lipids is typically both species- and sex-specific although behavioral cross-reactions between closely related species suggest that even lipid profiles which are discriminable by chemical and statistical methods may encode similar information (Buellesbach et al., 2013, 2018; Mair et al., 2017). In comparison to volatile insect sex pheromones which attract potential mates over longer distances, cuticular lipids are characterized by relatively low volatilities. Hence, lipids used for mate recognition are typically perceived at close range or even only after antennal contact with the insect cuticle (Ruther et al., 2000; Böröczky et al., 2009; Silk et al., 2009, 2011; Ginzel, 2010).
One challenge in cuticular lipid research is the question whether the CHCs alone mediate information transfer, or whether more polar lipids are also involved. Often, the behavior-modifying effects of insect-derived whole body extracts are attributed to the CHCs without explicitly testing this, e.g., by assaying fractions containing only CHCs, in part, because CHCs may be the only compound class detected in these extracts by coupled gas chromatography-mass spectrometry (GC/MS), the most common method used to analyze extracts of insect lipids. However, potentially bioactive, more polar minor compounds might be obscured by the major CHCs, or compound classes such as triacylglycerides (TAGs) might be missed because they are not sufficiently volatile to be detected by standard GC/MS techniques (Kühbandner et al., 2012; Kühbandner and Ruther, 2015). Another important question concerns the contribution of the different CHC classes (n-alkanes, methyl-branched alkanes, alkenes) to the bioactivity of cuticular lipid extracts. CHCs may be perceived by the responder as a whole profile, or single compound classes or even individual key compounds may be sufficient to trigger a behavioral response (Ginzel et al., 2003; Carlson et al., 2005; Lacey et al., 2008; Silk et al., 2009, 2011). To address the potential role of different CHC classes, fractionation techniques such as molecular sieving (Bello et al., 2015) and silver ion chromatography (Mander and Williams, 2016) can be applied to isolate methyl-branched alkanes and alkenes, respectively, as classes of compounds from bioactive lipid extracts. These fractions can then be tested separately in bioassays (Greene and Gordon, 2007; Böröczky et al., 2009). The gold standard, however, is the demonstration of bioactivity elicited by synthetic CHCs of explicitly known composition, which are, however, often not commercially available and have to be synthesized laboriously as needed (Millar, 2010).
One insect group for which cuticular lipids have been shown to be important mate recognition cues is the parasitoid wasps, a speciose taxon developing in or on arthropod hosts (Quicke, 1997; Ruther, 2013). After contact with female-derived cuticular lipids, males of many species respond by showing stereotypic, pre-copulatory courtship behavior. These behavioral sequences include courtship elements such as wing fanning, mounting, antennal movements/stroking, or series of movements termed head nodding (van den Assem and Putters, 1980; van den Assem et al., 1980; van den Assem, 1989; Ruther et al., 2000; Benelli et al., 2012, 2013). CHCs have been shown to be crucial in eliciting appropriate behavioral responses in several species (Howard, 1998; Ruther et al., 2000, 2011; Krokos et al., 2001; Sullivan, 2002; Steiner et al., 2005, 2006; Ablard et al., 2012; Sullivan and Erbilgin, 2015; Weiss et al., 2015; Pfeiffer et al., 2018; Böttinger et al., 2019). Sometimes, however, more polar components are the active compounds (Finidori-Logli et al., 1996; Weiss et al., 2013), or act synergistically with CHCs to evoke the full behavioral response from males (Kühbandner et al., 2012; Weiss et al., 2015; Mair et al., 2017; Böttinger et al., 2019). In the pteromalidae wasp Lariophagus distinguendus, for example, female-derived CHCs elicit a certain degree of wing fanning, mounting, antennal stroking, and even copulation attempts, but responses by males increase significantly if the CHCs are offered together with synergizing cuticular TAGs (Kühbandner et al., 2012). In the parasitoid wasp genus Leptopilina parasitizing Drosophila species, female-derived CHCs interact with more polar iridoids from the mandibular gland to mediate courtship behavior. The importance of the two substance classes varies greatly between species, ranging from those that rely exclusively on CHCs, through species that use both substance classes, to L. heterotoma, in which female-derived iridoids alone mediate both long-range attraction and courtship behavior in males (Weiss et al., 2013, 2015; Pfeiffer et al., 2018; Böttinger et al., 2019). In L. clavipes, co-extracted iridoids disturb the pheromone function of CHCs and crude extracts are much less active than the purified CHCs alone (Pfeiffer et al., 2018). Also in the genus Nasonia, an intensively studied model system for the study of parasitoid wasp biology (Werren et al., 2010; Mair and Ruther, 2019), the use of CHCs and more polar lipids for signaling varies among the species. In the cosmopolitan species N. vitripennis, CHCs are sufficient for elicitation of male courtship behavior (Steiner et al., 2006), whereas males of N. giraulti (sympatric with N. vitripennis in eastern North America) also need more polar, hitherto unknown lipids to recognize conspecific females (Mair et al., 2017).
Relatively little is known about the role of the different CHC classes or individual CHCs in the courtship pheromones of parasitoid wasps because in many species, only crude whole body washes or CHC fractions were tested (Ruther et al., 2000, 2011; Sullivan, 2002; Steiner et al., 2006; Buellesbach et al., 2013, 2018; Sullivan and Erbilgin, 2015). Synthetic alkadienes have been shown to elicit male courtship in the braconid wasp Cardiochiles nigriceps (Syvertsen et al., 1995) and in the chalcidoid wasp Eurytoma amygdali (Eurytomidae) (Krokos et al., 2001; Mazomenos et al., 2004). In Ooencyrtus kuvanae (Encyrtidae), two methyl-branched alkanes (5-MeC27 and 5,17-DiMeC27) stimulated male contact in an enantioselective manner (Ablard et al., 2012). In L. distinguendus, 3-MeC27 has been identified as a key component in the CHC-based contact sex pheromone (Kühbandner et al., 2012).
In the present study, we investigated the contact sex pheromone of the solitary parasitoid wasp Urolepis rufipes (Pteromalidae). The genus Urolepis Walker 1846 is closely related to the genera Nasonia Ashmead 1904 and Trichomalopsis Crawford 1913, and has been suggested to form a monophyletic taxon, the so-called “Nasonia group” (Burks, 2009). Urolepis rufipes is a pupal parasitoid of several Diptera such as house flies and stable flies (Stenseng et al., 2003) and has been found together with N. vitripennis in North American (Smith and Rutz, 1991; Gibson and Floate, 2004) and European livestock production facilities (Skovgard and Jespersen, 2000). Males of U. rufipes attract and arrest females using a substrate-borne sex pheromone [(2S,6S)-2,6-dimethyl-7-octene-1,6-diol] (Ruther et al., 2019; Melnik et al., 2020). Males produce this pheromone in the rectal vesicle and deposit it on the ground by dabbing movements of the abdominal tip. Males stay at the marked areas and wait for virgin females to arrive (Cooper and King, 2015). Once near a female, a male will immediately attempt to mount her. After mounting, the male shows occasionally bouts of wing fanning and establishes antennal contact with the female. Simultaneously, he extrudes his maxillary and labial palps, likely to release an (unknown) aphrodisiac pheromone from an oral gland that makes the female receptive to mating (Cooper et al., 2013). When receptive, the female folds her antennae against her head and opens her genital orifice. The male then backs up and copulates with the female. Copulation is typically followed by post-copulatory courtship that generally resembles the described pre-copulatory behavior (Cooper et al., 2013). While the courtship and mating behavior of U. rufipes have been studied in detail, the cues used by males to recognize females and to discriminate the sexes are unknown. Here, we investigated whether female-derived cuticular lipids are involved in the courtship behavior of U. rufipes. We investigated the responses of males to freeze-killed cadavers (dummies) of either sex, and to dummies from which cuticular lipids had been removed by solvent extraction. We tested whether extracts and fractions thereof, differing in polarity, contain chemical compounds that elicit male courtship behavior. Furthermore, we investigated the importance of methyl-branched and n-alkanes as possible bioactive compound classes responsible for eliciting behavioral responses. Finally, we analyzed the composition of cuticular extracts from males and females, and tested whether two components which were consistently more abundant in female-derived extracts were sufficient to render male-derived CHCs attractive to males.
Materials and Methods
Insects
The U. rufipes strain used in this study was collected by K. Floate and originated from cattle feedlots in southern Alberta, Canada. Wasps were reared on freeze-killed pupae of the green bottle fly Lucilia caesar at 25°C as described previously (Ruther et al., 2019). Under these conditions, U. rufipes has a generation time of approximately 14 days. After 13 days, parasitized fly pupae were isolated and kept singly in 1.5-ml reaction tubes to ensure emergence of virgin and naïve wasps. Dead males and females used as dummies in the experiments were virgin and either 1- or 2-day-old. Responding males were 1–2-day-old and naïve. To obtain enough males as responders for the bioassays, all-male broods were produced by using virgin females for parasitization. Because of the haplodiploid sex determination in parasitic wasps, these females produce only male offspring. Wasps used as dummies and responders in the behavioral experiments were used only once.
Preparation of Extracts and Fractionation
For bioassays, whole body extracts from 1 to 2-day-old male and female wasps were produced by washing batches of 50–100 freeze-killed wasps for 45 min with dichloromethane (DCM, 25 μl per wasp). We chose dichloromethane as a solvent and a relatively long extraction time based on previous studies (Steiner et al., 2006; Ruther et al., 2011; Kühbandner et al., 2012) having shown that these conditions result in the highest bioactivity. Furthermore, dichloromethane effectively extracts both CHCs and more polar TAGs that had been shown to act synergistically with CHCs in L. distinguendus (Kühbandner et al., 2012). Crude extracts were carefully concentrated under a stream of nitrogen and reconstituted in DCM to a final concentration of two wasp equivalents per μl. To test the response of males to lipids of differing polarity, we fractionated whole body extracts from males and females separately by adsorption chromatography. For this purpose, whole body crude extracts (70 wasps each, prepared as described above) were concentrated under nitrogen and reconstituted in 100 μl hexane. The hexane extracts were applied to silica gel cartridges (Chromabond 100 mg, Macherey & Nagel, Düren, Germany) which were then eluted consecutively with 800 μl each of hexane, DCM, and methanol. Eluates were concentrated under nitrogen, and reconstituted in DCM to a final concentration of two wasp equivalents per μl and stored at −20°C until being used in the bioassays. To test the response of males to female-derived methyl-branched alkanes, n-alkanes were removed from the bioactive female-derived hexane fraction (see section “Results Bioassays”) by treatment with activated molecular sieves (Bello et al., 2015). For activation, 50 g molecular sieves (5 Å, 45–60 mesh, Sigma-Aldrich, Taufkirchen, Germany) were conditioned for 2 h at 300°C under a stream of nitrogen. The hexane fraction (70 female equivalents, prepared as described above) was transferred to a 4-ml glass vial, concentrated under nitrogen and reconstituted in 2.5 ml isooctane. Subsequently, 50 mg activated molecular sieves were added and the solution was stirred for 16.5 h with a magnetic stirrer. The molecular sieves were removed by passing the solution through a silica gel cartridge and rinsing the cartridge with 500 μl isooctane. The isooctane was evaporated under nitrogen, and the purified methyl-branched alkanes were reconstituted in DCM to a final concentration of two female equivalents per μl. Fractions were stored at −20°C until being used in bioassays. To test the response of males to n-alkane blends in female specific ratios, synthetic n-alkanes (C25–31, C33) were dissolved in DCM and aliquots were combined to match the mean concentration found in female whole body extracts as determined by GC/MS analysis (Table 1, final concentration representing two female equivalents per μl). To test whether the addition of the two female-biased hydrocarbons 7-MeC31 and 7-MeC33 rendered male-derived CHCs behaviorally active, we first prepared a male-derived hexane fraction (200 individuals) as described above. Before the concentration step, we split the fraction into two equal aliquots of 100 wasp equivalents each and added to one sub-fraction 7.80 μg racemic 7-MeC31 and 1.1 μg racemic 7-MeC33 dissolved in hexane. Subsequently, both sub-fractions were concentrated under nitrogen and reconstituted with DCM to a final concentration of two wasp equivalents per μl. The added amounts of the synthetic compounds represented the mean difference between female- and male-derived extracts as determined by GC/MS analysis (Table 1). After the finding that the CHC fraction is active alone in the present study (see section “Results Bioassays”), we used hexane as the extraction solvent for the comparative chemical analyses of male- and female-derived CHC extracts to minimize co-extraction of non-volatile TAGs that could potentially contaminate the GC column. Two freeze-killed males or females per sample were extracted for 35 min with 25 μl hexane containing 10 ng/μl tetracosane (absent in the insect extracts) as an internal standard (n = 10 for each sex).
Chemical Analyses
Aliquots (2 μl) of whole body extracts, fractions thereof, isolated methyl-branched alkanes, and male-derived CHCs supplemented with 7-methylalkanes were analyzed on a Shimadzu QP2010 Plus GC/MS system equipped with a non-polar BPX5 capillary column (60 m × 0.25 mm inner diameter, 0.25 μm film thickness) (SGE Analytical Science Europe, Milton Keynes, United Kingdom). Samples were injected at 300°C in splitless mode using an AOC 20i autosampler. Helium was used as carrier gas at a linear velocity of 40 cm s–1. The initial oven temperature of 150°C was increased at 3°C min–1 to 300°C and held for 30 min. The mass spectrometer was operated in the electron ionization (EI) mode at 70 eV, and the mass range was m/z 35–600. Linear retention indices (LRI) of methyl-branched hydrocarbons were calculated by co-injection of straight-chain hydrocarbons (van den Dool and Kratz, 1963). Methyl-branched hydrocarbons were identified using diagnostic ions resulting from the favored fragmentation at the branching points (Nelson, 1993) and by comparing LRI values with literature data (Carlson et al., 1998; Steiner et al., 2006, 2007; Ruther et al., 2011; Buellesbach et al., 2018). For statistical comparison of CHC profiles of males and females, peak areas of individual compounds were normalized to the largest peak for each sample (at LRI 3545 representing 13,17-DiMeC35 plus 11,15-DiMeC35, = 100%). For quantification of CHCs in the extracts, individual peak areas were compared to that of the internal standard (10 ng/μl tetracosane).
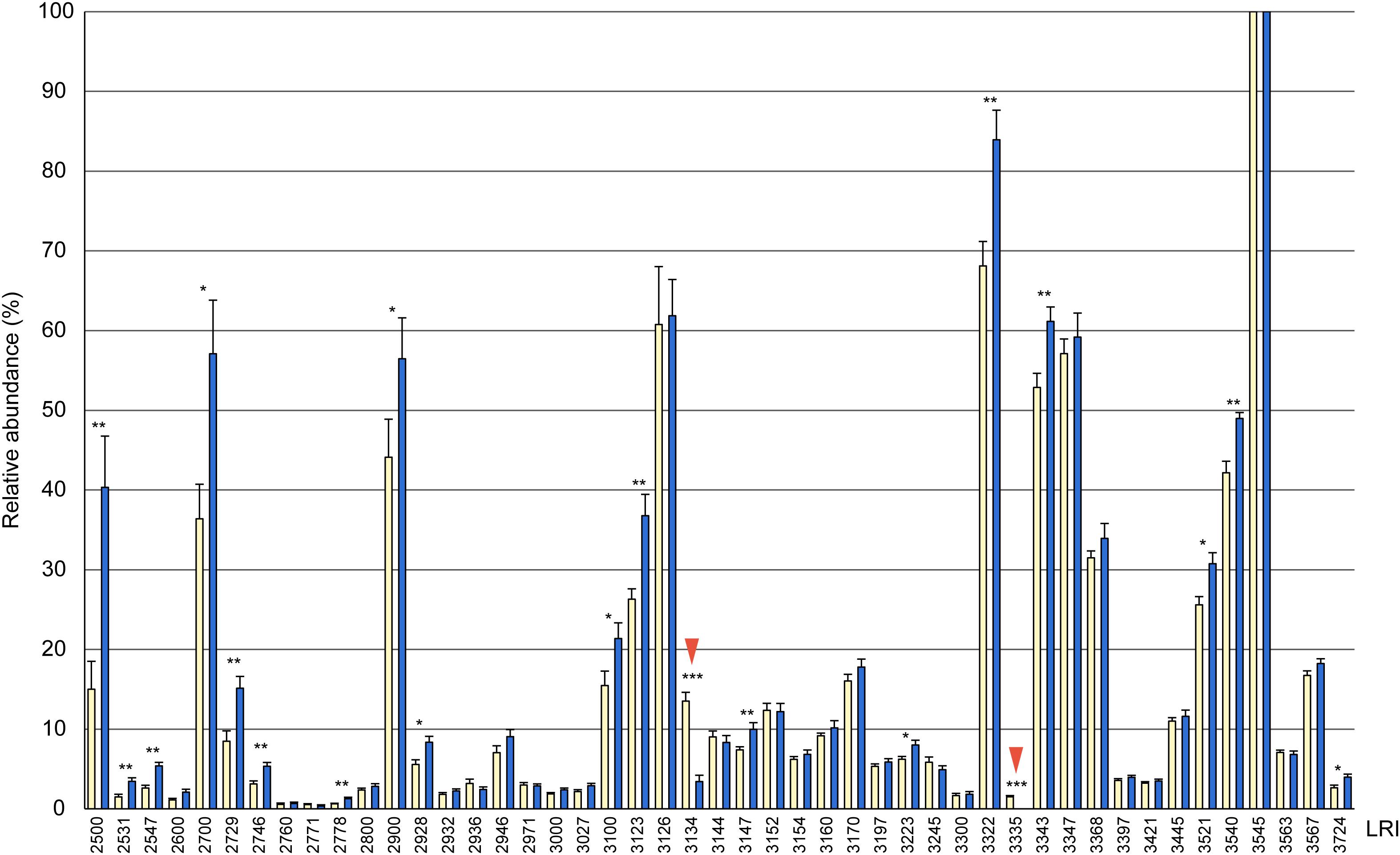
Figure 1. Relative composition of cuticular hydrocarbons (CHCs) in whole body extracts from female (yellow columns) and male (blue columns) Urolepis rufipes. Individual peak areas were related to the largest peak with a linear retention index (LRI) of 3545 (=100%). Error bars represent standard errors of means. Asterisks indicate significant differences between males and females at p < 0.05 (Mann-Whitney U-test, n = 10 for each sex). LRIs refer to the compounds listed in Table 1. Red arrows indicate the two female-biased CHCs 7-MeC31 and 7-MeC33.
Bioassays
Behavioral observations were performed in a round bioassay chamber (10 mm diameter × 3 mm high) made from acrylic glass which was covered by a cover slip (Ruther et al., 2000). Behaviors were observed with a stereo microscope under illumination from a microscope light source. Data were recorded using The Observer XT 11.0 computer software (Noldus Information Technology, Wageningen, Netherlands). Freeze-killed wasps (dummies) treated with test solutions were offered to responding males for an observation time of 5 min. During this time, we recorded (a) the time responding males spent mounted on the dummies, (b) the number of wing-fanning bouts, and (c) the duration of copulation attempts. In experiment 1, responding males were exposed to untreated dummies of either sex (1- or 2-day-old) or dummies stripped of cuticular lipids by Soxhlet-extraction (DCM, 7 h). In experiment 2, we tested the response of males to Soxhlet-extracted male dummies treated with 1 μl (representing 2 wasp equivalents) of a female-derived whole body extract as well as the respective hexane, DCM, or methanol fractions of the crude extract. Additionally, we tested solvent-extracted dummies treated with 1 μl (representing 2 wasp equivalents) of a male-derived whole body extract and the non-polar hexane fraction thereof. As controls, we tested Soxhlet-extracted dummies treated with solvent only. In experiment 3, we offered to male responders Soxhlet-extracted male dummies treated with female-derived methyl-branched alkanes or synthetic n-alkanes composed in the same ratios as found in female-derived cuticular extracts (2 wasp equivalents per dummy). In experiment 4, we tested Soxhlet-extracted male dummies treated with a male-derived hexane fraction or a sub-fraction supplemented with 7-MeC31 and 7-MeC33, respectively (see section “Preparation of Extracts and Fractionation”). Extracts, fractions, and synthetic compounds were applied to the dummies under a stereo microscope using a Hamilton 5-μl GC syringe designed for on-column gas chromatography (Fisher Scientific, Schwerte, Germany) mounted to a micromanipulator (World Precision Instruments, Sarasota, FL, United States). The whole body of the dummies was treated with the extracts or solutions in three to four partial doses. The number of replicates for each treatment was n = 20 for experiment 1 and n = 25 for experiments 2–4.
Statistical Analysis
The time males spent mounted on the dummies, the number of wing-fanning bouts, and the duration of copulation attempts were compared for each experiment by a Kruskal-Wallis H-test followed by pairwise Mann-Whitney U-tests with sequential Bonferroni correction. The relative abundances of CHCs (in relation to the largest peak) in cuticular extracts of males and females were compared by Mann-Whitney U-tests. All statistical analyses were done using PAST 3.26 scientific software (Hammer et al., 2001).
Chemical Synthesis
7-Methyltetracosane (7-MeC31) and 7-methyltetracosane (7-MeC33) were synthesized as shown in Supplementary Scheme S1, described in the Supplementary Material.
Results
Chemical Analyses
Based on fragmentation patterns and LRIs, we identified 67 compounds by GC/MS in cuticular extracts from male and female U. rufipes, all of them methyl-branched and n-alkanes, whereas unsaturated compounds were absent (Table 1). All compounds, except for 7-MeC33, occurred in both sexes. Due to co-elution of some components, these compounds eluted in 47 peaks that were compared statistically with respect to their relative abundances (normalized to the largest peak representing 11,15- and 11,17-DiMeC35). According to this analysis, 15 peaks were more abundant in males, while only two peaks were female-biased (Figure 1 and Table 1). 7-MeC31 (LRI 3134) showed an almost 4-fold higher relative abundance in females, and 7-MeC33 (LRI 3335) occurred exclusively in female-derived extracts. Hence, these compounds were considered potential key compounds, and synthetic versions were used to supplement male-derived CHCs for the behavioral bioassays (see section “Results Bioassays”). After fractionation on silica gel, all compounds detected in the crude extracts, except for cholesterol which eluted in the methanol fraction, were recovered in the non-polar hexane fraction (Supplementary Figure S1). Treatment of a female-derived hexane fraction with molecular sieves resulted in the selective removal of n-alkanes, while the composition of methyl-branched CHCs remained largely unchanged (Supplementary Figure S1). The non-polar sub-fraction of a male-derived whole body extract supplemented with synthetic 7-MeC31 and 7-MeC33 appeared to differ only in the abundance of the added compounds while the composition of the other CHCs was equivalent to that in the unsupplemented sub-fraction (Supplementary Figure S2).
Bioassays
In experiment 1, U. rufipes males spent significantly longer on, showed significantly more bouts of wing-fanning toward, and tried to copulate significantly longer with 1- and 2-day-old female dummies when compared to female dummies stripped of cuticular lipids or to untreated or solvent stripped male dummies (Figures 2A–C and Supplementary Table S1). In experiment 2, U. rufipes males spent significantly longer on, showed significantly more bouts of wing-fanning toward, and tried to copulate significantly longer with Soxhlet-extracted male dummies treated with female whole body extract and the non-polar hexane fraction thereof, when compared to dummies treated with the pure solvent or with the more polar lipid fractions eluting with DCM or methanol (Figures 3A–C and Supplementary Table S2). For mounting time and copulation duration, the hexane fraction was significantly more active than the crude extract. Neither male-derived whole body extracts nor the non-polar fraction thereof elicited stronger behavioral responses from males than the solvent-treated control dummies (Figures 3D–F and Supplementary Table S2). In experiment 3, neither female-derived methyl-branched alkanes nor synthetic n-alkanes combined in female-specific ratios elicited any behavioral responses from U. rufipes males (Figures 4A–C and Supplementary Table S3). In experiment 4, supplementation of a male-derived hexane fraction with racemic 7-MeC31 and 7-MeC33 did not influence the male response when compared to the non-manipulated control fraction (Figures 5A–C and Supplementary Table S4).
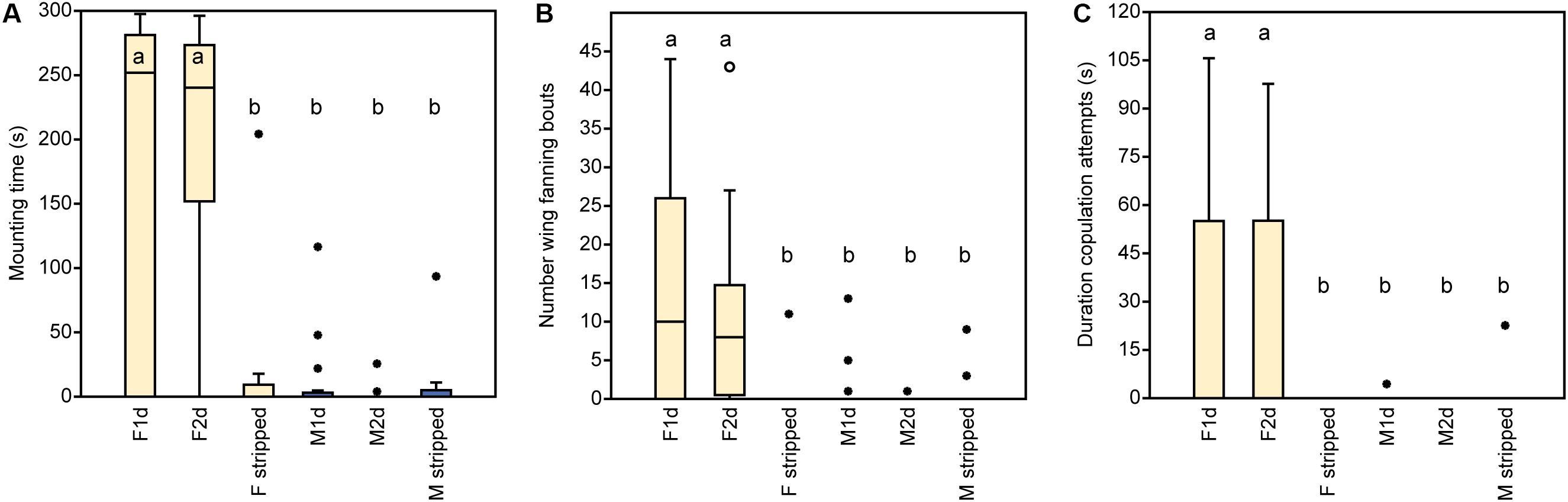
Figure 2. Behavioral responses of U. rufipes males to freeze-killed 1- and 2-day-old females (F1d and F2d) and males (M1d and M2d) as well as to female and male wasps that were stripped of CHCs by solvent extraction. Panels show (A) the time males spent mounted on the dummy, (B) the number of wing-fanning bouts, and (C) the duration of copulation attempts. Box-and-whisker plots show median (horizontal line), 25–75% quartiles (box), maximum/minimum range (whiskers) and outliers (∙ > 3× box height). Different lowercase letters indicate significant differences between treatments for each parameter at p < 0.05 (Kruskal-Wallis H-test followed by multiple Mann-Whitney U-tests with sequential Bonferroni-correction, n = 20). Statistical results are given in detail in Supplementary Table S1.
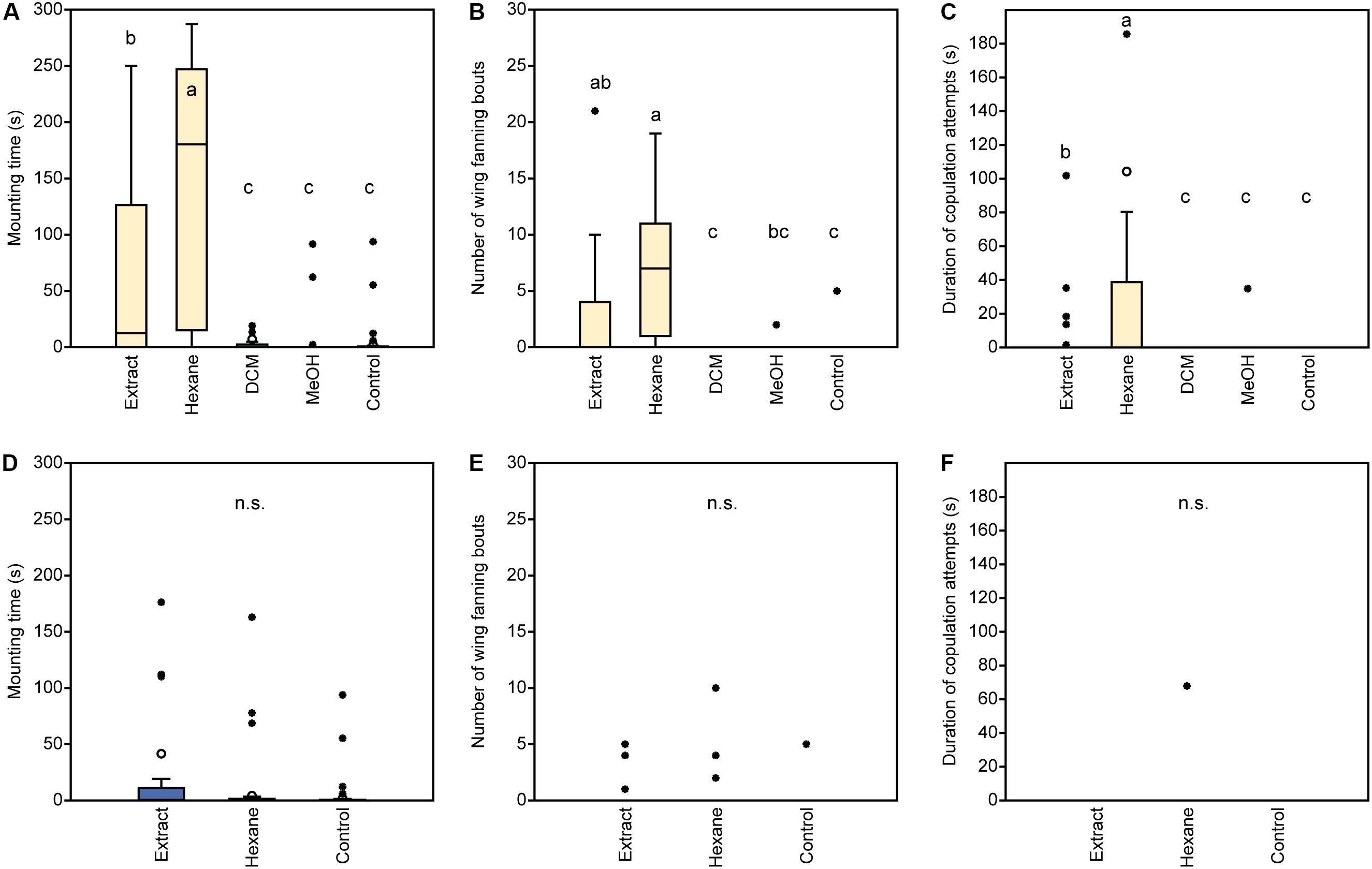
Figure 3. Behavioral responses of U. rufipes males to Soxhlet-extracted male dummies treated with female- (A–C) or male-derived (D–F) whole body extracts and fractions thereof obtained by silica gel chromatography using hexane, dichloromethane (DCM), or methanol (MeOH) as eluents. Control dummies were treated with the pure solvent. Given are (A,D) the time males spent mounted on the dummy, (B,E) the number of wing-fanning bouts, and (C,F) the duration of copulation attempts. Box-and-whisker plots show median (horizontal line), 25–75% quartiles (box), maximum/minimum range (whiskers) and outliers (°> 1.5× box height; ∙ > 3× box height). Different lowercase letters indicate significant differences between treatments for each parameter at p < 0.05 (Kruskal-Wallis H-test followed by multiple Mann-Whitney U-tests with sequential Bonferroni-correction; n.s. = non-significant; n = 25). Statistical results are given in detail in Supplementary Table S2.
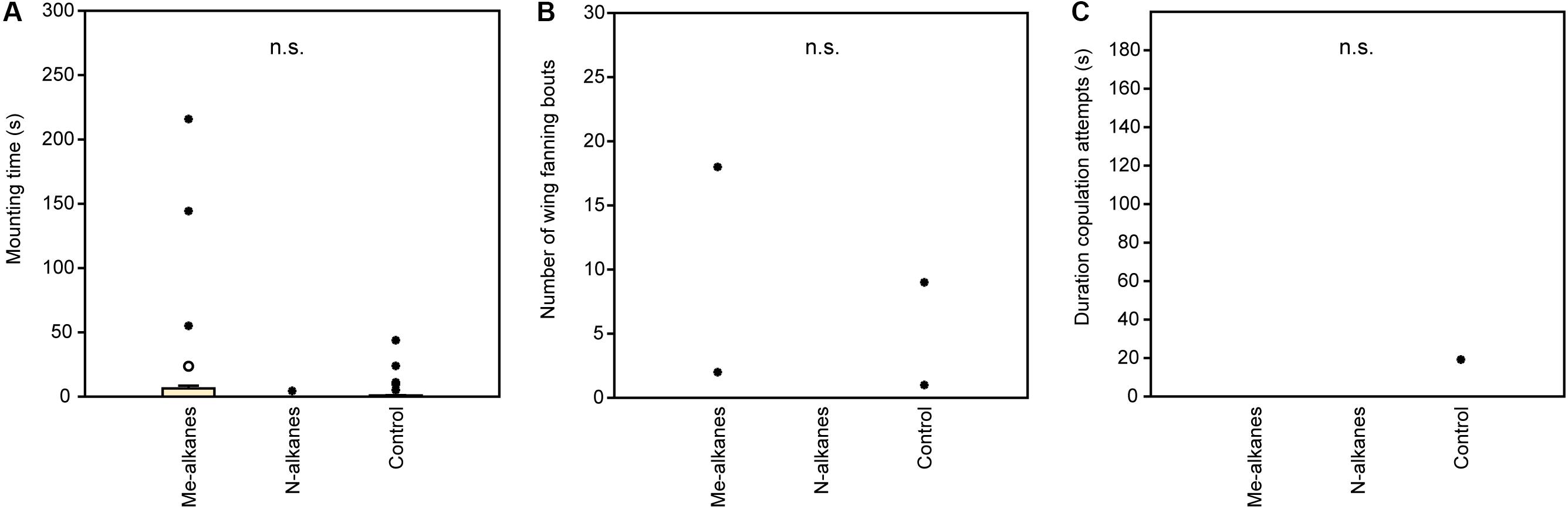
Figure 4. Behavioral responses of U. rufipes males to Soxhlet-extracted male cadavers (dummies) treated with female-derived methyl-branched cuticular hydrocarbons (Me-alkanes) or synthetic n-alkanes (N-alkanes) reconstructed in a female-specific ratio. Control dummies were treated with the pure solvent. Given are (A) the time males spent mounted on the dummy, (B) the number of wing-fanning bouts, and (C) the duration of copulation attempts. Box-and-whisker plots show median (horizontal line), 25–75% quartiles (box), maximum/minimum range (whiskers) and outliers (° > 1.5× box height; ∙ > 3× box height; Kruskal-Wallis H-test, n.s. = not significant; n = 25). Statistical results are given in detail in Supplementary Table S3.
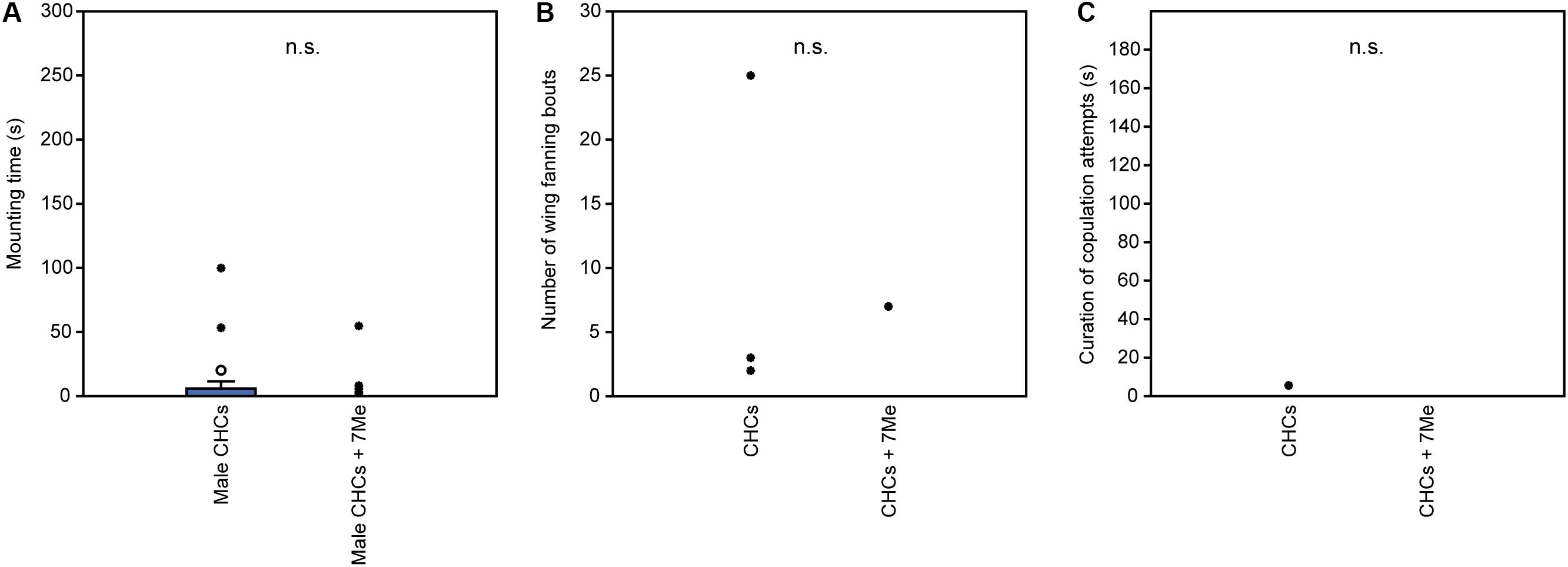
Figure 5. Behavioral responses of U. rufipes males to Soxhlet-extracted male cadavers (dummies) treated with male-derived cuticular hydrocarbons (CHCs) purified by silica gel chromatography or a subfraction supplemented with synthetic 7-MeC31 and 7-MeC33. Given are (A) the time males spent mounted on the dummy, (B) the number of wing-fanning bouts, and (C) the duration of copulation attempts. Box-and-whisker plots show median (horizontal line), 25–75% quartiles (box), maximum/minimum range (whiskers) and outliers (° > 1.5× box height; ∙ > 3× box height; Mann-Whitney U-test; n.s. = not significant; n = 25). Statistical results are given in detail in Supplementary Table S4.
Discussion
The present study demonstrates that U. rufipes males respond to freeze-killed females by mounting, wing fanning, and copulation attempts, behaviors which they did not exhibit when presented with dummies from which cuticular lipids had been removed by solvent extraction. Reapplication of female-derived extracts or the non-polar fraction thereof to solvent extracted dummies reestablished the full courtship response in males. All the compounds detected in the bioactive non-polar fraction were CHCs. In contrast, freeze-killed males and male-derived extracts did not elicit mating responses from test males. Likewise, purified male-derived CHCs [no longer containing the co-extracted (2S,6S)-2,6-dimethyl-7-octene-1,6-diol] did not elicit any behavioral responses, showing that it was not perception of the male sex pheromone (Ruther et al., 2019) that prevented males from responding to male dummies and male-derived whole body extracts. More polar lipids are not necessary for a full behavioral response from U. rufipes males, which is in contrast to L. distinguendus where cuticular TAGs synergize the responses of males to female-derived CHCs (Kühbandner et al., 2012). As for mounting time and copulation duration, the purified female-derived CHCs were significantly more active than whole body crude extracts suggesting that co-extracted polar lipids disturb the behavioral responses of males. The U. rufipes CHC profiles consisted exclusively of methyl-branched and n-alkanes, and the qualitative composition of male- and female-derived CHCs was very similar, whereas the relative amounts showed sex-specific differences. However, either group alone (methyl-branched alkanes isolated from females and synthetic n-alkanes in female-specific ratios) did not elicit responses from males. We therefore conclude that, like in other pteromalid wasps (Sullivan, 2002; Steiner et al., 2006; Ruther et al., 2011), female-derived CHCs elicit courtship behavior in U. rufipes males, and that n-alkanes and methyl-branched alkanes act synergistically to elicit the full behavioral response from males.
The only female-biased peaks in the CHC profiles were those of 7-MeC31 and 7-MeC33. However, supplementation of male-derived CHCs with natural proportions of racemic 7-MeC31 and 7-MeC33 was not sufficient to render these CHCs behaviorally active. This might be due to male-derived CHCs disrupting the perception of 7-MeC31 and/or 7-MeC33. Another possible reason for the biological inactivity of 7-MeC31 and 7-MeC33 is the fact that we used racemic compounds. There is increasing evidence that chiral methyl-branched CHCs are produced and perceived enantioselectively by a range of insect taxa (Bello et al., 2015; Hughes et al., 2015; Sharma et al., 2015; de Narbonne et al., 2016), and this is also true for parasitic wasps (Ablard et al., 2012). In Ooencyrtus kuvanae (Encyrtidae), male and female-derived CHC-profiles did not show any qualitative differences, but 5-MeC27 and 5,17-DiMeC27 were more abundant in males than in females. Males of O. kuvanae responded to the synthetic analogs of these compounds enantioselectively, with 5R-MeC27/5R,17R-DiMeC27 inhibiting and 5S-MeC27/5R,17S-DiMeC27 stimulating male contact (Ablard et al., 2012). Hence, future experiments might reveal whether 7-MeC31 and 7-MeC33 are behaviorally active in U. rufipes when tested as pure enantiomers. As they stand, our results suggest that these female-biased CHCs do not play a key role in the U. rufipes contact sex pheromone or play such a role only when presented simultaneously with other as-yet unidentified compounds. This is unlike what has been shown with 3-MeC27 in L. distinguendus. In this species, freeze-killed females of differing age and newly emerged males elicit courtship behavior (wing-fanning) from test males. With increasing age, however, males lose their attractiveness, and this process correlates with a loss of 3-MeC27 from the CHC profile (Steiner et al., 2005, 2007). Supplementation of behaviorally inactive CHCs from older males with synthetic 3-MeC27 resulted in a restoration of sexual attractiveness of the CHC profile. Somewhat surprisingly, this effect was elicited by both enantiomers of 3-MeC27, but variation of the added compound with respect to either chain length or position of the methyl-branch resulted in a loss of activity in the manipulated profiles, indicating that the response was compound-specific (Kühbandner et al., 2012, 2013).
The results of the present study suggest that U. rufipes males perceive the CHC-based contact sex pheromone sex-specifically “as a whole” rather than relying on the presence/abundance of individual key compounds. To test this hypothesis, it would be necessary to formulate the U. rufipes CHCs in sex-specific ratios and compare the responses of males to these synthetic profiles. However, this approach would be laborious and time-consuming, because most methylated CHCs are not commercially available, and their absolute configurations are unknown. However, even if the reconstruction of artificial CHC profiles is logistically unrealistic, it is still possible to manipulate them. For example, addition of 150 ng doses of several synthetic monomethyl- and n-alkanes to intrinsically bioactive dummies eliminated wing-fanning responses of male L. distinguendus (Kühbandner et al., 2013). All compounds tested in the cited study are constituents of the natural CHC profile of L. distinguendus, demonstrating that a correctly balanced ratio of all constituents is necessary to elicit proper courtship responses from males of this species. Comparable experiments with U. rufipes might therefore confirm the putative holistic perception of sex-specific CHC profiles that underlies sex discrimination and the initiation of courtship in this species.
Another open question concerns the physiological adaptations by which parasitoid wasps might be able to perceive and discriminate complex CHC blends that differ only in the relative proportions of more or less the same compounds. An answer to this question might be obtained by recent studies on ants, which have an analogous problem in the context of nestmate and caste recognition. In these hymenopteran insects, three sub-classes of multiply innervated sensilla basiconica have been characterized that are differentially sensitive to CHC blends as well as to individual CHCs, and likely underlie the ability of ants to discriminate caste- and colony-specific CHC mixtures (Sharma et al., 2015). Future comparative studies with the genetic model organism N. vitripennis might help to identify both the morphological structures and the olfactory receptors underlying the sex-specific perception of CHCs by parasitoid wasps in the context of mate recognition and courtship.
Data Availability Statement
The datasets generated for this study are available on request to the corresponding author.
Ethics Statement
Observational experiments with live insects are excluded from legislation in Germany. The studied species is not endangered. Before extraction, insects were freeze-killed. Insects were kept under near natural conditions on freeze-killed hosts.
Author Contributions
JR initiated the study, conceived the experiments, analyzed the data, and wrote the manuscript. JWü, TP, and JWi performed the experiments and analyzed the data. JM synthesized 7-MeC31 and 7-MeC33. All authors read and revised the manuscript.
Conflict of Interest
The authors declare that the research was conducted in the absence of any commercial or financial relationships that could be construed as a potential conflict of interest.
Funding
JM acknowledged the core funding from Hatch project CA-R∗ENT-5181-H.
Acknowledgments
The authors thank Bethia H. King for providing the starter culture of U. rufipes (originally collected by K. Floate) and Sonja Fleischmann for rearing the insects.
Supplementary Material
The Supplementary Material for this article can be found online at: https://www.frontiersin.org/articles/10.3389/fevo.2020.00180/full#supplementary-material
References
Ablard, K., Gries, R., Khaskin, G., Schaefer, P. W., and Gries, G. (2012). Does the stereochemistry of methylated cuticular hydrocarbons contribute to mate recognition in the egg parasitoid wasp Ooencyrtus kuvanae? J. Chem. Ecol. 38, 1306–1317. doi: 10.1007/s10886-012-0189-4
Bello, J. E., Mcelfresh, J. S., and Millar, J. G. (2015). Isolation and determination of absolute configurations of insect-produced methyl-branched hydrocarbons. Proc. Natl. Acad. Sci. U.S.A. 112, 1077–1082. doi: 10.1073/pnas.1417605112
Benelli, G., Bonsignori, G., Stefanini, C., and Canale, A. (2012). Courtship and mating behaviour in the fruit fly parasitoid Psyttalia concolor (Szepligeti) (Hymenoptera: Braconidae): the role of wing fanning. J. Pest Sci. 85, 55–63. doi: 10.1007/s10340-011-0394-x
Benelli, G., Bonsignori, G., Stefanini, C., Dario, P., and Canale, A. (2013). Male Wing fanning performance during successful and unsuccessful mating in the parasitic wasp Lariophagus distinguendus Förster (Hymenoptera: Pteromalidae). J. Insect Behav. 26, 228–237. doi: 10.1007/s10905-012-9356-2
Blomquist, G. J., and Bagneres, A. G. (2010). Insect Hydrocarbons - Biology, Biochemistry, and Chemical Ecology. Cambridge: Cambridge University Press.
Böröczky, K., Crook, D. J., Jones, T. H., Kenny, J. C., Zylstra, K. E., Mastro, V. C., et al. (2009). Monoalkenes as contact sex pheromone components of the woodwasp Sirex noctilio. J. Chem. Ecol. 35, 1202–1211. doi: 10.1007/s10886-009-9693-6
Böttinger, L. C., Hofferberth, J., Ruther, J., and Stökl, J. (2019). Semiochemicals mediating defense, intraspecific competition, and mate finding in Leptopilina ryukyuensis and L. japonica (Hymenoptera: Figitidae), parasitoids of Drosophila. J. Chem. Ecol. 45, 241–252. doi: 10.1007/s10886-019-01052-w
Buckner, J. S. (1993). “Cuticular polar lipids of insects,” in Insect Lipids. Chemistry, Biochemistry & Biology, eds D. W. Stanley-Samuelson and D. R. Nelson (Lincoln: University of Nebraska Press), 227–270.
Buellesbach, J., Gadau, J., Beukeboom, L. W., Echinger, F., Raychoudhury, R., Werren, J. H., et al. (2013). Cuticular hydrocarbon divergence in the jewel wasp Nasonia: evolutionary shifts in chemical communication channels? J. Evol. Biol. 26, 2467–2478. doi: 10.1111/jeb.12242
Buellesbach, J., Vetter, S. G., and Schmitt, T. (2018). Differences in the reliance on cuticular hydrocarbons as sexual signaling and species discrimination cues in parasitoid wasps. Front. Zool. 15:22. doi: 10.1186/s12983-018-0263-z
Burks, R. A. (2009). Phylogenetics of Pteromalidae and Eulophidae (Hymenoptera: Chalcidoidea) with a study of cranial bridges in Chalcidoidea. Doctor of Philosophy PhD, University of Califormia Riverside, Riverside, CA.
Carlson, D. A., Bernier, U. R., and Sutton, B. D. (1998). Elution patterns from capillary GC for methyl-branched alkanes. J. Chem. Ecol. 24, 1845–1865. doi: 10.1023/a:1022311701355
Carlson, D. A., Mramba, F., Sutton, B. D., Bernier, U. R., Geden, C. J., and Mori, K. (2005). Sex pheromone of the tsetse species, Glossina austeni: isolation and identification of natural hydrocarbons, and bioassay of synthesized compounds. Med. Vet. Entomol. 19, 470–479. doi: 10.1111/j.1365-2915.2005.00596.x
Chung, H., and Carroll, S. B. (2015). Wax, sex and the origin of species: dual roles of insect cuticular hydrocarbons in adaptation and mating. Bioessays 37, 822–830. doi: 10.1002/bies.201500014
Cooper, J. L., Burgess, E. R., and King, B. H. (2013). Courtship behavior and detection of female receptivity in the parasitoid wasp Urolepis rufipes. J. Insect Behav. 26, 745–761. doi: 10.1007/s10905-013-9390-8
Cooper, J. L., and King, B. H. (2015). Substrate-borne marking in the parasitoid wasp Urolepis rufipes (Hymenoptera: Pteromalidae). Environ. Entomol. 44, 680–688. doi: 10.1093/ee/nvv017
de Narbonne, M. M., Van Zweden, J. S., Bello, J. E., Wenseleers, T., Millar, J. G., and D’ettorre, P. (2016). Biological activity of the enantiomers of 3-methylhentriacontane, a queen pheromone of the ant Lasius niger. J. Exp. Biol. 219, 1632–1638. doi: 10.1242/jeb.136069
Finidori-Logli, V., Bagneres, A. G., Erdmann, D., Francke, W., and Clement, J. L. (1996). Sex recognition in Diglyphus isaea Walker (Hymenoptera: Eulophidae): role of an uncommon family of behaviorally active compounds. J. Chem. Ecol. 22, 2063–2079. doi: 10.1007/BF02040095
Gibbs, A. G. (1998). Water-proofing properties of cuticular lipids. Am. Zool. 38, 471–482. doi: 10.1093/icb/38.3.471
Gibbs, A. G., and Rajpurohit, S. (2010). “Cuticular lipids and water balance,” in Insect Hydrocarbons, eds G. J. Blomquist and A. G. Bagneres (Cambridge: Cambridge University Press), 100–120. doi: 10.1017/cbo9780511711909.007
Gibson, G. A. P., and Floate, K. D. (2004). Filth fly parasitoids; on dairy farms in Ontario and Quebec, Canada. Can. Entomol. 136, 407–417. doi: 10.4039/n03-006
Ginzel, M. D. (2010). “Hydrocarbons as contact pheromones in longhorned beetles (Coleoptera: Cerambycidae),” in Insect Hydrocarbons. Biology, Biochemistry, and Chemical Ecology, eds G. J. Blomquist and A. G. Bagneres (Cambridge: Cambridge University Press), 375–389. doi: 10.1017/cbo9780511711909.018
Ginzel, M. D., Blomquist, G. J., Millar, J. G., and Hanks, L. M. (2003). Role of contact pheromones in mate recognition in Xylotrechus colonus. J. Chem. Ecol. 29, 533–545. doi: 10.1023/a:1022894419521
Greene, M. J., and Gordon, D. M. (2007). Structural complexity of chemical recognition cues affects the perception of group membership in the ants Linephithema humile and Aphaenogaster cockerelli. J. Exp. Biol. 210, 897–905. doi: 10.1242/jeb.02706
Hammer, Ø, Harper, D. A. T., and Ryan, P. D. (2001). PAST: paleontological statistics software package for education and data analysis. Palaeontol. Electron. 4:4.
Howard, R. W. (1998). Ontogenetic, reproductive, and nutritional effects on the cuticular hydrocarbons of the host-specific ectoparasitoid Cephalonomia tarsalis (Hymenoptera: Bethylidae). Ann. Entomol. Soc. Am. 91, 101–112. doi: 10.1093/aesa/91.1.101
Howard, R. W., and Blomquist, G. J. (2005). Ecological, behavioral, and biochemical aspects of insect hydrocarbons. Annu. Rev. Entomol. 50, 371–393. doi: 10.1146/annurev.ento.50.071803.130359
Hughes, G. P., Bello, J. E., Millar, J. G., and Ginzel, M. D. (2015). Determination of the absolute configuration of female-produced contact sex pheromone components of the longhorned beetle, Neoclytus acuminatus acuminatus (F). J. Chem. Ecol. 41, 1050–1057. doi: 10.1007/s10886-015-0639-x
Krokos, F. D., Konstantopoulou, M. A., and Mazomenos, B. E. (2001). Alkadienes and alkenes, sex pheromone components of the almond seed wasp Eurytoma amygdali. J. Chem. Ecol. 27, 2169–2181. doi: 10.1023/a:1012218618218
Kühbandner, S., Bello, J. E., Mori, K., Millar, J. G., and Ruther, J. (2013). Elucidating structure-bioactivity relationships of methyl-branched alkanes in the contact sex pheromone of the parasitic wasp Lariophagus distinguendus. Insects 4, 743–760. doi: 10.3390/insects4040743
Kühbandner, S., and Ruther, J. (2015). Solid phase micro-extraction (SPME) with in situ transesterification: an easy method for the detection of non-volatile fatty acid derivatives on the insect cuticle. J. Chem. Ecol. 41, 584–592. doi: 10.1007/s10886-015-0592-8
Kühbandner, S., Sperling, S., Mori, K., and Ruther, J. (2012). Deciphering the signature of cuticular lipids with contact sex pheromone function in a parasitic wasp. J. Exp. Biol. 215, 2471–2478. doi: 10.1242/jeb.071217
Lacey, E. S., Ginzel, M. D., Millar, J. G., and Hanks, L. M. (2008). 7-methylheptacosane is a major component of the contact sex pheromone of the cerambycid beetle Neoclytus acuminatus acuminatus. Physiol. Entomol. 33, 209–216. doi: 10.1111/j.1365-3032.2008.00624.x
Mair, M. M., Kmezic, V., Huber, S., Pannebakker, B. A., and Ruther, J. (2017). The chemical basis of mate recognition in two parasitoid wasp species of the genus Nasonia. Entomol. Exp. Appl. 164, 1–15. doi: 10.1111/eea.12589
Mair, M. M., and Ruther, J. (2019). Chemical ecology of the parasitoid wasp genus Nasonia (Hypenoptera: Pteromalidae). Front. Ecol. Evol. 7:184. doi: 10.3389/fevo.2019.00184
Mander, L. N., and Williams, C. M. (2016). Chromatography with silver nitrate: part 2. Tetrahedron 72, 1133–1150. doi: 10.1016/j.tet.2016.01.004
Mazomenos, B. E., Athanassiou, C. G., Kavallieratos, N., and Milonas, P. (2004). Evaluation of the major female Eurytoma amygdali sex pheromone components, (Z,Z)-6,9-tricosadiene and (Z,Z)-6,9-pentacosadiene for male attraction in field tests. J. Chem. Ecol. 30, 1245–1255. doi: 10.1023/b:joec.0000030275.17006.f3
Melnik, K., Grimm, C., Wittbrodt, J., Ruther, J., and Schulz, S. (2020). Enantioselective synthesis and determination of the absolute configuration of the male sex pheromone of the parasitoid wasp Urolepis rufipes. Organ. Biomol. Chemi. 18, 3463–3465. doi: 10.1039/D0OB00614A
Menzel, F., Morsbach, S., Martens, J. H., Räder, P., Hadjaje, S., Poizat, M., et al. (2019). Communication versus waterproofing: the physics of insect cuticular hydrocarbons. J. Exp. Biol. 222(Pt 23):jeb.210807. doi: 10.1242/jeb.210807
Millar, J. G. (2010). “Chemical synthesis of insect cuticular hydrocarbons,” in Insect Hydrocarbons. Biology, Biochemistry, and Chemical Ecology, eds G. J. Blomquist and A. G. Bagneres (Cambridge: Cambridge University Press), 163–186. doi: 10.1017/cbo9780511711909.009
Nelson, D. R. (1993). “Methyl-branched lipids in insects,” in Insect Lipids - Chemistry, Biochemistry and Biology, eds D. W. Stanley-Samuelson and D. R. Nelson (Lincoln, NE: University of Nebraska Press), 271–315.
Pfeiffer, L., Ruther, J., Hofferberth, J., and Stökl, J. (2018). Interference of chemical defence and sexual communication can shape the evolution of chemical signals. Sci. Rep. 8:321. doi: 10.1038/s41598-017-18376-w
Ruther, J. (2013). “Novel insights into pheromone-mediated communication in parasitic hymenopterans,” in Recent Advances in Chemical Ecology of Insect Parasitoids, eds E. Wajnberg and S. Colazza (Chichester: Wiley-Blackwell), 112–143.
Ruther, J., Döring, M., and Steiner, S. (2011). Cuticular hydrocarbons as contact sex pheromone in the parasitoid Dibrachys cavus. Entomol. Exp. Appl. 140, 59–68. doi: 10.1111/j.1570-7458.2011.01129.x
Ruther, J., Homann, M., and Steidle, J. L. M. (2000). Female-derived sex pheromone mediates courtship behaviour in the parasitoid Lariophagus distinguendus. Entomol. Exp. Appl. 96, 265–274. doi: 10.1046/j.1570-7458.2000.00705.x
Ruther, J., Wittman, T., Grimm, C., Feichtner, F. S., Fleischmann, S., Kiermaier, J., et al. (2019). Male sex pheromone of the parasitoid wasp Urolepis rufipes demonstrates biosynthetic switch between fatty acid and isoprenoid metabolism within the Nasonia group. Front. Ecol. Evol. 7:26. doi: 10.3389/fevo.2019.00026
Sharma, K., Enzmann, B., Schmidt, Y., Moore, D., Jones, G., Parker, J., et al. (2015). Cuticular hydrocarbon pheromones for social behavior and their coding in the ant antenna. Cell Rep. 12, 1261–1271. doi: 10.1016/j.celrep.2015.07.031
Silk, P. J., Ryall, K., Lyons, D. B., Sweeney, J., and Wu, J. P. (2009). A contact sex pheromone component of the emerald ash borer Agrilus planipennis Fairmaire (Coleoptera: Buprestidae). Naturwissenschaften 96, 601–608. doi: 10.1007/s00114-009-0513-1
Silk, P. J., Sweeney, J., Wu, J. P., Sopow, S., Mayo, P. D., and Magee, D. (2011). Contact sex pheromones identified for two species of longhorned beetles (Coleoptera: Cerambycidae) Tetropium fuscum and T. cinnamopterum in the subfamily Spondylidinae. Environ. Entomol. 40, 714–726. doi: 10.1603/en10213
Skovgard, H., and Jespersen, J. B. (2000). Seasonal and spatial activity of hymenopterous pupal parasitoids (Pteromalidae and Ichneumonidae) of the house fly (Diptera: Muscidae) on Danish pig and cattle farms. Environ. Entomol. 29, 630–637. doi: 10.1603/0046-225x-29.3.630
Smith, L., and Rutz, D. A. (1991). Seasonal and relative abundance of hymenopterous parasitoids attacking house fly pupae at dairy farms in central New York. Environ. Entomol. 20, 661–668. doi: 10.1093/ee/20.2.661
Stanley-Samuelson, D. W., and Nelson, D. R. (1993). Insect Lipids: Chemistry, Biochemistry and Biology. Lincoln: University of Nebraska Press.
Steiner, S., Hermann, N., and Ruther, J. (2006). Characterization of a female-produced courtship pheromone in the parasitoid Nasonia vitripennis. J. Chem. Ecol. 32, 1687–1702. doi: 10.1007/s10886-006-9102-3
Steiner, S., Mumm, R., and Ruther, J. (2007). Courtship pheromones in parasitic wasps: comparison of bioactive and inactive hydrocarbon profiles by multivariate statistical methods. J. Chem. Ecol. 33, 825–838. doi: 10.1007/s10886-007-9265-6
Steiner, S., Steidle, J. L. M., and Ruther, J. (2005). Female sex pheromone in immature insect males - a case of pre-emergence chemical mimicry? Behav. Ecol. Sociobiol. 58, 111–120. doi: 10.1007/s00265-005-0930-x
Stenseng, L., Skovgard, H., and Holter, P. (2003). Life table studies of the pupal parasitoid Urolepis rufipes (Hymenoptera: Pteromalidae) on the house fly Musca domestica (Diptera: Muscidae) in Denmark. Environ. Entomol. 32, 717–725. doi: 10.1603/0046-225x-32.4.717
Sullivan, B. T. (2002). Evidence for a sex pheromone in bark beetle parasitoid Roptrocerus xylophagorum. J. Chem. Ecol. 28, 1045–1063. doi: 10.1023/A:1015270003717
Sullivan, B. T., and Erbilgin, N. (2015). Evidence for divergence in cuticular hydrocarbon sex pheromone between California and Mississippi (United States of America) populations of bark beetle parasitoid Roptrocerus xylophagorum (Hymenoptera: Pteromalidae). Can. Entomol. 147, 472–475. doi: 10.4039/tce.2014.59
Syvertsen, T. C., Jackson, L. L., Blomquist, G. J., and Vinson, S. B. (1995). Alkadienes mediating courtship in the parasitoid Cardiochiles nigriceps (Hymenoptera: Braconidae). J. Chem. Ecol. 21, 1971–1989. doi: 10.1007/BF02033856
van den Assem, J. (1989). “Mating behaviour in parasitic wasps,” in Insect Parasitoids, eds J. Waage and D. Greathead (London: Academic Press), 137–167.
van den Assem, J., Jachmann, F., and Simbolotti, P. (1980). Courtship behavior of Nasonia vitripennis (Hym., Pteromalidae): some qualitative, experimental evidence for the role of pheromones. Behaviour 75, 301–307. doi: 10.1163/156853980X00456
van den Assem, J., and Putters, F. A. (1980). Patterns of sound produced by courting chalcidoid males and its biological significance. Entomol. Exp. Appl. 27, 293–302. doi: 10.1111/j.1570-7458.1980.tb02977.x
van den Dool, H., and Kratz, P. (1963). A generalization of the retention index system including linear temperature programmed gas—liquid partition chromatography. J. Chromatogr. A 11, 463–471. doi: 10.1016/S0021-9673(01)80947-X
Weiss, I., Hofferberth, J., Ruther, J., and Stökl, J. (2015). Varying importance of cuticular hydrocarbons and iridoids in the species-specific mate recognition pheromones of three closely related Leptopilina species. Front. Ecol. Evol. 3:19. doi: 10.3389/fevo.2015.00019
Weiss, I., Roessler, T., Hofferberth, J., Brummer, M., Ruther, J., and Stökl, J. (2013). A nonspecific defensive compound evolves into a competition avoidance cue and a female sex pheromone. Nat. Commun. 4:2767. doi: 10.1038/ncomms3767
Keywords: cuticular hydrocarbons, mate finding, parasitoid wasp, contact sex pheromone, Urolepis rufipes
Citation: Würf J, Pokorny T, Wittbrodt J, Millar JG and Ruther J (2020) Cuticular Hydrocarbons as Contact Sex Pheromone in the Parasitoid Wasp Urolepis rufipes. Front. Ecol. Evol. 8:180. doi: 10.3389/fevo.2020.00180
Received: 09 February 2020; Accepted: 19 May 2020;
Published: 12 June 2020.
Edited by:
Sebastien Lebreton, Aix-Marseille Université, FranceReviewed by:
Maria Carolina Blassioli Moraes, Brazilian Agricultural Research Corporation (EMBRAPA), BrazilAyako Wada-Katsumata, North Carolina State University, United States
Marcelo Gustavo Lorenzo, Oswaldo Cruz Foundation (Fiocruz), Brazil
Copyright © 2020 Würf, Pokorny, Wittbrodt, Millar and Ruther. This is an open-access article distributed under the terms of the Creative Commons Attribution License (CC BY). The use, distribution or reproduction in other forums is permitted, provided the original author(s) and the copyright owner(s) are credited and that the original publication in this journal is cited, in accordance with accepted academic practice. No use, distribution or reproduction is permitted which does not comply with these terms.
*Correspondence: Joachim Ruther, am9hY2hpbS5ydXRoZXJAdXIuZGU=